Integration between Engineering and Knowledge Systems: Evaluation of Performance of a Wastewate
AVEVA最新三维工厂设计软件E3D

“精益建造”将加快项目整体进度,并减少损耗巴黎2012年10月11日电 /美通社/ -- AVEVA 今天在巴黎 AVEVA 世界峰会 (AVEVA World Summit) 上推出 AVEVA Everything3D (E3D)。
AVEVA E3D 将各种技术整合在一起,提出了一项新的愿景,让客户能够在工程、设计和建造等行业使用“精益”工作实践。
这将有助于加快项目的整体进度,并减少损耗。
作为 AVEVA 一体化工程与设计方案的一部分,AVEVA E3D 将多个重要创新成果融为一体,大大增强了新建工厂设计和全生命周期改造项目。
该产品最重要的一点就是将激光扫描数据和三维几何学、强大的绘图工具以及直观的用户界面整合在一起,满足一般常见设计任务的需求。
AVEVA 工程设计系统首席技术官兼主管 Dave Wheeldon 表示:“工厂设计解决方案市场日新月异,需要提出一个新的理念。
仅仅取得零误差设计已经远远不够。
经济实惠型新技术的出现已经让工厂设计的触角切实可行地延伸至供货商、制造商和施工团队等其它利益相关者。
”“采用‘精益’理念实现成本节约的一个关键在于随着工程进展远离设计办公室的团队沟通状态和建造信息的能力。
”AVEVA 战略与营销高级副总裁 Bruce Douglas 表示:“我们的客户正面临许多挑战。
力求获得更多能源和矿产资源的压力将使得工程项目面临更多、更大的技术难题。
发达市场工程师人口的老龄化与新兴市场经验的严重匮乏都要求工厂设计软件更易于使用、便于快速培训、能够缩短投产时间。
”“此外,商业市场出现了消费市场上的移动和云计算也使得异地工作人员,特别是那些在施工现场的人员能够通过状态更新和激光扫描数据将重要的完工资料反馈给设计团队。
这有助于迅速且具有成本效益地采取纠正措施,最大程度地保证施工质量,同时确保工程项目进度。
”AVEVA E3D 将包括:工厂设计系统,包含工程和原理图等专业三维建模功能,通过结合最优质的三维图形和激光扫描点云展现“真实世界”完全自动化的二维绘图能力,并与三维模型全面集成设计过程中与其它设计系统完全协同工作采用的架构支持项目重用与模块化设计,另外还支持与云/移动基础设施的集成支持与时下行业领先的系统 AVEVA PDMS 同时操作AVEVA E3D 将于2012年12月中旬面市。
多元融合联合毕业设计教学改革研究与实践
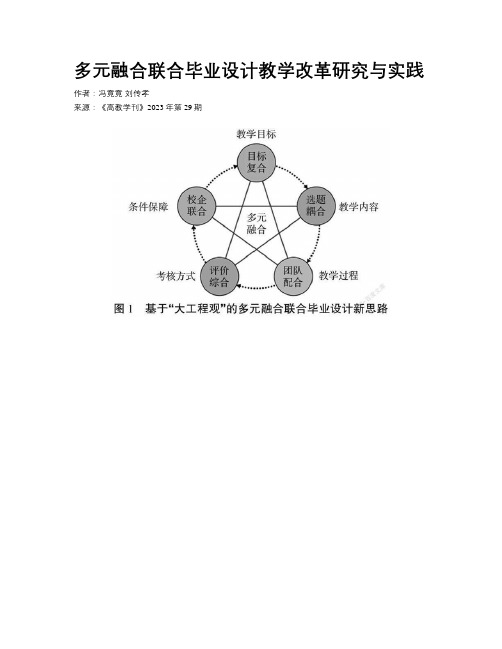
多元融合联合毕业设计教学改革研究与实践作者:冯竟竟刘传孝来源:《高教学刊》2023年第29期摘要:毕业设计作为本科阶段的最后综合实践环节,是实现人才培养目标的关键。
目前土木建筑类专业毕业设计普遍存在专业化培养与行业对复合型人才需求有机融合不够、毕业设计与工程实践深度融合不足、评价方法不够多元、不利于持续改进等问题。
为解决以上问题,在新工科建设背景下,该论文以“大工程观”教育理念为指导,以4个土木建筑类专业毕业设计为研究对象,通过教学目标复合、设计选题耦合、学生团队配合及评价综合、校企联合,探索“多元融合”联合毕业设计实践教学新模式,教学效果显著,推广应用性强,可为土木建筑类专业毕业设计实践教学改革提供参考和借鉴。
关键词:联合毕业设计;大工程观;多元融合;土木建筑类专业;教学改革中图分类号:G640 文献标志码:A 文章编号:2096-000X(2023)29-0149-04Abstract: As the last comprehensive practice link in the undergraduate stage, graduation design is the key to achieve the goal of talent training. At present, there are many problems in graduation design of civil engineering and architecture majors, such as insufficient organic integration between professional training and the industry's demand for compound talents,insufficient in-depth integration between graduation design and engineering practice, and insufficient diversity of evaluation methods, which is not conducive to continuous improvement. In order to solve the above problems, under the background of new engineering construction, guided by the "big engineering view", this paper takes the graduation design of four civil engineering and architecture majors as the research object. By the combination of teaching objectives, design topic selection coupling, student teamwork, comprehensive evaluation, and school-enterprise cooperation, this paper explores a new teaching practice mode of "multiple integration" joint graduation design, which has remarkable teaching effect and strong popularization and application, and can provide reference for practical teaching reform of graduation design of civil engineering and architecture majors.Keywords: joint graduation design; big engineering view; multi-integration; civil engineering and architecture majors; teaching reform基金項目:山东省本科教学改革研究项目“基于专业认证教育模式开展人才培养的‘三维’进度管理和质量控制体系研究”(M2021182)第一作者简介:冯竟竟(1981-),女,汉族,山东淄博人,博士,教授,副院长。
Engineering Physics

Engineering PhysicsEngineering Physics is a multidisciplinary field that combines principles of engineering and physics to solve complex problems and develop innovative technologies. This field encompasses a wide range of applications, including materials science, optics, nanotechnology, and renewable energy. The integration of engineering and physics principles has led to groundbreaking advancements in various industries, from aerospace and defense to healthcare and telecommunications. In this response, we will explore the historical background and development of engineering physics, analyze different perspectives and opinions surrounding the topic, provide case studies and examples to illustrate key points, offer a critical evaluation of the topic, and conclude with future implications and recommendations related to engineering physics. The historical background of engineering physics can be traced back to the late 19th and early20th centuries when the fields of engineering and physics began to converge. During this time, rapid industrialization and technological advancements created a demand for professionals who could apply scientific principles to practical engineering problems. This led to the emergence of engineering physics as a distinct discipline, with universities and research institutions offering specialized programs and conducting research in this area. One notable development in the history of engineering physics is the invention of the transistor in 1947, which revolutionized the field of electronics and paved the way for the development of modern computing and communication technologies. From a historical perspective, engineering physics has played a crucial role in driving innovation and technological progress. The integration of physics principles into engineering applications has led to the development of numerous groundbreaking technologies, such as semiconductors, lasers, and advanced materials. These advancements have had a profound impact on various industries, improving efficiency, performance, and sustainability. For example, in the field of renewable energy, engineering physics has contributed to the development of solar cells, wind turbines, and energy storage systems, enabling the transition towards a more sustainable and environmentally friendly energy infrastructure. From a contemporary standpoint, engineering physics continues to be a dynamic and evolving field, with ongoingresearch and development in areas such as quantum computing, photonics, and nanotechnology. The interdisciplinary nature of engineering physics allows for collaboration between engineers, physicists, and other scientific disciplines, leading to innovative solutions to complex challenges. However, there arediffering perspectives and opinions surrounding the role and significance of engineering physics in the modern world. Some individuals may view engineering physics as a niche field with limited practical applications, overshadowed by more specialized engineering disciplines such as mechanical, electrical, or chemical engineering. However, this perspective overlooks the unique contributions of engineering physics in driving fundamental scientific research and enabling technological breakthroughs. For instance, the development of quantum computingand nanoscale materials relies heavily on principles of engineering physics, demonstrating the far-reaching implications of this field. On the other hand, proponents of engineering physics emphasize its role in fosteringinterdisciplinary collaboration and pushing the boundaries of scientific knowledge. By integrating engineering and physics principles, engineering physics offers a holistic approach to problem-solving, addressing complex challenges that require a deep understanding of both scientific and engineering principles. This perspective highlights the versatility and adaptability of engineering physics in addressing diverse technological and societal needs. To illustrate the key pointssurrounding engineering physics, let us consider a case study in the field of medical imaging. Advances in engineering physics have led to the development of sophisticated imaging technologies such as magnetic resonance imaging (MRI) and computed tomography (CT) scans, which have revolutionized medical diagnostics and treatment planning. These technologies rely on principles of physics to generate detailed images of the human body, enabling healthcare professionals to identify and treat various medical conditions with precision and accuracy. The integration of engineering physics in medical imaging has significantly improved patient outcomes and has become an indispensable tool in modern healthcare. Another example that exemplifies the significance of engineering physics is the development of advanced materials with unique properties and functionalities. By leveraging principles of quantum mechanics and materials science, engineers andphysicists have collaborated to design and fabricate materials with tailored characteristics, such as superconductivity, high strength-to-weight ratio, and enhanced thermal conductivity. These materials have applications in diverse industries, including aerospace, automotive, and electronics, and have opened new possibilities for technological innovation and performance enhancement. While engineering physics has undoubtedly contributed to numerous technological advancements and scientific breakthroughs, it is essential to critically evaluate the benefits and drawbacks of this interdisciplinary field. One potential benefit of engineering physics is its capacity to drive innovation and address complex challenges that require a comprehensive understanding of scientific and engineering principles. By fostering collaboration between experts from different disciplines, engineering physics can lead to the development of transformative technologies with far-reaching implications for society. However, one drawback of engineering physics is the inherent complexity and interdisciplinary nature of the field, which can pose challenges in terms of education and training. Professionals in engineering physics must possess a broad skill set encompassing knowledge of physics, mathematics, and engineering principles, which may require a more extensive and rigorous training curriculum compared to traditional engineering disciplines. Additionally, the rapid pace of technological advancement in engineering physics necessitates continuous learning and adaptation to stay abreast of the latest developments, posing a challenge for professionals in this field. Looking towards the future, engineering physics is poised to play apivotal role in addressing global challenges such as climate change, energy sustainability, and healthcare innovation. As the demand for advanced technologies and scientific solutions continues to grow, engineering physics will be instrumental in driving progress and shaping the future of various industries. To maximize the potential of engineering physics, it is crucial to invest in research and education initiatives that promote interdisciplinary collaboration and cultivate the next generation of engineering physicists. In conclusion, engineering physics represents a unique and dynamic field that integrates principles of engineering and physics to drive innovation and solve complex challenges. The historical development of engineering physics has led to numeroustechnological advancements and scientific breakthroughs, with ongoing research and development in diverse areas. While there are differing perspectives and opinions surrounding the significance of engineering physics, its role in fostering interdisciplinary collaboration and enabling technological innovation cannot be understated. By critically evaluating the benefits and drawbacks of engineering physics, we can gain a deeper understanding of its potential and limitations. Looking ahead, engineering physics holds great promise for addressing global challenges and shaping the future of technology and science. As we continue to invest in research and education initiatives, engineering physics will undoubtedly play a crucial role in driving progress and innovation in the years to come.。
Engineering Systems Integration
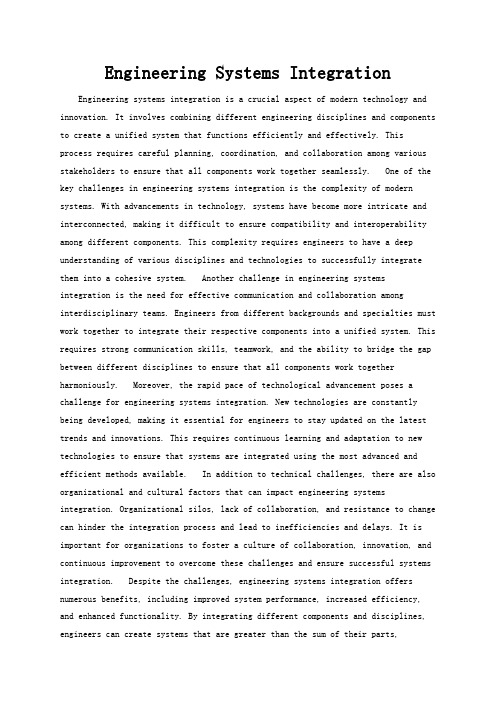
Engineering Systems Integration Engineering systems integration is a crucial aspect of modern technology and innovation. It involves combining different engineering disciplines and components to create a unified system that functions efficiently and effectively. This process requires careful planning, coordination, and collaboration among various stakeholders to ensure that all components work together seamlessly. One of the key challenges in engineering systems integration is the complexity of modern systems. With advancements in technology, systems have become more intricate and interconnected, making it difficult to ensure compatibility and interoperability among different components. This complexity requires engineers to have a deep understanding of various disciplines and technologies to successfully integrate them into a cohesive system. Another challenge in engineering systemsintegration is the need for effective communication and collaboration among interdisciplinary teams. Engineers from different backgrounds and specialties must work together to integrate their respective components into a unified system. This requires strong communication skills, teamwork, and the ability to bridge the gap between different disciplines to ensure that all components work together harmoniously. Moreover, the rapid pace of technological advancement poses a challenge for engineering systems integration. New technologies are constantly being developed, making it essential for engineers to stay updated on the latest trends and innovations. This requires continuous learning and adaptation to new technologies to ensure that systems are integrated using the most advanced and efficient methods available. In addition to technical challenges, there are also organizational and cultural factors that can impact engineering systems integration. Organizational silos, lack of collaboration, and resistance to change can hinder the integration process and lead to inefficiencies and delays. It is important for organizations to foster a culture of collaboration, innovation, and continuous improvement to overcome these challenges and ensure successful systems integration. Despite the challenges, engineering systems integration offers numerous benefits, including improved system performance, increased efficiency, and enhanced functionality. By integrating different components and disciplines, engineers can create systems that are greater than the sum of their parts,delivering value to users and stakeholders. Successful systems integration requires a holistic approach that considers technical, organizational, and cultural factors to create systems that meet the needs of today's complex and interconnected world.。
英语作文-工程技术与设计服务行业推动工业升级引领制造业转型

英语作文-工程技术与设计服务行业推动工业升级引领制造业转型In today's rapidly evolving industrial landscape, the engineering, technology, and design services sector plays a pivotal role in driving the advancement and transformation of manufacturing industries. This sector not only facilitates innovation but also serves as a cornerstone for industrial upgrades worldwide.Engineering and technology services encompass a broad spectrum of activities critical to enhancing manufacturing capabilities. At the forefront are design services, which integrate creative solutions with practical engineering principles to develop products that meet market demands effectively. These services encompass everything from conceptual design to detailed engineering, ensuring that products are not only functional but also optimized for production efficiency and cost-effectiveness.Moreover, technological advancements in fields such as automation, robotics, and artificial intelligence have revolutionized manufacturing processes. Engineering firms specializing in these areas provide tailored solutions that streamline production, improve quality control, and reduce operational costs. By leveraging these technologies, manufacturers can achieve higher productivity levels while maintaining stringent quality standards, thus enhancing their competitive edge in the global market.Furthermore, the role of design in shaping consumer perception and product appeal cannot be overstated. Design services extend beyond mere aesthetics; they encompass user experience (UX) research, human-centered design principles, and ergonomic considerations. By prioritizing these aspects, engineering and design firms ensure that products are not only visually appealing but also user-friendly and aligned with consumer expectations.In addition to product development, engineering services play a crucial role in optimizing manufacturing processes. This includes implementing lean manufacturing principles, supply chain management strategies, and sustainability initiatives. Byimproving efficiency and minimizing waste, these services contribute to reducing the environmental footprint of manufacturing operations while maximizing resource utilization.Another significant aspect of the engineering and technology services sector is its role in fostering innovation ecosystems. Collaborations between engineering firms, research institutions, and industry stakeholders facilitate the exchange of knowledge and ideas. This collaborative approach accelerates the pace of innovation, leading to breakthroughs in materials science, renewable energy technologies, and advanced manufacturing techniques.Moreover, the global nature of modern industries necessitates engineering and technology services that are adaptable and responsive to diverse market needs. International firms specializing in cross-border projects bring expertise in navigating regulatory frameworks, cultural considerations, and logistical challenges. This enables manufacturers to expand their operations globally while ensuring compliance with local regulations and standards.Ultimately, the engineering, technology, and design services sector serves as a catalyst for industrial transformation and economic growth. By continuously pushing the boundaries of innovation and efficiency, these services empower manufacturers to embrace new opportunities and overcome emerging challenges in an increasingly competitive market landscape.In conclusion, the integration of engineering, technology, and design services is indispensable in driving industrial upgrades and leading the transformation of manufacturing industries worldwide. As technological advancements continue to reshape the manufacturing landscape, the role of these services in fostering innovation, optimizing processes, and enhancing product development remains pivotal. By harnessing the power of engineering expertise and creative design solutions, manufacturers can navigate complexities, seize opportunities, and achieve sustainable growth in a rapidly evolving global economy.。
【工学】土木工程英语词汇共(80页)

【工学】土木工程英语词汇共(80页)structural controlstructure control 结构控制结构控制: structural control 结构控制剂: constitution controller 裂缝宽度容许值: allowable value of crack width 裂缝宽度容许值装配式预制: precast装配式预制的: precast-segmental 装配式预制装配式预制混凝土环: precast concrete segmental ring安装预应力: prestressed 安装预应力optimization最优化: Optimum Theory|optimization|ALARA 最优化使最优化: optimized 次最优化: suboptimization空心板梁: hollow slab beam 空心板梁主梁截面: girder section 主梁截面边、中跨径: side span &middle spin 边、中跨径girder主梁: girder|main beam|king post 主梁桥主梁: bridge girder主梁翼: main spar单墩: single pier单墩尾水管: single-pier draught tube 单墩单墩肘形尾水管: one-pier elbow draught tube结构优化设计: optimal structure designing扩结构优化设计: Optimal Struc ture Designing 结构优化设计液压机结构优化设计软件包: HYSOP 多跨连续梁: continuous beam on many supports 连续多跨splice barsplice plate拼接板: splice bar|scab|splice plate 拼接板端头拼接板: end matched lumber 销钉拼接板: pin splicecrack 裂缝 creviceto step acrossstep over跨越: stride leap|across|spanning 跨越跨越杆: cross-over pole|crossingpole 跨越点: crossingpoint|crossover point rigid frame bridge刚构桥: rigid frame bridge 刚构桥形刚构桥: T-shaped rigid frame bridge 连续刚构桥: continuous rigid frame bridge stiffness ratio ratio of rigidity刚度比: ratio of rigidity|stiffness ratio 刚度比动刚度比: dynamic stiffenss ratio 刚度比劲度比: stiffnessratio uniform beam 等截面粱等截面粱: uniform beam|uniform cross-section beambridge constructionbridgework桥梁工程: bridgeworks|LUSAS FEA|Bridge Engineering 桥梁工程桥梁工程师: Bridge SE铁路桥梁工程: railway bridge engineering suspension bridge悬索桥: suspension bridge|su e io ridge 悬索桥加劲悬索桥: stiffenedsuspensionbridge prestressed concrete预应力混凝土: prestressed concrete|prestre edconcrete 预应力混凝土预应力混凝土梁: prestressed concrete beam 预应力混凝土管: prestressed concrete pipe 预应力钢筋束: pre-stressing tendon|pre-stre ingtendon 预应力钢筋束抛物线型钢丝束(预应力配钢筋结构用): parabolic cable minimum steel ratio 最小配筋率axial tensionaxial tensile force轴向拉力: axial tension|axial te ion 轴向拉力轴向拉力, 轴向拉伸: axial tension 轴向拉力轴向张力: axialtensileforce cushion cap承台: bearing platform|cushioncap|pile caps 承台桩承台: pilecap|platformonpiles低桩承台: low pile caparch bridge拱桥: hump bridge|arch bridge|arched bridge 拱桥拱橋: Archbridge|Puente en arco|Pont en arc 鸠拱桥: Khājūintensitystrength强度: intensity|Strength|Density 强度刚强度: stiffness|stiffne|westbank stiffness 光强度: lightintensity|intensityhooping箍筋: stirrup|reinforcement stirrup|hooping 箍筋箍筋柱: tiedcolumn|hooped column 形箍筋: u stirrup u预应力元件: prestressed element 预应力元件equivalent load等效荷载: equivalent load 等效荷载等效荷载原理: principle of equivalent loads 等效负载等效荷载等值负载: equivalentload matrix model 模型 mouldpattern承载能力极限状承载能力极限状态: ultimate limit states 态serviceability limit state 正常使用极限状正常使用极限状态: serviceability limit state 态正常使用极限状态验证: verification of serviceability limit stateselasticityspringinessspringgive 弹性flexibility弹性: elasticity|Flexibility|stretch 弹性体: elastomer|elasticbody|SPUA plane cross-section assumption 平截面假定平截面假定: plane cross-section assumption intensity of tension 抗拉强度 tensile strength safety factor 安全系数standard value标准值: standard value,|reference value 标准值作用标准值: characteristic value of an action 重力标准值: gravity standardvalue of calculationdesign value设计值: design value|value|designed value 设计值作用设计值: design value of an action 荷载设计值: design value of a load confidence levelreliabilityfiduciary level 可靠度可靠度: Reliability|degree of reliability 不可靠度: Unreliability高可靠度: High Reliabilitygeometrical characteristic几何特征: geometrical characteristic 几何特征配位几何特征: coordinated geometric feature 流域几何特征: basin geometric characteristics plastic nature 塑性 plasticitystress diagram应力图: stress diagram|stress pattern 应力图谷式应力图: Cremona's method机身应力图: fuselage stress diagram crushing stress压应力: compressive stress|compression stress 压应力抗压应力: compressive stress|pressure load 内压应力: internal pressure stress ratio of reinforcementreinforcement ratioreinforcement percentage 配筋率配筋率: reinforcement ratio平均配筋率: balanced steel ratio纵向配筋率: longitudinal steel ratio finite element analysis有限元分析: FEA|finite element analysis (FEA)|ABAQUS 有限元分析反有限元分析: inverse finite element analysis 有限元分析软件: HKSABAQUS|MSC/NASTRAN MSC/NASTRANfinite element method有限元法: FInite Element|finite element method 有限元法积有限元法: CVFEM线性有限元法: Linear Finite Element Method 裂缝控制: crack control 控制裂缝钢筋: crack-control reinforcement 裂缝控制检查~核对~抑制~控制~试验~裂缝~支票~账单~牌号~名牌: check stress concentration应力集中: stress concentration 应力集中应力集中点: hard spot|focal point of stress 应力集中器: stress concentratorsprincipal tensile stress 主拉应力主拉应力: principal tensile stress nonlinearity 非线性nonlinear oscillationsnonlinear vibration非线性振动: nonlinear vibration 非线性振动非线性振动理论: theory of non linear vibration 非线性随机振动: Nonlinear random vibration flexural momentment of flexion (moment of flexure)bending momentflexural torque 弯矩弯矩: bending moment|flexural moment|kN-m 弯矩图: bending moment diagram|moment curve 双弯矩: bimomentcenter of momentsmoment center 弯矩中心弯矩中心: center of moments|momentcenter moment distributionmomentdistribution 弯矩分配法弯矩分配法: hardy cross method|cross method bending moment diagram moment curvemoment diagram 弯矩图弯矩图: bending moment diagram|moment curve 最终弯矩图: final bending moment diagram 最大弯矩图: maximum bending moment diagram shearing force剪力: shearing force|shear force|shear 剪力剪力墙: shearwall|shearing wall|shear panel 剪力钉: shear nails|SHEAR CONCRETE STUD elasticity modulusyoung's moduluselastic modulus 弹性模量modulus of elasticityelastic ratioshear diagram剪力图: shear diagram|shearing force diagram 剪力和弯矩图: Shear and Moment Diagrams 剪力图绘制剪力和弯矩图的图解法: Graphical Method for Constructing Shear and Moment Diagramsshear wall剪力墙: shear wall|shearing wall|shear panel 剪力墙抗剪力墙: shearwall剪力墙结构: shear wall structure 轴力: shaft force|axial force 螺栓轴力测试仪: Bolt shaft force tester 轴力轴向力: axial force|normal force|beam frame construction 框架结构等参数单元等参元: isoparametricelement 等参单元板单元: plate unit托板单元: pallet unit 板单元骨板骨单元: lamella/lamellaeosteon (surname)beam of roof 梁bridgebridge 桥梁curvature 曲率mechanics of materials 材料力学structural mechanics结构力学: Structural Mechanics|theory of structures 结构力学重结构力学: barodynamics船舶结构力学: Structual Mechamics for Ships flexural rigiditybending rigidity弯曲刚度: bending stiffness|flexural rigidity 弯曲刚度截面弯曲刚度: flexural rigidity of section 弯曲刚度~抗弯劲度: bending stiffness encased structures 钢管混凝土结构钢管混凝土结构: encased structuresultimate load极限荷载: ultimate load 极限荷载极限荷载设计: limit loaddesign|ultimate load design 设计极限荷载: designlimitloadDLL|design ultimate load limit load designultimate load analysisultimate load design 极限荷载设计极限荷载设计: limit load design|ultimate load design 设计极限荷载: designlimitloadDLL|design ultimate load mechanics of board shell 板壳力学: Plate Mechanics 板壳力学板壳非线性力学: Nonlinear Mechanics of Plate and Shell 本构模型: constitutive model体积本构模型: bulk constitutive equation 本构模型本构模型屈服面: yield surfacemain reinforcing steelmain reinforcement 主钢筋主钢筋: main reinforcement|Main Reinforcing Steel 钢筋混凝土的主钢筋: mainbarsocle beam悬臂梁: cantilever beam|cantilever|outrigger 悬臂梁悬臂梁长: length of cantilever双悬臂梁: TDCBcatenary悬链线: Catenary,|catenary wire|chainette 悬链线伪悬链线: pseudocatenary悬链线长: catenary lengthribbed stiffener加劲肋: stiffening rib|stiffener|ribbed stiffener 加劲肋短加劲肋: short stiffener支承加劲肋: bearing stiffenertechnology standard 技术标准水文: Hydrology水文学: hydrology|hydroaraphy|すいもんがく水文水文图: hydrograph|hydrological maps invite public bidding 招标(v) submit a bid 投标 bid forthrough beam连续梁: continuous beam|through beam 连续梁多跨连续梁: continuous beam on many supports 悬臂连续梁: gerber beamstiff girder加劲梁: stiffening girder|buttress brace 加劲梁加劲梁节点: stiff girder connection 支撑刚性梁~加劲梁~横撑: buttress brace hydrology 水文学: hydrology|hydroaraphy|すいもんがく水文学水文學: Hydrologie|水文学|??? ?????? 古水文学: paleohydrology桥梁抗震加固: bridge aseismatic strengthening 桥梁抗震wind resistance抗风: Withstand Wind|Wtstan Wn|wind resistance 抗风抗风锚: weather anchor抗风性: wind resistancebasal 基础的bridge construction control survey 桥梁控制测量桥梁控制测量: bridge construction control survey 桥梁施工控制综合程序系统: FWD 桥梁最佳施工指南: Bridge Best Practice Guidelines 桥梁施工桥梁工程施工技术咨询: Bridge Construction Engineering Serviceoverall designintegrated design总体设计: Global|overall design|general arrangement 总体设计总体设计概念: totaldesignconcept 工厂总体设计图: general layout scheme predesign 初步设计 preliminary plantechnical design技术设计: technical design|technical project 技术设计技术设计员: Technical Designer|technician 技术设计图: technical drawing construction documents design 施工图设计: construction documents design 施工图设计阶段: construction documents design phase 施工图设计基本建设项目施工图设计: design of working drawing of a capitalconstruction projectabutment 桥台 bridge abutmentfoundationbase 基础basisstructural style结构形式: Type of construction|form of structure 结构形式表结构形式: list structure form 屋顶结构形式: roof formearthquake 地震earthquake activityseismic activityseismic motionseismicity 地震活动地震活动: Seismic activity|seismic motion 地震活动性:seismicity|seismic 地震活动图: seismicity map支撑体系: bracing system|support system 支撑体系物流企业安全平台支撑体系: SSOSP 公路施工手册-桥涵: Optimization of Road Traffic Organization-Abstract 公路桥涵approach roadramp wayapproach 引道引道: approach|approach road引道坡: approach ramp|a roachramp 引道版: Approach slab装配式桥: fabricated bridge|precast bridge 装配式房屋: Prefabricated buildings 装配式装配式钢体: fabricated steel bodywear耐久性: durability|permanence|endurance 耐久性不耐久性:fugitiveness耐久性试验: endurance test|life test|durability test 持久状况: persistent situation 持久状况短暂状况: transient situation 短暂状况偶然状况: accidental situation 偶然状况永久作用: permanent action 永久作用永久作用标准值: characteristic value of permanent action可变作用: variable action可变作用标准值: characteristic value of variable action 可变作用可变光阑作用: iris action偶然作用: accidental action偶然同化,作用,: accidental assimilation 偶然作用作用效应偶然组合: accidental combination for action effects作用代表值: representative value of an action 作用代表值作用标准值: characteristic value of an action 地震作用标准值: characteristic value of earthquake action 作用标准值可变作用标准值: characteristic value of variable action作用频遇值 Frequent value of an action 作用频遇值safe class安全等级: safety class|Security Level|safeclass 安全等级生物安全等级: Biosafety Level生物安全等級: Biosafety Levelactionactivityactions 作用 actseffectto play a roledesign reference period 设计基准期设计基准期: design reference period作用准永久值: quasi,permanentvalueofanaction 作用准永久值作用效应: effects of actions|effect of an action 互作用效应: interaction effect 作用效应质量作用效应: mass action effect作用效应设计值 Design value of an action effect 作用效应设计值分项系数: partial safety factor|partial factor 作用分项系数: partial safety factor for action 分项系数抗力分项系数: partial safety factor for resistance 作用效应组合: combination for action effects 作用效应基本组合: fundamental combination for action effects 作用效应组合作用效应偶然组合: accidental combination for action effects结构重要性系数Coefficient for importance of a structure 结构重要性系数桥涵跟桥梁比较类似~主要区别在于:单孔跨径小于5m或多孔跨径之桥涵和小于8m的为桥涵,大于这个标准的为桥梁公路等级: highway classification 标准:公路等级代码: Code for highway classification 公路等级标准:公路路面等级与面层类型代码: Code for classification and type of highway pavementfair current 顺流设计洪水频率: designed flood frequency 设计洪水频率water powerwater conservancyirrigation works 水力水力: hydraulic power|water power|water stress 水力学: Hydraulics|hydromechanics|fluid mechanics 水力的:hydraulic|hydrodynamic|hydriver channel河槽: stream channel|river channel|gutter 河槽古河槽: old channel 河槽线: channel axisriversidestrand河岸: bank|riverside|river bank 河岸河岸林: riparian forest河岸权: riparian rightstream bank erosion河岸侵蚀: bank erosion|stream bank erosion 河岸侵蚀河岸侵蚀河岸侵食: bank erosion 河岸侵蚀, 堤岸冲刷: bank erosion 高架桥桥墩: viaduct pier 高架桥桥墩高潮时桥梁净空高度: bridge clearance 桥梁净空lane行车道: carriageway|traffic lane|Through Lane 行车道快行车道: fast lane西行车道: westbound carriageway A roadarterial roadarterial highway 一级公路一级公路: A road arterial road arterial highway一级公路网: primaryhighwaysystem b roadsecondary road 二级公路二级公路: B road, secondary road culvert涵洞: culvert 涵洞梁涵洞: Beam Culverts木涵洞: timber culvertriverbedrunway河床: river bed|bed|stream bed 河床冰河床: glacier bed型河床: oxbow|horseshoe bend|meander loopflood plainriver beach河滩: river shoal|beach|river flat 河滩河滩地: flood land|overflow land 河滩区: riffle areahigh-type highway 高级公路高级公路: high-typehighwaytrestleviaduct高架桥: viaduct|overhead viaduct 高架桥高架橋: Viadukt|Viaducto|高架橋高架桥面: elevated deckvolume of floodflood dischargeflooddischarge 洪水流量洪水流量: flood discharge|flood flow|peak discharge洪水流量预报: flooddischargeforecast 平均年洪水流量: average annual flood design speed设计速度: design speed|designed speed|design rate设计速度~构造速度: desin speed|desin speed <haha 设计速度最大阵风强度的设计速度: VB Design Speed for Maximum GustIntensionspan 跨度emergency shutdown (cut-off)emergency cut-off紧急停车: abort|panic stop|emergency stop 紧急停车紧急停车带: lay-by|emergency parking strip 紧急停车阀: emergency stop valve gear downretardmentspeed-down 减速decelerationslowdowntraffic lane 车道side tripmarginal strip 路缘带 margin verge路缘带: marginal strip|side strip|margin vergeshoulder of earth body路肩: shoulder|verge|shoulder of road 路肩硬路肩: hardshoulder|hardened verge 软路肩: Soft Shoulderminimum value最小值: minimum|Min|least value 最小值求最小值: minimization 找出最小值: minmax. 最大值principle of the maximummaximum principlemaximal principle 最大值原理最大值原理: maximum principle,|maximal principle 离散最大值原理: discrete maximum principle 极大值原理~最大值原理: maximum principle 车道宽度: lane-width 车道宽度cycle-track自行车道: bicycle path|cycle path|cycle track 自行车道旗津环岛海景观光自行车道: Cijin Oceanview Bike Path自行车道专供自行车行驶的车道。
mpa与kn之间的换算关系
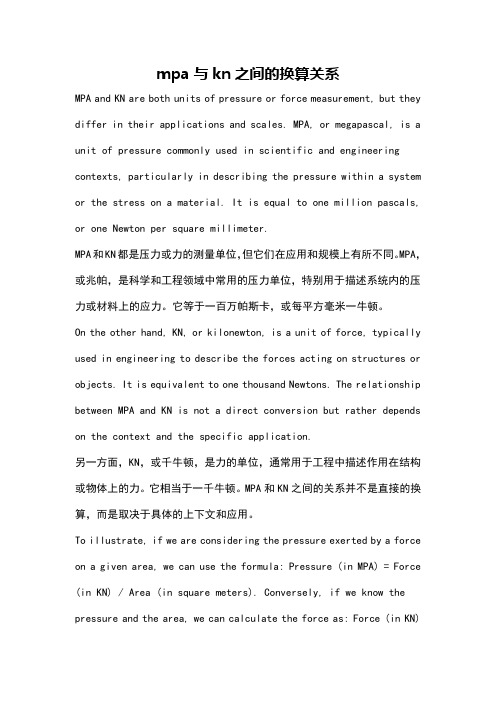
mpa与kn之间的换算关系MPA and KN are both units of pressure or force measurement, but they differ in their applications and scales. MPA, or megapascal, is a unit of pressure commonly used in scientific and engineering contexts, particularly in describing the pressure within a system or the stress on a material. It is equal to one million pascals, or one Newton per square millimeter.MPA和KN都是压力或力的测量单位,但它们在应用和规模上有所不同。
MPA,或兆帕,是科学和工程领域中常用的压力单位,特别用于描述系统内的压力或材料上的应力。
它等于一百万帕斯卡,或每平方毫米一牛顿。
On the other hand, KN, or kilonewton, is a unit of force, typically used in engineering to describe the forces acting on structures or objects. It is equivalent to one thousand Newtons. The relationship between MPA and KN is not a direct conversion but rather depends on the context and the specific application.另一方面,KN,或千牛顿,是力的单位,通常用于工程中描述作用在结构或物体上的力。
engineering failure analysis的novelty statement模板

engineering failure analysis的novelty statement模板Title: Novelty Statement for Engineering Failure AnalysisIntroduction:Engineering Failure Analysis plays a crucial role in identifying the root causes of failures in various engineering systems and assessing their impact. To further enhance this field, it is essential to highlight the novelty and advancements in approaching failure analysis. Therefore, the following template aims to guide the creation of a novelty statement for Engineering Failure Analysis.[Statement Template]1. Introduce the Field of Engineering Failure Analysis:The field of Engineering Failure Analysis investigates the causes, mechanisms, and consequences of engineering failures. It involves analyzing the technical, operational, and environmental factors that contribute to the failure, with the ultimate goal of preventing future occurrences. The recent advancements in this field have led to significant contributions to industry standards, safety practices, and technological improvements.2. Highlight the Existing Methods and Tools:Traditional engineering failure analysis methods rely on visual inspection, laboratory testing, and expert knowledge. These methods, although effective, have limitations in terms of time, cost, and the ability to analyze complex failure scenarios. Despite these challenges, they have provided a solid foundation for understanding failures, diagnosing their causes, and proposing remedial actions.3. Address the Need for Improved Approaches:While existing methods have been valuable, novel approaches in engineering failure analysis are crucial to address emerging challenges and enhance the accuracy, efficiency, and effectiveness of failure investigations. These approaches should strive to overcome limitations by incorporating advancements in various fields, such as materials science, data analysis, computational modeling, and remote sensing.4. Present the Novelty and Advancements:[a. Methodological Innovations]Describe novel methodologies or techniques that offer a fresh perspective on failure analysis. These may include advancements in non-destructive testing, image recognition, machine learning, or statistical analysis that enable faster, more accurate identification of failure causes.[b. Multi-disciplinary Approaches]Highlight the integration of multiple scientific and engineering disciplines in failure analysis. Novel collaborations that bring together experts from diverse fields, such as materials engineering, mechanical engineering, electrical engineering, and computer science, can lead to holistic and comprehensive failure investigations.[c. Application of Advanced Technologies]Discuss the utilization of cutting-edge technologies, such as remote sensing, unmanned aerial vehicles (UAVs), or virtual reality, to enhance the data collection, visualization, and analysis in failureinvestigations. These technological advancements can facilitate remote monitoring, real-time data acquisition, and simulation-based analyses.5. Anticipated Impact and Future Directions:Conclude by emphasizing the impact of these novel approaches on the field of Engineering Failure Analysis. Highlight their potential to improve failure prevention, enhance safety, optimize designs, and ultimately reduce economic losses. Also, address the future research directions, emphasizing the importance of continuous innovation and collaborations to advance engineered systems' reliability and resilience.[Example Novelty Statement]Title: Advancing Failure Analysis through Non-destructive Testing and Machine LearningIntroduction:Engineering Failure Analysis is a critical discipline that investigates the causes and consequences of failures in various engineering systems. Although traditional methods, such as visual inspection and laboratory testing, have been valuable, there is a need to embrace novel approaches that leverage advanced technologies to enhance failure analysis accuracy and efficiency. This novelty statement focuses on the integration of non-destructive testing (NDT) and machine learning methodologies to improve failure investigations and prevent future occurrences.1. Introduce the Field of Engineering Failure Analysis: Engineering Failure Analysis aims to understand the reasonsbehind failures, enabling the development of preventive measures and design improvements. It plays a vital role in industries such as aerospace, automotive, civil engineering, and energy production, ensuring safety and reliability.2. Highlight the Existing Methods and Tools:Traditional failure analysis methods involve visual inspection, laboratory testing, and expert judgment. While effective, these methods often require significant time and cost investment, limiting their applicability in large-scale or time-sensitive scenarios.3. Address the Need for Improved Approaches:To overcome existing limitations, novel approaches integrating NDT and machine learning offer promising avenues for more efficient and accurate failure analysis. These approaches maximize the usefulness of the data collected during NDT by leveraging machine learning algorithms to automatically identify patterns and anomalies.4. Present the Novelty and Advancements:[a. Methodological Innovations]By combining NDT techniques, such as ultrasonic testing, radiography, and thermography, with machine learning algorithms, engineers can quickly and accurately analyze large datasets obtained from failure investigations. Machine learning algorithms can identify intricate failure patterns and establish correlations between failure causes and various factors, such as material properties and operational conditions.[b. Multi-disciplinary Approaches]The integration of NDT and machine learning requires collaboration between engineers, materials scientists, computer scientists, and data analysts. This multidisciplinary approach ensures comprehensive failure investigations by considering various aspects of the failure mechanism and addressing the limitations of individual disciplines.[c. Application of Advanced Technologies]Utilizing advanced sensors and robotics, NDT techniques can be deployed remotely, allowing continuous monitoring and inspection of critical engineering systems. Machine learning also enables real-time analysis of sensor data, facilitating rapid identification of potential failure causes and the evaluation of remedial actions.5. Anticipated Impact and Future Directions:The integration of NDT and machine learning offers the potential to revolutionize failure analysis in engineering. By enabling rapid and accurate identification of failure causes, this approach can significantly reduce downtime, prevent catastrophic failures, optimize maintenance schedules, and enhance the overall reliability of engineered systems. Further research is needed to explore the full potential of this novel approach and identify additional applications for advanced NDT and machine learning technologies in failure analysis.Note: The provided novelty statement template is just a suggested guide. Users should modify and adapt it according to their specific research or engineering failure analysis context.。