微生物分析方法
微生物的鉴定(精)

引言概述:微生物的鉴定是微生物学中的一个重要课题,它涉及到鉴定和分类微生物的种类、特性和功能等方面。
微生物的鉴定对于环境保护、农业生产、医学诊断等领域起着重要的作用。
本文将从微生物的分离培养、形态学特征、生理生化特性、分子生物学鉴定和抗生素敏感性等五个大点进行详细阐述微生物的鉴定方法和技术。
正文:一、微生物的分离培养1. 选择培养基:根据待鉴定微生物的特性,选择合适的培养基,如富含营养物质的寒天培养基、蔗糖琼脂培养基等。
2. 分离培养:采用传统的分离培养方法,如涂布法、稀释法等,将微生物分离为单个菌落。
3. 纯化培养:通过多次传代培养,并观察菌落形态和特性,将单菌落转移到新的培养基上,获得纯种微生物。
二、微生物的形态学特征1. 形态学观察:利用显微镜观察微生物的形态特征,如形状、大小、颜色、胞壁结构等。
2. 细胞结构分析:通过染色方法,观察微生物的细胞结构,如细胞壁、细胞膜、核质、细胞器等。
3. 胞内含物分析:利用染色剂或特定试剂,观察微生物胞内含物,如颗粒、颜色、气泡等。
三、微生物的生理生化特性1. 新陈代谢特性:通过培养微生物在不同营养条件下的生长情况观察其代谢特性,如需氧性、产酸性、产气性等。
2. 饮食特性:测定微生物对不同营养物质的利用情况,如碳源、氮源、矿物质等。
3. 酶活性分析:应用酶学方法检测微生物体内的酶活性,如蛋白酶、淀粉酶、脂肪酶等。
四、微生物的分子生物学鉴定1. 16S rRNA基因测序:提取微生物的基因组DNA,并进行PCR 扩增和测序分析,根据16S rRNA基因序列比对和构建系统发育树,进行物种鉴定。
2. 基因组学方法:通过全基因组测序和比较基因组学分析,探索微生物的基因组特征,如基因组大小、基因编码等。
3. 荧光原位杂交技术:利用具有特异性的探针标记微生物的特定序列,通过荧光显微镜观察微生物的存在和分布情况。
五、微生物的抗生素敏感性1. 抗生素敏感试验:采用纸片扩散法或高通量平板法,将不同浓度的抗生素施加在微生物生长培养板上,观察不同抗生素对微生物生长的影响。
微生物群落分析的技术与方法综述
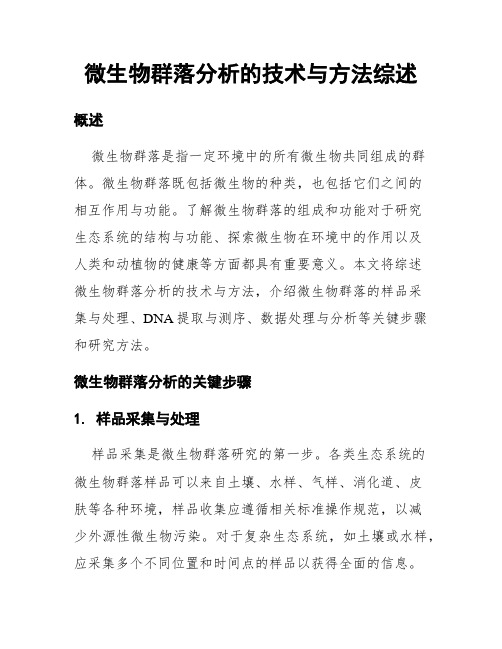
微生物群落分析的技术与方法综述概述微生物群落是指一定环境中的所有微生物共同组成的群体。
微生物群落既包括微生物的种类,也包括它们之间的相互作用与功能。
了解微生物群落的组成和功能对于研究生态系统的结构与功能、探索微生物在环境中的作用以及人类和动植物的健康等方面都具有重要意义。
本文将综述微生物群落分析的技术与方法,介绍微生物群落的样品采集与处理、DNA提取与测序、数据处理与分析等关键步骤和研究方法。
微生物群落分析的关键步骤1. 样品采集与处理样品采集是微生物群落研究的第一步。
各类生态系统的微生物群落样品可以来自土壤、水样、气样、消化道、皮肤等各种环境,样品收集应遵循相关标准操作规范,以减少外源性微生物污染。
对于复杂生态系统,如土壤或水样,应采集多个不同位置和时间点的样品以获得全面的信息。
在采集过程中还要注意样品处理,如快速冷冻或添加保护剂,以保持微生物群落的原样。
2. DNA提取与测序DNA提取是微生物群落分析的关键步骤之一。
常用的方法包括化学裂解、机械裂解和热激裂解等,以从样品中提取微生物细胞的DNA。
DNA浓度和纯度的测定对于后续的测序和分析非常重要。
提取到的DNA可通过PCR扩增特定区域的基因(如16S rRNA或ITS等)以获得微生物群落的信息。
目前,高通量测序技术如Illumina MiSeq或PacBio Sequel等已经逐渐取代传统的Sanger测序,成为微生物群落测序的主流技术。
3. 数据处理与分析对于微生物群落测序数据的处理与分析是微生物群落研究的最关键部分。
首先,需要对原始测序数据进行质量控制、截断和过滤,以去除低质量序列和噪音。
然后,对截断、过滤后的序列进行聚类,得到OTUs(操作分类单元)或ASVs(对应序列变体)。
随后,可以计算微生物群落的多样性指数,如物种丰度、Shannon指数等。
对不同样品之间的微生物群落进行比较,可以使用多样性分析、主坐标分析(PCoA)、非参数多元分析(NMDS)等方法。
检验科常见病原微生物检测方法
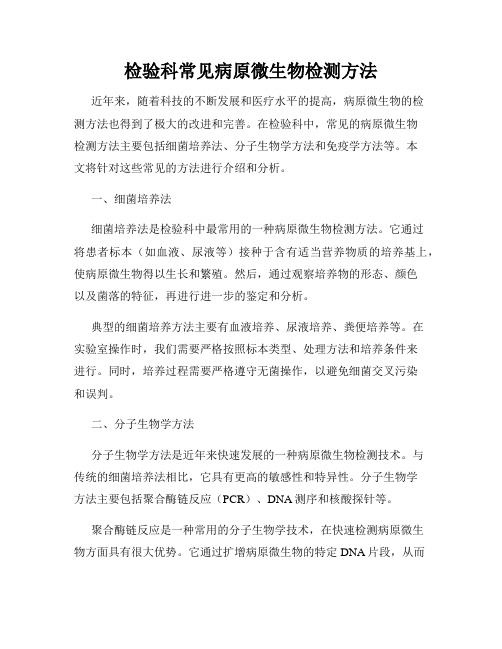
检验科常见病原微生物检测方法近年来,随着科技的不断发展和医疗水平的提高,病原微生物的检测方法也得到了极大的改进和完善。
在检验科中,常见的病原微生物检测方法主要包括细菌培养法、分子生物学方法和免疫学方法等。
本文将针对这些常见的方法进行介绍和分析。
一、细菌培养法细菌培养法是检验科中最常用的一种病原微生物检测方法。
它通过将患者标本(如血液、尿液等)接种于含有适当营养物质的培养基上,使病原微生物得以生长和繁殖。
然后,通过观察培养物的形态、颜色以及菌落的特征,再进行进一步的鉴定和分析。
典型的细菌培养方法主要有血液培养、尿液培养、粪便培养等。
在实验室操作时,我们需要严格按照标本类型、处理方法和培养条件来进行。
同时,培养过程需要严格遵守无菌操作,以避免细菌交叉污染和误判。
二、分子生物学方法分子生物学方法是近年来快速发展的一种病原微生物检测技术。
与传统的细菌培养法相比,它具有更高的敏感性和特异性。
分子生物学方法主要包括聚合酶链反应(PCR)、DNA测序和核酸探针等。
聚合酶链反应是一种常用的分子生物学技术,在快速检测病原微生物方面具有很大优势。
它通过扩增病原微生物的特定DNA片段,从而提高检测的准确性和灵敏度。
此外,PCR还可以进行多重扩增和实时扩增,进一步提高了检测效果。
DNA测序是一种更加精确的病原微生物检测方法。
通过将扩增得到的DNA片段进行测序,可以准确地确定其序列,进而进行比对和分析。
这种方法在对未知病原微生物的鉴定和新病原体的发现上具有重要的意义。
核酸探针是一种利用亲核反应原理进行病原微生物检测的方法。
它通过将已知病原微生物特异性序列的亲核核酸标记上特定荧光物质,通过特异性结合来检测目标病原微生物的存在。
三、免疫学方法免疫学方法是利用人体自身免疫系统对抗病原微生物的原理进行病原微生物检测的一种方法。
它主要包括血清学检测、免疫组化法、免疫电镜等。
血清学检测是一种通过检测患者血清中的抗体来判断病原微生物感染情况的方法。
微生物毒素的分析和检测技术
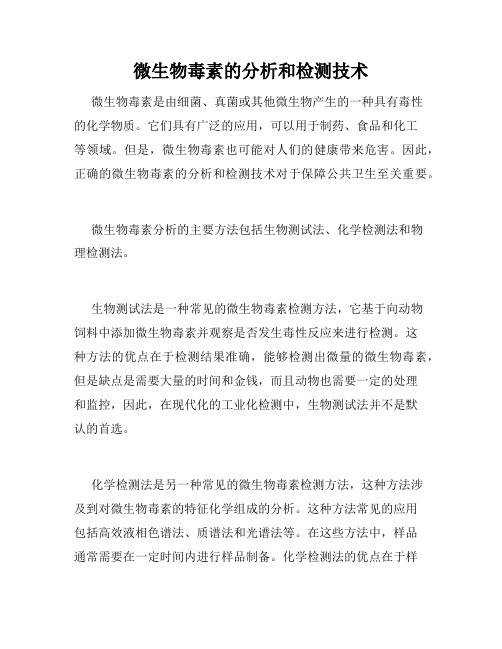
微生物毒素的分析和检测技术微生物毒素是由细菌、真菌或其他微生物产生的一种具有毒性的化学物质。
它们具有广泛的应用,可以用于制药、食品和化工等领域。
但是,微生物毒素也可能对人们的健康带来危害。
因此,正确的微生物毒素的分析和检测技术对于保障公共卫生至关重要。
微生物毒素分析的主要方法包括生物测试法、化学检测法和物理检测法。
生物测试法是一种常见的微生物毒素检测方法,它基于向动物饲料中添加微生物毒素并观察是否发生毒性反应来进行检测。
这种方法的优点在于检测结果准确,能够检测出微量的微生物毒素,但是缺点是需要大量的时间和金钱,而且动物也需要一定的处理和监控,因此,在现代化的工业化检测中,生物测试法并不是默认的首选。
化学检测法是另一种常见的微生物毒素检测方法,这种方法涉及到对微生物毒素的特征化学组成的分析。
这种方法常见的应用包括高效液相色谱法、质谱法和光谱法等。
在这些方法中,样品通常需要在一定时间内进行样品制备。
化学检测法的优点在于样品的制备和处理相对快速,而且能够实现较大规模的检测,不需要动物等生物实验。
物理检测法也是一种微生物毒素分析和检测的方法。
这种方法的主要缺点在于它需要对样品进行物理加工,以便检测出特定的微生物毒素。
因此,如果样品未得到适当的处理,该方法的准确度和可靠性都可能大打折扣。
在实际应用中,选择针对特定微生物毒素的特定检测方法,通常会通过分析样品中的化学组成来寻找可能存在的毒素。
一些公认权威的方法可用于检测目标微生物毒素的存在,这可能是基于与已知化学结构(例如四环素类合成物),或者特定细菌具有的毒素。
例如,拉曼光谱法是一种通过使用光学技术来检测微生物毒素的方法。
这种方法是非侵入性和非破坏性的,因此在检测环节对样品的影响非常小。
对于样品的处理准备也非常简单。
芗草羧琥珀酸酯盐酸盐(PAT)和四环素(TET)中毒株产生的毒素可以通过拉曼光谱检测 arotene环的特定振动模式而得到鉴定。
在实际应用中,选择适当的检测技术对于检测微生物毒素的存在非常关键。
微生物化验方法
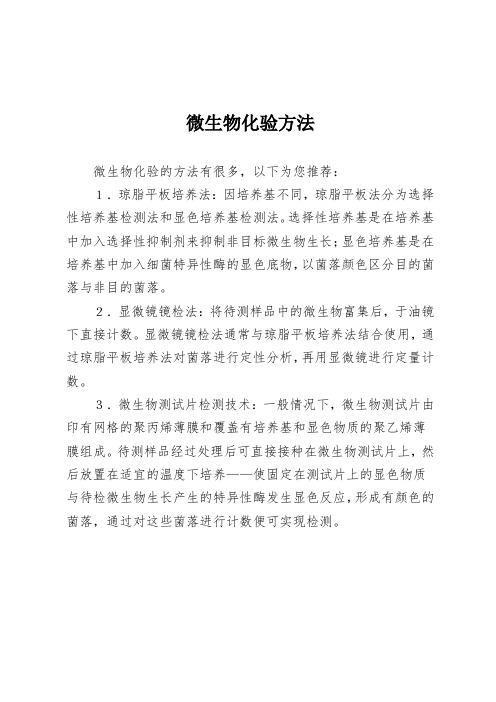
微生物化验方法
微生物化验的方法有很多,以下为您推荐:
1.琼脂平板培养法:因培养基不同,琼脂平板法分为选择性培养基检测法和显色培养基检测法。
选择性培养基是在培养基中加入选择性抑制剂来抑制非目标微生物生长;显色培养基是在培养基中加入细菌特异性酶的显色底物,以菌落颜色区分目的菌落与非目的菌落。
2.显微镜镜检法:将待测样品中的微生物富集后,于油镜下直接计数。
显微镜镜检法通常与琼脂平板培养法结合使用,通过琼脂平板培养法对菌落进行定性分析,再用显微镜进行定量计数。
3.微生物测试片检测技术:一般情况下,微生物测试片由印有网格的聚丙烯薄膜和覆盖有培养基和显色物质的聚乙烯薄膜组成。
待测样品经过处理后可直接接种在微生物测试片上,然后放置在适宜的温度下培养——使固定在测试片上的显色物质与待检微生物生长产生的特异性酶发生显色反应,形成有颜色的菌落,通过对这些菌落进行计数便可实现检测。
微生物群落结构与环境因素相关性分析

微生物群落结构与环境因素相关性分析随着生物技术的快速发展,微生物群落结构与环境因素相关性分析成为了研究的热点之一。
微生物群落是指在特定环境中共存的微生物的总体,它对环境的变化非常敏感,能够反映环境的健康状况和改变。
因此,了解微生物群落结构与环境因素之间的关系对于环境保护和生物多样性的监测具有重要意义。
一、微生物群落结构的分析方法为了研究微生物群落结构与环境因素之间的关系,首先需要对微生物群落进行分析。
常用的微生物群落分析方法包括:1. 16S rRNA基因分析:通过对微生物群落中16S rRNA基因的测序和分析,可以获得微生物群落的丰富度、多样性和组成。
2. 基于功能基因的分析:通过测定微生物群落中特定功能基因的存在情况,可以了解微生物群落的功能潜力和代谢能力。
3. 元基因组学分析:通过测序微生物群落中的全基因组DNA,可以研究微生物群落的功能特征和代谢通路。
二、环境因素对微生物群落结构的影响环境因素是影响微生物群落结构的重要因素之一。
多种环境因素,如温度、pH 值、适宜的营养条件、氧气含量、盐度等,都能够显著影响微生物群落的结构和组成。
下面以土壤微生物群落为例,具体分析环境因素对微生物群落结构的影响。
1. pH值:土壤的酸碱度通过影响微生物的生理特征和代谢途径,进而影响微生物群落结构。
不同pH值环境下,微生物群落的物种组成和丰度差异明显。
2. 湿度:湿度对土壤微生物的生存和代谢有着重要的影响。
适宜的水分含量可以促进微生物的生长、繁殖和代谢,从而影响微生物群落的结构。
3. 温度:不同温度条件下,微生物的生理特征会发生显著变化,从而导致微生物群落结构的差异。
4. 营养物质:营养物质是微生物生长和代谢的重要来源。
土壤中不同种类和含量的有机和无机物质会影响微生物群落结构的组成和多样性。
三、微生物群落结构与环境因素的相关性分析方法微生物群落结构与环境因素之间的相关性分析是揭示微生物群落对环境变化的响应机制的重要方法之一。
土壤微生物分析方法
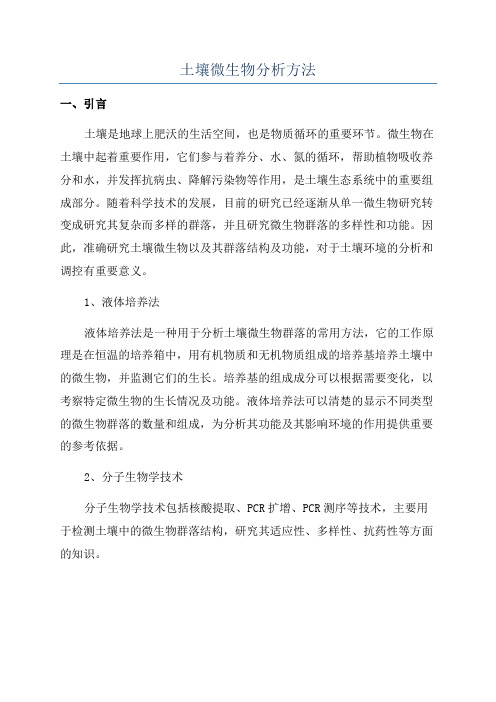
土壤微生物分析方法
一、引言
土壤是地球上肥沃的生活空间,也是物质循环的重要环节。
微生物在土壤中起着重要作用,它们参与着养分、水、氮的循环,帮助植物吸收养分和水,并发挥抗病虫、降解污染物等作用,是土壤生态系统中的重要组成部分。
随着科学技术的发展,目前的研究已经逐渐从单一微生物研究转变成研究其复杂而多样的群落,并且研究微生物群落的多样性和功能。
因此,准确研究土壤微生物以及其群落结构及功能,对于土壤环境的分析和调控有重要意义。
1、液体培养法
液体培养法是一种用于分析土壤微生物群落的常用方法,它的工作原理是在恒温的培养箱中,用有机物质和无机物质组成的培养基培养土壤中的微生物,并监测它们的生长。
培养基的组成成分可以根据需要变化,以考察特定微生物的生长情况及功能。
液体培养法可以清楚的显示不同类型的微生物群落的数量和组成,为分析其功能及其影响环境的作用提供重要的参考依据。
2、分子生物学技术
分子生物学技术包括核酸提取、PCR扩增、PCR测序等技术,主要用于检测土壤中的微生物群落结构,研究其适应性、多样性、抗药性等方面的知识。
常用的微生物检验方法
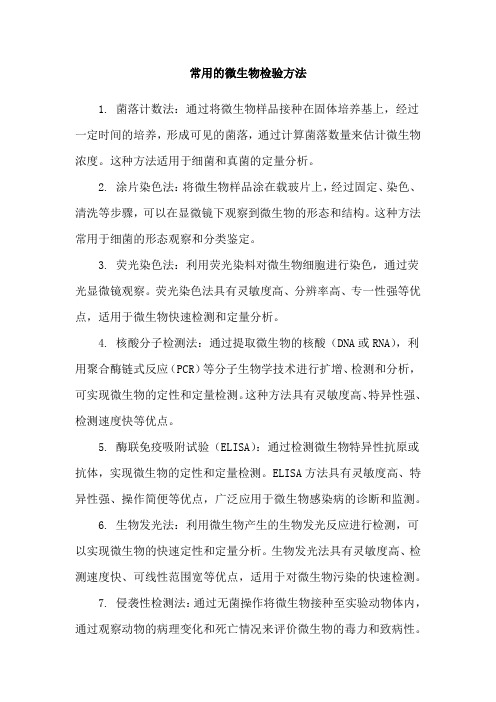
常用的微生物检验方法1. 菌落计数法:通过将微生物样品接种在固体培养基上,经过一定时间的培养,形成可见的菌落,通过计算菌落数量来估计微生物浓度。
这种方法适用于细菌和真菌的定量分析。
2. 涂片染色法:将微生物样品涂在载玻片上,经过固定、染色、清洗等步骤,可以在显微镜下观察到微生物的形态和结构。
这种方法常用于细菌的形态观察和分类鉴定。
3. 荧光染色法:利用荧光染料对微生物细胞进行染色,通过荧光显微镜观察。
荧光染色法具有灵敏度高、分辨率高、专一性强等优点,适用于微生物快速检测和定量分析。
4. 核酸分子检测法:通过提取微生物的核酸(DNA或RNA),利用聚合酶链式反应(PCR)等分子生物学技术进行扩增、检测和分析,可实现微生物的定性和定量检测。
这种方法具有灵敏度高、特异性强、检测速度快等优点。
5. 酶联免疫吸附试验(ELISA):通过检测微生物特异性抗原或抗体,实现微生物的定性和定量检测。
ELISA方法具有灵敏度高、特异性强、操作简便等优点,广泛应用于微生物感染病的诊断和监测。
6. 生物发光法:利用微生物产生的生物发光反应进行检测,可以实现微生物的快速定性和定量分析。
生物发光法具有灵敏度高、检测速度快、可线性范围宽等优点,适用于对微生物污染的快速检测。
7. 侵袭性检测法:通过无菌操作将微生物接种至实验动物体内,通过观察动物的病理变化和死亡情况来评价微生物的毒力和致病性。
这种方法适用于对微生物的生物学特性进行研究。
8. 培养法:通过将微生物样品接种在适宜的培养基中,进行一定时间的培养,通过观察培养物的生长情况和变化来判断微生物的种类和数量。
这种方法适用于多种微生物的检测和鉴定。
- 1、下载文档前请自行甄别文档内容的完整性,平台不提供额外的编辑、内容补充、找答案等附加服务。
- 2、"仅部分预览"的文档,不可在线预览部分如存在完整性等问题,可反馈申请退款(可完整预览的文档不适用该条件!)。
- 3、如文档侵犯您的权益,请联系客服反馈,我们会尽快为您处理(人工客服工作时间:9:00-18:30)。
Microorganisms play an integral and often unique role in ecosystem functions, yet we know little about dominant populations that presumably play vital roles in these functions. Soil microbial communities are the most complex, diverse, and important assemblages in the biosphere.Analysis of genetic diversity in soil communities by DNA renaturation suggests that there are at least 103 or even more species per gram of soil (Torsvik et al., 1990). However, many previous studies revealed function groups with few or no known the dominant populations at the division or phylum level, perhaps because only a limited number of microorganisms have been obtained in the isolating experiments. Virtually, in many ecosystems, activity of microorganisms is limited by the availability of substrate (Belser, 1979); thus we can image how difficulty for one thousand or even more species survive in one gram of soil! Hence, most of the species cells probably are dormant in soil in without extra carbon input condition. Several experiments on organic matter decomposition support our inference, when fresh plant residues in soil, fluctuations of microbial biomass N and C could be observed (Calderόn et al., 2001; Griffiths et al., 2001; Priemé and Christensen, 2001).So, the dominant microorganism populations in soil are not only have great number of cells, but also must be active in soil. To better understand the dominant microorganism populations in soil, all activity microorganisms were assumed to take up carbon only in the form of soluble, directly consumable substrate dissolved in soil solution. Soil-water-extraction media were used (Semenov et al., 1996; Zelenev et al.,2000), two trophic groups of microorganisms (copiotrophs and oligotrophs) were selected.Semenov, A.M., Batomunkueva, B.P., Nizovtseva, D.V., Panikov, N.S., 1996. Method of determination of cellulase activity in soils and in microbial cultures, and its calibration. Journal of Microbiological Methods 24, 259–267.Zelenev, V.V., Berkelmans, R., van Bruggen, A.H.C., Bongers, T., Semenov, A.M., 2004. Daily changes in bacterial feeding nematode populations oscillate with similar periods as bacterial populations after a nutrient impulse in soil. Applied Soil Ecology 26, 93–106.Torsvik, V., Goksoyr, J., Daae, F., 1990. High diversity in DNA of soil bacteria. Applied Environmental Microbiology 56, 782–787.Belser L. W. (1979) Population ecology of nitrifying bacteria. Annual Review of Microbiology 33, 309-333.Calderόn, F.J., Jackson, L.E., Scow, K.M., Rolston, D.E., 2001. Shortterm dynamics of nitrogen, microbial activity, and phospholipids fatty acids after tillage. Soil Science Society of America Journal 65,118–126.Griffiths, B.S., Bonkowski, M., Roy, J., Ritz, K., 2001. Functional stability, substrate utilisation and biological indicators of soils following environmental impacts. Applied Soil Ecology 16, 49–61.Priemé, A., Christensen, S., 2001. Natural perturbations, drying-wetting andfreezing-thawing cycles, and the emission of nitrous oxide, carbon dioxide and methane from farmed organic soils. Soil Biology &Biochemistry 33, 2083–2091.premise received from due owing signature筛选habitat.However, none of these studies have reached the level of diversity revealed by DNA–DNA hybridization methods, Thus, despite recent expansion in divisions by several previous studies (Dojka et al., 1998; Hugenholtz et al.,1998a,b), our understanding of the extent of microbial diversity is still incomplete. More studies on a variety of soil types and habitats are needed to obtain a comprehensive view of microbial community composition and structure in soil environments.Conversion of natural ecosystems to agriculture as well as increasing intensity of tillage are known to decrease soil organic matter (SOM) levels and contribute significantly to the increase in atmospheric CO2 concentration (Lal et al., 1998). Between 1850 and 1995, SOM mineralization emitted 136 ±55 ×1015 g of CDuring several years we investigated the stages of corn residue decomposition in Mollisols and observed significant oscillations of microorganism populations: Pseudomonas is the dominant population during 40-80d decomposition period; and then at the period of 80-100d decomposition, Bacillus, Penicillium, Aspergillus, Mucor are the dominant populations; At 180d decomposition, Stachybotrys, Trichoderma, Dematium and Phoma are the dominant populations; At 240d decomposition, Polyangium, Cellvibro, Nocardia, Micromonospora and Penicillium are the dominant populations.two trophic groups of microorganisms: Zymogeneous microorganisms and Autochthonous microorganisms. The former have low substrate affinity and grow relatively fast on C rich media (1000mgCL-1), while the latter have high substrate affinity and grow more slowly, but better on C poor (10mgCl_1) than on C rich media. Copiotrophic and oligotrophic bacteria roughly correspond to r- andK-strategists, but there are many exceptions in both trophic groups.or incorporation ofCorn is the most important cash crops of China, and are mostly produced in Northeast China. This large area is covered by different type of Mollisols. These soils vary greatly in their inherent properties, like morphology, texture and mineralogy. Soils were under grassland vegetation up to about 200 years ago. Since then, they have different agricultural histories, which resulted in different degradation levels soils lost 42 and 59% of their soil organic carbon (SOC) stock upon conversion from forest to crop and from grassland to crop, respectively.In contrast, Mann (1986) calculated losses <20% of the initial stock, which wascorroborated by Kern and Johnson (1993) andConversion of natural ecosystems to agriculture as well as increasing intensity of tillage are known to decrease soil organic matter (SOM) levels and contribute significantly to the increase in atmospheric CO2 concentration (Lal et al., 1998). Between Lal, R., Follett, R.F., Kimble, J.M., Cole, C.V., 1998. The Potential of US Cropland to Sequester Carbon and Mitigate the Greenhouse Effect. Lewis Publisher, Boca Raton, FL, 128 pp.residues returned 1993).From the 1990 decade the farmers grew soybean using zero tillage (ZT) systems, which progressively replaced the more aggressive conventional tillage (CT) systemsbroth blended horizonThe main objectives of this paper are: (1) to evaluate environmental factors that regulate bacterial abundance during guano decomposition; (2) to estimate the rate of decomposition of guano with an emphasis on percentage changes in fats, protein, chitin, ash, TOC (total organic carbon), nitrogen, phosphorus, and some other elements; (3) to examine the quantitative and qualitative transformations in the bacterial population during penguin guano decomposition; (4) to isolate and identify the dominant culturable bacteria as a first step in evaluating their diversity, and to preselect strains for further phylogenetic analysis.Culturable bacteria (colony forming units, CFU) were enumerated by the spread plate method on: (a) full strength nutrient Soil Extract Agar (SEA) for the isolation of copiotrophic bacteria, and 10! diluted NA for the bacteria more oligotrophic (Ogram and Feng, 1997). Agar plates were inoculated with 100 ml from each dilution in a decimal series of the homogenate. One liter of NA includes bacto beef extract (3 g), bacto-tryptone (5 g), bacto-agar 15 g (Fenchel and Hemmingsen, 1974) in 1 l of soil extract prepared according to Klement et al. (1990). CFU growth was examined every 2–3 days through 25–30 days incubation at 10 8C. This was done to compare the kinetics of bacterial colony increment among bacterial populations, isolated in different stages of guano decomposition. At theend of incubation, CFU counts were calculated. The standard error (SE) in CFU counts was determined from four replicates. For each experimental day (0, 2, 6, 10, 15, 20, 25, 30, 42), an average of 15 colonies were randomly selected, and subcultured for purification through repeated transfers on the same medium, and physiological investigations. In total, 136 colonies were so isolated.Chitinolytic bacteria were enumerated on a solid medium containing solubledye-labeled chitin (CM-Chitin-RBV solution; LOEWE Biochemica GmbH) prepared according to Klement et al. (1990).Morphological, physiological and biochemical features of the isolated bacteria were determined on the basis of 49 tests, including growth at 4, 22 and 32 8C, Gram stain, morphology (rods or cocci), motility (det ermined in hanging drop preparations), colony pigmentation (examined macroscopically), growth in 4% NaCl, and responses in API 20NE and API ZYM systems (API bioMe´rieux; Tearle and Richard, 1987; Zdanowski and Donachie, 1993; Zdanowski and Weglenski, 2001). A dendrogram showing the hierarchical classification of isolates based on the tests described was constructed by the simple matching coefficient of Sokal and Michener (1958) in association with the weightedpair-group-average algorithm (Sneath and Sokal,1974).Ogram, A., Feng, X., 1997. Methods of soil microbial community analysis. In: Hurst, C.J., Knudsen, G.H., McInerney, M.J., Stetzenbach, L.D., Walter, M.V. (Eds.), Manual of Environmental Microbiology. ASM Press, Washington, DC, pp. 422–430.Fenchel, T., Hemmingsen, B., 1974. Manual of Microbial Ecology.Akademisk Forlag. University of Aarhus, pp. 1–235.Mollisols are the soils of grassland ecosystems. They are characterized by a thick, dark surface horizon. This fertile surface horizon, known as a mollic epipedon, results from the long-term addition of organic materials derived from plant roots.Mollisols primarily occur in the middle latitudes and are extensive in prairieregions such as the Great Plains of the US. Globally, they occupy ~7.0% of the ice-free land area. In the US, they are the most extensive soil order, accounting for ~21.5% of the land area.Mollisols are among some of the most important and productive agricultural soils in the world and are extensively used for this purpose.Mollisols are divided into 8 suborders: Albolls, Aquolls, Rendolls, Gelolls, Cryolls, Xerolls, Ustolls, and Udolls. Click here for more information about these suborders. Click here to view a map of their distribution in the US. Microbes play an important role in soil formation, ecosystem biogeochemistry,(Konopka and Turco, 1991; Hiebert and Bennett, 1992; Madsen, 1995; Richter and Markewitz, 1995), Konopka, A., Turco, R., 1991. Biodegradation of organic compounds invadose zone and aquifer sediments. Applied and EnvironmentalMicrobiology 57, 2260–2268.Hiebert, F.K., Bennett, P.C., 1992. Microbial control of silicate weatheringin organic-rich ground water. Science (Washington DC) 258, 278–281.Madsen, E., 1995. Impacts of agricultural practices on subsurface microbialecology. Advances in Agronomy 54, 1–67.Richter, D., Markewitz, D., 1995. How deep is soil? BioScience 45,600–609.Microbial community composition may be one important control on soil processes (Schimel, 1995; Cavigelli and Robertson, 2000; Balser et al., 2002).Schimel, J., 1995. Ecosystem consequences of microbial diversity andcommunity structure. In: Iii, F.C., Korner, F. (Eds.), Arctic and AlpineBiodiversity, Ecological Studies, vol. 113. Springer, New York, pp.239–254.Cavigelli, M.A., Robertson, G.P., 2000. The functional significance ofdenitrifier community composition in a terrestrial ecosystem. Ecology(Washington DC) 81, 1402–1414.Balser, T., Kinzig, A., Firestone, M., 2002. The functional consequences ofbiodiversity. In: Kinzig, A., Pacala, S., Tilman, D. (Eds.), TheFunctional Consequences of Biodiversity, Princeton University Press,Princeton, pp. 265–293.From 2003 to 2004,108 black soil samples of corn crop were taken fromYushu,Dehui,Changchun and GongzhulingoWe used three kinds of isolating methods(Thin liquid plate method, Soil particle washing technique and Soil plate method ),131 Isolates obtained were identified. The partial soil sample mycobiota and ecological characteristics were studied.Mycobiota studies were focused on soil fungi quantity, genus and species distribution of black soil corn crop .The varity of soil fungi quantity of black soil corn crop in different films were studied,the varity of genus and species in different films were studied,too. We analysed and discussed seasonal variation and veritical distribution of black soil fungi.Ecological characteristics preliminary studied the movement of black soil fungi number and the relationship with 6 kinds of environmental factors(Water contents,pH, available nitrogen, available phosphorus,available postassium and organic matter) through the timing of the corn growing.And studied the varity of black soil mycobiota in different fertilizational black soil.On the basis of morphological observation, we identified as 25 genera,55 species and 4 varieties of fungi. 14 species are the new recorded species in Jilin.25 genera, Acremonium Link, Alternaria Nees, Arthrobotrys Corda.、Aspergillus (Mich.) Link, Beauveria Vuill., Circinella sp.v.Tiegh. et Monn., Cladosporium Link, Curvularia Boed.、Epicoccum Link, Exophiala Carm., Fusarium Link, Geotrichum Link., Humicola Traaen, Kabatiella Bub.、Mucor Mich. ex Fr.、Myrothecium Tode, Neurospora Shear et Didge.、Paecilomyces Bain.、Papulaspora Preuss.、Penicillium Link, Phialophora sp.Medlar.、Pythium Pringsh.、Rhizopus Ehrenb.、Scopulariopsis Bain. and Trichoderma Pers.. 59 species刀cremonium kiliense Griitz., Acremonium strictum WGams. Alternaria alternata (Fr.) Keissl.、Arthrobotrys superba Corda.、Aspergillus sydowii (Bain.et Sart.)Thom et church.、Aspergillus carnus IV (v.Tiegh.)Thom., Aspergillus niger v.Tiegh.、Aspergillus terreus Thom var.aureus Thom&Raper.、Aspergill us如vus Link:Fr.var.flavus, Aspergillus melleus Yukawa., Aspergillus penicilloides Speg.、Aspergillus puniceus Kwon&Fenn.、Aspergillus fumigates Fres.、Aspergillus ustus (Bain)Thom&Church.、Aspergillus ochraceus Wilh.、Aspergillus versicolor(Vuill.)Tirab.vanversicolor, Aspergillus terricola March., Beauveria brongniarth (Sacc.) Petch.、Cladosporium herbarum (Pers.) Link blab Sacc, Cladosporium carpophilum Thum.、Cladosporium tenissimum Cooke.、Curvularia lunata (Walk.) Boed.、Epicoccum purpurascens Ehrenb.、Exophiala jeanselmer (Langeron) McGinnis.、Fusarium oxysporum Schlecht., Fusarium mondiforme.var.inteimedium, Fusarium merismoides Corda.、Geotrichum candidum Link.、Humicola grisea Traaen.、Humicola fuscoatra Traaen., Kabatia microsticta Bubak.、Mucor hiemalis Wehmer., Myrothecium roridum Tode, Neurospora crassa Shear et Dodge.、Paecilomyces marquandii (Mass.) Hughes., Paecilomyces varioti Bain.、Paecilomyces inflatus (Burnside) Carmichael., Paecilomyces lilacinus (Thom)Samson., Papulaspora equi Shadomy&Dixon.、Penicillium citrinum Thom.、Penicillium decumbens Thom.、Penicillium nigricans (Bain.) Thom.、Penicillium purpurogenum stoll.、Penicillium corylophilum Dierckx.、Penicillium citreomigrum Dierckx.、Penicillium rubrum Stoll.、Penicillium implicatum Biourge.、Penicillium thomii Maire.、Penicillium spinulosum Thom.、Penicillium islandicum Sopp.、Penicillium waksmanii Zal.、Penicillium restrictum Gilm.et Abbott.、Penicillium janthinellum Biourge.、Pythium acanthophoron Sid., Pythium caroliniarum Matth., Rhizopus stolonifer (Ehrenb.) Lind.、Scopulariopsis canadensis Morton&Smith.、Trichoderma atroviride P.karsten,Finl. And Trichoderma harziamum Rifai,Mycol..The results of mycobiota studies showed that Penicillium and Aspergillus is the main genus.The seasonal variation of soil fungi quantity of black soil corn crop in different films is different.There are more fungi in the topsoil(0-20cm deep) than in the subsoil layers(20-40cm deep), the composition of fungi in topsoil and subsoil horizon of all plot s is different.The results of ecological characteristics studies showed that the water contents have straight relationship with the soil fungi numbers in soil layers of normal fertilization soil, it isBacterial and fungal cell counts in the commercial formulations were determined using standard serial dilution plating on 0.1% tryptic soy agar (TSA) for bacteria and potato dextrose agar (PDA) with 50 mg chlortetracycline and 1 ml/L tergitol for fungi (Larkin, 2003). Bacterial counts of_1_109 CFU/g formulation for Bsub and fungal countsof 6.5–7_106 CFU/g for Tvir and Tharz were observed.EM (EM-1; EMRO USA, Tucson, AZ, USA), a mixLarkin, R.P., 2003. Characterization of soil microbial communities under different potato cropping systems by microbial population dynamics, substrate utilization, and fatty acid profiles. Soil Biology & Biochemistry 35, 1451–1466.The study area is located on the Snake River Plain, between4283000000 and 4383000000N and 11482000000 and 11683000000W. The sites occur across an elevation gradient ranging from 860 to1300 m. The area is classified as a temperate semi-desertActive bacteria and fungal numbers in soil were determined for each treatment using methods described by Ingham and Klein (1984). Active bacteria and fungi were estimated by taking a 1.0 ml water sample that was diluted in 9ml of a phosphate buffer (pH 6.0) and shaken at approximately 120 rpm for 5 min. For fungal biomass, a 1 ml aliquot was removed and stained with 1 ml of a 20 fg ml_1 fluorescein diacetate (FDA) solution in a 0.2 M phosphate buffer for 3min.One milliliter of 1.5% agar in a 0.1 M phosphate buffer (pH 9.5) was added to the FDA suspension. The sample was mixed and an aliquot placed on a microscope slide containing a cavity of known volume (Ingham and Klein, 1984). Immediately after preparation, slides were examined for FDA-stained hyphal length by epifluorescent microscopy.Total fungal biomasswas estimated by measuring the length and diameter ofhyphae in 3–6 fields with phase—contrast microscopy. Threeslides were evaluated from each sample and ten fields perslide were evaluated with phase contrast microscopy for totalhyphal length, and three transects were evaluated for FDAstained(active) hyphal length at 160 total magnification.Iodonitrotetrazolium (INT) stain was used for countingactive bacteria (Stadmatiadis et al., 1990). A 1 ml sample ofinitial soil suspension was diluted to a final dilution in a 0.2 mgsoil in 4ml buffer. The suspension was incubated with 4ml offiltered INT buffer for 60 min in the dark at 20 8C. Total bacteriaper ml of water were estimated from the mean number ofbacteria (fluorescent and non-fluorescent bacteria), theiraverage diameter and length per field. Three slides wereevaluated for each sample and ten fields per slide wereevaluated using epifluorescent oil-immersion microscopy todetermine numbers and size of fluorescent and total bacteria(Lodge and Ingham, 1991).Bacterial biomasswas computed fromthe numbers of activeand total bacteria and active and total fungal biomass was determined fromhyphal length. Bacterial biomass was computedfromthe number of soil bacteria per gramof soil by consideringthat the bacterial spheres were 1 mm in diameter. Active andtotal fungal biomass were computed by considering averagehyphal diameter to be 1 mm in diameter and them multiplyingIngham, E.R., Klein, D.A., 1984. Soil fungi relationships between hyphal activity and staining with fluorescein diacetate. Soil Biol. Biochem. 16, 273–278. Microorganisms exhibit an impressive diversity in their metabolic activities and in their interactions with other microbes, plants, and animals. Microbes have much shorter turnover rates than higher plants and therefore, respond more quickly to changes in land management than plants and may be the most sensitive indicator to anthropogenic activities. In the past it has been nearly impossible to discern underlying patterns within ecosystems because of the intrinsic structural and functional diversity of microbial communities. To date, only 1–5% of the world’s microorganisms have been identified (Mills et al., 2007; Nannipieri et al., 2003; Hugenholtz et al.,1998) As a result, microbial processes, the microbes are often placed in a ‘black box’, inputs and outputs measured, but most of the processes that go on inside ‘the box’ are based on inference (Mills et al., 2007; Swift et al., 2004; Brussaard et al.,1997). Recently, there has been an explosion in the identification of microorganisms in the natural world using culture independent techniques. The structure and function of these microbial communities using new molecular technologies can be determined (Mills et al., 2007; Thies, 2007; Nakatsu, 2007; Rogers et al., 2007; Suzuki et al., 1998).Mills, D.K., Entry, J.A., Gillevet, P.M., Mathee, K., 2007. Assessing microbial community diversity using amplicon length heterogeneity polymerase chain reaction. Soil Sci. Soc. Am. J. 71, 572–578.Thies, J.E., 2007. Soil microbial community analysis using terminal restriction fragment polymorphisms. Soil Sci. Soc. Am. J. 71, 579–591.Rogers, S.W., Moorman, T.B., Ong, S.K., 2007. Fluorescent in situ hybridization and micro-autoradiography applied to ecophysiology in soil. Soil Sci. Soc. Am. J.71, 620–631.Suzuki, M., Rappe, M.S., Giovannoni, S.J., 1998. Kinetic bias in estimates of Coastal Picoplankton community structure obtained by measurements ofsmall-subunit rRNA Gene PCR amplicon length heterogeneity. Appl. Environ. Microbiol. 64, 4522–4529.Thies, J.E., 2007. Soil microbial community analysis using terminal restriction fragment polymorphisms. Soil Sci. Soc. Am. J. 71, 579–591.Changes in SOM by different agricultural land uses and practices have been extensively reviewed by Mann (1986), Davidson and Ackerman (1993), Guo and Gifford (2002), and Murty et al. (2002).Guo, L.B., Gifford, R.M., 2002. Soil carbon stocks and land use change: a meta analysis. Glob. Change Biol. 8, 345–360.Murty, D., Kirschbaum, M.U.F., McMurtie, R.E., McGilvray, A., 2002. Does conversion of forest to agricultural land change soil carbon and nitrogen? A review of the literature. Glob. Change Biol. 8, 105–123.ISP 4号培养基、察氏(Czapack)培养基;添加2% 玉米秸秆species S.F (%) S.E.M species S.F (%) S.E.M Acremonium strictum 20.4%Mucor hiemalis43.2% Alternaria alternato27.2%Mucor spinescens39.6% Aspergillus carnus20.0%Neurospora crassa42.4% Aspergillus flavus18.8%Paecilomyces inflatus44.4% Aspergillus fumigatus27.2%Paecilomyces lilacinus 38.8% Aspergillus melleus21.2%Paecilomyces marquandii 34.4% Aspergillus niger84.0%Paecilomyces varioti31.2% Aspergillus ochraceus 18.8%Papulaspora equi27.6% Aspergillus penicilloides 20.8%Penicillim rubrum44.8% Aspergillus puniceus16.4%Penicillim thomii41.2% Aspergillus sydowil18.0%Penicillium citreonjgrum 23.2% Aspergillus terreus31.2%Penicillium citrinum36.8% Aspergillus terricola23.2%Penicillium corylophilum 40.8% Aspergillus ustus15.2%Penicillium decumbens 34.8% Aspergillus versicolor14.8%Penicillium implicatum39.2% Beauverja brongniartii 26.0%Penicillium janthinellum 23.6% Cladosporium berbarum 18.8%Penicillium lslandicum 23.2% Cladosporium carpophilum 24.4%Penicillium purpurogenum 23.6% Cladosporium tenissimum 20.0%Penicillium restrictum94.0% Curvularia lunata25.2%Penicillium spinulosum 27.2% Epicoccum purpurascens 23.2%Penicillium waksmanii 26.8% Exophiala jeanselmer 19.6%Penicillum nigricans21.2% Fusarium merismoides 78.4%Phoma nebulosa49.2% Fusarium moniliforme26.8%Phoma putaminum84.4% Fusarium oxysporum19.6%Rhizopus stolonifer79.2% Geotrichum candidurn 28.8%Scopulariopsis canadensis 7.6% Humicola fuscoatra24.4%Trichoderma atroviride 83.6% Humicola grisea27.2%Trichoderma harziamum 21.6% Kabatia microsticta22.4%Aspergillus niger, Fusarium merismoides, Penicillium restrictum, Phoma putaminum, Trichoderma atroviride, Arthrobacter globiformis, Bacillus subtilis, Cellulomonas flavigena, Pseudomonas synxantha, Streptomyces albus,species S.F (%) S.E.M species S.F (%) S.E.M Achromobacter cholinophagum 27.6%Flavobacterium johnsoniae21.2% Acinetobacter calcoaceticus 31.6%Micrococcus halobius 39.2% Acinetobacter johnsonii 24.8%Micrococcus luteus51.6% Agrobacterium radiobacter var.I28.4%Nocardia rubropertincta50.8% Agrobacterium radiobacter var.II30.4%Pantoea agglomerans49.6% Alcaligenes faecalis 35.6%Pantoea soilia22.4% Arthrobacter citreus 8.8%Proteus vulgaris25.6% Arthrobacter globiformis 85.6%Pseudomonas fluorescens var.Ⅰ7.6% Bacillus cerecus 20.8%Pseudomonas pseudoalaligenes11.2% Bacillus megaterium 25.2%Pseudomonas stutzeri14.8% Bacillus mycoides 18.8%Pseudomonas synxantha83.6% Bacillus subtilis 86.8%Streptomyces albus87.6% Brevibacillus brevis 24.4%Streptomyces caeruleus35.6% Brevibacterium linens 19.2%Streptomyces canescens9.2% Cellulomonas fimi 19.6%Streptomyces chromfuscus10.4% Cellulomonas flavigena 87.2%Streptomyces rseoviolaceus48.4% Corynebacterium mucifaciens 18.8%Xanthobacter flavus14.4% Erwinia amylovora 22.4%species S.F (%) S.E.M species S.F (%) S.E.M Acremonium strictum 20.4%Penicillium purpurogenum 23.6% Alternaria alternato27.2%Penicillium restrictum94.0% Aspergillus carnus20.0%Penicillium spinulosum 27.2% Aspergillus flavus18.8%Penicillium waksmanii 26.8% Aspergillus fumigatus27.2%Penicillum nigricans21.2% Aspergillus melleus21.2%Phoma nebulosa49.2% Aspergillus niger84.0%Phoma putaminum84.4% Aspergillus ochraceus 18.8%Rhizopus stolonifer79.2% Aspergillus penicilloides 20.8%Scopulariopsis canadensis 7.6% Aspergillus puniceus16.4%Trichoderma atroviride 83.6% Aspergillus sydowil18.0%Trichoderma harziamum 21.6% Aspergillus terreus31.2%Aspergillus terricola23.2%Aspergillus ustus15.2%Aspergillus versicolor14.8%Beauverja brongniartii 26.0%Cladosporium berbarum 18.8%Cladosporium carpophilum 24.4%Cladosporium tenissimum 20.0%Curvularia lunata25.2%Epicoccum purpurascens 23.2%Exophiala jeanselmer 19.6%Fusarium merismoides 43.2%Fusarium moniliforme26.8%Fusarium oxysporum19.6%Geotrichum candidurn 28.8%Humicola fuscoatra24.4%Humicola grisea27.2%Kabatia microsticta22.4%Mucor hiemalis78.4%Mucor spinescens39.6%Neurospora crassa42.4%Paecilomyces inflatus44.4%Paecilomyces lilacinus 38.8%Paecilomyces marquandii 34.4%Paecilomyces varioti31.2%Papulaspora equi27.6%Penicillim rubrum44.8% Penicillim thomii41.2% Penicillium citreonjgrum 23.2% Penicillium citrinum36.8% Penicillium corylophilum 40.8% Penicillium decumbens 34.8% Penicillium implicatum39.2% Penicillium janthinellum 23.6% Penicillium lslandicum 23.2%。