Synthesis_of_zinc_oxide_nanotetrapods_by_a_novel_fast_microemulsion-based_hydrothermal_method
MaterialsLetters
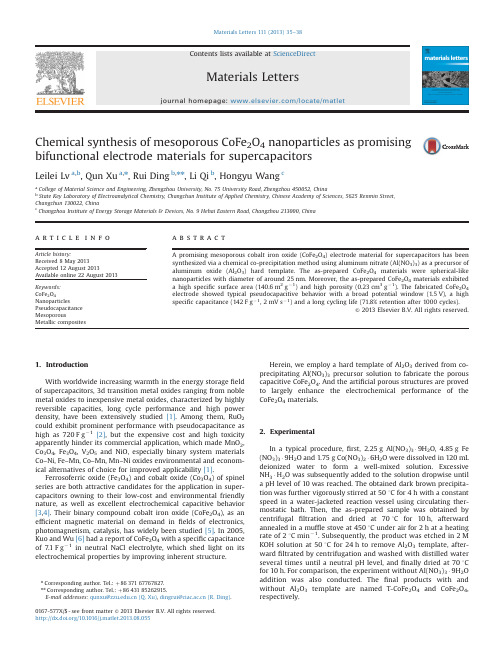
Chemical synthesis of mesoporous CoFe2O4nanoparticles as promising bifunctional electrode materials for supercapacitorsLeilei Lv a,b,Qun Xu a,n,Rui Ding b,nn,Li Qi b,Hongyu Wang ca College of Material Science and Engineering,Zhengzhou University,No.75University Road,Zhengzhou450052,Chinab State Key Laboratory of Electroanalytical Chemistry,Changchun Institute of Applied Chemistry,Chinese Academy of Sciences,5625Renmin Street,Changchun130022,Chinac Changzhou Institute of Energy Storage Materials&Devices,No.9Hehai Eastern Road,Changzhou213000,Chinaa r t i c l e i n f oArticle history:Received8May2013Accepted12August2013Available online22August2013Keywords:CoFe2O4NanoparticlesPseudocapacitanceMesoporousMetallic compositesa b s t r a c tA promising mesoporous cobalt iron oxide(CoFe2O4)electrode material for supercapacitors has beensynthesized via a chemical co-precipitation method using aluminum nitrate(Al(NO3)3)as a precursor ofaluminum oxide(Al2O3)hard template.The as-prepared CoFe2O4materials were spherical-likenanoparticles with diameter of around25nm.Moreover,the as-prepared CoFe2O4materials exhibiteda high specific surface area(140.6m2gÀ1)and high porosity(0.23cm3gÀ1).The fabricated CoFe2O4electrode showed typical pseudocapacitive behavior with a broad potential window(1.5V),a highspecific capacitance(142F gÀ1,2mV sÀ1)and a long cycling life(71.8%retention after1000cycles).&2013Elsevier B.V.All rights reserved.1.IntroductionWith worldwide increasing warmth in the energy storagefieldof supercapacitors,3d transition metal oxides ranging from noblemetal oxides to inexpensive metal oxides,characterized by highlyreversible capacities,long cycle performance and high powerdensity,have been extensively studied[1].Among them,RuO2could exhibit prominent performance with pseudocapacitance ashigh as720F gÀ1[2],but the expensive cost and high toxicityapparently hinder its commercial application,which made MnO2,Co3O4,Fe3O4,V2O5and NiO,especially binary system materialsCo–Ni,Fe–Mn,Co–Mn,Mn–Ni oxides environmental and econom-ical alternatives of choice for improved applicability[1].Ferrosoferric oxide(Fe3O4)and cobalt oxide(Co3O4)of spinelseries are both attractive candidates for the application in super-capacitors owning to their low-cost and environmental friendlynature,as well as excellent electrochemical capacitive behavior[3,4].Their binary compound cobalt iron oxide(CoFe2O4),as anefficient magnetic material on demand infields of electronics,photomagnetism,catalysis,has widely been studied[5].In2005,Kuo and Wu[6]had a report of CoFe2O4with a specific capacitanceof7.1F gÀ1in neutral NaCl electrolyte,which shed light on itselectrochemical properties by improving inherent structure.Herein,we employ a hard template of Al2O3derived from co-precipitating Al(NO3)3precursor solution to fabricate the porouscapacitive CoFe2O4.And the artificial porous structures are provedto largely enhance the electrochemical performance of theCoFe2O4materials.2.ExperimentalIn a typical procedure,first,2.25g Al(NO3)3Á9H2O,4.85g Fe(NO3)3Á9H2O and1.75g Co(NO3)2Á6H2O were dissolved in120mLdeionized water to form a well-mixed solution.ExcessiveNH3ÁH2O was subsequently added to the solution dropwise untila pH level of10was reached.The obtained dark brown precipita-tion was further vigorously stirred at501C for4h with a constantspeed in a water-jacketed reaction vessel using circulating ther-mostatic bath.Then,the as-prepared sample was obtained bycentrifugalfiltration and dried at701C for10h,afterwardannealed in a muffle stove at4501C under air for2h at a heatingrate of21C minÀ1.Subsequently,the product was etched in2MKOH solution at501C for24h to remove Al2O3template,after-wardfiltrated by centrifugation and washed with distilled waterseveral times until a neutral pH level,andfinally dried at701Cfor10h.For comparison,the experiment without Al(NO3)3Á9H2Oaddition was also conducted.Thefinal products with andwithout Al2O3template are named T-CoFe2O4and CoFe2O4,respectively.Contents lists available at ScienceDirectjournal homepage:/locate/matletMaterials Letters0167-577X/$-see front matter&2013Elsevier B.V.All rights reserved./10.1016/j.matlet.2013.08.055n Corresponding author.Tel.:þ8637167767827.nn Corresponding author.Tel.:þ8643185262915.E-mail addresses:*************.cn(Q.Xu),***************.cn(R.Ding).Materials Letters111(2013)35–38X-ray diffraction (XRD)patterns of the samples were recorded on a Rigaku D/max-2500diffractometer equipped with monochromated Cu K α(λ¼0.15406nm)radiation.Scanning electron microscopy (SEM)images were taken using Philips XL 30and a JEOL JSM-6700F microscope.N 2adsorption –desorption measurements were performed on a Micromeritics ASAP 2020apparatus.Electrochemical examinations were carried out with a CoFe 2O 4working electrode,a Pt mesh counter electrode and a Hg/HgO (2M KOH aqueous solution)reference electrode.The working electrodes were prepared by pressing the homogenous mixture of 70wt%CoFe 2O 4active materials,15wt%acetylene black,and 15wt%poly(tetra fluoroethy-lene)(Sigma Aldrich)onto a stainless steel mesh collector.Cyclic voltammetry (CV)and cyclic stability were collected on CHI700D electrochemical workstation and land CT2001A tester,respectively.The gravimetric speci fic capacitance (C m )is calculated accord-ing to the following equation:C m ¼12vm ðΔV ÞZ V bV a I d V ð1Þwhere m ,νand (V a –V b )i.e.ΔV denote the mass of CoFe 2O 4or T-CoFe 2O 4active powders,scan rate and the potential window (1.5V),respectively.3.Results and discussionFig.1a displays the XRD patterns of T-CoFe 2O 4and CoFe 2O 4materials.All the resultant peaks can be indexed as a face-centered-cubic spinel phase.The identi fied eight diffraction peaks at 2θvalue of 30.211,35.701,37.131,43.161,54.131,57.361,62.961and 74.321correspond to the (220),(311),(222),(400),(422),(511),(440)and (533)crystal planes,respectively,which is in well agreement with the standard patterns for CoFe 2O 4(JPCDS No.22-1086).No signals of Al 2O 3phase (JCPDS No.10-0425)aredetected in the patterns [7].Moreover,neither does Al emerge in XPS nor in EDAX spectra of T-CoFe 2O 4(seen in Fig.S1and S2).All suggested the successful removal of the template.Furthermore,it is clearly seen that diffraction peaks of T-CoFe 2O 4are duller indicating T-CoFe 2O 4comprises of smaller nanoparticles than CoFe 2O 4and this inference is further con firmed by their SEM images (Fig.1b and c).The CoFe 2O 4in Fig.1b,shows basically microsized agglomerate particles with few pores and voids,and a smooth surface,whereas the T-CoFe 2O 4exhibits basically dis-persed and uniform spherical-like particles of around 25nm size with rough surface and high porosity,as can be seen in the domain of Fig.1c.Thus by etching the hard template,T-CoFe 2O 4exhibits a porous framework with smaller granular size rather than bulk.To accommodate super ficial electroactive species as much as possible,it is essential for the electrode material to enrich its inner surface area and pores so as to ease the mass transfer of electrolytes [8].The surface area and porosity of the T-CoFe 2O 4and CoFe 2O 4were further veri fied by nitrogen sorption measurements which are shown in Fig.1d.The N 2adsorption –desorption isotherms of CoFe 2O 4and T-CoFe 2O 4representative of type II and IV curves with distinct hyster-esis loops.The BET surface area of T-CoFe 2O 4is 140.6m 2g À1which is far larger than the value of 27.6m 2g À1for CoFe 2O 4.The main pore size distributes narrowly in the range of 4–8nm centered at 5.6nm and 2–4nm centered at 2.2nm for the T-CoFe 2O 4and CoFe 2O 4,respectively,(shown in the insets of in Fig.1d).Besides,average pore size and mesoporous volume of T-CoFe 2O 4are quantitatively eval-uated as 6.53nm and 0.23cm 3g À1,and the corresponding values of CoFe 2O 4are 2.2nm and 0.225cm 3g À1,respectively.High speci fic surface area and porosity is critical to enhance the electrochemical performances of electrode materials for supercapacitors [1].Thereby,improved electrochemical properties for the porous T-CoFe 2O 4,such as speci fic capacitance,high-rate capability,and longer cycling life,can be expectedaccordingly.Fig.1.XRD patterns (a)and N 2adsorption –desorption isotherms with insets of BJH pore size distribution (d)of CoFe 2O 4and T-CoFe 2O 4;SEM images of CoFe 2O 4(b)and T-CoFe 2O 4(c).L.Lv et al./Materials Letters 111(2013)35–3836The CV plots of CoFe 2O 4and T-CoFe 2O 4electrodes tested in the potential range of À1.0–0.5V were plotted in Fig.2a and b.Two obvious pseudocapacitive blocks are observed in the positive (À0.1–0.5V)and negative (À0.5–À1.0V)potential regions which contribute to most of the capacitance.While the middle intervals (À0.1–À0.5V)contribute to the minor EDL capacitance [9].Here,the observed redox couples well elucidate the pseudocapacitive properties of CoFe 2O 4electrodes.The three couples of redox peaks located at around 0.28/0.05V,0.5/0.38V,À0.75/À0.85V indicate the reversible redox processes of Co 3þ/Co 2þ,Co 4þ/Co 3þ,Fe 3þ/Fe 2þredox couples in alkaline electrolytes [4,9,10],which can be expressed as the following equations:1/3Co 3O 4þ1/3OH Àþ1/3H 2O 2CoOOH þ1/3e À(2)CoOOH þOH À2CoO 2þH 2O þe À(3)2/3Fe 3O 4þ2/3OH ÀþH 2O 22FeOOH þ2/3e À(4)Even though a series of reports have studied the capacitive properties of M Fe 2O 4(M ¼Mn,Fe,Co,Ni),CoFe 2O 4in our work may draw fresh attention as it can both act as anode and cathode electrode materials by virtue of the broad potential window area.The speci fic capacitance values of CoFe 2O 4and T-CoFe 2O 4electrodes are shown in Fig.2c,both speci fic capacitance decreases with increasing scan rate because of insuf ficient active material involved in the redox reactions under higher scan rate.The CoFe 2O 4electrode exhibits a low speci fic capacitance range of 44–13F g À1while the T-CoFe 2O 4electrode exhibits a much higher and considerable speci fic capacitance range of 142–23F g À1in the scan rate range of 2–50mV s À1.Obviously,the porous T-CoFe 2O 4has much higher electrochemical activity than the bulk CoFe 2O 4thanks to suf ficient electroactive sites for electrochemical reac-tions and easy ion diffusion pathways for electrolyte ions transfer process.Long cycle life is a crucial parameter for electrode materials used for supercapacitors.The cyclic performances of CoFe 2O 4and T-CoFe 2O 4electrodes are shown in Fig.2d.The CoFe 2O 4electrodeshowed a capacitance ′s decay in the first 200cycles,then gradually went up with the increasing cycle numbers and finally a retention rate of 68.7%was obtained.The speci fic capacitance of T-CoFe 2O 4,which is nearly three times of CoFe 2O 4,slightly decreased before 200cycles and remained almost stable in the subsequent cycles.A retention rate of 71.8%was obtained after 1000cycles for the T-CoFe 2O 4electrode.Here,the decline in the speci fic capacitance with cycle number may be ascribable to the loss of active material caused by the dissolution and/or detach-ment during early cycle number [11].Further work on searching the most appropriate ratio of template to improve the cycling behavior is currently under progress.4.ConclusionsIn summary,the mesoporous CoFe 2O 4materials for super-capacitors have been successfully synthesized with assistance of co-precipitating Al 2O 3template.High BET speci fic surface and porosity of 140.6m 2g À1and 0.23cm 3g À1were obtained which facilitate the Faradaic pseudocapacitive performance by virtue of suf ficient electroactive sites and easy ions pathways.The unique mesoporous CoFe 2O 4electrode delivered a wide potential window of 1.5V and a high speci fic capacitance of 142F g À1at 2mV s À1,which can be expected to take important roles in both anode and cathode materials for supercapacitors.AcknowledgmentsWe gratefully acknowledge the financial support of this research by National Basic Research Program of China (2012CB932800),Scienti fic Research Foundation for the Returned Overseas Chinese Scholars and State Education Ministry (SRF for ROCS,SEM).Fig.2.CV plots of CoFe 2O 4electrode (a)and T-CoFe 2O 4electrode (b);speci fic capacitance values under different scan rates (c)and cycling performances (d)of CoFe 2O 4and T-CoFe 2O 4electrodes.L.Lv et al./Materials Letters 111(2013)35–3837Appendix A.Supporting informationSupplementary data associated with this article can be found in the online version at /10.1016/j.matlet.2013.08.055. References[1]Wang GP,Zhang L,Zhang JJ.Chemical Society Reviews2012;41:797–828.[2]Zheng JP,Cygan PJ,Jow TR.Journal of the Electrochemical Society1995;142:2699–703.[3]Xu JA,Gao L,Cao JY,Wang WC,Chen ZD.Electrochimica Acta2010;56:732–6.[4]Du X,Wang CY,Chen MM,Jiao Y,Wang J.Journal of Physical Chemistry C2009;113:2643–6.[5]Li XH,Xu CL,Han XH,Qiao L,Wang T,Li FS.Nanoscale Research Letters2010;5:1039–44.[6]Kuo SL,Wu NL.Electrochemical and Solid State Letters2005;8:A495–9.[7]Shang XF,Wang XG,Nie WX,Guo XF,Zou XJ,Ding WZ,et al.Materials Letters2012;83:91–3.[8]Wei TY,Chen CH,Chien HC,Lu SY,Hu CC.Advanced Materials2010;22:347–51.[9]Li YH,Huang KL,Yao ZF,Liu SQ,Qing XX.Electrochimica Acta2011;56:2140–4.[10]Wu JB,Lin Y,Xia XH,Xu JY,Shi QY.Electrochimica Acta2011;56:7163–70.[11]Lokhande CD,Gujar TP,Shinde VR,Mane RS,Han SH.ElectrochemistryCommunications2007;9:1805–9.L.Lv et al./Materials Letters111(2013)35–38 38。
无机材料的乙醇溶剂热合成及应用
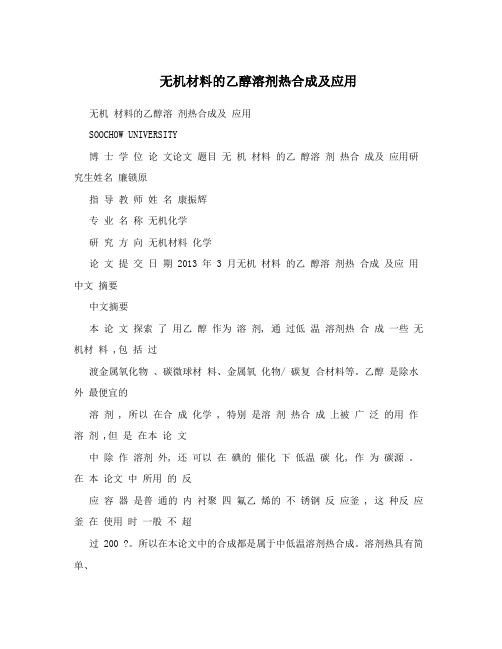
无机材料的乙醇溶剂热合成及应用无机材料的乙醇溶剂热合成及应用SOOCHOW UNIVERSITY博士学位论文论文题目无机材料的乙醇溶剂热合成及应用研究生姓名廉锁原指导教师姓名康振辉专业名称无机化学研究方向无机材料化学论文提交日期 2013 年 3 月无机材料的乙醇溶剂热合成及应用中文摘要中文摘要本论文探索了用乙醇作为溶剂, 通过低温溶剂热合成一些无机材料 ,包括过渡金属氧化物、碳微球材料、金属氧化物/ 碳复合材料等。
乙醇是除水外最便宜的溶剂 , 所以在合成化学 , 特别是溶剂热合成上被广泛的用作溶剂 ,但是在本论文中除作溶剂外, 还可以在碘的催化下低温碳化, 作为碳源。
在本论文中所用的反应容器是普通的内衬聚四氟乙烯的不锈钢反应釜 , 这种反应釜在使用时一般不超过 200 ?。
所以在本论文中的合成都是属于中低温溶剂热合成。
溶剂热具有简单、反应物与温度都易调控的特点。
合成的无机材料主要有以下表征手段 : 扫描电子显微镜 (SEM ) 、能谱 (EDS ) 、透射电子显微镜 (TEM)、X 射线粉末衍射(XRD)、氮气吸附脱附 (BET ) 、红外 (IR ) 、紫外-可见 (UV-Vis ) 、拉曼(Raman)、X 射线光电子能谱 (XPS ) 及电化学工作站等。
最后根据所合成的无机材料的各自性质的不同分别对其应用做了初步研究。
具体工作包括以下几部分:1、以乙醇为溶剂, 以 FeCl ? 6H O 为溶质, 高温下令其醇解可以得到形态不同3 2氧化铁材料, 包括核-壳有分层结构的α-Fe O 。
具体研究了时间、浓度、2 3温度等条件对所合成的α-Fe O 材料形态和结构的影响。
通过对反应时间2 3的研究给出了可能的形成机理。
以核-壳结构的α-Fe O 为催化剂,研究2 3了苯甲醇氧化制备苯甲醛的催化反应, 研究发现以此氧化铁为催化剂同时 3+ 可以提高反应的选择性和转化率。
若以 Fe 为催化剂时转化率高, 但是选择性很低,主要生成苯甲酸,若以商业α-Fe O 或 Fe O 为催化剂,则转 2 3 3 4 化率很低。
微反应器水热法耦合制备纳米片状氧化铝
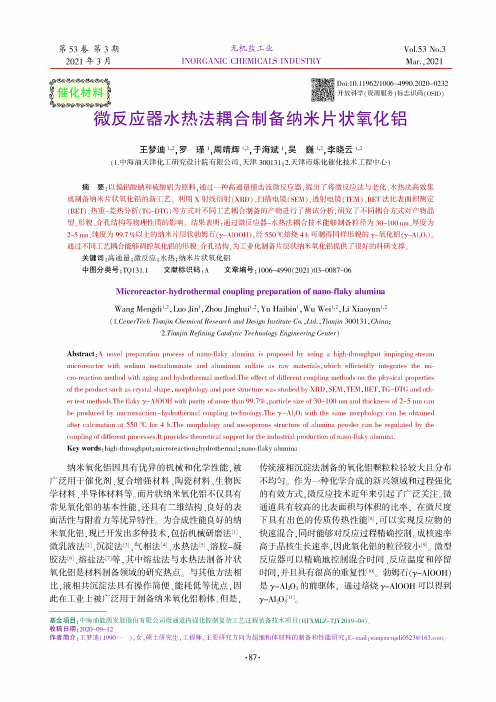
第53卷第3期2021年3月Vol.53No.3Mar.,2021无机盐工业INORGANIC CHEMICALS INDUSTRY催化材料Doi:10.11962/1006-4990.2020-0232开放科学(资源服务)标志识码(OSID)微反应器水热法耦合制备纳米片状氧化铝王梦迪“,罗瑾周靖辉",于海斌吴巍",李晓云"(1.中海油天津化工研究设计院有限公司,天津300131;2.天津市炼化催化技术工程中心)摘要:以偏铝酸钠和硫酸铝为原料,通过一种高通量撞击流微反应器,提岀了将微反应法与老化、水热法高效集成制备纳米片状氧化铝的新工艺。
利用X射线衍射(XRD)、扫描电镜(SEM)、透射电镜(TEM)、BET法比表面积测定(BET)、热重-差热分析(TG-DTG)等方式对不同工艺耦合制备的产物进行了测试分析,研究了不同耦合方式对产物晶型、形貌、介孔结构等物理性质的影响。
结果表明:通过微反应器-水热法耦合技术能够制备粒径为30~100nm、厚度为2~5nm、纯度为99.7%以上的纳米片层状勃姆石(酌-AlOOH),经550益焙烧4h可制得同样形貌的酌-氧化铝(酌-Al J O i)。
通过不同工艺耦合能够调控氧化铝的形貌、介孔结构,为工业化制备片层状纳米氧化铝提供了很好的科研支撑。
关键词:高通量;微反应;水热;纳米片状氧化铝中图分类号:TQ131.1文献标识码:A文章编号:1006-4990(2021)03-0087-06Microreactor-hydrothermal coupling preparation of nano-flaky aluminaWang Mengdi1,2,Luo Jin1袁Zhou Jinghui1,2,Yu Haibin1,Wu Wei1,2,Li Xiaoyun1,2(1.CenerTech Tianjin Chemical Research and Design Institute Co.,Ltd.,Tianjin300131,China;2.Tianjin Refining Catalytic Technology Engineering Center)Abstract: A novel preparation process of nano-flaky alumina is proposed by using a high-throughput impinging stream microreactor with sodium metaaluminate and aluminum sulfate as raw materials,which efficiently integrates the micro-reaction method with aging and hydrothermal method.The effect of different coupling methods on the physical properties of the product such as crystal shape,morphology and pore structure was studied by XRD,SEM,TEM,BET,TG-DTG and other test methods.The flaky酌-AlOOH with purity of more than99.7%,particle size of30~100nm and thickness of2~5nm can be produced by microreaction-hydrothermal coupling technology.The酌-Al2O3with the same morphology can be obtained after calcination at550益for4h.The morphology and mesoporous structure of alumina powder can be regulated by the coupling of different processes.It provides theoretical support for the industrial production of nano-flaky alumina.Key words:high-throughput;microreaction;hydrothermal;nano-flaky alumina纳米氧化铝因具有优异的机械和化学性能,被广泛用于催化剂、复合增强材料、陶瓷材料、生物医学材料、半导体材料等。
核酸适配体

a b s t r a c t
Herein, we have successfully built up connections between nanoparticles and nanoclusters, and further constructed a surface-enhanced fluorescence (SEF) strategy based on the two types of nanomaterials for selectively assaying carcinoembryonic antigen (CEA). Specifically, silver nanoclusters provided the original fluorescence signal, while gold nanoparticles modified with DNA served as the fluorescence enhancer simultaneously. On the basis of this proposed nano-system, the two nanomaterials were linked by CEA–aptamer, thus facilitating SEF occurring. Nevertheless, more competitive interactions between CEA and CEA–aptamer emerged once CEA added, leading to SEF failed and their fluorescence decreased. Significantly, this creative method was further applied to detect CEA, and showed the linear relationship between the fluorescence intensity and CEA concentrations in the range of 0.01–1 ng mL À 1 with a detection limit of 3 pg mL À 1 at a signal-to-noise ratio of 3, demonstrating its sensitivity and promising towards multiple applications. On the whole, this approach we established may broaden potential ways of combining nanoparticles and nanoclusters for detecting trace targets in bioanalytical fields. & 2014 Elsevier B.V. All rights reserved.
J Polym Sci, Part A Polym. Chem. 2013,51,1099-1106.

Synthesis of PMHDO-g-PDEAEA Well-Defined Amphiphilic Graft Copolymer via Successive Living Coordination Polymerizationand SET-LRPGuolin Lu,1Yongjun Li,1Hongsheng Gao,2,3Hao Guo,2Xingliang Zheng,3Xiaoyu Huang1 1Key Laboratory of Organofluorine Chemistry and Laboratory of Polymer Materials,Shanghai Institute of Organic Chemistry, Chinese Academy of Sciences,345Lingling Road,Shanghai200032,People’s Republic of China2Department of Chemistry,Fudan University,220Handan Road,Shanghai200433,People’s Republic of China3College of Chemistry and Biological Engineering,Changsha University of Science and Technology,Changsha410114, People’s Republic of ChinaCorrespondence to:H.Guo(E-mail:hao_guo@)or X.Huang(E-mail:xyhuang@)Received30October2012;accepted6November2012;published online5December2012DOI:10.1002/pola.26470ABSTRACT:A series of well-defined amphiphilic graft copoly-mer containing hydrophobic polyallene-based backbone and hydrophilic poly(2-(diethylamino)ethyl acrylate)(PDEAEA) side chains was synthesized by sequential living coordination polymerization of6-methyl-1,2-heptadiene-4-ol(MHDO)and single electron transfer-living radical polymerization(SET-LRP)of2-(diethylamino)ethyl acrylate(DEAEA).Ni-catalyzed living coordination polymerization of MHDO was first performed in toluene to give a well-defined double-bond-containing poly(6-methyl-1,2-heptadiene-4-ol)(PMHDO)homo-polymer with a low polydispersity(M w/M n¼ 1.10).Next, 2-chloropropionyl chloride was used for the esterification of pendant hydroxyls in every repeating unit of the homopolymer so that the homopolymer was converted to PMHDO-Cl macroi-nitiator.Finally,SET-LRP of DEAEA was initiated by the macroi-nitiator in tetrahydrofuran/H2O using CuCl/tris(2-(dimethy-lamino)ethyl)amine as catalytic system to afford well-defined PMHDO-g-PDEAEA graft copolymers(M w/M n 1.22)through the grafting-from strategy.The critical micelle concentration (cmc)was determined byfluorescence spectroscopy with N-phenyl-1-naphthylamine as probe and the micellar morphol-ogy was visualized by transmission electron microscopy. V C2012Wiley Periodicals,Inc.J.Polym.Sci.,Part A:Polym. Chem.2013,51,1099–1106KEYWORDS:graft copolymers;grafting-from;polyallene;self-assembly;single electron transfer-living radical polymerization (SET-LRP)INTRODUCTION As well-known,allene derivatives have cumulated double bonds so that they can be regarded as the isomers of propargyl ether derivatives.They have been used for sigmatropic rearrangements as well as for cyclization reactions in the field of organic synthesis.Also,allene deriva-tives are attractive monomers to produce polyallenes,a se-ries of reactive polymers bearing exo-methylene moieties directly attached to polymeric backbone or the internal dou-ble bonds in the main chain,by vinyl polymerization of ei-ther part of the cumulated double bonds.Polyallenes can be used as attractive synthetic precursors for the versatile materials including various functional groups due to the ver-satility of the addition reactions of the double bonds.1 Polyallenes can be obtained by different polymerization approaches of allene derivatives including radical,cationic, coordination,and zwitterionic polymerization,etc.2In compar-ison with radical or cationic polymerization of allene derivatives,controlled synthesis of polyallenes(i.e.,the control of structure,molecular weight and molecular weight distribu-tion,end functionalization,and block copolymerization,etc.) was a great challenge for polymer chemists.To overcome these obstacles,Endo and coworkers developed living coordi-nation polymerization of allene derivatives,which could afford well-defined polyallenes in high yields using[(g3-allyl)NiO-COCF3]2as initiator.3–5They also investigated the effect of ani-onic ligands6or protic solvents7on polymerization behavior and polymer structure.With the advent of this living coordi-nation polymerization,some well-defined polyallene-based polymers8–10and corresponding block copolymers11,12were synthesized.Moreover,block copolymers of allene derivatives with butadiene,isocyano monomers or PEG were also reported.13–15However,well-defined polyallenes-based copoly-mers of vinyl monomers cannot be obtained by this kind of Ni-catalyzed living coordination polymerization because of their different polymerization mechanism.Guolin Lu and Yongjun Li contributed equally to this work. V C2012Wiley Periodicals,Inc.Thus,we have been developing various strategies for the synthesis of well-defined copolymers of allene derivatives and vinyl monomers.Our main purpose is to synthesize polyallene-based graft copolymers with commercial vinyl monomers.As we know,graft copolymers have more compli-cated and confined structures,16,17which may provide more various parameters to control the polymers’properties and further design new nanomaterials.Nevertheless,synthesis of a well-defined graft copolymer with a controlled molecular weight and a narrow molecular weight distribution is much more difficult than that of a linear block copolymer,which has limited its application.With the development of reversi-ble deactivation radical polymerization(RDRP)in the past decades,such as nitroxide-mediated polymerization,18atom transfer radical polymerization(ATRP),19–21single electron transfer-living radical polymerization(SET-LRP),22–26and reversible addition-fragmentation chain transfer polymeriza-tion,27,28well-defined graft copolymers containing diverse segments29–37have been prepared.Generally,three different strategies,including grafting-through,grafting-onto,and grafting-from,are used to synthesize graft copolymers.38 Graft copolymers can be obtained via the polymerization of macromonomers via the grafting-through method;but,the resulting graft copolymers via conventional radical polymer-ization of macromonomers possessed a broad chain-length distribution39and living polymerization of macromonomers yielded well-defined graft copolymers with low molecular weights.40The grafting-onto technique is to graft as-prepared side chains onto the backbone by a coupling reaction,normally with insufficient grafting efficiency.41The grafting-from approach used the pendant initiating groups on the backbone to initiate the polymerization of another monomer to form the side chains.42The recent boom of RDRP techniques have rendered the grafting-from strategy more prevailing.29,31–33,43,44In our early work,we used the graft-from strategy to synthe-size hydrophobic well-defined graft copolymers bearing polyallene backbone through sequential living coordination polymerization of allene derivative and ATRP of vinyl mono-mer.45–47After these successful synthesis of polyallene-based hydrophobic graft copolymers,we were then focused on the synthesis and self-assembly behavior of polyallene-based amphiphilic graft copolymers because that self-assembly behavior of amphiphilic copolymers could led to potential applications in many fields including solubilizer,48drug deliv-ery,49,50catalysis,51and microelectronics.52In2009,we synthesized a polyallene-based amphiphilic graft copolymer consisting of hydrophilic PEG side chains via the grafting-onto strategy.53However,the grafting density was not high (<40%)and most hydrophilic monomers are not suitable for this strategy.Herein,we used the grafting-from strategy to synthesize poly(6-methyl-1,2-heptadiene-4-ol)-g-poly(2-(diethylamino)ethyl acrylate)(PMHDO-g-PDEAEA)amphiphilic graft copolymers with well-defined structures and a high grafting density (>80%)by sequential Ni-catalyzed living coordination polymerization of6-methyl-1,2-heptadiene-4-ol(MHDO)and SET-LRP of2-(diethylamino)ethyl acrylate(DEAEA)as shown in Scheme1.Fluorescence spectroscopy and transmission elec-tron microscopy(TEM)were used to study the micellization behavior of these amphiphilic copolymers in aqueous media. EXPERIMENTALMaterialsDEAEA(Aldrich,97%)was passed through a basic alumina column and distilled from CaH2under reduced pressure before use.Copper(I)chloride(CuCl,Aldrich,99%)was puri-fied by stirring overnight over CH3CO2H at room tempera-ture followed by washing the solid with ethanol,diethyl ether,and acetone before drying in vacuo at40 C for1day. Allyltrifluoroacetate(Aldrich,98%)was redistilled before use.Triphenyl phosphorate(PPh3,Acros,99%)was recrys-tallized with CH2Cl2/hexane.Triethylamine(TEA,Aldrich, 99.5%)was dried over KOH and distilled from CaH2under N2before use.Tetrahydrofuran(THF,Aldrich,99%)and tolu-ene(Aldrich,99%)were dried over CaH2and distilled from sodium and benzophenone under N2before use.N-Phenyl-1-naphthylamine(PNA,Alfa Aesar,97%)was purified by recrystallization in ethanol for three times.2-Chloropro-pionyl chloride(2-CPC,Acros,95%),bis(1,5-cyclooctadiene)-nickel(0)(Ni(COD)2,Aldrich),4-(dimethylamino)pyridine (DMAP,Aldrich,99%),and tris(aminoethyl)amine(TREN, Aldrich,96%)were used as received.MHDO was synthesized according to previous literature.54Tris(2-(dimethylamino) ethyl)amine(Me6TREN)was prepared from TREN according to previous literature.55MeasurementsFTIR spectra were recorded on a Nicolet AVATAR-360FTIR spectrophotometer with a resolution of4cmÀ1.All1H(300 MHz)and13C(75MHz)NMR analyses were performed on a Varian Mercury300spectrometer in CDCl3,TMS(1H NMR), and CDCl3(13C NMR)were used as internal standards.Chlo-rine content was determined by the titration with Hg(NO3)2. Relative molecular weights and molecular weight distribu-tions were measured by conventional gel permeation chromatography(GPC)system equipped with a Waters1515 Isocratic HPLC pump,a Waters2414refractiveindex Synthesis of PMHDO-g-PDEAEA amphiphilic graft copolymer.detector,and a set of Waters Styragel columns(HR3(500–30,000),HR4(5000–600,000),and HR5(50,000–4,000,000), 7.8Â300mm,particle size:5l m).GPC measurements were carried out at35 C using THF as eluent with a flow rate of1.0mL/min.The system was calibrated with linear polystyrene standards.Steady-state fluorescent spectra of PNA were measured on a Hitachi F-2700spectrophotometer with the band width of5nm for excitation and emission;the emission intensity at418nm was recorded to determine the critical micelle concentration(cmc)where k ex was340nm. TEM images were obtained by a JEOL JEM-1230instrument operated at80kV.Esterification of PMHDO Homopolymer with2-CPCThe homopolymer of MHDO,PMHDO1(M n¼5700g/mol, M w/M n¼ 1.10),was first obtained by living coordination homopolymerization of MHDO in toluene at50 C using a feeding ratio of[MHDO]:[Ni(COD)2]:[allyltrifluoroaceta-te]:[PPh3]¼50:1:1:1,the procedure was similar to those in our previous reports45–47and the yield was78.9%.To a50-mL sealed three-neck flask,PMHDO1(0.4425g,M n¼5700g/mol,M w/M n¼1.10,3.512mmol A OH groups)and DMAP(0.0470g,3.847mmol)were first introduced for degass-ing and kept under N2.Next,dry THF(45mL)and anhydrous TEA(0.65mL, 4.664mmol)were charged via a gastight syringe.The solution was cooled to0 C and2-CPC(0.50mL, 5.139mmol)was added dropwise.The mixture was stirred at 0 C for1h followed by stirring at room temperature for another20h.The solution was concentrated and precipitated into CH3OH/H2O.After repeated purification of dissolving in THF and precipitating in CH3OH/H2O,0.6418g of PMHDO-Cl2 macroinitiator was obtained after drying in vacuo overnight. GPC:M n¼7500g/mol,M w/M n¼ 1.11.FTIR:m(cmÀ1): 2958,2932,2871,1736,1638,1468,1369,1342,1162.EA: Cl%:14.50%.1H NMR:d(ppm):0.92(6H,CH2CH(C H3)2), 1.26–1.78(3H,C H2C H(CH3)2and3H,CHCl(C H3)CO), 2.09–3.70(2HÂy,¼¼C A C H2and1HÂx,¼¼C A C H),4.34(1HÂx, CO2C H and1H,C H Cl(CH3)CO),4.93(1HÂy,CO2C H),5.44 (2HÂx,¼¼C H2and1HÂy,¼¼C H).13C NMR:d(ppm):22.1, 23.5,24.8,40.3,44.0,52.7,71.7,115.6,127.3,129.1,137.9, 144.6,169.6.SET-LRP Graft Copolymerization of DEAEAIn a typical procedure,CuCl(20.7mg,0.21mmol)and PMHDO-Cl2macroinitiator(M n¼7500g/mol,M w/M n¼1.11.Cl%¼14.50%,0.0549g,0.22mmol initiating groups) were first added to a25-mL Schlenk flask(flame-dried under vacuum before use)sealed with a rubber septum for degassing and kept under N2.Next,freshly distilled THF(8.0 mL),redistilled H2O(1.0mL),and DEAEA(7.9mL,42.6 mmol)were introduced via a gastight syringe.The solution was degassed by three cycles of freezing-pumping-thawing. Finally,Me6TREN(60l L,0.22mmol)was charged via a gas-tight syringe and the flask was immersed into an oil bath preset at40 C.The polymerization lasted8h and was terminated by immersing the flask into liquid N2.The con-version of DEAEA monomer was calculated from1H NMR spectrum of raw sample in CDCl3by comparing the integra-tion area ratio of3ethylenic protons of remaining DEAEA at 6.43,6.05,and5.80ppm to2methylene protons of remain-ing DEAEA and PDEAEA at4.23ppm.Before GPC measure-ment,raw sample was diluted in THF and passed through a neutral alumina column to remove residual copper complex followed by concentration and precipitation in cold n-hexane. The crude product was purified by repeated dissolution and precipitation followed by drying in vacuo overnight to give 0.6265g of a yellow sticky solid,PMHDO-g-PDEAEA3b.GPC:M n¼21,700g/mol,M w/M n¼1.18.1H NMR:d(ppm): 0.88(6H,CH2CH(C H3)2),1.14(6H,CO2CH2N(CH2C H3)2),1.22–2.27(3H,C H2C H(CH3)2of the backbone,4H,C H(C H3)CO,and 3H,C H2C H of PDEAEA side chains),2.76(2HÂy,¼¼C A C H2, 1HÂx,¼¼C A C H,and6H,CO2C H2N(C H2CH3)2), 4.23(2H, CO2C H2N(CH2CH3)2),5.35(2HÂx,¼¼C H2and1HÂy,¼¼C H). Determination of Critical Micelle ConcentrationPNA was used as fluorescence probe to measure the cmc of PMHDO-g-PDEAEA3graft copolymer in aqueous media.Ace-tone solution of PNA(1mM)was added to a large amount of water until the concentration of PNA reached0.002mM. Next,different amounts of THF solutions of PMHDO-g-PDEAEA3graft copolymer(2,0.2,or0.01mg/mL)were added to water containing PNA([PNA]¼0.002mM).The concentration of PMHDO-g-PDEAEA3graft copolymer ranged from 2.0Â10À5g/L to0.1g/L.All fluorescence spectra were recorded at25 C.TEM ImagesPMHDO-g-PDEAEA3graft copolymers were first dissolved in THF(2mg/mL)followed by adding deionized water slowly (0.4mL/h)to0.4mL of THF solution until the water content reached25%.The solution was sealed with a PTFE plug for equilibration under stirring for another12h.The solution was dialyzed against deionized water with slow stirring for 5days to remove THF and deionized water was changed twice a day.For TEM studies,a drop of micellar solution was deposited on an electron microscopy copper grid coated with carbon film and the water evaporated at room temperature. RESULTS AND DISCUSSIONPreparation of PMHDO-Cl MacroinitiatorThe[(g3-allyl)NiOCOCF3]2-initiated living coordination homo-polymerization of MHDO was performed in toluene at50 C with a similar reported procedure45–47to afforded the poly-allene-based backbone,a well-defined PMHDO1homopoly-mer.The living nature of the homopolymerization of MHDO was demonstrated by the good conformity of the measured molecular weight(M n,GPC¼5700)of PMHDO1homopoly-mer with the theoretical molecular weight(M n,the¼124Â50þ203¼6400,124,and203are the molecular weights of MHDO and initiator,respectively)and the unimodal and symmetrical GPC curve of this homopolymer with a narrow molecular weight distribution of 1.10.The ratio of1,2-polymerized units to2,3-polymerized units in the current homopolymer was found to57:43via1H NMR spectrum, which was similar to our previous results.45–47Furthermore, we can estimate from the data of molecular weight thatevery PMHDO chain possesses 44.3double-bond-containing MHDO repeating units.In the current work,PDEAEA side chains were chosen for the construction of polyallene-based amphiphilic graft copolymers via the grafting-from strategy and DEAEA mono-mer was generally polymerized via RDRP.The pendant hydroxyls of PMHDO 1homopolymer obviously could not initiate RDRP of DEAEA monomer,which indicated the nec-essary conversion of these hydroxyls to RDRP initiating groups.These pendant hydroxyls were then esterified with 2-CPC so that PMHDO 1homopolymer was transformed into Cl-containing PMHDO-Cl 2macroinitiator,not our previously reported Br-containing macroinitiator.45–47This macroinitiator was characterized by 1H NMR,13C NMR,FTIR,and element analysis.The occurrence of esterification reaction was illustrated by the rising of Cl content from 0%before the reaction with 2-CPC to 14.50%after the reaction.The introduction of CHCl(CH 3)CO groups,that is,RDRP ini-tiating groups,was verified by the appearance of a new peak at 1736cm À1attributed to the carbonyls in FTIR spectrum after the reaction while the stretching peak of pendant hydroxyls around 3403cm À1became very weaker.Moreover,the molecular weight after the reaction was found by GPC to be raised to 7500g/mol in comparison with the value before the reaction (5700g/mol)due to the newly grafted CHCl(CH 3)CO groups.The unimodal and symmetrical GPC after the reaction (M w /M n ¼1.11)verified that the carbon-hydrogen backbone was inert during the reaction.Figure 1shows 1H NMR spectrum of PMHDO-Cl 2macroinitiator,which displayed the appearance of all typical proton signals of MHDO repeating including the broad peak around 5.44ppm of the protons of double bond.In particular,the promi-nent raising of the integration area of the peak at 4.34ppm also evidenced the new attachment of C H Cl(CH 3)CO group.In addition,a new signal at 169.6ppm corresponding to the carbon of carbonyl (CHCl(CH 3)C O)appeared in 13C NMR spectrum after the reaction,this indicating the presence ofCHCl(CH 3)CO ing the similar calculation formula in our previous reports,45–47the approximate grafting density of CHCl(CH 3)CO initiating group was evaluated to be 0.82/1from the result of Cl content (14.50%).Thus,it can be con-cluded from the aforementioned results that 36.3(¼44.3Â0.82)CHCl(CH 3)CO RDRP initiating groups were successfully attached onto PMHDO backbone.Synthesis of PMHDO-g-PDEAEA Graft CopolymerThe pendant CHCl(CH 3)CO initiating groups of PMHDO-Cl 2macroinitiator obviously could initiate RDRP of DEAEA monomer to yield the desired PMHDO-g -PDEAEA graft copol-ymer via the grafting-from strategy.As well-known,recently a new RDRP method,named SET-LRP ,was developed by Per-cec and coworkers,which provides a method for the ultra-fast synthesis of ultrahigh molecular weight polymers with narrow molecular weight distribution at ambient and subam-bient temperature.22–25The relative simplicity and versatility by which SET-LRP process can be performed is based on the catalysis by Cu(0)powder or Cu(0)wire in conjunction with a suitable combination of ligand and solvent.SET-LRP has emerged as a powerful tool for the rapid synthesis ofvarious1H NMR spectrum of PMHDO-Cl 2macroinitiator inCDCl 3.TABLE 1Synthesis of PMHDO-g -PDEAEA Amphiphilic Graft Copolymer aSample Time (h)Conv.b (%)M n c(g/mol)M w /M n c cmc d (g/L)3a 4 3.6719,300 1.11 3.45Â10À33b 88.3321,700 1.18 4.32Â10À33c1616.6727,1001.229.49Â10À3aInitiated by PMHDO-Cl 2macroinitiator (M n ¼7,500g/mol,M w /M n ¼1.11,density of grafted SET-LRP initiating group:0.82/1)at 40 C,solvent:THF/H 2O (v:v ¼8:1),[DEAEA]:[Cl group]:[CuCl]:[Me 6TREN]¼200:1:1:1.bObtained by 1H NMR.cMeasured by GPC in THF at 35 C.dDetermined by fluorescence spectroscopy using N -phenyl-1-naphthyl-amine as probe at 25C.1H NMR spectrum of PMHDO-g -PDEAEA 3graftcopolymer in CDCl 3.polymers with excellent control of molecular weight,molecu-lar weight distribution,perfect chain end fidelity,and ultra-high molecular weight.56–76In the current case,RDRP of DEAEA was conducted in THF/H 2O using CuCl/Me 6TREN as catalytic system and a control experiment was run to confirm SET-LRP mechanism of this polymerization.The results of SET-LRP graft copolymeriza-tion of DEAEA are summarized in Table 1and it was found that all graft copolymers’molecular weights were much higher than that of the macroinitiator,which indicated SET-LRP of DEAEA was performed.All graft copolymers showedunimodal and symmetrical GPC curves with narrow molecu-lar weight distributions (M w /M n 1.22),which meant that intermolecular coupling reactions could be neglected 29because a high feeding ratio of DEAEA monomer to the ini-tiating group (200:1)and a low conversion of monomer (<17%)were used to suppress the intermolecular coupling reactions.29,38Figure 2shows 1H NMR spectrum of the prod-uct of SET-LRP of DEAEA.All characteristic signals of the corresponding protons of PMHDO backbone and PDEAEA side chains appeared in this spectrum.The peak at 5.35ppm was attributed to the protons of the double bonds intheA:Kinetic plot for solution SET-LRP of DEAEA initiated by PMHDO-Cl 2in THF/H 2O at 40 C and (B)dependence of mo-lecular weight (M n )and molecular weight distribution (M w /M n )on the conversion of DEAEA for solution SET-LRP of DEAEA initi-ated by PMHDO-Cl 2in THF/H 2O at 40C.A:Fluorescence emission spectra of PNA in aqueous solution of PMHDO-g -PDEAEA 3c graft copolymer ([PNA]¼0.002mM)and (B)dependence of fluorescence intensity ratio I /I 0of PNA fluorescence emission spectra on the concentration of PMHDO-g -PDEAEA 3c graft copolymer.backbone.The signals at 1.14ppm,2.76ppm,and 4.23ppm belonged to 14protons of CH 2CH 2N(CH 2CH 3)2group of the side chains.From the data of conversions of DEAEA listed in Table 1,the semilogarithmic plot of Ln([M]0/[M])versus time is depicted in Figure 3(A).The plot shows a linear dependence of the conversion of DEAEA monomer on the time and the appa-rent polymerization rate is first order with respect to the concentration of DEAEA,which indicated a constant number of propagating species during the polymerization of DEAEA.Furthermore,the linear increasing of molecular weight (M n )with the raising of the conversion of DEAEA and low poly-dispersity throughout the whole polymerization process [Fig.3(B)]proved that SET-LRP graft polymerization of DEAEA proceeded under good control.These phenomena accorded with the characteristics of SET-LRP .22–25From these results,it is clear PMHDO-g -PDEAEA 3well-defined graft copolymers were successfully synthesized by SET-LRP of DEAEA via the grafting-from strategy.Self-Assembly of PMHDO-g-PDEAEA in Aqueous Solution PDEAEA is soluble in acidic solution as a weak cationic poly-electrolyte due to the protonation of tertiary amine group.So PMHDO-g -PDEAEA graft copolymer can aggregate in acidic solution to form micelles with hydrophobic PMHDO backbone as core and hydrophilic PDEAEA side chains as co-rona.The cmc of PMHDO-g -PDEAEA graft copolymer in aqueous media was determined by fluorescence technique using PNA as probe.PNA is a more suitable fluorescent probe than pyrene in terms of reproducibility because it dis-plays higher fluorescence activity in nonpolar environments and it can be very easily quenched by polar solvents such as water.77Fluorescence emission spectra of PNA in aqueous solution of PMHDO-g -PDEAEA 3c graft copolymer with dif-ferent concentrations are shown in Figure 4(A)and the rela-tionship of the fluorescence intensity ratio (I /I 0)of PNA as a function of the concentration of PMHDO-g -PDEAEA 3c graft copolymer was plotted in Figure 4(B).We can find that I /I 0increased sharply when the concentration exceeded a certain value,which affirmed the incorporation of PNA probe into the hydrophobic region of micelles.Thus,cmc of PMHDO-g -PDEAEA 3c graft copolymer with a value of 9.49Â10À3g/L was determined to be the intersection of two straight lines.As summarized in Table 1,cmc values of PMHDO-g -PDEAEA graft copolymers increased with the rising of molecular weights,that is,the length of PDEAEA sidechains.FIGURE 5TEM images of micelles formed by PMHDO-g -PDEAEA 3graft copolymer,initial copolymer content in THF:2mg/mL,water content:25%,(A)3c in pure water,(B)and (C)3a in brine,[NaCl]¼0.05M,(D)3a in brine,[NaCl]¼0.15M.Finally,the micellar morphology of PMHDO-g-PDEAEA graft copolymer was visualized by TEM as shown in Figure5.All graft copolymers formed spherical micelles either in water or in brine.CONCLUSIONSIn summary,well-defined PMHDO-g-PNIPAM amphiphilic graft copolymer were synthesized by the combination of liv-ing coordination polymerization of MHDO and SET-LRP of DEAEA via the grafting-from approach.The construction of the backbone and side chains was both controllable.The graft copolymers aggregated in aqueous media to form spherical micelles.These copolymers may find potential applications in nanotechnologies,such as serving templates to fabricate nanocomposites.ACKNOWLEDGMENTSThe authors thank the financial supports from National Natural Science Foundation of China(20974117and21274023), National Basic Research Program of China(973Program, 2012CB720304),Shanghai Pujiang Program(11PJD004), Shanghai Rising Star Program(11QH1402800),and the Speci-alized Research Fund for the Doctoral Program of Higher Edu-cation of China(20110071120004).REFERENCES AND NOTES1M.P.McGrath,E.D.Sall,S.J.Tremont,Chem.Rev.1995, 95,381–389.2T.Endo,I.Tomita,Prog.Polym.Sci.1997,22,565–600.3I.Tomita,Y.Kondo,K.Takagi,T.Endo,Macromolecules 1994,27,4413–4414.4K.Takagi,I.Tomita,T.Endo,Macromolecules1997,30, 7386–7390.5K.Takagi,I.Tomita,T.Endo,Macromolecules1998,31, 6741–6747.6K.Takagi,I.Tomita,Y.Nakamura,T.Endo,Macromolecules 1998,31,2779–2783.7K.Mochizuki,I.Tomita,Macromolecules2006,39, 7474–7478.8M.Taguchi,I.Tomita,T.Endo,Angew.Chem.Int.Ed.Engl. 2000,39,3667–3669.9J.Wang,I.Tomita,T.Endo,Macromolecules2001,34, 4294–4295.10M.Kyohei,I.Tomita,Macromolecules2006,39,6336–6340. 11I.Tomita,T.Abe,K.Takagi,T.Endo,J.Polym.Sci.Part A: Polym.Chem.1995,33,2487–2492.12K.Takagi,I.Tomita,T.Endo,mun.1998,6, 681–682.13M.Taguchi,I.Tomita,Y.Yoshida,T.Endo,J.Polym.Sci. Part A:Polym.Chem.1999,37,3916–3921.14I.Tomita,M.Taguchi,K.Takagi,T.Endo,J.Polym.Sci.Part A:Polym.Chem.1997,35,431–437.15M.Taguchi,I.Tomita,Y.Yoshida,T.Endo,J.Polym.Sci. Part A:Polym.Chem.2001,39,495–499.16S.Forster,T.Plantenberg,Angew.Chem.Int.Ed.Engl. 2002,41,688–714.17G.Riess,Prog.Polym.Sci.2003,28,1107–1170.18C.Cheng,K.Qi,E.Khoshdel,K.L.Wooley,J.Am.Chem. Soc.2006,128,6808–6809.19J.S.Wang,K.Matyjaszewski,J.Am.Chem.Soc.1995,117, 5614–5615.20V.Percec,B.Barboiu,Macromolecules1995,28,7970–7972. 21M.Kato,M.Kamigaito,M.Sawamoto,T.Higashimura,Mac-romolecules1995,28,1721–1723.22V.Percec,A.V.Popov,E.Ramirez-Castillo,M.Mon-teiro,B. Barboiu,O.Weichold, A. D.Asandei, C.M.Mitchell,J.Am. Chem.Soc.2002,124,4940–4941.23V.Percec,T.Guliashvili,dislaw, A.Wistrand, A. Stjerndahl,M.J.Sienkowska,M.J.Monteiro,S.Sahoo,J.Am. Chem.Soc.2006,128,14156–14165.24N.H.Nguyen,M.E.Levere,J.Kulis,M.J.Monteiro,V.Per-cec,Macromolecules2012,45,4606–4622.25M. E.Levere,N.H.Nguyen,V.Percec,Macromolecules 2012,45,8267–8272.26B.M.Rosen,V.Percec,Chem.Rev.2009,109,5069–5119.27G.Moad, E.Rizzardo,S.H.Thang,Polymer2008,49, 1079–1131.28G.Moad,E.Rizzardo,S.H.Thang,Acc.Chem.Res.2008, 41,1133–1142.29G.L.Cheng,A.Boker,M.F.Zhang,G.Krausch,A.H.E. Muller,Macromolecules2001,34,6883–6888.30H.F.Gao,K.Matyjaszewski,J.Am.Chem.Soc.2007,129, 6633–6639.31L.N.Gu,Z.Shen,S.Zhang,G.L.Lu,X.H.Zhang,X.Y. Huang,Macromolecules2007,40,4486–4493.32C.Feng,Z.Shen,L.N.Gu,S.Zhang,L.T.Li,G.L.Lu,X.Y. Huang,J.Polym.Sci.Part A:Polym.Chem.2008,46,5638–5651. 33Y.Q.Zhang,Z.Shen,D.Yang,C.Feng,J.H.Hu,G.L.Lu, X.Y.Huang,Macromolecules2010,43,117–125.34Y.G.Li,Y.Q.Zhang,D.Yang,Y.J.Li,J.H.Hu,C.Feng,S. J.Zhai,G.L.Lu,X.Y.Huang,Macromolecules2010,43, 262–270.35C.Feng,Y.J.Li, D.Yang,J.H.Hu,X.H.Zhang,X.Y. Huang,Chem.Soc.Rev.2011,40,1282–1295.36S.J.Zhai,J.Shang,D.Yang,S.Y.Wang,J.H.Hu,G.L.Lu, X.Y.Huang,J.Polym.Sci.Part A:Polym.Chem.2012,50, 811–820.37Y.G.Li,M.Du,Y.Q.Zhang,Y.J.Li,L.Sui,G.L.Lu,X.Y. Huang,J.Polym.Sci.Part A:Polym.Chem.2012,50,1890–1899. 38M.Zhang,T.Breiner,H.Mori, A.H. E.Muller,Polymer 2003,44,1449–1458.39P.Dziezok,S.S.Sheiko,K.Fischer,M.Schmidt,M.Moller, Angew Chem.Int.Ed.Engl.1997,36,2812–2815.40Y.H.Zhang,Z.Z.Xu,X.K.Li,Y.M.Chen,J.Polym.Sci. Part A:Polym.Chem.2007,45,3994–4001.41A.Deffieux,M.Schappacher,Macromolecules1999,32, 1797–1802.42K.L.Beers,S.G.Gaynor,K.Matyjaszewski,S.S.Sheiko,M. Moller,Macromolecules1998,31,9413–9415.43K.Matyjaszewski,J.H.Xia,Chem.Rev.2001,101,2921–2990. 44M. F.Wang,S.Zou,G.Guerin,L.Shen,K.Q.Deng,M. Jones,G.C.Walker,G.D.Scholes,M.A.Winnik,Macromole-cules2008,41,6993–7002.45X.H.Zhang,D.Peng,G.L.Lu,L.N.Gu,X.Y.Huang,J. Polym.Sci.Part A:Polym.Chem.2006,44,6888–6893.46X.H.Zhang,Z.Shen,L.T.Li,S.Zhang,G.L.Lu,X.Y. Huang,J.Polym.Sci.Part A:Polym.Chem.2007,45, 5509–5517.。
α-MnO2
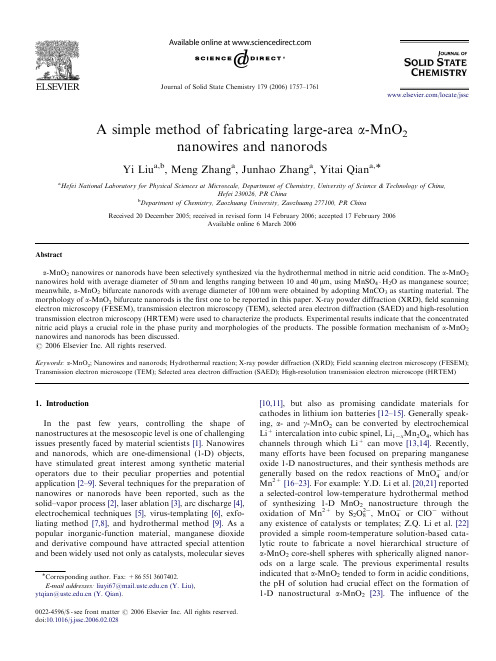
Journal of Solid State Chemistry 179(2006)1757–1761A simple method of fabricating large-area a -MnO 2nanowires and nanorodsYi Liu a,b ,Meng Zhang a ,Junhao Zhang a ,Yitai Qian a,ÃaHefei National Laboratory for Physical Sciences at Microscale,Department of Chemistry,University of Science &Technology of China,Hefei 230026,PR ChinabDepartment of Chemistry,Zaozhuang University,Zaozhuang 277100,PR ChinaReceived 20December 2005;received in revised form 14February 2006;accepted 17February 2006Available online 6March 2006Abstracta -MnO 2nanowires or nanorods have been selectively synthesized via the hydrothermal method in nitric acid condition.The a -MnO 2nanowires hold with average diameter of 50nm and lengths ranging between 10and 40m m,using MnSO 4ÁH 2O as manganese source;meanwhile,a -MnO 2bifurcate nanorods with average diameter of 100nm were obtained by adopting MnCO 3as starting material.The morphology of a -MnO 2bifurcate nanorods is the first one to be reported in this paper.X-ray powder diffraction (XRD),field scanning electron microscopy (FESEM),transmission electron microscopy (TEM),selected area electron diffraction (SAED)and high-resolution transmission electron microscopy (HRTEM)were used to characterize the products.Experimental results indicate that the concentrated nitric acid plays a crucial role in the phase purity and morphologies of the products.The possible formation mechanism of a -MnO 2nanowires and nanorods has been discussed.r 2006Elsevier Inc.All rights reserved.Keywords:a -MnO 2;Nanowires and nanorods;Hydrothermal reaction;X-ray powder diffraction (XRD);Field scanning electron microscopy (FESEM);Transmission electron microscope (TEM);Selected area electron diffraction (SAED);High-resolution transmission electron microscope (HRTEM)1.IntroductionIn the past few years,controlling the shape of nanostructures at the mesoscopic level is one of challenging issues presently faced by material scientists [1].Nanowires and nanorods,which are one-dimensional (1-D)objects,have stimulated great interest among synthetic material operators due to their peculiar properties and potential application [2–9].Several techniques for the preparation of nanowires or nanorods have been reported,such as the solid–vapor process [2],laser ablation [3],arc discharge [4],electrochemical techniques [5],virus-templating [6],exfo-liating method [7,8],and hydrothermal method [9].As a popular inorganic-function material,manganese dioxide and derivative compound have attracted special attention and been widely used not only as catalysts,molecular sieves[10,11],but also as promising candidate materials for cathodes in lithium ion batteries [12–15].Generally speak-ing,a -and g -MnO 2can be converted by electrochemical Li +intercalation into cubic spinel,Li 1Àx Mn 2O 4,which has channels through which Li +can move [13,14].Recently,many efforts have been focused on preparing manganese oxide 1-D nanostructures,and their synthesis methods are generally based on the redox reactions of MnO 4Àand/orMn 2+[16–23].For example:Y.D.Li et al.[20,21]reported a selected-control low-temperature hydrothermal method of synthesizing 1-D MnO 2nanostructure through theoxidation of Mn 2+by S 2O 82À,MnO 4Àor ClOÀwithout any existence of catalysts or templates;Z.Q.Li et al.[22]provided a simple room-temperature solution-based cata-lytic route to fabricate a novel hierarchical structure of a -MnO 2core-shell spheres with spherically aligned nanor-ods on a large scale.The previous experimental results indicated that a -MnO 2tended to form in acidic conditions,the pH of solution had crucial effect on the formation of 1-D nanostructural a -MnO 2[23].The influence of the/locate/jssc0022-4596/$-see front matter r 2006Elsevier Inc.All rights reserved.doi:10.1016/j.jssc.2006.02.028ÃCorresponding author.Fax:+865513607402.E-mail addresses:liuyi67@ (Y.Liu),ytqian@ (Y.Qian).anion on growth of the products had been investigated by Kijima et al.[19],and their results showed that a -MnO 2could be prepared in concentrated H 2SO 4rather than HCl or HNO 3.Thus far,the synthesis of a -MnO 2nanowires or nanorods has seldom been reported under concentrated nitric acidic conditions.Here we report a novel,large-area synthesis method for obtaining nanowires and nanorods with uniform sizes.The a -MnO 2nanowires have average diameter of 50nm and lengths of 10–40m m,using MnSO 4ÁH 2O as manganese source;meanwhile,a -MnO 2bifurcate nanorods with average diameter of 100nm were obtained by adopting MnCO 3as starting material.In our presentation,we choose concentrated nitric acid as acid source to tune the pH of the system.Our experiments show that pure-phase a -MnO 2can be readily obtained in a wide range of nitric acid concentrations.This result may be a useful comple-mentarity to previous experimental results that a -MnO 2could be only produced in H 2SO 4surroundings.2.Experimental procedureAll the reagents of analytical grade were purchased from Shanghai Chemical Reagent Company and used without further purification.In a typical procedure,1mmol MnSO 4ÁH 2O or MnCO 3and 2mmol KClO 3powders were successively put into a beaker with 15mL concen-trated nitric acid,the solution was magnetically stirred for 20min at 801C to form brown colloid.The slurry solution was transferred into a 50mL stainless-steel autoclave with a Teflon-liner,the beaker was washed with 25–30mL distilled water,and washing solution was put into above-mentioned Teflon-liner.The autoclave was sealed and maintained at 1201C for 12h,then air cooled to room temperature.The brown products were filtered off,washed several times with distilled water and absolute ethanol,and then dried in vacuum at 801C for 1h.The X-ray powder diffraction (XRD)pattern of the as-prepared samples was determined using a Philips X’Pert PRO SUPER X-ray diffractometer equipped with graphitemonochromatized Cu K a radiation (l ¼1:541874A)in the 2y ranging from 101to 701.The morphology and size of the final products were determined by field scanning electron microscopy (FESEM)images,taken with JEOL-6700F scanning electronic microanalyzer.Transmission electron microscope (TEM)image and selected area electrondiffraction (SAED)pattern,which were characterized by Hitachi H-800TEM with a tungsten filament and an accelerating voltage of 200kV.High-resolution transmis-sion electron microscope (HRTEM)image was recorded on a JEOL 2010microscope.The samples used for TEM and HRTEM characterization were dispersed in absolute ethanol and were ultrasonicated before observation.3.Results and discussionThe synthesis of a -MnO 2nanowires and nanorods is based on the hydrothermal method in a strong acidic (nitric acid)circumstance.The experimental results by using nitric acid as acidification agent,different manganese sources,and KClO 3as the oxidizer are summarized in Table 1.Under our experimental conditions,the different size and morphological products can be obtained by varying the concentration of nitric acid.From this table we can see that only under concentrated nitric acid condition pure a -MnO 2can be obtained.The volume of concentrated nitric acid can be in the range of 3–20mL.The yields and morphology change greatly when different amounts of nitric acid were introduced.We found that the most optimal conditions of obtaining uniform a -MnO 2nanowires were fixed on 15mL concentrated nitric acid and reaction temperature of 1201C.Moreover,when different Mn compounds were selected as starting materi-als,the size and morphologies can be changed greatly,as shown in the lines 1and 4of Table 1.The result of experiments clearly indicates concentrated nitric acid plays a crucial role in the formation of a -MnO 2with 1-D structure.The phase and purity of the products were firstly examined by XRD.Fig.1shows a typical XRD pattern of the as-synthesized samples at 1201C for 12h,all the reflection peaks can be readily indexed to body-centered tetragonal a -MnO 2phase (space group I 4/m ),with latticeconstants of a ¼9:816A,and c ¼2:853A,which are in agreement with the standard values (JCPDS 72-1982,a ¼9:815A;c ¼2:847A Þ.No other phase was detected in Fig.1indicating the high purity of the final products.The morphologies and structure information were further obtained from FESEM,TEM and SAED.Fig.2provides FESEM images of the as-prepared a -MnO 2single-crystal nanowires.Figs.2(a)and (b)are the low-and high-magnification FESEM images of the as-prepared a -MnO 2Table 1Summary of the results on the products obtained under different manganese sources,the content of concentrated nitric acid and reaction temperature for 12h,using KClO 3as the oxidizer Sample no.Manganese source Concentrated nitric acid (mL)Reaction temperature (1C)Product morphology 1MnSO 4ÁH 2O 15120a -MnO 2nanowires 2MnSO 4ÁH 2O 0120Nonexistence of MnO 23MnSO 4ÁH 2O 0180Minor b -MnO 24MnCO 315120Flowery a -MnO 2nanorods 5MnCO 3120Nonexistence of MnO 2Y.Liu et al./Journal of Solid State Chemistry 179(2006)1757–17611758single-crystal nanowires when MnSO 4ÁH 2O served as manganese source.These images show that the products of a -MnO 2consisted of a large quantity of uniform nanowires,with diameters of 50nm and lengths up to several hundreds of micrometers.Fig.3(a)shows the TEM image of as-prepared a -MnO 2nanowires,and the TEM images further demonstrate that the obtained product has a uniform wire-like morphology.The results reveal the product of a -MnO 2was composed of nanowires.The diameters and lengths of nanowires were consistent with(541)(002)(521)(600)(411)(510)(321)(301)(420)(330)(211)(400)(310)(220)(101)(200)(110)i n t e n s i t y2θ/degreeFig.1.Typical XRD pattern of as-prepared a -MnO 2.Fig.2.Low-magnification FESEM image (a)and high-magnification FESEM image (b)of a -MnO 2nanowires (MnSO 4ÁH 2O as manganesesource).Fig.3.TEM images of as-prepared single-crystal a -MnO 2nanowires (a),TEM image (b),SAED pattern (c)and HRTEM image (d)of the single a -MnO 2nanowire.Y.Liu et al./Journal of Solid State Chemistry 179(2006)1757–17611759those of FESEM results.The TEM image (Fig.3(b))of representative single nanowires and HRTEM observation for individual nanowire provide additional insight into the structure of a -MnO 2with MnSO 4ÁH 2O as manganese source.The typical SAED pattern of the single a -MnO 2nanowire is shown in the inset of Fig.3(c).Fig.3(d)is the HRTEM image taken from the single a -MnO 2nanowire,which shows the clearly resolved lattice fringes.Theseparated spacings of 2.73and 3.12Acorrespond to ð101Þand (310)planar of a -MnO 2,respectively.This image clearly reveals that the as-synthesized nanowire has no defect of dislocation and further substantiates that the nanowires are single crystalline,which is consistent with the SAED pattern.According to HRTEM image and SAED pattern recorded on the single a -MnO 2nanowire,the deduced growth direction of nanowire is ½101 .If MnCO 3was introduced into the reaction system,the products are mainly composed of nanorods,as revealed by the corresponding FESEM images.Figs.4(a)and (b)are the low-and high-magnification FESEM images of the as-prepared a -MnO 2nanorods with MnCO 3as manganese source.The low-magnification FESEM image (Fig.4(a))reveals that the product of a -MnO 2is consisted of a large quantity of flowery nanorods with average diameter of 100nm.Fig.4(b)is the high-magnification FESEM image of the as-prepared a -MnO 2,in which we seem to observe obvious features of bifurcate rod-like structure.It is worth to note that the morphology of a -MnO 2bifurcate nanorods has never been reported paring Figs.4(a)and (b)to Figs.2(a)and (b),it can be found that the nanowires with MnSO 4ÁH 2O as manganese source are much slenderer than the bifurcate nanorods with MnCO 3as manganese source.Generally,pH is believed to have great impact on the crystal forms of final products [17,19,24,25].In our experiment,a series of hydrothermal synthesis were carried out in a wide range of acidity with pH value less than 7,we found that the final products to be a -MnO 2nanowires or nanorods with 1-D morphology whether MnSO 4ÁH 2O or MnCO 3as manganese source.Therefore,this method is very effective for the large-scale synthesis of a -MnO 2with 1-D nanostructures.The influence of the reaction time on the growth of the nanowires and nanorods was investigated.The correspond-ing samples were tested by FESEM.Fig.5shows FESEM images of the as-obtained samples measured (a)after 0.5h,(b)after 3h,(c)after 6h,(d)after 12h,and other conditions kept constant at the same time.Thereinto,Figs.5(a)–(d)are FESEM images of the products with MnSO 4ÁH 2O as manganese source.As can be seen,the reaction lasted for 0.5h;the products were composed of aggregated particles (see Fig.5(a)).When the reaction timeFig.4.Low-magnification FESEM image (a)and high-magnification FESEM image (b)of a -MnO 2nanorods (MnCO 3as manganesesource).Fig.5.The FESEM images of products obtained by heating in the acidic solution for various reaction times,MnSO 4ÁH 2O (a–d)as manganese source:(a)0.5h,(b)3h,(c)6h,(d)12h and MnCO 3(e–h)as manganese source:(e)0.5h,(f)3h,(g)6h,(h)12h.Y.Liu et al./Journal of Solid State Chemistry 179(2006)1757–17611760prolonged to3h,on the surfaces of these particles,lamellar structures appeared,and some of these lamellar split to tiny nanowires,indicating the beginning of the formation of a-MnO2nanowires(see Fig.5(b)).This process continued and more nanowires formed after6h(see Fig.5(c)).Until the reaction time was extended to12h,most of the products are nanowires with average diameter of50nm and lengths ranging between10and40m m,as shown in Fig.5(d).Further elongating the reaction time shows little effects on the size and phase-purity of the products. This growth process is similar to the results of C.Z.Wu et al.[26],we call this a‘‘rolling-broken-growth’’process. According to above results and previous research [20,21,27],the possible formation mechanism of a-MnO2 nanowires by adopting MnSO4ÁH2O as manganese source could be explained as follows:(1)when temperature was maintained at801C,the interaction of KClO3and manganese source with Mn2+ion happened only when concentrated nitric acid exists.In the synthetic process,a large number of the MnO2colloidal particles had been formed in concentrated nitric acid before hydrothermal operation.(2)Under hydrothermal conditions,owing to the absence of surfactants,the MnO2colloidal particles are prone to aggregate and form bigger particles.(3)The surface of aggregated big particles grows gradually into sheets of a-MnO2with lamellar structure through an elevated temperature and pressure,and then these sheets of a-MnO2will curl by extending reaction time to form a-MnO21-D nanostructres.(4)Much evidence has demonstrated that the lamellar structure had a strong tendency to form1-D nanostructures[20,27].The structure of a-MnO2comprises a macromolecular lamellar net with octahedral[MnO6]units coordinated Mn and O atoms [20],which can give rise to formation of1-D nanostruc-tures.As the layer structure of a-MnO2is in a metastable state,these sheets of a-MnO2with lamellar structure split into nanowires.(5)Anisotropic nature of crystal growth makes thefinal products turn into a large number of uniform a-MnO2nanowires.Moreover,we found when MnCO3serves as manganese source,a similar growth procedure was observed,as shown in Figs.5(e)–(h).We believe this a-MnO21-D nanostructural formation process is universal despite different manganese sources were involved in the hydrothermal process.This observation may spread to other nanomaterials synthesis.The above mechanism is in good agreement with our experiment results.4.ConclusionIn summary,a-MnO2nanowires and nanorods with a uniform diameter have been successfully synthesized on a large scale via a simple nitric-acid-assisted hydrothermal process at low temperature.It belongs tofirstly report that the morphology of a-MnO2bifurcate nanorods can be acquired when MnCO3serves as manganese source.The concentrated nitric acid plays a crucial role in the formation of a-MnO2nanowires and nanorods.This experimental result is different from the previous conclu-sion that the concentrated nitric acid seems to be an unfavorable condition to form a-MnO2.This observation may be expanded to synthesize other nanomaterials. AcknowledgmentsFinancial support from the National Natural Science Foundation of China and the973Project of China is greatly appreciated.References[1]A.P.Alivisatos,Science271(1996)933.[2]Y.Wu,P.Yang,Chem.Mater.12(2000)605.[3](a) A.M.Morales,C.M.Lieber,Science279(1998)208;(b)M.S.Gudiken,C.M.Lieber,J.Am.Chem.Soc.122(2000)8801.[4](a)S.Iijima,Nature354(1991)56;(b)T.Seeger,P.Kohler-Redlich,M.Ruhle,Adv.Mater.12(2000)279.[5]Y.Zhou,S.H.Yu,X.P.Cui,C.Y.Wang,Z.Y.Chen,Chem.Mater.11(1999)545.[6]C.Mao,D.J.Solis,B.D.Reiss,S.T.Kottmann,R.Y.Sweeney,A.Hayhurst,G.Georgiou,B.Iverson,A.M.Belcher,Science303(2004) 213.[7]G.H.Du,L.-M.Peng,Q.Chen,S.Zhang,W.Z.Zhou,Appl.Phys.Lett.83(2003)1638.[8]G.H.Du,Q.Chen,Y.Yu,S.Zhang,W.Z.Zhou,L.M.Peng,J.Mater.Chem.14(2004)1437.[9]G.H.Du,Q.Chen,R.C.Che,L.M.Peng,Appl.Phys.Lett.79(2001)3702.[10]M.M.Thackeray,Prog.Solid State Chem.25(1997)1.[11]A.R.Armstrong,P.G.Bruce,Nature381(1996)499.[12]B.Ammundsen,J.Paulsen,Adv.Mater.13(2001)943.[13]Q.Feng,H.Kanoh,K.Ooi,J.Mater.Chem.9(1999)319.[14]L.I.Hill,A.Verbaere,D.Guyomard,J.Power Sources226(2003)119.[15]M.M.Thackeray,J.Am.Ceram.Soc.82(1999)3347.[16]Y.F.Shen,R.P.Zerger,S.L.Suib,L.McCurdy,D.I.Potter,C.L.O’Young,Science260(1993)511.[17]R.N.DeGuzman,Y.F.Shen,h,S.L.Suib,C.L.O’Young,S.Levine,J.M.Newsam,Chem.Mater.6(1994)815.[18]M.Benaissa,M.Jose-Yacaman,T.D.Xiao,P.R.Strutt,Appl.Phys.Lett.70(1997)2120.[19]N.Kijima,H.Yasuda,T.Sato,Y.Yoshimura,J.Solid State Chem.159(2001)94.[20]Y.D.Li,X.L.Li,R.R.He,J.Zhu,Z.X.Deng,J.Am.Chem.Soc.124(2002)1411.[21]X.Wang,Y.D.Li,Chem.Eur.J.9(2003)300.[22](a)Z.Q.Li,Y.Ding,Y.J.Xiong,Q.Yang,Y.Xie,mun.(2005)918;(b)Z.Q.Li,Y.Ding,Y.J.Xiong,Y.Xie,Cryst.Growth Des.5(2005)1953.[23](a)Y.Q.Gao,Z.H.Wang,J.X.Wan,G.F.Zou,Y.T.Qian,J.Cryst.Growth279(2005)415;(b)Y.Chen,C.Liu,F.Liu,H.M.Cheng,J.Alloy Compd.19(2005)282.[24]J.Luo,S.L.Suib,J.Phys.Chem.B101(1997)10403.[25]T.D.Xiao,P.R.Strutt,M.Benaissa,H.Chen, B.H.Kear,Nanostruct.Mater.10(1998)1051.[26]C.Z.Wu,Y.Xie,D.Wang,J.Yang,T.W.Li,J.Phys.Chem.B107(2003)13583.[27]Y.D.Li,X.L.Li,Z.X.Deng,B.C.Zhou,S.S.Fan,J.W.Wang,X.M.Sun,Angew Chem.Int.Ed.Engl.41(2002)333.Y.Liu et al./Journal of Solid State Chemistry179(2006)1757–17611761。
- 1、下载文档前请自行甄别文档内容的完整性,平台不提供额外的编辑、内容补充、找答案等附加服务。
- 2、"仅部分预览"的文档,不可在线预览部分如存在完整性等问题,可反馈申请退款(可完整预览的文档不适用该条件!)。
- 3、如文档侵犯您的权益,请联系客服反馈,我们会尽快为您处理(人工客服工作时间:9:00-18:30)。
Synthesis of zinc oxide nanotetrapods by a novel fast microemulsion-based hydrothermal methodJunying Jiang,Yanfen Li,Shengwei Tan,Zaiyin Huang ⁎College of Chemistry and Ecological Engineering,Guangxi University for Nationalities,Nanning 530006,PR Chinaa b s t r a c ta r t i c l e i n f o Article history:Received 25April 2010Accepted 8July 2010Available online 14July 2010Keywords:ZnO nanotetrapodsMicroemulsion-mediated hydrothermal Electrochemical analysisZnO nanotetrapods have been successfully synthesized via a novel microemulsion-mediated hydrothermal route at 120°C for 12h.X-ray power diffraction (XRD),field-emission scanning electron microscopy (FESEM),transmission electron microscopy (TEM),selected-area electron diffraction analysis (SAED),and electrochemical analysis (EA)were employed to study the structural features and electrochemical behavior of the products.It was found that these tetrapod ZnO nanostructures had a single crystal hexagonal wurtzite structure with lattice constants of a =0.3249nm and c =0.5205nm.And they exhibited a clearly electrocatalytic response,showing potential applications for sensor constructions.©2010Elsevier B.V.All rights reserved.1.IntroductionZnO is an attractive semiconductor material with a wide direct band gap (3.37eV at room temperature),large exciton binding energy (60meV),and high refractive index (N 2).It has attracted intensive research for its unique properties and versatile applications in transparent electronics,ultraviolet light emitters,piezoelectric devices,chemical sensors,spin electrics and so on [1,2].As we all know,the ability to control particle morphology is an important object in the synthesis of nanocrystals,as size and shape can signi ficantly in fluence various properties [3–5].Therefore,the target-oriented preparation of ZnO has become a big issue.Many techniques involving chemical vapor deposition,aqueous solution precipitation,microwave irradiation,thermal evaporation technique,hydrothermal synthesis,microemulsion method,gel –sol process,and electrodeposition have been used to prepare ZnO nanostructures.And lots of morphologies,such as nanobelts,nanowires,nanoneedles,nanotubes,nanosheets,and nanotetrapods have been obtained [6–18].Among them,nanote-tropod is a common morphology for ZnO and it has been extensively studied.Several methods have been developed to synthesize ZnO nanote-tropods,such as thermal evaporation methods [19–21],chemical vapor deposition [22–24],aqueous solution route and rapid thermal annealing technique [25],and so on.However,the most reported synthesis techniques are complicated,time and energy consuming.In particular,when organo-metallic precursors are used,complex procedures,high temperatures and sophisticated equipment to control the growth process are involved.For the further use of ZnOnanotetropods,a simple and inexpensive process with gentle conditions is required.Here,we present a novel fast microemulsion-based hydrothermal method to prepare ZnO nanotetrapods,which overcomes the shortcomings of previous preparation methods.Also,their electrochemical behavior was investigated by EA.2.ExperimentalZn(Ac)2·2H2O,NaOH,n-octanol,TritonX-100and cyclohexane were from Xilong Chemical Reagent Factory,which were of analytical grade and used without further puri fication.Poly (vinyl alcohol)(PVA MW 89,000–98,000)was bought from Sigma-Aldrich.Hemoglobin (Hb)from bovine blood was obtained from Fluka.In a typical procedure,firstly,one reverse microemulsion solution was prepared by adding 0.66mL 0.1M Zn(Ac)2aqueous solution into TritonX-100/cyclohexane/n-octanol system (according to the mass ratio TritonX-100/cyclohexane/n-octanol =3/8/2),which was stirred for 10min at room temperature until the microemulsion became transparent.Secondly,0.66mL 0.6M NaOH aqueous solution was added dropwise into the as-prepared reverse microemulsion solution and was kept stirred for another 20min.Then the mixed solutions were transferred to a 25mL Te flon-lined stainless-steel autoclave and kept at 120°C for 12h.After being cooled to room temperature naturally,white products were harvested by centrifugation and washed several times with acetone,deionized water and absolute ethanol,and then dried in vacuum at room temperature.The as-prepared products were characterized by X-ray diffraction (XRD,Philips PW1710with Cu K αradiation,λ=1.5406Å),field-emission scanning electron microscopy (FESEM,JEOL JSM-6700F),transmission electron microscopy (TEM,JEOL JEM-2010,200KV)andMaterials Letters 64(2010)2191–2193⁎Corresponding author.Tel.:+867713262120;fax:+867713261718.E-mail address:hzy210@ (Z.Huang).0167-577X/$–see front matter ©2010Elsevier B.V.All rights reserved.doi:10.1016/j.matlet.2010.07.026Contents lists available at ScienceDirectMaterials Lettersj o u r na l ho m e p a g e :w w w.e l s ev i e r.c o m /l o c a t e /m a t l e telectrochemical analysis(CHI660A and CHI440A electrochemical workstation,Shanghai Chenhua Instrument Co.,China).3.Results and discussionPowder XRD pattern of the as-prepared products is shown in Fig.1a.All of the diffraction peaks can be indexed to hexagonal wurtzite structural ZnO,and match well with standard hexagonal ZnO (a=3.249Å,c=5.205Å,JCPDS Card No.005-0664).The sharp and narrow diffraction peaks reveal that the synthesized nanotetrapods are highly crystallized[26].No impurity peaks were detected, indicating the formation of pure products.A typical FESEM image of the ZnO nanotetrapods is shown in Fig.1b, from which it can be seen that the as-prepared ZnO nanostructures were mainly tetrapods with leg length between200and300nm.To obtain further information about the nanostructure of these ZnO nanotetra-pods,TEM,HRTEM,and SAED analysis were performed.Fig.1c and d shows two typical TEM images of ZnO nanotetrapods,which have a typical leg length of~250nm.Fig.2a is the TEM image of the core of ZnO nanotetrapod corresponding to Fig.1d.Fig.1f,g and Fig.2b present the corresponding SAED patterns.The presence of the sharp diffraction spots rather than an amorphous ring is suggestive of the predicted formation of single crystalline ZnO.And these SAED patterns can be indexed to pure ZnO crystals of a hexagonal wurtzite structure.The HRTEM images(Fig.1e,Fig.2c and d)further indicate that the observed ZnO nanotetrapods are single crystalline with no defects of dislocations. The lattice fringes reveal that the single crystalline ZnO nanotetrapods possess interplanar spacing of about0.53nm,corresponding to the (002)plane of hexagonal ZnO.This clearly indicates that the ZnO nanoleg preferred growth along the[001]direction(c-axis).The HRTEM and SAED results also indicate that all four legs of the nanotetrapods grow along the[001]direction.Tetrapod-like ZnO with200–300nm length legs were prepared by a microemulsion-mediated hydrothermal process,which was due to the preferential growth of ZnO crystal growth along the[001] direction.Based on surface energy minimization,ZnO crystallites form zinc hydroxyl nucleate together because of excess saturation. Each of them individually grows along the c-axis into rod-like crystal, and then tetrapod-like architectures arefinally formed.A possible growth mechanism for the formation of nano-ZnO via a microemul-sion-mediated hydrothermal process was reported in the literature [27].The formation mechanism of ZnO nanoparticles was proposed based on the restriction effect of microemulsions in the crystal growth process.It is possible that many internal or external factors determined thefinal morphologies of nanocrystals during the process of nucleation and growth.The clear formation mechanism is not clear yet,we believe that the restricting effect of the microemulsion,the surfactant and cosurfactant molecules play critical roles in the morphology control.The details need further research.To investigate the electrochemical characteristics of ZnO nanote-trapods,we prepared a hydrogen peroxide(H2O2)sensor by doping ZnO nanotetrapods to the hybrid materials PVA/TiO2.Fig.3shows a typical cyclic voltammograms of the different modified electrodes in the absence of H2O2.It was found that the reduction peak current of Hb-PVA/TiO2/ZnO nanotetrapods/GC electrode(b)increased obvi-ously comparing with that of Hb-PVA/TiO2/GC electrode(a), indicating that the as-prepared ZnO nanotetrapods accelerate electrontransfer.Fig.1.(a,b)Power XRD pattern and typical FESEM image of the as-synthesized ZnO nanotetrapods,respectively,(c)TEM image of a single ZnO nanotetrapod,and the corresponding SAED pattern of the core(f),(d)TEM image of a single ZnO nanotetrapod,(e)HRTEM image from the outlined area in(d),and the corresponding SAED pattern(g).2192J.Jiang et al./Materials Letters64(2010)2191–21934.ConclusionIn summary,ZnO nanotetrapods have been successfully synthe-sized via a simple microemulsion-mediated hydrothermal route.The as-prepared products are high phase-purity with hexagonal wurtzite in crystal structure.Clearly EA at room temperature suggests that these materials may have good potential applications for sensor constructions.Further studies on the growth mechanism and characterization experiments are underway.AcknowledgementsThis work is financially supported by the National Natural Science Foundation of China (No.20963001);and theGuangxi Natural Science Foundation of China (No.0575030and No.0832078).References[1]Wang ZL.Chinese Sci Bull 2009;54:4021–34.[2]Wang ZL.Mater Sci Eng R 2009;64:33–71.[3]Ischenko V,Polarz S,Grote D,Stavarache V,Fink K.Adv Funct Mater 2005;15:1945–54.[4]Gao PX,Ding Y,Wang ZL.Nano Lett 2003;3:1315–20.[5]Zhang N,Yi R,Shi RR,Gao GH,Chen G,Liu XH.Mater Lett 2009;63:496–9.[6]Zhang YG,Lu F,Wang ZY,Zhang LD.J Phys Chem C 2007;111:4519–23.[7]Fu YS,Du XW,Kulinich SA,Qiu JS,Qin WJ,Li R,et al.J Am Chem Soc 2007;129:16029–33.[8]Seungho C,Seung HJ,Kun HL.J Phys Chem C 2008;112:12769–76.[9]Zhang J,Yang YD,Xu BL,Jiang FH,Li JP.J Cryst Growth 2005;280:509–15.[10]Deng Y,Wang GS,Li N,Guo L.J Lumin 2009;129:55–8.[11]Niederberger M.Acc Chem Res 2007;40:793–800.[12]Xu LF,Guo Y,Liao Q,Zhang JP,Xu DS.J Phys Chem B 2005;109:13519–22.[13]Pan ZW,Dai ZR,Wang ZL.Science 2001;291:1947–9.[14]Huang MH,Mao S,Feick H,Yan HQ,Wu YY,Kind H,et al.Science 2001;292:1897–9.[15]Zhang ZX,Yuan HJ,Zhou JJ,Liu DF,Luo SD,Miao YM,et al.J Phys Chem B 2006;110:8566–9.[16]Gao PX,Ding Y,Mai WJ,Hughes WL,Lao CS,Wang ZL.Science 2005;309:1700–4.[17]Yan HQ,He RR,Pham J,Yang PD.Adv Mater 2003;15:402–5.[18]Dai Y,Zhang Y,Wang ZL.Solid State Commun 2003;126:629–33.[19]Al-Azri K,Nor RM,Amin YM,Al-Ruqeishi MS.Appl Surf Sci 2010;256:5957–60.[20]Liu F,Zhang HR,Li JQ,Gao HJ.Nanotechnology 2004;15:949–52.[21]Wei J,Yang C,Man BY,Liu M.Physica B 2010;405:1976–9.[22]Ahmad M,Pan CF,Zhao J,Iqbal J,Zhu J.Mater Chem Phys 2010;120:319–22.[23]Zhang ZX,Sun LF,Zhao YC,Liu Z.Nano Lett 2008;8:652–5.[24]Yan BH,He RR,Pham J,Yang PD.Adv Mater 2003;15:402–5.[25]Lupan O,Chow L,Chai GY,Roldan B.Mater Sci Eng B 2007;145:57–66.[26]Marques APA,Picon FC,Melo DMA,Pizani PS,Leite ER,Varela JA,et al.J Fluoresc2008;18:51–9.[27]Li XC,He GH,Xiao GK,Liu HJ,Wang M.J Colloid Interface Sci 2009;333:465–73.Fig.2.TEM images of the core of ZnO nanotetrapod corresponding to Fig.1d (a),junction of three legs (c),and junction between two legs (d),respectively.(b)The SAED pattern of the tetrapod in(a).Fig.3.The cyclic voltammograms of different modi fied electrodes:(a)Hb-PVA/TiO2/GC electrode and (b)Hb-PVA/TiO2/ZnO nanotetrapods/GC electrode in the absence of H2O2in 0.12M pH 6.2PBS.2193J.Jiang et al./Materials Letters 64(2010)2191–2193。