MicrostructuralevaluationandnanohardnessofanAlCoCu
CALPHAD软件介绍
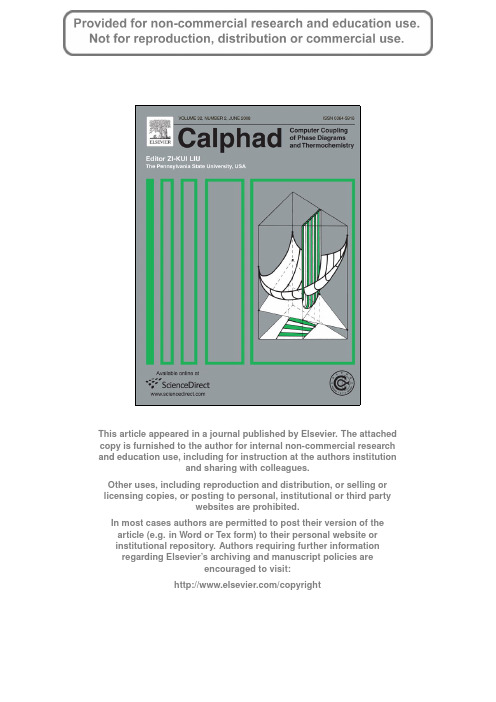
Abstract
The phase-field method has become an important and extremely versatile technique for simulating microstructure evolution at the mesoscale. Thanks to the diffuse-interface approach, it allows us to study the evolution of arbitrary complex grain morphologies without any presumption on their shape or mutual distribution. It is also straightforward to account for different thermodynamic driving forces for microstructure evolution, such as bulk and interfacial energy, elastic energy and electric or magnetic energy, and the effect of different transport processes, such as mass diffusion, heat conduction and convection. The purpose of the paper is to give an introduction to the phase-field modeling technique. The concept of diffuse interfaces, the phase-field variables, the thermodynamic driving force for microstructure evolution and the kinetic phase-field equations are introduced. Furthermore, common techniques for parameter determination and numerical solution of the equations are discussed. To show the variety in phase-field models, different model formulations are exploited, depending on which is most common or most illustrative. c 2007 Elsevier Ltd. All rights reserved.
美实验室开发出三维纳米圆锥太阳能电池
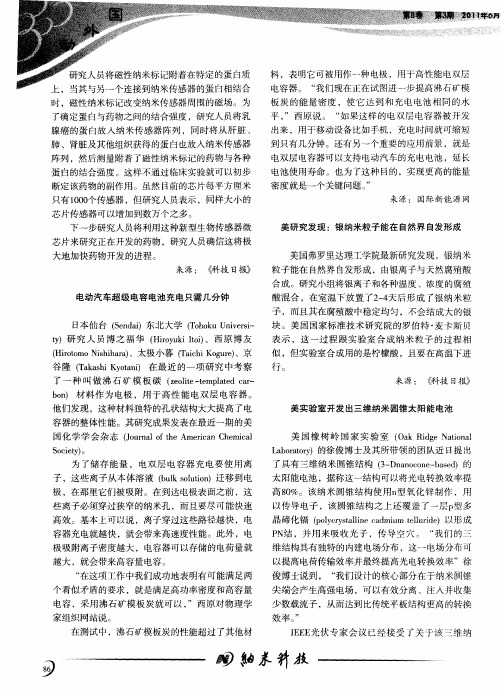
特的电子和光学性能,如能成功合成 ,将成为材料 学领域的一大突破。相关论文6 日发表在 ( 月7 ( 物理 评论快报B 杂志网络版上。 》 为了探讨是否存在 比钻石密度更大的碳结构 ,
研 究 人 员 朱 强 ( ) 和 地 质 与 物 理 学 教 授 阿特 穆 音
线数据的传输都 由设备 自行供 电,用一个电容器来 实现电力存储 。 这种传感器不仅仅用于医疗 ,还可以用于空中 摄 像机 、可穿戴 电子产 品等 ,套 用威廉吉布森 的 话 ,未来 已经来临。
容器充电就越快 ,就会带来高速度性能。此外 ,电 极吸附离子密度越大 ,电容器可 以存储的电荷量就 越大 ,就会带来高容量 电容 。 “ 在这项工作 中我们成功地表明有可能满足两 个看似矛盾 的要求 ,就是满足高功率密度和高容量 电容 ,采用沸石矿模板炭就可以 , ”西原对物理学
家 组织 网站说 。
行。
来源: 技 日报》
日本仙 台 ( n a S di e )东北大学 ( hk n e i T ouU i r— o v s t y )研究 人员博 之福 华 ( i yk I i Hr u i t) o o 、西 原博友
( rt i iaa、太极 小暮 fa h K gr) Hi o Ns hr) o mo h Ti i oue、京 c 谷 隆 (aah ytn1 在 最 近 的 一 项 研 究 中考 察 T ksi oai K
果 表 明 ,即使 在 10 米 的 尺度 上 ,人 造皮 肤 和 老 0纳
结构的一项重要特征。其 中t1具有 目前碳 同素异 P2 形体中最大的带 隙,电流在上面运行时将几乎毫无 阻力 ,这使其有望成为超导体和新型电子工程材料
的有 力 候选 人 。
鼠皮肤的反应都几乎相同。科学家称 ,这意味着在
国家纳米科学中心石墨烯纳米生物传感器研究取得新进展
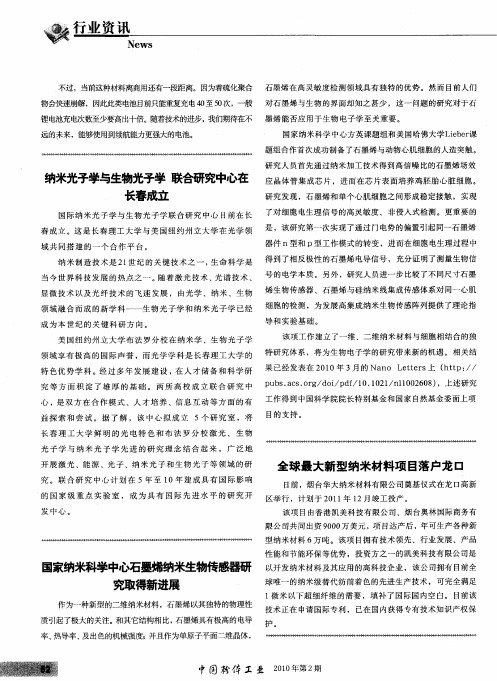
中 围糟 铸 工 韭 21年 期 0 第2 0
领 域融 合 而 成 的新 学 科一一 生 物光 子 学 和 纳米 光 子学 已经
成 为本 世 纪 的 关 键科 研 方 向。 美 国纽 约州 立 大学 布 法 罗 分校 在 纳 米学 、生 物 光子 学
细胞的检 测 ,为发展高 集成纳 米生物 传感 阵列 提供 了理 论指
导 和实验 基础 。
行业 资讯
Ne ws
不过 ,当前这种材料离商用还有一段距离。因为着硫化聚合 石墨烯 在高 灵敏度 检测领 域具 有独特 的优势 。然 而 目前 人们 物会快速崩解,因此此类电池目前只能重复充 电4 至 5 次 ,—般 对石 墨烯 与生物 的界面 却知之 甚少 ,这一 问题 的研究对 于石 J 0 D
球 唯一的 纳米级替 代纺前着 色 的先 进生产 技术 ,可 完全满足
1 米 以下超细 纤维 的需要 ,填 补 了国 际国 内空 白。 目前该 微
技术 正在 申请 国际专利 , 已在 国 内获得 专有技 术知识产 权保 质引起了极大的关注 。 和其它结构相 比, 石墨烯具有极高的电导 护 。
u s a s o g d i p f 0. 0 1 n l 0 6 8 ,上述研 究 究 等 方 面 积 淀 了 雄 厚 的 基 础 。 两 所 高 校 成 立 联 合 研 究 中 p b . c . r / o / d /l 1 2 / l 0 2 0 ) 心 ,是 双 方 在合 作 模 式 、人 才培 养 、信息 互 动 等 方 面 的 有 工作 得到 中国科学 院 院长特别基 金和 国家 自然基 金委面上项
该项 工作建 立了一 维 、二维纳 米材料 与细胞相 结合 的独
作者姓名:卢滇楠
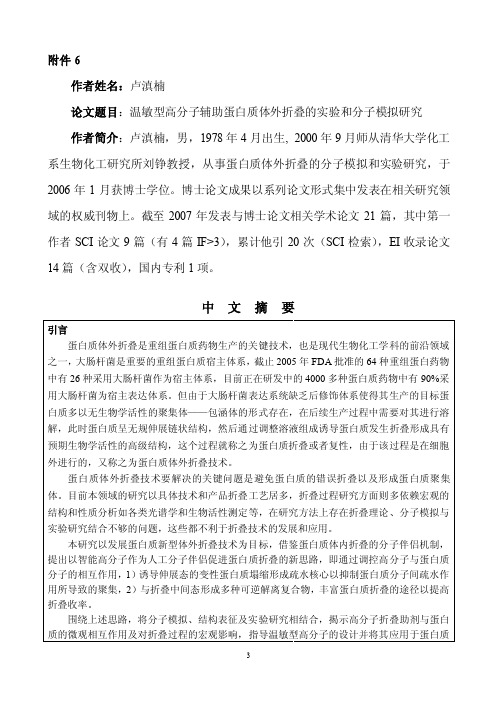
附件6作者姓名:卢滇楠论文题目:温敏型高分子辅助蛋白质体外折叠的实验和分子模拟研究作者简介:卢滇楠,男,1978年4月出生, 2000年9月师从清华大学化工系生物化工研究所刘铮教授,从事蛋白质体外折叠的分子模拟和实验研究,于2006年1月获博士学位。
博士论文成果以系列论文形式集中发表在相关研究领域的权威刊物上。
截至2007年发表与博士论文相关学术论文21篇,其中第一作者SCI论文9篇(有4篇IF>3),累计他引20次(SCI检索),EI收录论文14篇(含双收),国内专利1项。
中文摘要引言蛋白质体外折叠是重组蛋白质药物生产的关键技术,也是现代生物化工学科的前沿领域之一,大肠杆菌是重要的重组蛋白质宿主体系,截止2005年FDA批准的64种重组蛋白药物中有26种采用大肠杆菌作为宿主体系,目前正在研发中的4000多种蛋白质药物中有90%采用大肠杆菌为宿主表达体系。
但由于大肠杆菌表达系统缺乏后修饰体系使得其生产的目标蛋白质多以无生物学活性的聚集体——包涵体的形式存在,在后续生产过程中需要对其进行溶解,此时蛋白质呈无规伸展链状结构,然后通过调整溶液组成诱导蛋白质发生折叠形成具有预期生物学活性的高级结构,这个过程就称之为蛋白质折叠或者复性,由于该过程是在细胞外进行的,又称之为蛋白质体外折叠技术。
蛋白质体外折叠技术要解决的关键问题是避免蛋白质的错误折叠以及形成蛋白质聚集体。
目前本领域的研究以具体技术和产品折叠工艺居多,折叠过程研究方面则多依赖宏观的结构和性质分析如各类光谱学和生物活性测定等,在研究方法上存在折叠理论、分子模拟与实验研究结合不够的问题,这些都不利于折叠技术的发展和应用。
本研究以发展蛋白质新型体外折叠技术为目标,借鉴蛋白质体内折叠的分子伴侣机制,提出以智能高分子作为人工分子伴侣促进蛋白质折叠的新思路,即通过调控高分子与蛋白质分子的相互作用,1)诱导伸展态的变性蛋白质塌缩形成疏水核心以抑制蛋白质分子间疏水作用所导致的聚集,2)与折叠中间态形成多种可逆解离复合物,丰富蛋白质折叠的途径以提高折叠收率。
新兴材料研究的热点纳米材料期刊评介
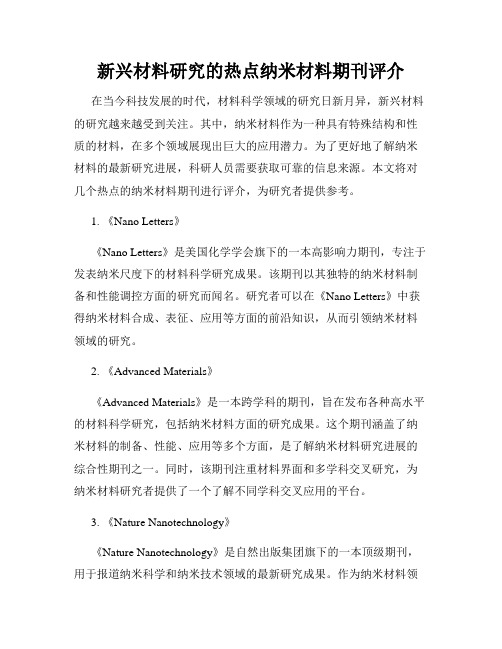
新兴材料研究的热点纳米材料期刊评介在当今科技发展的时代,材料科学领域的研究日新月异,新兴材料的研究越来越受到关注。
其中,纳米材料作为一种具有特殊结构和性质的材料,在多个领域展现出巨大的应用潜力。
为了更好地了解纳米材料的最新研究进展,科研人员需要获取可靠的信息来源。
本文将对几个热点的纳米材料期刊进行评介,为研究者提供参考。
1. 《Nano Letters》《Nano Letters》是美国化学学会旗下的一本高影响力期刊,专注于发表纳米尺度下的材料科学研究成果。
该期刊以其独特的纳米材料制备和性能调控方面的研究而闻名。
研究者可以在《Nano Letters》中获得纳米材料合成、表征、应用等方面的前沿知识,从而引领纳米材料领域的研究。
2. 《Advanced Materials》《Advanced Materials》是一本跨学科的期刊,旨在发布各种高水平的材料科学研究,包括纳米材料方面的研究成果。
这个期刊涵盖了纳米材料的制备、性能、应用等多个方面,是了解纳米材料研究进展的综合性期刊之一。
同时,该期刊注重材料界面和多学科交叉研究,为纳米材料研究者提供了一个了解不同学科交叉应用的平台。
3. 《Nature Nanotechnology》《Nature Nanotechnology》是自然出版集团旗下的一本顶级期刊,用于报道纳米科学和纳米技术领域的最新研究成果。
作为纳米材料领域最负盛名的期刊之一,《Nature Nanotechnology》向读者展示了涵盖纳米材料制备、性能调控、应用探索等各个方面的前沿研究。
该期刊以其高质量的论文和独特的视角享有崇高的声誉,对于研究者来说是全面了解纳米材料领域的重要渠道。
4. 《ACS Nano》《ACS Nano》是美国化学学会旗下的一本专注于纳米材料研究的期刊。
该期刊接收并发表了大量关于纳米尺度材料合成、表征、应用以及理论计算方面的研究成果。
研究人员可以在《ACS Nano》中找到有关纳米材料最新研究进展的详尽信息,并了解到该领域的前沿发展动态。
定量电子显微学方法与氧化钛纳米结构研究获国家自然科学二等奖
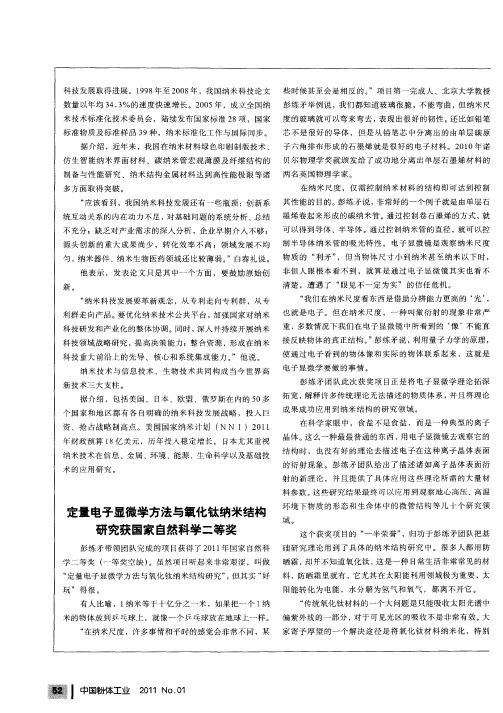
个 国家 和地 区都有 各 自明确 的纳 米 科技 发展 战略 ,投入 巨 成果 成功 应用 到纳米 结构 的研究 领域 。 在 科 学 家 眼 中 ,食 盐 不 是 食 盐 , 而 是 一 种 典 型 的 离 子 资 、抢 占战略制 高点 。美 国国家 纳米讨‘ ( 划 NN I)2 1 01 晶体 。 么一种 最最普 通的东 西 , 电子显微 镜去观 察它 的 这 用 年 财 政 预 算 l 美 元 ,历 年 投 入 稳 定 增 长 。 日本 尤 其 重 视 8亿 结构 时 ,也 没有 好的理 论去 描述 电子在 这种 离子 晶体 表面 纳 米技术在信 息 、金 属 、环境 、能源 、生命 科学 以及基础 技 的衍 射现 象 。彭 练矛 团 队给 出了描 述诸 如离 子 晶体表 面衍
仿 生智 能纳 米界 面材料 、碳纳 米管 宏观 薄膜 及纤 维结 构 的 贝 尔 物 理 学 奖 就 颁 发 给 了 成 功 地 分 离 出 单 层 石 墨 烯 材 料 的
制备与 性 能研究 、纳 米结 构金 属材 料达 到高 性 能极 限等诸 两 名 英 国 物 理 学 家 。
多 方面取得 突破 。
像 科 技 研 发 和 产 业 化 的 整 体 协 调 。同 时 , 入 并 持 续 开 展 纳 米 重 ,多 数 情 况 下 我 们 在 电 子 显 微 镜 中所 看 到 的 ‘ ’不 能 直 深 接反 映物体 的真正结 构 。 练矛 说 , 用量 子力学 的原理 , ”彭 利 科技领 域战 略研 究 ,提高 决策 能力 ;整合资 源 ,形成在纳 米
nmfe算法 rna折叠最小自由能 -回复

nmfe算法rna折叠最小自由能-回复【NMFE算法RNA折叠最小自由能】RNA(核糖核酸)是一类生物分子,其序列中的碱基决定了其结构和功能。
折叠是指RNA分子通过碱基之间的互补配对形成特定的结构,进而发挥特定的功能。
RNA分子的折叠状态对于生物体的正常生理过程起着关键作用。
RNA折叠的问题可以形式化为在给定RNA序列的条件下,如何找到能量最小的折叠构象。
这是一个计算复杂度很高的问题,需要应用数学和计算机科学的技术。
近年来,科学家们提出了多种算法用于求解RNA折叠最小自由能的问题。
其中一种重要的算法是NMFE(Nucleotide Mutational Folding Evaluation)算法。
以下是NMFE算法的一步一步介绍:第一步:选择RNA序列作为输入,我们需要选择一个特定的RNA序列作为算法的初始输入。
RNA 序列是由四种不同的核苷酸(腺嘌呤A、尿嘧啶U、鸟嘌呤G和胞嘧啶C)组成的。
第二步:建立目标函数在NMFE算法中,我们需要定义一个目标函数来刻画给定的折叠结构的自由能。
自由能是描述了系统处于某个状态的稳定程度的物理量,其数值越小,表示该状态越稳定。
第三步:构建动态规划模型NMFE算法通过动态规划的方式来求解RNA折叠最小自由能问题。
动态规划是一种将复杂问题分解为相对简单的子问题并重复利用子问题解的方法。
在NMFE算法中,我们采用了自底向上的策略来构建动态规划模型。
具体地,我们从RNA序列的末端开始,逐渐向前计算,直到获得整个序列的最优折叠结构。
第四步:计算最小自由能在动态规划模型中,我们需要定义一系列的状态和转移方程来计算每一步的最小自由能。
具体来说,我们可以定义一个二维数组,每个元素代表了从第i个碱基到第j个碱基之间的最小自由能。
通过填充这个数组,我们可以递推地计算每一个子问题的最小自由能。
第五步:回溯获得最优解在计算得到最小自由能的同时,我们还需要记录每一步的选择,以便于最后回溯获得最优解的折叠结构。
可见光响应催化剂BiVO4六角形微米棒的水热合成

( oeeo hmir n hm cl nie r g C nr o t U i ri, hnsa4 0 8 , hn ) lg C e sya dC e ia E gn ei , et l uh nv sy C agh 1 0 3 C ia Cl f t n aS e t
A s a t s g B ( 0)・ H 0 a d N 4 O s rw ma r l i O coo sw t hx gn sa e w r b t c:U i iN 3 5 , n H V 3a a t i s V 4mi rd i eao h p ee r n 3 e a ,B r h
snh s e v h doh r lme o i oyiy pro d n (V )a e pae T epo et so e a‘ v tei d b y rtema z t d w t p l n l yrl o e P P stm lt. h rp re ft s h h v i i h
p e a e a l s we e c a a trz d b r p r d s mp e r h r c e ie y XRD, EM , R, ma n S I Ra n a d UV— s s c r s o y Vi pe to c p .Th e u t e e Jt t e r s ls r v a ha u il m a d we1 r salz d nfr 0 n 1 c y tlie Bi VO4he a o . c o o s n x g n mir r d i mo o ln c r sa sr c u e c ul b o t i e t n ci i cy t l tu t r o d e b a n d wih d mee f 1 5~ 00 m n e gh f . i tr0 2 3 n a d l n t o 10~16 L v a a h d oh r l o t s ie b . Lm i y r t e ma r u e a std y PVP.Co a e wih mp r d t Bi VO4 atce ,t b v b a n d mir r d y 2. VP h w ma lr d s i n t e a s r to a d a t ril s he a o e o t i e c o o sb 0 g P p s o a s l e h f i h b o p in b n nd l t s
- 1、下载文档前请自行甄别文档内容的完整性,平台不提供额外的编辑、内容补充、找答案等附加服务。
- 2、"仅部分预览"的文档,不可在线预览部分如存在完整性等问题,可反馈申请退款(可完整预览的文档不适用该条件!)。
- 3、如文档侵犯您的权益,请联系客服反馈,我们会尽快为您处理(人工客服工作时间:9:00-18:30)。
International Journal of Minerals, Metallurgy and Materials Volume 26, Number 5, May 2019, Page 634https:///10.1007/s12613-019-1771-3Corresponding author: C.D. Gómez-Esparza E-mail: cynthia.gomez@.mx© University of Science and Technology Beijing and Springer-Verlag GmbH Germany, part of Springer Nature 2019Microstructural evaluation and nanohardness of an AlCoCuCrFeNiTihigh-entropy alloyC.D. Gómez-Esparza1), R. Peréz-Bustamante2), J.M. Alvarado-Orozco3), J. Muñoz-Saldaña4),R. Martínez-Sánchez1), J.M. Olivares-Ramírez5), and A. Duarte-Moller1,6)1) Centro de Investigación en Materiales Avanzados (CIMAV), Laboratorio Nacional de Nanotecnología, Miguel de Cervantes No. 120, Chihuahua 31136, Chihuahua,México2) CONACYT-Corporación Mexicana de Investigación en Materiales S.A. de C.V. (COMIMSA), Ciencia Y Tecnología 790, Fracc. Saltillo 400, Saltillo 25290, Coahuila,México3) Centro de Ingeniería y Desarrollo Industrial, Querétaro Campus, Querétaro 76130, Qro., México4) Centro de Investigación y de Estudios Avanzados del IPN, Unidad Querétaro, Libramiento Norponiente No. 2000, Fracc. Real de Juriquilla, Querétaro, Qro., 76230,México5) Universidad Tecnológica de San Juan del Río, San Juan del Río 76800, Querétaro, México6) Escuela de Ingeniería Civil, Industrial y Mecánica, Universidad De La Salle Bajío, León 37150, Guanajuato., México(Received: 6 July 2018; revised: 1 December 2018; accepted: 2 December 2018)Abstract: An AlCoCuCrFeNiTi high-entropy alloy (HEA) was prepared by mechanical alloying and sintering to study the effect of Ti addi-tion to the widely studied AlCoCuCrFeNi system. The structural and microstructural characteristics were investigated by X-ray diffraction (XRD), scanning electron microscopy (SEM), and transmission electron microscopy (TEM). The formation of four micrometric phases was detected: a Cu-rich phase with a face-centered cubic (fcc) structure, a body-centered cubic (bcc) solid solution with Cu-rich plate-like preci-pitates (fcc), an ordered bcc phase, and a tetragonal structure. The XRD patterns corroborate the presence of a mixture of bcc-, fcc-, and te-tragonal-structured phases. The Vickers hardness of the alloy under study was more than twice that of the AlCoCuCrFeNi alloy. Nanoinden-tation tests were performed to evaluate the mechanical response of the individual phases to elucidate the relationship between chemical composition, crystal structure, and mechanical performance of the multiphase microstructure of the AlCoCuCrFeNiTi HEA.Keywords: high-entropy alloys; mechanical alloying; microstructure; nanoindentation1. IntroductionConventional alloys consist of one or two elements as ma-jor constituents with small amounts of other elements, which promotes the improvement of properties. High-entropy alloys (HEAs), defined by Yeh et al. [1] as those formed by at least five main elements, may exhibit characteristics superior to those of conventional alloys, such as solid solution forma-tion, nanometer microstructures, good thermal stability, high hardness, and good mechanical properties [2]. The solid so-lutions containing more than five major elements tend to have structures such as face-centered cubic (fcc), body-centered cubic (bcc), and bcc + fcc. They are more stable than the many conventional intermetallic structures that can be formed according to the predictions of binary phase dia-grams reported in the specialized literature. The structure and phases formed in the HEAs depend on the number of alloying elements and their nature (atomic radii, crystalline structure, electronegativity, melting point), as well as their synthesis process. HEAs have been mainly processed by liquid routes; however, interest in the use of powder metal-lurgy to synthesize these advanced materials has increased. Mechanical alloying has been used to produce nanocrystal-line solid-solution phases under the concept of HEAs [3–4].The AlCoCrCuFeNi system has been the most studied HEA in terms of microstructure and mechanical properties.C.D. Gómez-Esparza et al., Microstructural evaluation and nanohardness of an AlCoCuCrFeNiTi high-entropy alloy 635In principle, most of the investigated systems contain Cu, which improves plasticity. Ni, Co, and Fe are of interest in engineering applications because of their excellent mechan-ical performance, high-temperature mechanical resistance, and resistance to corrosion. They are constituents of most of the reported HEAs. Although Al is a low-melting and very ductile element, its effect has been extensively studied in HEAs. It tends to enhance the mechanical properties of HEAs because it is a bcc phase former. Cr is a high-melting-point element that improves mechanical strength and corrosion re-sistance. Ti is a high-melting element with an atomic radius similar to that of Al. Some authors have attributed the en-hancement of mechanical strength and ductility of HEAs to Ti content [5–6]. Although the effect of Ti in AlCoCrCuFeNi alloy has been investigated, the relationship between alloying elements and the crystalline structure of the formed phases in an equiatomic AlCoCrCuFeNiTi alloy is not yet clear.On the other hand, knowledge of local mechanical prop-erties in multiphase materials in micrometric scale, such as HEAs, can represent a great advance in understanding and predicting their bulk properties. Low-load indentation, or nanoindentation, using the Oliver–Pharr method is a very versatile technique that enables mechanical properties to be measured at small scale (micro and submicrometric) [7]. In past years, investigations related to nanoindentation tests of HEAs have been conducted to obtain more information about specific properties of individual phases [8–9]. Be-cause of the nature of alloying elements, equiatomic Al-CoCrCuFeNiTi alloy is expected to possess a multiphase microstructure. Hence, the aim of this work is to study an equiatomic AlCoCrCuFeNiTi HEA produced by mechanical alloying and conventional sintering to understand the effect of Ti on the chemical distribution and crystalline structure of the resulting phases. In addition, the evaluation of hardness and reduced elastic modulus of its individual phases through nanoindentation tests could establish a relationship between chemical composition, crystalline structure, and mechanical properties of AlCoCrCuFeNiTi-type HEAs.2. Experimental2.1. MaterialsElemental powders of Al, Co, Cr, Cu, Fe, Ni, and Ti with a purity level greater than 99% were used as raw materials. These powders were mixed in equiatomic composition to form a high-entropy AlCoCrCuFeNiTi alloy. The milling process was performed in a high-energy mill (SPEX-8000M) under an argon atmosphere to avoid excessive oxidation of the powders. A hardened steel vial and hardened steel balls were used to mill the samples and were pre-coated by mil-ling an equiatomic NiCoAl powder mixture for 10 h under an Ar atmosphere to avoid iron contamination from the vial and grinding media. Methanol was used as a process control agent to balance welding and fracturing processes during milling. The milling time was 10 h, and the ball-to-powder weight ratio was 5:1. After milling, the alloyed powders were cold compacted at a pressure of 1.5 GPa, sintered at 1200°C under vacuum for 3 h, and cooled in the furnace to room temperature.2.2. Microstructural characterizationThe sintered alloy was microstructurally characterized by field-emission scanning electron microscopy (FESEM; JEOL JSM-7401). The microscope was equipped with an X-ray scattering spectrometer. In order to characterize the phase composition, a sintered sample was prepared using a focused ion beam system (JEM-9320FIB) equipped with an Omniprobe 200 nanomanipulator system and then analyzed by transmission electron microscopy (TEM; JEOL JEM2200F). X-ray diffraction (XRD) analysis was performed on a Pana-lytical X'Pert PRO diffractometer equipped with a Cu Kαradiation source (λ = 0.15406 nm).2.3. Mechanical testingThe mechanical behavior of the alloy was evaluated by Vickers microhardness tests on a Future-Tech Corp. tester model FM-7 using a load of 300 g and a dwell time of 15 s. The nanoindentation tests were carried out on a Ubi1 nano-mechanical tester, Hysitron, USA, using a Berkovich trian-gular pyramid diamond tip.3. Results and discussion3.1. Microstructural characterization3.1.1. Transmission electron microscopyBecause of the complex chemical interaction among the different HEA elements during sintering, TEM analyses were carried out on sintered samples to study the micro-structural details and compositional analysis. The equili-brium microstructure of the AlCoCrCuFeNiTi alloy synthe-sized by mechanical alloying and sintering and the respec-tive chemical composition of their phases, as determined by energy-dispersive X-ray spectroscopy, are shown in Fig. 1. Four micrometric homogeneously distributed phases were observed (labeled as A, B, C, and D). The dark phase de-picted as A corresponds to the high-Fe matrix with low con-tents of Al and Ti and with rich-Cu plate-like nanoprecipi-tates well dispersed in it. The formation of nanoprecipitates636 Int. J. Miner. Metall. Mater ., Vol. 26, No. 5, May 2019in HEAs due to high mixing entropy has been reported [10]. In addition, the Cu segregation is related to the difference in electronegativities and enthalpies of formation with the rest of the elements present in the alloy under study. According to the binary phase diagrams, Cu has a very limited solid solubility with Fe, Co, and Cr, which explains the precipita-tion of Cu in a matrix with large Cr and Fe contents.In the zone denoted as B, a high content of Ni, Co, and Ti, an intermediate Al content, and low concentrations of Cu and Cr were detected. The phase C shows a high content of Cr, Fe, and Co and a very low concentration of Al and Ni. Also, the zone marked as D shows a Cu-rich crystallized phase. Cu tends to segregate at grain boundaries in HEAs synthesized via liquid routes [11]. Cu has a high positive mixing enthalpy with Co, Cr, and Fe, whereas it has a lowerpositive enthalpy with Ni and Al [12].Fig. 1. Z-contrast image by scanning transmission electron microscopy (STEM) of AlCoCrCuFeNiTi alloy, and chemical composi-tion of individual phases by EDS.To show the effect of Ti in the AlCoCrCuFeNiTi HEA, TEM micrographs of the microstructures of AlCoCuFeNi and AlCoCrCuFeNi alloys, previously reported and synthe-sized under the same conditions [13], are displayed together with a micrograph of the AlCoCrCuFeNiTi HEA alloy in Fig. 2 for comparative purposes. The AlCoCuFeNi alloy (Fig. 2(a)) has two main phases. The first phase is composed of a high-Fe-content matrix and Cu-rich nanometric preci-pitates in a plate-shape arrangement disposed in perpendi-cular and parallel angles, forming a basket-like microstruc-ture. The second phase has a high content of Fe, Co, and Ni, approximately 28at% each. The microstructure of the Al-CoCrCuFeNi alloy (Fig. 2(b)) is very similar to the AlCo-CuFeNi alloy. The effect of Cr addition is mainly evidenced by the formation of a third phase with high Cr content (˃80at%). In comparison with the previous alloys, the Al-CoCrCuFeNiTi alloy (Fig. 2(c)) has a similar phase with a high-Fe-content matrix with immersed Cu-rich plate-like precipitates (phase A). The spacing and size of the plate-like precipitates increase as more alloying elements are added (Cr and Ti). The phase identified as B has a high content of Ni, Co, Ti, and Al; in comparison with the previous alloys, the Fe content decreases, whereas substantial amounts of Al and Ti are detected. Phase C also has a high Cr content with a substantial increase in Fe and Co amounts. Phase D is a Cu-rich solid solution that was not observed in the previousFig. 2. TEM micrographs of the sintered alloys to compare the microstructural evolution as a function of chemical composition: (a) AlCoCuFeNi; (b) AlCoCrCuFeNi; (c) AlCoCrCuFeNiTi.C.D. Gómez-Esparza et al., Microstructural evaluation and nanohardness of an AlCoCuCrFeNiTi high-entropy alloy637alloys. The enthalpy of mixing values for the pairs Ti–Ni and Ti–Al are highly negative [14], which promotes the sol-id solubility of Ti in enriched Al and/or Ni phases. Hence, the results suggest that the complex microstructure observed is mainly due to the limited solid solubility of Cu with the other alloying elements.Figs. 3 and 4 show TEM images and selected-area elec-tron diffraction (SAED) patterns of the crystal-structure phases in the AlCoCrCuFeNiTi alloy. On the basis of the collected SAED pattern of phase A (Fig. 3), the matrix ([–111] zone axis) has a bcc structure, whereas the plate-like precipitates ([011] zone axis) have a fcc structure. A Z-contrast STEM micrograph (Fig. 4(a)) correlates the B, C, and D phas-es (Figs. 4(b)–4(d)) with their respective dot patterns.Fig. 3. TEM micrograph of phase A in the AlCoCrCuFeNiTi alloy (a), and SAED patterns of the matrix (b) and plate-like precipi-tates (c).Fig. 4. TEM micrograph (a) and SAED patterns of phase B (b), phase C (c) and phase D (d) in the AlCoCrCuFeNiTi alloy.Phases B and D were observed along the [200] and [321] zone axes, having a bcc and an fcc crystal structure, respec-tively. Phase C, which was observed along the [321] zone axis, is consistent with a tetragonal structure. Notably, both fcc phases, which correspond to plate-like precipitates of phase A and phase D, have a similar chemical composition; however, a Cu content greater than 70at% was detected. Phase B has a high Al content (an fcc-structured element) and crystallizes with a bcc structure, corroborating the as-sumption that Al is a bcc former [15].The precipitation of nanocrystalline phases in the HEA synthesized via a liquid route and subsequent thermal treat-ment is considered an important factor affecting the me-chanical properties of the alloys [16]. In this study, the alloy638 Int. J. Miner. Metall. Mater., Vol. 26, No. 5, May 2019AlCoCrCuFeNiTi processed by mechanical alloying gave rise to the formation of nanocrystalline phases without the need for a subsequent thermal treatment. These phases re-mained on the nanometer scale even after the conventional high-temperature sintering process.3.1.2. X-ray diffractionThe diffraction pattern of the sintered AlCoCrCuFeNiTi alloy is presented in Fig. 5. Two fcc-structured phases, two bcc-structured phases, one of which is ordered, and a fifth phase that does not correspond to a cubic structure are present. The lattice parameters of the phases were calculated from their peak positions. The lattice parameter of the two fcc phases (0.362 and 0.360 nm) is similar to that of pure Cu. These phases correspond to phase D and the plate-like precipitates of phase A. The ordered bcc phase (B2) [17] has a lattice parameter of 0.292 nm, similar to that reported for an Al11.1(TiVCrMnFeCoNiCu)88.9 alloy (a bcc = 0.293 nm) [10]. In a previous AlCoCrCuFeNi alloy, an ordered bcc solid solution was not detected [13]. Howev-er, Chen et al. [18] reported that ordering of a bcc phase in an Al0.5CoCrCuFeNiTi1.4 alloy occurs as a function of Ti content. Their finding corroborates that phase B, which has a bcc structure and contains a substantial amount of Ti (21at%), possesses a bcc-B2 phase. The solid solutions are formed by solute atoms of different elements. In HEAs, each atom is surrounded by different types of atoms with different atomic sizes depending on the chemical composi-tion of the alloy. Therefore, the crystalline structure suffers distortion, causing its lattice parameter to differ from that previously reported for a similar solid solution.Fig. 5. XRD pattern of AlCoCrCuFeNiTi alloy.The lattice parameter of the second bcc phase is 0.304 nm, which corresponds to the matrix of phase A. The fifth phase presents diffraction peaks at 46°, 46.98°, and 48.18° 2θ. Some authors have reported this phase as γ-NiCoCr [15] or a CoCr tetragonal phase [15,19], and the peak at 36.2° 2θin Fig. 1 has been attributed to a Ti2Ni-type phase [19]. The XRD peak locations were verified with the Inorganic Crys-tal Structure Database (ICSD), and the diffraction peaks were found to correspond to a structure similar to σ-CoCr (ICSD card No. 102316), differing slightly from the peaks of the isolated CoCr system [20]. The diffraction peaks at 34.09°, 36.73°, 40.42°, 56.69°, 73.00°, and 78.16° 2θ (not identified in the spectrum) correspond to aluminum oxide. HEAs have a tendency to form random solid solutions in-stead of intermetallic or complex phases, but some com-pounds with a high enthalpy of formation, such as oxides, carbides, and nitrides, will be formed [21]. The formation of aluminum oxide in sintered HEAs was reported in our pre-vious work [22]. The XRD results related to the formation of solid-solution phases corroborate the results obtained from SAED–TEM patterns.3.2. Mechanical testing3.2.1. Vickers microhardnessThe microstructure of HEA processed by a liquid route exhibits the peculiarity of segregation, which is a characte-ristic of this processing technique [23]. Although the alloy under study has a multiphase microstructure, the use of me-chanical alloying produces a homogeneous distribution of phases in the bulk material, which may exceed the distribu-tion achieved by the liquid route. The sintered AlCoCrCu-FeNiTi alloy was subjected to Vickers microhardness tests, and a value of HV 602 ± 44 was obtained. The microhard-ness values reported for the previous alloys AlCoCuFeNi and AlCoCrCuFeNi synthesized under the same conditions were HV 261 ± 31 and HV 269 ± 39, respectively [13]. Notably, the addition of Ti promoted an increase of hardness to more than twice that of its predecessors. A similar beha-vior was reported by Chen et al. [18], where the Al0.5CoCrCuFeNiTi x system exhibited an increase in me-chanical response as a function of the Ti content. Specifical-ly, the Al0.5CoCrCuFeNiTi x alloy exhibited a hardness value of HV 650.The atomic radii of the constituent elements of the stu-died alloy differ from each other. Al and Ti have the largest atomic radii of 0.143 and 0.146 nm, respectively. The ob-tained microhardness values can be attributed to the effect of solid-solution hardening, which increases the lattice distor-tion and avoids interplanar displacement and dislocations. Al has been reported to promote the formation of a bcc phase that is responsible for the enhancement of the me-chanical properties of AlCoCuCrFeNi-type alloys [24]. In the studied AlCoCrCuFeNiTi alloy, the formation of a bcc phase with high Al content is evident (phase B). However, phase precipitation of Cu at the nanoscale (plate-like preci-pitates in phase A) favors hardening of the alloy. These har-C.D. Gómez-Esparza et al., Microstructural evaluation and nanohardness of an AlCoCuCrFeNiTi high-entropy alloy 639dening mechanisms also contribute to the observed increase in hardness.The multiphase microstructure in advanced metallic ma-terials is responsible for their superior mechanical properties. The evaluation of local mechanical properties in multiphase HEAs can support future experimental chemical designs, giving deeper evidence of the relationship between chemical composition, crystal structure, and mechanical properties. Hence, nanoindentation tests were used to determine the lo-cal mechanical behavior of the sintered AlCoCrCuFeNiTi alloy.3.2.2. NanoindentationThe mechanical properties of individual phases in the AlCoCrCuFeNiTi alloy were evaluated by the nanoindenta-tion technique. Notably, the evaluated mechanical properties of the individual phases of the alloy were supported by mi-croscopy techniques. Nanoindentation impression images taken by in situ scanning probe microscopy (SPM) were compared with SEM micrographs collected in secondary electron (SE) mode (where the phases had already been pre-viously identified). An example is displayed in Fig. 6. The SEM–SE image (Fig. 6(a)) shows the morphology of the phases, whereas the SPM image (Fig. 6(b)) shows the na-noindentation impressions. At least 10 nanoindentation mea-surements were conducted for each phase. For practical pur-poses, the graph in Fig. 6(c) shows a single load–displacement curve for each tested phase. The chosen curves are repre-sentative of the individual phase response. The average val-ues of nanohardness and reduced elastic modulus are sum-marized in Table 1. The tetragonal phase exhibited the high-est nanohardness value (~19 GP), followed by the bcc-B2 phase (~17 GP). However, the reduced elastic modulus (E r ) value for the tetragonal phase was only 9% higher than that of the bcc-B2 phase. The tetragonal phase influences the hardening of the bulk alloy without sacrificing the ductility of the material. Even phase A (bcc matrix with fcc plate-like precipitates) reached only half the hardness of the bcc-B2 phase; its E r value was only 5% lower. Phase D (high Cu content and fcc structure) exhibited the worst mechanical properties among the phases. We assumed that the basket-like arrangement of Cu precipitates has a hardening effect on phase A, as evidenced by the slight reduction of modulus in comparison with those of the bcc-B2 and tetra-gonal phases.Fig. 6. SEM-SE micrograph (a), SPM micrograph of nanoindentation impressions (b) and representative load-displacement curves (c) for the four identified phases in the AlCoCrCuFeNiTi alloy.Table 1. Average and standard deviation (SD) of nanohardness and reduced modulus obtained from nanoindentation tests (1000 mN of load).Phase Crystal structure Nanohardness / GPa E r / GPaAverage SD Average SDAbcc matrix + fcc2 plate-like precipitates8.720.7433718B bcc-B2 17.25 0.75 356 20C Tetragonal 19.24 0.71 389 16D fcc1 6.48 0.65 242 25The experimental evidence suggests that, in addition to the entropy effect, other factors also influence the formation of phases in the HEA. In terms of relevance, a well-known principle in metallurgy describes the formation of phases: the Hume–Rothery rules on the formation of binary solid solutions. These rules establish that the high solubility of one element in another depends directly on a small differ-ence in their electronegativity, atomic radius, crystal struc-640 Int. J. Miner. Metall. Mater., Vol. 26, No. 5, May 2019ture, valence [25], and their melting temperature [26]. Even though there are different solubility levels of some elements in others, the formation of solid solutions instead of inter-metallic compounds is evident. One of the hardening me-chanisms of HEAs is solid-solution hardening [27]; atoms with larger sizes, such as Ti and Al, induce lattice distortion. In addition, high-melting-point elements exhibit less diffu-sion; the high-melting points are related to the increase in the modulus of elasticity. Tong et al. [28] reported a nano-sized spinodal microstructure in an AlCoCrCuFeNi alloy produced by a liquid route. They attributed the increase in hardness to the hardening effect of nanocomposite forma-tion. The Cu-rich plate-like nanoprecipitates are expected to enhance the mechanical properties of HEAs; therefore, some authors have adjudged this phenomenon as the cause of the hardening. However, in the present study, the Cu-rich phases were demonstrated to be those with the poorest me-chanical properties, even when they exist as precipitates with nanoscale size and spacing. However, previously re-ported nanoindentation results for AlCoCuFeNi and Al-CoCrCuFeNi alloys [13] show that Ti addition increases the matrix hardness of phases with Cu-rich nanoprecipitates. The phases with Cu-rich plate-like precipitates in the Al-CoCuFeNi and AlCoCrCuFeNi alloys exhibit a lower hard-ness and lower E r value compared with those reported for the similar phase in the studied AlCoCrCuFeNiTi alloy, which has a matrix Ti content of ~2at%. The solid-solution hardening effect is greater than the precipitation hardening effect. In addition, the phase sizes in the AlCoCrCuFeNiTi alloy are smaller than those observed in the AlCoCuFeNi and AlCoCrCuFeNi alloys (Fig. 2). In materials with mi-crometric-scale phase sizes, the volumetric fraction of grain boundaries increases with decreasing phase size. Grain boundaries act as obstacles to the movement of dislocations.4. ConclusionsAn AlCoCrCuFeNiTi HEA was synthesized by mechan-ical alloying and conventional sintering. The sintered alloy exhibits a multiphase microstructure composed of four phases with fcc, bcc, and tetragonal structures. According to TEM analyses, Cu promotes the formation of fcc phases, whereas Al and Ti are bcc formers. The tetragonal phase possesses high Cr, Fe, and Co contents. The elastic modulus and hardness for individual phases were successfully eva-luated by nanoindentation tests. The tetragonal phase exhi-bited the best mechanical properties, with average hardness and modulus values of 19.24 and 389 GPa, respectively, followed by the bcc-B2 phase, with average hardness and modulus values of 17.25 and 356 GPa, respectively. Ac-cording to the modulus values, the tetragonal phase achieved the highest hardening without substantially in-fluencing the ductility of the material. The Cu-rich phase with an fcc structure exhibited the worst mechanical proper-ties among the investigated phases. AcknowledgementsThe authors would like to thank W. Antunez-Flores and C.E. Ornelas-Gutiérrez for their technical assistance. References[1] J.W. Yeh, S.K. Chen, J.Y. Gan, S.J. Lin, T.S. Chin, T.T.Shun, C.H. Tsau, and S.Y. Chang, Formation of simple crys-tal structures in Cu–Co–Ni–Cr–Al–Fe–Ti–V alloys with mul-tiprincipal metallic elements, Metall. Mater. Trans. A,35(2004), p. 2533.[2] S.T. Chen, W.Y. Tang, Y.F. Kuo, S.Y. Chen, C.H. Tsau, T.T.Shun, and J.W. Yeh, Microstructure and properties of age-hardenable Al x CrFe1.5MnNi0.5 alloys, Mater. Sci. Eng. A,527(2010), No. 21-22, p. 5818.[3] R. Sriharitha, B.S. Murty, and R.S. Kottada, Phase formationin mechanically alloyed Al x CoCrCuFeNi (x = 0.45, 1, 2.5, 5 mol) high entropy alloys, Intermetallics,32(2013), p. 119. [4] N.T.B.N. Koundinya, C.S. Babu, K. Sivaprasad, P. Susila,N.K. Babu, and J. Baburao, Phase evolution and thermal analysis of nanocrystalline AlCrCuFeNiZn high entropy alloyproduced by mechanical alloying, J. Mater. Eng. Perform.,22(2013), No. 10, p. 3077.[5] Y.F. Wang, S.G. Ma, X.H. Chen, J.Y. Shi, Y. Zhang, andJ.W. Qiao, Optimizing mechanical properties of AlCoCrFe-NiTi x high-entropy alloys by tailoring microstructures, ActaMetall. Sin.-Engl., 26(2013), No. 3, p. 277.[6] P. Cui, Y.M. Ma, L.J. Zhang, M.D. Zhang, J.T. Fan, W.Q.Dong, P.F. Yu, G. Li, and R.P. Liu, Effect of Ti on micro-structures and mechanical properties of high entropy alloys based on CoFeMnNi system, Mater. Sci. Eng. A,737(2018),No. 8, p. 198.[7] W.Y. Yan, C.L. Pun, and G.P. Simon, Conditions of applyingOliver–Pharr method to the nanoindentation of particles in composites, Compos. Sci. Technol., 72(2012), No. 10, p.1147.[8] C.S. Babu, N.T.B.N. Koundinya, K. Sivaprasad, and J.A.Szpunar, Thermal analysis and nanoindentaion studies on nanocrystalline AlCrNiFeZn high entropy alloy, ProcediaMater. Sci.,6(2014), p. 641.[9] V. Maier-Kiener, B. Schuh, E.P. George, H. Clemens, and A.Hohenwarter, Nanoindentation testing as a powerful screen-ing tool for assessing phase stability of nanocrystalline high-entropy alloys, Mater. Des.,115(2017), p. 479.[10] C.M. Lin and H.L. Tsai, Evolution of microstructure, hard-。