The Forward Time Projection Chamber (FTPC) in STAR
PhysRevLett.110.012301

Charge separation relative to the reaction plane in Pb-Pb collisions at ffiffiffiffiffiffiffiffiffis NN p ¼2:76TeVB.Abelev et al.*(ALICE Collaboration)(Received 5July 2012;published 2January 2013)Measurements of charge-dependent azimuthal correlations with the ALICE detector at the LHC arereported for Pb-Pb collisions at ffiffiffiffiffiffiffiffis NN p ¼2:76TeV .Two-and three-particle charge-dependent azimuthal correlations in the pseudorapidity range j j <0:8are presented as a function of the collision centrality,particle separation in pseudorapidity,and transverse momentum.A clear signal compatible with a charge-dependent separation relative to the reaction plane is observed,which shows little or no collision energy dependence when compared to measurements at RHIC energies.This provides a new insight for under-standing the nature of the charge-dependent azimuthal correlations observed at RHIC and LHC energies.DOI:10.1103/PhysRevLett.110.012301PACS numbers:25.75.Ld,11.30.Er,11.30.Qc,12.38.AwThe possibility to observe parity violation in the strong interaction using relativistic heavy-ion collisions has been discussed for many years [1–3].In quantum chromody-namics (QCD),this symmetry violation originates in the interaction between quarks and topologically nontrivial gluonic fields,instantons,and sphalerons [4].This interac-tion,which is characterized by the topological charge [5],breaks the balance between the number of quarks with different chirality,resulting in a violation of the P and CP symmetry.In [6,7],it was suggested that in the vicinity of the deconfinement phase transition,and under the influ-ence of the strong magnetic field generated by the colliding nuclei,the quark spin alignment along the direction of the angular momentum (i.e.the direction of the magnetic field)and the imbalance of the left-and right-handed quarks,generates an electromagnetic current.The experimental search of these effects has intensified recently,following the realization that the consequent quark fragmentation into charged hadrons results in a charge separation along the direction of the magnetic field,and perpendicular to the reaction plane (the plane of symmetry of a collision defined by the impact parameter vector and the beam direction).This phenomenon is called the chiral magnetic effect (CME).Because of fluctuations in the sign of the topologi-cal charge,the resulting charge separation averaged over many collisions is zero.This makes the observation of the CME possible only via P -even observables,expressed in terms of two-particle and multiparticle correlations.The previous measurement of charge separation by the STAR Collaboration [8]is consistent with the qualitative expec-tations for the CME and has triggered an intense discussion [9–13].A significant source of uncertainty in the theoretical consideration of the CME is related to the expected center-of-mass energy dependence.In [7],the authors argued that the uncertainty in making any quantitative prediction relies on the time integration over which the magnetic field develops and decays.As long as a decon-fined state of matter is formed in a heavy-ion collision,the magnitude of the effect should either not change or should decrease with increasing energy [7].In addition,in [12]it is also suggested that there should be no energy depen-dence between the top RHIC and the LHC energies,based on arguments related to the universality of the underlying physical process,without however explicitly quantifying what the contribution of the different values and time evolution of the magnetic field for different energies will be.On the other hand,in [13]it is argued that the CME should strongly decrease at higher energies,because the magnetic field decays more rapidly.Such spread in the theoretical expectations makes it important to measure the charge-dependent azimuthal correlations at the LHC,where the collision energy is an order of magnitude higher compared to the RHIC.In this Letter we report the measurement of charge-dependent azimuthal correlations at midrapidity in Pb-Pb collisions at the center-of-mass energy per nucleon pair ffiffiffiffiffiffiffiffis NN p ¼2:76TeV by the ALICE Collaboration at the LHC.Azimuthal correlations among particles produced in a heavy-ion collision provide a powerful tool for the experi-mental study of particle production with respect to the reaction plane.They are usually quantified by the aniso-tropic flow coefficients,v n ,in a Fourier decomposition [14].Local violation of parity symmetry may result in the additional P -odd sinus terms [3,8,15]:dNd’ $1þ2X n ½v n; cos ðn Á’ Þþa n; sin ðn Á’ Þ ;(1)where Á’ ¼’ ÀÉRP is the azimuthal angle ’ of the charged particle of type relative to the reaction plane*Full author list given at the end of the article.Published by the American Physical Society under the terms of the Creative Commons Attribution 3.0License .Further distri-bution of this work must maintain attribution to the author(s)and the published article’s title,journal citation,and DOI.angle,ÉRP.The leading order coefficient a1; reflects the magnitude while the higher orders(a n; for n>1)describe the specific shape in azimuth of the effects from local parity violation.We thus employ a multiparticle correlator [15]that probes the magnitude of the a1coefficient,and at the same time suppresses the background correlations unrelated to the reaction plane:h cosð’ þ’ À2ÉRPÞi¼h cosÁ’ cosÁ’ iÀh sinÁ’ sinÁ’ i:(2) The indices and refer to the charge of the particles.The brackets denote an average over the particle pairs within the event as well as an average over the analyzed events.In practice,the reaction plane angle is not known and is esti-mated by constructing the event plane using azimuthal par-ticle distributions.In Eq.(2),the terms h cosÁ’ cosÁ’ i and h sinÁ’ sinÁ’ i quantify the correlations in-and out-of plane,respectively.The latter is sensitive to the charge correlations resulting from the CME:h sinÁ’ sinÁ’ i$ h a1; a1; i.The construction of the correlator in Eq.(2)as the difference between these two contributions suppresses correlations not related to the reaction plane orientation (nonflow).The contribution from the CME to the correla-tions of pairs of particles with same and opposite charge is expected to be similar in magnitude and opposite in sign. This expectation could be further modified by the medium created in a heavy-ion collision,that may result in the dilution of the correlations between particles with opposite sign[6,7].In order to evaluate each of the two terms in Eq.(2),we also measure the two-particle correlator:h cosð’ À’ Þi¼h cosÁ’ cosÁ’ iþh sinÁ’ sinÁ’ i;(3) which in contrast to the correlator in Eq.(2)is independent of the reaction plane angle and susceptible to the large P-even background contributions.The combination of these correlators provides access to both components, h cosÁ’ cosÁ’ i and h sinÁ’ cosÁ’ i,which is impor-tant for detailed comparisons with model calculations.It should be pointed out that both correlators of Eq.(2) and Eq.(3)could be affected by background sources.In [10],it is argued that the effect of momentum conservation influences in a similar way the pairs of particles with opposite and same charge,and could result in a potentially significant correction to both h cosð’ þ’ À2ÉRPÞi and h cosð’ À’ Þi.Also in[10],it was suggested that local charge conservation effects may be responsible for a sig-nificant part of the observed charge dependence of the correlator h cosð’ þ’ À2ÉRPÞi.Recent calculations [16]suggest that the correlator in Eq.(2)may have a negative(i.e.out-of-plane),charge-independent,dipole flow contribution originating fromfluctuations in the initial energy density of a heavy-ion collision.A description of the ALICE detector and its perform-ance can be found in[17,18].For this analysis,the follow-ing detector subsystems were used:the time projection chamber(TPC)[19],the silicon pixel detector(SPD), two forward scintillator arrays(VZERO),and two zero degree calorimeters(ZDC)[17].We analyzed a sample of about13Â106minimum-bias trigger events of Pb-Pb collisions atffiffiffiffiffiffiffiffis NNp¼2:76TeV collected with the ALICE detector.The standard ALICE offline event selection criteria[20]were applied,including a collision vertex cut ofÆ7cm along the beam axis.The collision centrality is estimated from the amplitude mea-sured by the VZERO detectors[17].The data sample is divided into centrality classes which span0%-70%of the hadronic interaction cross section,with the0%-5%class corresponding to the most central(i.e.smaller impact pa-rameter)collisions.Charged particles reconstructed by the TPC are accepted for analysis within j j<0:8and0:2< p T<5:0GeV=c.A set of requirements described in[20] were applied in order to ensure the quality of the tracks but also to reduce the contamination from secondary particles. To evaluate the systematic uncertainties in the analysis, events recorded with two different magneticfield polarities were analyzed leading to an uncertainty that is less than7% for all centrality classes.The cut on the collision vertex was varied fromÆ7cm toÆ10cm from the nominal collision point,with steps of1cm,contributing a maximum of5%to the total uncertainty.A bias due to the centrality determina-tion was studied by using multiplicities measured by the TPC or the SPD,rather than the VZERO,and was found to be less than10%.Contamination due to secondary tracks that do not originate from the collision vertex was reduced by requiring that the distance of closest approach between tracks and the primary vertex is less than2cm.The effect of secondary tracks on the measurement was estimated by varying the cut from2to4cm in steps of0.5cm and was calculated to be below15%.Effects due to nonuniform acceptance of the TPC were estimated to be below2%and are corrected for in the analysis.A significant contribution to the systematic error is coming from the uncertainty in the v2measurement,which is used as an estimate of the reaction plane resolution.The v2 estimate is obtained from the2-and4-particle cumulant analyses[20],which are affected in different ways by non-flow effects andflowfluctuations.For this analysis,v2was taken as the average of the two values,with half of the difference between v2f2g and v2f4g being attributed as the systematic uncertainty.The values of this uncertainty range from9%for the20%–30%centrality to18%(24%)for the 50%–60%(60%–70%)centrality class.The differences in the results from the four independent analysis methods (described below)were also considered as part of the system-atic uncertainty and were estimated to be3%for the 20%–30%and the50%–60%centrality bins and47%for the most peripheral centrality class.The contributions from all effects were added in quadrature to calculate the totalsystematic uncertainty.For the correlation between pairs of particles with the same charge it varies from 19%(28%)for the 20%–30%(50%–60%)centrality up to 55%for the 60%–70%centrality class.The correlations between oppo-site charged particles for 0%–60%centrality and for the same charge pairs for 0%–20%centrality are compatible with zero with a systematic error below 5:5Â10À5.Figure 1(a)presents the centrality dependence of the three-particle correlator,defined in Eq.(2).The correla-tions of the same charge pairs for the positive-positive and negative-negative combinations are found to be consistent within statistical uncertainties and are combined into one set of points,labeled same .The difference between the correlations of pairs with same and opposite charge indi-cates a charge dependence with respect to the reaction plane,as may be expected for the CME.To test the bias from the reaction plane reconstruction,four independent analyses were performed.The first analysis uses a cumu-lant technique [21],whereas for the three other analyses the orientation of the collision symmetry plane is estimated from the azimuthal distribution of charged particles in the TPC,and hits in the forward VZERO and ZDC detectors [22].There is a very good agreement between the results obtained with the event plane estimated from different detectors covering a wide range in pseudorapidity.This allows us to conclude that background sources due to corre-lations not related to the orientation of the reaction plane are negligible,with perhaps the exception of the peripheral collisions for the pairs of particles with opposite charge.Figure 1(b)shows the centrality dependence of the two-particle correlator h cos ð’ À’ Þi ,as defined in Eq.(3),which helps to constrain experimentally the P -even back-ground correlations.The statistical uncertainty is smaller than the symbol size.The two-particle correlations for the same and opposite charge combinations are always posi-tive and exhibit qualitatively similar centrality depen-dence,while the magnitude of the correlation is smaller for the same charged pairs.Our two-particle correlation results differ from those reported by the STARCollaboration for Au-Au collisions at ffiffiffiffiffiffiffiffis NN p ¼200GeV [8]for which negative correlations are observed for the same charged pairs.Figure 1(c)shows the h cosÁ’ cosÁ’ i and h sinÁ’ sinÁ’ i terms separately.For pairs of particles of the same charge,we observe that the h sinÁ’ sinÁ’ i correlations are larger than the h cosÁ’ cosÁ’ i ones.On the other hand,for pairs of opposite charge,the two terms are very close except for the most peripheral collisions.Further interpretation of the results presented in Fig.1(c)in terms of in-and out-of-plane correlations is complicated due to the significant nonflow contribution in h cos ð’ À’ Þi .Figure 2presents the three-particle correlator h cos ð’ þ’ À2ÉRP Þi as a function of the collision centrality com-pared to model calculations and results for RHIC energies.The statistical uncertainties are represented by the errorbars.The shaded area around the points indicates the systematic uncertainty based on the different sources described above.Also shown in Fig.2are STAR results [8].The small difference between the LHC and the RHIC data indicates little or no energy dependence for the three-particle correlator when changing from the collisionenergy of ffiffiffiffiffiffiffiffis NN p ¼0:2TeV to 2.76TeV .In Fig.2,the ALICE data are compared to the expectations from the HIJING model [23].The HIJING results for the three-particle correlations are divided by the experimentally〉)R P Ψ - 2βϕ + αϕ c o s (〈-0.50.5-3〉)βϕ-αϕ c o s (〈00.0020.0040.006centrality, %0.0020.003FIG.1(color online).(a)Centrality dependence of the correla-tor defined in Eq.(2)measured with the cumulant method and from correlations with the reaction plane estimated using the TPC,the ZDC,and the VZERO detectors.Only statistical errors are shown.The points are displaced slightly in the horizontal direction for visibility.(b)Centrality dependence of the two-particle corre-lator defined in Eq.(3)compared to the STAR data [8].The width of the solid red lines indicates the systematic uncertainty of the ALICE measurement.(c)Decomposition of the correlators into h cosÁ’ cosÁ’ i and h sinÁ’ sinÁ’ i terms.The ALICE results in (b)and (c)are obtained with the cumulant method.measured value of v 2(i.e.h cos ð’ þ’ À2’c Þi =v 2f 2g )as reported in [20]due to the absence of collective azimuthal anisotropy in this model.Since the points do not exhibit any significant difference between the correlations of pairs with same and opposite charge,they were averaged in the figure.The correlations from HIJING show a significant increase in the magnitude for very peripheral collisions.This can be attributed to correlations not related to the reaction plane orientation,in particular,from jets [8].The results from ALICE in Fig.2show a strong corre-lation for pairs with the same charge and simultaneously a very weak correlation for the pairs of opposite charge.This difference in the correlation magnitude depending on the charge combination could be interpreted as ‘‘quenching’’of the charge correlations for the case when one of the particles is emitted toward the center of the dense medium created in a heavy-ion collision [6,7].An alternative ex-planation can be provided by a recent suggestion [16]that the value of the charge-independent version of the corre-lator defined in Eq.(2)is dominated by directed flow fluctuations.The sign and the magnitude of these fluctua-tions based on a hydrodynamical model calculation for RHIC energies [16]appear to be very close to the mea-surement.Our results for charge-independent correlations are given by the shaded band in Fig.2.The thick solid line in Fig.2shows a prediction [13]for the same sign correlations due to the CME at LHC ener-gies.The model makes no prediction for the absolute magnitude of the effect and can only describe the energy dependence by taking into account the duration and time evolution of the magnetic field.It predicts a decrease of correlations by about a factor of 5from RHIC to LHC,which would significantly underestimate the observed magnitude of the same sign correlations seen at the LHC.At the same time in [7,12],it was suggested that the CME might have the same magnitude at the LHC and at RHIC energies.Figure 3shows the dependence of the three-particle correlator on the transverse momentum difference,j p T ; Àp T ; j ,the average transverse momentum,ðp T ; þp T ; Þ=2,and the pseudorapidity separation,j À j ,of the pair for the 30%-40%centrality range.The pairs of opposite charge do not show any significant dependence on the pseudorapidity difference,while there is a dependencecentrality, %〉)R P Ψ - 2βϕ + αϕ c o s (〈-0.6-0.4-0.200.20.40.6-3FIG.2(color online).The centrality dependence of the three-particle correlator defined in Eq.(2).The circles indicate the ALICE results obtained from the cumulant analysis.The stars show the STAR data from [8].The triangles represent the three-particle correlations [h cos ð’ þ’ À2’c Þi ]from HIJING [23]corrected for the experimentally measured v 2f 2g [20].Points are displaced horizontally for visibility.A model prediction for the same sign correlations incorporating the chiral magnetic effect for LHC energies [13]is shown by the solid line.The shaded band represents the centrality dependence of the charge-independent correlations.)| (GeV/c T,β - p T,α|p 〉)R P Ψ - 2βϕ + αϕc o s (〈-0.4-0.20.2-3))/2 (GeV/c T,β + p T,α(p |βη - αη = |η∆FIG.3(color online).The three-particle correlator defined in Eq.(2)as a function of (a)the transverse momentum difference,j p T ; Àp T ; j ,(b)the average transverse momentum,ðp T ; þp T ; Þ=2,and (c)the rapidity separation,j À j ,of the charged particle pair of same (closed symbols)and opposite (open symbols)sign.on j p T ; Àp T ; j (stronger)and ðp T ; þp T ; Þ=2(weaker).The correlations for pairs of particles of the same charge show no strong dependence on the p T difference,allowing one to exclude any type of short range correlations (e.g.quantum statistics correlations)as the main source of the effect.In addition,it is seen that the magnitude of the same charge correlations increases with increasing average p T of the pair.This observation is in contradiction to the initial expectations from theory [7]that the effect should originate from low p T particles.The dependence of the correlations on the j À j indicates a width of one unit in pseudor-apidity,beyond which the value of h cos ð’ þ’ À2ÉRP Þi is close to zero up to Á %1:5.Similar results were reported also at RHIC energies [8].At the moment there are no quantitative model calculations of the charge-dependent differential correlations.In summary,we have measured the charge-dependentazimuthal correlations in Pb-Pb collisions at ffiffiffiffiffiffiffiffis NN p ¼2:76TeV at the LHC using the ALICE detector.Both two-and three-particle correlations are reported.A clear signal compatible with a charge-dependent separation rela-tive to the reaction plane is observed.However,our results are not described by the only available quantitative model prediction of the CME for the LHC energy.The lack of realistic model calculations for the centrality and pair differential dependencies based on models incorporating CME and possible background contributions does not allow us to make a firm conclusion regarding the nature of the charge-dependent correlations originally observed at RHIC and now established at the LHC.The observation of a small collision energy dependence of the three-particle correlation and the simultaneous significant change in the two-particle correlations between top RHIC and LHC energies put stringent constraints on models built to interpret such correlations.Analyses of higher harmonic correlations are planned and may yield a better understanding of the com-plex charge-dependent correlations seen at LHC energies.The ALICE Collaboration would like to thank all its engi-neers and technicians for their invaluable contributions to the construction of the experiment and the CERN accelerator teams for the outstanding performance of the LHC complex.The ALICE Collaboration acknowledges the following fund-ing agencies for their support in building and running the ALICE detector:Calouste Gulbenkian Foundation from Lisbon and Swiss Fonds Kidagan,Armenia;ConselhoNacional de Desenvolvimento Cientı´fico e Tecnolo ´gico (CNPq),Financiadora de Estudos e Projetos (FINEP),Fundac ¸a˜o de Amparo a `Pesquisa do Estado de Sa ˜o Paulo (FAPESP);National Natural Science Foundation of China (NSFC),the Chinese Ministry of Education (CMOE),and the Ministry of Science and Technology of China (MSTC);Ministry of Education and Youth of the Czech Republic;Danish Natural Science Research Council,the Carlsberg Foundation,and the Danish National Research Foundation;The European Research Council under the EuropeanCommunity’s Seventh Framework Programme;Helsinki Institute of Physics and the Academy of Finland;French CNRS-IN2P3,the ‘‘Region Pays de Loire,’’‘‘Region Alsace,’’‘‘Region Auvergne,’’and CEA,France;German BMBF and the Helmholtz Association;General Secretariat for Research and Technology,Ministry of Development,Greece;Hungarian OTKA and National Office for Research and Technology (NKTH);Department of Atomic Energy and Department of Science and Technology of the Government of India;Istituto Nazionale di Fisica Nucleare (INFN)of Italy;MEXT Grant-in-Aid for Specially Promoted Research,Japan;Joint Institute for Nuclear Research,Dubna;National Research Foundation of Korea (NRF);CONACYT,DGAPA,Me´xico,ALFA-EC,and the HELEN Program (High-Energy Physics Latin-American–European Network);Stichting voor Fundamenteel Onderzoek der Materie (FOM)and the Nederlandse Organisatie voor Wetenschappelijk Onderzoek (NWO),Netherlands;Research Council of Norway (NFR);Polish Ministry of Science and Higher Education;National Authority forScientific Research-NASR (Autoritatea Nat ¸ionala˘pentru Cercetare S ¸tiint¸ifica ˘-ANCS);Federal Agency of Science of the Ministry of Education and Science of Russian Federation,International Science and Technology Center,Russian Academy of Sciences,Russian Federal Agency of Atomic Energy,Russian Federal Agency for Science and Innovations and CERN-INTAS;Ministry of Education of Slovakia;Department of Science and Technology,South Africa;CIEMAT,EELA,Ministerio de Educacio´n y Ciencia of Spain,Xunta de Galicia (Consellerı´a de Educacio ´n),CEADEN,Cubaenergı´a,Cuba,and IAEA (International Atomic Energy Agency);Swedish Research Council (VR)and Knut &Alice Wallenberg Foundation (KAW);Ukraine Ministry of Education and Science;United Kingdom Science and Technology Facilities Council (STFC);the United States Department of Energy,the United States National Science Foundation,the State of Texas,and the State ofOhio.[1]T.D.Lee,Phys.Rev.D 8,1226(1973);T.D.Lee andG.C.Wick,Phys.Rev.D 9,2291(1974).[2]P.D.Morley and I.A.Schmidt,Z.Phys.C 26,627(1985).[3] D.Kharzeev,R.D.Pisarski,and M.H.G.Tytgat,Phys.Rev.Lett.81,512(1998);D.Kharzeev and R.D.Pisarski,Phys.Rev.D 61,111901(2000).[4]T.Scha¨fer and E.V .Shuryak,Rev.Mod.Phys.70,323(1998).[5]S.S.Chern and J.Simons,Ann.Math.99,48(1974).[6] D.Kharzeev,Phys.Lett.B 633,260(2006);K.Fukushima, D.E.Kharzeev,and H.J.Warringa,Phys.Rev.D 78,074033(2008).[7] D.Kharzeev,L.D.McLerran,and H.J.Warringa,Nucl.Phys.A803,227(2008).[8] B.I.Abelev et al.(STAR Collaboration),Phys.Rev.Lett.103,251601(2009); B.I.Abelev et al.(STAR Collaboration),Phys.Rev.C 81,54908(2010).[9] B.Mu¨ller and A.Scha¨fer,Phys.Rev.C82,057902(2010).[10]S.Schlichting and S.Pratt,Phys.Rev.C83,014913(2011);S.Pratt,S.Schlichting,and S.Gavin,Phys.Rev.C84,024909(2011).[11] A.Bzdak,V.Koch,and J.Liao,Phys.Rev.C81,031901(2010);A.Bzdak,V.Koch,and J.Liao,Phys.Rev.C83, 014905(2011).[12] A.R.Zhitnitsky,Nucl.Phys.A853,135(2011);A.R.Zhitnitsky,Nucl.Phys.A886,17(2012).[13]V.D.Toneev and V.V oronyuk,arXiv:1012.1508.[14]S.V oloshin and Y.Zhang,Z.Phys.C70,665(1996);A.M.Poskanzer and S.A.V oloshin,Phys.Rev.C58,1671 (1998).[15]S.A.V oloshin,Phys.Rev.C70,057901(2004).[16] D.Teaney and L.Yan,Phys.Rev.C83,064904(2011).[17]K.Aamodt et al.(ALICE Collaboration),JINST3,S08002(2008).[18]K.Aamodt et al.(ALICE Collaboration),J.Phys.G30,1517(2004);K.Aamodt et al.(ALICE Collaboration), J.Phys.G32,1295(2006).[19]J.Alme et al.(ALICE Collaboration),Nucl.Instrum.Methods Phys.Res.,Sect.A622,316(2010).[20]K.Aamodt et al.(ALICE Collaboration),Phys.Rev.Lett.105,252302(2010).[21] A.Bilandzic,R.Snellings,and S.V oloshin,Phys.Rev.C83,044913(2011).[22]S.V oloshin, A.M.Poskanzer,and R.Snellings,inRelativistic Heavy Ion Physics,Landolt-Bornstein V ol.1 (Springer-Verlag,Berlin,2010),pp.554.[23]M.Gyulassy and X.N.Wang,mun.83,307(1994);X.N.Wang and M.Gyulassy,Phys.Rev.D44,3501(1991).B.Abelev,1J.Adam,2D.Adamova´,3A.M.Adare,4M.M.Aggarwal,5G.Aglieri Rinella,6A.G.Agocs,7 A.Agostinelli,8S.Aguilar Salazar,9Z.Ahammed,10N.Ahmad,11A.Ahmad Masoodi,11S.A.Ahn,12S.U.Ahn,13A.Akindinov,14D.Aleksandrov,15B.Alessandro,16R.Alfaro Molina,9A.Alici,17,18A.Alkin,19E.Almara´z Avin˜a,9J.Alme,20T.Alt,21V.Altini,22S.Altinpinar,23I.Altsybeev,24C.Andrei,25A.Andronic,26V.Anguelov,27J.Anielski,28C.Anson,29T.Anticˇic´,30F.Antinori,31P.Antonioli,17L.Aphecetche,32H.Appelsha¨user,33N.Arbor,34S.Arcelli,8A.Arend,33N.Armesto,35R.Arnaldi,16T.Aronsson,4I.C.Arsene,26 M.Arslandok,33A.Asryan,24A.Augustinus,6R.Averbeck,26T.C.Awes,36J.A¨ysto¨,37M.D.Azmi,11M.Bach,21A.Badala`,38Y.W.Baek,39,13R.Bailhache,33R.Bala,16R.Baldini Ferroli,18A.Baldisseri,40A.Baldit,39F.Baltasar Dos Santos Pedrosa,6J.Ba´n,41R.C.Baral,42R.Barbera,43F.Barile,22G.G.Barnafo¨ldi,7L.S.Barnby,44V.Barret,39J.Bartke,45M.Basile,8N.Bastid,39S.Basu,10B.Bathen,28G.Batigne,32B.Batyunya,46C.Baumann,33I.G.Bearden,47H.Beck,33I.Belikov,48F.Bellini,8R.Bellwied,49E.Belmont-Moreno,9G.Bencedi,7S.Beole,50I.Berceanu,25A.Bercuci,25Y.Berdnikov,51D.Berenyi,7 A.A.E.Bergognon,32D.Berzano,16L.Betev,6A.Bhasin,52A.K.Bhati,5J.Bhom,53L.Bianchi,50N.Bianchi,54 C.Bianchin,55J.Bielcˇı´k,2J.Bielcˇı´kova´,3A.Bilandzic,56,47S.Bjelogrlic,57F.Blanco,58F.Blanco,49D.Blau,15C.Blume,33M.Boccioli,6N.Bock,29S.Bo¨ttger,59A.Bogdanov,60H.Bøggild,47M.Bogolyubsky,61L.Boldizsa´r,7M.Bombara,62J.Book,33H.Borel,40A.Borissov,63S.Bose,64F.Bossu´,50M.Botje,56B.Boyer,65E.Braidot,66P.Braun-Munzinger,26M.Bregant,32T.Breitner,59T.A.Browning,67M.Broz,68R.Brun,6E.Bruna,50,16G.E.Bruno,22D.Budnikov,69H.Buesching,33S.Bufalino,50,16K.Bugaiev,19O.Busch,27 Z.Buthelezi,70D.Caballero Orduna,4D.Caffarri,55X.Cai,71H.Caines,4E.Calvo Villar,72P.Camerini,73 V.Canoa Roman,74G.Cara Romeo,17W.Carena,6F.Carena,6N.Carlin Filho,75F.Carminati,6A.Casanova Dı´az,54J.Castillo Castellanos,40J.F.Castillo Hernandez,26E.A.R.Casula,76V.Catanescu,25 C.Cavicchioli,6C.Ceballos Sanchez,77J.Cepila,2P.Cerello,16B.Chang,37,78S.Chapeland,6J.L.Charvet,40S.Chattopadhyay,10S.Chattopadhyay,64I.Chawla,5M.Cherney,79C.Cheshkov,6,80B.Cheynis,80V.Chibante Barroso,6D.D.Chinellato,81P.Chochula,6M.Chojnacki,57S.Choudhury,10P.Christakoglou,56 C.H.Christensen,47P.Christiansen,82T.Chujo,53S.U.Chung,83C.Cicalo,84L.Cifarelli,8,6,18F.Cindolo,17J.Cleymans,70F.Coccetti,18F.Colamaria,22D.Colella,22G.Conesa Balbastre,34Z.Conesa del Valle,6 P.Constantin,27G.Contin,73J.G.Contreras,74T.M.Cormier,63Y.Corrales Morales,50P.Cortese,85I.Corte´s Maldonado,86M.R.Cosentino,66F.Costa,6M.E.Cotallo,58E.Crescio,74P.Crochet,39E.Cruz Alaniz,9E.Cuautle,87L.Cunqueiro,54A.Dainese,55,31H.H.Dalsgaard,47A.Danu,88D.Das,64K.Das,64I.Das,65S.Dash,89A.Dash,81S.De,10G.O.V.de Barros,75A.De Caro,90,18G.de Cataldo,91J.de Cuveland,21A.De Falco,76D.De Gruttola,90H.Delagrange,32A.Deloff,92V.Demanov,69N.De Marco,16E.De´nes,7 S.De Pasquale,90A.Deppman,75G.D.Erasmo,22R.de Rooij,57M.A.Diaz Corchero,58D.Di Bari,22T.Dietel,28C.Di Giglio,22S.Di Liberto,93A.Di Mauro,6P.Di Nezza,54R.Divia`,6Ø.Djuvsland,23A.Dobrin,63,82T.Dobrowolski,92I.Domı´nguez,87B.Do¨nigus,26O.Dordic,94O.Driga,32A.K.Dubey,10A.Dubla,57L.Ducroux,80P.Dupieux,39M.R.Dutta Majumdar,10A.K.Dutta Majumdar,64D.Elia,91D.Emschermann,28。
原子核物理——气体探测器发展

多漂移单元模块MDM
MDM (Multidrift Module)
顶点探测器位于最内部,维修难。不仅要求定位精度高和计数率限高, 还要求高可靠性、抗辐射性能强。
一、结构 • 单元:半径 1.5mm
1+6; 31m的不 锈钢丝性能最好 • 模块:70个单元包在 30mm的六角形碳纤 维管内。管子作为机械 支撑、气体密封。 • 每根径迹30次取样
1
空间丝上带电粒子的径迹坐标就由丝上雪崩 在感应片上感应信号的重心所决定。 (100m)
•
• 漂移时间给出z向的位置。 (300m)
• 两者结合,给出带电粒子的三维坐标。
2
二、PEP-4的高压TPC
圆柱半径1m,长2m。 圆柱中央有一加负高压 (-150kV)的电极将圆柱 分为左右对称的两半。 圆柱的两端面是栅丝平面、 阳极丝平面(包括灵敏丝和 场丝)和阴极感应平面。 磁场B=1.5T,与电场平行, 测动量,减小漂移电子的横 向扩散。
微条气体室MSGC
MSGC (MicroStrip Gas Chamber)
一、结构和工作原理
在绝缘或半绝缘基质板 上通过蚀刻而成的两组细 金属条: 阳极条(5-10m), 电场条(50-100 m)
产生雪崩倍增的阳极和 阴极之间的距离大大缩短
21
二、性能
空间分辨: 30 m,双径迹分辨400 m。
17
二、性能
• 空间分辨率:100m
• 双径迹分辨: ~4mm,由管径决定
• 单元死时间: ~40ns • 机械性能好,不怕断丝 • 进一步减小管子的直径、增加长度是对制作工 艺的挑战。
18
MWPC和漂移室是具有代表性的气体探测器。不仅在 粒子物理实验中得到广泛的应用,且在X射线晶体学、 生物医学和天体物理等领域发挥重要作用。
TW 117设计学中英对照术语
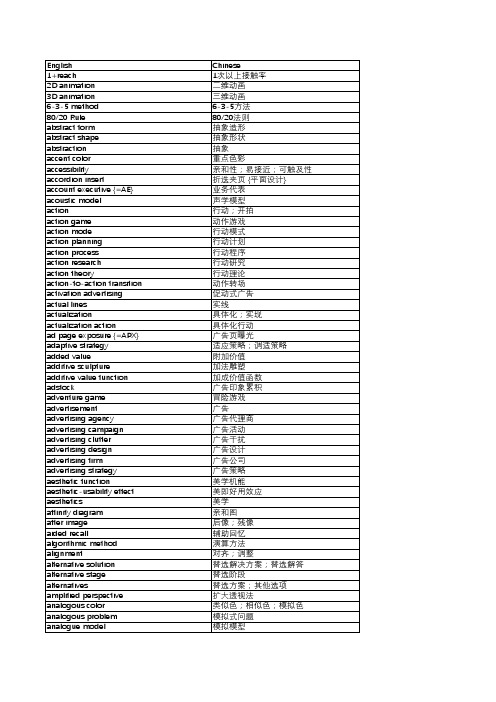
Chinese 1次以上接触率 二维动画 三维动画 6-3-5方法 80/20法则 抽象造形 抽象形状 抽象 重点色彩 亲和性;易接近;可触及性 折迭夹页 {平面设计} 业务代表 声学模型 行动;开拍 动作游戏 行动模式 行动计划 行动程序 行动研究 行动理论 动作转场 促动式广告 实线 具体化;实现 具体化行动 广告页曝光 适应策略;调适策略 附加价值 加法雕塑 加成价值函数 广告印象累积 冒险游戏 广告 广告代理商 广告活动 广告干扰 广告设计 广告公司 广告策略 美学机能 美即好用效应 美学 亲和图 后像;残像 辅助回忆 演算方法 对齐;调整 替选解决方案;替选解答 替选阶段 替选方案;其他选项 扩大透视法 类似色;相似色;模拟色 模拟式问题 模拟模型
模拟;模拟法 分析 辅助角色 取镜角度 动画 人体计测学 应用程序 外观;外貌;表象 应用艺术 原型;原形 建筑设计 建筑 艺术;艺术作品 艺术指导;艺术导演;艺术总监 器物分析;文物分析 人工智能;人工智能 旁白 组装图;组合图 装配时间 助理创意总监 联想方法 联想理论 非对称平衡 非对称互补 大气透视法;空气透视法 吸引力 属性表 属性模拟 观众;阅听众 阅听众累计 阅听众组成 阅听众流量 阅听分众 音响;声音 视听觉 扩增实境 授权 自动优化 前卫;前卫风格 化身 背景 背景故事 平衡 频带;乐队 带宽;带宽 横幅{网络广告} 条形码 基本概念 批次 行为 行为识别 行为模型;行为模式 行为对映 行为科学 标竿
Hale Waihona Puke cognition cognitive dissonance cohesive strength collaborate design collaborative design collage collateral material color associations color chart color contrast color correction color filter color forecasting color harmony color intensity color management color marketing color marketing group color match color scale color scheme color sensation color separation color system color temperature color value color wheel combinatorial explosion comic comic supplement command line commercial commercial audience commercial film {=CF} commercial impression commercial pool commercial time common language communication communication design communication skill community community relations company policy compatibility compatible solution competing product analysis competitor analysis complementary color completeness complexity component composition comprehensive designing compression
英文对照(摄影类)
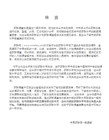
focusing hood 对焦遮光罩
focusing mount 对焦接环
focusing range 对焦范围
focusing ring 对焦环
focusing scale 对焦刻度
focusing screen 对焦屏
focusing 对焦定位片
hard water 硬水
hard(paper) 反差高(相纸)
hardener 坚膜药
hardening bath 坚膜液
hardening fixer 坚膜定影药
haze 雾气
haze filter 雾化滤光片
heat absorbing filter 吸热滤片
heat filter 隔热片
filter factor 滤光片曝光系数
filter holder 滤光片夹
filter turrets 滤片圆盘,安装三个以上的滤光片在一个圆盘中
fine grain 幼微粒
fine grain developer 幼微粒颜影药
fisheye lens 鱼眼镜头 (取景角度达180度)
focal plane 焦点平面
focal plane shutter 焦点平面快门
focal poing 【光】聚焦点
foci 焦点focus 的众数
focus 焦点
focus extension 镜头对焦伸长度,亦称lens extension
focusing 对焦
focusing adaptor 对焦附加器
Fourier techniques 傅里叶法
Fourier transformation 傅里叶换式
衍生工具远期合同会计处理方法

衍生工具远期合同会计处理方法英文回答:Derivative instruments, such as forward contracts, are financial contracts whose value is derived from an underlying asset or benchmark. These instruments are commonly used for hedging purposes or for speculative trading.The accounting treatment for forward contracts depends on their classification as either a cash flow hedge or a fair value hedge.For cash flow hedges, any changes in the fair value of the forward contract are initially recognized in other comprehensive income (OCI) and later reclassified to the income statement when the hedged transaction affects the income statement. The gain or loss on the forward contract is recognized in the income statement in the same period as the hedged item.For fair value hedges, any changes in the fair value of the forward contract are recognized in the income statement. The gain or loss on the forward contract is recognized in the income statement along with the gain or loss on the hedged item.In both cases, if the forward contract is notdesignated as a hedge or does not qualify for hedge accounting, any changes in its fair value are recognized in the income statement.Furthermore, when a forward contract is initially recognized, it is measured at fair value. Subsequent measurement depends on whether the contract is designatedas a fair value hedge or a cash flow hedge. If designatedas a fair value hedge, the contract is measured at fair value with changes recognized in the income statement. If designated as a cash flow hedge, the contract is measuredat fair value with changes recognized in OCI.Overall, the accounting treatment for forward contractsinvolves recognizing changes in fair value in either the income statement or OCI, depending on the classification as a cash flow hedge or a fair value hedge.中文回答:衍生工具远期合同的会计处理方法取决于其被分类为现金流量套期保值还是公允价值套期保值。
5-5半导体探测器的应用

å S µZ
i
2
Front-end electronics
p-side
n-side
VA64hdr
10 VAs on the p-side (Y direction) 6 VAs on the n-side (X direction)
Each VA reads 64 channels
• Each VA produces a signal with different characteristics • In particular differences in the gain are observed • FEE response curve is deliberately non-linear, different for p and n
2012/11/14
12
CMS硅微条径迹探测器
• 每个花瓣安装17-28个 不等的硅探测器
2012/11/14
Байду номын сангаас
中国科大 汪晓莲
13
五、在空间物理和宇宙线实验中的应用
1. AMS
丁肇中先生领导的AMS组, 计划 把磁谱仪AMS(Alpha Magnetic Spectromenter) 送到国际空间站 ISSA (International Space Station Alpha), 在宇宙线中寻找反物质和 暗物质。AMS 的中间核心部分的 多层径迹室都是采用双边读出的 硅微条探测器。谱仪的体积不大 (直径和高才1m多) , 但这些精密 的径迹探测器与谱仪中的永久磁 铁、飞行时间计数器、契伦科夫 探测器、量能器等紧密配合, 可能 会为天体物理和宇宙线科学作出 非常卓越的贡献。
每一片SVXIIb就有128 路读出。
Chamber
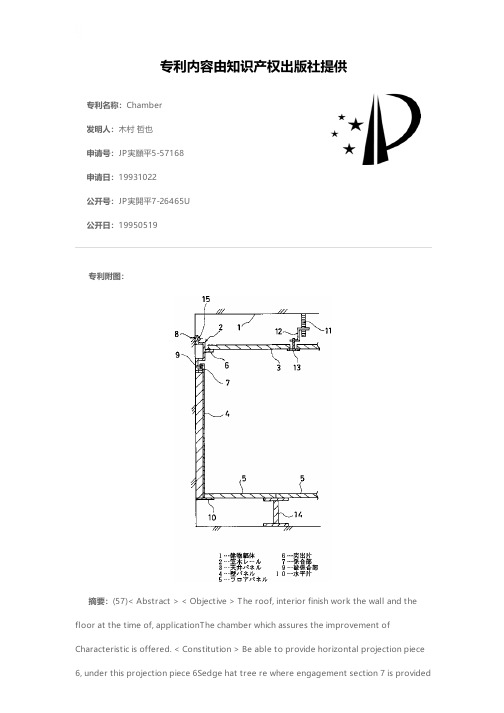
专利名称:Chamber发明人:木村 哲也申请号:JP実願平5-57168申请日:19931022公开号:JP実開平7-26465U公开日:19950519专利内容由知识产权出版社提供专利附图:摘要:(57)< Abstract > < Objective > The roof, interior finish work the wall and the floor at the time of, applicationThe chamber which assures the improvement ofCharacteristic is offered. < Constitution > Be able to provide horizontal projection piece 6, under this projection piece 6Sedge hat tree re where engagement section 7 is providedon side and is locked to building body body 1The roof panel 3 where the edge is mounted in ru 2 and projection piece 6With, be able to provide the engaged portion 9 which is engaged in engagement section 7 on top, the waterThe wall panel 4 where it can provide flat piece 10 in lower part and horizontal piece 10It had with the floor panel 5 where the edge is mounted.申请人:松下電工株式会社地址:大阪府門真市大字門真1048番地国籍:JP代理人:宮井 暎夫更多信息请下载全文后查看。
CLEANROOM PROJECT 3 Evaluating baseline recipes fo
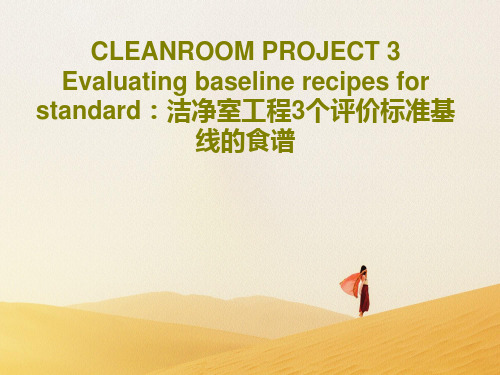
8. Evaluate sufficient development of each wafer by checking its profile using the P15 profilometer or Alpha Step 500.
CLEANROOM PROJECT 3 Evaluating baseline recipes for standard:洁净室工程3个评价标准基
线的食谱
16、云无心以出岫,鸟倦飞而知还。 17、童孺纵行歌,斑白欢游诣。 18、福不虚至,祸不易来。 19、久在樊笼里,复得返自然。 20、羁鸟恋旧林,池鱼思故渊。
4. Obtain a post-etch profile of each wafer using P15 Profilometer or the Alpha Step 500 after stripping the sample of its photoresist using 1165 Remover and use the data obtained to determine the etch rate for each process.
3. Place the wafer in the center of the chamber for the Adv. Vac or the front right position of the PT RIE (for consistency purposes only), and run the standard etching recipe for the specified time using the appropriate etching tool.
- 1、下载文档前请自行甄别文档内容的完整性,平台不提供额外的编辑、内容补充、找答案等附加服务。
- 2、"仅部分预览"的文档,不可在线预览部分如存在完整性等问题,可反馈申请退款(可完整预览的文档不适用该条件!)。
- 3、如文档侵犯您的权益,请联系客服反馈,我们会尽快为您处理(人工客服工作时间:9:00-18:30)。
a r X i v :n u c l -e x /0211014v 1 15 N o v 2002The Forward Time Projection Chamber (FTPC)inSTARK.H.Ackermann 5,F.Bieser 4,F.P.Brady 2,D.Cebra 2,J.E.Draper 2,V .Eckardt 5,T.Eggert 5,H.Fessler 5,K.J.Foley 1,V .Ghazikhanian 3,T.J.Hallman 1,M.Heffner 2,H.H¨u mmler 5,J.Klay 2,S.R.Klein 4,A.Lebedev 1,M.J.LeVine 1,T.Ljubicic 1,G.Lo Curto 5,R.S.Longacre 1,M.Oldenburg 5,HG.Ritter 4,J.L.Romero 2,N.Schmitz 5,A.Sch¨u ttauf 5,J.Seyboth 5,P.Seyboth 5,M.Vidal 5,C.Whitten Jr.3,E.Yamamoto 31BrookhavenNational Laboratory,Upton,New York 11973,USA 2University of California,Davis,California 95616,USA3University of California,Los Angeles,California 90095,USA4Lawrence Berkeley National Laboratory,Berkeley,California 94720,USA5Max-Planck-Institut f¨u r Physik,80805M¨u nchen,Germany1IntroductionThe Forward Time Projection Cham-bers (FTPC)were constructed to ex-tend the acceptance of the STAR ex-periment [1].They cover the pseu-dorapidity range of 2.5<|η|<4.0on both sides of STAR and measure momenta and production rates of posi-tively and negatively charged particles as well as neutral strange particles.Also,due to the high multiplicity,ap-proximately 1000charged particles ina central Au+Au collision,event-by-event observables like p T ,fluctua-tions of charged particle multiplicity and collective flow anisotropies can be studied.The increased acceptance improves the general event character-ization in STAR and allows the study of asymmetric systems like p+A col-lisions.The design and construction was carried out by the group from MPI Munich with contributions from LBNL Berkeley,BNL Brookhaven,UC Davis,UCLA Los Angeles,and MEPhI Moscow [1,2].2Detector Design2.1Conceptual DesignThe FTPC concept was determined mainly by two considerations:Firstly by the high particle density with tracks under small angles with respect to the beam direction and secondly by the restricted available space inside the TPC[3],where the FTPCs are located. In Fig.1thefinal design is shown. It is a cylindrical structure,75cm in diameter and120cm long,with a ra-dial driftfield and readout chambers located in5rings on the outer cylinder surface.Each ring has two padrows and is subdivided azimuthally into 6readout chambers.The radial drift configuration was chosen to improve the two-track separation in the region close to the beam pipe where the par-ticle density is highest.Thefield cage is formed by the inner HV-electrode, a thin metalized plastic tube,and the outer cylinder wall at ground poten-tial.Thefield region at both ends is closed by a planar structure of con-centric rings,made of thin aluminum pipes.The front end electronics(FEE), which amplifies,shapes,and digitizes the signals,is mounted on the back of the readout chambers.Each particle trajectory is sampled up to10times. The ionization electrons are drifted to the anode sense wires and induced signals on the adjacent cathode sur-face are read out by9600pads(each 1.6×20mm2).The above design has some unusual and new features for a TPC:•The electrons drift in a radial elec-tricalfield perpendicular to thesolenoidal magneticfield.•Curved readout chambers are used to keep the radialfield as ideal aspossible.•A two-track separation of1-2mm is expected,which is an order of mag-nitude better than in all previously built TPCs with pad readout.To meet these requirements a R+D pro-gram was initiated,including the se-lection of the most suitable gas mix-ture,the development of the fabrica-tion technology for the curved readoutchambers,and the optimization of thewire and pad geometry for the readoutchambers.2.2Selection of Gas MixtureDue to the short drift length of only23cm a cool gas mixture with CO2orDME can be used.It has a low diffusioncoefficient for electrons and a smallLorentz angle[4].After extensivemeasurements an Ar/CO2(50%/50%)mixture was selected which is non-flammable,shows no or little ageingeffect in comparison to hydrocarbonsand is chemically less agressive thana mixture with DME.Fig.2showsthe measurements of drift time,clus-ter sizes,deflection angle due to theLorentz force in the magneticfield andtwo track resolution for the Ar/CO2gas mixture[1].2.3Readout ChambersIn a conventional TPC the anode(am-plification)wires are orthogonal toFig.1.Schematic diagram of an FTPC for the STAR experimentthe axial direction of the pads.This is impossible in the case of a curved readout chamber.The wires can not be parallel to the pads and therefore to the cylinder axis either,because focusing effects then lead to periodic shifts in the position measurement. This is demonstrated infig.3.How-ever,if two or more wires cross the pad under a small angle this effect al-ready vanishes.For the FTPC design an angle of17.40was chosen resulting in three wires crossing each pad for the selected pad-wire geometry.The anode wires arefirst glued on theflat pad plate with conductive epoxy.Af-terwards the plate is bent between3 rollers to thefinal curvature without breaking the wires.A complete read-out chamber with2padrows is shown infig.4.With only1.5mm distance between the anode wires and the pad plane the spread of the signal(the so-called Pad Response Function)is of similar narrow width.This together with the low electron diffusion and the radial drift principle results in the required2-track separation of about1 mm as can be seen infig.5.2.4Readout ElectronicsThe two FTPCs have19,200chan-nels of electronics,capable of measur-ing the charge drifting to the readout chambers in short time samples.The drift time of about50µs for the23cm maximum drift length is subdivided into256time bins.Because of the slow drift gas and the resulting long dura-tion of the collection of the electron cloud from a track crossing a shaping time of350ns is used.The sampling102030405010203012345671020300.20.40.60.811.2102030FTPC: Ar-CO 2(50%) in E →⊥B →(V c =10kV, B=5kG, L pad =2cm)a) Drift time: T d (µs)r (cm)b) Deflection angle: Ψd (deg)r (cm)c) Cluster widths:σtim (µs) (solid)(τshp =0.20µs)σpad (mm) (dash)(σprf =0.6mm)r (cm)d) Two-track resolution (3σ):σr (mm) (solid)σφ(mm) (dash)r (cm)123456789102030Fig.2.Electron cluster properties as a function of the radial position of the track hit in the FTPC.(a)drift time;(b)deflection angle;(c)cluster widths in time (µs,solid line)and pad (mm,dashed line)directions;(d)two-track resolution (3σ)in the radial (solid line)and azimuthal (dashed line)directions.rate is 5MHz.The design of the front end electronics closely follows that of the central TPC [5].Each pad is read out by a low-noise STAR preampli-fier/shaper (SAS),which sends signals to a switched capacitor array/ADC chip (SCA/ADC).Four of these chip sets,handling 64channels,are mounted on a small FEE card,which is posi-tioned directly on the detector,paral-lel to the readout chambers.Fifteen FEE cards are read out by a readout board,which sends the signals via a 1.2Gbit/s fiber-optic link to the data acquisition system.The readout board also controls the FEE cards,utilising signals from the clock and trigger dis-tribution system,and the slow controlFig.4.Photograph of a FTPC readout chamber.The bending radius is305mm and each of the two padrows has160pads.Fig.3.Measured position of a laser beam for two crossing angles between the an-ode wires and the pad axis.For0o(top) systematic shifts due to the wire structure are observed,which disappear for9o angle (bottom).links.For maintaining the proper oper-ating temperature the FEE and readout boards are water cooled using a leak-12345010203040r [ cm ]λφ[mm]Fig. 5.Measured2-track resolution in the prototype RTPC(data points)and ex-pected reolution in the FTPC(line)as a function of the radial distance r from the beam axis.less,low pressure circulation system [9].The FTPCs are remotely operated through a VME based supervision sys-tem.Data logging and visualisation are performed by a software package de-veloped within the EPICS mainframe [10].2.5Laser Calibration SystemA laser calibration system serves the following primary purposes:•Provides straight ionized tracks of known position to infer corrections for spatial distortions caused by me-chanical or driftfield imperfections.•Helps to calibrate the drift velocity in the non-uniform radial driftfield.•Tests the detector independent of collider operation.The design of this system,similar to the STAR-TPC laser system[6],uses a frequency quadrupled Nd:YAG laser to provide a UV(266nm)beam.The beam is expanded to30mm,split and transported via mirrors to the two FTPC detectors.Remote angle con-trol of two mirrors in each path plus a CCD readout maintains precise steer-ing of the two beams to the detectors. At the detector the beam is subdivided with pickoff mirrors into3,8mm beams which pass into the gas volume through fused silica windows.Each of these beams are further split with smaller pickoff mirrors into5,1mm beams,producing a total of15fidu-cial,ionizing beams distributed in the active volume.3Simulation and Reconstruction of Experimental DataThefirst step in the reconstruction of tracks is to calculate the track points (clusterfinding)from the charge distri-bution measured by the readout elec-tronics.In a second step(trackfinding),these track points are grouped to tracks. Using the magneticfield map,the up to ten position measurements per track are then used tofit the momentum. 3.1Cluster FindingThe reconstruction of track points is done by the FTPC clusterfinding pro-gram[7].It is optimized to deal with high track densities while minimiz-ing the use of computing time.The program reads in the electronic signal data from the data acquisition sys-tem,looks for areas of nonzero charge (cluster),deconvolutes clusters andfits the point coordinates.The transforma-tion from pad position and drift time into cartesian coordinates includes the correction of distortions introduced by the magneticfield.For a typical central Au+Au collision with1000particles in both FTPCs and an occupancy of 25%in the inner region the program needs about2seconds on a930MHz Intel PentiumII processor.3.2Track ReconstructionThe second step in the analysis of FTPC data is the reconstruction of the particle tracks and their momenta. The FTPC track reconstruction code is based on an algorithm developed for fast online reconstruction[8].It is a conventional track-following algo-rithm optimized for minimum use of computing power.In this code all po-sition calculations are done in a trans-formed coordinate system in which points appear on a straight line if theyFig.6.Reconstructed tracks in the FTPCsfrom a Au+Au collision at√s NN=200GeV simulated withthree different models.Fig.8.Distributions of the temperature pa-rameter T reconstructed event-by-event inthe FTPCs for three different event gener-ators.follow the p-¯p distribution and char-acterise the baryon stopping in the re-action.Fig.8shows histograms of theeffective temperature as determinedevent-by-event.Such measurementswill be used to study and search forfluctuations of event properties and toselect special event classes.5SummaryBased on the prototype measurementsand simulations one expects to obtaina position resolution of100µm,a two-Fig.9.One FTPC installed inside the STAR detector track-separation of1mm,a momentumresolution between12and15%,andan overall reconstruction efficiency be-tween70and80%.A summary of the main characteris-tics of the FTPCs is given in table1.The completed and tested FTPCs havebeen installed in the STAR experimentforfirst data taking during summer andfall of2001.One of them can be seenmounted inside the STAR detector inFig.9.Table1FTPC parametersPARAMETER V ALUErows per TPC10pads per sector2×160outer radius30.5cmacceptance2.5<|η|<4.0(2.0o<θ<9.3o) drift electricalfield240-1400V/cm(radial)Gasgas mixture Ar(50%)-CO2(50%) trans.diffusion D T100-130µm/√long.diffusion D L100-130µm/√Lorentz angle4deg.(at0.5T)Readout#of pads19200pad pitch 1.9mmanodewire–pad gap 1.5mmSCA time bin size218nsReferences[1] F.Bieser et al.,The Forward TimeProjection Chamber for the STARDetector,MPI PhE/98-3,1998 [2] A.Sch¨u ttauf et al.,A Forward TPCfor STAR,Nucl.Phys.A661(1999)677c[3]H.Wieman et al.,STAR TPC atRHIC,IEEE Trans.Nucl.Sci.44(1997)671[4]X.Bittl et al.,Diffusion and DriftStudies of Ar-DME/CO2/CH4GasMixtures for a radial TPC in the E×BField,Nucl.Instr.Meth.A398(1997)249[5]S.Klein et al.,Front-End Electronicsfor the STAR TPC,IEEE Trans.Nucl.Sci.43(1996)1768[6]M.Alyushin et al.,Laser CalibarationSystem for STAR TPC,IEEENucl.Sc.Symp.Conference Record96CH35974(1996),Proceedings of the9th ViennaConference on Intrumentation,Nucl.Instr.Meth.in print[7]H.H¨u mmler,Doctorate Thesis,Technical University,M¨u nchen,Germany,2000,MPI PhE/2001-042001,H.H¨u mmler,Simulationand Reconstruction of Data from theSTAR FTPC,STAR Note SN0429(2000)[8]P.Yepes,A fast track patternrecognition,Nucl.Instr.Meth.A380(1996)582M.Oldenburg,Doctorate Thesis,Technical University,M¨u nchen,Germany,2001,in preparation [9]M.Bosteels,Cern internal report,CERN/LHCC/99-33(1999)[10]A.J.Kozubal et al.,ICALEPS89Proceedings,288,(Vancouver,1989)。