Abstract Semi-Immersive Space Mission Design and Visualization Case Study of the “Terrestr
科技发展宇航员的作文英语
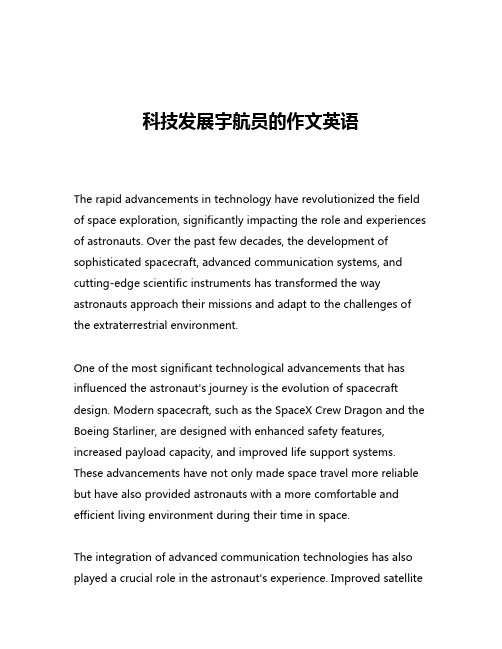
科技发展宇航员的作文英语The rapid advancements in technology have revolutionized the field of space exploration, significantly impacting the role and experiences of astronauts. Over the past few decades, the development of sophisticated spacecraft, advanced communication systems, and cutting-edge scientific instruments has transformed the way astronauts approach their missions and adapt to the challenges of the extraterrestrial environment.One of the most significant technological advancements that has influenced the astronaut's journey is the evolution of spacecraft design. Modern spacecraft, such as the SpaceX Crew Dragon and the Boeing Starliner, are designed with enhanced safety features, increased payload capacity, and improved life support systems. These advancements have not only made space travel more reliable but have also provided astronauts with a more comfortable and efficient living environment during their time in space.The integration of advanced communication technologies has also played a crucial role in the astronaut's experience. Improved satellitenetworks, high-speed data transmissions, and real-time video conferencing have enabled astronauts to maintain constant communication with mission control and their families on Earth. This connectivity has not only enhanced the astronauts' sense of support and well-being but has also facilitated the exchange of critical information and the coordination of mission-critical tasks.Furthermore, the development of scientific instruments and equipment has transformed the way astronauts conduct research and experiments in the microgravity environment of space. Advanced telescopes, spectrometers, and other specialized instruments have enabled astronauts to make groundbreaking discoveries and gain a deeper understanding of the universe. Additionally, the integration of robotic systems and autonomous technologies has allowed astronauts to focus on more complex and specialized tasks, reducing the workload and enhancing the efficiency of their missions.One of the most remarkable technological advancements that has impacted astronauts is the field of life support systems. The development of sophisticated life support systems, including air purification, water recycling, and temperature regulation, has significantly improved the astronauts' ability to survive and thrive in the harsh conditions of space. These systems not only ensure the astronauts' physical well-being but also contribute to theirpsychological and emotional well-being by providing a more comfortable and familiar living environment.The impact of technological advancements on astronauts extends beyond the physical aspects of space exploration. The availability of advanced medical technologies, such as telemedicine and remote diagnostics, has enabled astronauts to receive prompt and effective medical care even in the most remote and challenging environments. This has improved the overall health and safety of astronauts, reducing the risks associated with long-duration spaceflight.Moreover, the integration of virtual reality (VR) and augmented reality (AR) technologies has transformed the training and preparation of astronauts. These immersive technologies allow astronauts to simulate various mission scenarios, practice emergency procedures, and familiarize themselves with the layout of spacecraft and space stations. This not only enhances the astronauts' skills and confidence but also reduces the risk of errors during actual missions.As the pace of technological advancement continues to accelerate, the future of space exploration holds even more promising possibilities for astronauts. Emerging technologies, such as artificial intelligence, robotics, and advanced propulsion systems, are poised to further revolutionize the astronaut's experience. These advancements may lead to more efficient mission planning,autonomous systems that can assist astronauts, and propulsion technologies that can reduce travel times and expand the reach of space exploration.In conclusion, the impact of technological advancements on astronauts has been profound and multifaceted. From the design of spacecraft and life support systems to the integration of advanced communication and scientific instruments, these advancements have transformed the way astronauts approach their missions and adapt to the challenges of the extraterrestrial environment. As space exploration continues to evolve, the role of technology in shaping the astronaut's experience will only become more crucial, paving the way for even greater achievements and discoveries in the vast expanse of the cosmos.。
哈工大论文撰写规范060912
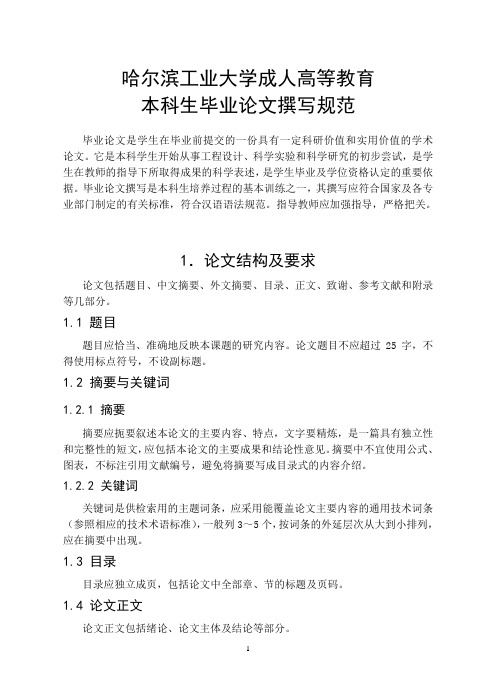
哈尔滨工业大学成人高等教育本科生毕业论文撰写规范毕业论文是学生在毕业前提交的一份具有一定科研价值和实用价值的学术论文。
它是本科学生开始从事工程设计、科学实验和科学研究的初步尝试,是学生在教师的指导下所取得成果的科学表述,是学生毕业及学位资格认定的重要依据。
毕业论文撰写是本科生培养过程的基本训练之一,其撰写应符合国家及各专业部门制定的有关标准,符合汉语语法规范。
指导教师应加强指导,严格把关。
1.论文结构及要求论文包括题目、中文摘要、外文摘要、目录、正文、致谢、参考文献和附录等几部分。
1.1 题目题目应恰当、准确地反映本课题的研究内容。
论文题目不应超过25字,不得使用标点符号,不设副标题。
1.2 摘要与关键词1.2.1 摘要摘要应扼要叙述本论文的主要内容、特点,文字要精炼,是一篇具有独立性和完整性的短文,应包括本论文的主要成果和结论性意见。
摘要中不宜使用公式、图表,不标注引用文献编号,避免将摘要写成目录式的内容介绍。
1.2.2 关键词关键词是供检索用的主题词条,应采用能覆盖论文主要内容的通用技术词条(参照相应的技术术语标准),一般列3~5个,按词条的外延层次从大到小排列,应在摘要中出现。
1.3 目录目录应独立成页,包括论文中全部章、节的标题及页码。
1.4 论文正文论文正文包括绪论、论文主体及结论等部分。
1.4.1 绪论绪论一般作为第1章。
绪论应说明选题的目的、背景和意义,国内外文献综述,以及论文所要研究的主要内容。
文管类论文的绪论是毕业论文的开头部分,一般包括说明论文写作的目的、意义,对所研究问题的认识以及提出问题。
绪论只是文章的开头,不必写章号。
1.4.2 论文主体论文主体是论文的主要部分,应该结构合理,层次清楚,重点突出,文字简练、通顺。
论文主体的内容要求参照《哈尔滨工业大学关于本科生毕业设计(论文)的若干规定》第六条。
论文主体各章后应有一节“本章小结”。
1.4.3 结论结论作为单独一章排列,但不加章号。
研究生一年级英语课本翻译
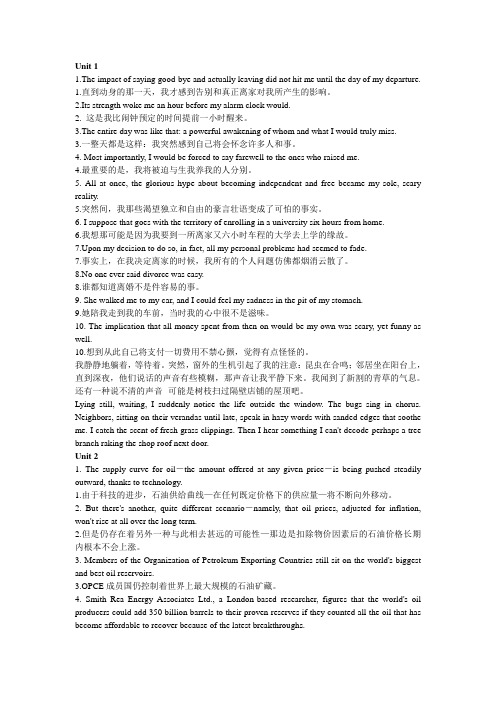
Unit 11.The impact of saying good-bye and actually leaving did not hit me until the day of my departure.1.直到动身的那一天,我才感到告别和真正离家对我所产生的影响。
2.Its strength woke me an hour before my alarm clock would.2. 这是我比闹钟预定的时间提前一小时醒来。
3.The entire day was like that: a powerful awakening of whom and what I would truly miss.3.一整天都是这样:我突然感到自己将会怀念许多人和事。
4. Most importantly, I would be forced to say farewell to the ones who raised me.4.最重要的是,我将被迫与生我养我的人分别。
5. All at once, the glorious hype about becoming independent and free became my sole, scary reality.5.突然间,我那些渴望独立和自由的豪言壮语变成了可怕的事实。
6. I suppose that goes with the territory of enrolling in a university six hours from home.6.我想那可能是因为我要到一所离家又六小时车程的大学去上学的缘故。
7.Upon my decision to do so, in fact, all my personal problems had seemed to fade.7.事实上,在我决定离家的时候,我所有的个人问题仿佛都烟消云散了。
8.No one ever said divorce was easy.8.谁都知道离婚不是件容易的事。
在航天的基础上展开想象写一篇作文

在航天的基础上展开想象写一篇作文英文回答:Space exploration has always been a fascinating subject for many people. The advancements in technology and the curiosity of human beings have led to numerous achievements in the field of space exploration.One of the most significant achievements in space exploration is the landing of humans on the moon. This monumental event took place on July 20, 1969, when Neil Armstrong became the first person to set foot on the lunar surface. This event marked a new era in human history and opened up possibilities for further exploration and colonization of other celestial bodies.The exploration of Mars has also been a major focus in space exploration. Multiple spacecraft have been sent to Mars to gather information about its atmosphere, geology, and the possibility of past or present life. The Mars Rovermissions have provided valuable data and images, giving scientists a better understanding of the red planet.The International Space Station (ISS) is another remarkable achievement in space exploration. It is a habitable space station that serves as a laboratory for scientific research and international cooperation. Astronauts from different countries live and work on the ISS, conducting experiments and studying the effects of long-duration space travel on the human body.Space exploration has not only contributed to scientific knowledge but has also led to technological advancements. The development of satellite technology has revolutionized communication, weather forecasting, and navigation systems. Satellites orbiting the Earth provide us with real-time information and have become an integral part of our daily lives.中文回答:航天探索一直以来都是许多人着迷的话题。
外太空的英文作文
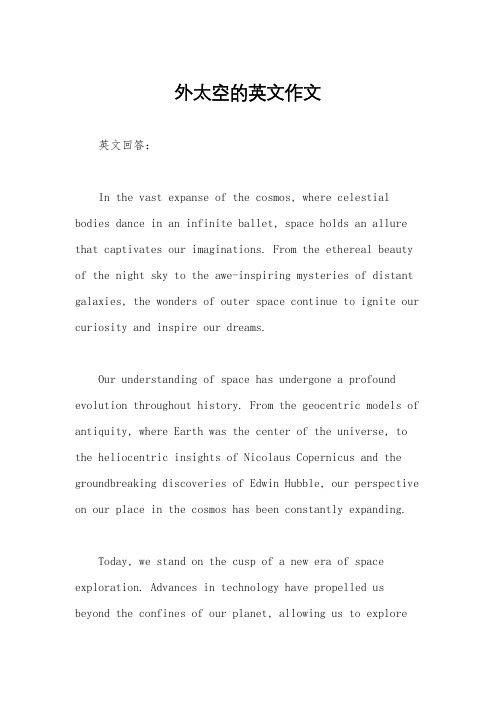
外太空的英文作文英文回答:In the vast expanse of the cosmos, where celestial bodies dance in an infinite ballet, space holds an allure that captivates our imaginations. From the ethereal beauty of the night sky to the awe-inspiring mysteries of distant galaxies, the wonders of outer space continue to ignite our curiosity and inspire our dreams.Our understanding of space has undergone a profound evolution throughout history. From the geocentric models of antiquity, where Earth was the center of the universe, to the heliocentric insights of Nicolaus Copernicus and the groundbreaking discoveries of Edwin Hubble, our perspective on our place in the cosmos has been constantly expanding.Today, we stand on the cusp of a new era of space exploration. Advances in technology have propelled us beyond the confines of our planet, allowing us to explorethe depths of our solar system and venture into the uncharted territories of interstellar space. Robotic missions, such as the Voyager probes and the Curiosity rover, have provided invaluable data and stunning images, transforming our knowledge of distant worlds. Human spaceflight, exemplified by the Apollo missions and the International Space Station, has demonstrated our capacityto overcome the challenges of living and working in the harsh environment of space.As we venture further into the cosmos, we areconfronted by a myriad of questions and possibilities. Are we alone in the universe? What lies beyond the observable universe? What is the nature of dark matter and dark energy? These are just a few of the profound mysteries that await exploration.The pursuit of knowledge about outer space is notmerely an academic endeavor. It has practical implications for our lives on Earth. Satellites orbiting our planet provide vital services such as navigation, communication, and weather forecasting. Space exploration also drivesinnovation, leading to advancements in materials science, engineering, and computing.Beyond its practical benefits and scientific revelations, outer space holds a profound aesthetic and philosophical appeal. The contemplation of the cosmic vastness inspires a sense of awe and humility, reminding us of our insignificance in the grand scheme of things. At the same time, it fuels our aspirations and fuels our desire to comprehend our place in the universe.As we continue to explore outer space, we are not only expanding the boundaries of human knowledge but also broadening our perception of ourselves and our potential. The wonders of the cosmos await our discovery, and it is our privilege to embark on this extraordinary journey.中文回答:宇宙浩瀚无垠,天体在其中宛如一场永恒的芭蕾舞,太空的魅力吸引着我们的想象力。
The Future of Space Exploration A New Frontier
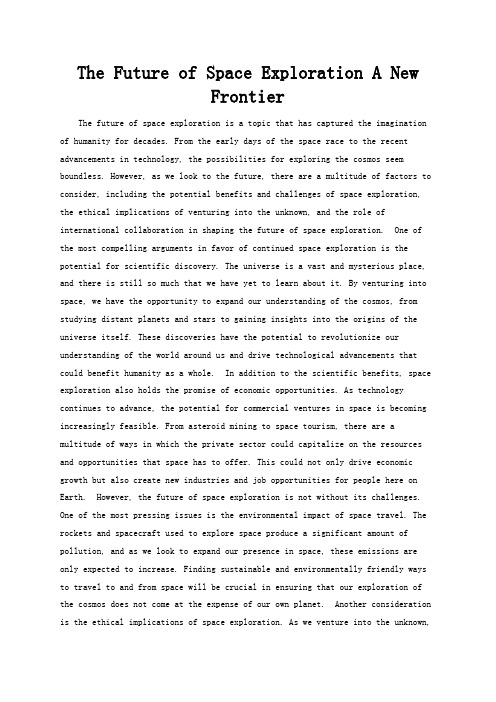
The Future of Space Exploration A NewFrontierThe future of space exploration is a topic that has captured the imagination of humanity for decades. From the early days of the space race to the recent advancements in technology, the possibilities for exploring the cosmos seem boundless. However, as we look to the future, there are a multitude of factors to consider, including the potential benefits and challenges of space exploration, the ethical implications of venturing into the unknown, and the role of international collaboration in shaping the future of space exploration. One of the most compelling arguments in favor of continued space exploration is the potential for scientific discovery. The universe is a vast and mysterious place, and there is still so much that we have yet to learn about it. By venturing into space, we have the opportunity to expand our understanding of the cosmos, from studying distant planets and stars to gaining insights into the origins of the universe itself. These discoveries have the potential to revolutionize our understanding of the world around us and drive technological advancements that could benefit humanity as a whole. In addition to the scientific benefits, space exploration also holds the promise of economic opportunities. As technology continues to advance, the potential for commercial ventures in space is becoming increasingly feasible. From asteroid mining to space tourism, there are a multitude of ways in which the private sector could capitalize on the resources and opportunities that space has to offer. This could not only drive economic growth but also create new industries and job opportunities for people here on Earth. However, the future of space exploration is not without its challenges. One of the most pressing issues is the environmental impact of space travel. The rockets and spacecraft used to explore space produce a significant amount of pollution, and as we look to expand our presence in space, these emissions are only expected to increase. Finding sustainable and environmentally friendly ways to travel to and from space will be crucial in ensuring that our exploration of the cosmos does not come at the expense of our own planet. Another consideration is the ethical implications of space exploration. As we venture into the unknown,we may encounter new forms of life or come across resources that are of great value. It will be important for us to approach these discoveries with a sense of responsibility and respect, ensuring that we do not exploit or harm other life forms for our own gain. Additionally, we must consider the impact that our presence in space may have on other celestial bodies, such as the potential for contaminating other planets with Earth-based microorganisms. Furthermore, the future of space exploration will undoubtedly be shaped by international collaboration. The challenges and costs associated with space exploration are immense, and no single country can tackle them alone. By working together, nations can pool their resources and expertise to achieve common goals, whether it be establishing a permanent human presence on another planet or conducting large-scale scientific experiments in space. International collaboration will also be crucial in establishing guidelines and regulations for space exploration, ensuring that it is conducted in a safe and responsible manner. In conclusion, the future of space exploration holds great promise, from the potential for scientific discovery to economic opportunities and technological advancements. However, it is important for us to approach this new frontier with a sense of responsibility and mindfulness, considering the ethical implications and environmental impact of our actions. By working together on an international scale, we can ensure that the future of space exploration is one that benefits all of humanity and respects the vast and wondrous cosmos that we are so eager to explore.。
Abstract Design and Implementation of Semi-preemptible IO

is thus undesirable. Making disk IOs preemptible would reduce blocking and improve the schedulability of realtime disk IOs. Another domain where preemptible disk access is essential is that of interactive multimedia such as video, audio, and interactive virtual reality. Because of the large amount of memory required by these media data, they are stored on disks and are retrieved into main memory only when needed. For interactive multimedia applications that require short response time, a disk IO request must be serviced promptly. For example, in an immersive virtual world, the latency tolerance between a head movement and the rendering of the next scene (which may involve a disk IO to retrieve relevant media data) is around 15 milliseconds [2]. Such interactive IOs can be modeled as higher-priority IO requests. However, due to the typically large IO size and the non-preemptible nature of ongoing disk commands, even such higherpriority IO requests can be kept waiting for tens, if not hundreds, of milliseconds before being serviced by the disk. To reduce the response time for a higher-priority request, its waiting time must be reduced. The waiting time for an IO request is the amount of time it must wait, due to the non-preemptibility of the ongoing IO request, before being serviced by the disk. The response time for the higher-priority request is then the sum of its waiting time and service time. The service time is the sum of the seek time, rotational delay, and data transfer time for an IO request. (The service time can be reduced by intelligent data placement [27] and scheduling policies [26]. However, our focus is on reducing the waiting time by increasing the preemptibility of disk access.) In this study, we explore Semi-preemptible IO (previously called Virtual IO [5]), an abstraction for disk IO, which provides highly preemptible disk access (average preemptibility of the order of one millisecond) with little loss in disk throughput. Semi-preemptible IO breaks the components of an IO job into fine-grained physical diskcommands and enables IO preemption between them. It
4美航宇局2013年度新概念研究项目新鲜出炉

一 . j 糖
L
安托尼 ‘ 朗曼独立提 出的 “ 自适应生长的张拉整体 结构”( 项目5 ) 描述 的是 一个全新的创建并制造经济可行 的空间栖息 发展技 术 : 在地月第二拉格 朗日点部署一个轻量级 的张拉 整体 栖息地结构 。在那里 ,机器人使用 空间为基础 的材料提供 辐射 屏蔽 、灌溉 、生活支持 、生态系统 发展 的土壤 ,并维护和增强 结构 。栖 息地可以成为旅游胜地 、经济枢纽和 一个多 用途 的用 于月球表面开发和空 间生态系统的生命科学研究与支持设施 。 这次得到资 助的还有 项目 1 0: “ 极端环 境中的变形金 刚” 。这是一个可 以改变形状和 功能的多功能 平台 ,它们可以原位
行主任福克尔 ( J a y F a l k e r )应 “ 行星协 幻小 说 。它们有 科学 研究 作 为基础 ,技 局 新概 念 研究项 目的前身 是 “ 美 航宇 局 宇局确定未来的发展 方向。
先 进理念研究 所” ,由大学空间研究协 会
会” 的要求介 绍 了这 次的评 选 。 “ 美航 宇 术上 有成 功 的希望 。它们 可以帮 助美 航 共 同特 点 : 雄 心勃 勃 。不 怕做 不 到 ,就 怕想不到 。虽然只有 1 2个项 目,但是却
一
种不 同于 常规 的思 路 ,必须 试 图打破
技术 上的 限制 ,挑战 常规 ,而 且 必须 使
“ 美航宇局新概念研 究项 目”的目标 评审人 员 能够理 解项 目中 的基本 原理 还
五十万美元的资助。美该局希望通过这些项 是 通 过那些 真正 革命性 的 富有远 见的 新 能解放 思 想 ,对项 目得 出 一个合 理的 评
“ 美航宇局 新概 念研究项 目”每两年 覆盖 了航 空和 航天 的多 个领 域 。福 克 尔
- 1、下载文档前请自行甄别文档内容的完整性,平台不提供额外的编辑、内容补充、找答案等附加服务。
- 2、"仅部分预览"的文档,不可在线预览部分如存在完整性等问题,可反馈申请退款(可完整预览的文档不适用该条件!)。
- 3、如文档侵犯您的权益,请联系客服反馈,我们会尽快为您处理(人工客服工作时间:9:00-18:30)。
Semi-Immersive Space Mission Design and Visualization:Case Study of the“Terrestrial Planet Finder”Mission.Ken Museth Alan Barr Martin W.LoComputer Science DepartmentCalifornia Institute of TechnologyPasadena,CA91125AbstractThis paper addresses visualization issues of the Terrestrial PlanetFinder Mission[2].The goal of this mission is to search for chem-ical signatures of life in distant solar systems usingfive satellitesflying in formation to simulate a large telescope.To design and vi-sually verify such a delicate mission one has to analyze and interactwith many different3D spacecraft trajectories,which is often dif-ficult in2D.We employ a novel trajectory design approach usinginvariant manifold theory,which is best understood and utilized inan immersive setting.The visualization also addresses multi-scaleissues related to the vast differences in distance,velocity,and timeat different phases of the mission.Additionally,the parameteri-zation and coordinate frames used for numerical simulations maynot be suitable for direct visualization.Relative motion presents amore serious problem where the patterns of the trajectories can onlybe viewed in particular rotating frames.Some of these problemsare greatly relieved by using interactive,animated stereo3D visu-alization in a semi-immersive environment such as a ResponsiveWorkbench.Others were solved using standard techniques suchas a stratify approach with multiple windows to address the multi-scale issues,re-parameterizations of trajectories and associated2Dmanifolds and relative motion of the camera to“evoke”the desiredpatterns.1IntroductionModern space missions are increasingly more complex as advancedinstrument and spacecraft technologies enable more demanding sci-entific queries during space exploration.Visualization of complexscenarios and high dimensional parameter spaces are extremelyuseful for the design of such missions.We are developing an in-teractive,semi-immersive visualization tool called LVis as part ofa trajectory design tool(LTool)to support new space missions atthe Jet Propulsion Laboratory(JPL).We selected the visualizationof the Terrestrial Planet Finder Mission(TPF)as a case study toidentify and analyze the requirements for LVis using trajectory datagenerated in a previous study for NASA[3].The goal of TPF istofind Earth-like planets around stars near our Sun using interfer-ometry.The mission concept for this case study involvesflying aconstellation offive satellites in formation around a3D periodichalo orbit near a so called Lagrange Point(in Fig.2).For thishalo orbit mission we also employ a novel design technique forthe low-fuel transfer of the TPF mothership from Earth to the haloorbit.Specifically the transfer trajectory is selected from a fam-ily of low-energy trajectories which form a tube-like surface calledthe stable manifold emanating from the halo orbit.The main con-tribution of this paper is to demonstrate how this novel approachto low-energy trajectory design can conveniently be analyzed andFigure2:The Lagrange points arefive equilibrium(i.e.zero total force)points in the orbit of one body around another,such as the Earth around the Sun.,,and are unstable with respect to small perturbations,whereas and are stable equilibriumpoints.The halo orbital considered for the TPF mission is a3D periodic orbit around the Lagrange point.2.1The Model for the TPF SimulationWe model the dynamics of TPF in the Circular Restricted Three Body Problem(CRTBP)where the Sun and the Earth are moving in circular orbits around the center of mass,and the third body(a massless spacecraft)moves under their influence in the XY-plane [3].The most natural frame to use in the CRTBP is a rotating frame with the origin at the center of mass,the X-axis along the Sun-Earth line,and the Earth on the positive X-axis,as shown in Fig.2.is one of thefive Lagrange points.It lies on the far side of the Earth along the Sun-Earth line as depicted in Fig.2.A halo orbit is a3D,large,unstable periodic orbit around with a shape simular to the edge of a potato chip.The instability of the halo orbit is very small(time constant on the order of a month)and it provides a whole family of low energy transfer trajectories from the Earth to the halo,forming a tube called the stable manifold of the halo orbit.Fig.4(b)on the color plate depicts a portion of the stable manifold(green)with a family of transfer trajectories(red)from the Earth to the halo orbit.The trajectories and the2D manifold are actually objects in the6D phase space composed of position and velocity.We are visualizing the projection of these objects into 3D position space.In fact,if we continued the computation of the stable manifold,the visualization of its projection in position space will show self-intersections.Astrodynamicists have determined how to break a trajectory into blocks that may be designed somewhat independently.These blocks can be assembled in tinker-toy fashion,following rules and constraints,to produce an end-to-end trajectory.The gluing of these blocks is an art and typically uses multiple shooting algorithms or two-point-boundary value methods[3].For halo orbit missions,the blocks are the launch orbit,the parking orbit,the transfer orbit,and the halo orbit.2.2Low-Energy Transfer to Halo OrbitFor this study,we assume that we already have the halo orbit and its stable manifold(see[3]for details).The designer next selects an insertion point on one of the red transfer orbits(color plate Fig.4(a) and4(b))embedded on the stable manifold with the picking device. During this step LVis provides useful information to the designer, like fuel consumption to reach the halo orbit,velocity(red arrow) etc.,that will help him in this decision process.LVis then computes a circular parking orbit around the Earth that connects to the inser-tion point on the selected white trajectory on the manifold.We do not compute and visualize the launch orbit from Earth to the park-ing orbit because this is considered a separate problem that does not have to be considered during the early study phase of the mission.2.3Formation Flight Around Halo OrbitOnce an end-to-end trajectory from a parking orbit to the halo or-bit has been designed,we move to the next phase of the simula-tion,the Observation Phase.We assume the spacecraft carrying thefive satellites for the TPF mission is now on the halo orbit.At a predetermined moment,thefive satellites are deployed from the spacecraft and maneuver into the initial formation where four of the satellites are equally spaced on the diameter of a100meter spinning circle,and thefifth satellite(the beam combiner)is off-set from the center of the circle by50meters.The observations of the four satellites are beamed to the collector satellite.The spin of the circle synthetically creates a telescope with a100m diameter. For this simulation,the satellites are actually moving along a20 sided polygon using small impulsive rocket burns at the corners of the20-gon.These impulsive burns also include corrections due to the small instability of the halo orbit and gravitational perturbations from other planets.The angular momentum vector of the spinning circle point at the star that TPF is currently observing.After mak-ing several revolutions in the direction of one star,TPF reorients the spinning20-gon by reconfiguring the satellites into the next spin-ning20-gon pointing at the next star to be observed.Fig.4(c)on the color plate shows the spirals generated by the TPF formation as it makes its observation along the halo orbit(straight blue orbit across the image).3VisualizationThe TPF mission design strategies presented in the previous section form a complex problem that may be studied with an interactive simulation environment that offers constant visual feedback.The goals and requirements of the graphics tool for the TPF simulation presented in this paper can be broken into the following steps.1.Animate the time-propagation of the stable manifold of thehalo orbit associated with the Lagrange points of the Sun-Earth system.2.Select a low-energy transfer trajectory on the stable manifoldand compute a conic low-Earth parking orbit for the space-craft that intersects the manifold trajectory at the selected in-sertion point.unch the spacecraft with TPF satellites from the intersec-tion point of the parking orbit along the low-energy trajectory onto the halo orbit.4.Once the spacecraft reaches the halo orbit,deploy thefivesatellites into initial formation.5.Begin satellite pattern maintenance on a20-gon by makingimpulsive rocket burns at the vertices to form a large virtual telescope.6.Satellites make reconfiguration maneuvers to reorient the lineof sight by tilting the plane of the20-gon.This requirements list for LVis,the interactive design and visu-alization tool of the TPF mission,presents a number of graphical problems which is the topic of the remaining of this section.3.1Multi-Scaling IssuesA problem often encountered when visualizing astronomical data is the enormous differences in scale that they typically represent.The differences can be both in spatial distances,time lines for events and relative velocities to somefixed frame of reference.This is es-pecially pronounced in the present case study of the TPF mission. For instance,the distance from Earth to is1.5million km and the baseline halo orbit has amplitudes on the order of700,000km whereas the formationflight of thefive satellites around it has to beaccurate to within 2centimeters.Also the velocity of the satellites relative to each other is typically a few m/hour whereas their relative speed to the halo orbit is several thousand km/hour.The transfer of the spacecraft from a parking orbit around Earth,along a low-energy transfer trajectory,onto the halo orbit takes several months.In contrast,the pattern formation of the satellites typically spans a few hours.These enormous differences in scales (a factor offor distance)clearly cause problems both for the visualization as well as the numerical simulation.The latter is conveniently solved by partitioning the numerical simulation of the mission into two phases:a computation of the stable manifold in a Sun-Earth rotat-ing frame,and the computation of the satellite motion in a moving frame relative to the baseline halo orbit.This simple but effective strategy suggests a similar stratified approach to the visualization.Thus,the design and visualization of the mission is rendered in two separate windows on the monitor or as two different applications on the RWB -one for the transfer from Earth to the halo orbit on a scale of astronomical units (149,600,000km)and a time-scale of days (step 1through 3in the list in Section 3)-and one for the for-mation flight on a scale of meters and minutes (step 4-6).However,one adjustment had to be made to this approach;since the forma-tion flight is simulated in a frame that moves with a velocity equal to the halo baseline this gave a misleading visual illusion of the satellites flying in static periodic orbits around the baseline.To fix this we introduced a new frame of reference in which the camera is fixed,but the satellites move slowly forward and the spacecraft slowly backward.This enables the visualization of the pattern of the satellite formation.3.2Parameterization IssuesA fundamental problem when rendering the trajectories is the pa-rameterization.For the numerical simulation a natural parame-terization of the trajectories is by time.Specifically the sampled representations of all the trajectories were given by fixed time-increments.However,due to the rather complex nature of the fam-ily of trajectories embedded on the stable manifold in 6D phase-space,such a parameterization is not suitable for visualization -see Fig.3.Since the velocity on the trajectory changesdramati-Figure 3:Arclength parameterized orbit antialiases the rendering to produce a smooth curve (left),time parameterized does not (right).cally in space,a fixed time parameterization produces very jagged or aliased lines when the trajectory is projected to the 3D posi-tion space.On the other hand a fixed arclength parameterization would produce many redundant sampling points in regions near the halo orbit where the velocity is very low.Thus,we devel-oped a simple scheme for the arclength parameterization of the manifold trajectories that preserves as many of the original data points as ing the three coordinate and three velocity components at each pair of two adjacent original sampling pointsin the 6D phase-space,,a cubic poly-nomial is analytically fit to the corresponding curve segment.Ifthe arclengthis smaller than a given minimum arclength the point at is removed and if it is inte-ger times larger than a given maximum arclength new samplingpoints are inserted at timessuch that.The times are numerically foundby a Newton-Raphson iteration as described in reference [7]and the bounds on the arclength are chosen to produce sufficiently smooth trajectories.This subdivision algorithm proved efficient for the re-parameterization of the manifold trajectories (see Fig.3),and ad-ditionally generates important information about the accumulated arclength along each of the transfer trajectories that is returned to the user upon selections in step 2of the list above.However,a different approach had to be employed for the triangulation of the manifold tube.The growth of the manifold has to be animated as time evolves requireing a time parameterization that ensures a well-defined tube-like geometry for the manifold during all stages of the animation.Furthermore,the subdivision scheme outlined above generates a different number of sampling points for each manifold trajectory.This prevents the creation of a simple triangulation with rings of triangle strips from sets of two connecting points on each trajectory.On the other hand the original time-parameterized points undersample the tube in regions of space with large velocity (typ-ically near Earth)producing bad aspect-ratio triangles.A robust solution was simply a finer re-sampling of the trajectories in time.3.3Semi-Immersive VisualizationWe found it extremely useful for this study to employ the Respon-sive Workbench (RWB)at Caltech (see Fig.1).This is a 3D in-teractive virtual reality system with a tabletop metaphor originally developed by Wolfgang Krueger at GMD[6].The user of the RWB sees the virtual objects as floating over the tabletop and can interact with them just as they would with real objects on an actual work-bench.This allows for the mission designer to quickly develop a perception of the 3D structure of the stable manifold which in turn is very valuable both for general analysis,as well as the selection of initial conditions for the transfer trajectory embedded on the mani-fold (step 2in the list above).To create this 3D environment,users wear shutter glasses (V-sync at 120Hz)to view computer-generated stereoscopic images that are projected on the tabletop display sur-face by a projector/mirror system.The user’s head position and orientation are tracked to create the correct perspective for the com-puter to use when rendering the environment.A 6DOF input device from Polhemus is also tracked by the system allowing the users to accurately interact with the trajectories,see Fig.1.We especially found the stereoscopic feature of the RWB to be very useful for this study primarily because we are analyzing unfamiliar trajecto-ries with complicate 3D structures.The immersive interaction with the data also seems more natural on the RWB than on a 2D mon-itor where picking is typically implemented by ray intersection of bounding boxes and can lead to ambiguous selections such as points on either side of the manifold tube.3.4Animation SystemsPhysically-based animation[7]is naturally implemented using aprocedural approach where the simulation explicitly defines the movements of objects as a function of time.However,to allow for the animation to include objects that were not explicitly included in the actual calculations,(e.g.rotating stars and moon)and to prop-erly synchronize events and phasing of the mission like the growth of the manifold and launch time,we also implemented an event-driving scripting system.This allows the user to completely control the sequence of events and to “play”the mission forward or back-ward at any desired speed.To conveniently communicate a completed mission design by an automated animation or to generate video animations a 3D key frame animation system was also implemented.It allows the user to interactively select the camera position and orientation at differenttimes of the mission,which are then played back by interpolatingthe key frames.The camera orientation is explicitly represented byquaternions and the interpolation is done as three successive spher-ical cubic interpolations as explained in reference[4].The sys-tem then performs off-screen rendering of the scene and dumps theframe-buffer to imagefiles.3.5Implementations And Visual CluesAn important requirement for LVis is that it should be interactive ona non-immersive desktop workstation for fast prototyping,and alsoflexible enough to be ported to a semi-immersive environment onhigh-end SGI hardware.The real-time requirement constrained thegraphics to onlyflat or Gouraud-shaded polygons with texture map-ping,and the portability issues lead us to select the open-sourcedOpenInventor API[5].As emphasized in Section3.1it was numerically convenient tostratify the visualization of the TPF mission into two phases orscene graphs-the transfer followed by the formationfl-mon to both scene graphs was a background star-map implementedby projecting the true ephemeris on a rotating sphere placed at thecenter of the bounding-box.Both scenes also have a directionallight source oriented according to the position of the Sun in orderto obtain the correct lighting of all objects.The“transfer-scene-graph”,Fig.4(b),has a grid in the XY-planeof the rotating Sun-Earth frame and texture-maps of the spinningEarth with the Moon in a conic orbit around it.The direction of theSun is indicated by a glowing yellow arrow and the static position of by a simple colored sphere placed on the Sun-Earth X-axis.The scene also includes a texture-mapped spacecraft withfive satellitesorbiting around Earth in a conic orbit which by construction inter-sects the user-selected transfer trajectory.The halo orbit is shownas a closed curve around,and the stable manifold is grown as a transparent tube and has the embedded family of trajectories twist-ing around it.After the transfer trajectory is highlighted and the corresponding parking orbit is computed from a selected insertion point the spacecraft makes a small burn andflies on the stable man-ifold onto the halo orbit.The“formation-scene-graph”,Fig.4(c),shows a very small seg-ment of the halo orbit with the spacecraft andfive satellites attachedto it.For this visualization,we implemented a number of visual andaudio cues to emphasize the rather complex dynamics.As alreadymentioned the illustration of motion relative to the halo orbit wasartificially introduced,and to enhance this thefive spiraling trajec-tories of the satellites are dynamically rendered with color coding.To emphasize the detailed relative motion of the satellites a trans-parent20-gon is added to the scene and the orientation of the virtualtelescope is illustrated by an arrow pointing in the line of sight ofthe IR interferometer.The rocket burns of the satellites at each ver-tex of the20-gon are emphasized by short pulsating animations offire accompanied by a sound effect.4Conclusions And Future WorkWe have presented an interactive design,visualization,and anima-tion tool,LVis,for the TPF mission.Our investigations and pro-totypes show the RWB metaphor to be very useful for visualizingcomplex geometries.The RWB is able to provide3D visual cuesand nuances absent in non-immersive environments,which is par-ticularly important for this study since most of the trajectories arenew and unfamiliar to mission designers.In the case of the low-energy transfer from Earth to the halo orbit we employ a noveldesign strategy that produces unfamiliar trajectories embedded oncomplicated3D manifolds.In the case of the trajectories spinningaround the halo orbit,this is thefirst demonstration that such tightformationflight is possible.Hence,it is even more important to provide additional insight through immersive visualization to help the aerospace and scientific communities understand this new de-sign approach.We solved the multi-scale problems using stratification approach with multiple windows.We solved the aliasing problems by re-parameterizing the orbits by arclength and by re-sampling the man-ifolds in time.We solve the manifold animation problem by prop-agating the manifold tube in annular strips which are dynamically triangulated.We solved the problem of visualizing the formation pattern by moving the camera in a proper manner.We provided picking on the trajectories and manifolds to enable intuitive and convenient exploration of large data sets.We provided a scripting language to create efficient3D key-frame animations using quater-nions.We plan to conduct a detailed user analysis of our visualization and design tool in the near future when it has further matured.One of the improvements that we would like to see is a different solution to the multi-scale problem that will allow all phases of the mission design to be handled in the same window/application.For this we are currently investigating a camera model by Blinn[1]which al-lows for the specification of spacecraft and planets as respectively foreground and background objects in the same scene.Other plans for future work include the computation and visualization of in-tersections of different manifolds to provide new options for low energy trajectory design.We plan to study the use of haptic devices to add another dimension to the immersive experience.We are also exploring the option of providing semi-immersive capabilities on a desktop workstation as a low-cost alternative to the RWB. AcknowledgmentThis work was carried out at the Jet Propulsion Laboratory and the California Institute of Technology under a contract with National Aeronautics and Space Administration.The work was partially supported by the National Science Foundation(ASC-89-20219and ACI-9982273).We thank Josep Masdemont and Gerald G´o mez for the TPF simulation performed in a previous collaboration[3].Fi-nally we thank David Breen for many useful comments and Mark Montague for assisting the video production with this paper. References[1]J.Blinn.Where Am I?What Am I Looking At?IEEE Com-puter Graphics And Applications,8(4):76–81,July1988. [2]Beichman C.A.,Woolf N.J.,and Lindensmith C.A.The ter-restrial planetfinder:A NASA origins program to search for habitable planets.JPL Publication99-3,May1999.[3]G.Gomez,M.Lo,J.Masdemont,and K.Museth.Simulationof formationflight near Lagrange points for the TPF mission.In Proceedings of Astrodynamics Specialist Conference,Que-bec City,Canada,2001.American Astronautical Society. [4]K.Shoemake.Quaternion calculus and fast animation.InCourse Notes,volume10,pages101–21.SIGGRAPH,1987.[5]P.S.Strauss and R.Carey.An object-oriented3D graphicstoolkit.In Computer Graphics,volume26,pages341–349.SIGGRAPH,1992.[6]Krueger W.and Froehlich B.The responsive workbench.IEEEComputer Graphics and Applications,14(3):12–15,May1994.[7] A.Watt and M.Watt.Advanced Animation and RenderingTechniques,chapter15.Addison-Wesley,1992.(a)Photo of immersive orbit selection on the RWB(b)Screen shot showing the picking of a white transfer trajectory embedded on a green stable manifold.Thearrow indicates the direction and magnitude of the velocity at the selected insertion point on the trajectory goingfrom Earth to the halo orbit at the far end of the manifold tube.Notice the spacecraft in the orbit around Earth.(c)Screen shot of5satellites with color-coded trajectories in formationflight around a segment of the halo orbit(straight blue line)forming a virtual telescope(the orange20-gon)with a line of sight indicated by the red arrow.Figure4:Semi-Immersive Space Mission Design and Visualization:Case Study of the“Terrestrial Planet Finder”mission.。