Aeroelastic Stability of a Four-Bladed Semi-Articulated Soft-Inplane Tiltrotor Model
Aerobics英英解释

AerobicsAerobic is a form of physical exercise that combines rhythmic aerobic exercise with stretching and strength training routines with the goal of improving all elements of fitness (flexibility, muscular strength, and cardio-vascular fitness). It is usually performed to music and may be practiced in a group setting led by an instructor (fitness professional), although it can be done solo and without musical accompaniment. With the goal of preventing illness and promoting physical fitness, practitioners perform various routines comprising a number of different dance-like exercises. Formal aerobics classes are divided into different levels of intensity and complexity. Aerobics classes may allow participants to select their level of participation according to their fitness level. Many gyms offer a variety of aerobic classes. Each class is designed for a certain level of experience and taught by a certified instructor with a specialty area related to their particular class.Contents1 History2 Aerobic gymnasticsHistoryBoth the term and the specific exercise method were developed by Dr. Kenneth Cooper, M.D., an exercise physiologist, and Col. Pauline Potts, a physical therapist, both of the United States Air Force. Dr. Cooper, an avowed exercise enthusiast, was personally and professionally puzzled about why some people with excellent muscular strength were still prone to poor performance at tasks such as long-distance running, swimming, and bicycling. He began measuring systematic human performance using a bicycle ergometer, and began measuring sustained performance in terms of a person's ability to use oxygen. His groundbreaking book, Aerobics, was published in 1968, and included scientific exercise programs using running, walking, swimming and bicycling. The book came at a fortuitous historical moment, when increasing weakness and inactivity in the general population was causing a perceived need for increased exercise.Aerobic gymnasticsAerobic gymnastics, also known as sport aerobics and competitive aerobics, is a type of competitive aerobics involving complicated choreography, rhythmic and acrobatic gymnastics with elements ofaerobics.[1] Performance is divided into categories by age, sex and groups (individual, mixed pairs and trios) and are judged on the following elements: dynamic and static strength, jumps and leaps, kicks, balance and flexibility. Ten exercises are mandatory: four consecutive high leg kicks, patterns. A maximum of ten elements from following families are allowed: push-ups, supports and balances, kicks and splits, jumps and leaps. Elements of tumbling such as handsprings, handstands, back flips, and aerial somersaults are prohibited. Scoring is by judging of artistic quality, creativity, execution, and difficulty of routines. Sport aerobics has state, national, and international competitions, but is not an Olympic sport.Best wishesBy Phoebe。
刀具角度介绍英文作文

刀具角度介绍英文作文Title: Introduction to Knife AnglesIntroduction:Knife angles play a crucial role in determining the performance and functionality of a blade. The angle at which a blade is sharpened influences its cutting ability, durability, and overall effectiveness. In this article, we will explore the different knife angles commonly used, their advantages, and how they impact various cutting tasks.Body:1. The Importance of Knife Angles:Knife angles are defined as the bevel or slope formed on the edge of a blade during sharpening. They are crucial because they determine how the blade interacts with the material being cut. A correct angle ensures efficient cutting, while an incorrect one may result in reduced performance or even damage to the blade.2. Common Knife Angles:a. Acute Angle:An acute angle, typically ranging between 10 to 20 degrees, is commonly used for razor-sharp blades. This angle provides excellent cutting performance on delicate tasks such as slicing fruits, vegetables, or meat. However, due to its thin edge, blades sharpened at acute angles may require more frequent maintenance.b. Standard Angle:A standard angle, usually between 20 to 25 degrees, is commonly found on most kitchen knives and general-purpose blades. This angle strikes a balance between sharpness and durability, making it suitable for everyday cutting tasks like chopping, dicing, and mincing. Blades sharpened at this angle offer a good compromise between edge retention and cutting ability.c. Obtuse Angle:An obtuse angle, ranging from 25 to 30 degrees or more, is often used for heavy-duty tasks that require durability over sharpness. This angle is commonly found on knives used for chopping tough materials, such as cleavers or survival knives.Blades with obtuse angles tend to have stronger edges but sacrifice some cutting ability.3. Factors Affecting Knife Angle Selection:Several factors influence the choice of knife angle, including the intended use, blade material, and user preference.A thinner blade material may require a lower angle for optimal performance, while tougher materials may benefit from a higher angle to enhance durability. Additionally, user experience and cutting technique also play a role in determining the ideal angle.Conclusion:Knife angles are a critical aspect of blade performance, determining the balance between sharpness and durability. Acute angles excel in precision cutting, standard angles offer versatility, and obtuse angles provide durability forheavy-duty tasks. Understanding the different knife angles and their applications allows users to select the most suitable blade for their specific cutting needs.。
Aerobics for a Fit Body

**Title: Aerobics for a Fit Body**In the realm of fitness pursuits, aerobics stands out as a dynamic and effective way to achieve a fit body. Let's explore the world of aerobics and uncover its many benefits.The theme is centered on the power of aerobics to sculpt and tone the body. We can express this by sharing stories of individuals who have transformed their physiques through regular aerobics sessions. The structure could begin with an engaging introduction that grabs the reader's attention and sets the stage for the exploration of aerobics. Then, describe the various aspects of aerobics, including the actions, suitable venues, necessary equipment, and the sensations it evokes. Finally, discuss the benefits of aerobics in terms of physical health, mental well-being, and social interaction.When it comes to the details of aerobics, it's a symphony of movement and energy. Picture a group of people in a brightly lit studio, moving in unison to the upbeat tempo of music. Their bodies sway, jump, and step with grace and precision. The actions range from simple steps to more complex combinations, all designed to get the heart pumping and the muscles working. The studio, with its mirrors and cushioned floors, is a haven for fitness enthusiasts. The participants wear comfortable workout clothes and shoes that allow for freedom of movement. The feeling of sweat trickling down and the rush of endorphins is exhilarating.For those who prefer outdoor aerobics, the connection with nature adds an extra dimension. The fresh air and natural surroundings provide a sense of freedom and rejuvenation. The sound of birds chirping and the warmth of the sun create a pleasant backdrop for the workout.Aerobics offers a multitude of benefits. Physically, it is a great cardiovascular workout that improves heart health, increases stamina, and burns calories. It also helps tone the muscles and improve flexibility. It's like a sculptor shaping the body into a masterpiece. Mentally, aerobics reduces stress and anxiety, boosting mood and enhancing mental clarity. As the saying goes, "A healthy body is a temple of the soul." Aerobics helps maintain this temple in pristine condition. Socially, aerobics brings people together. Joining an aerobics class or group provides an opportunity to meet new people, make friends, and share the journey to fitness. It creates a sense of community and support.After a careful grammar and spelling check, and some optimization ofexpressions, the article becomes an invitation to embrace aerobics. Let's move and groove our way to a fit body and a healthier life. For in aerobics, we find a path to a more vibrant and fulfilling self.。
isecondaryReference温度标准说明书
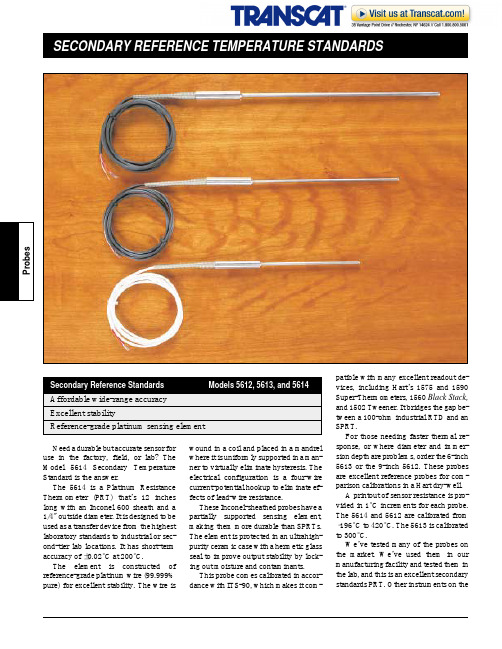
Need a durable but accurate sensor for use in the factory,field,or lab?The Model5614Secondary Temperature Standard is the answer.The5614is a Platinum Resistance Thermometer(PRT)that’s12inches long with an Inconel600sheath and a 1/4"outside diameter.It is designed to be used as a transfer device from the highest laboratory standards to industrial or sec-ond-tier lab locations.It has short-term accuracy of ±0.02°C at 200°C.The element is constructed of reference-grade platinum wire(99.999% pure)for excellent stability.The wire is wound in a coil and placed in a mandrelwhere it is uniformly supported in a man-ner to virtually eliminate hysteresis.Theelectrical configuration is a four-wirecurrent-potential hookup to eliminate ef-fects of lead-wire resistance.These Inconel-sheathed probes have apartially supported sensing element,making them more durable than SPRTs.The element is protected in an ultrahigh-purity ceramic case with a hermetic glassseal to improve output stability by lock-ing out moisture and contaminants.This probe comes calibrated in accor-dance with ITS-90,which makes it com-patible with many excellent readout de-vices,including Hart’s1575and1590Super-Thermometers,1560Black Stack,and1502Tweener.It bridges the gap be-tween a100-ohm industrial RTD and anSPRT.For those needing faster thermal re-sponse,or where diameter and immer-sion depth are problems,order the6-inch5613or the9-inch5612.These probesare excellent reference probes for com-parison calibrations in a Hart dry-well.A printout of sensor resistance is pro-vided in1°C increments for each probe.The5614and5612are calibrated from–196°C to420°C.The5613is calibratedto 300°C.We’ve tested many of the probes onthe market.We’ve used them in ourmanufacturing facility and tested them inthe lab,and this is an excellent secondarystandards PRT.Other instruments on themarket are priced much higher,have lower stability, or have lower quality.Remember,these instruments are in-expensive and have excellent durability. Each probe is individually calibrated and includes a report of calibration from the manufacturer.Contact Hart for calibra-tion in Hart’s NVLAP accredited lab. Ordering Information5612-X Secondary StandardPRT, 3/16" x 9", –200 to420°C5613-X Secondary StandardPRT, 3/16" x 6", –200 to300°C5614-X Secondary StandardPRT, 1/4" x 12", –200 to420°C2601Probe Carrying CaseX = termination. Specify “B” (bare wire), “S”(spade lugs), “D” (5-pin DIN for Tweener Thermometer), or “I” (INFO-CON for 1521 or 1522 Handheld Thermometer).Terminations are available as spade lugs, DIN connectors, banana plugs, INFO-CON, or bare wire.。
重型燃气轮机高雷诺数CDA_叶型转捩特性数值计算

收稿日期:2021-08-26作者简介:王润禾(1997),女,硕士。
引用格式:王润禾,童歆,羌晓青,等.重型燃气轮机高雷诺数CDA 叶型转捩特性数值计算[J].航空发动机,2023,49(5):136-142.WANG Runhe ,TONG Xin ,QIANG Xiaoqing ,et al.Numerical calculation of controlled diffusion airfoils of transition characteristics for heavy-duty gas turbine at high Reynolds number[J].Aeroengine ,2023,49(5):136-142.航空发动机Aeroengine重型燃气轮机高雷诺数CDA 叶型转捩特性数值计算王润禾1,童歆1,羌晓青2,3,杜朝辉1,3,欧阳华1,3(上海交通大学机械与动力工程学院1,航空航天学院2:上海200240;3.燃气轮机与民用航空发动机教育部工程研究中心,上海201306)摘要:为研究重型燃气轮机的压气机叶片在高雷诺数工况下的气动性能,基于Gamma-Theta 转捩模型的雷诺时均方程对某可控扩散叶型进行了数值计算。
通过对比不控制马赫数与控制马赫数,分析高雷诺数对可控扩散叶型气动性能及转捩特性的影响。
结果表明:在不控制马赫数条件下,在零攻角时,雷诺数从7×105增大为9×105,总压损失增加了约391.95%;在高雷诺数工况下随着雷诺数的增大,叶片流动损失不断增大,叶片可用攻角范围减小,同时在叶片吸力面出现激波,干扰转捩的产生。
在控制马赫数条件下,当Ma =0.6时,在零攻角工况下,雷诺数从8.2×105增大为1×107,总压损失减小了约38.98%,吸力面转捩起始点从4.78%弦长处前移至1.11%弦长处;在高雷诺数工况下,叶片流动损失随着雷诺数的增大不断减小,吸力面转捩位置前移。
机械工程(包括机械工程学专业词汇)EC

English Chinese abandon抛弃abaxial离轴的ablation烧蚀abnormal li异常液体abrasion磨耗abrasion ha耐磨硬度abrasion te磨损试验abrasion te磨损试验机abrasives研磨材料abrupt pert突然扰动abscissa横坐标absence of 无碰撞absence of 失重absence of 无应力absence of 无旋涡absolute绝对的absolute ac绝对加速度absolute an绝对角动量absolute ce绝对升限absolute co绝对坐标absolute de绝对偏差absolute el完全弹性体absolute eq绝对平衡absolute ex绝对极值absolute fl绝对飞行高度absolute fr绝对频率absolute ge绝对位势absolute hu绝对湿度absolute in绝对不稳定性absolute mo绝对运动absolute pa绝对路径absolute pe绝对扰动absolute pr绝对压力absolute re绝对静止absolute ro绝对旋转absolute si完全相似性absolute st绝对稳定性限度absolute te绝对温度absolute th绝对温度表absolute ve绝对速度absolute vi绝对粘度absolute vo绝对涡度absolute we绝对重量absolute ze绝对零度absolute ze绝对零度absorbabili吸收性absorbing a吸收剂absorbing m吸收媒质absorbing r吸收阻抗absorptiome吸收率计absorption吸收;缓冲absorption 吸收曲线absorption 吸收功率计absorption 吸收热absorption 吸收损失absorption 吸收模型absorption 吸收势absorption 吸收管absorption 吸收式波长计absorptive吸收的absorptive 吸收本领absorptivit吸收率accelerated加速蠕变accelerated加速运动acceleratin加速能力acceleratin加速场acceleratin加速梯度acceleratin加速撞击acceleratin加速周期acceleratin加速管acceleratin加速装置acceleratio加速度acceleratio加速度图acceleratio加速度能量acceleratio加速度场acceleratio加速度计acceleratio加速喷嘴acceleratio蠕变加速度acceleratio重力加速度acceleratio牵连加速度acceleratio加速势acceleratio加速压acceleratio加速度时间图acceleratio加速度矢量acceleratio加速波acceleratio加速功acceleratio无加速的accelerogra加速度记录仪acceleromet加速度计accessibili可达性accessible 可达点accidental偶然的accidental 偶然符合accidental 随机误差accidental 偶然共振accommodati适应accommodati适应系数accumulated累积误差accumulator蓄压器储压器accuracy准确度accuracy of测量准确度accuracy of读数准确度accurate ad精确蝶accurate me精确测量accurate mo准确模型accurate si准确相似accurate si准确模拟acicular st针状组织acoustic声的acoustic ab声吸收acoustic ab声吸收acoustic ab声吸收率acoustic ad声导纳acoustic an声学分析acoustic ba隔音板acoustic co声导率acoustic di声弥散acoustic di声音干扰acoustic ef声效率acoustic en声能acoustic fi声场acoustic fi滤声器acoustic fr音频acoustic im声阻抗acoustic lo声负载acoustic ma隔声材料acoustic me声机械效率acoustic po声功率acoustic pr声压acoustic ra声辐射压acoustic re声抗acoustic re声阻acoustic re共鸣acoustic ve声速acoustical声的acoustical 声波acting forc织力action at d超距酌action cent活动中心action inte酌积分action of d爆燃酌action of f力的酌action prin酌原理action sphe酌区action turb冲唤涡轮action vari酌变量action wave酌波activation活化active eart织土压active flig织飞行active forc织力active gas活性气体active load有功负载反酌active pres织压力activity of力功率actual load实际载荷actual stre实际应力actual valu实际值actuator执行器acyclic非循环的adaptabilit适应性adapter转接器adaptometer适应计addition附加additional 附加阻力additional 附加载荷additional 附加质量additional 附加压力adhere粘着adherence附着adhesion附着adhesion co粘着系数adhesive fo粘附力adhesive te粘附张力adiabat绝热线adiabatic绝热的adiabatic a绝热近似adiabatic a绝热大气adiabatic c绝热式量热器adiabatic c绝热变化adiabatic c态的绝热变化adiabatic c绝热压缩adiabatic c绝热冷却adiabatic c绝热线adiabatic e绝热效率adiabatic e绝热椭圆adiabatic e绝热平衡adiabatic e绝热膨胀adiabatic f绝热因子adiabatic h绝热加热adiabatic h绝热假设adiabatic i绝热不变量adiabatic i涡旋的绝热不变量adiabatic i涡旋的绝热不变量adiabatic p绝热扰动adiabatic p绝热原理adiabatic p绝热过程adiabatic p绝热脉动adjoint ope伴算子adjustable 可导叶adjusting d蝶装置adjusting s蝶弹簧adjusting v蝶阀adjusting w蝶楔块adjustment蝶adjustment 照准标志admissible 容许浓度admissible 容许误差admissible 容许荷载admissible 容许压力admissible 容许应力admissible 容许值admission进气admission p进气管admission p进气压力admixture混合物adsorb吸附adsorbent吸附剂adsorption吸附adsorption 吸附力adsorption 吸附层advancing r超前速率advancing w前进波advection平流advection f平另advective平聊aeration充气aerator通风机aerial空气的aerial rope架空死aeroballist航空弹道学aerodonetic滑翔学aerodromics滑翔力学aerodromome空气速度测量法aerodynamic空气动力酌aerodynamic空气动力比拟aerodynamic气动迎角aerodynamic气动迎角aerodynamic风洞天平aerodynamic气动中心aerodynamic气动力系数aerodynamic空气动力导数aerodynamic气动阻力aerodynamic气动力aerodynamic气动力加热aerodynamic气动升力aerodynamic气动阻力aerodynamic空气动力稳定性aerodynamic空气动力学的aerodynamic气动力矩aerodynamic空气动力学aerodyne重航空器aeroelastic气动弹性aeroelastic气动弹性力学aeroengine飞机发动机aerohydrody空气铃动力学aerology高空气象学aeromechani航空力学aerometer气体比重计aeromotor飞机发动机aeronautica航空的aeronautics航空学aeroplane飞机aeropulse e脉动式喷气发动机aerosol气溶胶aerospace e航空航天技术aerostat轻航空器aerostatics气体静力学aerothermod气动热力学aerothermoe空气热弹性学;气动热弹性aerotonomet气体张力测量法affine tran仿射变换affinity亲合力after effec后效after effec后效函数afterbody后部afterburner加力燃烧室aftershock 余震波age hardeni时效硬化ageing应变时效ageing test老化试验agglomerati凝聚aileron副翼air amount空气量air brake空气制动器air bubble气泡air buffer空气缓冲器air burst空中爆炸air cap空气冠air circula空气环流air column空气柱air compres空气压缩机air condens空气电容器air conveyi气龄送air coolant空气冷却剂air cooling空气冷却air coordin空间坐标air current气流air cushion气垫air cylinde压气缸air damper空气阻尼器air damping空气阻尼air density空气密度air drag空气阻力air duct风道air eddy空气涡旋air flow气流空气量air flow me气疗air frictio空气摩擦air gap空隙air gas风煤气air hammer空气锤air hardeni空气淬火air hoist风动起重滑车air humidit空气湿度air inflati充气air injecti空气喷射柴油发动机air inrush空气侵入air intake 进气阀air jet空气喷射air pipe气管air plasma空气等离子体air pressur空气压力计air pressur空气压头air pressur空气减压器air proof气密的air pump空气泵air registe空气活门air relief 放气塞air reservo储气筒air resista空气阻力air shock w空气激波air speed空气速率air speed i空气速率指示器air streaml空气吝air tank空气箱air tempera气温air tight气密的air to air 空对空导弹air traject空气轨迹air wave空气波air wedge空气楔aircraft dy飞机动力学airfoil机翼airfoil pro翼型airfoil sec翼剖面airfoil the机翼理论airless inj无气喷射airline飞行航线airlock气锁airmechanic空气力学airplane飞机airscrew空气螺旋桨airship飞艇airway航路airy stress爱里应力函数algebraic s代数应力模型aligned com定向复合材料aligned str定向结构allocation分配allotropic 同素异形变化allotropic 同素异形变化allowable c容许间隙allowable d容许挠度allowable d容许偏差allowable e容许误差allowable l容许荷载allowable p容许压力allowable s容许应力allowable t容许温度allowed cro容许截面allowed ene容许能带allowedness容许度alternate i反复浸没试验alternating交变弯曲alternating交变弯曲应力alternating交变弯曲试验alternating交替方向alternating交变滞后alternating交变冲讳曲试验alternating交变冲辉验alternating交替载荷alternating交变负载变形alternating交变压力alternating交替应力alternation交变alternation负载交变altimeter高度表altitude高度altitude ci等高圈altitude di高度差altitude fr高空锋面ambient air周围空气ambient med周围介质ambient pre周围压力ambient tem周围温度ambipolar d两极性扩散amendment修正amoeboid mo阿米巴式运动amorphous s无定形状态amount of e能量amount of e蒸发量amplificati放大amplificati放大极限频率amplifier放大器amplitude振幅amplitude c脉冲幅度限幅amplitude c幅角余弦amplitude d振幅甄别器amplitude d波幅畸变amplitude f幅频图amplitude f振幅函数amplitude l幅值轨迹amplitude m爹amplitude r振幅共振amplitude r振幅响应amplitude s振幅选择器amplitude s振幅谱amplitude s幅度变动anabatic fr上升锋面anabatic wi上坡风anafront上滑锋anallobar气压上升区analog模拟analog digi模拟数字计算机analog meth模拟法analogue模拟analogue co模拟计算机analogue me相似法analogy模拟analogy of 磁场相似analysis分析学analysis of振荡分析analytic解析的analytic tr解析变换analytical 分析力学anchoring系泊anelastic m滞弹性材料anemogram风力自记曲线anemograph风速计anemometer风速表anemoscope风向仪angle modul相角灯angle of ad超前角angle of ar到达角angle of at迎角angle of ba倾斜角angle of ca毛细角angle of co接触角angle of co切线角angle of cr交叉角angle of de偏斜角angle of de起飞角angle of di畸变角angle of do下洗角angle of el仰角angle of em出角angle of fl襟翼偏转角angle of fl燎angle of fr摩擦角angle of im碰撞角angle of in倾角angle of in内摩擦角angle of re静止角angle of ro转动角angle of si侧滑角angle of sl倾斜角angle of tw扭转角angle of ya偏航角angle pipe弯管angle prese保角映射angle strag角离散angle varia角变量angular acc角加速度angular coe角系数angular coo角坐标angular cor角相关angular der角偏向损失angular der角微商angular dis角分散angular dis角位移angular dis角距angular dis角分布angular div角分度angular fre角频率angular imp角冲量angular mom角动量angular mom角动量守恒定律angular mom角动量算符angular mom角动量张量angular mom角动量定理angular mot角运动angular sep方向夹角angular spe角速度angular uni角单位angular var角变量angular vel角速度anharmonic非低的anharmonic 非谐振动anharmonic 非谐振子anharmonic 非低比anisentropi非等熵怜anisotherma非等温渗透流anisotropic蛤异性的anisotropic蛤异性体anisotropic蛤异性液体anisotropic蛤异性材料anisotropic蛤异性岩石anisotropic蛤异性湍流anisotropy蛤异性anisotropy 蛤异性常数anisotropy 蛤异性比annular flo环流annular foc环形焦点annular mis环形雾状流anomalous a异常吸收anomalous d反常扩散anomalous s反常散射anti diffus反扩散anticlockwi反时针方向旋转anticoincid反重合anticoincid反符合法anticycloly反气旋消散anticycloni反气旋涡度antinode波腹antipodal s对映空间antiresonan反共振antiresonan反共振频率antisymmetr反对称张量antisymmetr反对称态antisymmetr反对称antitriptic减速风aperiodic非周期的aperiodic d非周期衰减aperiodic m非周期运动aperiodicit非周期性aperture孔径aperture ra孔径比aphelion远日点apocenter远心点apogee远地点apolar无极的apolune远月点apparent ab表观吸收系数apparent co表观粘力apparent di表观扩散率apparent el表观弹性极限apparent en表观能量apparent eq表观平衡apparent ex表观膨胀apparent fo表观力apparent mo表观弹性模量apparent ph表观相角apparent ve表观速度apparent vi表观粘度apparent wo表观功appearance 疲劳现象appell equa阿佩尔方程application酌点applied ela应用弹性学applied mec应用力学approach ve驶近速度approximate近似解析approximate近似模型approximate近似相似approximate近似模拟approximate近似解approximati近似approximati近似计算approximati拉格朗日函数近似approximati近似理论approximati近似值arbitrary u任意单位arch拱arch bridge拱桥arch dam拱坝arch gravit拱形重力坝arch struct拱形结构arch truss拱形桁架arched beam拱形梁arched gird拱形梁archimedes 阿基米德原理area curve面积曲线area load表面负载area moment面积矩area of con接触面积area ratio面积比areal accel面加速度areal coord面积坐标areal veloc面积速度areometer比重计arm边arm of coup力偶臂arrest制动器arrhenius e阿雷尼厄斯方程arrhenius l阿雷尼厄斯定律artesian he自廉头artesian pr自廉压artesian wa自廉artesian we自廉artificial 人工压缩artificial 人造地球卫星artificial 人工地震artificial 人造重力artificial 人工扰动artificial 人造卫星artificial 人工通风ascending a向上气流ascending m上升运动ascending s上行冲程ascension升起aseismic耐震的ash ejector吹灰器排灰器aspect rati展弦比assembly装配associate c结合累积associated 附加质量associated 缔合波astromechan天体力学astronaviga天文导航astronomica天文方位角astronomica天文单位asymmetric非对称的asymmetrica非对称性分布asymmetrica非对称负载asymmetry非对称性asymptotic 渐近模型athodyd冲压喷气发动机atmosphere大气atmospheric大气边界层atmospheric大气飞行atmospheric大气湿度atmospheric大气层atmospheric大气湿度atmospheric大气扰动atmospheric大气压atmospheric气压图atmospheric大气下沉atmospheric大气温度atmospheric大气湍流atmospheric大气波atom collis原子碰撞因子atomic blas原子爆炸atomic crac原子龟裂atomic dist原子间距atomic ener原子能atomic ener原子能级atomic hard原子硬化atomization喷雾化atomization雾化压力attachment附着attenuation衰减attenuation衰减振动attenuator衰减器attitude姿态attraction吸引attraction 引力势attractive 引力中心attractive 引力audibility可听性augmentatio增加augmenter加速室auto spectr自乘谱autocontrol自动控制autocorrela自相关autoexcitat自激autogeneous自点火autogiro自动陀螺仪automatic c自动控制系统automatic c自动控制理论automatic d计算机辅助设计automatic f自动频率控制automatic p自动编程序automatic r自动第器autonomous 自治系统autonomous 自激振荡autopilot自动导航装置autorotatio自转available e有效能available h有效水头available h有效热average平均;平均的average acc平均加速度average cur平均曲率average dev平均加速度average dia平均直径average err平均误差average gra平均坡度average lif平均寿命average loa平均负载average pot平均势average pow平均功率average pre平均压力average qua平均量average spe平均速度average tem平均温度average val平均值average vel平均速度averaged or平均轨迹元averaging取平均值averaging m平均方法avertence偏斜axes of coo座标轴axial appli轴向施加力axial compr轴向压缩axial elong轴向伸长axial flow轴流轴向气流axial flow 轴撩axial force轴向力axial impac轴向碰撞axial load轴向荷载axial magni轴向放大率axial mode轴向模axial momen轴向力矩axial momen轴惯性矩axial pitch轴向节距axial press轴向压力axial press轴向压力负载axial stres轴向应力axial symme轴对称axial tensi轴向拉力axial thrus轴向推力axial turbi轴两涡轮axial vecto轴矢量axially sym轴对称流轴对称怜axiom of co约束公理axiomatics公理系统axipetal向心的axis of abs横坐标轴axis of cen弯曲中心轴axis of cur曲率轴axis of ord纵坐标轴axis of rev回转轴axis of rot转动轴axis of sym对称轴axis of tra牵引轴axisymmetri轴对称的axisymmetri轴对称单元axisymmetri轴对称流axisymmetri轴对称问题axle load轴负载azimuth ang方位角back flow回流逆流back mixing反混back pressu背压力back reacti逆反应back substi回代backpressur反向压力;反压力backscatter逆散射法backstreami逆流backward di后向差分backward mo逆向运动backward pr逆旋进backward th逆推力backward wa逆向行波backwash后涡流backwater回水backwater c回水曲线backwater d回水距离backwater f回水函数backwater s回水面backwater s回水浪baer law巴尔定律baeyer stra贝耶尔应变理论balance平衡balance con平衡条件balance equ平衡方程balance for平衡力balance wei平衡锤balanced lo平衡负载balanced sy平衡力系balancing平衡balancing f补偿力balancing i本机平衡balancing m平衡试验机balancing m平衡法balancing s平衡速率ball suppor球面支座ballast压载物ballistic弹道的ballistic c弹道系数ballistic c弹道常数ballistic c弹道曲线ballistic d弹道偏差ballistic e冲惑差ballistic p冲悔ballistic r弹道火箭ballistic t弹道轨迹ballistic w弹道波ballistics弹道学balloon气球balloon bor气球发射火箭banded stru带状结构bandwidth带宽bank侧向倾斜bar巴bar constru棒构造baric topog气压形势baric wave气压波barocline斜压baroclinic 斜压铃baroclinity斜压性barodynamic重结构力学barograph气压计barometer气压计barometric 气压高度barometric 气压柱barometric 气压梯度barometric 气压高度barosphere气压层barotropic 正压方程barotropic 正压平衡barotropic 正压流barotropic 正压铃barotropic 正压不稳定barotropy正压性barrel vaul圆柱壳barrier障碍barycenter重心barycentric重心的barycentric重心坐标barycentric重心系barycentric重心速度base flow底流base pressu底面压力basic equat基本方程basic load基本载荷basin硫basset forc巴塞特力bathyal reg半深海区bauschinger包辛格效应beach海岸beam梁beam axle梁式轴beam balanc杠杆秤beam column梁杆beam struct梁结构beam suppor简支梁beam truss桁架梁beam with v变截面梁bearing方位;轴承bearing cap承载力bearing fri支承摩擦bearing fri轴承摩擦损失bearing lin方位线bearing loa轴承荷载bearing pow承重能力bearing pre支承压力bearing str承载应变bearing str承载强度bearing str承载应力bearing sur支承面beat差拍beat freque拍频beat period拍频周期bed load推移质beginning o曲线起点behavior行为bell pressu钟型压力计bell type m钟型压力计bellows波纹管belt tensio皮带张力bend test弯曲试验bending弯曲bending cen弯曲中心bending fat弯曲疲劳极限bending lin弯曲线bending loa弯曲载荷bending mom弯矩bending mom弯矩密度bending mom弯矩图bending rad弯曲半径bending rig抗弯刚度bending str弯曲应变bending str抗弯强度bending str弯曲应力bending tes弯曲试验bending vib弯曲振动bending wav弯曲波beneding st挠应力bernoulli c伯努利常数bernoulli e伯努利方程bessel func贝塞耳函数beta ratio比压biaxial str双轴向应力bifurcated 分岔激波bifurcation分支bifurcation分支点biharmonic 双谐函数bilateral双向的bilateral c双边约束billow大浪bimoment双力矩bimotor双发动机飞机binary coll双碰撞binary diff二元扩散系数binder结合剂binding age结合剂binding ene结合能binding for结合力bingham bod宾汉物体bingham flo宾汉怜bingham flu宾汉铃bingham mod宾汉模型binodal sei双节点驻波振荡biological 生物学相似性biomechanic生物力学biomechanic骨力学biomechanic运动生物力学biorheology生物龄学biot savart毕奥 萨伐尔定律biplane双翼飞机bipolar coo双极坐标birefringen双折射bivector双矢black body绝对黑体;黑体black body 黑体辐射blade叶片blade effic叶片效率blade exit 叶片出口角blade grid叶栅blade inlet叶片入口角blade loss叶片损失blade outle叶片出口角blade pitch叶片距blading叶片装置blast送风blast nozzl喷气嘴blast of wi阵风blast press风压blast tuyer风口blast volum风量blasting爆破blasting ch起爆室blasting ef爆破效率blimp软式飞艇blocking ph堵塞现象blood flow血流blood visco血液粘弹性blood visco血液粘度blower吹风器blown flap吹气襟翼bluff body钝体blunt body钝头体body centro本体极迹body fixed 物体固定坐标系body force体力body of rev旋转体boger fluid保格铃boiling沸腾boiling poi沸点boiling tem沸点温度boltzmann c玻耳兹曼常数boltzmann d玻耳兹曼分布boltzmann f玻耳兹曼因子boltzmann h玻耳兹曼 h 定理boltzmann l玻耳兹曼定律boltzmann t玻耳兹曼输运方程bolus flow团流bond energy结合能bond streng附着强度bonding for耦合力boost升压booster加速器bootstrap d靴袢动力学border边缘borderline边线bore内径borehole钻孔born green 玻陡窳址匠眺born von ko玻斗肟 呓缣跫 bottom chor底弦bottom curr底流bottom stan底层驻波bottom velo底层速度bouguer wav布格波数bounce回跳bound energ束缚能bound state束缚态bound vecto束缚矢量bound vorte约束涡boundary边界boundary co边界配置boundary co边界条件boundary ef边界效应boundary el边界元法boundary fr边界摩擦boundary la边界层boundary la边界层控制boundary la边界层方程boundary la边界层怜boundary la边界层法boundary la边界层区域boundary la边界层分离boundary la边界层厚度boundary la边界层转捩boundary me边界解法boundary of气团的边界boundary pa边界部分boundary po边界点boundary su边界面boundary va边界值boundary va边界值问题boundary wa界面波bourdon gag布尔登压力计boussinesq 布辛涅斯克近似boussinesq 布辛涅斯克运动方程bow船首bow shock w头波bow wave头波brachistoch最速降线bracket悬臂梁brake制动器brake horse制动马力brake parac制动伞brake power制动功率brake press闸压力brake test闸试验braking制动braking dis制动距离braking for制动功率braking mom制动力矩braking roc制动火箭break throu穿透breakaway分离breakdown t断裂试验breaker破浪breaking an断裂角breaking el断裂伸长breaking lo断裂负截breaking of海浪断裂breaking po断点breaking st断裂应变breaking st裂断强度breaking st破坏应力breaking te致断试验breaking wa碎波breaking we断裂负截bridge trus桥桁架brinell har布氏硬度brinell har布里涅耳硬度试验brittle脆的brittle beh脆性行为brittle coa脆性涂层brittle cre脆性蠕变brittle fra脆裂brittle mat脆性材料brittle str抗脆裂强度brittleness脆性broken line折线brownian mo布朗运动brunt vaisa布伦特 韦伊塞拉频率bubble气泡bubble cent气泡中心bubble clou气泡云bubble dens气泡密度bubble doma泡畴bubble flow泡状流bubble form气泡形成bubble of t湍联bubble pres泡压bubbling气泡形成bubbling fl鼓泡怜床bucket叶片buckingham 伯金汉姆势buckling屈曲buckling be翘曲行为buckling co屈曲系数buckling lo屈曲负载buckling of板的翘曲buckling of壳的皱损buckling st抗屈曲强度buckling st屈曲应力buckling te纵弯试验buffer缓冲器减震器buffer acti缓冲酌buffer beam缓冲梁buffer solu缓冲溶液buffer spri缓冲弹簧buffeting扰炼震built in ar固定拱bulge隆起bulk accele牵连加速度bulk forces体积力bulk modulu体积弹性横量bulk modulu体积弹性横量bulk motion牵连运动bulk potent体积势bulk resona体积共振bulk scatte体积散射bulk strain体积应变bulk temper总体温度bulk veloci牵连速度bulk viscos体积粘度buoyancy浮力buoyant fre浮力频率burgers mat伯格斯材料burning燃烧burning loa燃烧负荷burning poi燃点burning tem燃点burning vel燃烧速度bursting爆裂bursting pr爆炸压力bursting st破裂强度bursting st破裂应力bursting te爆破试验busemann re布泽曼关系式cable staye斜拉桥cad计算机辅助设计calculator计算机calculus of近似计算calculus of差分演算calibration校准calibrator校准器calorific c热容量calorimetri量热测量camber chan改变机翼弯度的襟翼canal管道canal vorte沟渠涡旋canal wave沟渠波canard鸭翼canonical c正则坐标canonical d正则分布canonical e正则运动方程canonical e正则方程canonical f正则形式canonical m正则动量canonical t正则变换canonical v正则变量cantilever悬臂梁caoutchouc 橡胶弹性capacitive 电容传感器capacity功率capacity me容积量度capacity st电容应变计capillarity毛细现象capillary a毛细管吸收capillary a毛细酌capillary a毛细引力capillary c毛细凝缩capillary c毛细常数capillary e表面张力能capillary f毛细裂纹capillary f毛管流capillary f毛细力capillary g毛细重力波capillary l毛细面振动capillary p毛细现象capillary p毛细压力capillary r毛细升高capillary t毛细张力capillary t毛细管capillary v毛细管粘度计capillary w毛细波capture俘获carbon fibe碳纤维cardan angl卡登角cardan ring卡登环cardiac dyn心脏动力学cardiac wor心脏的工作cargo货物carrier gas气体载体carrier ine牵连惯性力carrier liq载体液体carrier osc载波振荡carrier roc运载火箭carrier vel牵连速度carrying ca负荷量cartesian c笛卡儿坐标cartesian v笛卡儿矢量cascade exc级联激发cascade flo翼栅怜cascade of 翼型叶栅cascade tun叶栅风洞case depth渗碳层深度case harden表面硬化casing外壳castigliano卡斯蒂利亚诺定理casting str铸造应力catapult弹射器catenary悬链线catenoid悬链曲面cauchy defo柯挝变张量cauchy equa柯嗡动方程cauchy inte柯锡分定理cauchy law 柯梧似性定律cauchy resi柯涡数定理cauchy riem柯卫杪 匠眺cauchy stre柯桅力张量caudad acce尾向加速度causality因果律caving空泡形成cavitating 气穴流涡空流cavitation气蚀现象cavitation 空泡cavitation 空化损坏cavitation 空化效应cavitation 空蚀cavitation 空化核cavitation 空化数cavitation 空化参数cavitation 气蚀现象cavitation 气蚀冲击cavitation 空泡试验筒cavity空腔cavity coll空泡破裂cavity drag空泡阻力cavity flow气穴流涡空流cavity flow空泡另论cavity form空泡形成cavity pres空腔压力cavity reso空腔共振器cavity vibr共振腔振动ceiling上升限度celestial m天体力学cell model笼子模型cell reynol网格雷诺数cellular gr网状栅格cellular st栅格结构center中心center line中心线center line中线平均高度center line杆件轴线center of a面积中心center of b浮心center of c曲率中心center of g重心center of i惯性中心center of i惯性中心坐标系center of i反演中心center of m质心center of m质心坐标系center of m质心定理center of m质心运动center of m质心坐标系center of m质心定理center of o震荡中心center of p撞恍心center of r转动中心center of s相似中心center of t扭转中心center of v涡心center to c中心距center to c中心距centered co有心压缩波centered ra有心稀疏波centi厘centigrade 摄氏温度centimetre厘米centimetre 厘米 克 秒central中心的central ang中心角;圆心角central axi惯性中心轴central bod中枢体central dif中心差分法central for有心力central for有心力场central gas中心气流central imp对心碰撞central lim中心极限定理central lin中线central mom中心力矩central poi中心点central pot有心力势central pri中心惯性轴central pri中心知动惯量centre中心centre of b浮心centre of c曲率中心centre of f力心centre of g重心centre of g旋转中心centre of i质心centre of m质心centre of m质心运动centre of m质心系centre of p撞恍心centre of p压力中心centrifugal离心的centrifugal离心加速度centrifugal离心效应centrifugal离心力场centrifugal离心力centrifugal离心式蒂器centrifugal惯性离心力centrifugal离心荷载centrifugal离心摆centrifugal离心势centrifugat离心分离centrifuge离心机centrifuge 离心模拟centripetal向心的centripetal向心加速度centripetal向心力centrode瞬心轨迹centroid质心centroid ax重心轴线centroid of面心centroidal 重心诌性轴centrosymme中心对称的cgs system c gs 单位制chain drive链条传动chain graph链图chain react连锁反应chain trans链条传动chance even随机事件chance quan随机量chance vari随机变量change变化change of c坐标变换change of f形态变化change of s物态变化change of t变数更换change of t风向改变changing lo交变负载channel渠道channel dif通道扩散channel flo渠道怜chaos混沌chaplygin c恰普雷金对应原理chaplygin e恰普雷金方程chaplygin f恰普雷金铃chaplygin f恰普雷金函数chapman ens查普曼 豆科格方法chapman ens查普曼 豆科格解chapman jou查普曼 儒盖条件chapman jou查普曼 儒盖假设chapman kol查普曼 柯尔莫果洛夫方程chapman lay查普曼层characteris特性characteris特性曲线characteris特盏characteris特招列式characteris特者图characteris爱因斯坦特章度characteris特哲量characteris特性方程characteris特盏率characteris特寨数characteris特蘸压数characteris特栅度characteris特性线characteris特肇阵characteris特性参数characteris特战面characteris特性量characteris特怔面characteris特性浪涌阻抗characteris特章度characteris特盏characteris特崭量characteris特召度characteris特炸长characteris特性charge装药charpy impa却贝冲辉验机charpy impa却贝冲辉验chatter amp颤动幅度chatter mar振颤痕chatter vib颤动chattering颤动check校验check analy检验分析chilling冷硬choke扼力choke free无阻塞的choked nozz壅塞式喷管choked wind阻塞式风洞choking壅塞choking coi扼力choking mac壅塞马赫数choking reg壅柳choking vel壅塞速度chopped wav斩波choppy sea三角浪chord翼弦chord lengt弦长chronometry测时ciliary mot纤毛运动circle erro陀螺盘旋误差circle of c曲率圆circle of i惯性圆circle of i挠曲圆circular co圆锥circular cy圆柱circular fu圆函数circular mo圆周运动circular or圆形轨道circular pe圆摆circular sh圆轴circular tu圆管circular ve圆速度circular vi圆振动circular vo圆涡circular vo圆涡circular wa圆波矢量circular wh圆涡circulating循环空气circulating环流circulating循环水circulation循环circulation环林支circulation环恋数circulation环粒数circulation无环聊circulation环粮数circulation环零circulation循环管路circulation矢量场环路circulation水循环circulation环良circulation环量守恒运动circulation循环过程circulation环哩理circulation循环水槽circulator 扇形circulatory环流circulatory循环积分circulatory环了动circulatory循环系统circumferen圆周circumferen周缘分量circumferen周向circumferen切向力circumferen周向速率circumgloba球面总辐射circumgyrat回转circumpolar环极涡旋circumspher外接球cistern bar液槽气压计civil engin土木工程clamped bea固支梁clapeyron e克拉佩隆弹性体clapotis驻波clapp oscil克拉普振荡器classical m经典力学classical p经典物理学classical s经典统计力学classical t经典概率论classical t经典热力学clausius cl克劳修斯 克拉佩隆方程clausius cl克劳修斯 克拉佩隆方程clausius du克劳修斯 迪昂不等式clausius pl克劳修斯 普朗克不等式clearance间隙cleavage劈裂cleavage cr解理裂纹cleavage fr解理断裂cleavage fr解理断裂强度cleavage pl裂开面cleavage st解理强度cleft裂纹cleft famil裂口族cleftiness裂隙climbing攀移climbing sp上升速率clinoaxis斜轴clipper快速帆船clockwise顺时针方向的clockwise r顺时针旋转closed loop闭环closed shel闭壳closed syst闭合系closed wind闭式回羚洞closing pre关闭压力co oscillat共振潮co oscillat合振荡co tidal li等潮线coagulation凝聚coalescence聚并coanda effe柯安达效应coasting mo惯性运动coating thi涂层厚度cockpit驾驶舱coefficient放大系数coefficient表观膨胀系数coefficient压缩系数coefficient凝结系数coefficient固结系数coefficient收缩系数coefficient体胀系数coefficient扩散系数coefficient弥散系数coefficient湍脸性系数coefficient弹性系数coefficient伸长系数coefficient膨胀系数coefficient摩擦系数coefficient热传导系数coefficient传热系数coefficient浸渗系数coefficient内摩擦系数coefficient动摩擦系数coefficient线伸长系数coefficient相互扩散系数coefficient枢轴摩擦系数coefficient加压扩散系数coefficient回归系数coefficient阻力系数coefficient恢复系数coefficient滚动摩擦系数coefficient滑动摩擦系数coefficient稳定性系数coefficient静摩擦系数coefficient表面膨胀系数coefficient导热系数coefficient热膨胀系数coefficient透射系数coefficient粘性系数coherence d相干距离coherence f相干函数coherence l相干距离coherence s相干散射coherent st湍拎干结构coherent wa相干波cohesion内聚cohesion fo内聚力cohesion pr内聚压力cohesion st内聚强度cohesionles无粘聚性土cohesive en内聚能cohesive so粘聚性土coiled spri螺旋形弹簧cold bendin冷弯曲试验cold brittl冷脆性cold crack冷裂纹cold cuttin冷切cold deform冷变形cold drawin冷拉cold energy冷能cold flow冷流cold plasma冷等离子体cold pressu冷压cold rollin冷轧cold wave冷波cold workin冷加工collapse崩溃collapse me破坏机构collapsible可折皱管collapsing 破坏力collar vort涡环collateral 次级运动collective 集体运动模式collective 集体运动collimation视准collision碰撞collision c碰撞链collision c碰撞截面collision d碰撞直径collision d碰撞扩散collision e碰撞激发collision f碰撞频率collision h碰撞加热collision i碰撞积分collision i碰撞不变量collision l碰撞定律collision l碰撞损失collision m碰撞矩阵collision m碰撞平均自由程collision o第一类碰撞collision o第二类碰撞collision p碰撞参数collision p碰撞点collision r碰撞半径collision s碰撞强度collision t碰撞理论collisionle无碰撞的collisionle无碰撞冲花colloid胶体colloid osm胶体渗透力colloidal p胶粒colloidal s胶体溶液colloidal s胶态colloidal s胶状结构colloidal s胶质column柱column vect列矢量combination组合combination组合共振combination组合振动combined lo复合载荷combined mo组合模型combined mo复合运动combined st复合应力combustible可燃物combustion燃烧combustion 燃烧曲线combustion 燃烧前沿combustion 燃烧热combustion 燃烧率compactibil可夯实性compaction压实comparative比较实验comparative比较测量comparative比较量compatibili相容性方程compatibili相容性方程compensated补偿摆compensated补偿粘度计compensatin补偿运动compensatin补偿槽compensatin补偿水槽compensatio补偿compensatio误差补偿compensatio补偿管线compensator补偿器compensator补偿压力complementa附加加速度complementa余能量complete eq完全平衡complete ha总哈密顿算符complete in完全积分complete mo完整模型complete re整转complete si完全相似complete si完全模拟complex复合complex amp复振幅complex ben复合弯曲complex imp复数阻抗complex mag复磁雷诺数complex mod复模态complex mod复模量complex num复数complex osc复合振荡complex pla复平面complex pot复势complex str复合应力complex tra复合变换complex tru复式桁架complex var复变数法complex vel复数速度势complex vis复粘度compliance顺度compliance 柔度法component成分component f分力component m运动分量composite复合物composite b混合梁composite m复合材料composite m复合运动composite r多级火箭composite s复合谱composite s集合强度composite s复合应力composite t复合潮composite w复合波composition合成composition加速度合成composition力的合成composition运动的合成composition速度的合成compound cu复合曲线compound gi合成梁compound pe复摆compressed 压缩空气compressed 压缩气体compressibi压缩性compressibi压缩性效应compressibi压缩因数compressibi压缩性积分compressibi铃压缩性compressibi土的压缩性compressibi介质可压缩性compressibl可压缩的compressibl可压缩边界层compressibl可压缩效应compressibl可压缩流compressibl可压缩铃compressibl可压缩铃射流compressibl可压缩参数compression压缩compression压缩锥compression压缩蠕变compression压缩曲线compression压缩效率compression压缩成形compression压缩断裂compression压缩加热compression压缩指数compression压缩损失compression压缩马赫波compression压缩压力compression压缩比compression压缩激波compression压缩速率compression抗压缩度compression压缩应力compression压缩试验compression压缩时间compression压缩波compression压缩功compression压缩膨胀波compression压缩振荡compression抗压刚度compressive压杆compressive压弯杆compressive压缩变形compressive压缩力compressive压缩载荷compressive压缩合力compressive压缩变形compressive压缩应力compressive抗压屈服点compressor 压气机功率computation计算computation计算动力学computation计算铃力学computation计算力学computation计算误差computation计算图式computation计算结构力学computer计算机computer ai计算机辅助设计computer si计算机模拟computing e运算元件computing t计算时间concentrate集中常数concentrate集中力偶concentrate集中力concentrate集中负载concentrate集中涡旋concentrate集中涡旋concentrati浓度concentrati浓度相关的concentrati浓度差concentrati浓度效应concentrati浓度方程concentrati集中系数concentric同心的concept概念concurrent 汇交力concussion 地面振动condensate冷凝物condensatio冷凝腔condensatio凝结热condensatio凝聚高度condensatio凝聚点condensatio冷凝物condensatio凝结速度condensatio凝结激波condensatio凝结理论condensed f冷凝液体condensed m冷凝物质condensed p凝聚相condensed s凝聚状态condition f平衡条件condition o等熵条件conditional有条件的conditional条件方程conditional条件稳定性conditionin第conductivit电导率cone of she切应力锥configurati构型configurati配置改变configurati位形配分函数confinement封闭confocal pa共焦抛物面坐标conformal c保角坐标conformal m保角映射congruent t全等变换conical flo锥形怜conical pen锥摆conical she锥壳conical sho锥形激波coning锥旋conjugate共轭的conjugate a共轭轴connecting 连结棒connection结合conservatio守恒conservatio守恒场conservatio守恒定律conservatio角动量守恒conservatio能量动量守恒conservatio陀螺定轴性conservatio质量守恒conservatio机械能守恒conservativ保守力conservativ保守力场conservativ守恒运动conservativ守恒系。
丽声英语百科分级读物 things with wings
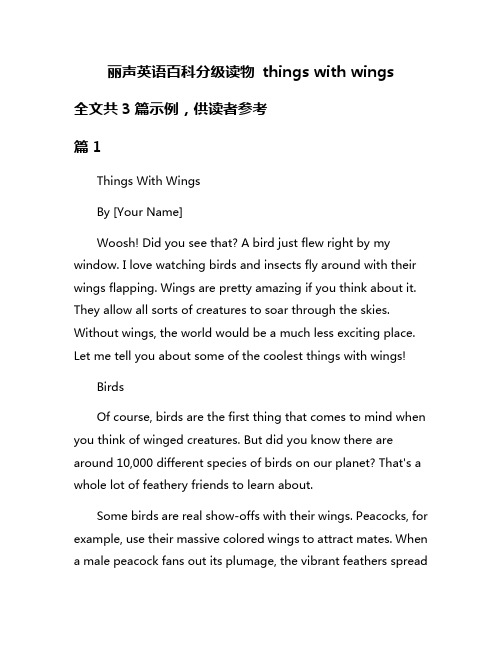
丽声英语百科分级读物things with wings全文共3篇示例,供读者参考篇1Things With WingsBy [Your Name]Woosh! Did you see that? A bird just flew right by my window. I love watching birds and insects fly around with their wings flapping. Wings are pretty amazing if you think about it. They allow all sorts of creatures to soar through the skies. Without wings, the world would be a much less exciting place. Let me tell you about some of the coolest things with wings!BirdsOf course, birds are the first thing that comes to mind when you think of winged creatures. But did you know there are around 10,000 different species of birds on our planet? That's a whole lot of feathery friends to learn about.Some birds are real show-offs with their wings. Peacocks, for example, use their massive colored wings to attract mates. When a male peacock fans out its plumage, the vibrant feathers spreadout into a dazzling display. Other birds like swans and geese have powerful wings that allow them to fly incredibly long distances during migration season.My personal favorite winged buddies are hummingbirds. These tiny marvels can flap their wings over 50 times per second! That's the fastest wing-flapping of any bird. Hummingbirds are the only birds that can hover in place and even fly backwards. Their wings are specially adapted to allow such aerial acrobatics.InsectsWhile not as obvious as birds, so many insects have wings too. In fact, there are way more species of winged insects than there are birds. Butterflies, bees, flies, dragonflies, moths, and more - the insect world is full of amazing little aviators.Butterflies have to be some of the most beautiful winged insects around. Their colorful, patterned wings almost look like miniature stained glass windows. Some butterfly species migrate hundreds of miles each year using their delicate wings to travel. Can you imagine flying that far when you only weigh as much as a paperclip?Another super cool insect is the dragonfly. These guys are basically the fighter jets of the insect kingdom! Dragonflies canfly straight up and down, hover like helicopters, and even mate while flying. Their two pairs of powerful wings allow them to be some of the fastest insects in the air. Just don't get too close - those jaws pack a punch if you bother a dragonfly!BatsYou might be surprised to learn that bats are the only mammals that can truly fly. While flying squirrels and other animals can glide from place to place, bats are the masters of powered flight in the mammal world thanks to their wings.A bat's wings are pretty weird when you think about it. They are made from a double layer of skin that is stretched across the bones of the bat's hands and arms. Can you imagine having wings made out of skin stretched between your finger bones? Weird, right?Even weirder is how bats can fly equally well whether they are upside down, sideways, or right-side up. Their wings and aerodynamic bodies allow bats to be the most agile and maneuverable flyers in the skies. As if that's not enough, many bats also use echolocation (like sonar) to navigate and hunt insects in total darkness just by listening for echoes. Now that's an impressive set of wings!Flying ReptilesSadly, there are no more flying reptiles roaming the skies today. Pterosaurs like the famous pterodactyl went extinct around 66 million years ago. But these amazing winged lizards ruled the prehistoric skies back when dinosaurs walked the Earth.Pterosaurs had wings formed by a massive stretching of the skin membrane between their incredibly elongated fourth fingers and their bodies. Some pterosaurs had wingspans as big as a giraffe is tall! Just imagine a dragon-like creature that large soaring overhead. No wonder ancient humans were so terrified of these beasts.While they are long gone now, paleontologists continue to study fossils of pterosaurs to learn more about these first vertebrates to evolve the ability of true powered flight. Maybe one day scientists will even bring them back to life using DNA from fossils. A real life Jurassic World with flying reptiles? Now that would be amazing!The Awesome Ability of FlightWhether it's a majestic bald eagle, a busy little honeybee, or a zipping hummingbird, all of these incredible creatures share the awesome power of being able to fly. Their wings allow themto soar high above the ground, travel long distances, escape predators, and more.Just think how limiting life would be if we only had wings for swimming through the water or crawling across land. Wings have unlocked the skies for so many amazing lifeforms. The world is an infinitely more vibrant, varied place because of all the creatures with wings sharing the air around us.Next time you see a bird effortlessly gliding by or a dragonfly zipping across a pond, stop for a moment and appreciate the wonder of wings. We are so lucky to share a planet with such beautiful, graceful, gravity-defying animals. Their wings have made the impossible possible in nature's wildest dreams. Flying truly is one of life's most liberating superpowers!篇2Things With WingsBy [Your Name]Have you ever watched a bird soaring high in the sky and wished you could fly too? Or marveled at the colorful wings of a butterfly fluttering from flower to flower? There's something magical about winged creatures that has captivated humans forcenturies. In this essay, we'll explore some of the amazing things that possess wings and the incredible abilities they provide.Birds - The Masters of the SkiesWhen we think of winged animals, birds are usually the first that come to mind. With over 10,000 different species, birds are incredibly diverse and can be found on every continent. Their wings, made up of feathers, allow them to do something that most other animals cannot - fly.From the mighty eagle soaring over mountains to the tiny hummingbird hovering to sip nectar from a flower, the ability of birds to take to the air is truly remarkable. Their wings, combined with their lightweight, hollow bones, make them perfectly adapted for flight.But birds don't just use their wings for flying. They also serve other purposes, such as helping them steer while in the air, attracting mates with colorful plumage, and even swimming in the case of some waterfowl like ducks and geese.Insects - The Tiny Winged WondersWhile not as well-known as birds, insects make up the majority of winged creatures on our planet. From the delicatebutterfly to the industrious bee, these tiny creatures have evolved an incredible diversity of wing types and flying abilities.Many insects, such as butterflies and moths, have two pairs of wings covered in colorful scales. Others, like dragonflies and damselflies, have two pairs of transparent wings that allow them to hover and dart through the air with incredible agility.Insect wings don't just enable flight, they also play important roles in communication and survival. For example, some moths use their wings to produce ultrasonic sounds that deter predators, while butterflies use their wings to regulate body temperature by basking in the sun or closing them to retain heat.Bats - The Nighttime AviatorsWhile birds and insects are the most well-known winged creatures, there is one group of mammals that has also taken to the skies - bats. These fascinating creatures are the only mammals capable of true, sustained flight.Unlike birds and insects, bats don't have feathers or scales on their wings. Instead, their wings are made up of a thin membrane of skin stretched between their elongated finger bones. This unique wing structure, combined with theirlightweight, furry bodies, allows them to navigate the night skies with incredible precision.Bats use their wings not only for flight but also for a variety of other purposes, such as catching insects, roosting, and even giving birth and nursing their young while hanging upside down.The Importance of WingsWhile winged creatures may seem small and delicate, they play vital roles in ecosystems around the world. Birds and bats, for example, are important pollinators and seed dispersers, helping to spread plants and maintain healthy forests and ecosystems.Insects, too, are crucial for pollination and serve as food sources for many other animals, from birds and bats to lizards and frogs. Without these winged wonders, entire ecosystems could collapse.Wings have also played an important role in human culture and imagination throughout history. From the ancient Greek myth of Icarus to the dreamers who first envisioned human flight, wings have long symbolized freedom, exploration, and the desire to soar above the mundane world.ConclusionWhether you're watching a flock of geese fly overhead in their distinctive V-formation or marveling at the intricate patterns on a butterfly's wings, there is no denying the beauty and wonder of winged creatures. Their ability to take to the skies and navigate the world from above is a testament to the incredible diversity and adaptability of life on our planet.So the next time you see something with wings flitting or soaring by, take a moment to appreciate the incredible evolutionary journey that has allowed these creatures to conquer the air around us. Who knows, maybe one day we too will find a way to spread our wings and join them in their aerial adventures.篇3Things with WingsWhen I was a little kid, I loved watching birds fly through the sky. I would gaze up in wonder at their graceful movements, flapping their wings to propel themselves effortlessly on the breeze. Birds seemed so free and unburdened. I wished I could sprout wings and soar up there with them.As I grew older, I learned that many other creatures besides birds have wings too. Insects like butterflies, moths, bees and flies all have delicate little wings that allow them to flit about.Some of the largest animals on Earth, the flying foxes and other bat species, use wings formed from skin membranes to navigate the night skies in search of food.My fascination with winged beings only grew over time. I yearned to understand what enables these diverse creatures to defy gravity and take to the air. How did wings evolve? What purposes do they serve beyond just flying? I decided to research the incredible world of "things with wings" for my science fair project.Evolution of WingsThe earliest winged creatures were insects that emerged over 400 million years ago during the Devonian period. Fossil evidence suggests wings first formed from extensions of the insect exoskeleton, allowing primitive insects to glide from trees down to the ground.Over millions of years of evolution, wings became more sophisticated as flying insects developed better stabilizers, musculature and aerodynamic forms. This gave many species the ability to truly fly and remain airborne for long periods.Birds are believed to have evolved from feathered,tree-dwelling dinosaurs around 150 million years ago. Initiallyusing feathers for regulating body temperature, some dinosaur species developed longer feathers on their forelimbs that may have aided gliding or short bursts of flapping flight from branch to branch.The wings of bats, a type of flying mammal, evolved much more recently, around 50 million years ago. Stretchy skin membranes developed between their elongated finger bones, allowing them to easily maneuver and hunt insects on the wing at night.Purposes of WingsWhile enabling flight is the most obvious function of wings, these appendages serve many other critical roles. Insect wings help drive air circulation for respiration. Birds use their wings for balance while perched, swimming underwater, and shading their young. Some winged insects like butterflies incorporate bright colors and patterns into their wings to attract mates.The wing venation or patterns of veins across the surface also vary from species to species. In dragonflies, the network of veins near the front of the wing forms a strong leading edge for agile aerial maneuvers. Moths have more dense, furry wings optimized for quiet, stealth flight at night.Aerodynamics & BiomechanicsWhat allows winged creatures to generate enough lift and thrust to become and stay airborne? The physical mechanics involve complex interactions between the shape, movement and surface area of the wings, the strength and flexibility of wing muscles and bones, and the effects of air pressure and aerodynamic forces.The wings of birds are true marvels of natural engineering. The contoured airfoil shape of their wings, coupled with the gentle curvature of the feather vanes, generates incredibly efficient lift. Birds can adjust the geometry and angle of their wings with remarkable dexterity to perform amazing aerial feats.Many insects rapidly vibrate their wings to generate the lift required for powered flight. Some flies can beat their wings an astonishing 1,000 times per second! The corrugated patterns on insect wings also help provide lift at low speeds.I can hardly comprehend the advanced physics and biological mechanics involved in winged flight. All I know is that the sight of birds wheeling across the heavens or a kaleidoscope of butterflies dancing among the flowers fills me with profound awe and wonder at the natural world's ingenuity.Through my research project, I've developed a much deeper appreciation for the remarkable evolutionary journey that enabled creatures to sprout wings and take to the skies over vast eons of time on this planet. What once seemed like a simple anatomical feature is actually an exquisitely refined mechanism, finely tuned by nature's hand over millions of years.If given the chance to ask every winged being one question, I would ask: Does the freedom of flight make up for all the struggles and dangers you face? I imagine they would answer that the ability to soar unfettered through the vast open skies, to experience perspectives unavailable to earthbound creatures, makes every hardship worthwhile.My childlike sense of wonder about winged creatures has never faded. If anything, it has grown even stronger through learning about the intricate brilliance of wing design. I still occasionally gaze up at the birds riding effortlessly on unseen currents of air with a tinge of envy and longing. To truly fly like them, unburdened by gravity's shackles, and with the whole wide world visible below—what could be more freeing than that?。
飞行器稳定性与操纵性(英)_西北工业大学中国大学mooc课后章节答案期末考试题库2023年

飞行器稳定性与操纵性(英)_西北工业大学中国大学mooc课后章节答案期末考试题库2023年1.是飞机横向静稳定性的最大来源。
答案:机翼2.短周期自然频率主要取决于以下哪个参数?答案:3.对于无上反的后掠机翼来说,侧滑角会改变哪些参数?答案:弦向速度_局部动压_展向速度4.为降低操纵力,调整片应与操纵面同向偏转。
答案:错误5.右侧扰流板打开时,飞机会向右滚。
答案:正确6.升力系数越高,后掠角对横向静稳定性的贡献越小。
答案:7.同样的飞机,重心适当后移可使飞机的配平性能提高。
答案:8.对于后掠机翼,左侧滑情况下,右侧机翼动压大于左侧机翼动压。
答案:9.侧洗会垂尾前缘处的侧滑角。
Sidewash will the sideslip angle of the verticaltail leading edge.答案:增大increase10.按照本课程的符号定义习惯(国际坐标系),绕x轴向右滚转为,绕z轴向左偏航为。
According to the sign convention in this course (international coordinate system), roll to the right about the x-axis is , and yaw to the left about the z-axis is .答案:正,负positive, negative11.惯性轴系与地轴系之间相差了一个。
The difference between the Inertialsystem and Earth-fixed system is .答案:地球自转earth rotation12.方向舵偏为正,会产生偏航力矩 Rudder deflect to the is positive, and ayawing moment will be generated.答案:左,左left, left13.以下哪些角度是基础体轴系与风轴系间的夹角?Which of the followingangles are the angles between the basic body Axes System and the Wind Axes System?答案:迎角Angle of attack_侧滑角Sideslip angle14.舰载机在起飞离舰瞬间,升力会突然增加。
- 1、下载文档前请自行甄别文档内容的完整性,平台不提供额外的编辑、内容补充、找答案等附加服务。
- 2、"仅部分预览"的文档,不可在线预览部分如存在完整性等问题,可反馈申请退款(可完整预览的文档不适用该条件!)。
- 3、如文档侵犯您的权益,请联系客服反馈,我们会尽快为您处理(人工客服工作时间:9:00-18:30)。
AEROELASTIC STABILITY OF A FOUR-BLADED SEMI-ARTICULATED SOFT-INPLANE TILTROTOR MODEL Mark W.Nixon1,Chester ngston2,Je rey D.Singleton3,David J.Piatak4,Raymond G.Kvaternik5,Lawrence M.Corso6,and Ross K.Brown71,2,3U.S.Army Vehicle Technology Directorate,ARLMS340,NASA Langley Research Center,Hampton,VA23681USA mark.w.nixon@,ngston@,je rey.d.singleton@4,5Aeroelasticity Branch,NASA LangleyMS340,NASA Langley Research Center,Hampton,V A23681,USAdavid.j.piatak@,raymond.g.kvaternik@6,7Bell Helicopter Textron,Inc.PO Box482,Fort Worth,TX76101,USAlcorso@,rbrown2@Key words:Tiltrotor,Soft-Inplane,Aeroelasticity,Structural Dynamics,IF ASD2003. Abstract.A new four-bladed,semi-articulated,soft-inplane rotor system,designed as a candidate for future heavy-lift rotorcraft,was tested at model scale on the Wing and Rotor Aeroelastic Testing System(WRATS),a1/5-size aeroelastic wind-tunnel model based on the V-22.The experimental investigation included a hover test with the model in helicopter mode subject to ground resonance conditions,and a forwardflight test with the model in airplane mode subject to whirl-flutter conditions.An active control system designed to augment system damping was also tested as part of this investigation. Results of this study indicate that the new four-bladed,soft-inplane rotor system in hover has adequate damping characteristics and is stable throughout its rotor-speed envelope. However,in airplane mode it produces very low damping in the key wing beam-bending mode,and has a low whirl-flutter stability boundary with respect to airspeed.The active control system was successful in augmenting the damping of the fundamental system modes,and was found to be robust with respect to changes in rotor speed and airspeed. Finally,conversion-mode dynamic loads were measured on the rotor and these were found to be significantly lower for the new soft-inplane hub than for the previous baseline sti -inplane hub.4INTRODUCTIONCurrent tiltrotor designs for production aircraft use gimballed sti -inplane rotor systems. Sti -inplane rotor systems are desirable for tiltrotors because in hover there is no concern for ground resonance,and in high-speed airplane mode the stability boundaries associ-ated with whirl-flutter have been established at velocities slightly beyond aircraft power limits,with adequate damping margins at subcritical airspeeds.The disadvantage of a sti -inplane rotor system is that significant inplane dynamic blade loads may develop, particularly during maneuvers.Presented at the2003International Forum on Aeroelasticity and Structural Dynamics,June4-6, 2003,Amsterdam,The Netherlands.This paper is declared a work of the ernment and is not subject to copyright protection in the United States.Soft-inplane rotor systems can greatly reduce the inplane blade loads in tiltrotor aircraft, thereby reducing strength requirements for the hub,leading to reduced structural weight and improved aircraft agility.It is for similar reasons that conventional helicopters with three or more blades have soft-inplane rotor systems.However,soft-inplane rotor systems generally have reduced damping margins and lower stability boundaries than sti -inplane rotor systems.Therefore,before soft-inplane rotor systems can be applied to tiltrotor aircraft,design concepts must be developed which can ensure adequate stability characteristics in both hover and forwardflight.One of thefirst soft-inplane tiltrotor designs to be proposed was the Boeing Model222. Aeromechanical behavior of this soft-inplane hingeless rotor system was addressed in sev-eral experimental and analytical studies using di erent size rotor test apparatus,beginning with a1/10-scale wind-tunnel model described in Ref.1,and ending with a full-scale26-ft.diameter semispan model tested in the NASA Ames40-x80-ft.tunnel described in Refs.2and3.The Boeing soft-inplane design had a relatively high inplane natural fre-quency(about0.9/rev at low airspeeds),such that the design rotor speed in hover mode did not create a ground resonance condition.The only experimental results associated with an instability of this configuration were obtained with the system in airplane mode subject to air resonance conditions.In general,this configuration(in airplane mode) exhibited unacceptably low damping in the wing beam mode at all airspeeds.In2001, a gimballed-hub,soft-inplane rotor system was tested on the WRATS model in hover as described in Ref.4.This rotor system had a fundamental lag frequency of0.5/rev,and hover testing showed that aeromechanical instabilities could occur at rotor speeds well be-low the design rotor speed.As this system exhibited inadequate stability characteristics in hover,it was not tested in airplane mode.More recently,a new full-scale,semi-articulated,soft-inplane rotor was designed by Bell Helicopter as part of the Army Variable Geometry Advanced Rotor Technology(VGART) program.The goals of the Bell VGART study were to satisfy Army Technical E ort Objectives for reduced weight,increased maneuverability,and reduced vibratory loads; and as part of the design to satisfy scalability issues and include growth potential to allow for more than three blades.The new soft-inplane rotor does not have a gimbal, but instead uses a standardflaphinge for the blades.It also adds a highly-damp ed elastomeric-bearing in the blade lag direction(the term semi-articulated is used because the lag mechanism with elastomeric-bearing has both hinge andflexural qualities).The inplane(lag)frequency of the rotor was designed to be in a range of0.55/rev to0.75/rev to maximize the dynamic loads reduction capabilities of the soft-inplane system while retaining feasibility for full-scale application.The new soft-inplane rotor also has four blades,rather than three as used on the previous sti -inplane ing the results from this VGART study,a new1/5-size,four-bladed,soft-inplane hub was designed and fabricated for the WRATS tiltrotor model.The new four-bladed,semi-articulated,soft-inplane rotor system was tested on the Wing and Rotor Aeroelastic Testing System(WRATS),a1/5-size aeroelastic tiltrotor wind-tunnel model based on the V-22.The experimental investigation included a hover test with the model in helicopter mode subject to ground resonance conditions,and a forward flight test with the model in airplane mode subject to whirl-flutter conditions.The ob-jectives of the investigation were to determine the damping margins,stability boundaries, and load reduction factors associated with the new soft-inplane rotor as compared to the current baseline sti -inplane rotor system.Also included as part of this investiga-tion was testing of an active control system designed to augment system damping.Thethree-bladed sti -inplane rotor system(used in several past investigations documented inRefs.5-8)was examined under the same conditions as the four-bladed soft-inplane hub toprovide a baseline for comparison.2APPARATUSThe WRATS1/5-size semi-span tiltrotor model was used as the test-bed for these exper-iments,and the important characteristics of this wind-tunnel model have been describedin several previous reports such as Ref.9,Ref.10,and most recently Ref.5.The wind-tunnel test was performed at the Langley Transonic Dynamics Tunnel(TDT),and thehover test was performed in a30x30-ft.hover cell located in an adjacent high-bay build-ing.While the TDT can use R-134a refrigerant as a test medium,the current experimentwas conducted using air at atmospheric pressures.The key geometric features of the new soft-inplane hub and the baseline sti -inplane hubare illustrated in Fig.1,and several important attributes of these rotor systems are listedin Table1.Some significant features of the soft-inplane hub are a0.5inch pre-lead usedto reduce the steady lag response associated with blade drag,aflap hinge o set of1.76inches(e q=0.039),and a lag pivot point of5.76inches(e l=0.126).The outer pitch bearing is coincident with the lag pivot.As shown,the pitch links have been movedfrom a trailing-blade position on the three-bladed sti -inplane hub to a leading-bladeposition on the four-bladed soft-inplane hub.Both hubs have a nominal geometric B3(pitch-flap skew angle)of about15 ,but for the soft-inplane systemflap-up movementproduces pitch-down rotation while for the sti -inplane hubflap-up movement producespitch-up rotation.The pitch-flap and pitch-lag couplings for the soft-inplane hub weremeasured as a function of blade collective pitch position(collective measured at the75%span station),and are plotted as the e ective geometric B3and B4angles,respectively,inFig.2.The pitch-flap coupling is shown to change rapidly at low collectives where it hasa higher than nominal value,but in the normal collective range associated with airplanemode(20 to50 )the pitch-flap coupling remains in a±1 band about the nominal15value.The pitch-lag coupling is shown to be about9 over the20 to50 collectiverange with about the same deviation band of±1 ,and lag(aft)movement produces apitch-down rotation.The four-bladed,soft-inplane rotor system had two sets of elastomeric dampers thatwere used in the tests so that the e ects of lag mode frequency placement could beexamined(the dampers provide both damping and sti ness to the lag hinge).The softerset of dampers produced a nominal lag mode frequency of0.57/rev while the sti er set ofdampers produced a nominal lag mode of0.63/rev(based on an888RPM design rotorspeed in hover).Only the soft damper set was used in the hover test,while both setswere used in the wind-tunnel test.3HOVER TESTINGThe four-bladed,soft-inplane rotor system was tested in both isolated-rotor and coupled-system configurations.For the isolated-rotor case the pylon was clamped to the rotortest stand such that thefixed-system frequencies were well above the rotor frequenciesof interest,and for coupled-system testing the wing was cantilevered to the test standwith fundamental elastic wing bending modes free to interact with the rotor system.Frequencies of the three key coupled-system modes are plotted as a function of rotorspeed in Fig.3.The three modes are the rotor lag mode(with the0.57/rev damper set installed),the wing beam mode,and the wing torsion/chord(WTC)mode.Coupling between the rotor lag and WTC modes increases as the lag mode frequency(nonrotating frame)approaches the WTC frequency at the upper rotor speed range(lag perturbation stick-stirs produce significant WTC response).Without su!cient damping,this condition will generally result in a ground resonance type instability.The coupled-system damping associated with these three modes is shown in Figs.4,5,and6,where it is seen that there is no instability associated with any of the modes over the rotor speed range tested.A likely reason for these results is the high value of lag mode damping provided by the elastomeric damper as indicated in Fig.4.For the isolated rotor the nominal value for damping is about12%with little variation over rotor speed,while the coupled system the damping is generally higher and varies from12%to18%over the rotor speed range shown.The measured frequency of the rotor lag mode was approximately the same for the isolated and coupled configurations.An important result from the hover testing was that both the measured frequency and measured damping of the rotor lag mode are in close proximity to those expected for full-scale applications of soft-inplane tiltrotor systems.It should be noted when viewingfigures that most plots presented in this paper show error bars.This indicates that a minimum of5data points are available for the indicated condition,with the data point representing the average of the available records and the error bar indicating the standard deviation from the average.In some cases there were less data acquired,and for these cases the individual data records are shown(Figs.5and 6for example).On most plots,faired lines(either linear or cubic spline)are used to help indicate the data trends.The frequency of the WTC mode,which is the key wing mode associated with inplane hub motion and ground resonance behavior in hover,is about5.6Hz and remains steady with respect to rotor speed as shown in Fig.3.Damping of this crucial mode is shown in Fig.5for two collective pitch settings,0 and10 as measured at the75%radial station. As shown,the damping begins at about2%critical in the lower rotor speed range,then falls to a minimum of about1%at800RPM.The soft-inplane system did not encounter an instability under normal operating conditions.In previous studies with a soft-inplane gimballed rotor system(Ref.4)the WTC mode was found to become unstable.Thus,it appears that the new semi-articulated hub design,with use of highly-damped elastomeric materials,provides adequate damping to avoid aeromechanical instability over the design rotor speed range.The wing beam mode in hover is not highly coupled with the rotor lag mode(lag pertur-bation stick-stirs produce little wing beam response),and previous studies indicate that this mode is not likely to become unstable.However,as this is the lowestfixed-system mode(5.4Hz natural frequency)it was monitored carefully throughout the hover test. Fig.6shows the damping associated with the wing beam mode as a function of rotor speed,and indeed this mode is more highly damped than the WTC mode(for l>300 RPM).The damping does,however,decrease with rotor speed from about5%critical at the peak to about2%critical at the upper end of the rotor speed spectrum.Figs.5and6also show that,for the semi-articulated,soft-inplane rotor,the collective pitch setting has little e ect on the WTC and wing beam mode dampings.This is contrary to the behavior observed for the gimballed,soft-inplane rotor system investigated in Ref.4 where the blade pitch setting was found to have a significant impact on damping.Theexact cause of the damping change with collective that was observed in Ref.4has yet to be determined,but may be associated with the particular design and not a general characteristic of gimballed soft-inplane rotor systems.4WIND-TUNNEL TESTINGThe new four-bladed,soft-inplane rotor system,oriented in airplane mode for high-speed wind-tunnel testing,is shown in Fig.7mounted on the WRATS testbed in the NASA Langley Transonic Dynamics Tunnel(TDT).The basic dynamics of the wing/pylon/rotor system shifts substantially with conversion to airplane mode,as the mass o set of the py-lon/rotor moves from above to forward of the elastic axis,and thus creates a significant coupling between the wing beam and torsion modes and the rotor lag mode.The wing chord mode becomes predominantly isolated from these modes in the airplane configura-tion.For airplane-mode aeroelastic stability testing,the rotor system is normally operated windmilling(unpowered and disconnected from the drive system),with the collective blade pitch used to adjust the rotor speed,and a near-zero torque at the rotor shaft.This represents the most conservative manner to test the stability of the system(no damping from the drive system).Under windmilling operation,damping of the key mode associated with system stability(the wing beam mode)was determined to be significantly less for the new four-bladed soft-inplane hub than for the three-bladed sti -inplane(baseline)system, as shown in Fig.8.Damping of the wing beam mode was generally less than1.0%in windmillingflight for all the soft-inplane configurations considered(on-downstop(D/S), o -D/S;0.57/rev dampers,0.63/rev dampers;550,742,and888RPM rotor speeds). Unfortunately,these damping characteristics are inadequate for full-scale operation.In powered-mode(200in-lb torque maintained)the system damping and the stability boundary both increased significantly as illustrated in Fig.9(note on-D/S configuration shown rather than o -D/S as used in Fig.8because of low damping associated with the o -D/S case).Although not a solution for the low-damping behavior associated with the windmilling condition,these results represent a substantial deviation from previous results associated with the baseline system,wherein the e ect of power is not significant with respect to the stability boundary.Fig.10shows that while the subcritical damping values increase significantly with power for the sti -inplane rotor system,the instability condition is about the same.5STABILITY AUGMENTATION TESTINGThe active control system examined in this study incorporates wing-root bending mea-surements(strain gages)for feedback and applies control signals to three independent swashplate hydraulic actuators.The active control algorithm was developed cooper-atively between Bell and NASA Langley,and is based on the Generalized Predictive Control(GPC)theory presented in Refs.11and12.Past studies that have successfully demonstrated the stability augmentation capability of the GPC theory for tiltrotors are documented in Refs.13and14.The GPC active stability augmentation system was highly successful in application to both the new soft-inplane and the baseline sti -inplane rotor systems in high-speedflight. Fig.11shows very significant increases in damping associated with closed-loop control of the baseline system that are extended well beyond the open-loop stability boundary.For the open-loop system the instability occurs at a velocity of about105kts.Closed-looptesting proceeded to a velocity of150kts.before testing was terminated,which is45 kts.beyond the open-loop stability boundary(100kts beyond for full-scale).In fact,the damping of the wing beam mode is shown to be increasing as a function of the airspeed, rather than decreasing,as is the custom for the open-loop system.Similar results were also obtained for the new soft-inplane rotor system as shown in Fig.12,although the system was not tested as far beyond the stability boundary as the baseline system. While not shown on a plot,damping of the wing chord and torsion modes also increased substantially under GPC,otherwise the system would eventually become unstable in these modes.Data were also acquired within the same run at several rotor speeds between550 and888RPM,and the GPC control system was not adversely a ected by these changes in rotor speed.Data from this test show that it is possible to attain the damping levels required for acceptable operation of a soft-inplane rotor system using GPC,and the control system shows robustness with respect to both rotor speed and airspeed deviations.6CONVERSION LOAD MEASUREMENTSThe last objective of this test was to demonstrate the reduction in hub and blade dy-namic loads,which is the key benefit of using soft-inplane rotor systems.Blade and hub loads were measured for a defined set of pylon conversion angles(0,15,30,45,60, 75,and90degrees)and cyclic pitch settings(flapping up to3degrees)in combination, which are designed to simulate tiltrotor free-flight maneuvers.The dynamic loads at each instrumented blade station were measured for each of variousflight conditions,and the maximum sustained dynamic loads(half-peak-to-peak of all conditions considered to-gether)are plotted in Fig.13as a function of span.As expected,the soft-inplane rotor system produces significantly lower dynamic loads.A reduction of approximately50%in the highest(midspan)loads is indicated on the plot.7CONCLUSIONSAn experimental study of a new four-bladed,semi-articulated,soft-inplane hub designed for the WRATS tiltrotor testbed was conducted in hover and forwardflight.Based on results of the tests,the following conclusions are indicated:1.The lag-mode frequency and damping of the new soft-inplane rotor system were measured and found to be representative what can be obtained at full-scale.2.In hover,the new soft-inplane rotor system produced adequate levels of damping throughout the rotor speed spectrum.Ground resonance does not appear be a problem for the current soft-inplane design.3.In windmilling airplane mode,damping levels for the new soft-inplane rotor system were extremely low and insu!cient for full-scale application.4.For the soft-inplane rotor,there is a large increase in system damping associated with moving from the windmilling to the powered-mode operating condition.For the baseline sti -inplane design,subcritical damping increases,but there is not a significant change in the stability boundary.5.The GPC-based active stability augmentation system was very e ective at increas-ing damping in all the fundamental wing modes simultaneously,for both the soft-inplane and sti -inplane rotor systems.6.The GPC controller was very robust with respect to rotor speed and airspeed, with the system damping for the sti -inplane rotor still increasing at45knots beyond the corresponding open-loop instability condition.7.A substantial reduction of blade and hub dynamic loads was obtained for the new soft-inplane design as compared to the baseline sti -inplane design during conversion mode operations.8REFERENCES1.Alexander,H.R.,Hengen,L.H.,and Weiberg,J.A.:“Aeroelastic-Stability Char-acteristics of a V/STOL Tilt-Rotor Aircraft with Hingeless Blades:Correlation of Analysis and Test.”American Helicopter Society30th Annual National Forum, Washington D.C.,May,1974.2.Magee,J.P.,Alexander,H.R.,Gillmore,K.B.,Richardson,D.A.,and Peck,W.B.:“Wind Tunnel Tests of a Full Scale Hingeless Prop/Rotor Design for the Boeing Model222Tiltrotor Aircraft.”Report No.D222-10059-1,Contract NAS2-6505, April,1973.3.Johnson,W.:“Dynamics of Tilting Proprotor Aircraft in Cruise Flight.”NASATN D-7677,May,1974.4.Nixon,M.W.,Langston,C.W.,Singleton,J.D.,Piatak,D.J.,Kvaternik,R.G.,Corso,L.M.,and Brown,R.K.:“Hover Test of a Soft-Inplane Gimballed Tiltro-tor Model.”Journal of the American Helicopter Society,Vol.48,No.1,January, 2003.5.Piatak,D.J.,Kvaternik,R.G.,Nixon,M.W.,Langston,C.W.,Singleton,J.D.,Ben-nett,R.L.,and Brown,R.K.:“A Parametric Investigation of Whirl-Flutter Stability on the WRATS Tiltrotor Model.”Journal of the American Helicopter Society, Vol.47,No.3,July,2002.6.Nixon,M.W.,Piatak,D.J.,Corso,L.M.,and Popelka,D.A.:“Aeroelastic Tailoringfor Stability Augmentation and Performance Enhancements of Tiltrotor Aircraft.”Journal of the American Helicopter Society,Vol.45,No.4,October,2000.7.Corso,L.M.,Popelka,D.A.,and Nixon,M.W.:“Design,Analysis,and Test of aComposite Tailored Tiltrotor Wing.”Journal of the American Helicopter Society, Vol.45,No.3,July,2000.8.Nixon,M.W.,Kvaternik,R.G.,and Settle,T.B.:“Tiltrotor Vibration ReductionThrough Higher Harmonic Control.”Journal of the American Helicopter Society, Vol.43,No.3,July,1998.9.Settle,T.B.,and Kidd,D.L.:“Evolution and Test History of the V-220.2ScaleAeroelastic Model.”Journal of the American Helicopter Society,Vol.37,No.1, January,1992.10.Settle,T.B.and Nixon,M.W.:“MA VSS Control of an Active Flaperon for TiltrotorVibration Reduction.”American Helicopter Society53rd Annual Forum Proceed-ings,pp.1177—1193,Virginia Beach,Virginia,April29-May1,1997.11.Clarke,D.W.,Mohtadi,C.,and Tu s,P.S.:“Generalized Predictive Control—Parts I and II.”Automatica,Vol.23,No.2,1987,pp.137-160.12.Juang,J.-N.and Phan,M.Q.:Identification and Control of Mechanical Systems.Cambridge Univ.Press,New York,NY,2001.13.Kvaternik,R.G.,Juang,J.,and Bennett,R.L.:“Exploratory Studies in Gen-eralized Predictive Control for Active Aeroelastic Control of Tiltrotor Aircraft.”Presented at the American Helicopter Society Northeast Region Specialists’Meet-ing on Active Controls Technology,Bridgeport,CT,October4-5,2000(Paper is also available as NASA/TM-2000-210552).14.Kvaternik,R.G.,Piatak,D.J.,Nixon,M.W.,Langston,C.W.,Singleton,J.D.,Ben-nett,R.L.,and Brown,R.K.:“An Experimental Evaluation of Generalized Predic-tive Control for Tiltrotor Aeroelastic Stability Augmentation in Airplane Mode of Flight.”Journal of the American Helicopter Society,Vol.47,No.3,July,2002.Table1:Key rotor system properties.Twist147.5 47.5Rotor Weight214.59lb16.94lbHub Weight37.189lb9.703lbBlade Weight4 2.167lb 1.770lbBlade FlapInertia0.2330slug-ft20.1265slug-ft2Hover RPM888888Cruise RPM742742Airfoil start8.0in7.5inLift curve slope(nom.) 5.9/rad 5.9/radTipchord 4.470in 3.250inRoot chord 6.510in 4.190in0.75R chord 5.069in 3.757inSolidity,j50.1060.105Precone 2.50 2.75 6Geometric B3-15.0 +15.0Geometric B4—+9.012Includes all blades,hub,pitch links,and hub attachment to mast hardware.3Includes hub,nose cone,pitch links,bearings,and blade cu s.4Per blade,from hub attachment point outboard,includes ballast weight inserts.5Based on chord at75%radius.6This number has little meaning for the current study because there was noflaphinge spring.Fig. 1. Schematics of the two hub types tested.05101520KinematicCoupling,deg.Rotor Lag Damping (Rotating System),% Crit.Rotor Speed, RPM Rotor Speed, RPM Fig. 4. Rotor lag mode damping as a function of rotorspeed (0.57/rev damper set).Fig. 5. Wing torsion/chord mode (WTC) dampingas a function of rotor speed (0.57/rev damper set).(a) The 4-bladed semi-articulated soft-inplane hub. (b) The 3-bladed gimballed stiff-inplane hub.Wing BeamDamping,% Crit.Rotor Speed, RPM7654321Fig. 6. Wing beam mode damping as a function of rotor speed (0.57/rev damper set).Fig. 7. The four-bladed soft-inplane rotor mountedon the WRATS testbed for airplane mode testingin the TDT.-0.50.51.01.52.02.5Velocity, kts.Wing Beam Damping,% crit.-101234Velocity, ktsWing BeamDamping, % crit.Fig. 8. Comparison of wing beam mode dampingbetween the soft-inplane (0.63/rev damper set) andthe stiff-inplane rotor system (742 RPM, off-D/S,windmilling).Fig. 9. Comparison of wing beam mode damping between the windmilling and powered conditions for the soft-inplane rotor system (0.63/rev damper set, 742 RPM, on-D/S).Blade Station, in.HPTPBending Moment, in-lb.02001601208040on wing beam mode damping for the soft-inplane rotor system (742 RPM, on-D/S, wind-milling,0.63/rev damper set)Fig. 13. Effect of hub type on blade dynamic loads (half-peak).1234567Wing Beam Damping,% crit.Velocity, kts1020Velocity, kts.Wing Beam Damping,% crit.Fig. 10. Comparison of wing beam mode damping between the windmilling and powered conditions for the stiff-inplane rotor system (742RPM, off-D/S).Fig. 11. Effect of GPC active stability augmentation on wing beam mode damping for the stiff-inplane rotor system (742 RPM, off-D/S).11。