The Star Cluster Population of M51 III. Cluster disruption and formation history
tpo32三篇托福阅读TOEFL原文译文题目答案译文背景知识

tpo32三篇托福阅读TOEFL原文译文题目答案译文背景知识阅读-1 (2)原文 (2)译文 (5)题目 (7)答案 (16)背景知识 (16)阅读-2 (25)原文 (25)译文 (28)题目 (31)答案 (40)背景知识 (41)阅读-3 (49)原文 (49)译文 (53)题目 (55)答案 (63)背景知识 (64)阅读-1原文Plant Colonization①Colonization is one way in which plants can change the ecology of a site.Colonization is a process with two components:invasion and survival.The rate at which a site is colonized by plants depends on both the rate at which individual organisms(seeds,spores,immature or mature individuals)arrive at the site and their success at becoming established and surviving.Success in colonization depends to a great extent on there being a site available for colonization–a safe site where disturbance by fire or by cutting down of trees has either removed competing species or reduced levels of competition and other negative interactions to a level at which the invading species can become established.For a given rate of invasion,colonization of a moist,fertile site is likely to be much more rapid than that of a dry, infertile site because of poor survival on the latter.A fertile,plowed field is rapidly invaded by a large variety of weeds,whereas a neighboring construction site from which the soil has been compacted or removed to expose a coarse,infertile parent material may remain virtually free of vegetation for many months or even years despite receiving the same input of seeds as the plowed field.②Both the rate of invasion and the rate of extinction vary greatly among different plant species.Pioneer species-those that occur only in the earliest stages of colonization-tend to have high rates of invasion because they produce very large numbers of reproductive propagules(seeds,spores,and so on)and because they have an efficient means of dispersal(normally,wind).③If colonizers produce short-lived reproductive propagules,they must produce very large numbers unless they have an efficient means of dispersal to suitable new habitats.Many plants depend on wind for dispersal and produce abundant quantities of small,relatively short-lived seeds to compensate for the fact that wind is not always a reliable means If reaching the appropriate type of habitat.Alternative strategies have evolved in some plants,such as those that produce fewer but larger seeds that are dispersed to suitable sites by birds or small mammals or those that produce long-lived seeds.Many forest plants seem to exhibit the latter adaptation,and viable seeds of pioneer species can be found in large numbers on some forest floors. For example,as many as1,125viable seeds per square meter were found in a100-year-old Douglas fir/western hemlock forest in coastal British Columbia.Nearly all the seeds that had germinated from this seed bank were from pioneer species.The rapid colonization of such sites after disturbance is undoubtedly in part a reflection of the largeseed band on the forest floor.④An adaptation that is well developed in colonizing species is a high degree of variation in germination(the beginning of a seed’s growth). Seeds of a given species exhibit a wide range of germination dates, increasing the probability that at least some of the seeds will germinate during a period of favorable environmental conditions.This is particularly important for species that colonize an environment where there is no existing vegetation to ameliorate climatic extremes and in which there may be great climatic diversity.⑤Species succession in plant communities,i.e.,the temporal sequence of appearance and disappearance of species is dependent on events occurring at different stages in the life history of a species. Variation in rates of invasion and growth plays an important role in determining patterns of succession,especially secondary succession. The species that are first to colonize a site are those that produce abundant seed that is distributed successfully to new sites.Such species generally grow rapidly and quickly dominate new sites, excluding other species with lower invasion and growth rates.The first community that occupies a disturbed area therefore may be composed of specie with the highest rate of invasion,whereas the community of the subsequent stage may consist of plants with similar survival ratesbut lower invasion rates.译文植物定居①定居是植物改变一个地点生态环境的一种方式。
Ionized gas kinematics and massive star formation in NGC 1530
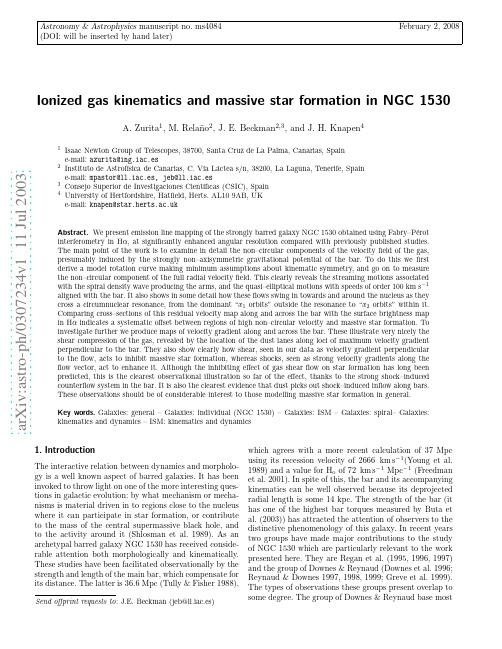
a r X i v :a s t r o -p h /0307234v 1 11 J u l 2003Astronomy &Astrophysics manuscript no.ms4084February 2,2008(DOI:will be inserted by hand later)Ionized gas kinematics and massive star formation in NGC 1530A.Zurita 1,M.Rela˜n o 2,J.E.Beckman 2,3,and J.H.Knapen 41Isaac Newton Group of Telescopes,38700,Santa Cruz de La Palma,Canarias,Spaine-mail:azurita@ing.iac.es 2Instituto de Astrof ´ısica de Canarias,C.V´ıa L´a ctea s/n,38200,La Laguna,Tenerife,Spain e-mail:mpastor@ll.iac.es,jeb@ll.iac.es 3Consejo Superior de Investigaciones Cient´ıficas (CSIC),Spain 4University of Hertfordshire,Hatfield,Herts.AL109AB,UK e-mail:knapen@Abstract.We present emission line mapping of the strongly barred galaxy NGC 1530obtained using Fabry–P´e rot interferometry in H α,at significantly enhanced angular resolution compared with previously published studies.The main point of the work is to examine in detail the non–circular components of the velocity field of the gas,presumably induced by the strongly non–axisymmetric gravitational potential of the bar.To do this we first derive a model rotation curve making minimum assumptions about kinematic symmetry,and go on to measure the non–circular component of the full radial velocity field.This clearly reveals the streaming motions associated with the spiral density wave producing the arms,and the quasi–elliptical motions with speeds of order 100km s −1aligned with the bar.It also shows in some detail how these flows swing in towards and around the nucleus as they cross a circumnuclear resonance,from the dominant “x 1orbits”outside the resonance to “x 2orbits”within paring cross–sections of this residual velocity map along and across the bar with the surface brightness map in H αindicates a systematic offset between regions of high non–circular velocity and massive star formation.To investigate further we produce maps of velocity gradient along and across the bar.These illustrate very nicely the shear compression of the gas,revealed by the location of the dust lanes along loci of maximum velocity gradient perpendicular to the bar.They also show clearly how shear,seen in our data as velocity gradient perpendicular to the flow,acts to inhibit massive star formation,whereas shocks,seen as strong velocity gradients along the flow vector,act to enhance it.Although the inhibiting effect of gas shear flow on star formation has long been predicted,this is the clearest observational illustration so far of the effect,thanks to the strong shock–induced counterflow system in the bar.It is also the clearest evidence that dust picks out shock–induced inflow along bars.These observations should be of considerable interest to those modelling massive star formation in general.Key words.Galaxies:general –Galaxies:individual (NGC 1530)–Galaxies:ISM –Galaxies:spiral–Galaxies:kinematics and dynamics –ISM:kinematics and dynamics1.IntroductionThe interactive relation between dynamics and morpholo-gy is a well known aspect of barred galaxies.It has been invoked to throw light on one of the more interesting ques-tions in galactic evolution:by what mechanism or mecha-nisms is material driven in to regions close to the nucleus where it can participate in star formation,or contribute to the mass of the central supermassive black hole,and to the activity around it (Shlosman et al.1989).As an archetypal barred galaxy NGC 1530has received conside-rable attention both morphologically and kinematically.These studies have been facilitated observationally by the strength and length of the main bar,which compensate for its distance.The latter is 36.6Mpc (Tully &Fisher 1988),2Zurita et al.:Ionized gas kinematics and massive star formation in NGC1530of their work on velocity and surface luminosity mapping in CO with some additional stellar information presented in Greve et al.(1999),while Regan et al.’s most important contributions come from their velocity and surface bright-ness mapping in Hαwith a useful contribution from CO supplemented by near–IR mapping in the initial paper of their published series.NGC1530is a dramatic twin-armed spiral galaxy,with arms breaking away sharply from the ends of the long primary bar.It is notable that these arms,though trail-ing,have shorter opposing lengths,curving inward from the tips of the bar as prolongations of the main arms. They are well brought out in Hαas seen in Regan et al. (1995)or in Fig.1a of the present paper.The bar ima-ged in broad band is wide and contains prominent dust lanes which,as the publications by Regan et al.and by Downes&Reynaud listed above well point out,pick out shocked gas following trajectories generally aligned with the bar.These are almost straight along the bar but begin to curve inwards from a galactocentric distance of some 2kpc,bending sharply in towards the nucleus.They link the prominent star–forming regions at the ends of the bar, seen very well here in Hαin Fig.1a,with the even more prominent star–forming ring feature with radius∼2kpc noted in blue images by Buta(1984)and by Wray(1988) and also clearly picked out in Hαin bining near–IR with optical data to give colour maps,Regan et al.(1995)explored the stellar and dust morphology in the bar and in the central zone following the lanes right into the nucleus.One of the aims of the present paper is to elucidate further the relation between gasflows and star formation which are implied in these morphological data described above.However,this aim has been pursued with conside-rable achievement previously by the two groups men-tioned above.Regan et al.(1996)combined atomic hy-drogen21cm measurements with ionized hydrogen HαFabry–P´e rot spectroscopy to derive a rotation curve for NGC1530.From this they derived a bar pattern speed of 20km s−1kpc−1and used it to predict resonances at radii coinciding with prominent morphological features,inclu-ding an outer gaseous ring seen in H i,an inner ring seen in H i and Hα,and the nuclear star–forming ring men-tioned above.Downes et al.(1996)mapped the galaxy in CO and identified the major concentrations of potentially star forming molecular gas;these are located over the cen-tral prominent inner disc zone of radius∼2kpc and extend out more weakly over the bar to less prominent concentra-tions over the ends of the bar.They added interferometric mapping of the central zone,finding a circumnuclear ring structure with gas in orbits apparently perpendicular to the elongated orbits in the outer parts of the bar.They re-late these patterns to the predictions of resonance models (Athanassoula1984;Jenkins&Binney1994)in which gas within corotation moves along x1orbits along the bar un-til it reaches an inner Lindblad resonance(ILR)where it moves into the x2orbits perpendicular to the bar.A neat schematic diagram infig.10of Downes et al.(1996)illus-trates the predicted behaviour of the gas under these cir-cumstances.Although the stars follow quasi–elliptical x1 orbits,in the case of gas,the shocks are produced along the bar and specially at the ends of the bar,where orbit crowd-ing occurs,as was well predicted in Roberts et al.(1979). However,as the gas reaches the outer limit of the zone dominated by x2dynamics,it suffers another shock,and is pulled through a right–angle,falling in again towards the nucleus.Gas on x1orbits with an impact parameter a little larger with respect to the nucleus is less affected by the effects of the resonance,and proceeds along the out-going edge of the bar,albeit with declining velocities.It is just this kind of arrangement for allowing gas to fall to the centres of barred galaxies which was invoked by Shlosman et al.(1989)in their“bars within bars”scenario as a fu-eling mechanism for the different types of circumnuclear activity:starbursts and AGN.Using Fabry–P´e rot Hαmapping of NGC1530,Regan et al.(1997)demonstrated that the two–dimensional Hαvelocityfield in the bar region shows the skew pattern predicted for x1orbits superposed on the general galac-tic rotation.They went on to combine hydrodynamical models with their observations to compute a net inflow rate to the nuclear zone of∼1M⊙per year,with the re-maining gas spraying back outwards along the major bar axis before coupling into a new inflow ing molecu-lar emission from CO and HCN,the former at angular res-olution of1.8′′and the latter at3.6′′,Reynaud&Downes (1997)detected steep velocity gradients spatially confined to two arcs coupling into a ring.These arcs represent shock fronts where the inflow along the bar is being constrained to change direction and assume the x2orbital configura-tion along the nucleus.These arcs correspond to two small arm features picked out in Hαto the north and south of the circumnuclear zone of enhanced star formation(see Fig.1a of the present paper).The points where the arcs couple to the circumnuclear ring are the main emitters in HCN,suggesting points of maximum molecular density. Reynaud&Downes postulate the presence of two ILR’s: one at1.2kpc from the nucleus where the shock arcs are rooted,and one very close to the nucleus,at the inner edge of the molecular ring.They support these inferences using resonance theory and their rotation curve,which is de-rived kinematically but supported by a dynamical model. In a subsequent article Reynaud&Downes(1999)map the same area in two emission lines each of12CO and13CO, showing that the molecular gas density is highest in the circumnuclear ring and not so high in the arcs referred to above.Perhaps of most interest and relevance for the study we present here is that of Reynaud&Downes(1998),who use their CO kinematics of the whole bar region in NGC1530 to explore the relation between velocity changes and star formation.They conclude that strong velocity changes, which represent shocks and shear,are negatively corre-lated with star formation and that the distribution of star formation does not correspond fully to the distribution of available molecular gas,as traced by CO.Although itZurita et al.:Ionized gas kinematics and massive star formation in NGC15303is true that the major concentration is found in the cen-tral circumnuclear zone where star formation is most en-hanced,there is considerable CO measured along the bar as well as at its ends,while the star formation is clearly weak along of the bar.Reynaud&Downesfind veloci-ty jumps across the dust lanes from velocities of order 100km s−1along the bar in one direction,to similar velo-cities in the reverse direction.These jumps maximize half way out along the bar from the nucleus on both sides. They conclude that steep velocity gradients,both shear and shock,prevent molecular clouds from condensing to form stars.One of the main aims of the present paper is to examine this hypothesis in a little more detail using an Hαvelocityfield.We present new high resolution velocity and inten-sity maps of NGC1530,obtained in Hαemission using a Fabry–P´e rot interferometer.The instrument provides a three–dimensional“data cube”,akin to a conventional ra-diastronomical set of line maps,in which the emission in-tensity is given as a function of three coordinates:two positional coordinates and the velocity.From this“data cube”one goes on to compute maps in integrated line intensity,line peak velocity,and velocity dispersion.The next step is to obtain the rotation curve of the galaxy and to subtract it offtwo–dimensionally from the com-plete observed velocityfield.This allows us to obtain the non-circular motions due to non-axisymmetric elements of the galaxy.For NGC1530the dominant source of non–axisymmetry is the strong bar of length almost28kpc, which dominates the appearance of the galactic disk.We alsofind kinematic signatures of the streaming motions in the sharply defined spiral arms.Besides we also see,in the central5kpc,evidence for a distinct kinematic structure predicted by classical resonance theory,and already care-fully observed and characterised in the studies by Regan and coworkers,and by Reynaud&Downes in the referen-ces given above.As a further step we have prepared plots of the velocity gradients in the whole of the bar and arm structure as ima-ged in Hαemission.We have related the velocity gradient morphology to the distribution of the massive star forma-tion in arms,bar,and the central zone.Our main steps forward compared with previous studies are due to the uniformly high angular resolution we could obtain over the full image.This has enabled us to make a more precise de-finition of the rotation curve,and hence an improved map of the non-circular velocityfield,both around the main bar,and in the central zone.From the two–dimensional display of the non-circular velocities we have gone on to derive a map of the velocity gradients,with a resolution better than2′′over the wholefield of the galaxy.Such a map has not been previously produced,so we are able to make progress here in characterising the geometrical and physical relationships between velocity gradients and star formation.2.Observations and data reductionThe Fabry–P´e rot observations of NGC1530were taken at the Roque de los Muchachos Observatory with the 4.2m William Herschel Telescope(WHT)on the night of September2nd,1998.The TAURUS-II instrument, equipped with a TEK CCD camera was used to observe the galaxy in the Hαemission line,with a plate scale of0.292′′per pixel,which corresponds to a pixel scale 179pc arcsec−1at the distance of the galaxy(37Mpc). The observations consisted of exposures of150s at equally spaced positions of the etalon,at intervals of0.407˚A,co-rresponding to18.62km s−1.The total number of steps was55,allowing us to scan the full wavelength range of the Hαline in the galaxy.We used the appropriately red-shifted narrow band Hαfilter as an order-sortingfilter (λc=6613˚A,∆λ=15˚A,corresponding to the galaxy’s sys-temic velocity v sys=2461km s−1;de Vaucouleurs et al. 1991;hereinafter RC3).Wavelength and phase calibration were performed using observations of a calibration lamp (taken before and after the observations of the galaxy),u-sing the TAUCAL package in the FIGARO environment. The spatial resolution of the resulting data set is∼1′′.The data set was placed on a correctly oriented spatial grid by comparing positions of point–like features in the HαFabry–P´e rot intensity map with those in the narrow-band Hαimage from Rela˜n o et.al(2003b),yielding maximum uncertainties in positions of0.3′′.Thefinal calibrated data cube has941×941pixels×55‘planes’in wavelength,se-parated by18.62km s−1.We examined plane by plane the data cube and found that it had a considerable number of cosmic ray events.We eliminated them with the task imedit in IRAF,which allowed us to replace the anoma-lous pixels by a mean background value.We determined which channels of the data set were free of Hαline emission after smoothing the data to a resolution of2.5′′×2.5′′.We found10channels free of line emission on the low-velocity side,and13channels on the high–velocity side.The con-tinuum was determined byfitting a linear relation to the line–free channels,and was then subtracted from the line emission channels.In order to study the kinematics on different scales,the data–cube was convolved down from the original resolu-tion of1′′×1′′to resolutions of2′′×2′′,3′′×3′′,6′′×6′′and 10′′×10′′.For each data set we obtained the total Hαintensity(zeroth moment),velocity(first moment)and velocity dispersion(second moment)maps.The proce-dure to obtain the moment maps is equivalent for each smoothed data cube so we describe the procedure followed for the full resolution data set only.A reader wanting a fuller description of the procedure canfind it in Knapen (1998).The points with low signal were eliminated pro-ducing a conditionally transferred data cube,in which values were retained only at positions where the inten-sity in the smoothed data cube of3′′×3′′was larger than 2.5times the rms noise of the smoothed cube;pixel va-lues at all other positions were set to undefined.We then removed noise peaks outside the area where Hαemission4Zurita et al.:Ionized gas kinematics and massive star formation in NGC1530Fig.1.a)Intensity map(zeroth order moment)of the Hαemission in NGC1530.The map was obtained from the 2′′resolution data cube.The kinematic centre is marked with a black cross.b)Velocity map(first moment)of the Hαemission in NGC1530,derived from the2′′resolution data cube.c)Model velocity map of NGC1530,obtained from the Hαrotation curve of the galaxy shown in Fig.3,with Hαisointensity contours overlaid.We can clearly see“wiggles”at the radii of the spiral arms,due to the density wave streaming motions not subtracted offfrom the rotation curve.d)Map of the residual velocities of NGC1530,obtained by subtracting the model velocityfield (Fig.1c,after taking out the ripples due to the streaming motions in the spiral arms,see text for details)from theZurita et al.:Ionized gas kinematics and massive star formation in NGC15305 is expected by setting the pixel values there to undefinedtoo.This was done interactively by inspecting the highresolution channel maps one by one and comparing themwith the corresponding and adjacent channel maps in the3′′×3′′data set.The resulting data cube was then used tocalculate the moment maps using the moments task inthe GIPSY package.moments obtains the zeroth,first and second mo-ments of the emission at each spatial pixel in the galaxy and produces a corresponding map for each moment.In the procedure,moments takes into account the signal only when two conditions are met:the signal is higher than2.5times the rms noise of the high-resolution cube, in at least three adjacent channels.Theintensity map for the2′′resolution data cube is shown in Fig.1a and the first moment(velocity)map is shown in Fig.1b.Although the moments procedure is a more useful tech-nique for studying the global kinematics in the galaxy,it must be treated with caution when it is used for a detailed description of the kinematics of individual H ii regions. The moments procedure gives an underestimate of the ve-locity dispersion of the profile(van der Kruit&Shostak 1982),thus,if a precision measurement of this quantity is required,a betterfit to the line profiles,using Gaussian functions,is needed.3.Kinematics3.1.Rotation curveThe rotation curve of the galaxy can be derived from the velocity map,which gives the projected velocity along the line of sight in each position of the galaxy.Assuming that the measurements refer to a position on a single inclined plane,the projected velocity can be related to the rotation velocity in the plane of the galaxy by this expression:v(x,y)=v sys+v rot(r)cos(θ)sin(i)(1) where v(x,y)denotes the radial velocity at the sky coor-dinates x and y,v sys the systemic velocity,v rot the ro-tational velocity,i the inclination angle of the normal to the galaxy plane to the line of sight,andθthe azimuthal distance from the major axis in the plane of the galaxy,re-lated to the rotation centre(x0,y0)and the position angle of the galaxy,PA.We derived the rotation curve following the procedure described by Begeman(1989),where the rotation curve is determined byfitting tilted rings to the observed velocityfield.For each ring independently,the parameters v sys,v rot,i and x0,y0and PA are obtained making a least–squaresfit to Eq.1using the observed velocityfield v(x,y).We discarded the points within a certain free–angle θmax around the minor axis since they carry less informa-tion about the underlying circular velocity of the galaxy and the values obtained in this zone are less accurate due to projection effects.In the case of NGC1530a value of θmax=45◦was used to avoid the non-circular motions along the bar.Besides,we give more weight to positionsRadius (arcsec)020*********100200300Radius (kpc)Fig.2.Hαrotation curve of the disc of NGC1530,for the receding and the approaching sides of the galaxy separately.The agreement between the two sides shows the symmetry of the velocityfield and the reliability of the rotation centre.close to the major axis using a weighting function pro-portional to|cosθ|in thefit.The procedure used to ob-tain each of the parameters which characterise the rotation curve is the following.The parameters v sys,x0and y0depend on the symme-try of the velocityfield and are the same for each ring. We obtained the position of the kinematic centre(x0,y0) using the velocity map of6′′resolution.We took annuli of 3′′width,fixed values for the heliocentric systemic velo-city at2461km s−1(RC3),inclination angle at i=50◦(a mean between the values of Downes et al.1996and Regan et al.1996)and position angle at188◦(the value found by Regan et al.1996)and obtained(x0,y0)using Eq.1.We found a large scatter in the values of the kinematic centre for annuli far from the centre of the galaxy.This is due to the paucity of Hαemission in the disc of the galaxy outside the arms and to the fact that lower resolution data cubes are more spatially affected by the smoothed non-circular motions along the bar in the data cubes.The mean value of(x0,y0)for the rings inside12′′corresponds to the coor-dinates4h23m26.94s(±0.02s)75◦17′44.0′′(±0.1′′),J2000. We checked this value using the same procedure for the velocity maps of lower and higher(10′′and2′′)resolution, and found that the differences in the rotation centre from the value adopted were inside the error bars.The systemic velocity was obtained from the10′′reso-lution velocity map with rings of width5′′.The centre po-sition wasfixed to the value found previously,and the in-clination and position angle werefixed to the same values used to derive the rotation centre.The mean value for v sys in all the rings is2466±4km s−1,where the error is the6Zurita et al.:Ionized gas kinematics and massive star formation in NGC1530 standard deviation from the mean.This value is in agree-ment with the value found by Reynaud et al.(1997)ofv sys=2466km s−1for CO data,but differs from that ob-tained by Regan et al.1996in Hα,v sys=2447±2km s−1.A way to test whether the rotation centre and thesys-temic velocity are well determined is shown in Fig.2, where we have plotted rotation curves for the receding and approaching halves of the galaxy derived separately u-sing the rotation centre and systemic velocity found above. The coincidence of the velocity points for both sides,espe-cially in the central zone of the galaxy,provides evidence of the symmetry of the velocityfield and the validity of the values for the rotation centre and systemic velocity. We also derived the rotation curves for the receding and a-pproaching halves with the rotation centre found in Regan et al.(1996),but in that case we found discrepancies which appeared unphysically large.It is the better resolution of our data(1′′,while Regan et al.(1996)has a net resolution of4′′)which allows us to obtain a more accurate positionfor the rotation centre.Finally,we obtained the rotation curve using the ve-locity map at2′′resolution in Fig1b.For the internal part of the galaxy,out to12.5′′,we used rings of width1′′,and rings of width6′′for larger radii.The rotation curve ob-tained in this way is shown in Fig.3,where the PA and the inclination werefixed at188◦and50◦respectively.Fig.3 also displays the rotation curve from Regan et al.1996, which is similar to that found here,but we obtain higher values of the rotation velocity in the inner part,10′′–15′′, and also in the70′′–90′′radial range.In order to check the PA adopted,we left it as a free parameter in thefit,find-ing that the values given by the program for most of the annuli are close to188◦and that the rotation curve did not change significantly from the curve with afixed PA.The adopted inclination angle i=50◦was also checked.We fitted v rot and i with Eq.1,keeping the other kinematic parametersfixed,and found a mean value of i=(53±8)◦, in good agreement with the value adopted.To test the importance of a careful definition of the the free–angle we reduced its value to20◦and rederived the rotation curve.As seen in Fig.4this produced“ro-tational velocities”rising to values of over500km s−1,as there is an obvious contribution from the non–rotational motion along the bar projected into the line of sight.As we will see below the amplitude of the gas motion along the bar is over100km s−1,and it is easy to see that this will yield the unrealistically high velocities shown in Fig.4. Fortunately,the bar orientation in NGC1530does not make it too difficult to isolate and avoid the emission from gas which contains a significant non–circular velocity com-ponent,but Fig.4offers a warning to those using maps of much lower resolution in similar circumstances.3.2.The residual velocity mapAfter deriving the mean rotation curve,an easy way to explore non–circular motions within the galactic disc isRadius (arcsec)020*********100200300Radius (kpc)051015Fig.3.Hαrotation curve of the disc of NGC1530,up to18kpc from the galactic centre.The rotation curve was obtained byfitting tilted rings to the observed ve-locityfield in Fig.1b.Also shown is the rotation curve obtained by Regan et al.(1996).to make a two–dimensional projection of this curve and subtract it from the observed velocityfield.The two–dimensional projection of the initial rotation curve is seen in Fig.1c,where we have superposed an isophote map of intensities to show the positions of the emitting compo-nents.The projection has classical symmetry,but shows a few small features:“wiggles”which occur at some three quarters of the radial distance from the centre to the edge of thefield.These can be explained as effects of stream-ing motions in the spiral arms,which have not been sub-tracted adequately from the mean rotation curve.While it is difficult to get an exact separation of ro-tation and streaming motions,we have made afirst a-pproximation by assuming that the true rotation curve is smooth.Applying a second order polynomialfit to the part that is observed as smooth,i.e.between27′′and63′′from the centre,we then extrapolate this to cover the range between63′′and the edge of thefield at99′′from the centre,and go on to combine it with the original curve out to63′′radius,to give thefinal“true”rotation curve. The result of this procedure is shown in Fig.5,where we have compared our original rotation curve with the new curve and also with the curve by Regan et al.(1996).Our procedure has isolated the wiggles between63′′and93′′.We can then proceed to produce a new two–dimensional projected model for the rotation in which the wiggles are no longer present,and to subtract offthis new rotation model from the original velocityfield,yielding a resulting map of residuals as shown in Fig.1d.There areZurita et al.:Ionized gas kinematics and massive star formation in NGC 15307Radius (arcsec)204060801000200400600Radius (kpc)51015freeangle 20 degfreeangle 45 deg Fig.4.H αrotation curves of the disc of NGC 1530ob-tained with different “free–angles”and with rings of 1′′width.For free–angles less than ∼30◦there is an obvi-ous contribution from the non–rotational motion along the bar in the mean calculated rotational velocity that can “contaminate”the rotation curve of the galaxy.clearly ordered fields of non–circular velocity both around the bar,and in the arms,but we can check on the mo-del subtraction by examining the residuals of the regions which are in the disc outside the arms and bar.These in general show,as they should if the rotation curve sub-traction is valid,net residual velocities close to zero:less than ∼10km s −1,which is close to the precision limit of the observations.We can nowsee clearly the streaming motions across the arms in Fig.1d.These have projected values between 10and 30km s −1,with similar amplitudes in both arms.3.2.1.The residual velocities along the barOf most striking interest is the fairly complex non–circular velocity field associated with the bar.Approaching veloc-ities of up to 120km s −1are observed along the north side of the bar,and receding velocities of the same am-plitude along the south side.These values,and the im-plied vectorial direction in elongated quasi–elliptical or-bits around the bar with long axis parallel to the bar axis,are in line with classical predictions of the dynamical ef-fects of a stellar bar on the rotating gas which date back to Huntley et al.(1978)and Roberts et al.(1979)as well as to more recent studies by Athanassoula (1992).Most of the observational work has been performed either in H i or more recently in CO emission.There have been relatively few H αstudies of this phenomenon,for two probable rea-sons:slit spectroscopy is costly in time if more than a couple of position angles are taken (one good early studyRadius (arcsec)204060801000100200300Radius (kpc)Fig.5.H αrotation curve of the disc of NGC 1530.This rotation curve has been obtained by subtracting the ef-fect of the streaming motions in the spiral arms of the galaxy from the mean rotation curve shown in Fig.3(see text for further details).in this mode using six slit positions on NGC 1512was published by Lindblad &Jorsater 1981);and H αemis-sion is much patchier than either H i or CO.However,as we can see from the data in the present article,the use of a scanning Fabry–P´e rot for this work permits full two–dimensional coverage at an angular resolution better than that of essentially all H i or CO measurements,which can compensate to an interesting degree for the intrinsic patchiness of the observed field.In Fig.1d there are several complexities in the non–circular velocity field which are worth pointing out.Taking the north side of the bar we can see a zone with high bar–streaming velocities,of order 120km s −1in a direction approaching us to the east of the centre,and another re-gion,more restricted in size and velocity range,with simi-lar velocities to the west.To the south of the bar we can see a diametrically symmetric flow,with high receding ve-locities,again of order 120km s −1receding on west side,and two smaller zones with similar velocities to the east.It is tempting to consider these flows as direct evidence for motion aligned with the bar and terminating in zones of shock towards the ends of the bar.We will see later that there is considerable evidence in favour of this,and of the direct causal links between properties of these flows,and both the stimulation and inhibition of massive star forma-tion.Examining Fig.1d,the question arises as to how the flow can apparently be stopped half way along the bar,yet continue on beyond the central zones as described above.This appearance does not give a true picture of the ve-locity field and is essentially due to the patchiness of the。
玉米籽粒皱缩突变体sh2021_的表型分析和基因定位
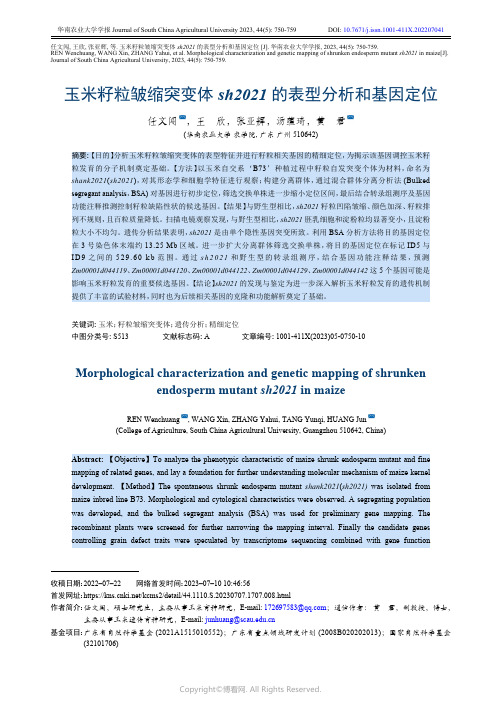
任文闯, 王欣, 张亚辉, 等. 玉米籽粒皱缩突变体sh2021的表型分析和基因定位[J]. 华南农业大学学报, 2023, 44(5): 750-759.REN Wenchuang, WANG Xin, ZHANG Yahui, et al. Morphological characterization and genetic mapping of shrunken endosperm mutant sh2021 in maize[J].Journal of South China Agricultural University, 2023, 44(5): 750-759.玉米籽粒皱缩突变体sh2021的表型分析和基因定位任文闯 ,王 欣,张亚辉,汤蕴琦,黄 君(华南农业大学 农学院, 广东 广州 510642)摘要: 【目的】分析玉米籽粒皱缩突变体的表型特征并进行籽粒相关基因的精细定位,为揭示该基因调控玉米籽粒发育的分子机制奠定基础。
【方法】以玉米自交系‘B73’种植过程中籽粒自发突变个体为材料,命名为shank2021(sh2021),对其形态学和细胞学特征进行观察;构建分离群体,通过混合群体分离分析法(Bulked segregant analysis ,BSA)对基因进行初步定位,筛选交换单株进一步缩小定位区间,最后结合转录组测序及基因功能注释推测控制籽粒缺陷性状的候选基因。
【结果】与野生型相比,sh2021籽粒凹陷皱缩、颜色加深、籽粒排列不规则,且百粒质量降低。
扫描电镜观察发现,与野生型相比,sh2021胚乳细胞和淀粉粒均显著变小,且淀粉粒大小不均匀。
遗传分析结果表明,sh2021是由单个隐性基因突变所致。
利用BSA 分析方法将目的基因定位在3号染色体末端约13.25 Mb 区域。
进一步扩大分离群体筛选交换单株,将目的基因定位在标记ID5与I D 9之间的529.60 k b 范围。
Three clusters of the SMC from ACSWFC HST archive data NGC 265, K~29 and NGC 290 and their
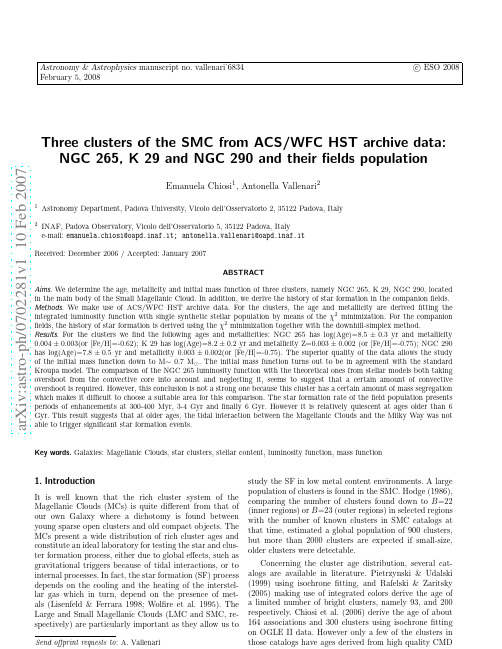
a r X i v :a s t r o -p h /0702281v 1 10 F eb 2007Astronomy &Astrophysics manuscript no.vallenari˙6834cESO 2008February 5,2008Three clusters of the SMC from ACS/WFC HST archive data:NGC 265,K 29and NGC 290and their fields populationEmanuela Chiosi 1,Antonella Vallenari 21Astronomy Department,Padova University,Vicolo dell’Osservatorio 2,35122Padova,Italy 2INAF,Padova Observatory,Vicolo dell’Osservatorio 5,35122Padova,Italye-mail:emanuela.chiosi@oapd.inaf.it;antonella.vallenari@oapd.inaf.itReceived:December 2006/Accepted:January 2007ABSTRACTAims.We determine the age,metallicity and initial mass function of three clusters,namely NGC 265,K 29,NGC 290,located in the main body of the Small Magellanic Cloud.In addition,we derive the history of star formation in the companion fields.Methods.We make use of ACS/WFC HST archive data.For the clusters,the age and metallicity are derived fitting the integrated luminosity function with single synthetic stellar population by means of the χ2minimization.For the companion fields,the history of star formation is derived using the χ2minimization together with the downhill-simplex method.Results.For the clusters we find the following ages and metallicities:NGC 265has log(Age)=8.5±0.3yr and metallicity 0.004±0.003(or [Fe/H]=-0.62);K 29has log(Age)=8.2±0.2yr and metallicity Z=0.003±0.002(or [Fe/H]=-0.75);NGC 290has log(Age)=7.8±0.5yr and metallicity 0.003±0.002(or [Fe/H]=-0.75).The superior quality of the data allows the study of the initial mass function down to M ∼0.7M ⊙.The initial mass function turns out to be in agreement with the standard Kroupa model.The comparison of the NGC 265luminosity function with the theoretical ones from stellar models both taking overshoot from the convective core into account and neglecting it,seems to suggest that a certain amount of convective overshoot is required.However,this conclusion is not a strong one because this cluster has a certain amount of mass segregation which makes it difficult to choose a suitable area for this comparison.The star formation rate of the field population presents periods of enhancements at 300-400Myr,3-4Gyr and finally 6Gyr.However it is relatively quiescent at ages older than 6Gyr.This result suggests that at older ages,the tidal interaction between the Magellanic Clouds and the Milky Way was not able to trigger significant star formation events.Key words.Galaxies:Magellanic Clouds,star clusters,stellar content,luminosity function,mass function1.IntroductionIt is well known that the rich cluster system of the Magellanic Clouds (MCs)is quite different from that of our own Galaxy where a dichotomy is found between young sparse open clusters and old compact objects.The MCs present a wide distribution of rich cluster ages and constitute an ideal laboratory for testing the star and clus-ter formation process,either due to global effects,such as gravitational triggers because of tidal interactions,or to internal processes.In fact,the star formation (SF)process depends on the cooling and the heating of the interstel-lar gas which in turn,depend on the presence of met-als (Lisenfeld &Ferrara 1998;Wolfire et al.1995).The Large and Small Magellanic Clouds (LMC and SMC,re-spectively)are particularly important as they allow us to2 E.Chiosi&A.Vallenari:Three clusters of the SMC Table1.Equatorial coordinates of the SMC regions containingthe three clusters.NGC2650:47:12-73:28:38K290:51:53-72:57:14 NGC2900:51:14-73:09:41Cluster∆m F555WP SF−AP(3pix)∆m F814WP SF−AP(3pix)-0.14±0.02-0.15±0.02-0.14±0.02-0.15±0.02-0.14±0.02-0.13±0.02the aperture photometry is calculated with an aperture of3pixels,the point spread function(PSF)is derived for each colour and each CCD,and the PSF photome-try is performed.Data arefinally corrected for the charge transfer efficiency(CTE)using the Riess&Mack(2004) transformations.This correction goes from0.01mag for the brightest magnitudes to0.1mag for the faintest mag-nitudes.Calibration.The transformation from the instrumen-tal magnitudes to the ACS/WFC-Vega system,taken from Bedin et al.(2005),is given bym filter≡−2.5log10I e−E.Chiosi &A.Vallenari:Three clusters of the SMC318202224260.10.080.060.040.02magF555WF814WFig.1.Mean photometric errors σas function of the magni-tude for the F555W and F814W pass-bands for the studiedfields.of the saturated stars in the ACS photometry from the OGLE II catalog (see the sections below for more detail).We estimate the photometric errors in the two pass-bands by means of the artificial stars experiments as the difference between the assigned and recovered magnitude for any simulated star.The results are shown in Fig.1as a mean of all the studied fields.This is justified by the fact that differences from field to field are not relevant.The observational CMDs of the three clusters are pre-sented in the top left panels of the Figs.2,3,and 4.They will be examined in detail below.They refer to a circular area having 22–25”of radius (depending on the cluster)inside which the vast majority of the cluster stars falls as shown by the surface brightness profiles in the top right panels of the Figs.2,3,and 4(see the discussion below).Incompleteness .Correction for incompleteness is de-rived as usual by means of artificial star experiments where artificial stars of known magnitude are added to the original image and data are re-reduced.The ratio between the number of recovered stars and the original number of added stars gives the completeness correction Λ.The re-sults are shown in the bottom right panels of the Figs.2,3,and 4which present Λas function of the magnitude for the inner radius of the clusters.and for the external regions.More details can be found in the sections below.3.Distance modulus,reddening and metallicityThe distance modulus (m −M )0=18.9is assumed for the SMC,in agreement with recent determinations by Storm et al.(2004),Weldrake et al.(2004),Caputo et al.(1999)and Sandage et al.(1999).No assumptions are made for the reddening and metal-licity of each cluster.They are derived together with the age from the analysis of the CMD by means of the χ2minimization technique to be discussed below.In the study of the field population,assumptions have to be made for the SMC depth along the line of sight,inter-stellar extinction and age-metallicity relation.We proceed as follows:(1)We adopt the SMC depth along the line of sight of 4kpc,which implies a difference in the distance modulus of about 0.14mag (Welch et al.1987;Zaritsky et al.2002).(2)The extinction map across the SMC derived by Zaritsky et al.(2002)shows that the interstellar redden-ing is spatially varying,i.e.increasing along the SMC ridge from North-East to South-West.A mean reddening E (B −V )=0.08mag is derived by Rachford et al.(2002)and Hunter et al.(2003).However,we prefer to use for the field population the same extinction we have obtained for the study of the CMD of the companion cluster using the minimization procedure.(3)Our knowledge of the age-metallicity relation in the SMC is mainly based on clusters.The interpretation of the existing SMC age-metallicity relation widely varies from author to author.It goes from continuous enrichment from the oldest to the youngest objects as found by Da Costa &Hatzidimitriou (1998)and by Dolphin (2000b)to a burst-mode of star formation and enrichment as derived by Olszewski et al.(1996),Pagel &Tautvaiˇs ien˙e (1999),Piatti et al.(2001),Harris &Zaritsky (2004).To study the star formation history in the fields,we assume the enrichment history by Pagel &Tautvaiˇs ien˙e (1999).4.Method to derive ages,metallicities,and IMFsAge,metal content,and IMF are estimated by comparing theoretical luminosity functions (LF)that depend on the three parameters under examination to the observational one and by plotting the corresponding isochrones on the CMD to check the mutual consistency of the results.The determination is first made by visual inspection of the CMD and it is refined with the aid of the χ2minimization.Theoretical luminosity functions and isochrones are taken from the library of Girardi et al.(2002).Corrections for completeness and field subtraction are the preliminary step to be undertaken to derive good ex-perimental luminosity functions.The analysis articulates as follows:1.Equal areas are considered for each cluster and com-panion field.2.The luminosity interval spanned by the cluster and field stars in each pass-band is divided in number of intervals of 0.5mag width.In each interval,and separately for the cluster and field,we count the stars and apply the correc-tion for incompleteness (the cluster and field completeness4 E.Chiosi&A.Vallenari:Three clusters of the SMC factors are used as appropriate)andfinally we calculatethe ratio1N true=E.Chiosi &A.Vallenari:Three clusters of the SMC5-112325201510F555W-F814W0.511.5-5-4.8-4.6-4.4-4.2log(r(arcsec))-1012325201510F555W-F814WmagnitudeF555W clusterF814W cluster 20222426283000.511.5F555W field F814W fieldFig.2.Summary panels for NGC 265.In the top left panel is the CM diagram (inner 22”)with a superposed isochrone of log(age)=8.5,Z=0.004and E(F555W-F814W)=0.08.The brightest stars settle at F555W=16.In the top right panel the surface brightness profile (in arbitrary units)is fitted by one of the King functions (dashed line)and by Elson profile (continuous line).From this distribution we can determine core and tidal radius.Vast majority of the stars sets within a radius of 22”corresponding to log(r)=1.3.In the bottom left panel the CMD of field stars is shown.The field population is taken at about 1.6’from the cluster center and the area is comparable to the area of the cluster (see text for details).The bottom right panel shows the completeness factors for the cluster and field regions as indicatedcoordinates αand δusing the task IRAF STSDAS ”xy2rd”.Then,the center of a cluster is determined calculating the luminosity weighted centroid at increas-ing radii.The photometric data of the stars that are saturated in the ACS/WFC observations are taken from OGLE II data,converted to the F555W pass-band,andfinally used to construct the surface brightness profile.We are confident that this does not introduce errors in the derived profiles as we have already checked the consistency between the OGLE II photometry in the V magnitude and the ACS/WFC HST V calibration.6E.Chiosi &A.Vallenari:Three clusters of the SMC-112325201510F555W-F814W0.511.5-4.8-4.6-4.4-4.2-4log(r(arcsec))-1012325201510F555W-F814W0magnitudeF555W clusterF814W cluster 2022242628300.511.5F555W field F814W fieldFig.3.Summary panels for K 29.In the top left panel is the CM diagram (inner 22”)with a superposed isochrone of log(age)=8.2,Z=0.003and E(F555W-F814W)=0.14.The OGLE bright stars are indicated by the triangles.The brightest stars settle at F555W=15.In top right is the brightness profile (in arbitrary units)fitted with one of the King (dashed line)and with the Elson functions (solid line).From this distribution we can determine core and tidal radius.Vast majority of the stars sets within a radius of 22”(or log(r)=1.3).In the bottom left panel the CMD of field stars is shown.The field population is taken at about 1.6’from the cluster center and the area is comparable to the area of the cluster (22”).The bottom right panel shows the completeness factors for the cluster and field regions as indicatedMany SMC clusters have elliptical isophotes.This is not the case of the objects studied in this paper,which have only moderate ellipticity,1−b/a ∼0.13−−0.3(Hill &Zaritsky 2006).Thanks to this,we can use circular aper-tures to calculate the profile.Because of the high resolu-tion of our images,the surface brightness given as log (f )inside the i-th circle having radius b i is obtained simply by adding up the flux of the stars falling inside the circle itself:log (f )=1/(πb 2i )×jΛj F jE.Chiosi &A.Vallenari:Three clusters of the SMC7-1012325201510F555W-F814W0.511.5-4.5-4-3.5log(r(arcsec))-1012325201510F555W-F814W0magnitudeF555W clusterF814W cluster 2022242628300.511.5F555W field F814W fieldFig.4.Summary panels for NGC 290.In the top left panel is the CM diagram (inner radius of 25”)with a superposed isochrone of log(age)=7.8,Z=0.003,E(F555W-F814W)=0.15.The OGLE bright stars are indicated by the triangles.The brightest stars settle at F555W=14.In top right is surface brightness profile (in arbitrary units)fitted with the King profile (dashed line)and with the Elson function (solid line).From this distribution we can determine the core and tidal radius.Vast majority of the stars sets within a radius of 25”.In the bottom left panel the CMD of field stars is shown (see text for details).The field population is taken at about 1.6’from the cluster center and the area is comparable to the area of the cluster (25”).The bottom right panel shows the completeness factors for the cluster and field regions as indicatedwhere Λj is the completeness factor inside the ring and F j the stellar flux.Crowding can cause a significant number of stars to be missed by ALLSTAR.Their flux can af-fect the surface brightness profile and must be accounted for.This is the reason why the completeness correction is applied to the flux determination.We restrict the de-termination of the surface profile to the area completely falling inside the small field of view of the ACS/WFC cam-era.In all the cases,the profiles have maximum radii of log(r)∼1.5arcsec.The sky background subtraction is a critical step in the determination of the surface profile especially in the8E.Chiosi &A.Vallenari:Three clusters of the SMC141618202004006008001000magNGC 265141618202004006008001000magK 29141618202004006008001000magNGC 290Fig.5.The luminosity functions of HST (solid line)data and OGLE II (dashed line)data for the field area are compared.OGLE II field areas are taken at a distance of 1deg from the clusters.The number of objects is normalized to an area of 22′′outer regions of a cluster.The field population is sampled as discussed in the previous section,the contamination is calculated as the mean flux in a field area of about 22′′,and is finally subtracted.Two families of models are fitted on the brightness profiles.The first one is the tidally truncated functions of King (1962):f (x )=πr 2c kln (1+x )−4(1+x )1/2−11+x t(4)with x =(r/r c )2and x t =(r t /r c )2where k is a constant and r t and r c are the searched parameters,specifically r t is the tidal radius and r c the core radius.The second one is the Elson et al.(1987)family of functions:f (r )=2πµ0a 2a 21−γ/2(5)where a is a scale length and γthe power law index.These models are essentially the same as the King (1962)models,but they do not assume tidal truncation.The parameter a is related to the King (1962)core radius r c by:r c =a (22/γ−1)1/2The profile fit is made using the χ2minimization.The un-certainties on the profiles are calculated as Poisson errors.5.2.1.Results and comparison with previous workFigs.2,3,4present the surface brightness profiles of each cluster and Table 3gives the derived parameters.The core radii obtained using Elson et al.(1987)and King (1962)profiles are substantially in agreement,as expected be-cause the main differences between the models arise at larger radii.The tidal radius is quite uncertain,first be-cause it strongly depends on the sky subtraction and sec-ond,because our photometry does not extend to large enough radii.Table 4compares our results on cluster pa-rameters with those by Hill &Zaritsky (2006).In compar-ison with our results,their values of r c are in agreement for NGC 265,but they tend to be overestimated for the other two clusters,especially concerning the fit with Elson et al.(1987)profiles.The values of γby Hill &Zaritsky (2006)are overestimated for all the clusters.The question whether MC clusters are tidally limited or not is a long lasting one.The King (1962)models are expected to describe bound systems that have isothermal and isotropic stellar distribution functions and are lim-ited by a strong tidal field.The Elson et al.(1987)models are empirically derived in order to reproduce the profiles of young MC clusters.The lack of tidal cut-offwas origi-nally explained with the dynamical youth of those clusters having extra-tidal stars.In this view,it is possible for a cluster to evolve or not from an Elson et al.(1987)pro-file to a King (1962)one,depending on the environment and on the cluster’s characteristics.However,on an ob-servational ground,no clear correlation is found between the age of the clusters and the presence of tidal cut-off.Hill &Zaritsky (2006)find that the SMC clusters are fit-ted in a satisfactory way by both models,although the King (1962)profiles provide a slightly superior fit in ∼90%of the cases.McLaughlin &van der Marel (2005)compare the King (1962)models,the Elson et al.(1987)profiles,and the modified isothermal spheres based on the Wilson (1975)models.These latter are spatially more ex-tended than the King (1962)functions,but still include a finite,tidal cut-offin the density.The authors come to the conclusion that the un-truncated power-law distri-butions,Wilson (1975)models,and King (1962)profiles yield comparable good fits of MC clusters.McLaughlin &van der Marel (2005)conclude that extended star en-velopes around MC clusters may not be transient features due to the age,but more probably halos of self-gravitating objects.For the LMC clusters,Elson et al.(1987)find a clear trend that the spread in core radius is an increasing function of the cluster age.The observed trend probably represents a real structural evolution of the clusters:all clusters form with a relatively small radius and subse-quently some of them experience a core expansion,while others do not.This result is confirmed by other authors (we quote as the most recent Mackey &Gilmore 2003).To check whether the trend found for the LMC is a property of SMC clusters as well,we combine the cluster age deter-minations derived from isochrone fitting by Chiosi et al.(2006)with the values of the King (1962)core r c obtainedE.Chiosi&A.Vallenari:Three clusters of the SMC9 Table3.Core and tidal radius as determined by thefit with the King(1962)and Elson et al.(1987)functions.King(1962)r c aγχ2(pc)(pc)2.610.75 1.062.40 2.50 1.270.87 1.50 1.55Table4.Structural parameters of the clusters as determinedby Hill&Zaritsky(2006).Elson et al.(1987)r c r90(pc)(pc)NGC265 3.51 3.20K29 5.63 6.00NGC290 1.83 3.25Cluster log(Age)(yr)Z E(F555W−F814W)NGC2658.5±0.30.004±0.0030.08±0.06K298.2±0.20.003±0.0020.14±0.08NGC2907.8±0.50.003±0.0020.15±0.05NGC2658.7±0.20.004±0.0030.08±0.06K298.3±0.20.003±0.0020.14±0.08NGC2908.0±0.30.003±0.0020.15±0.0510 E.Chiosi&A.Vallenari:Three clusters of the SMCstatistically subtracted from the LF and the CMD.Inside the radius of22′′about1000cluster stars are detected down to F555W=25where the incompleteness reaches the50%level.Starting from an initial guess obtained from visual inspection of the CMD,and using the CMD and LF simulator,by means of theχ2minimization we derive:log(Age)=8.5±0.3yr,interstellar redden-ing E(F555W-F814W)=0.08±0.06,corresponding to A F555W=0.21(Bedin et al.2005),andfinally metallicity Z=0.004±0.003.All the errors on the determinations of the parameters are given at the68%confidence level.The top left panel of Fig.2presents the CMD where the isochrone for the age and metallicity we have determined is superposed.The isochrone gives a good fit of the observational CMD.Different combinations of extinction,metallicity and age(inside the quoted errors) give reasonablefits of the CMD.For instance,when log(Age)=8.4yr,E(F555W-F814W)=0.1,and Z=0.007, the main sequence color,the turnoffmagnitude,and the luminosity of the loop of the core He-burning stars are well reproduced,while the theoretical loop is too red in comparison with the data.When log(Age)=8.6yr, E(F555W-F814W)=0.15,and Z=0.001,then the turnoffand the loop magnitudes and colors are well reproduced, but the colour of the unevolved part of the main sequence, two magnitudes about below the turnoffis poorlyfitted. On the other hand,it is clear that these features of the CMD depend on the details of the stellar models (treatment of convection,mixing length,bolometric corrections,adopted chemical mixture...).For this reason, at some extent,the derived set of bestfitting parameters is depending on the adopted stellar models.The turn-offmass is about4M⊙.The IMF slope in the mass range M>1M⊙turns out to beα2=2.5±0.5,while in the mass range(0.7<M<1M⊙)isα1=2.4±0.4, substantially in agreement with the Kroupa(2000)IMF. We recall that the Kroupa(2000)IMF hasα1=2.2for 0.5M⊙<M<1M⊙,α2=2.7for M>1M⊙.When binary stars are included a slightly older age is found.We add a percentage of binaries amounting to 30%of the stars.Instead of an age of log(Age(yr))=8.5 wefind the bestfit at an age of log(Age(yr))=8.7±0.2. As a consequence,the determination of the exponents of the IMF changes.For all the studied clusters,the summary of the IMF determination is presented in Table 6.The quality of thefit is shown in Fig.7where the observational LF is compared with the bestfit simulation.5.3.2.K29We sample the cluster population inside a radius of22”where about700stars are detected down to F555W=25 mag.As for NGC265,this radius is larger than the core radius.Thefield population of K29is located outside the core radius.Table6.The bestfit IMF coefficient in the range of low masses α1(0.7M⊙<M<1M⊙)and in the range of high massesα2 (M>1M⊙)is given for the three different clusters.The68% confidence interval is also given(σ1andσ2).No binariesBinariesFig.3(top left panel)presents the CMD of the cluster (inside the22′′radius),where bright stars that are sat-urated in the ACS/WFC photometry are taken from the OGLE II data,analogously to the procedure adopted in the determination of the brightness.The age derived by χ2minimization is log(Age)=8.2±0.2yr,the metallicity Z=0.003±0.002,and the reddening E(F555W-F814W) =0.14±0.08.The turn-offmass is about4.5M⊙.The slope of the IMF in the mass interval from4M⊙to1M⊙is α2=2.7±0.5,while in the mass range(0.7<M<1M⊙) isα1=1.8±0.2in marginal agreement with the Kroupa law.The LF of the observational data is compared with the bestfit model in Fig.8.When binaries are included, the age of the cluster becomes log(Age)=8.3±0.2yr.See the entries of Table6for the effect of binaries.5.3.3.NGC290Inside a radius of∼25′′about660stars are found.Once more this radius is larger than the cluster core.Evolved stars brighter than F555W=17.3mag are beyond the satu-ration level of the photometry.To cope with this we inte-grate our data for the red giants with those by OGLE II,whose photometry is converted in the F555W and F814W pass-bands,as described in the previous sections. Mounting the two sets of data has been made using equal areas in the two frames.No stars are missing in the HST photometry at the turnofflevel F555W=18.This ensures that no spurious effects due to the use of ground-based and HST photometry influences the age determination. The top left panel of Fig.4presents the composite CMD.As for the other two clusters,the preliminary inspec-tion of the CMD provides estimates of age and metallicity which is then refined by the Monte Carlo technique.The automaticfit gives log(Age)=7.8±0.5yr withχ2=1.5, metallicity Z=0.003±0.002,and E(F555W-F814W) =0.15±0.05.The IMF slope givesα1=2.2±0.2in the mass range0.7M⊙≤M≤1M⊙,andα2=2.7±0.4 in the mass range M≥1M⊙.Fig.4presents the CMD of NGC290compared with our bestfit isochrone.Fig9 presents the observational LF compared with the bestfit model.An older age is found when30%of binary stars16182022240500100015002000F555WNGC 265simulated population log(age(yr))=8.5observational dataFig.7.LF of the cluster NGC 265.The best fit is done with a population of log(Age)=8.5yr 1618202224050010001500F555WK 29simulated population log(age(yr))=8.2observational dataFig.8.LF of K 29fitted with a population of log(Age)=8.2yrare included:log(Age)=8.0±0.3yr.Once again see the entries of Table 6for the effect of binaries on the IMF parameters.5.4.Revisiting an old question:convective overshootBecker &Mathews (1983),comparing observational and synthetic CMDs of NGC 1866,first noticed that classical stellar models they used predicted a number of red gi-161820222402004006008001000F555WNGC 290simulated population log(age(yr))=7.8observational dataFig.9.LF of the cluster NGC 290fitted with a simulated population having log(Age)=7.8yrants larger than observed,and a smaller number of main sequence stars in turn as compared to the observations.The same authors suggested that a more careful treat-ment of core convection (rger convective cores)could remove the discrepancy.A number of subsequent studies confirmed this idea and demonstrated that the inclusion of overshooting in the description of convective motions could reproduce the correct ratio of the number of evolved stars N R over the number of main sequence stars,N MS .Chiosi et al.(1989a),in particular,clearly showed that the overshooting scheme,by reducing the ratio between the lifetime of the stars in the He-burning and the H-burning phase,t He /t H ,offers a good and simple solution to the problem.This conclusion was also reinforced by Lattanzio et al.(1991),who analysed the CMD of NGC 1866by means of overshooting and classical models.The classical theory of stellar structure predicts that in a star whenever the condition ∇r ≤∇a(6)is violated the region becomes unstable to convective mo-tions and fully mixed.This region is surrounded by sta-ble radiative layers.This is only an approximation:what happens physically at the border region passing from un-stable to stable conditions?If we define the Schwarzschild core as the region at border of which the acceleration of convective bubbles vanishes there is still a shell above in which the velocity is not zero (the so-called overshoot re-gion).The thickness,the thermal properties,and mixing efficiency in the overshooting region are still a matter of discussion.In any case,it is worth recalling that first con-vective overshoot is a consequence of the inertia principle,so that neglecting its existence would not be physicallysound,second it is quite common in Nature(Deardorffet al.1969).As demonstrated in a number of studies,in-cluding numerical simulations(Freytag et al.1996),the penetration depth of convective elements into a formally stable region represents a non-negligible fraction of the size of the unstable zone.In addition to this,there is a number of astrophysical situations in which the hypoth-esis of substantial convective overshoot has been found to offer better and more elegant solutions than other ex-planations(see Bertelli et al.1986a;Chiosi et al.1992). Among others,suffice it to recall here the so-called mass discrepancy of Cepheid stars(Bertelli et al.1986b;Chiosi et al.1992).Thefirst studies of this subject were by Shaviv &Salpeter(1973);Prather&Demarque(1974);Maeder (1975);Cloutman&Whitaker(1980b);Bressan et al. (1981);Stothers&Chin(1981),followed over the years by more sophisticated formulations standing on turbulence theories(Cloutman&Whitaker1980a;Xiong1980)and fluid hydro-dynamics(Canuto&Mazzitelli1991;Canuto et al.1996;Unno&Kondo1989;Canuto2000).The ballis-tic description long ago proposed by Bressan et al.(1981) is particularly suitable for the calculation of stellar models. It has been proved to best reproduce the numerical results of laboratoryfluid-dynamics simulations(Zahn1991).The Bressan et al.(1981)procedure will be shortly summarized below.Despite all this,it has been often argued that unre-solved binaries could mimic the effects of convective over-shoot and easily account for the low ratio of evolved stars to main sequence stars observed in the young LMC clus-ters.This hypothesis has been investigated by many au-thors,both in open clusters of the Milky Way(see Carraro et al.1994)and young clusters of the LMC(Chiosi et al. 1989a,b;Vallenari et al.1992)with somewhat contrast-ing results.Worth of mention is the study by Testa et al. (1999)of NGC1866,who introducing about30%of binary and using the classical models of Dominguez et al.(1999) came to the conclusion that convective overshooting is not required.The subsequent analysis of Barmina et al.(2002) of the same cluster carried out on the Testa et al.(1999) data,discovered that the correction for completeness ap-plied by Testa et al.(1999)was not correct thus invalidat-ing their conclusions.In addition to this,they performed a systematic analysis of the effects of binary and stochastic fluctuations in the IMF,and came to the opposite conclu-sion:convective overshoot in unavoidable.Here we have three clusters whose turn-offmass is about4M⊙so it might be worth of interest to contribute further to this subject.In order to check which model bet-ter reproduces the data we need to have different sets of stellar populations.They are the two sets with convective overshoot,i.e.the models by Girardi et al.(2002)and those by Pietrinferni et al.(2004)with a different pre-scription so that comparison is possible.As already recalled,the ballistic description of con-vective overshoot by(Bressan et al.1981)is particularly suited to stellar models.The algorithm adopts a non-local treatment of convection in the context of the mixing-length theory(MLT)by B¨o hm-Vitense(1958):it looks for the layers where the velocity of convective elements (accelerated by the buoyancy force in the formally unsta-ble regions)gets zero into the surrounding stable regions, then adopts a suitable temperature stratification in the overshoot regions,andfinally assumes straight mixing over-there.Since the Bressan et al.(1981)formalism makes use of the MLT,it expresses the mean free path of the convective elements as l=Λc×H p where H p is the local pressure scale height.According to this definition adopted by Bressan et al.(1981)Λc represents the overshoot distance across the Schwarzschild radius in units of the pressure scale height.In Bressan et al. (1981)Λc was assumed to be the same for all stellar masses.Over the years,this was slightly modified to treat overshoot in that particular range of mass which sees the transition from radiative core H-burning to convective core H-burning and during this phase the convective core starts small,grows to a maximum and then decreases.In this mass intervalΛc is not kept constant but let vary with mass according to the recipe in Girardi et al.(2000):1)Λc=0for M≤1.0M⊙(when the core is radiative)2)Λc=M/M⊙−1.0for1M⊙<M≤1.5M⊙3)Λc=0.5for M>1.5M⊙The last value stands also for the whole He-burning phase.Pietrinferni et al.(2004)adopt a similar prescription:1)Λc=0for M≤1.1M⊙(when the core is radiative)2)Λc=(M/M⊙−0.9)/4for1M⊙<M≤1.5M⊙3)Λc=0.2for M≥1.7M⊙where we have to keep in mind thatΛc is”measured”from the Schwarzschild border.Therefore it corresponds to about half of the Girardi et al.(2000)definition. Considered these premises,the two sets of tracks are prac-tically coincident.Thisfinding also ensures that using the classical models by Pietrinferni et al.(2004)is safe as the input physics adopted by the two groups is nearly the same.The analysis is made comparing the integrated lumi-nosity function of main sequence stars normalized to the number of evolved stars(thereinafter NILF)first intro-duced by Chiosi et al.(1989a),which has been proved to be the only way to effectively discriminate between the two different evolutionary schemes.It is worth reminding the reader that the NILF is by definition proportional to the lifetime ratio t H/t He(Chiosi et al.1989a).The NILF requires that the number of evolved stars truly belonging to the cluster is known.The task is not trivial because it requires an accurate decontamination by field stars.The procedure is as follows.First of all,we ap-ply to the CMD of the cluster andfield the correction for completeness and area coverage.Second in the area of the cluster andfield CMD we perform star counts.We statistically subtract from the cluster counts a number of。
On the massive stellar population of the Super Star Cluster Westerlund 1
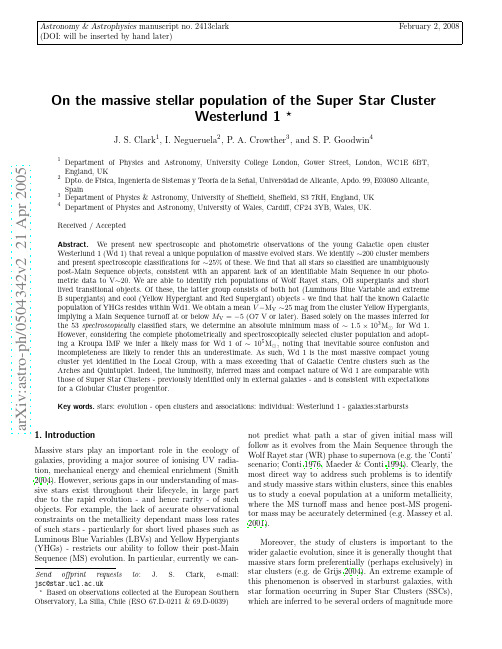
a r X i v :a s t r o -p h /0504342v 2 21 A p r 2005Astronomy &Astrophysics manuscript no.2413clark February 2,2008(DOI:will be inserted by hand later)On the massive stellar population of the Super Star ClusterWesterlund 1⋆J.S.Clark 1,I.Negueruela 2,P.A.Crowther 3,and S.P.Goodwin 41Department of Physics and Astronomy,University College London,Gower Street,London,WC1E 6BT,England,UK 2Dpto.de F ´ısica,Ingenier ´ıa de Sistemas y Teor ´ıa de la Se˜n al,Universidad de Alicante,Apdo.99,E03080Alicante,Spain3Department of Physics &Astronomy,University of Sheffield,Sheffield,S37RH,England,UK 4Department of Physics and Astronomy,University of Wales,Cardiff,CF243YB,Wales,UK.Received /AcceptedAbstract.We present new spectroscopic and photometric observations of the young Galactic open cluster Westerlund 1(Wd 1)that reveal a unique population of massive evolved stars.We identify ∼200cluster members and present spectroscopic classifications for ∼25%of these.We find that all stars so classified are unambiguously post-Main Sequence objects,consistent with an apparent lack of an identifiable Main Sequence in our photo-metric data to V ∼20.We are able to identify rich populations of Wolf Rayet stars,OB supergiants and short lived transitional objects.Of these,the latter group consists of both hot (Luminous Blue Variable and extreme B supergiants)and cool (Yellow Hypergiant and Red Supergiant)objects -we find that half the known Galactic population of YHGs resides within Wd1.We obtain a mean V −M V ∼25mag from the cluster Yellow Hypergiants,implying a Main Sequence turnoffat or below M V =−5(O7V or later).Based solely on the masses inferred for the 53spectroscopically classified stars,we determine an absolute minimum mass of ∼1.5×103M ⊙for Wd 1.However,considering the complete photometrically and spectroscopically selected cluster population and adopt-ing a Kroupa IMF we infer a likely mass for Wd 1of ∼105M ⊙,noting that inevitable source confusion and incompleteness are likely to render this an underestimate.As such,Wd 1is the most massive compact young cluster yet identified in the Local Group,with a mass exceeding that of Galactic Centre clusters such as the Arches and Quintuplet.Indeed,the luminosity,inferred mass and compact nature of Wd 1are comparable with those of Super Star Clusters -previously identified only in external galaxies -and is consistent with expectations for a Globular Cluster progenitor.Key words.stars:evolution -open clusters and associations:individual:Westerlund 1-galaxies:starbursts1.IntroductionMassive stars play an important role in the ecology of galaxies,providing a major source of ionising UV radia-tion,mechanical energy and chemical enrichment (Smith 2004).However,serious gaps in our understanding of mas-sive stars exist throughout their lifecycle,in large part due to the rapid evolution -and hence rarity -of such objects.For example,the lack of accurate observational constraints on the metallicity dependant mass loss rates of such stars -particularly for short lived phases such as Luminous Blue Variables (LBVs)and Yellow Hypergiants (YHGs)-restricts our ability to follow their post-Main Sequence (MS)evolution.In particular,currently we can-2J.S.Clark et al.:The stellar content of Wd1massive than galactic open clusters.Such objects may have very different basic properties from their smaller rela-tives,with many authors arguing for different kinds of top-heavy mass distributions(e.g.M82-F;Smith&Gallagher 2001).The relevance of such a possibility is clear when one considers that a large fraction of the stellar popula-tion of galaxies may have formed in starburst episodes in the distant past.If such episodes,in which very large num-bers of stars form,are in any way fundamentally different from the much more modest star formation episodes that we observe in the present Milky Way,our modelling of the history and evolution of galaxies could be profoundly biased.Unfortunately,dense clusters of massive stars are rare in the galaxy.The Arches&Quintuplet Galactic Centre clusters(with masses of∼1×104M⊙;Figer2004)are the most massive known;however,observations are hampered by their extreme reddening.Recently,observations of the apparently unremarkable cluster Westerlund1(henceforth Wd1)have demonstrated that for over4decades the as-tronomical community has possibly overlooked the pres-ence of a SSC in our own galaxy.Discovered by Westerlund(1961),photometric (Borgman et al.1970,Lockwood1974and Koornneef 1977)and spectroscopic(Westerlund1987;West87) surveys suggested the presence of a population of highly luminous early and late type supergiants.However, despite these observations Wd1languished in relative obscurity throughout the past decade,due in large part to the significant reddening(A v∼12.9mag.Piatti et al.1998)which made high resolution spectroscopic observations difficult.Motivated by the unusual radio and mid-IR properties of two cluster members(Clark et al.1998),we have un-dertaken an extensive program of spectroscopic and pho-tometric observations of Wd1.Thefirst results of this program-the discovery of an unexpected large popula-tion of WRs and a new LBV-have already been reported in Clark&Negueruela(2002,2004).Here we report on the remainder of the observations,specifically their use in constraining the properties of the population of massive post MS objects within Wd1.The paper is therefore structured as follows.In Sect. 2we briefly describe the observations and the data reduc-tion techniques employed.Sections3&4are dedicated to the presentation and analysis of both spectroscopic and photometric datasets.Finally,we present an analysis of the global properties of the cluster in Sect.5and sum-marise the results in Sect.6.2.Observations&Data ReductionLow resolution spectroscopy of cluster members over the red/near-IR spectral region(∼6000to11000˚A)was taken on2001June23rd to25th from the ESO1.52m tele-scope at La Silla Observatory,Chile.The telescope was equipped with the Boller&Chivens spectrograph,the Loral#38camera and the#1(night1)and#13(nights 2and3)gratings,giving dispersions of∼5˚A/pixel and ∼7˚A/pixel-leading to resolutions of≈11˚A and≈16˚A -respectively.Intermediate-resolution spectroscopy of the brighter cluster members was carried out on2002June7th,us-ing the ESO Multi-Mode Instrument(EMMI)on the3.6 m New Technology Telescope(NTT)at La Silla.The Red Medium Resolution mode was used.The instrument was equipped with gratings#6and#7in the red arm and the new2048×4096MIT CCD mosaic(in the2×2binning mode).Grating#7covered theλλ6310–7835˚A region at a dispersion of∼0.8˚A/pixel,while grating#6covered the λλ8225–8900˚A range at∼0.4˚A/pixel.Data pre-reduction was carried out with MIDAS soft-ware,while image processing and reduction were ac-complished with the Starlink packages ccdpack(Draper 2000)and figaro(Shortridge1997).Finally photometric observations of thefield contain-ing Wd1were made with the SUperb-Seeing Imager2 (SuSI2)direct imaging camera on the NTT;the2mo-saiced2k×4k CCDs providing a5.5×5.5arcminfield of view.Broadband UBVRI1images were obtained in ser-vice time on2001August21,with integration times for individual frames ranging from1200s(U band)to2s(R &I bands).Given the significant interstellar reddening an-ticipated for Wd1a selection of nearby red Landolt(1992) standards was observed.Debiasing andflatfielding(both dome and twilightflatfield frames were obtained)were accomplished with the Starlink package kappa.Final photometry was kindly extracted for us by Peter Stetson, using the daophot package in IRAF2(Stetson1991). 3.Spectroscopic classification.In total,27stars were observed at intermediate resolution between6500and7900˚A and8200and8900˚A,and an additional26stars-including the11WRs identified in Clark&Negueruela(2002)-were observed at low resolu-tion between6500and8900˚A.Afinding chart for all spec-troscopically observed stars is presented in Fig.1.Spectra are presented in Figs.2(new WR candidates;Sect.3.1), 3-7(Super-and Hyper-giants;Sect.3.2-3.5)and8(the unusual sgB[e]star W9;Sect.3.3.1).The6500to9000˚A window offers the prospect of spectral classification of moderately reddened objects for which traditional optical criteria are unavailable.Given the presence of transitions from H i,He i and numerous low-e.g.Fe i,Ca ii and N i-and high-e.g.He ii,C iii and N iv-ionisation species it would appear possible to construct an accurate classification scheme.While au-thors have recognised the importance of this window(e.g.J.S.Clark et al.:The stellar content of Wd134J.S.Clark et al.:The stellar content of Wd1Andrillat etal.1995),comparatively few observations ex-ist within the literature,particularly of high luminosity and/or early type stars.We were therefore forced to adopt a twin approach for spectral classification of our spectra -comparison to observational data where possible(spec-tral type mid-B→G)supplemented by construction of a grid of synthetic spectra where required(OB supergiants; Appendix A).The results of the spectral classification are presented in Table1,along with the original classification by West87. Where possible we have adopted the nomenclature of West87;if an individual object is resolved into two or more stars in our data we adopt the convention of retaining the designation of West87,with the addition of the suffix a for the brightest component,b for the second brightest etc.We further present the J2000co-ordinates and the BVRI broadband photometry for each object if available.Note that while we have utilised a grid of synthetic spectra for the classification of the OB star population of Wd1we do not claim that these represent tailored analyses of individual stars.Given the breadth and com-plexity of the spectra presented,quantitative analysis is clearly beyond the scope of the current work,which aims to provide coarse spectral classifications for the objects presented.3.1.The candidate Wolf Rayet StarsClark&Negueruela(2002)reported the serendipitous dis-covery of11WR stars within Wd1in the low resolution2001ESO1.52m dataset,comprising6WN and5WC stars.Analysis of the2002intermediate resolution NTT data reveals a further two candidates within the cluster. These are W44and W66(=Candidates L&M respec-tively,following the notation of Clark&Negueruela2002); 6500to7900˚A spectra for both stars are presented in Fig 2.Turningfirst to W66,Fig.2reveals prominent blended emission in He ii6560+C ii6578˚A,C ii6725-42+C iii 6727-73˚A,C iii7037+He i7065+C iv7064˚A and C ii 7236+C iii7210-12˚A.Difficulty in defining a continuum shortwards of7000˚A prevents the accurate measurement of line strengths for thefirst3blends,but we measure an EW∼−130±15˚A for the C ii7236+C iii7210-12˚A blend. For the lowest excitation WC8star(WR53),the C iv 7065˚A and C ii7236˚A lines are of similar strength,while all WC9stars have C ii7236˚A>>C iv7065˚A,indicating a classification of WC9for W66.W44demonstrates emission in the comparatively low ionisation lines of Hα(∼−30˚A),He i6678(∼−8˚A)and 7065(∼−14˚A),with no emission from either higher(e.g. He ii,N iii-iv or C iii-iv)or lower ionisation species(e.g. Fe ii).The spectrum in the8200to8900˚A range appears completely featureless(to a limit of EW±0.5˚A)and hence is not presented.Given the apparent magnitude of W44,combined with the strength of the emission lines, the lack of Paschen lines(emission and absorption)and Fig.2.R band spectra of the2newly discovered WR stars in the cluster;Candidate L(=W44),a probable WN9star and Candidate M(=W66),a sixth WCL star(WC9). low ionisation species,we exclude a B emission line star classification-such as classical Be or sgB[e]star-and in-stead suggest a WN identification.The lack of N iv7116˚A precludes a classification earlier than WN9,with a clas-sification of WN9rather than WN9ha more likely due to the comparative strength of the He i lines.This is thefirst such star identified within Wd1,although we note that the low resolution spectrum of W5is possibly indicative of an even later WNL classification-this is detailed in Sect.3.3.3.3.2.The late O&early-B supergiantsIntermediate resolution spectra of all13stars in Fig.4 and W7,33,70&71in Fig.5fulfill the criteria for OB spectral types defined in Appendix A and individual clas-sifications are listed in Table1.The FWHM of the Paschen lines clearly satisfy the criterion of Caron et al.(2003)for supergiants,forming a continuous sequence with the later A-F stars in Fig.5,which we demonstrate in Sect.3.4to also be super-or hyper-giants.J.S.Clark et al.:The stellar content of Wd15 Table1.Summary of the53cluster members with spectroscopic classifications.Column1provides the notation of West87,while Column2provides our additional notation if required.Columns3&4provide the Equ J2000co-ordinates,while broadband BVRI photometric values are presented in Columns5-8where available.Columns9& 10list spectral classifications from West87and this work,respectively.Classifications listed in italics were derived from low resolution spectra.In general wefind an encouraging correspondence between the two works,although our classifications appear to be systematically earlier for those stars classified as late B-early G by West87.Importantly we confirm of the classification of West87’s four YHG candidates,while identifying a further2candidates.Finally,we highlight one important difference;we are unable to identify any Main Sequence or giant stars,with all the OB stars in our study being classified as Supergiants(W6,10,11,28,33,70&71receiving revised luminosity classes).(new)W87New6J.S.Clark et al.:The stellar content of Wd 1Fig.3.Intermediate resolution R band(6400to7800˚A)spectroscopy of O-F supergiant and hypergiant members of Wd1.Prominent transitions of H i,He i and O i are indicated.Left panel:O and mid B supergiants;wefind no evidence for He ii absorption in the spectra.Right panel:mid-B to F supergiants in Wd1.Weak C ii6578/82˚A absorption is visible in the red wing of the Hαline in the mid-B supergiants.We tentatively identify the three prominent absorption features between7420-7450˚A in W243and W16a as N i7423.6,7442.3&7468.3˚A.Weak Fe i lines at7112.2and 6999.9˚A also appear to be present in the spectrum of W12a(A5Ia+)and later objects.Possible identifications of the absorption features in the mid-A and later spectral types at∼7711˚A and∼7748˚A are Fe ii7712and Fe i7748.3˚A respectively.Wefind W57a,70&71to have the latest spectral type -B3Ia-of the continuous sequence of OB supergiants present in the sample.This classification may be assigned on the basis of the presence and strength of weak N i and O i8446˚A absorption.While W7&33apparently are of later spectral type,their spectra demonstrate several un-usual properties that suggest they have more in common with the transitional objects such as the LBV W243and the YHGs;hence we defer discussion until Sect.3.3.The remaining spectra display solely H i and He i pho-tospheric absorption lines,implying subtypes of B1.5Ia or earlier(T eff≥20kK).In addition,wefind no signature of the He ii8238˚A line in any object,suggesting subtypes no earlier than O9.5Ia.Further subclassification is ham-pered by the poor S/N of most spectra,which precludes the use of the Pa16+C iii/Pa15line ratio.Consequently,we may only attempt additional clas-sification based on the strengths of the Paschen lines. Measuring the strengths of the Paschen lines in the ob-served(and synthetic)spectra relative to W70&71(B3 Ia model)suggests an approximate range of spectral types for W61a,60,43a,19,23a,11,2a&28(earliest→latest) of O9.5Ia→B0.5Ia(30kK→24kK).While the system-atic weakening in line strength-resulting in the absence of higher Paschen series lines-of stars such as W61a& 60compared to W2a&28is clearly indicative of gradu-ations in spectral type within this group,we refrain from attempting any further subclassification based on current data.Finally,while the spectra of W30,13,61b&24are almost completely devoid of photospheric features and hence suggest spectral types of O9Ia or earlier,the ab-sence of He ii8238˚A apparently precludes such a possi-bility.Instead we consider it likely that possible binarity or blending of2nearby objects leads to the featureless spectra and hence refrain from a classification other than generic early OB supergiant for these4objects.Although the S/N for many spectra is rather poor, wefind no evidence for a photospheric Hαprofile as pre-dicted by our synthetic spectra in the temperature range 10-34kK.A montage of the region containing the Hαline for those spectra with the largest S/N is presented in Fig. 6;wefind evidence for either infilling(W19,23a,24,43a, 57a60,61b&70)or emission(W11,13,28,30,61a& 71)in all spectra.Preempting the results of Sect.4,in the absence of an accurate photometric determination of bolo-metric luminosity,we may use the presence of Hαemission to infer a large luminosity and initial mass for the OB su-J.S.Clark et al.:The stellar content of Wd178J.S.Clark et al.:The stellar content of Wd1J.S.Clark et al.:The stellar content of Wd19Fig.6.Montage of the region of the spectrum containing Hαfor a selection of the OB supergiants.The left panel shows sample spectra for the O9.5Ia to B3Ia stars,the presence of either infilling or Hαemission indicating a Ia luminosity classification.The right panel shows the Hαprofiles for the two highly luminous mid-B hypergiants W7&33,note the narrow line superimposed on a broader plinth of emission.pergiants.Specifically,McErlean et al.(1999)demonstrate that of their sample all the most luminous(M∼30-40M⊙) B supergiants demonstrate Hαemission.Fig.7presents a montage of the low resolution spec-tra of OB star candidates;where intermediate resolution spectra exist,these have been overplotted.Given both the lower resolution and S/N of the spectra,detailed classifica-tion is difficult.Hence we simply choose to divide the spec-tra into three morphological groups based on the presence of various photospheric features.Group1contains those stars without an obvious Paschen series and consists of W13,14a,15&44.Group2contains those stars with the Paschen series in absorption and consists of W2a,5,6,10, 11,23a,24,29,43a,55,56&238.Finally,group3consists of those stars with both Paschen and the O i7774˚A blend in absorption,and consists of W7,33,41,42,70&71.Of the group1objects W44and W13have already been discussed.With an essentially featureless spectrum we suspect that W15is likely a binary or blend of one or more OB SGs.The strong N iv emission seen in W14a is unexpected since this would imply an early O supergiant classification,at odds with the expected age of the cluster (Sect.5).However,we note that W14a appears to be com-posed of2or more stars;hence an alternative explanation would be a blend containing at least one hitherto unde-tected WNE star.Clearly,future observations are required to resolve this apparent inconsistency.With a lack of O i7774˚A absorption,the group2ob-jects appear to have earlier spectral types than∼B3Ia. Indeed all those objects for which intermediate resolution observations are available are of spectral type O9.5Ia to B0.5Ia-hence we assume a late O/early B supergiant classification for the remaining objects,with the sole ex-ception of W5,which,together with W7and33,we discuss in Sect.3.3.3.Of the group3spectra W41&42have only been ob-served at low resolution.W41apparently shows weak O i absorption,although it is coupled with an unexpectedly weak Paschen series.Hαis clearly seen in absorption;the only such object in our dataset.Given the rather unusual nature of the spectrum we tentatively suggest the spec-trum may be a blend of two or more stars.By contrast W42a has pronounced Hαemission and appears similar to the mid B supergiants W7&33(Sect.3.3.3)and is discussed therein.Finally,comparison of the two epochs of observations of W23a and43a are suggestive of variability,with Hαemission conspicuous in2001,but apparently absent in 2002.3.3.Anomalous B supergiantsAmongst the observations of the OB supergiants within the cluster wefind a significant number of objects with spectra demonstrating unusual properties.In this subsec-tion we detail these individually.3.3.1.The sgB[e]star W9The spectrum of W9is presented in Fig.8and is dom-inated by H i,He i and low ionisation metallic emis-sion(Fe ii,Ca i,N i and O i),with narrow,single peaked emission lines(FWHM(Hα)=125kms−1)and is notable for the complete lack of any photospheric features and the strength of certain emission features(e.g.Wλ(Hα)=-520±30˚A,Wλ(OI)=-48±2˚A).To the best of our knowledge such an equivalent width for Hαis completely unprece-dented for an early type emission line star,with the ex-ception of the extreme systems SS433(X-ray binary;e.g. Falomo et al.1987)andηCar(LBV;e.g.Davidson et al. 1999).Radio observations(Clark et al.1998,Dougherty et al.2004)reveal a strong,compact radio source to be associated with W9,while mid IR observations likewise10J.S.Clark et al.:The stellar content of Wd 1Fig.7.Low resolution spectra of cluster OB supergiants(solid black lines).Where available high resolution spectra (from Figs.4-6)are smoothed and overplotted(dotted red lines)on the corresponding low resolution spectra for comparison.Due to the low resolution an accurate determination of spectral type is impossible;hence we simply divide the spectra into3morphological classes based on the presence or otherwise of the Paschen lines and the O i 7774˚A feature;see Sect.3.2for further details.show strong emission(F10µm=50Jy),although the emis-sion mechanism(thermal dust or f-f/b-f)is still uncer-tain.Finally,an ISO-SWS spectrum reveals the presence of[O iv]emission,implying an exciting source with a tem-perature in excess of∼80kK must be present(Clark et al. 1998).While a quantitative analysis of the combined dataset is beyond the scope of this work,we note that the ob-servational properties of W9are unique within Wd1,and apparently amongst the wider galactic population of early OB stars.While it fulfills the classification criteria for su-pergiant B[e]stars of Lamers et al.(1998)we suspect that the emission line spectrum arises at least partially in the compact circumstellar envelope rather than in a stellar wind(Clark et al.1998).As such it could closely resem-ble the unusual object NaSt1,which Crowther&Smith (1999)propose to consist of a compact ejection nebula sur-rounding-and obscuring-an exciting WNE object.Such an hypothesis would naturally explain the[O iv]emission present in W9,although one might question the compact nature-and hence implied youth-of the radio nebula when contrasted to other LBV nebulae,given the require-ment for the central star to evolve through both the LBV and WNL phases.Clearly,while such an objection could be overcome by proposing a compact WR+LBV binary system,we also suggest an alternative possibility,namely that W9is the result of a recent merger event.Given the severely crowded nature of the central regions of the cluster(Sect.5.4) stellar collisions and interactions appear likely.A recent (∼103yr)interaction in which the outer H mantle of one or both objects were lifted offto form the compact radio nebula would reveal the hot interior layers of the star(s)so affected.This would then naturally explain the high exci-tation emission-until the remnant relaxed to a new equi-librium structure on a thermal timescale(∼104yrs);a sce-nario proposed by Clark et al.(2000)to explain the stellar and nebular morphology of the candidate LBV G25.5+0.2.3.3.2.The Luminous Blue Variable W243Clark&Negueruela(2004)present multi-epoch data that clearly demonstrate that W243-initially classified as an early B supergiant by West87-has evolved in the inter-vening decades to demonstrate a much cooler,A super-giant spectrum,albeit with anomalous emission features. The overall spectrum closely resembles that of the lumi-nous A supergiant IRC+10420(e.g.Oudmaijer1998), with the notable exception of emission in the He i6678 and7065˚A lines.Clark&Negueruela(2004)advanced the suggestion that W243was an LBV,with the unusual com-posite spectrum resulting from the formation of a pseudo-photosphere in a dense stellar wind.Fig.8.R and I band spectra of the sgB[e]star W9.Note that we are unable to identify the narrow emission features at7134,7233&7280˚A.Subsequently,de Koter(2004,m.)suggested that as presented such an hypothesis would predict sig-nificantly stronger Hαemission(EW∼−100˚A)than ob-served.However,the presence of He i emission and strong electron scattering wings(extending to±103kms−1)in the Hαemission profile apparently excludes a simple alterna-tive explanation that W243now has the physical proper-ties-and in particular a rather low mass loss rate-of a bonafide A supergiant.Consequently,we suspect that a hybrid model may provide the correct explanation-viz.a cooling of the un-derlying star to a late B spectral type-still sufficient to produce He i emission-coupled with an increase in mass loss rate to simulate a later spectral type.3.3.3.Extreme B supergiants W5,7,33&42aBased on the strength of the O i8446˚A and N i features present in the intermediate resolution spectra(Fig.5),W7 &33appear to be B5supergiants.However,closer exami-nation shows a weak P Cygni profile in the O i8446˚A line of both stars-to the best of our knowledge absent from any other mid-B supergiant,with the notable exception of the2002spectrum of the LBV W243.Examination of the Hαprofiles also shows that they differ from the weak P Cygni profiles expected for B su-pergiants,being composed of a single narrow peak super-imposed on a broad emission base(Fig.6).In this re-spect they again resemble the LBV W243(Fig.3),while the broad emission component is observed for the clus-ter member YHG W16a(Sect.3.4).Moreover,similar composite Balmer line profiles are also obtained for the ‘B-supergiant LBVs’studied by Walborn&Fitzpatrick (2000).Consequently,we propose that both objects are to be found in a shortlived,transitional evolutionary state more closely related to the LBV and YHG population of Wd1than the late O/early B supergiant population. Indeed,both stars are a magnitude more luminous than any other OB supergiant within the cluster,with an ob-served V band magnitude directly comparable to the YHG population.Comparable objects might therefore be the highly lu-minous B1.5a+hypergiants HD80077(Carpay et al. 1991)and HD152236(Van Genderen et al.1984),which are also characterised by strong Hαemission due to mass loss rates an∼order of magnitude greater than Ia and Ib supergiants of similar spectral type(e.g.Leitherer et al. 1995).Of the stars for which only low resolution spectra exist, wefind that W42a has a comparable morphology to both W7&33,suggesting a similar classification.W5demon-strates both dramatic Hαand also C ii∼7236˚A emis-sion,previously only identified in the WCL population. However,the lack of C iii9705˚A emision(not shown in Fig.7)precludes an identification as a binary/blend con-taining a WCL star.Recent high resolution spectra ob-tained by us(Negueruela&Clark2004,in prep)confirm the identification of C ii emission,while also revealing the presence of weak He i P Cygni emission profiles.Such a morphology,with a lack of N iv7116emission,is indica-tive of either a very late WN star(WN9or later)or an extreme early BIa+supergiant,the lack of a He ii transi-tion in the current data precluding a further classification.3.4.The Yellow HypergiantsThe remaining6spectra in Figs.3&5are seen to be dom-inated by low ionisation metallic photospheric lines,sug-gesting spectral types later than prehensive classi-fication criteria and standard spectra for cool supergiants are provided by Munari&Tomasella(1999).Comparison to their data,employing the relative strengths of the Ca ii lines and adjacent Paschen series lines-providing the same diagnostic as the Ca ii H&K versus the Balmer line strength in the optical region-achieves a broad clas-sification for B8-F8supergiants.This may then be further refined by consideration of the presence and strength of the N i transitions between8650to8750˚A for A super-giants and the appearance of Fe i and Si i lines for the F supergiants in our sample.When combined with the (lower resolution)observations of Cenarro et al.(2001) we estimate a classification accuracy of±2spectral sub-types for our current observations,from which we identify two A and four F stars(Tables1&2).The narrow line widths,forming a smooth progres-sion from the OB supergiants,together with the pro-nounced N i absorption features(c.f.Figs.17-25of Munari &Tomasella1999)clearly justifies at least a supergiant classification for the6stars in question.However,deter-mination of the bolometric luminosity of A-G stars may be made directly via the strength of the O i7774˚A blend;a re-lationshipfirst identified by Merrill(1925).We make use of the most recent calibration of this relationship by Arellano Ferro et al.(2003;their Eqn.2);which is calibrated from M V=+0.35to−9.5mag.The results are presented in Table2,with four stars(W4,12a,16a&265)apparently demonstrating absolute visual magnitudes in excess of the current calibration for the relationship.Consequently,we simply limit ourselves to the conservative statement that these stars have M V∼−9.5(log(L∗/L⊙)∼5.7);placing them at the empirical Humphreys-Davidson limit for cool hypergiants(under the assumption of negligible bolomet-ric correction).We further note that both the presence of chemically processed material at the stellar surface and/or infilling of the blend due to emission from a stellar wind will only cause a systematic underestimate of the true luminosity;such an effect is apparently observed for the YHGρCas.Therefore,we conclude the six A-F stars in Wd1have luminosities that are at least directly compa-rable to those of thefield populations of YHGs found in both the galaxy and the Magellanic Clouds.However,as described by de Jager(1998)a high lumi-nosity(M V>-7)is not sufficient to designate a star as a bonafide YHG.Rather,he defines the‘Keenan-Smolinski’criteria for the spectroscopic classification of hypergiants;(i)the presence of one or more broad components of Hαand(ii)absorption lines that are significantly broader than Table2.Equivalent widths for the O i∼7774˚A blend for the six yellow hypergiants in Wd1,with absolute visual magnitude and resultant luminosity determined directly from the relationship of Arellano Ferro et al.(2003;formal error of±0.4mag.).4F2Ia+ 3.0-10.18F5Ia+ 2.1-8.712a A5Ia+ 2.8-9.916a A2Ia+ 2.7-9.832F5Ia+ 2.3-9.1265F5Ia+ 2.6-9.7。
The Globular Cluster System of NGC 1316 (Fornax A)
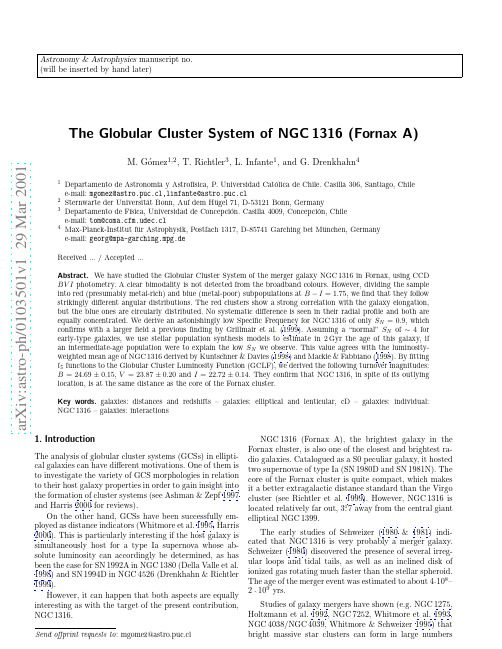
a r X i v :a s t r o -p h /0103501v 1 29 M a r 2001Astronomy &Astrophysics manuscript no.(will be inserted by hand later)The Globular Cluster System of NGC 1316(Fornax A)M.G´o mez 1,2,T.Richtler 3,L.Infante 1,and G.Drenkhahn 41Departamento de Astronom ´ıa y Astrof ´ısica,P.Universidad Cat´o lica de Chile.Casilla 306,Santiago,Chilee-mail:mgomez@astro.puc.cl,linfante@astro.puc.cl 2Sternwarte der Universit¨a t Bonn,Auf dem H¨u gel 71,D-53121Bonn,Germany 3Departamento de F ´ısica,Universidad de Concepci´o n.Casilla 4009,Concepci´o n,Chile e-mail:tom@coma.cfm.udec.cl 4Max-Planck-Institut f¨u r Astrophysik,Postfach 1317,D-85741Garching bei M¨u nchen,Germany e-mail:georg@mpa-garching.mpg.deReceived .../Accepted ...Abstract.We have studied the Globular Cluster System of the merger galaxy NGC 1316in Fornax,using CCD BV I photometry.A clear bimodality is not detected from the broadband colours.However,dividing the sample into red (presumably metal-rich)and blue (metal-poor)subpopulations at B −I =1.75,we find that they follow strikingly different angular distributions.The red clusters show a strong correlation with the galaxy elongation,but the blue ones are circularly distributed.No systematic difference is seen in their radial profile and both are equally concentrated.We derive an astonishingly low Specific Frequency for NGC 1316of only S N =0.9,which confirms with a larger field a previous finding by Grillmair et al.(1999).Assuming a “normal”S N of ∼4for early-type galaxies,we use stellar population synthesis models to estimate in 2Gyr the age of this galaxy,if an intermediate-age population were to explain the low S N we observe.This value agrees with the luminosity-weighted mean age of NGC 1316derived by Kuntschner &Davies (1998)and Mackie &Fabbiano (1998).By fitting t 5functions to the Globular Cluster Luminosity Function (GCLF),we derived the following turnover magnitudes:B =24.69±0.15,V =23.87±0.20and I =22.72±0.14.They confirm that NGC 1316,in spite of its outlying location,is at the same distance as the core of the Fornax cluster.Key words.galaxies:distances and redshifts –galaxies:elliptical and lenticular,cD –galaxies:individual:NGC 1316–galaxies:interactions1.IntroductionThe analysis of globular cluster systems (GCSs)in ellipti-cal galaxies can have different motivations.One of them is to investigate the variety of GCS morphologies in relation to their host galaxy properties in order to gain insight into the formation of cluster systems (see Ashman &Zepf 1997and Harris 2000for reviews).On the other hand,GCSs have been successfully em-ployed as distance indicators (Whitmore et al.1995,Harris 2000).This is particularly interesting if the host galaxy is simultaneously host for a type Ia supernova whose ab-solute luminosity can accordingly be determined,as has been the case for SN 1992A in NGC 1380(Della Valle et al.1998)and SN 1994D in NGC 4526(Drenkhahn &Richtler 1999).However,it can happen that both aspects are equally interesting as with the target of the present contribution,NGC 1316.2M.G´o mez et al.:The Globular Cluster System of NGC1316(Fornax A)during a merger event.But despite the strong evidence for a previous merger in NGC1316,the only indication for cluster formation is that Grillmair et al.(1999,here-after Gr99),could not see a turnover in the GCLF at the expected magnitude.They interpreted thisfinding as an indication for an enhanced formation of many less massive clusters,perhaps in connection with the merger.However, their HST study was restricted to the innermost region of the galaxy.In contrast to other galaxy mergers,where,presum-ably caused by the high star-formation rate(Larsen& Richtler1999,2000),the specific frequency of GCs in-creases,Gr99found an unusually small total number of clusters relative to the luminosity of NGC1316(M V∼−22.8,adopting a distance modulus to Fornax ofµ= 31.35,Richtler et al.2000).There is also evidence from stellar population synthesis of integrated spectra that NGC1316hosts younger popu-lations(Kuntschner&Davies1998,Kuntschner2000)and thus the question arises,whether the surprisingly low spe-cific frequency is caused by a high luminosity rather than by a small number of clusters.Goudfrooij et al.(2000, hereafter Go00)obtained spectra of27globular clusters and reported that the3brightest clusters have an age of about3Gyr,indicating a high star-formation activity 3Gyr ago,presumably caused by the merger event.Thesefindings and the hope for a good distance via the GCLF were the main motivation to do the present study of the GCS of NGC1316in a larger area than that of the HST study.As we will show,this galaxy resembles in many aspects the“old”merger galaxy NGC5018,whose GCS has been investigated by Hilker&Kissler-Patig(1996).The paper is organized as follows:in Sect.2we dis-cuss the observations,the reduction procedure and the selection of cluster candidates.The photometric and mor-phological properties of the GCS are discussed in Sect.3. Sect.4contains ourfindings concerning the Specific Frequency.We conclude this work with a general discus-sion in Sect.5.2.Observations and ReductionThe B,V and I images were obtained at the3.6m tele-scope at La Silla during the nights29th and30th of December,1997(dark moon),using the ESO Faint Object Spectrograph and Camera,EFOSC2.Thefield of view was 5.′6×5.′6with a scale of0.′′32/pixel.During thefirst night, short-and long-exposures in eachfilter were centred on the galaxy.In the second night,a backgroundfield located about5′away from the centre of NGC1316was observed, overlapping by1′the observations of thefirst night.In addition,severalfields containing standard stars from the Landolt catalog(Landolt1992)were acquired in eachfil-ter,as well as some short exposures of NGC1316.Fig.1 shows the combined frames from both nights and Table1 summarises the observations.Table1.Summary of the observations.Dec.29,1997B4×6001.′′1Dec.29,1997V5×3001.′′0Dec.29,1997I6×3001.′′0Dec.30,1997B4×6001.′′3Dec.30,1997V3×6001.′′3Dec.30,1997I3×6001.′′2M.G´o mez et al.:The Globular Cluster System of NGC1316(Fornax A)3 Table2.General parameters of the target galaxy,from de Vaucouleurs et al.(1991)and Poulain(1988).NGC131603h22m41.s6−37◦06′10′′240.◦16−56.◦69(R’)SAB(s)08.53±0.080.861793±12filter A j1A j2rms of thefitture radii between22.′′9and86.′′6are: ∆V =0.013,∆B−V =0.007and ∆V−I =0.006mag,well below therms of thefit(see Table3).We then defined5local stan-dard stars in thefield of NGC1316to set the photometryof both nights in a consistent way.2.3.Selection criteriaSeveral criteria have been applied to select cluster can-didates,according to colours,magnitude,photometric er-rors,stellarity index and projected position around thegalaxy.We assume that the clusters are similar to theMilky Way clusters.Adopting an absolute turnover mag-nitude(TOM)of V=−7.60for the galactic clusters(Drenkhahn&Richtler1999,Ferrarese et al.2000)andµ=31.35(Richtler et al.2000),the TOM of NGC1316isexpected to be V∼23.7mag,and the brightest clustersabout V∼20.Although no reddening corrections are normally ap-plied when looking towards Fornax(Burstein&Heiles1982),we cannot restrict the colours of the clusters inNGC1316to match exactly the galactic ones.One pointis the smaller sample of galactic clusters.Besides,we mustallow for significant photometric errors of faint cluster can-didates.Fig.2shows a colour-magnitude diagram for allobjects detected simultaneously in B,V and I in bothnights(before the selection),together with the cut-offval-ues adopted as criterium for this colour.As can be seen,the majority of objects have colours around V−I=1.0.Objects bluer than0.5mag are very probably foregroundstars.A fraction of the data points redder than V−I=1.6are background galaxies.We also tested in our images the robustness of the“stellarity index”computed by SExtractor.This indexranges from0.0(galaxy)to1.0(star)and varies for thesame object by about0.2when classifying under differentseeing conditions,except for the brightest objects,whichare clearly classified.By visual inspection of the images,we are quite confident that bright galaxies are always givenindices near to zero.However,this classification becomesprogressively more difficult with fainter sources.4M.G´o mez et al.:The Globular Cluster System of NGC 1316(Fornax A)−0.50.51 1.522.5V−I161820222426V Fig.2.A colour-magnitude diagram for all objects de-tected in B ,V and I in both nights before the selection criteria (911points).The dashedlines indicates the selection criterium in the V −I colour (see Sect.2.3.)V0.00.20.40.60.81.0s t e l l a r i t y i n d e xFig.3.The stellarity index computed by SExtractor as function of the V magnitude.The dashed lined indicates the cut value adopted in the selection criteria to reject background galaxies.Fig.3shows the “stellarity index”for the 911objects in our sample (before the selection criteria)as a function of the magnitude.Two groups of objects having indices of ∼0.0and ∼1.0can be seen,but it is apparent that faint clusters cannot be unambiguously distinguished from background galaxies due to the uncertainty of the stellar-ity index in the case of faint sources.Very similar results were obtained with our “artificial stars”(see Sect.2.5),where indices down to 0.2were measured for faint objects that are constructed using the PSF model and,therefore,are expected to have a stellarity index of ∼1.0.Guided by our experience with artificial stars,we de-fined the cut-offvalue for the stellarity index to be 0.35.Remaining galaxies will be statistically subtracted be-cause the same criteria are applied to the background field (see Sect.2.4).Finally,we rejected objects with photometric errorlarger than 0.15mag.To summarise,the following criteria were applied to our sample of 911sources detected in B ,V and I :i)V >19.5ii)0.4<B −V <1.4iii)0.3<V −I <1.8iv)stellarity index >0.35v)error(V ),error(B −V ),error(V −I )<0.15375objects met this set of criteria and are our globular cluster candidates.2.4.Background correctionAfter the selection,there might still be some contamina-tion by foreground stars and background galaxies in our sample.To subtract them statistically,one needs to ob-serve a nearby field,where no clusters are expected,and to apply the same detection and selection criteria as with the galaxy frame.However,the analysis of our background field still shows a concentration towards the galaxy centre,which means that only part of the field can be considered as background.We used the radial profile of the GC surface densi-ties (see Sect.3.3)to select all objects on the flat part of the profile,i.e.,where the number of globular cluster can-didates per area unit exhibits no gradient.Fig.11(top)shows that this occurs at r ≈300′′,where r is the distance from the optical centre.Thus,all objects with galactocen-tric distances larger than 300′′and matching the above criteria,constitute our background sample.We constructed a semi-empirical luminosity function of the background clusters with a technique described by Secker &Harris (1993),where a Gaussian is set over each data point,centred at the corresponding observed magni-tude.The sum of all Gaussians gives a good representation of the background,without introducing an artificial undu-lation or loss of information due to the binning process.Fig.4shows the histogram of the background objects,us-ing a bin size of 0.4mag,and the adopted semi-empirical function,which we use as background in the calculation of the luminosity function (see Sect.3.4)and the specific frequency.Admittedly,the numbers are small.The dip at V =23may be simply a result of bad statistics.On the other hand,as Table 6shows,the background counts are small compared to the clusters counts.Therefore,errors of the order of the statistically expected uncertainty do not significantly influence our results and are accounted for in the uncertainties of the total counts in Table 6.pleteness correctionTo correct statistically for completeness,one needs to de-termine which fraction of objects are actually detected by the photometry routines.These ‘artificial stars ex-periments’were performed using the task addstar inM.G´o mez et al.:The Globular Cluster System of NGC 1316(Fornax A)52021222324V05101520N Fig.4.The semi-empirical luminosity function for the background field (dashed line).A histogram with a bin size of 0.4mag is over-plotted for comparison.DAOPHOT.In one step,100stars were added in the sci-ence frames from V =20to V =25in steps of 0.1mag,distributed randomly to preserve the aspect of the image and not to introduce crowding as an additional param-eter,but with the same coordinates in the B ,V and I frames every loop.The colours of the stars were forced to be constant B −V =0.7and V −I =1.0,close to the mean colours of the globular clusters in NGC 1316(see Sect.3.1).The object detection,photometry,classi-fication and selection criteria were applied in exactly the same way as for the globular cluster candidates.To get sufficiently good statistics,we repeated the whole proce-dure 10times,using different random positions in each of them and averaging the results.In all,50000stars were added.However,the completeness is not only a function of the magnitude.Due to the remaining noise after galaxy subtraction,the probability of detecting an object near the centre of the galaxy is smaller than in the outer parts.We divided our sample of artificial stars into elliptical rings,in the same way as we did in deriving the radial profile (see Sect.3.3).The results of the completeness tests are summarised in Fig.5.It can be seen that the 50%limit goes deeper with increasing galactocentric distance.3.Photometric and morphological properties 3.1.Colour distributionIn this section we discuss the colours of our GC candidates.No interstellar reddening is assumed (Burstein &Heiles 1982)and therefore,only internal reddening might affect the colours.The histograms in Fig.6show the colour distribution of the cluster candidates.A fit of a Gaussian function with free dispersions returned the values listed in Table 4and is overplotted for comparison.As can be seen,the dispersion in the B −V and V −I histograms is completely explained by the photometric er-rors alone.This is not the case for the B −I histogram,due probably to the greater metallicity sensitivity of thisV0.00.20.40.60.81.0c o m p l e t e n e s spleteness factors in four elliptical annuli.The probability of detection strongly decreases near the centre of NGC 1316.Table 4.Fit of a Gaussian function to the colour his-tograms of the GC candidates.B −V 0.80±0.020.13±0.04V −I 0.95±0.010.17±0.02B −I 1.77±0.020.35±0.036M.G´o mez et al.:The Globular Cluster System of NGC 1316(Fornax A)0.00.51.0 1.52.0B−V0204060N0.00.51.0 1.52.0V−I0204060N0.81.31.82.3 2.8B−I010203040N Fig.6.Colour histograms of the cluster candidates.The bin size is 0.05mag in each colour.A Gaussian function has been fit to the histograms (dashed line,see text).The dot-dashed line is a Gaussian with σ=0.15.The long-dashed line at B −I =1.75(lower panel)was set to divide the sample in red and blue clusters.the clusters are on average ∼0.2mag bluer than the un-derlying galaxy light.This is a property commonly found also in normal early-type galaxies,where the blue and presumably metal-poor clusters indicate the existence of−3.0−2.0−1.00.0 1.0[Fe/H]1020304050NFig.7.Metallicity histogram of the GCS of NGC 1316,from its B −I broadband colour.The galactic system (dashed line)is overplotted for comparison.The long-dashed line near [Fe /H]=−1.0divides the sample in metal-rich and metal-poor clusters,using the calibration from Couture et al (1990)and B −I =1.75(see text and Fig.6.)a faint metal-poor stellar (halo?)population,as the case of NGC 1380suggests (Kissler-Patig et.al 1997).However,the non-existence of a colour gradient is consistent with the finding that blue and red clusters have similar surface density profiles (Sect.3.3).Differential reddening caused by the irregular dust structure might affect the width of the colour distribution,but apparently does not produce any colour gradient.3.2.Angular distributionFor all objects in our sample of clusters,a transformation from cartesian (x,y )coordinates to polar (r,θ)was done,with origin in the optical centre of NGC 1316.An offset of 50◦in θwas applied to match the PA of the galaxy quoted by RC3.In this way,θ=0represents the direction of the semi-major axis (sma)of the galaxy,a ,and θ=90◦the direction of b ,the semi-minor axis.To analyse the angular distribution,we rejected ob-jects inside a radius of 150pixels (corresponding to 4.3kpc with µ=31.35),where the completeness is significantly lower (see Fig.5)and some clusters appear over ripples and dust structures.Objects outside of 450pixels were also rejected,as one needs equally-sized sectors to do this analysis,and r =450is roughly the radius of the largest circle fully covered by our frame (see dotted line in Fig.10).Only candidates brighter than V =23.8(the 50%completeness level in the entire frame)were considered.The data were then binned in θ.Several bin sizes from 18◦to 30◦were tested,and Fig.9shows the histogram for a bin size of 22.◦5,which corresponds to dividing the sample into 16sectors.The bins were taken modulo πfor a better statistics,that is,we assume that the distribution of clusters is symmetric along the semi-mayor axis of the galaxy.Due to the substructures present in NGC 1316,andM.G´o mez et al.:The Globular Cluster System of NGC 1316(Fornax A)71.21.4 1.61.82.0 2.2 2.4log r (arcsec)1.02.03.0B −I1.02.03.0B −IFig.8.The B −I colour gradient along the projected galactic radius.Top:the cluster candidates and a least-square fit(solid line).The crosses indicate the B −I colour of the galaxy.Bottom:the mean colour in several rings,with the error bars indicating the σof the mean at that ring.090180270360θ [deg]10203040N Fig.9.The angular distribution of globular clusters for a radius from 150to 450pixels (48′′to 144′′)down to V =23.8.The bin size is 22.◦5and the data were taken mod π.The histogram from 0◦to 180◦is repeated from 180◦to 360◦for a better visualisation.The dashed line indicates the best fit of a double-cosine function.from the fact that it is a merger galaxy,one could expect some systematic differences in the azimuthal distribution of the clusters between both halves.Although this is not observed at any bin size,the small number of counts does not allow us to address this question clearly.The histogram shown in Fig.9demonstrates the strong correlation of the globular clusters with the galaxy light.By fitting isophotes to the galaxy,we obtained ellipticities ranging from 0.27to 0.32and position angles from 49◦to53◦,between semi-major axes of 150to 450pixels (thesame used with the clusters).From the least-square fit to this histogram (with fixed period π),we derived PA =63◦±9◦and an ellipticity of 0.38±0.06.There are,however,some problems that cannot be eas-ily resolved with our ground-based data.As indicated,the detection of cluster candidates near the centre of the galaxy is quite poor.This is,unfortunately,the most in-teresting region to search for young clusters which might be related to a merger event.3.3.Radial profileTo derive the radial surface density of GCs,we divide our sample into elliptical annuli,as shown in Fig.10.The annuli have a width of 100pixels along the major axis (a )and start from a =50pixels.Due to the small number of objects in the periphery of NGC 1316,rings beyond a =900were given a width of 300pixels.The ellipticity,position angle and centre of these rings were taken from the fit of the galaxy light (see Sect.3.2)and fixed for all annuli.In particular,the ellipticity e ,defined as 1−(b/a ),where a and b are the semi-major and semi-minor axis respectively,was set to 0.3and the PA to 50◦.We then counted the number of globular cluster can-didates per unit area in each ring,down to V =23.8,and corrected them with the corresponding completeness function.The results are presented in Table 5.The first column lists the mean semi-major axis of the ring.Column 2gives the raw number of clusters down to V =23.8,without correction for completeness.Column 3lists the corrected data with their errors.Column 4lists the visible area of the rings,in ⊓⊔′.Column 5gives the number of candidates per unit area.Column 6,7and 8are used to compute the Specific Frequency (see Sect.4).Fig.11shows the radial profile of the clusters’surface density,before and after the subtraction of the background counts.A fit of a power-law ρ(r )=A ·r α,where ρis the surface density and r the projected distance along the semi-major axis,gives αgcs =−2.04±0.20and αgal =−2.03±0.02for the clusters and the galaxy light,respectively.We are well aware that a King profile may be more adequate than a power function,but our purpose is to compare our result with previous work in other GCSs in Fornax,which quote only power functions.The similarity between both slopes indicates that the GCS of NGC 1316is not more extended than the galaxy light.Moreover,αGCS =−2.04is in good agreement with other GCS of “normal”early-type galaxies in Fornax (Kissler-Patig et al.1997).We divided the system at B −I =1.75mag into blue (presumably metal-poor)and red (metal-rich)clusters,and searched for systematic differences in the morpholog-ical properties between both subgroups.Again,only clus-8M.G´o mez et al.:The Globular Cluster System of NGC1316(Fornax A)Table5.This table gives the result of the radial profile of the cluster candidate surface density.Thefirst column lists the centre of each annulus(in pixels).Then follows the raw number counts down to V=23.8,before and after the correction for completeness.The fourth column gives the visible area of the annulus in⊓⊔′.Column5lists the mean density of GC per⊓⊔′.Columns6and7give the number of clusters down to the TOM in V,before and after the correction for completeness.The applied geometrical corrections are listed in column8.Finally,the number of the clusters in each annulus,after doubling the counts around the TOM.Note that the corrected and total number of clusters for the innermost annulus(sma=100)are NOT derived using the completeness correction.Instead,they were estimated by extrapolating the radial profile towards the centre(see text in Sect.4).1002058.6±18.1 1.25146.86±14.4720200.2±37.5 1.400±7520053100.3±17.9 2.50240.09±7.1553100.7±18.0 1.201±3630068104.4±15.2 3.75127.84±4.0569109.4±15.8 1.219±324005465.4±10.7 5.00613.06±2.135668.9±11.0 1.134±225004858.2±10.0 6.2349.34±1.605162.0±10.40.997124±216003742.9±8.5 6.1596.96±1.223744.5±8.80.821108±217001922.4±6.2 5.1454.35±1.211922.8±6.30.58878±219001923.2±6.49.4402.45±0.68————12001112.7±4.67.1591.77±0.64————150045.3±3.2 2.8801.84±1.11————M.G´o mez et al.:The Globular Cluster System of NGC 1316(Fornax A)9Fig.10.The elliptical annuli used in the calculation of the globular cluster density and specific frequency.The open circles indicate the position of all GC candidates brighter than V =23.8.Objects outside the annulus defined by r =150and r =450pixels (dotted line)were rejected for the analysis of the angular distribution (see Sect.3.2).Objects outside the dashed ellipse are considered as background.The scale and orientation is the same as in the Fig.1.There are two additional columns,namely the number of blue and red clusters.They were calculated in the same way and will be discussed in Sect.5.Bin sizes of 0.3,0.4and 0.5mag were tested,and the results were always in good agreement with each other.Finally,we have chosen 0.5mag as our bin size from the appearance of the fit histogram,and the absence of undu-lations which are present for the other cases.We note,however,that our derived TOM is close to the limit of the observations,and the last bins are strongly affected by the completeness correction.Nevertheless,the similarity of the results using different bin sizes and cen-ters,and the robustness of the fit against skipping the last bin,is encouraging.100110100G C /a r c m i n2100r (arcsec)110100G C /a r c m i n2Fig.11.Top:the radial profile of the density of glob-ular cluster candidates.The dashed line indicates thevalue adopted for the background,corresponding to 2.0objects /⊓⊔′.Bottom:the radial profile after subtrac-tion of the background counts.The crosses represent the profile of the galaxy light,arbitrarily shifted.1.61.82.02.2 2.4log r (arcsec)−0.50.00.51.01.52.0l o g (G C /a r c m i n2)Fig.12.The radial profile of the GC surface density for the red (triangles)and blue population (squares).No sys-tematic difference is seen and both are equally concen-trated.Fig.14shows the three luminosity functions in B ,V ,I .For fitting the LF we chose t 5functions,which are of the form:t 5(m )=85πσt1+(m −m 0)210M.G´o mez et al.:The Globular Cluster System of NGC1316(Fornax A)Table6.The counts(in bins of0.5mag)used in the determination of the GCLF.Given are the V-magnitudes(or B,I,respectively)of the bin centers.Then follow the raw counts of the four elliptical annuli N i(see text)together with the corresponding completeness factors f i.The background counts are then listed as defined in Sect.2.4.The completeness factors of the fourth annulus have been used for the background as well.The total number of clusters is the sum of the four annuli minus the background,normalised to the same area(see text).Also given is the number of clusters for the blue and the red population separately,where the separating colour was B−I=1.75.19.500.7110.981 1.002 1.000.04.0±2.01.0±1.03.0±1.720.010.7110.983 1.001 1.000.06.4±2.72.4±1.74.0±2.020.510.6700.984 1.002 1.000.26.9±3.13.9±2.63.0±1.721.020.6260.983 1.003 1.00 1.012.6±5.01.4±3.311.2±3.821.520.5830.94120.9970.99 2.518.8±7.07.8±4.611.3±5.222.020.5650.84140.97110.98 4.622.2±8.814.4±6.98.0±5.422.570.40110.68320.95140.95 4.269.8±12.536.6±9.033.3±8.623.030.35180.54390.93140.90 1.794.2±12.944.3±8.749.9±9.523.520.1480.28520.71190.79 3.7127.2±19.965.5±15.661.7±12.124.000.0720.20320.2280.25 3.8145.1±38.848.8±24.896.5±28.124.500.0000.0040.0310.030.5———B N B1f1N B2f2N B3f3N B4f4N B bkg.N B total N B blue N B red18.500.7110.980 1.002 1.000.03.0±1.70.0±0.03.0±1.719.000.7110.984 1.001 1.000.06.0±2.51.0±1.05.0±2.219.530.6710.983 1.000 1.000.08.5±3.34.0±2.34.5±2.320.000.6350.983 1.005 1.000.511.7±4.13.2±2.58.5±3.320.540.5840.94110.9950.99 2.819.8±7.49.5±4.910.3±5.521.020.5660.84160.97100.98 3.826.7±8.59.3±5.417.4±6.621.540.4090.68350.95170.95 5.163.1±12.133.0±9.030.1±8.022.050.35180.54370.93120.90 1.994.9±13.539.0±9.255.9±9.822.510.1490.28460.71150.79 2.3115.0±17.962.0±13.953.4±10.923.010.0700.20380.22150.25 4.3199.4±45.4130.6±36.468.8±24.623.500.0210.0030.0300.03 1.4———。
Stellar Populations and Variable Stars in the Core of the Globular Cluster M5

a rXiv:as tr o-ph/9711152v113Nov1997STELLAR POPULATIONS AND V ARIABLE STARS IN THE CORE OF THE GLOBULAR CLUSTER M51Laurent Drissen D´e partement de Physique and Observatoire du Mont M´e gantic,Universit´e Laval,Qu´e bec,QC,G1K 7P4Electronic mail:ldrissen@phy.ulaval.ca and Michael M.Shara Space Telescope Science Institute,3700San Martin Drive,Baltimore,Maryland 21218Electronic mail:mshara@ ABSTRACT We report the discovery of a variable blue straggler in the core of the globular cluster M5,based on a 12-hour long series of images obtained with the Planetary Camera aboard the Hubble Space Telescope .In addition,we present the light curves of 28previously unknown or poorly studied large-amplitude variable stars (all but one are RR Lyrae)in the cluster core.A (V,U-I)color-magnitude diagram shows 24blue stragglers within 2core radii of the cluster center.The blue straggler population is significantly more centrally concentrated than the horizontal branch and red giant stars.1.IntroductionGlobular cluster cores are being imaged intensively with the Hubble Space Telescope, and continue to yield surprises.The realizations that blue stragglers are commonplace,red giants are depleted,and that horizontal branch populations may depend on core properties has spurred on observers in the pastfive years.As part of our program to study the dis-tributions of blue stragglers and variable stars in globular cores,we have now imaged NGC 5904=M5.The globular cluster M5harbors one of the richest collections of RR Lyrae stars in the Galaxy.It is also home to one of only two known dwarf novae in Galactic globular clusters. Until now,however,not a single blue straggler candidate has been identified in M5.Is this because none exist,or because blue stragglers reside only in the(until now unobservable) core of M5?In section2we describe the observations and reductions;the CMD of the core of M5is presented in section3.The variable stars wefind are described in section4while our results are summarized in section5.2.Observations and reductions2.1.Search for variable starsA series of twenty-two600-second exposures of M5’s central(r≤50′′)region,spanning11.5hours,was obtained on March21,1993with the Planetary Camera(PC)aboard the Hubble Space Telescope(HST)before the repair mission.The F336Wfilter,similar to the Johnson U bandpass(Harris et al.al.1991),was used for the observations.Standard cali-bration(bias and dark subtraction,flatfield correction)was performed with STScI software within IRAF/STSDAS.The detection of variable stars was performed as follows.First,the22individual images were combined with IRAF’s combine task(with the crreject option)to create a deep image. This image is shown in Figure1.The individual images were remarkably well aligned with each other,so no shift was necessary.DAOPHOT’s Daofind algorithm(Stetson1987)was then used to build a masterlist of3144stars visible in the deep image.In order to eliminate the numerous cosmic rays,which are very difficult to remove on single frames,the22individual images were combined two by two(again with the combine task and the crreject option),with an overlap of1(image1+image2,image2+image3,...).Aperture photometry with an aperture radius of4pixels(0.17′′),and a sky annulus with inner and outer radii of10and15pixels was then performed on the21resulting images, using the masterlist of stars previously found.The standard deviation from the mean in the 21frames was then computed for all stars;this resulted in the discovery(or re-discovery)of 29variable stars,which will be discussed in section4.Note that since for any given star the conditions(PSF,flatfield,star location,aperture, background)are virtually identical from frame to frame(with the exception of faint stars near bright,large amplitude variables),the relative photometry from frame to frame is expected to be much more precise than the relative photometry among stars within the same frame (mostly because of PSF variations which induce position-dependent aperture corrections and overlapping PSFs in a crowdedfield).While the relative error from one star to the next within a single frame can be as large as0.2mag(if simple aperture photometry is used), even for bright stars,the typical standard deviation from the mean of the21frames for a given non-variable star is less than0.05mag at U=17.5,and reaches0.1mag at the cluster main sequence turnoff.2.2.The color-magnitude diagramIn order to study the stellar populations in M5,a70second F555W(equivalent of Johnson Vfilter;Harris et al.1991)and a70second F785LP(comparable to I)PC images were retrieved from the HST archives.Both images were obtained on December27,1991. Fortunately,the center of these frames is only∼8′′away from the center of our images (the totalfield of view of the4PC CCDs is70′′),so most stars detected in our F336W images are also located in the archive frames;the area common to all three sets of images is∼1.1arcmin2.Stellar photometry was performed in the most simple way on these frames:aperture photometry(with the same parameters as above)was performed on the deep,combined F336W frame using the masterlist.After proper shift and rotation of the coordinates,the same masterlist was used to obtain aperture photometry of the stars in the F555W and F785LP images.Although PSF-fitting techniques are known to improve the photometric accuracy(especially at the faint end of the luminosity function and near bright stars;see Guhathakurta et al.1992for a detailed discussion),simple core aperture photometry gives results which are reliable enough(typicallyσ∼0.2mag above the turnoff) for our purpose.A total of2153stars have been included in the color-magnitude diagram discussed below.Aperture corrections and photometric zero-points determined by Hunter et al.(1992) were used to calibrate the magnitudes and colors of the stars.Moreover,the absolute sen-sitivity of the CCDs is known to decrease slowly with time following each decontamination (Ritchie&MacKenty1993);this effect was taken into account.As a consistency check,the photometric calibration was compared with ground-based data.The average F555W and F336W magnitudes of the RR Lyrae stars are F555W RR= 15.2and F336W RR=15.55,while Buonanno et al(1981)find V RR=15.13and Richer& Fahlman(1987)obtained U HB=15.6.The agreement is excellent.3.Stellar PopulationsThe color-magnitude diagram for the2153stars located in the area common to the F336W(U),F555W(V)and F785LP(I)frames is shown in Figure2.In this plot,variable stars(see next section)are identified with special symbols;seven of29variables found in the F336W frames are located outside the F555W and F785LPfield of view,and are therefore not included in Figure2.Photometry is fairly complete down to∼0.5magnitude below the turnoff.The large wavelength difference between the U and Ifilters compensates for the relatively poor photometric accuracy,and allows us to clearly define the stellar populations. Two characteristics of the CMD are worth noting:the significant blue straggler population and the morphology of the horizontal branch.3.1.Blue StragglersThe boundaries of the BS region of the CMD have often been arbitrarily defined,vary from author to author,and also depend on thefilters used.As emphasized by Guhathakurta et al.(1994),the(V,U-I)CMD is well suited to define the blue straggler population.As a working definition,we decided to include in the BS region of the(V,U-I)CMD all stars significantly brighter(0.4mag)than the turnoffand bluer than the average main sequence at the turnofflevel;twenty-four stars are included in this region of the CMD(surrounded by dotted lines in Figure2)and can be considered as blue stragglers.Such a significant blue straggler population in the core of M5is in striking contrast to the outer regions of the cluster, where none have been found(Buonanno et al.1981,for2′≤r obs≤5.6′;Richer&Fahlman 1987,for3′≤r obs≤25′).Recent CCD observations of the central regions of M5(Brocato et al.(1995);Sandquist et al.(1996))also reveal some blue stragglers.The two-dimensional distribution of red giants,horizontal branch stars and blue stragglers is shown in Figures 3a-c.It is obvious from these plots that the BS population is preferentially concentrated in the very core of the cluster.This is quantified in Figure4a,which shows the cumulativedistribution of the different stellar populations within the inner50′′,and in Figure4b,within one core radius(24′′).A simple K-S test showed that there is a99.5%probability that the blue stragglers in the core of M5are more centrally concentrated than the horizontal branch stars,and a97.5%probability that they are more centrally concentrated than the red giants.This tendency for blue stragglers to be more centrally concentrated than other cluster members has been noted for most globular clusters observed to date(see Sarajedini(1993), Yanny et al.1994and references therein),and is consistent with the hypothesis that BS are more massive than main sequence stars.The luminosity-inferred lifetime of the shortest-lived,most luminous blue straggler in the core of M5is∼5×108yrs,assuming it lies on the hydrogen-burning main sequence. This corresponds to the star with V=16.0,L∼25L⊙and M∼2.15M⊙.The relaxation time in the core of M5is T rc=2×108yrs(Djorgovski1993).Hence,all BS in the core of M5are expected to be dynamically relaxed.Thus,the radial distribution of the BS is, indeed,a direct probe of their masses.In order to compare the blue straggler population from cluster to cluster,Bolte,Hesser &Stetson(1993)have defined the specific frequency,F BS,as the ratio of the number of blue stragglers to the total number of stars brighter than2magnitudes below the horizontal branch at the instability strip.In the case of the central region of M5(area in common to the U,V and I HST/PC frames),where V HB=15.2,24F BS=other clusters.He suggests that gaps may provide important constraints on mixing in earlier stages of stellar evolution.We see no evidence that the gap width changes between the inner and outer parts of the core.4.Variable StarsFigure5shows the standard deviation as a function of the average U magnitude for all the stars in the F336W frames.The usual problem with outliers in Figure5(due to mismatches in star lists)was almost completely avoided because(a)the same master list for all frames(see section2.1),and(b)all images were perfectly aligned with each other.Visual inspection of the few remaining outliers immediately showed them to be mismatches and they were eliminated from our photometry.Stars with RMS magnitude of variability higher than2sigma above the mean curve were considered possible variables and were examined more closely.Most of them were faint stars located close to bright,large-amplitude variables. Twenty-eight stars stand out as bright,large-amplitude variables in Figure5,as well as one fainter,low-amplitude variable star.Seven of these variable stars had never been identified previously,and two(HST-V20and V21)were considered as a single star.All but one of the large-amplitude variables have light curves typical of RR Lyrae stars,whereas the low-amplitude variable is a blue rmation on the variables,including cross-identification with previous papers,is presented in Table1.We note that the cataclysmic variable V101is36′′North and282′′West of the center of M5and is therefore too distant to have been included in thefield of view of the Planetary Camera(70′′×70′′).4.1.A Variable Blue StragglerOf24blue stragglers identified in Figure2,only one(HST-V28)shows evidence for variability above the noise level.Although only HST-V28showed up above the2-sigma variability level in Figure5,we have examined the light curve of every blue straggler and compared them with those of nearby stars of similar magnitude in order tofind possible variations undetected by our technique;no new variable showed up.But because the noise level is still relatively high between V=17and V=18(the2σvariability level varies from0.06 to0.12,corresponding to amplitudes∼0.2-0.3mag),we cannot exclude the possibility that some blue stragglers are actually small amplitude variables.The color-magnitude diagram(Figure2)shows that HST-V28is the bluest,and one of the brightest blue stragglers in M5(with M V=+2.2).Figure6shows its light curve,along with light curves of3nearby comparison stars of the same magnitude.HST-V28is obviously variable,with an amplitude∆U≥0.15mag.Unfortunately,our data do not allow us to determine the period with any precision(or even to determine if the variations are periodic).Phase dispersion minimization algorithms favor a periodicity of the order of ∼15hours(in which case this star could be an eclipsing binary),but cannot completely exclude periods shorter than∼1.9hours(typical for pulsating blue stragglers in clusters; Mateo1993).Better photometric accuracy and temporal sampling are obviously necessary to determine the period and nature of HST-V28.Guhathakurta et al.(1994)recently discovered2variables among the28blue stragglers in the core of M3.Both have photometric amplitudes∼0.4mag and periods∼6-12hours. Guhathakurta et al.suggest that both stars are dwarf cepheids.4.2.RR Lyrae StarsAmong the97genuine variables listed in Sawyer-Hogg’s(1973)catalogue,93are RR Lyrae.Recently,Kalda et al.(1987)and Kravtsov(1988,1992)searched for new variables in the central,crowded region of the cluster and found30more.So far,the light curves of only two of these new variables have been published(Kravtsov1992).A recent review of the M5RR Lyrae population has been published by Reid(1996).The U-band light curves of all RR Lyrae stars found in the HST images are shown in Figure7.Tentative periods have been determined for the9type RRc stars with a phase dispersion minimization algorithm,and the results are presented in Table1.The periods of RRab stars are too long to be determined from our observations alone.4.3.HST-V1Eclipsing binary stars are common in the periphery of M5’s core(Reid1996,Yan&Reid 1996),but star HST-V1is unique among the variables found in the core of M5.Although our observations do not cover an entire cycle(P∼0.55d−0.65d),the light curve suggests that HST-V1is a contact(or semi-detached)binary.The secondary minimum is very asymmetric, somewhat reminiscent of the near-contact binary V361Lyrae(Kaluzny1990).The strong asymmetry of the light curve of V361Lyr,more pronounced at shorter wavelengths,is thought to be caused by the presence of a hot spot on the secondary star resulting from the high mass transfer rate from the primary star.Unfortunately,HST-V1lies outside the boundaries of the F555W and F785LP frames, so its color cannot be determined.But with an average magnitude of U=15.7,HST-V1is more than two magnitudes brighter than the turnoff;if it is a cluster member,at least one of its components must be a giant.5.SummaryThe main results of our paper can be summarized as follows:(1)The core of M5contains a highly centrally concentrated population of blue stragglers, similar in size to that found in47Tuc(Paresce et al.1991).(2)Of24blue stragglers detected in the HST images,only one shows significant vari-ability above the noise level.Since our data are not sensitive enough to detect variables of amplitude≤0.2mag in the V=17-18range,we cannot exclude the possibility that some blue stragglers may be variable below that level.A more sensitive search with the refurbished HST is highly desirable.(3)We confirm the existence of a0.2mag gap in the color-magnitude distribution of the horizontal branch,first noted by Brocato et al..The nature of this gap is still unknown.(4)We have presentedfinding charts and light curves for27RR Lyrae variables and one probable contact binary.Among those variables,seven were previously unknown and21 had no published light curve.Support for this work was provided by NASA through grant number GO-3872from the Space Telescope Science Institute,which is operated by the Association of Universities for Research in Astronomy,Inc.,under NASA contract NAS5-26555.REFERENCESBolte,M.,Hesser,J.E.,&Stetson,P.B.1993,ApJ,408,L89Brocato,E.,Castellani,V.,&Ripepi,V.1995,AJ,109,1670Buonanno,R.,Corsi,C.E.,&Fusi Pecci,F.1981,MNRAS,196,435Djorgovski,S.G.1993,in Structure and Dynamics of Globular Clusters,ASP Conf.Ser., Vol.50,S.G.Djorgovski and G.Meylan,eds.,p.373Guhathakurta,P.,Yanny,B.,Schneider,D.P.,&Bahcall,J.N.1992,AJ,104,1790Guhathakurta,P.,Yanny,B.,Bahcall,J.N.,&Schneider,D.P.1994,AJ,108,1786 Harris,H.C.,Baum,W.A.,Hunter,D.A.,&Kreidl,T.J.1991,AJ,101,677Hesser,J.E.1988,IAU Symposium No.126,eds.J.E.Grindlay and A.G.Davis Philip,p. 65Hunter,D.A.,Faber,S.M.,Light,R.,&Shaya,E.1992,in Wide Field/Planetary camera Final Orbital/Science verification Report,ed.S.Faber(STScI,Baltimore),chap.12 Kaluzny,J.,1990,AJ,99,1207Kaluzny,J.,&Shara,M.M.1987,ApJ,314,585Kravtsov,V.V.,1988,Astron.Tsirk.,1526,6Kravtsov,V.V.,1992a,Sov.Astron.Lett.18(4),246Kravtsov,V.V.,1992b,Sov.Astron.Lett.17(6),455Kadla,Z.I.,Gerashchenko,A.N.,Jablokova,N.V.,&Irkaev,B.N.,Astron.Tsirk.,1502, 7Mateo,M.1993,in Blue Stragglers,ed.R.A.Saffer(ASP Conf.Ser.53),p.74 Paresce,F.,Meylan,G.,Shara,M.M.,Baxter,D.,&Greenfield,P.1991,Nature,352,297 Reid,N.1996,MNRAS,278,367Richer,H.B.,&Fahlman,G.G.1987,ApJ,316,189Sarajedini,A.1993,in Blue Stragglers,ASP Conf.Ser.,vol.53,ed.R.E.Saffer,p.14 Sawyer-Hogg,H.,1973,D.D.O.Pub.,Vol3.,No.6Yan,L.&Reid,I.N.1996,MNRAS,279,751Yanny,B.,Guhathakurta,P.,Schneider,D.P.,&Bahcall,J.N1994,ApJ,435,L59Fig.1.—Full HST/PCfield of view(70′′×70′′)of the central region of M5.This image is the average of twenty-two F336W(U-band)exposures.Variable stars and the cluster center are identified.-2-1012342019181716151413U-I Fig.2.—Color-magnitude diagram for the stars common to the U (F336W),V(F555W)and I(F785LP)frames.RR Lyrae stars,and the variable blue straggler are shown with special symbols.050010001500050010001500Fig. 3.—Distribution of the red giants,horizontal branch stars and blue stragglers in the V frame coordinate system.The location of the U images is shown.The cluster center is identified by a cross.The circle has a radius of 20′′(∼one core radius).050010001500050010001500Fig.3.—continued050010001500050010001500Fig.3.—continuedFig.4a.—(a)Cumulative distribution of the stellar populations (BS:Blue Stragglers;HB:Horizontal Branch stars;RG:Red Giants)in the inner 50′′region of M5;(b)same as (a),but within one core radiusFig.5.—The RMS magnitude of variability as a function of the F336W magnitudes for the stars in the21frame set.The line is the2σcutoff,above which the stars were considered as candidate variables and examined more closely.Genuine variables are shown with specialsymbols.Fig.6.—Light curve of HST-V28,the variable blue straggler and of three nearby(∆d≤5′′)comparison stars of similar mean magnitudeFig.7.—Light curves(filter F336W∼U-band)of the large amplitude variable stars found in the core of M5.All but one(HST-V1)are RR Lyrae stars.Fig.7.—ContinuedFig.7.—ContinuedFig.7.—Continued。
全天星图
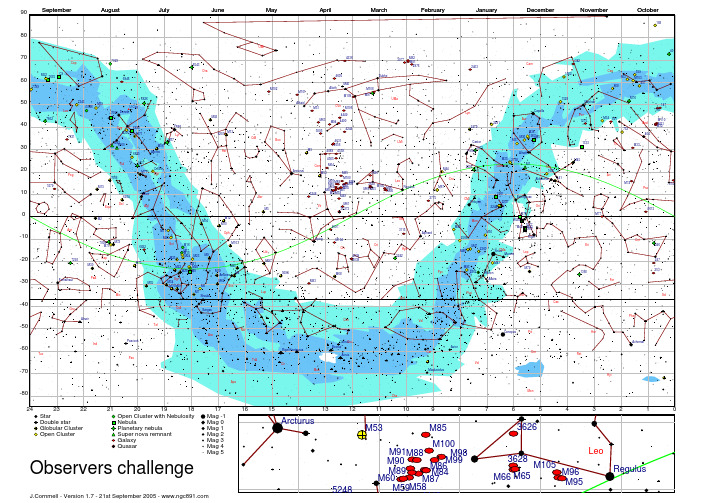
Observers challengeJ.Cornmell - Version 1.7 - 21st September 2005 - Name Size Dec RA Mag Type Cons ID’s Month Best SeenName Size Dec RA Mag Type Cons ID’s Month Best Seen 2+16d 08m 00h 03m 10.5Gx Peg C43/NGC7814Oct 1+72d 31m 00h 13m 11Pl Cep C2/NGC40Oct 5+48d 30m 00h 33m 9.3Gx Cas C17/NGC147Oct 5+48d 19m 00h 39m 9.2Gx Cas C18/NGC185Oct Satellite galaxy of Andromeda 8+41d 40m 00h 40m 8Gx And M110/NGC205Oct Andromeda Galaxy 63+41d 16m 00h 42m 3.5Gx And M31/NGC224Oct Satellite galaxy of Andromeda 4+40d 52m 00h 42m 8.2Gx And M32/NGC221Oct 14+85d 19m 00h 43m 8.1OC Cep C1/NGC188Oct 4-11d 52m 00h 46m 8Pl Cet C56/NGC246Oct 7-20d 46m 00h 47m 8.9Gx Cet C62/NGC247Oct Sculptor Galaxy 9-25d 16m 00h 47m 7.1Gx Scl C65/NGC253Oct 8+02d 07m 01h 04m 9.3Gx Cet C51/IC1613Oct 4+35d 43m 01h 09m 10.1Gx And NGC404Oct 13+58d 19m 01h 19m 6.4OC Cas C13/NGC457Oct 4+63d 17m 01h 29m 9.5OC Cas C8/NGC559Oct 6+60d 42m 01h 33m 7.4OC Cas M103/NGC581Oct Triangulum Galaxy/Pinwheel galaxy 28+30d 38m 01h 33m 5.7Gx Tri M33/NGC598Oct 5+15d 46m 01h 36m 9.2Gx Psc M74/NGC628Oct Little Dumbell/Cork/Barbell/Butterfly 5+51d 34m 01h 42m 12Pl Per M76/NGC650Oct 16+61d 15m 01h 46m 7.1OC Cas C10/NGC663Oct 50+37d 40m 01h 57m 5.7OC And C28/NGC752Oct Double Cluster, H Persei 30+57d 08m 02h 19m 4OC Per C14/NGC869Nov 4+42d 21m 02h 22m 10Gx And C23/NGC891Nov 35+42d 46m 02h 42m 5.2OC Per M34/NGC1039Nov 3-00d 01m 02h 42m 8.8Gx Cet M77/NGC1068Nov 1+41d 31m 03h 19m 11.6Gx Per C24/NGC1275Nov 9+31d 25m 03h 29m -Nb Per NGC1333Nov 7-25d 51m 03h 33m -Pl For NGC1360Nov 18+68d 05m 03h 46m 9Gx Cam C5/IC342Nov Pleiades 110+24d 07m 03h 46m 1.6OC Tau M45Nov 8+62d 19m 04h 07m 5.7OC Cam NGC1502Dec 24+51d 13m 04h 15m 6.4OC Per NGC1528Dec Hyades 330+16d 00m 04h 27m 0.5OC Tau C41Dec 45+19d 04m 04h 46m 6.4OC Tau NGC1647Dec Flaming Star Nebula 30+34d 16m 05h 16m -Nb Aur C31/IC405Dec 9-24d 33m 05h 24m 8Gb Lep M79/NGC1904Dec 7+35d 19m 05h 28m 8.2OC Aur NGC1907Dec 21+35d 49m 05h 28m 6.4OC Aur M38/NGC1912Dec Crab Nebula 6+22d 01m 05h 34m 8.4SNR Tau M1/NGC1952Dec 14-05d 54m 05h 35m -Nb Ori NGC1980Dec Great Nebula In Orion 66-05d 27m 05h 35m 4Nb Ori M42/NGC1976Dec 20-04d 52m 05h 35m -Nb Ori NGC1977Dec de Mairan’s Nebula 20-05d 16m 05h 35m 9Nb Ori M43/NGC1982Dec 12+34d 07m 05h 36m 6OC Aur M36/NGC1960Dec Tank track nebula 30-01d 51m 05h 41m -Nb Ori NGC2024Dec 8+00d 03m 05h 46m 8Nb Ori M78/NGC2068Dec 24+32d 32m 05h 52m 5.6OC Aur M37/NGC2099Dec 5+24d 06m 06h 07m 8.6OC Gem NGC2158Jan 28+24d 19m 06h 08m 5.1OC Gem M35/NGC2168Jan Rosette Nebula -+05d 02m 06h 30m -Nb Mon C49/NGC2237Jan 24+04d 52m 06h 32m 4.8OC Mon C50/NGC2244Jan Hubble’s Variable Nebula 2+08d 43m 06h 39m -Nb Mon C46/NGC2261Jan 38-20d 43m 06h 46m 4.5OC CMa M41/NGC2287Jan 16-08d 19m 07h 03m 5.9OC Mon M50/NGC2323Jan 13-15d 37m 07h 17m 7.2OC CMa C58/NGC2360Jan 8-24d 56m 07h 18m 4.1CN CMa C64/NGC2362Jan The Peanut Nebula 1+29d 28m 07h 25m 13Pl Gem NGC2371Jan Eskimo Nebula/Clown Face Nebula 1+20d 55m 07h 29m 10Pl Gem C39/NGC2392Jan 30-14d 30m 07h 36m 4.4OC Pup M47/NGC2422Jan 9+65d 35m 07h 36m 8.4Gx Cam C7/NGC2403Jan 4+38d 52m 07h 38m 10.4Gb Lyn C25/NGC2419Jan 27-14d 49m 07h 41m 6.1OC Pup M46/NGC2437Jan 22-23d 52m 07h 44m 6.2OC Pup M93/NGC2447Jan 7-10d 46m 08h 00m 7.6OC Mon C54/NGC2506Feb 54-05d 47m 08h 13m 5.8OC Hya M48/NGC2548Feb Beehive Cluster/Praesepe 95+19d 58m 08h 40m 3.1OC Cnc M44/NGC2632Feb 30+11d 49m 08h 50m 6.9OC Cnc M67/NGC2682Feb 2+07d 01m 09h 10m 10.3Gx Cnc C48/NGC2775Feb 2+67d 55m 09h 47m 10.2Gx UMa NGC2976Feb Bode’s Galaxy or Bode’s Nebula 10+69d 04m 09h 55m 6.9Gx UMa M81/NGC3031Feb The Cigar Galaxy 4+69d 40m 09h 55m 8.4Gx UMa M82/NGC3034Feb 2+68d 43m 10h 03m 9.9Gx UMa NGC3077Mar Spindle Galaxy 2-07d 43m 10h 05m 9.2Gx Sex C53/NGC3115Mar Ghost Of Jupiter/CBS eye 21-18d 37m 10h 24m 9Pl Hya C59/NGC3242Mar 3+11d 41m 10h 43m 9.7Gx Leo M95/NGC3351Mar 3+11d 49m 10h 46m 9.2Gx Leo M96/NGC3368Mar 5+12d 34m 10h 47m 9.3Gx Leo M105/NGC3379Mar 3+55d 40m 11h 11m 10.1Gx UMa M108/NGC3556Mar Owl Nebula 3+55d 01m 11h 14m 11.2Pl UMa M97/NGC3587Mar 3+13d 04m 11h 18m 9.3Gx Leo M65/NGC3623Mar 1+18d 21m 11h 20m 10.9Gx Leo C40/NGC3626Mar 3+12d 58m 11h 20m 9Gx Leo M66/NGC3627Mar 4+13d 35m 11h 20m 9.5Gx Leo NGC3628Mar 3+53d 22m 11h 57m 9.8Gx UMa M109/NGC3992Mar Antennae or Ring-Tail galaxy 2-18d 52m 12h 01m 10.7Gx Crv C60/NGC4038Apr Antennae or Ring-Tail galaxy 1-18d 52m 12h 01m 13Gx Crv C61/NGC4039Apr 3+14d 54m 12h 13m 10.1Gx Com M98/NGC4192Apr 7+69d 28m 12h 16m 9.7Gx Dra C3/NGC4236Apr 5+37d 49m 12h 17m 10.2Gx CVn C26/NGC4244Apr Pin-Wheel Nebula 5+14d 25m 12h 18m 9.8Gx Com M99/NGC4254Apr 6+47d 17m 12h 19m 8.3Gx CVn M106/NGC4258Apr 6+04d 28m 12h 21m 9.7Gx Vir M61/NGC4303Apr Winnecke 41+58d 04m 12h 22m 9.1D*UMa M40Apr 3+15d 49m 12h 22m 9.4Gx Com M100/NGC4321Apr 3+12d 52m 12h 25m 9.3Gx Vir M84/NGC4374Apr 7+18d 10m 12h 25m 9.2Gx Com M85/NGC4382Apr 4+12d 56m 12h 26m 9.2Gx Vir M86/NGC4406Apr 3+44d 06m 12h 28m 9.4Gx CVn C21/NGC4449Apr 3C273 Brightest Quasar -+02d 06m 12h 29m 12QSO Vir 3C273Apr 5+08d 00m 12h 29m 8.4Gx Vir M49/NGC4472Apr 2+41d 37m 12h 30m 9.8Gx CVn NGC4490Apr Virgo A 7+12d 24m 12h 30m 8.6Gx Vir M87/NGC4486Apr 3+14d 25m 12h 31m 9.5Gx Com M88/NGC4501Apr 2+14d 30m 12h 35m 10.2Gx Com M91/NGC4548Apr 4+12d 33m 12h 35m 9.8Gx Vir M89/NGC4552Apr 4+27d 58m 12h 35m 9.9Gx Com C36/NGC4559Apr Needle Galaxy5+25d 58m 12h 36m 9.6Gx Com C38/NGC4565Apr 4+13d 10m 12h 36m 9.5Gx Vir M90/NGC4569Apr 3+11d 49m 12h 37m 9.8Gx Vir M58/NGC4579Apr 12-26d 45m 12h 39m 8.2Gb Hya M68/NGC4590Apr 2+61d 37m 12h 40m 11Gx UMa NGC4605Apr Sombrero Galaxy3-11d 37m 12h 40m 8.3Gx Vir M104/NGC4594Apr 2+11d 39m 12h 41m 9.8Gx Vir M59/NGC4621Apr 5+32d 31m 12h 42m 9.3Gx CVn C32/NGC4631Apr 3+11d 33m 12h 43m 8.8Gx Vir M60/NGC4649Apr 5+32d 10m 12h 43m 10.4Gx CVn NGC4656Apr 3-05d 47m 12h 48m 9.3Gx Vir C52/NGC4697Apr 5+41d 07m 12h 50m 8.2Gx CVn M94/NGC4736Apr Black-Eye Galaxy4+21d 40m 12h 56m 8.5Gx Com M64/NGC4826Apr 1+27d 58m 13h 00m 11.4Gx Com C35/NGC4889Apr 2+37d 02m 13h 10m 9.8Gx CVn C29/NGC5005Apr 13+18d 10m 13h 12m 7.7Gb Com M53/NGC5024Apr Sunflower Galaxy 5+42d 01m 13h 15m 8.6Gx CVn M63/NGC5055Apr Whirlpool Galaxy5+47d 12m 13h 29m 8.4Gx CVn M51/NGC5194Apr 11-29d 52m 13h 37m 7.6Gx Hya M83/NGC5236Apr 3+08d 52m 13h 37m 10.2Gx Boo C45/NGC5248Apr 16+28d 22m 13h 42m 6.4Gb CVn M3/NGC5272Apr The Pinwheel Galaxy 27+54d 21m 14h 03m 7.7Gx UMa M101/NGC5457May 4-26d 31m 14h 39m 10.2Gb Hya C66/NGC5694May Possibly M1021+55d 46m 15h 06m 10Gx Dra M102/NGC5866May 13-21d 01m 15h 17m 8.6Gb Lib NGC5897May 17+02d 04m 15h 18m 5.8Gb Ser M5/NGC5904May 9-22d 58m 16h 16m 7.2Gb Sco M80/NGC6093Jun 26-26d 31m 16h 23m 5.9Gb Sco M4/NGC6121Jun 10-13d 03m 16h 32m 8.1Gb Oph M107/NGC6171Jun Great Cluster In Hercules17+36d 28m 16h 41m 5.9Gb Her M13/NGC6205Jun 1+36d 49m 16h 43m 11.6Gx Her NGC6207Jun 15-01d 57m 16h 47m 6.6Gb Oph M12/NGC6218Jun 15-04d 05m 16h 57m 6.6Gb Oph M10/NGC6254Jun 14-30d 07m 17h 01m 6.6Gb Oph M62/NGC6266Jun 14-26d 16m 17h 02m 7.2Gb Oph M19/NGC6273Jun 11+43d 07m 17h 17m 6.5Gb Her M92/NGC6341Jun 9-18d 31m 17h 19m 7.9Gb Oph M9/NGC6333Jun 12-03d 15m 17h 37m 7.6Gb Oph M14/NGC6402Jun Butterfly Cluster 15-32d 13m 17h 40m 4.2OC Sco M6/NGC6405Jun Ptolemy’s Cluster80-34d 49m 17h 53m 3.3OC Sco M7/NGC6475Jun 27-19d 01m 17h 56m 5.5OC Sgr M23/NGC6494Jun Cat’s Eye Nebula 6+66d 37m 17h 58m 9Pl Dra C6/NGC6543Jun Trifid Nebula29-23d 01m 18h 02m 6.3CN Sgr M20/NGC6514Jul Lagoon Nebula/Hourglass Nebula 90-24d 22m 18h 03m 5.8Nb Sgr M8/NGC6523Jul 13-22d 30m 18h 04m 5.9OC Sgr M21/NGC6531Jul Milky Way Patch 5-18d 25m 18h 18m 11OC Sgr M24/NGC6603Jul Eagle Nebula35-13d 46m 18h 18m 6CN Ser M16/NGC6611Jul 9-17d 07m 18h 19m 6.9OC Sgr M18/NGC6613Jul The Omega, Swan, or Horseshoe Nebula46-16d 10m18h 20m 6CN Sgr M17/NGC6618Jul 11-24d 52m 18h 24m 6.9Gb Sgr M28/NGC6626Jul 7-32d 21m 18h 31m 7.7Gb Sgr M69/NGC6637Jul 32-19d 15m 18h 31m 4.6OC Sgr M25/IC4725Jul 24-23d 53m 18h 36m 5.1Gb Sgr M22/NGC6656Jul 8-32d 17m 18h 43m 8.1Gb Sgr M70/NGC6681Jul 15-09d 24m 18h 45m 8OC Sct M26/NGC6694Jul Wild Duck Cluster 14-06d 16m 18h 51m 5.8OC Sct M11/NGC6705Jul Ring Nebula3+33d 01m 18h 53m 9Pl Lyr M57/NGC6720Jul 9-30d 28m 18h 55m 7.7Gb Sgr M54/NGC6715Jul 7+30d 10m 19h 16m 8.3Gb Lyr M56/NGC6779Jul The Coathanger or Brocchi’s Cluster 60+20d 10m 19h 25m 4OC Vul Cr399Jul 19-30d 58m 19h 40m 7Gb Sgr M55/NGC6809Jul Blinking Planetary 2+50d 31m 19h 44m 10Pl Cyg C15/NGC6826Jul Barnard’s Galaxy 10-14d 48m 19h 44m 9Gx Sgr C57/NGC6822Jul 7+18d 46m 19h 53m 8.3Gb Sge M71/NGC6838Jul Dumbbell Nebula15+22d 43m 20h 00m 8.1Pl Vul M27/NGC6853Jul 20+35d 46m 20h 05m 5.2OC Cyg NGC6871Aug 6-21d 55m 20h 06m 8.6Gb Sgr M75/NGC6864Aug 7+26d 28m 20h 11m 6OC Vul C37/NGC6885Aug Crescent Nebula20+38d 21m 20h 11m -Nb Cyg C27/NGC6888Aug 7+38d 31m 20h 23m 6.6OC Cyg M29/NGC6913Aug 6+07d 24m 20h 34m 8.9Gb Del C47/NGC6934Aug 11+60d 08m 20h 34m 8.9Gx Cep C12/NGC6946Aug Veil/Lace Work/Filamentary Neb 70+30d 43m 20h 45m -SNR Cyg C34/NGC6960Aug 6-12d 31m 20h 53m 9.4Gb Aqr M72/NGC6981Aug Veil/Lace Work/Filamentary Neb 60+31d 43m 20h 56m -SNR Cyg C33/NGC6992Aug North America Nebula120+44d 19m 20h 58m -Nb Cyg C20/NGC7000Aug 3-12d 37m 20h 58m 9OC Aqr M73/NGC6994Aug 18+68d 10m 21h 00m 7CN Cep C4/NGC7023Aug 3+16d 10m 21h 01m 10.6Gb Del C42/NGC7006Aug Saturn Nebula2-11d 22m 21h 04m 8Pl Aqr C55/NGC7009Aug 12+12d 10m 21h 30m 6.4Gb Peg M15/NGC7078Aug 32+48d 25m 21h 32m 4.6OC Cyg M39/NGC7092Aug 13-00d 49m 21h 33m 6.5Gb Aqr M2/NGC7089Aug 11-23d 10m 21h 40m 7.5Gb Cap M30/NGC7099Aug Cocoon Nebula 12+47d 16m 21h 53m 7.2CN Cyg C19/IC5146Aug 21+49d 52m 22h 15m 6.4OC Lac C16/NGC7243Sep Helix Nebula13-20d 48m 22h 29m -Pl Aqr C63/NGC7293Sep 4+34d 25m 22h 37m 9.5Gx Peg C30/NGC7331Sep 30+62d 36m 22h 56m -Nb Cep C9Sep 2+12d 19m 23h 04m 11Gx Peg C44/NGC7479Sep Bubble nebula 15+61d 12m 23h 20m -Nb Cas C11/NGC7635Sep 13+61d 34m 23h 24m 6.9OC Cas M52/NGC7654Sep Blue Snowball2+42d 32m 23h 25m 9Pl And C22/NGC7662Sep 16+56d 43m 23h 56m 6.7OC Cas NGC7789Sep。
- 1、下载文档前请自行甄别文档内容的完整性,平台不提供额外的编辑、内容补充、找答案等附加服务。
- 2、"仅部分预览"的文档,不可在线预览部分如存在完整性等问题,可反馈申请退款(可完整预览的文档不适用该条件!)。
- 3、如文档侵犯您的权益,请联系客服反馈,我们会尽快为您处理(人工客服工作时间:9:00-18:30)。
1. Introduction
The goal of this series of papers is to understand the properties of the entire star cluster population of the interacting spiral galaxy M51. These properties include the age and mass distribution of the cluster population. Additional properties are the survival rate of the clusters as well as any relations between the observed properties. These relations may be used to constrain cluster formation and destruction scenarios. In order to study the above properties, we exploit the large amount of HST broad-band archival data on M51, which covers roughly 50% of the observed surface area of M51, and covers a broad spectral range (UV to NIR). The large spatial coverage is necessary in order to obtain a large sample of clusters for carrying out a statistical analysis, and the broad spectral range allows accurate determinations of the individual cluster properties (Bik et al. 2003; Anders et al. 2004). A preliminary analysis of a subset of the M51 cluster population was carried out by Bik et al. (2003; hereafter Paper I) who introduced the method used to determine the cluster properties and derived the age and mass distributions of the cluster sample roughly 2 kpc to the North East of the nucleus.
arXiv:astro-ph/0506066v2 5 Jun 2005
M. Gieles1 , N. Bastian1 , H.J.G.L.M. Lamers1,2 , and J.N. Mout1
1 Astronomical Institute, Utrecht University, Princetonplein 5, NL-3584 CC Utrecht, The Netherlands e-mail: gieles@astro.uu.nl 2 SRON Laboratory for Space Research, Sorbonnelaan 2, NL-3584 CA Utrecht, The Netherlands
Send offprint requests to : gieles@astro.uu.nl
Bastian et al. (2005; hereafter Paper II) extended the survey to include the entire inner ∼ 5 kpc of M51, and found 1152 clusters, 305 of which had accurate size determinations. In that work we extended the age distribution analysis of Paper I and found evidence for a cluster formation rate increase ∼ 50 − 70 Myr ago. This corresponds to the last close passage of NGC 5195 and M51 (Salo & Laurikainen 2000). Additionally we found that 68 ±15% of the clusters forming in M51 will disrupt within the first ∼ 10 Myr after their formation, independent of their mass, so-called infant mortality. For the resolved cluster sample, we found that the size distribution (the number of clusters as a function of their effective radius) can be well fit by a power-law: N dreff ∝ reff −η dreff , with η = 2.2 ± 0.2, which is very similar to that found for Galactic globular clusters. Finally, we did not find any relation between the age and mass, mass and size, nor distance from the galactic center and cluster size. In this study we focus on the evolution of the population of clusters in M51, in particular the timescale of cluster disruption and possible variations in the cluster formation rate. Cluster disruption of multi-aged populations, which excludes the galactic globular clusters, has been the subject of many earlier studies (e.g. Hodge (1987) for the SMC and Battinelli & Capuzzo-Dolcetta (1991) for the
Astronomy & Astrophysics manuscript no. m51-v12 (DOI: will be iቤተ መጻሕፍቲ ባይዱserted by hand later)
February 2, 2008
The Star Cluster Population of M51: III. Cluster disruption and formation history
Received February 2, 2008; accepted ??, 2005 Abstract. In this work we concentrate on the evolution of the cluster population of the interacting galaxy M51 (NGC 5194), namely the timescale of cluster disruption and possible variations in the cluster formation rate. We present a method to compare observed age vs. mass number density diagrams with predicted populations including various physical input parameters like the cluster initial mass function, cluster disruption, cluster formation rate and star bursts. If we assume that the cluster formation rate increases at the moments of the encounters with .6 NGC 5195, we find an increase in the cluster formation rate of a factor of 3.0+4 −1.2 , combined with a disruption .3 8 timescale which is slightly higher then when assuming a constant formation rate (t4 = 2.0+2 −1.1 × 10 yr vs. +0.6 8 1.0−0.5 × 10 yr). The measured cluster disruption time is a factor of 5 shorter than expected on theoretical grounds. This implies that the disk of M51 is not a preferred location for survival of young globular clusters, since even clusters with masses of the order of 106 M⊙ will be destroyed within a few Gyr. Key words. Galaxies: spiral – Galaxies: individual: M51 – Galaxies: star clusters