纳米Ir/IrOx电极的制备及在过氧化氢检测中的应用
纳米电极与纳米孔电极的制备及电化学响应
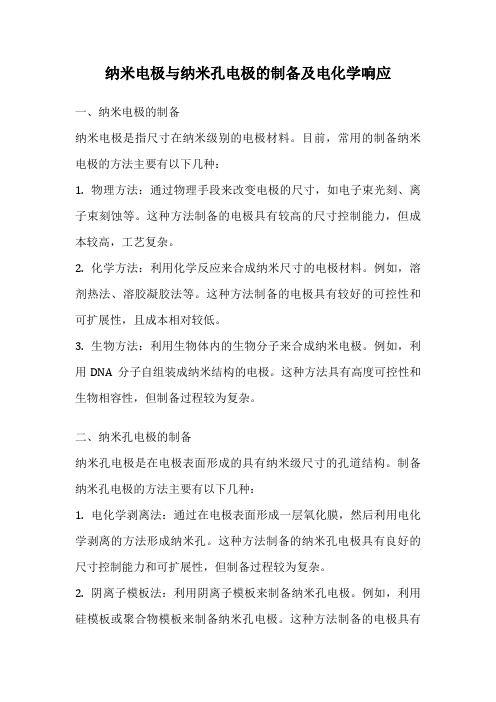
纳米电极与纳米孔电极的制备及电化学响应一、纳米电极的制备纳米电极是指尺寸在纳米级别的电极材料。
目前,常用的制备纳米电极的方法主要有以下几种:1. 物理方法:通过物理手段来改变电极的尺寸,如电子束光刻、离子束刻蚀等。
这种方法制备的电极具有较高的尺寸控制能力,但成本较高,工艺复杂。
2. 化学方法:利用化学反应来合成纳米尺寸的电极材料。
例如,溶剂热法、溶胶凝胶法等。
这种方法制备的电极具有较好的可控性和可扩展性,且成本相对较低。
3. 生物方法:利用生物体内的生物分子来合成纳米电极。
例如,利用DNA分子自组装成纳米结构的电极。
这种方法具有高度可控性和生物相容性,但制备过程较为复杂。
二、纳米孔电极的制备纳米孔电极是在电极表面形成的具有纳米级尺寸的孔道结构。
制备纳米孔电极的方法主要有以下几种:1. 电化学剥离法:通过在电极表面形成一层氧化膜,然后利用电化学剥离的方法形成纳米孔。
这种方法制备的纳米孔电极具有良好的尺寸控制能力和可扩展性,但制备过程较为复杂。
2. 阴离子模板法:利用阴离子模板来制备纳米孔电极。
例如,利用硅模板或聚合物模板来制备纳米孔电极。
这种方法制备的电极具有较好的可控性和可扩展性,但模板的制备过程较为复杂。
3. 液相剥离法:通过在电极表面涂覆一层液体,然后利用溶剂蒸发的方法形成纳米孔。
这种方法制备的电极简单易行,但尺寸控制能力较差。
三、纳米电极与纳米孔电极的电化学响应纳米电极和纳米孔电极在电化学领域具有广泛应用。
它们的电化学响应主要体现在以下几个方面:1. 电极反应:纳米电极和纳米孔电极可以用于催化反应和电催化反应。
由于其高比表面积和尺寸效应的存在,它们能够提供更多的活性位点和更快的反应速率,从而提高反应效率。
2. 电化学传感:纳米电极和纳米孔电极可以用于构建高灵敏度的电化学传感器。
通过改变电极表面的形貌和结构,可以提高传感器的灵敏度和选择性,实现对特定分子或离子的检测和分析。
3. 能量储存与转化:纳米电极和纳米孔电极在能量储存与转化领域也具有重要应用。
纳米氧化铱制备

纳米氧化铱制备全文共四篇示例,供读者参考第一篇示例:纳米氧化铱制备纳米氧化铱是一种具有广泛应用价值的纳米材料,具有较大的比表面积和特殊的物理化学性质,因此在催化、传感、电化学和生物医药等领域有着重要的应用。
在制备纳米氧化铱的过程中,选择合适的合成方法和控制材料的形貌和结构至关重要。
一种常见的制备纳米氧化铱的方法是溶胶-凝胶法。
溶胶-凝胶法是一种将溶胶转化为凝胶的方法,通过溶胶的胶凝反应形成固体颗粒。
通常,将铱盐与溶剂混合并加热搅拌,促使铱盐完全溶解,形成均匀的溶液。
然后通过加入沉淀剂或结晶剂,使溶液中的铱离子沉淀或结晶成固体颗粒。
通过烘干和煅烧等步骤,形成纳米氧化铱。
另一种制备纳米氧化铱的方法是气相沉积法。
气相沉积法是一种在高温环境下直接将气态前体物质输送到基底表面并沉积形成颗粒的方法。
通过选择合适的气态前体物质和控制沉积条件,可以得到具有优良性质的纳米氧化铱。
气相沉积法的优点是可以制备高纯度、晶体度高的纳米氧化铱,但也存在着设备成本高、操作复杂等缺点。
制备纳米氧化铱是一项具有挑战性的工作,需要选择合适的合成方法和控制材料的形貌和结构。
随着科技的不断发展和进步,纳米氧化铱在各个领域的应用前景必将更加广阔。
希望未来能够有更多的研究者投入到纳米氧化铱的制备和应用中,为推动纳米材料科学的发展做出更大的贡献。
第二篇示例:纳米氧化铱(IrO2)是一种具有重要应用价值的高性能材料,具有优良的电化学性能和热稳定性,被广泛应用于催化剂、电极材料、电池材料等领域。
制备纳米氧化铱的方法有很多种,其中包括溶胶-凝胶法、磁控溅射法、水热合成法等。
本文将重点介绍纳米氧化铱的制备方法及其在催化领域的应用。
一、溶胶-凝胶法制备纳米氧化铱溶胶-凝胶法是一种常用的制备纳米氧化铱的方法。
其基本原理是将金属离子溶解在有机溶剂中形成溶胶,经过适当的处理后形成胶体,最终进行热处理制备成纳米氧化铱。
此方法制备的纳米氧化铱具有较高的比表面积和较好的分散性,具有潜在的应用前景。
一种快速检测过氧化氢含量的方法[发明专利]
![一种快速检测过氧化氢含量的方法[发明专利]](https://img.taocdn.com/s3/m/15044f20aa00b52acec7cab6.png)
专利名称:一种快速检测过氧化氢含量的方法专利类型:发明专利
发明人:李剑锋,温宝英
申请号:CN201911174672.3
申请日:20191126
公开号:CN110779907A
公开日:
20200211
专利内容由知识产权出版社提供
摘要:本发明公开了一种用于快速检测过氧化氢含量的SERS芯片,所述SERS芯片包括基底以及滴加于所述基底上的增强纳米粒子,所述基底上修饰有苯硼酸类分子,所述增强纳米粒子包括金纳米粒子以及包裹于所述金纳米粒子表面的惰性壳层。
本发明还提供了一种利用所述SERS芯片快速检测过氧化氢含量的方法,包括将含有过氧化氢代谢产物的溶液滴在所述SERS芯片上,进行拉曼光谱测试,得到过氧化氢与苯硼酸类分子反应后的拉曼图谱,与标准曲线对照,从而计算出过氧化氢的含量。
本发明的检测方法具有前处理简单、快速、高效、特异性强的优点。
申请人:启东科赛尔纳米科技有限公司
地址:226200 江苏省南通市启东市经济开发区林洋路500号
国籍:CN
代理机构:苏州市中南伟业知识产权代理事务所(普通合伙)
代理人:郭磊
更多信息请下载全文后查看。
纳米银修饰石墨电极伏安法测定过氧化氢
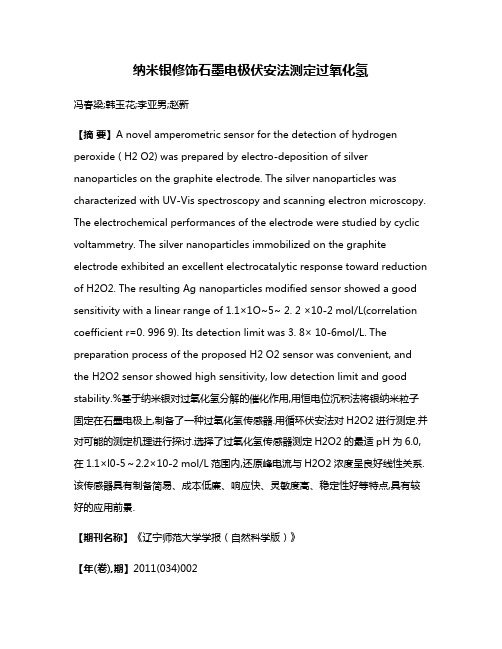
纳米银修饰石墨电极伏安法测定过氧化氢冯春梁;韩玉花;李亚男;赵新【摘要】A novel amperometric sensor for the detection of hydrogen peroxide ( H2 O2) was prepared by electro-deposition of silver nanoparticles on the graphite electrode. The silver nanoparticles was characterized with UV-Vis spectroscopy and scanning electron microscopy. The electrochemical performances of the electrode were studied by cyclic voltammetry. The silver nanoparticles immobilized on the graphite electrode exhibited an excellent electrocatalytic response toward reduction of H2O2. The resulting Ag nanoparticles modified sensor showed a good sensitivity with a linear range of 1.1×1O~5~ 2. 2 ×10-2 mol/L(correlation coefficient r=0. 996 9). Its detection limit was 3. 8× 10-6mol/L. The preparation process of the proposed H2 O2 sensor was convenient, and the H2O2 sensor showed high sensitivity, low detection limit and good stability.%基于纳米银对过氧化氢分解的催化作用,用恒电位沉积法将银纳米粒子固定在石墨电极上,制备了一种过氧化氢传感器.用循环伏安法对H2O2进行测定.并对可能的测定机理进行探讨.选择了过氧化氢传感器测定H2O2的最适pH为6.0,在1.1×l0-5~2.2×10-2 mol/L范围内,还原峰电流与H2O2浓度呈良好线性关系.该传感器具有制备简易、成本低廉、响应快、灵敏度高、稳定性好等特点,具有较好的应用前景.【期刊名称】《辽宁师范大学学报(自然科学版)》【年(卷),期】2011(034)002【总页数】4页(P188-191)【关键词】纳米银粒子;石墨电极;过氧化氢;电化学传感器;循环伏安法【作者】冯春梁;韩玉花;李亚男;赵新【作者单位】辽宁师范大学化学化工学院功能材料化学研究所。
纳米材料化学修饰电极的制备及其在药物分析中的应用
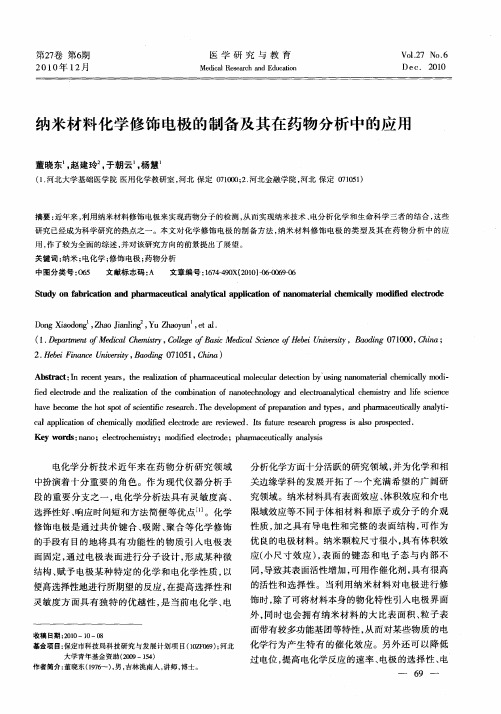
医 学 研 究 与 教 育
Me ia R s ac n d c t n dc l e e r h a d E u ai o
V0 .7 1 No. 2 6
D ec. 2 1 00
纳米材料化学修饰 电极 的制备及其在药物 分析 中的应 用
董晓 东 赵建 玲 于朝 云 杨 慧 , , ,
(. 1河北 大学基础 医学 院 医用化学教研室 , 河北 保定 0 10 ; . 7002 河北金融学 院, 河北 保定 0 15 ) 70 1
摘要 : 近年来 , 利用纳米材料修饰 电极 来实现药 物分 子的检测 , 从而实现纳米技术 、 电分析化学 和生命科学 三者的结合 , 这些 研究 已经成为科学研究的热点之一。本文 对化学 修饰 电极的制备方 法 , 纳米材 料修饰 电极 的类 型及其在 药物分 析 中的应
c la p iain o h mial di e lcrd l e iwe a p l t fc e c y mo f d ee to e ae r ve d.Isf t r s a c r ge s i s r s e td. c o l i t u u r e r h p rs s a o p p ce e e o l o
D n a o g , h oJa l g , u Z a y n ,t . o gXio n Z a ini = Y h o u e d n 1 a
( . eatetfMei l hmsy C lg B s ei l c neo H bi nv sy,B o i 7 00 C i ; 1D p r n m o d a e ir , oee c C t l o ai M d a Si c ee U i rt ad g0 10 , h a f c c e f ei n n
纳米材料化学修饰电极的制备及其在药物分析中的应用

Study on fabrication and pharmaceutical analytical application of nanomaterial chemically modified electrode Dong Xiaodong , Zhao Jianling , Yu Zhaoyun , et al. ( 1. Department of Medical Chemistry , College of Basic Medical Science o f Hebei University , Baoding 071000, China ; 2. Hebei Finance University , Baoding 071051, China ) Abstract : In recent years, the realizat ion of pharmaceutical molecular detection by using nanomaterial chemically modi fied electrode and the realizat ion of the combinat ion of nanotechnology and electroanalytical chemistry and life science have become the hot spot of scientific research. The development of preparation and types, and pharmaceut ically analyti cal application of chemically modified electrode are reviewed. Its future research progress is also prospected. Key words: nano; electrochemistry; modified electrode; pharmaceutically analysis 电化学分析技术近年来在药物分析研究领域 中扮演着十分重要的角色。作为现代仪器分析手 段的重要分支之一 , 电化学分析法具有灵敏度高、 选择性好、 响应时间短和方法简便等优点 。化学 修饰电极是通过共价键合、 吸附、 聚合等化学修饰 的手段有目的地将具有功能性的物质引入电极表 面固定, 通过电极表面进行分子设计, 形成某种微 结构、 赋予电极某种特定的化学和电化学性质 , 以 便高选择性地进行所期望的反应 , 在提高选择性和 灵敏度方面具有独特的优越性, 是当前电化学、 电
二氧化钌和核黄素纳米复合材料修饰的玻璃电极测定过氧化氢
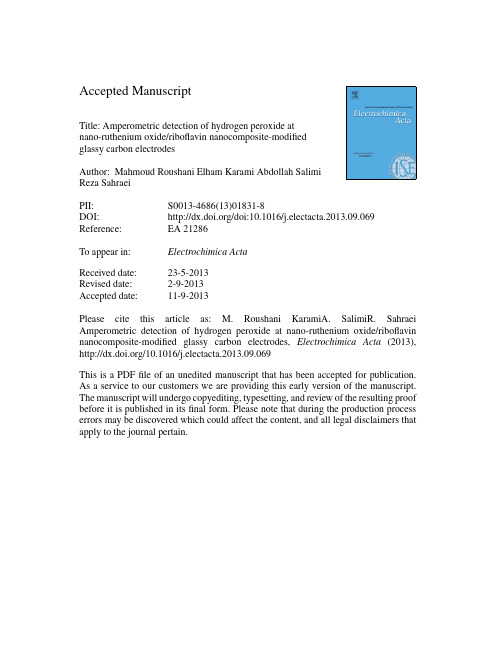
Accepted ManuscriptTitle:Amperometric detection of hydrogen peroxide atnano-ruthenium oxide/riboflavin nanocomposite-modifiedglassy carbon electrodesAuthor:Mahmoud Roushani Elham Karami Abdollah SalimiReza SahraeiPII:S0013-4686(13)01831-8DOI:/doi:10.1016/j.electacta.2013.09.069 Reference:EA21286To appear in:Electrochimica ActaReceived date:23-5-2013Revised date:2-9-2013Accepted date:11-9-2013Please cite this article as:M.Roushani KaramiA.SalimiR.Sahraei Amperometric detection of hydrogen peroxide at nano-ruthenium oxide/riboflavin nanocomposite-modified glassy carbon electrodes,Electrochimica Acta(2013), /10.1016/j.electacta.2013.09.069This is a PDFfile of an unedited manuscript that has been accepted for publication. As a service to our customers we are providing this early version of the manuscript. The manuscript will undergo copyediting,typesetting,and review of the resulting proof before it is published in itsfinal form.Please note that during the production process errors may be discovered which could affect the content,and all legal disclaimers that apply to the journal pertain.Accept ed Manus cript 1Amperometric detection of hydrogen peroxide at nano-ruthenium oxide/riboflavin nanocomposite-modified glassy carbon electrodesMahmoud Roushani a,*, Elham Karami a , Abdollah Salimi b , Reza Sahraei a a Department of Chemistry,Ilam of University, Ilam, Iran b Department of Chemistry, University of Kurdistan, Sanandaj, Iran Corresponding author. Tel/fax: +98 841 2227022E-mail address: mahmoudroushani@ (M. Roushani).A c c e p t e dM a n u s c r i p t2ABSTRACTA simple and sensitive electrochemical sensor based on nano-ruthenium oxide/riboflavin modified glassy carbon (GC/RuOx/RF) electrode was constructed and utilized to determine H 2O 2. By immersing the GC/RuOx modified electrode into RF solution for a short period of time (5–70s), a thin film of the proposed molecule was immobilized onto the electrode surface.The modified electrode showed stable and a well-defined redox couples at a wide pH range (1–10), with surface confined characteristics.Experimental results revealed that RF was adsorbed on the surface of RuOx nanoparticles (NPs) and in comparison to usual methods for the immobilization of RF, such as electropolymerization, the electrochemical reversibility and stability of this modified electrode has been improved.The heterogeneous electron transfer rate constant (k s ) and the surface coverage of immobilized RF on the GC/RuOx electrode was obtained as 16.8 s −1 and2.2×10−11 mole cm −2, respectively.The electrocatalytic activity of the modified electrode toward hydrogen peroxide reduction was investigated and it was found that the GC/RuOx/RF electrode illustrates electrocatalytic activity toward H 2O 2 reduction at reduced overpotential. The catalytic rate constant (k cat ) of the modified electrode toward H 2O 2 is 9.1 ×103 M -1 s -1. This biosensor shows excellent sensitivity towards H 2O 2 in buffer solution (pH 7) with a wide linear range from 0.15 µM to 3 mM. The detection limit and sensitivity are 150 nM and 35 nA µM -1 respectively.Furthermore, the fabricated H 2O 2 chemical sensor exhibited excellent stability, remarkable catalytic activity and reproducibility.Keyword s:Hydrogen peroxide; Nano-ruthenium oxide;RF; Chemical modified electrode;Electrocatalytic.A c c e p t e d M a n u s c r i p t 31. IntroductionThe simple, rapid, reliable, sensitive determination of a small quantity of hydrogen peroxide (H 2O 2)at physiological pH has become an extremely important topic of research in recent years,because of its wide and varied applications. As a well-known oxidizing agent, H 2O 2 is employed in textiles, cleaning products, organic compounds, the food industry, and for environmental treatments [1–3].Furthermore, H 2O 2 is the vital intermediate product of enzymatic reactions in the field of biosensing [4].Up to now, many traditional techniques including chemiluminescence [5], titrimetry [6], spectrophotometry [7], fluorescence [8] and electroanalytical methods [9–11] have been employed for detecting H 2O 2. Among these methods, electroanalytical method is attractive because of its low cost, operational simplicity, high sensitivity and the possibility for real-time detection [12–14].Design, fabrication and application of novel electrochemical sensors have been a topic of research in recent years [15, 16].Recently,more attention has been focused on the synthesis and application of NPs, since they have properties such as high electrical conductivity, high surface area and chemical stability [17, 18].The NPs can be used to promote electron transfer reactions when used as electrode material in electrochemical device. Therefore, modification of electrochemical interface with nanosized metal and metal oxide nanostructures is one of the recent approaches used extensively in the development of sensing platforms [19].Among most NPs reported, RuOx has been widely investigated because of its good electrochemical catalysis (e.g. chlorine and oxygen evolution) and photochemical catalysis (e.g. organic compound and water decomposition) excellent charge storage property [20, 21], and applications for modification of different electrode surfaces [22–24].A c c e p t e dM a n u s c r i p t4RF (6,7-dimethyl 9-(d-1-ribityl)-isoalloxazine), commonly called vitamin B 2, is a water soluble vitamin.RF is an essential precursor of these coenzymes [25].The electrochemical behavior of flavins has been studied by several investigations [26-28]. These compounds show reversible two electron reduction and oxidation waves, both due to overlapping one-electron waves.In the present study, RuOx nanostructures were used for the immobilization of RF. The prepared nanocomposite has been used as an excellent catalyst for H 2O 2 reduction at lower overpotentials. Cyclic voltammetry and amperometry have been used for the investigation of the electrochemical properties and electrocatalytic activity of the nanocomposite-modified electrode.The fabricated sensor was used for the detection of micromolar or even lowers concentrations of H 2O 2 and linear concentration rang at physiological pH, using hydrodynamic amperometry.To the best our knowledge, there is no report on the application of GC/RuOx/RFelectrode to for the detection of H 2O 2.2. Experimental2.1. Reagents and apparatusRF, RuCl 3·xH 2O and other reagents were purchased from Merck and used without purification. H 2O 2 (30%, w/w) was obtained from Merck, and its diluted solution was prepared daily. The buffer solutions (0.1 M) were made from Na 3PO 4, NaH 2PO 4 and Na 2HPO 4 and the pH was adjusted with 0.1 M H 3PO 4 or 1.0 M NaOH. The pH was measured with a Metrohm model 780 pH/mV meters. Solutions were deaerated by bubbling high purity (99.99%) N 2 gas through them prior to the experiments. All electrochemical experiments were carried out at a temperature of 25±0.1 ◦C.A c c e p t e d M a n u s c r i p t5All the electrochemical experiments were performed on a μ-AUTOLAB type III and FRA2 board computer controlled Potentiostat/Galvanostat (Eco-Chemie, The Switzerland) driven with NOVA software. A conventional three-electrode cell was used with a Ag/AgCl/(sat.KCl) reference electrode, a Pt wire as the counter electrode and a GC disk (modified and unmodified) as the working electrode. Voltammetry on electrodes coated with RuOx NPs and RFmodified electrodes was carried out in buffers free of RF molecules. The scanning electron micrographs (SEM) of the electrode surface were obtained by scanning electron microscopy (Philip’s Company, Netherlands) at an acceleration voltage of 20 kV.2.2.Modification of GC and GC/RuOx electrodes with RFPrior to modification, the bare GC electrode (2 mm in diameter) was polished successively with alumina on a polishing cloth and then rinsed with doubly distilled water. After being cleaned, it was modified by 20 cycles of potential sweep between −0.5 and 1.7 V at 50 mV s −1 in a 0.1 M HCl containing about 1.0 mM of ruthenium (III) chloride. The GC/RuOx electrode, was rinsed thoroughly with water and placed in a 0.1M phosphate buffer solution (pH 2). Subsequently, the potential was scanned for 10 cycles at 100mV s −1 over the range of -0.300mV to 0.600mV so as to obtain a stable redox response for the surface-immobilized film. The results show the current response of the redox couples decreases at the first and then remain almost constant.The effective surface area of the electrode modified with RuOx NPs was determined to be 0.08 cm 2 from the cyclic voltammogram of 1mM K 3[Fe(CN)6] in buffer solution, pH 7.An stable thin film of the RF molecule adsorbed on the electrode surfaces by immersing the GC/RuOx electrode in fresh buffer solution (pH 7) containing 0.1mM RF, for 5–70 s.A c c e p t e d M a n u sc r i p t 63.Result and discussion3.1.Electrochemical properties of RF modified electrodeScanning electron microscopy (SEM) was used to characterize the surface morphologies of different electrodes. Fig. 1 shows the SEM of bare GC electrode (Fig. 1A) and GC/RuOxelectrode (Fig. 1B). As it can be seen, when the RuOx NPs are electrodeposited on the GC electrode surface, the nanoparticles of the ruthenium oxide are observed clearly on the GC electrode surface (Fig. 1A).(Fig. 1)Fig. 2 shows the cyclic voltammograms of GC/RuOx and GC/RuOx/RF electrodes in buffer solution (pH 7). As shown for the GC/RuOx electrode, no redox peaks were observed (voltammogram “a”) at a potential range of -0.5 to 0.2 V, which provides a potential window of the GC/RuOx electrode for investigating the voltammetric behavior of RF.As shown for GC/RuOx/RF electrodes, a well-defined cyclic voltammogram with a peak potential separation of less than 20mV and a peak current of 0.95µA was observed at a formal potential of -0.41 V ((voltammogram “b”).In inset Fig. 2, demonstrates a comparison of cyclic voltammograms of as prepared GC/RuOx/RF and GC/RF in a buffer solution solution pH 7.0.As shown in inset Fig.2a by immersing the preanodized GC electrode in 0.1mM RF solution for 1 h, a cyclic voltammogram with low peak current (0.025µA) was observed.For voltammogram “inset Fig. 2a”the peak separation between the oxidation and reduction peaks is increased due to the increasing resistance of the thicker film. However, for the GC/RuOx/RF electrode, a pair of well-defined redox couples, with low peak potential separationA c c e p t e dM a n u s c r i p t7(ΔE= E c - E a = -0.4109 V + 0.4019 V = 0.009 V) and higher peak current (0.4µA)were observed (inset Fig. 2 voltammogram“b”),suggesting that the redox reversibility of RF is significantly improved. In addition to the fact that the porous interfacial layer of the GC/RuOx electrode with a high specific surface area increases the conductive area, RF can penetrate through the conductive porous channels onto the electrode more easily, leading to a higher redox response.Therefore, RuOx NPs can also be used as new materials for the immobilization and electron transfer reactions of RF.(Fig. 2)cyclic voltammetric response of GC/RuOx electrode with different surface concentration of RF, obtained by soaking the GC/RuOx electrode in 0.1 mM RF solution for different times in the blank solution of 0.1 M phosphate buffer (pH 7).The results indicate that, by immersing the electrode for 60 s in RF solution, a stable thin layer of RF was adsorbed at the surface of the electrode. By increasing the immersion time, the surface concentration of RF is increased and begins to level off after 70 s.The influence of potential scan rate on the electrochemical behavior of GC/RuOx/RF electrode in a 0.1 M phosphate buffer solution (pH 7.0) was investigated by cyclic voltammetry (Fig. 3).As shown in the inset of Fig. 3B the peak currents linearly increased with increasing the scan rate from 10 to 2000mV s -1 as expected for redox surface-controlled process. Moreover, the anodic peak currents were almost the same as the corresponding cathodic peak currents and the peak potential did not change with increasing scan rate.The peak-to-peak potential separation is about 9mV for sweep rates below 100mV s −1, suggesting facile charge transfer kinetics over this range of sweep rate.A c c e p t e dM a n u sc r i p t 8At higher sweep rates, the plot of the peak current vs. scan rate deviates from linearity and the peak current becomes proportional to the square root of the scan rate (Fig. 3C), indicating a diffusion-controlled process, which is reflective of the relatively slow diffusion of counter ions into the electrode surfaces. The shifts of the peak potentials were proportional to the logarithm of the scan rate for scan rates higher than 3.0 V s −1 (Fig. 3D). Based on the Laviron theory [29], the k s and charge transfer coefficient (α) can be determined.By measuring the variation of the peak potential with scan rate,using the following Eq. (1), the charge transfercoefficient (α) was determined:(1)The slope of the ΔE pc vs . log (v ) was about 59 mV and the electrons transferred for RF was 2,then, α was calculated as 0.50. By introducing this α value in the following Eq. (2), for ΔE p ≥200/n mV (ν=6000 mV s -1), the k s was estimated to be 16.8 s −1. (2)The large value of the electron transfer rate constant indicates a high ability of RuOx NPs for promoting electrons between RF and the electrode surface.(Fig. 3)The surface coverage can b e evaluated from the equation Γ=Q /nFA , where Q is the charge obtained by integrating the cathodic peak under the background correction, at a low scan rate of 10 mV s -1, and other symbols have their usual meanings. Several parameters such as the electron transfer kinetics between the adsorbed compound and the electrode, the stability of theA c c e p t e d M a n u s c r i p t9sensor and the nature of limitation of the produced current (diffusion or kinetic limited) are directly or indirectly affected by the degree of the surface coverage [30].When the population of redox molecules exceeds the current is not any more proportional to the redox population, since overlapping effect results in a decrease of the rate of increase of the current versus the surface coverage. This phenomenon is more profound at high scan rates [31]. Therefore the calculation of surface coverage was carried out at low scan rate.The surface coverage value for the GC/RuOx/RF electrode was 2.2 ×10−11 mol cm −2, which corresponds to the presence of multilayer of surface species. These results indicate high loading ability of the RuOx NPs for immobilization of RF.3.2. Stability and pH dependence of modified electrodeThe stability of the GC/RuOx/RF electrode was examined by continuous potential cycling between 0 and -0.65 V in 0.1 M phosphate buffer solution (pH 7; not shown). The results indicated that after 100 repetitive cycles at a scan rate of 150 mV s −1, no detectable change was observed at the peak height and potential separation. In addition, it was found that the current value is decreased only by 4% when the electrode was immersed in buffer solution (pH 7) for 24h. Therefore, the mediator is prevented from leaching. Since the method of electrode preparation is simple and fast (less than 2min), the current decay is not a serious disadvantage for this modified electrode. The high stability of adsorbed RF is resulted from the chemical and mechanical stability of the RuOx film and strong adsorption of RF on the surface of RuOx NPs.For investigating the effect of pH on the redox response of modified electrodes, cyclic voltammograms of the GC/RuOx/RF electrode at various pH values (1–10) were recorded (Fig.6). As can be seen in the inset of Fig. 6, the formal potential of the surface redox couple was pH dependent. A plot of E o ʹvs. pH values gives a straight line from pH 1–10 with a slope of -58 mVA c c e p t e d Ma nu s c r i p t10/pH, which is very close to the anticipated Nernstian value of 59mV for a two electron–two proton process.Furthermore, with increasing pH values, the peak current decreased, due to the desorption and dissolution of anionic forms of RF. At pH values higher than 10, the peaks were distorted and the cathodic peak began to disappear. This might be due to the adsorbed film destruction as a result of deprotonation process [32].(3)(Fig. 4)3.3. Electrocatalytic reduction of H 2O 2 at GC/RuOx/RFThe electrocatalytic reduction of H 2O 2 by GC/RuOx/RF electrode was investigated by cyclic voltammetry. Fig. 5 shows the cyclic voltammograms of GC/RuOx, GC/RF and GC/RuOx/RF electrodes in buffer solution (pH 7) in the absence and presence of hydrogen peroxide. There was no reduction peak observed at the GC/RuOx electrode in the absence or presence of H 2O 2 in the potential range of 0.0 to −0.65V, suggesting that the GC/RuOx electrode was inactive to the direct reduction of hydrogen peroxide (Fig. 5 voltammogram “b”).Although the GC/RF electrode is active in the direct reduction of hydrogen peroxide (Fig. 5 voltammogram “d”), but it is unstable and has short lifetimes. Such as RF films, GC/RF films are easily lost from the electrode surface and the catalytic activity is reduced.(Fig. 5)A c c e p t e d M a n u s c r i p t11However, for the GC/RuOx/RF electrode, the reduction of hydrogen peroxide starts at −0.3V and a catalytic reduction peak appears at a potential of −0.41 V. As is shown, the cathodic peak current was enhanced in the presence of hydrogen peroxide and the oxidation peak current decreased, suggesting a typical electrocatalytic reduction process. By increasing the concentration of H 2O 2, the cathodic peak current of the modified electrode increased, while itsanodic peak current decreased,indicating a typical electrocatalytic reduction process (EC ʹ) (Fig.6). The catalytic currents increased linearly with H 2O 2 concentration in the range of 2.0–12μM, and were fitted to the equation I p (μA) = 0.16 [H 2O 2] (μM)+0.043μA and R 2 = 0.995.(Fig. 6)For investigation the electrocatalytic mechanism of the modified electrode toward H 2O 2reduction, cyclic voltammograms of modified electrode in 5 mM H 2O 2 at different scan rates were recorded (not shown). The peak current for the cathodic reduction of H 2O 2 was proportional to the square root of the scan rate, suggesting that the process is controlled by diffusion of analyte as expected for a catalytic system. In addition, a plot of the scan rate-normalized current (I p /v 1/2) vs. scan rate exhibited the characteristic shape of a typical EC ʹcatalytic process. Based on these results, the reaction sequence in the reduction of H 2O 2 by RF redox center can be described by the following catalytic scheme:RF (oxdidized form) + 2H + +2e − RF (reduced form) (4)RF (reduced form) + H 2O 2KcatRF (oxdidized form) + 2H 2O (5)For an EC ʹ mechanism, Andrieux and Saveant theoretical model can be used to calculate the catalytic rate [33]. Based on this model, for a slow scan rate and a large catalytic rate, the relationship between the peak current and the analyte concentration reads:A c c e p t e d Ma n u s c r i p t 12(6)where D and C s are the diffusion coefficient (cm 2 s −1) and the bulk concentration (mol cm −3) of substrate (hydrogen peroxide), respectively, and other symbols have their usualmeanings. For low scan rates (5–20 mV s −1), the average coefficient value (α) in Eq. (6) is found to be 0.3 for a GC/RuOx/RF electrode, with a surface coverage of 2.2 × 10−11 molcm -2 and a geometric area A of 0.08 cm 2 in 0.1 mM hydrogen peroxide at pH 7.0. According to the approach of Andriex and Saveant, and using Fig. 1 in Ref. [33], the average values of the calculated K cat are 9.1×103 M −1 s −1 for modified electrode. The high K cat obtained for GC/RuOx/RF electrode implies that this system can be efficiently used as electrochemical sensorfor H 2O 2 detection.3.4. Amperometric detection of H 2O 2 at GC/RuOx/RFSince amperometry under stirred conditions has a much higher current sensitivity than cyclic voltammetry, it was used to estimate the lower limit of detection for the proposed electrochemical system. As discussed above, the proposed modified electrode showed excellent and strong mediation properties to facilitate the low potential amperometric measurements of hydrogen peroxide.Fig. 7 shows a typical current–time plot of the rotated GC/RuOx/RF electrode (rotation speed 1000 rpm) on successive additions of 250.0 μM and 4mM of hydrogen peroxide at an applied potential of −0.41 V (vs. Ag/AgCl). As shown, the modified electrode responded rapidly and approached 95% of the steady-state current within 3 s.The plot of current response vs. H 2O 2 concentration is shown in the inset of Fig. 7. The calibration plot is linear over a wide concentration range (1μM to 3 mM), while for a highA c c ep t e d M a n u s c r i p t13concentration of H 2O 2 the plot of current vs. analyte concentration deviates from linearity (insets of Fig. 7). The linear least squares calibration curve over the range of 1–10μM (10 points) is I (μA) = 0.035[H 2O 2]μM+ 0.001 μA with a correlation coefficient of 0.999, indicating that the regression line is fitted very well with the experimental data and the regression equation can be applied in the unknown sample determination. The detection limit (when signal to noise ratio was 3) and sensitivity were 150 nM and 35 nA μM −1, respectively. Highly stable amperometric response toward hydrogen peroxide is extremely attractive feature of the GC/RuOx/RF electrode. Fig. 7E shows the amperometric response of 10μM H 2O 2 during a prolonged 600 s experiment. The response remains stable throughout the experiment (only a 1% decrease in current was observed), indicating that RF is effectively stabilized with immobilization onto ruthenium oxide nanomaterials. Therefore, the modified electrode possesses excellent, stable and strong mediation properties and facilitates the low potential amperometric measurement of H 2O 2 at trace levels. Electron transfer rate constant, detection limit and linear calibration range of the proposed modified electrode were compared with those previously reported, and the results are summarized in Table 1. As can be seen, the analytical parameters are comparable or better than the results reported for H 2O 2 determination at the surface of other modified electrodes.(Fig. 7) and Table 14. ConclusionIn the present study, electrodeposited RuO x NPs were used for adsorption of RF.The nanocomposite-modified electrode showed stable and reproducible electrochemical behavior at a wide pH range (1–10).The RF immobilized onto RuO x NPs can be used as excellent electronA c c e p te d M a n u s c r i p t14transfer mediators to shuttle electrons between hydrogen peroxide and substrate electrodes. The proposed modified electrodes were used as sensitive amperometric hydrogen peroxide sensors. These sensors presented good stability as the current response remained almost constant even after a long period of use, confirming the strong adherence of the proposed RF on the RuOx NPs. The fabricated hydrogen peroxide sensor possesses excellent characteristics and performance, such as fast response time, good stability, low detection limit and high sensitivity, manifesting that the immobilization of electroactive RF onto RuOx nanocomposites is a suitable method for sensor construction.The detection limit, sensitivity and linear concentration range of the GC/RuOx/RF electrode were 150nM, 35 nA μM −1 and 1μM to 3mM, respectively. These analytical parameters are comparable or better than the results reported for H 2O 2 determination at the surface of other modified electrodes.A c c e p t e d Ma n u s c r i p t15References[1] M. Somasundrun, K. Kirtikara, M. Tanticharoen, Amperometric determination of hydrogen peroxide by direct and catalytic reduction at a copper electrode,Anal. Chim. Acta 319 (1996) 59.[2] J. Wang, Y. Lin, L. Chen, Organic-phase biosensors for monitoring phenol and hydrogen-peroxide in pharmaceutical antibacterial products, Analyst 118 (1993) 227.[3] M. Darder, K. Takada, F. Pariente, E. Lorenzo, H.D. Abruna, Dithiobissuccinimidyl propionate as an anchor for assembling peroxidases at electrodes surfaces and its application in a H 2O 2biosensor ,Anal. Chem. 71 (1999) 5530.[4] J. Wang, Glucose biosensors: 40 years of advances and challenges, Electroanalyasis 13 (2001) 983.[5] B.X. Li, Z.J. Zhang, Y. Jin,Chemiluminescence flow biosensor for hydrogen peroxide with immobilized reagents,Sens. Actuators B 72 (2001) 115.[6] E.C. Hurdis, H. Romeyn,Accuracy of determination of hydrogen peroxide by cerate oxidimetry,Anal. Chem. 26 (1954) 320.[7] K. Sunil, B. Narayana,Spectrophotometric determination of hydrogen peroxide in water and cream samples,Bull. Environ. Contam. Toxicol. 81 (2008) 422.[8] Y.Z. Li, A. Townshend, Evaluation of the adsorptive immobilisation of horseradish peroxidase on PTFE tubing in flow systems for hydrogen peroxide determination using fluorescence detection, Anal. Chim. Acta 359 (1998) 149.[9] S.Y. Xu, B. Peng, X.Z. Han,A third-generation H 2O 2 biosensor based on horseradish peroxidase-labeled Au nanoparticles self-assembled to hollow porous polymeric nanopheres,Biosens. Bioelectron. 22 (2007) 1807.[10] Q. Rui, K.K. Komori, Y. Tian, H.Q. Liu, Y.P. Luo, Y. Sakai,Electrochemical biosensor for the detection of H 2O 2 from living cancer cells based on ZnO nanosheets,Anal. Chim. Acta 670 (2010) 57.A c c e p t e d Ma n u s c r i p t 16[11] S. Liu, L.M. Li, Q.Y. Hao, X.M. Yin, M. Zhang, Q.H. Li, L.B. Chen, T.H. Wang,A novel non-enzymatic hydrogen peroxide sensor based on Mn-nitrilotriacetate acid (Mn-NTA) nanowires,Talanta 81 (2010) 727.[12] Y. Song, L. Wang, C. Ren, G. Zhu, Z. Li,A novel hydrogen peroxide sensor based on horseradish peroxidase immobilized in DNA films on a gold electrode,Sens. Actuators B 114(2006) 1001.[13] Q. Lu, X. Dong, L.J. Li, X. Hu, Direct electrochemistry-based hydrogen peroxide biosensor formed from single-layer graphene nanoplatelet–enzyme composite film, Talanta 82 (2010)1344.[14]A. Salimi, R. Rahmatpanah, R. Hallaj, M. Roushani, Covalent attachment of thionine onto gold electrode modified with cadmium sulfide nanoparticles: Improvement of electrocatalytic and photelectrocatalytic reduction of hydrogen peroxide,Electrochim. Acta 95 (2013) 60.[15]A. Noorbakhsh, A. Salimi,Amperometric detection of hydrogen peroxide at nano-nickel oxide/thionine and celestine blue nanocomposite-modified glassy carbon electrodes,Electrochim. Acta 54 (2009) 6312.[16]M. Roushani, M. Shamsipur, S. M. Pourmortazavi,Amprometric detection of Glycine, L-Serine, and L-Alanine using glassy carbon electrode modified by NiO nanoparticles, J. Appl. Electrochem. 42 (2012)1005.[17] X. Yan, H. Liu, K.Y. Liew, Size control of polymer-stabilized ruthenium nanoparticles by polyol reduction,J. Mater. Chem. 11 (2001) 3387.[18] A. Balamurugan, K.C. Ho, S.M. Chen, T.Y. Huang, Electrochemical sensing of NADH based on meldola blue immobilized silver nanoparticle-conducting polymer electrode,Colloids Surf. A 362 (2010) 1.[19] P. Biswas, Y. Nodasaka, M. Enyo, M. Haruta,Electro-oxidation of CO and methanol on graphite-based platinum electrodes combined with oxide-supported ultrafine gold particles,J. Electroanal. Chem. 381 (1995) 167.A c c e p t e d Ma n u s c r i p t 17[20] T. Arikawa, Y. Murakami, Y. Takasu,Simultaneous determination of chlorine and oxygen evolving at RuO2/Ti and RuO2–TiO2/Ti anodes by differential electrochemical mass spectroscopy, J. Appl. Electrochem. 28 (1998) 511.[21] K. Ikarashi, J. Sato, H. Kobayashi, N. Saito, H. Nishiyama, Y. Inoue,Photocatalysis for water decomposition by RuO 2-dispersed ZnGa 2O 4 with d 10configuration,J. Phys. Chem. B 106(2002) 9048.[22] H.R. Zare, S.H. Hashemi, A. Benvidi, Electrodeposited nano-scale islands of ruthenium oxide as a bifunctional electrocatalyst for simultaneous catalytic oxidation of hydrazine andhydroxylamine,Anal. Chim. Acta 668 (2010) 182.[23] M. Metikos-Hukovic, R. Babic, F. Jovic, Z. Grubac,Anodically formed oxide films and oxygen reduction on electrodeposited ruthenium in acid solution, Electrochim. Acta 51 (2006) 1157.[24] Hamid R. Zare, F. Chatraei,Preparation and electrochemical characteristics of electrodeposited acetaminophen on ruthenium oxide nanoparticles and its role as a sensor forsimultaneous determination of ascorbic acid, dopamine and N-acetyl-l-cysteine,Sens. Actuators B 160 (2011) 1450.[25] W. Friedrich,Vitamins.Walter de Gruyter, Berlin, 1988, p.404.[26] B. Breyer, T. Biegler,Estimation of riboflavin by A.C polarography,J. Electroanal Chem. 1(1960) 453.[27] A.M. Hartley, G.S. Wilson, Unusual adsorption effects in the electrochemical reduction of flavin mononucleotide at mercury electrodes,Anal. Chem. 38 (1966) 681.[28] L. Gorton, G. Johansson, Cyclic voltammetry of FAD adsorbed on graphite, glassy carbon, platinum and gold electrodes,J. Electroanal. Chem. 113 (1980)151.[29] E. Lavion,General expression of the linear potential sweep voltammogram in the case of diffusion less electrochemical systems, J. Electroanal. Chem. 101 (1979) 19.。
纳米银石墨烯修饰电极-电化学法测定血清中的过氧化氢

纳米银石墨烯修饰电极-电化学法测定血清中的过氧化氢姜浩杰;李盛富;王斌堂【摘要】建立纳米银-石墨烯修饰电极电化学法测定血清中过氧化氢的方法.在pH 7.0的磷酸缓冲溶液中,过氧化氢在-0.1 V处产生明显的还原峰.过氧化氢在纳米银-石墨烯修饰的电极上的反应是典型的表面控制反应过程.过氧化氢的浓度在0.5~2.7 mmol/L范围内与其还原峰峰电流呈良好的线性关系,线性相关系数r2=0.9930,检出限为0.17 mmol/L(信噪比S/N=3),测定结果的相对标准偏差小于5%(n=5),加标回收率为98%~103%.该方法灵敏度高,测定结果准确可靠,可用于血清中过氧化氢的测定.【期刊名称】《化学分析计量》【年(卷),期】2018(027)005【总页数】5页(P34-38)【关键词】纳米银石墨烯修饰电极;电化学法;过氧化氢;血清【作者】姜浩杰;李盛富;王斌堂【作者单位】核工业二一六大队,核工业新疆理化分析测试中心,乌鲁木齐 830000;核工业二一六大队,核工业新疆理化分析测试中心,乌鲁木齐 830000;核工业二一六大队,核工业新疆理化分析测试中心,乌鲁木齐 830000【正文语种】中文【中图分类】O657.1过氧化氢是一种重要的化学产品,被广泛应用于农业、工业、医用、生物、军工以及建材等。
过氧化氢的广泛应用对环境产生了污染,对人体危害较大,例如多次接触过氧化氢可以引起人体遗传物质DNA损伤及基因突变,加速人体衰老进程,导致脑中风、动脉硬化、白内障、老年痴呆、癌症[1–3]。
2018年5月1日国家卫生健康委员会在GBZ/T 300.48–2017中降低了过氧化氢的职业接触限值(时间加权平均容许浓度为1.5 mg/m3),由此可见测定过氧化氢含量,特别是直接测定人体中血液中的过氧化氢具有重要意义。
目前,检测过氧化氢的方法主要分为3类:化学发光法、波谱法和电化学法[4–5]。
其中电化学法因操作简单、检测快速、消耗低、灵敏度高而具有应用优势。
- 1、下载文档前请自行甄别文档内容的完整性,平台不提供额外的编辑、内容补充、找答案等附加服务。
- 2、"仅部分预览"的文档,不可在线预览部分如存在完整性等问题,可反馈申请退款(可完整预览的文档不适用该条件!)。
- 3、如文档侵犯您的权益,请联系客服反馈,我们会尽快为您处理(人工客服工作时间:9:00-18:30)。
广 州 化 学
Gu a n g z ho u Ch e mi s t r y
V 1 . 3 8 No. 1
Ma t . 2 O1 3
文章编号 :1 0 0 9 . 2 2 0 X ( 2 0 1 3 ) 0 1 . 0 0 0 1 . 0 7
关 键 词 :I r / I r O x电极 ;过 氧 化 氢 ; 电位 ;铱 电极
中图分类号 :0 6 5 7 . 1
文献标识码 :A
过氧 化氢 不 仅是 一些 高选 择性 氧化 酶反 应 的副产 物 ,而且 是 食 品、制 药 、I 临床 、环境和 工业 分 析 中一 种 重要 的 中间体 ,研 究低浓 度 过氧化 氢 的检 测方 法在 食 品 、 医 药 的检 测 中具有 重要 意 义 。许 多技术 如滴 定法 、光 谱法 、化 学 发光和 电化学 方法 已被 用于 过氧 化氢 的测 定 。 鉴 于金 属纳米 材 料 以及 金 属铱 对 O 2 析 出 的显 著 的催 化作 用 ,本研 究将 自制 的纳 米铱 及其氧 化物 电极 ( I r / I r Ox )应用 于低 浓度 过氧 化氢 的检 测 。
第3 8 卷
1 . 2 实验步 骤
采 用 方波 伏安 法 ,设 定参数 使 电极 在起 始 电位 静 置 , 通 过 高 电位 氧 化和 低 电位还 原 两个 过 程 ,将 该 电极制 成 纳米 I r / I r Ox 电极 。电极表 征 采用 循环 伏 安法 ;过 氧化 氢 的检测 、条件
进一 步纯 化 。 C HI 4 4 0电化 学 分析仪 ( 上 海辰 华仪 器 公司 ) ;集热 式磁 力搅 拌 器 HJ 一 8( 常州 国华 电器 有 限公 司 ) ;J S M一 6 7 0 0 F 场 发射 显微 镜 ( F E S E M,J E OL 日本 电子 公司 ) 。实验 中采 用三 电极 体系 , 工作 电极 为 1 5 0 g m 的铱 电极 , 参 比 电极 为饱 和甘 汞 电极 ( S C E , 上海 辰华 仪器 公司 ) , 对 电极 为铂片 电极 。
1 实验
1 . 1 试 剂 与仪 器 3 0 %过 氧化 氢 ( A R,国药集 团化学 试剂 有 限公 司 ) ;1 5 0 p m 铱丝 ( 9 9 . 9 9 %,F l u c k ) ;
E 一 4 4( 6 1 0 1 )环 氧树 脂 和 6 5 0 聚 酰胺 树 脂 ( 镇 江 市 丹徒 树 脂 厂 ) ;试 验 用水 均 为 去离 子水 ( Mi l l i p o r e Mi l l i — Q系 统净 化 ) ;其他 试剂 均 为分 析纯 ( 国药集 团化 学试 剂有 限公 司 ) ,未作
将制 得 的 1 5 0 m 的铱 电极置 于 1 mo l / L的 H2 S O4 溶 液 中 ,采 用 方波 伏安 法 ,起始 电位
为5 V,静 止 时间 2 . 5 h ,其它 参 数取 仪器 初 始值 ( 终 止 电位 为 0 V, 电位 增 量 0 . 0 0 4 V,振 幅0 . 0 2 5 V,频 率 1 5 Hz ) 。上 述 过程 中 , 电极 表面 由外而 内逐 渐变 成 棕褐 色 ,然后 逐渐 变 成 黑色 , 说 明 电极表面 的铱 被氧 化 生成 I r Ox ; 然后, 将起 始 电位调 整为 一0 . 5 V, 持续 时 间 1 . 5 h ,
纳米 I r / I r Ox电极 的制备 及在 过氧 化氢检测 中的应用
陈 家越
( 福建船政交通职 业学院 安全技术与环境工程 系,福建 福州 3 5 0 0 0 7 )
摘
要 :在 1 5 0 l a m 的铱 电极上 ,采用 高电位氧 化和低 电位还原的方法制备纳米多
孔I r / I r Ox电极 ,并用扫描 电镜 、循环伏 安方法对 其结构和 电极的 电化学进行表征。 纳米多孔 I f l r O x 电极用于过氧化氢的检测 ,灵敏度高 ,线性范 围宽 ,分别为 1 O ~ 5 9 0 g mo l / L和 2 -6 0 mmo l / L ,检测 限为 2 . 7 7 g mo l / L。实验 结果令人满意 。
优 化 以及 干扰 测 定过程 中均采 用 , ~f 曲线 法 ,取样 间 隔均 为 0 . 1 S 。
2 结果 与讨论
2 . 1 纳米 多孔 I r / I r O x电极 的制备 用质 量 比为 1:1的环 氧 树脂 和聚 酰 胺树 脂 ,将 1 5 0 u m 铱 丝 密封 在直 径 为 5 mm 的 玻
璃管 内,室温 干燥 4 8 h后 ,用粗 砂纸 打 磨至 铱丝 露 出,再用 粒径 依 次减 小 的砂纸 打磨 至 光 滑 ,然后 分 别用 丙酮 、乙醇 和二 次水 各超 声 清洗 5 mi n ,除 去表 面 的有机 杂质 ,最后 依次 用 粒径 为 0 . 1 、0 . 0 3 、0 . 0 5 u m 的 AI 2 03 粉 末 抛光 ,二次 水清 洗后 备用 。
收稿 日期 :2 0 1 2 . 1 2 — 1 3
基金项 目:福建省教育厅科技资助项 目 ( J Bl 1 3 2 8 ) 。 作者,副教授 ,硕士 ;主 要从事电化学分析检测、水污染治理 研究 。
2
广 州 化 学
电极表 面 的氧 化物 被 部 分还 原生 成 纳 米铱 。生 成 的 电极 为 I r / I r Ox 电极 ,上 述 制 备过 程 如
图1 所示。
S WV 1 5 0 0 0Hz ,
5 V 2 . 5 h ] S W 一 V O , . 5 1 5 V 0 0 1 0 . 5 H h z , ]