Nucleosynthesis in Core-Collapse Supernovae and GRB--Metal-Poor Star Connection
冲击波聚心的英文
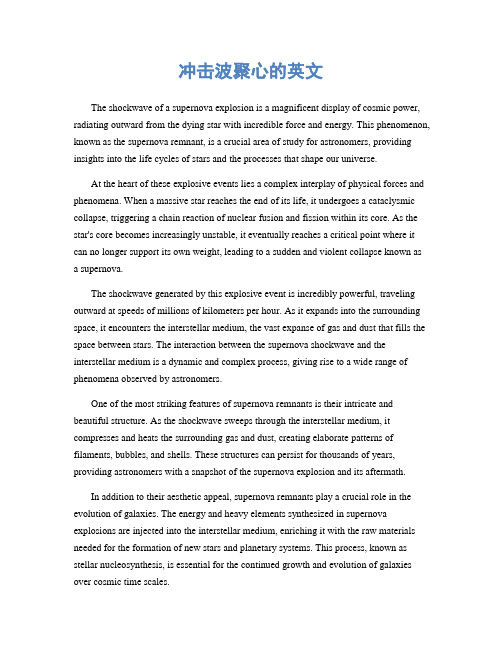
冲击波聚心的英文The shockwave of a supernova explosion is a magnificent display of cosmic power, radiating outward from the dying star with incredible force and energy. This phenomenon, known as the supernova remnant, is a crucial area of study for astronomers, providing insights into the life cycles of stars and the processes that shape our universe.At the heart of these explosive events lies a complex interplay of physical forces and phenomena. When a massive star reaches the end of its life, it undergoes a cataclysmic collapse, triggering a chain reaction of nuclear fusion and fission within its core. As the star's core becomes increasingly unstable, it eventually reaches a critical point where it can no longer support its own weight, leading to a sudden and violent collapse known as a supernova.The shockwave generated by this explosive event is incredibly powerful, traveling outward at speeds of millions of kilometers per hour. As it expands into the surrounding space, it encounters the interstellar medium, the vast expanse of gas and dust that fills the space between stars. The interaction between the supernova shockwave and the interstellar medium is a dynamic and complex process, giving rise to a wide range of phenomena observed by astronomers.One of the most striking features of supernova remnants is their intricate and beautiful structure. As the shockwave sweeps through the interstellar medium, it compresses and heats the surrounding gas and dust, creating elaborate patterns of filaments, bubbles, and shells. These structures can persist for thousands of years, providing astronomers with a snapshot of the supernova explosion and its aftermath.In addition to their aesthetic appeal, supernova remnants play a crucial role in the evolution of galaxies. The energy and heavy elements synthesized in supernova explosions are injected into the interstellar medium, enriching it with the raw materials needed for the formation of new stars and planetary systems. This process, known as stellar nucleosynthesis, is essential for the continued growth and evolution of galaxies over cosmic time scales.Furthermore, supernova remnants are also sites of intense physical processes, including the acceleration of charged particles to relativistic speeds. These high-energy particles can interact with magnetic fields and emit radiation across the entire electromagnetic spectrum, from radio waves to gamma rays. Studying the emission from supernova remnants allows astronomers to probe the underlying physics of particle acceleration and magnetic field dynamics in extreme environments.In recent years, advances in observational techniques have led to a deeper understanding of supernova remnants and their role in the universe. High-resolution imaging and spectroscopic observations have revealed the intricate details of shockwave dynamics and the distribution of heavy elements within these cosmic relics. Theoretical models and numerical simulations have provided valuable insights into the physical processes driving the evolution of supernova remnants and their impact on galactic ecosystems.Looking ahead, future observations with next-generation telescopes and space-based observatories promise to unveil even more secrets of supernova remnants. By studying these cosmic artifacts in greater detail, astronomers hope to unravel the mysteries of stellar death and the profound influence it has on the evolution of the cosmos. From the heart of a dying star to the farthest reaches of the universe, the shockwaves of supernova explosions continue to captivate our imagination and inspire scientific discovery.。
长链的非编码RNA生长停滞特异性转录本5在骨关节炎中的研究进展

长链的非编码RNA生长停滞特异性转录本5在骨关节炎中的研究进展张晓彤;郝婧婧;李芳;纪斌平;张芳芳【期刊名称】《安徽医药》【年(卷),期】2024(28)5【摘要】骨关节炎(OA)是一种以软骨退变、骨重建为主要病理特点的慢性关节疾病。
目前没有可治愈该疾病的药物及手段,治疗策略有限的根本原因是对其潜在发病机制的认识不足。
长链的非编码RNA(LncRNAs)被认为是许多疾病中的一类新的调控因子,是目前研究OA发病机制的一个重要切入点。
LncRNA生长停滞特异性转录本5(Cas5)参与调控细胞的增殖、分化及凋亡,是一种在软骨细胞中特异性高表达的LncRNA。
然而,由于LncRNA Cas5作用机制复杂,其与OA相关具体分子机制目前仍不明确。
该文针对LncRNA Cas5在OA中的软骨细胞凋亡、软骨基质代谢成骨分化及治疗方面的作用进行综述。
【总页数】4页(P870-873)【作者】张晓彤;郝婧婧;李芳;纪斌平;张芳芳【作者单位】山西医科大学第二临床医学院;山西医科大学第二医院风湿免疫科;山西华晋骨科医院骨科【正文语种】中文【中图分类】R73【相关文献】1.子痫前期患者胎盘组织中长链非编码RNA生长停滞特异性转录本5表达水平及临床意义2.长链非编码RNA生长停滞特异性转录本5在糖尿病肾病进展中作用机制研究3.长链非编码RNA生长停滞特异性转录本5在妇科恶性肿瘤中的研究进展4.长链非编码RNA生长停滞特异性转录本5(lncGAS5)促进同型半胱氨酸致肝细胞自噬作用5.长链非编码生长停滞特异性蛋白6反义RNA1靶向miRNA-374a-3p 调控高糖诱导的人肾小管上皮细胞损伤及纤维化分子机制的研究因版权原因,仅展示原文概要,查看原文内容请购买。
英语 人类的故事 从宇宙到地球

The birth of nebulae and stars
Gravity and form particle swarm on each particle, which attract more particles, gas ball under appropriate conditions, in the center of the hot, dense enough, can generate burning helium and hydrogen producing stars. Born in the right as the stars in the Eagle nebula.
The formation and early history of the earth
4.6 billion years ago, the primitive earth, dead frozen world, through three effect to gain energy and began to evolution and development. • 1 ° impact effect: interstellar dust high-speed collision, the kinetic energy to heat energy • 2 ° compression effect: interstellar dust landing on the surface, increase external quality, internal squeezed, compression can be converted into heat energy. • 3 ° radioactive decay effect: radioactive elements in the interior of the earth (uranium, thorium, rubidium, etc.). Release huge energy • Evolution results: composition of the molten iron flows to the center of the earth, the formation of iron core, it is a rocky composition form structures (silicate).
真核生物上游刺激因子家族的分子进化

第 3 3卷 第 4期
21 0 0年 1 2月
Vol 33 N o 4 _ .
De . c 2 O 0l
文 章 编 号 : 0 01 3 ( O 0)40 8 一 6 1 0 — 7 5 2 l O — 4 4O
真核生物上游刺激因子家族的分子进化
李 莹
( 阳 职 业技 术 学 院 化 T系 , 宁 辽 阳 1 1 0 ) 辽 辽 1 0 4
摘 要 : 基 酸序 列 和基 因 结构 的系 统 发 育分 析 表 明 , 游 刺 激 因 子 家族 uf 氨 上 s l和 U 基 因 的 产 生 是 基 因 复 制 的 结 S
果 , 时 , s l和 uJ 同 uf s2的 内含 子 位 置 以及 插 入 相位 分 析 揭 示 在 不 同 进 化 地 位 的 物 种 中 , 独 立 地 发 生 内含 子 的 插 入 可 或删除, 因此 , 似 同源 的 内含 子不 一 定 是 同源 的 , 说 明在 以 内含 子 为基 础 进 行 同源 性 分 析 时 应谨 慎 . 低 等 动 物相 看 这 与
而本 研 究 发 现 , 该结 构 域 是新 近 插 入 到 该 蛋 自家 族 中 的.
自
关 键词 : 游 刺 激 因子 ; 因复 制 ; 显 子一 上 基 外 内含子 结构 ; 选择 性 剪 接 中 图分 类 号 : 4 . Q3 3 1 文 献标 识 码 : A
作者姓名:卢滇楠
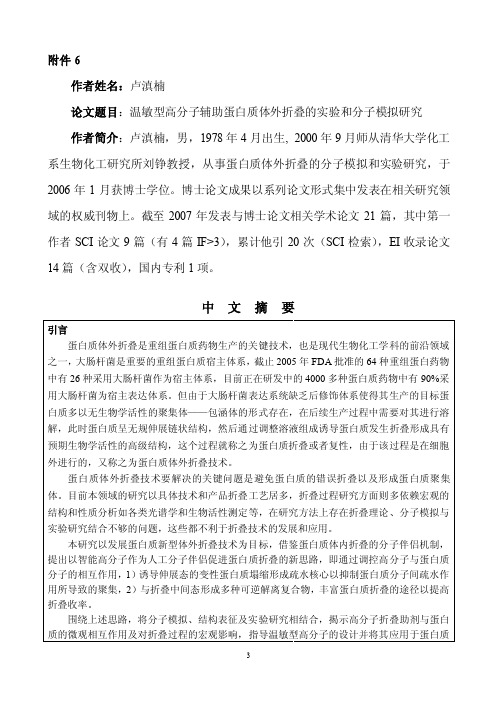
附件6作者姓名:卢滇楠论文题目:温敏型高分子辅助蛋白质体外折叠的实验和分子模拟研究作者简介:卢滇楠,男,1978年4月出生, 2000年9月师从清华大学化工系生物化工研究所刘铮教授,从事蛋白质体外折叠的分子模拟和实验研究,于2006年1月获博士学位。
博士论文成果以系列论文形式集中发表在相关研究领域的权威刊物上。
截至2007年发表与博士论文相关学术论文21篇,其中第一作者SCI论文9篇(有4篇IF>3),累计他引20次(SCI检索),EI收录论文14篇(含双收),国内专利1项。
中文摘要引言蛋白质体外折叠是重组蛋白质药物生产的关键技术,也是现代生物化工学科的前沿领域之一,大肠杆菌是重要的重组蛋白质宿主体系,截止2005年FDA批准的64种重组蛋白药物中有26种采用大肠杆菌作为宿主体系,目前正在研发中的4000多种蛋白质药物中有90%采用大肠杆菌为宿主表达体系。
但由于大肠杆菌表达系统缺乏后修饰体系使得其生产的目标蛋白质多以无生物学活性的聚集体——包涵体的形式存在,在后续生产过程中需要对其进行溶解,此时蛋白质呈无规伸展链状结构,然后通过调整溶液组成诱导蛋白质发生折叠形成具有预期生物学活性的高级结构,这个过程就称之为蛋白质折叠或者复性,由于该过程是在细胞外进行的,又称之为蛋白质体外折叠技术。
蛋白质体外折叠技术要解决的关键问题是避免蛋白质的错误折叠以及形成蛋白质聚集体。
目前本领域的研究以具体技术和产品折叠工艺居多,折叠过程研究方面则多依赖宏观的结构和性质分析如各类光谱学和生物活性测定等,在研究方法上存在折叠理论、分子模拟与实验研究结合不够的问题,这些都不利于折叠技术的发展和应用。
本研究以发展蛋白质新型体外折叠技术为目标,借鉴蛋白质体内折叠的分子伴侣机制,提出以智能高分子作为人工分子伴侣促进蛋白质折叠的新思路,即通过调控高分子与蛋白质分子的相互作用,1)诱导伸展态的变性蛋白质塌缩形成疏水核心以抑制蛋白质分子间疏水作用所导致的聚集,2)与折叠中间态形成多种可逆解离复合物,丰富蛋白质折叠的途径以提高折叠收率。
雷公藤内酯醇对阿尔茨海默病模型大鼠海马突触素表达及突触超微结构的影响
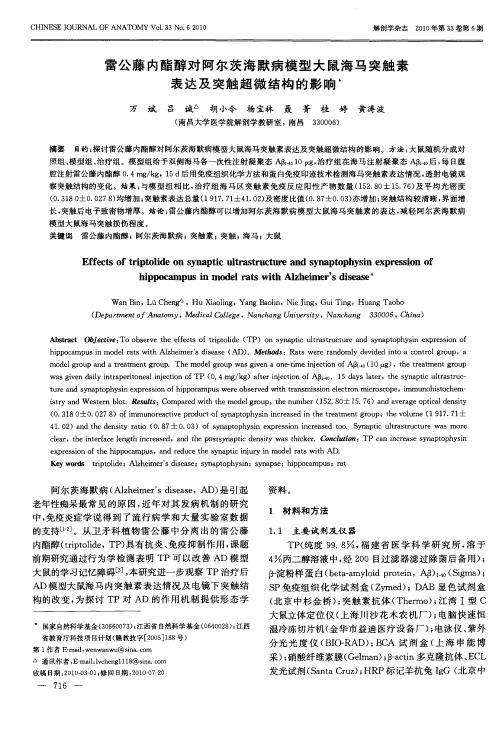
( . 1 土 0 0 78 o O 3 8O . 2 ) fi mmu o eciep o u to y a tp y i n r ae n t ete t n r u ;tev lme( 1 . 1 n ra t r d c fs n p o h sn ice sd i h rame tg o p h ou v 19 7 7 ±
( 南昌大学医学院解剖 学教研室 ,南 昌 30 0 ) 3 0 6
摘要 目的: 探讨雷公藤 内酯醇对 阿尔茨海默病模型大 鼠海马突触素表达及 突触超微 结构 的影 响。方法 : 鼠随机 分成对 大
照组、 模型组 、 治疗组 。模 型组给予 双侧海 马各一次性注射凝 聚态 A 。 0 , 1 g 治疗 组在海 马注射凝 聚态 A 。 , 日腹 后 每
腔注射雷公藤 内酯醇 0 4rg k ,1 . / g 5 a d后用免疫组织化学方法和蛋白免疫印迹技 术检 测海 马突触 素表 达情况 , 透射 电镜观 察 突触结构 的变化 。结果 : 与模 型组 相 比, 治疗 组海 马 区突触 素免疫 反应 阳性 产物 数量 ( 5 . 0 5 7 ) 1 28 士1 .6 及平 均光 密度 ( . 1 ±0 078 均增加 ; O38 O . 2 ) 突触素表达总量 ( 1 . 1 -1 0 ) 1 7 7 44 . 2及密度 比值 (. 7 . 3 亦增加 ; 9 O 8 ±0 0 ) 突触结构较清 晰 , 界面增 长, 突触后 电子致 密物增厚 。结论 : 雷公藤 内酯醇 可以增加 阿尔 茨海默病 模型大 鼠海马突触 素 的表 达 , 轻阿尔茨 海默病 减 模 型大 鼠海马突触损伤程度 。 关键词 雷公藤 内酯醇 ;阿尔茨海 默病 ; 突触素 ; 突触 ; 马 ; 鼠 海 大
circRNA_09505介导M2型巨噬细胞极化失衡在强直性脊柱炎模型中的作用
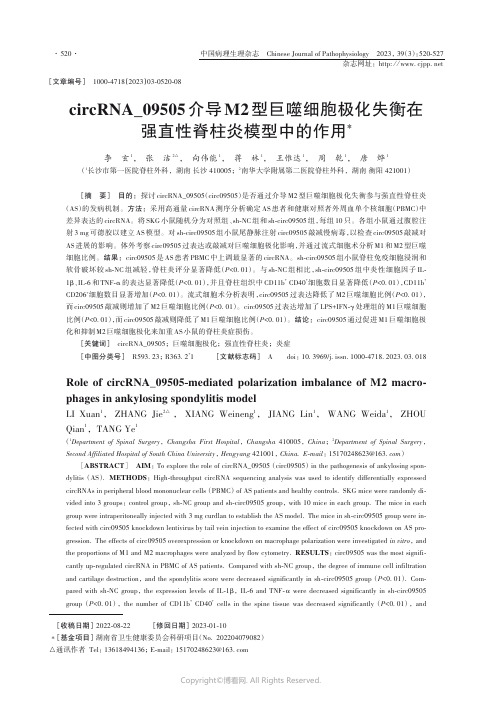
circRNA_09505介导M2型巨噬细胞极化失衡在强直性脊柱炎模型中的作用*李玄1, 张洁2△, 向伟能1, 蒋林1, 王惟达1, 周乾1, 唐烨1(1长沙市第一医院脊柱外科,湖南 长沙 410005;2南华大学附属第二医院脊柱外科,湖南 衡阳 421001)[摘要] 目的:探讨circRNA_09505(circ09505)是否通过介导M2型巨噬细胞极化失衡参与强直性脊柱炎(AS )的发病机制。
方法:采用高通量circRNA 测序分析确定AS 患者和健康对照者外周血单个核细胞(PBMC )中差异表达的circRNA 。
将SKG 小鼠随机分为对照组、sh -NC 组和sh -circ09505组,每组10只。
各组小鼠通过腹腔注射3 mg 可德胶以建立AS 模型。
对sh -circ09505组小鼠尾静脉注射circ09505敲减慢病毒,以检查circ09505敲减对AS 进展的影响。
体外考察circ09505过表达或敲减对巨噬细胞极化影响,并通过流式细胞术分析M1和M2型巨噬细胞比例。
结果:circ09505是AS 患者PBMC 中上调最显著的circRNA 。
sh -circ09505组小鼠脊柱免疫细胞浸润和软骨破坏较sh -NC 组减轻,脊柱炎评分显著降低(P <0.01)。
与sh -NC 组相比,sh -circ09505组中炎性细胞因子IL -1β、IL -6和TNF -α的表达显著降低(P <0.01),并且脊柱组织中CD11b + CD40+细胞数目显著降低(P <0.01),CD11b + CD206+细胞数目显著增加(P <0.01)。
流式细胞术分析表明,circ09505过表达降低了M2巨噬细胞比例(P <0.01),而circ09505敲减则增加了M2巨噬细胞比例(P <0.01)。
circ09505过表达增加了LPS+IFN -γ处理组的M1巨噬细胞比例(P <0.01),而circ09505敲减则降低了M1巨噬细胞比例(P <0.01)。
单细胞核测序和空间转录组学生物学重复
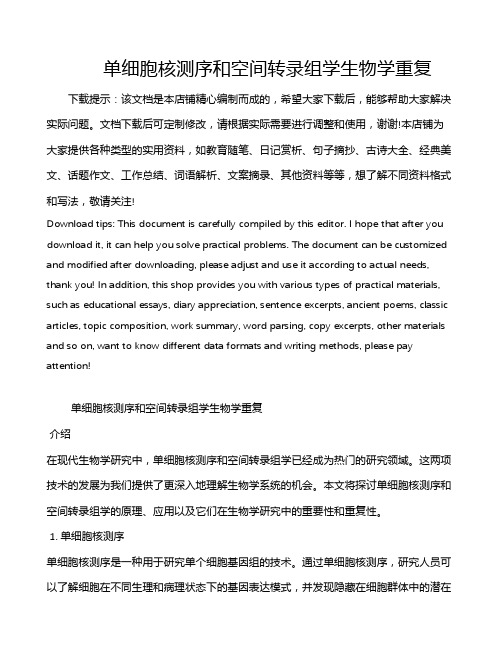
单细胞核测序和空间转录组学生物学重复下载提示:该文档是本店铺精心编制而成的,希望大家下载后,能够帮助大家解决实际问题。
文档下载后可定制修改,请根据实际需要进行调整和使用,谢谢!本店铺为大家提供各种类型的实用资料,如教育随笔、日记赏析、句子摘抄、古诗大全、经典美文、话题作文、工作总结、词语解析、文案摘录、其他资料等等,想了解不同资料格式和写法,敬请关注!Download tips: This document is carefully compiled by this editor. I hope that after you download it, it can help you solve practical problems. The document can be customized and modified after downloading, please adjust and use it according to actual needs, thank you! In addition, this shop provides you with various types of practical materials, such as educational essays, diary appreciation, sentence excerpts, ancient poems, classic articles, topic composition, work summary, word parsing, copy excerpts, other materials and so on, want to know different data formats and writing methods, please pay attention!单细胞核测序和空间转录组学生物学重复介绍在现代生物学研究中,单细胞核测序和空间转录组学已经成为热门的研究领域。
- 1、下载文档前请自行甄别文档内容的完整性,平台不提供额外的编辑、内容补充、找答案等附加服务。
- 2、"仅部分预览"的文档,不可在线预览部分如存在完整性等问题,可反馈申请退款(可完整预览的文档不适用该条件!)。
- 3、如文档侵犯您的权益,请联系客服反馈,我们会尽快为您处理(人工客服工作时间:9:00-18:30)。
a r X i v :0707.2187v 1 [a s t r o -p h ] 15 J u l 2007Nucleosynthesis in Core-Collapse Supernovae and GRB–Metal-Poor Star Connection K.Nomoto ∗,N.Tominaga ∗,M.Tanaka ∗,K.Maeda †and H.Umeda ∗∗Department of Astronomy,University of Tokyo,Bunkyo-ku,Tokyo 113-0033,Japan †Max-Planck-Institut für Astrophysik,85741Garching,Germany Abstract.We review the nucleosynthesis yields of core-collapse supernovae (SNe)for various stellar masses,explosion energies,and parison with the abundance patterns of metal-poor stars provides excellent opportunities to test the explosion models and their nucleosynthesis.We show that the abundance patterns of extremely metal-poor (EMP)stars,e.g.,the excess of C,Co,Zn relative to Fe,are in better agreement with the yields of hyper-energetic explosions (Hypernovae,HNe)rather than normal supernovae.We note that the variation of the abundance patterns of EMP stars are related to the diversity of the Supernova-GRB connection.We summarize the diverse properties of (1)GRB-SNe,(2)Non-GRB HNe/SNe,(3)XRF-SN,and (4)Non-SN GRB.In particular,the Non-SN GRBs (dark hypernovae)have been predicted in order to explain the origin of C-rich EMP stars.We show that these variations and the connection can be modeled in a unified manner with the explosions induced by relativistic jets.Finally,we examine whether the most luminous supernova 2006gy can be consistently explained with the pair-instability supernova model.Keywords:gamma rays:bursts —nuclear reactions,nucleosynthesis,abundances —stars:abun-dances —stars:Population II —supernovae:general PACS:26.20.+f,26.30.+k,26.50.+x,,97.60.Bw To appear in "Supernova 1987A:20Years After:Supernovae and Gamma-Ray Bursters",eds.S.Immler,K.Weiler,&R.McCray (American Institute of Physics)(2007)INTRODUCTION Massive stars in the range of 8to ∼130M ⊙undergo core-collapse at the end of their evolution and become Type II and Ib/c supernovae (SNe)unless the entire star collapsesinto a black hole with no mass ejection [e.g.,4,33,23].The explosion energies of core-collapse supernovae are fundamentally important quantities,and an estimate of E ∼1×1051ergs has often been used in calculating nucleosynthesis and the impact on the interstellar medium.(In the present paper,we use the explosion energy E for the final kinetic energy of explosion,and E 51=E /1051erg.)A good example is SN1987A in the Large Magellanic Cloud,whose energy is estimated to be E 51=1.0−1.5from its early light curve [e.g.,4,55].One of the most interesting recent developments in the study of supernovae is the dis-covery of some very energetic supernovae,whose kinetic energy (KE)exceeds 1052erg,more than 10times the KE of normal core-collapse SNe.The most luminous and power-ful of these objects,the Type Ic supernova (SN Ic)1998bw,was linked to the gamma-rayparison between the abundance pattern of VMP stars[11](filled circles with error bars)and the IMF integrated yield of Pop III SNe from10M⊙to50M⊙[75]burst GRB980425[25],thus establishing for thefirst time a connection between long-duration gamma-ray bursts(GRBs)and the well-studied phenomenon of core-collapse SNe[83].However,SN1998bw was exceptional for a SN Ic:it was as luminous at peak as a SN Ia,indicating that it synthesized∼0.5M⊙of56Ni,and its KE was estimated at E51∼30[35].In the present paper,we use the term’Hypernova(HN)’to describe such a hyper-energetic supernova with E∼>1052ergs without specifying the explosion mechanism [57].Following SN1998bw,other“hypernovae”of Type Ic have been discovered or recognized[59].Nucleosynthesis features in such hyper-energetic(and hyper-aspherical)supernovae must show some important differences from normal supernova explosions.This might be related to the unpredicted abundance patterns observed in the extremely metal-poor (EMP)halo stars[e.g.,32,6].This approach leads to identifying the First Stars in the Universe,i.e.,metal-free,Population III(Pop III)stars which were born in a primordial hydrogen-helium gas cloud.This is one of the important challenges of the current astronomy.ABUNDANCE PATTERS OF METAL-POOR STARSWe have calculated the nucleosynthesis yields for various stellar masses,explosion energies,and metallicities[61,39,75].From the light curve and spectrafitting of individual supernova,the relations between the mass of the progenitor,explosion energy, and produced56Ni mass have been obtained.The enrichment by a single SN can dominate the preexisting metal contents in the early universe.Therefore,the comparison between the SN model and the abundance patterns of EMP stars can provide a new way tofind out the individual SN nucleosyn-thesis.FIGURE3.Averaged elemental abundances of stars with[Fe/H]=−3.7[11]compared with the hypernova yield(20M⊙,E51=10).model of15M⊙and E51=1(Fig.2)[60,75].In the normal SN model(Fig.2),the mass-cut is determined to eject Fe of mass0.14 M⊙).Then the yields are in reasonable agreements with the observations for[(Na,Mg, Si)/Fe],but give too small[(Mn,Co,Ni,Zn)/Fe]and too large[(Ca,Cr)/Fe].In the HN model(Fig.3),these ratios are in much better agreement with observations. The ratios of Co/Fe and Zn/Fe are larger in higher energy explosions since both Co and Zn are synthesized in complete Si burning at high temperature region(see the next subsection).To account for the observations,materials synthesized in a deeper complete Si-burning region should be ejected,but the amount of Fe should be small.This is realized in the mixing-fallback models[78,79].SUPERNOV A–GAMMA-RAY BURST CONNECTIONWe have shown that nucleosynthesis in HNe is in better agreement with the abundance pattern of EMP stars.Thus it would be useful to examine the GRB-SN connection in relation to the GRB-First Star connection.GRBs at sufficiently close distances(z<0.2)have been found to be ac-companied by luminous core-collapse SNe Ic(GRB980425/SN1998bw[25]; GRB030329/SN2003dh[68,34];GRB031203/SN2003lw[43]).Such GRB-SN connection is now revealing quite a large diversity as follows.(1)GRB-SNe:The three SNe Ic associated with the above GRBs have similar prop-erties;showing broader lines than normal SNe Ic(Fig.4:so-called broad-lined SNe [83,50]).These three GRB-SNe have been all found to be Hypernovae(HNe),i.e.,very energetic supernovae,whose isotropic kinetic energy(KE)exceeds1052erg,about10 times the KE of normal core-collapse SNe[35,57,59].(2)Non-GRB HNe/SNe:These SNe show broad line features but are not associated with GRBs(SN1997ef[36];SN2002ap[44];SN2003jd[45]).These are either lessgive the estimates of M ej and E.The timescale of the LC around maximum brightness reflects the timescale for optical photons to diffuse[3].For larger M ej and smaller E, the LC peaks later and the LC width becomes broader because it is more difficult for photons to escape.From the synthetic spectra and light curves,it was interpreted as the explosion of a massive star,with E51∼30and M ej∼10M⊙[35].Also the very high luminosity of SN1998bw indicates that a large amount of56Ni(∼0.5M⊙)was synthesized in the explosion.The ejected56Ni mass is estimated to be M(56Ni)∼0.3−0.7M⊙(e.g.,[46])which is 4to10times larger than typical SNe Ic(M(56Ni)∼0.07M⊙[54]).The other two GRB-SNe,2003dh and2003lw,are also characterized by the very broad line features and the very high luminosity.M ej and E are estimated from synthetic spectra and light curves and summarized in Figure7[53,15,46].It is clearly seen that GRB-SNe are the explosions of massive progenitor stars(with the main sequence mass of M ms∼35−50M⊙),have large explosion kinetic energies(E51∼30−50),synthesized large amounts of56Ni(∼0.3−0.5M⊙).These GRB-associated HNe(GRB-HNe)are suggested to be the outcome of very energetic black hole(BH)forming explosions of massive stars(e.g.,[35]).Non-GRB HypernovaeThese HNe show spectral features similar to those of GRB-SNe but are not known to have been accompanied by a GRB.The estimated M ej and E,obtained from synthetic light curves and spectra,show that there is a tendency for non-GRB HNe to have smaller M ej and E,and lower luminosities as summarized in Figures7and8.SN1997ef is found to be the HN class of energetic explosion,although E/M ej is a factor3smaller than GRB-SNe.It is not clear whether SN1997ef is not associated with GRB because of this smaller E/M ej or it was actually associated with the candidate GRB 971115.SN2002ap was not associated a GRB and no radio has been observed.It has similar spectral features,but narrower and redder(Fig.4),which was modeled as a smaller energy explosion,with E51∼4and M ej∼3M⊙[44].The early time spectrum of SN2003jd is similar to SN2002ap.Interestingly,its neb-ular spectrum shows a double peak in O-emission lines[45].This has exactly confirmed the theoretical prediction by the asymmetric explosion model[41].In this case,the ori-entation effect might cause the non-detection of a GRB.XRF–SupernovaeGRB060218is the second closest event as ever(∼140Mpc).The GRB was weak [10]and classified as X-Ray Flash(XRF)because of its soft spectrum.The presence of SN2006aj was soon confirmed[64,49].Here we summarize the properties of SN2006aj by comparing with other SNe Ic.SN 2006aj has several features that make it unique.It is less bright than the other GRB/SNe (Fig.4).Its rapid photometric evolution is very similar to that of a dimmer,non-GRB SN 2002ap[44],but it is somewhat faster.Although its spectrum is character-ized by broader absorption lines as in SN 1998bw and other GRB/SN,they are not as broad as those of SN 1998bw,and it is much more similar to that of SN 2002ap (Fig.4).The most interesting property of SN 2006aj is surprisingly weak oxygen lines,much weaker than in Type Ic SNe.By modeling the spectra and the light curve,we derive for SN 2006aj M ej ∼2M ⊙and E 51∼ck of oxygen in the spectra indicates ∼1.3M ⊙of O,and oxygen is still the dominant element.We synthesize the theoretical light curve and find that the best match is achieved with a total 56Ni mass of 0.21M ⊙in which 0.02M ⊙is located above 20,000km s −1(Fig.4).The properties of SN 2006aj (smaller E and smaller M ej )suggest that SN 2006aj is not the same type of event as the other GRB-SNe known thus far.One possibility is that the initial mass of the progenitor star is much smaller than the other GRB-SNe,so that the collapse/explosion generated less energy.If M ms is ∼20−25M ⊙,the star would be at the boundary between collapse to a black hole or to a neutron star.In this mass range,there are indications of a spread in both E and the mass of 56Ni synthesized[29].The fact that a relatively large amount of 56Ni is required in SN 2006aj possibly suggests that the star collapsed only to a neutron star because more core material would be available to synthesize 56Ni in the case.Although the kinetic energy of E 51∼2is larger than the canonical value (1×1051erg,[54])in the mass range of M ms ∼20−25M ⊙,such an energy might be obtained from magnetar-type activity.XRFs may be associated with less massive progenitor stars than those of canonical GRBs,and that the two groups may be differentiated by the formation of a neutron star[52]or a BH.In order for the progenitor star to have been thoroughly stripped of its H and He envelopes,the progenitor may be in a binary system.Non-SN Gamma-Ray BurstsFor recently discovered nearby long-duration GRB 060505(z =0.089,[18])and GRB 060614(z =0.125,[26,18,13,27]),no SN has been detected.Upper limits to brightness of the possible SNe are about 100times fainter than SN 1998bw.These correspond to upper limits to the ejected 56Ni mass of M (56Ni )∼10−3M ⊙.Tominaga et al.[74]calculated the jet-induced explosions (e.g.,[42,51])of the 40M ⊙stars [79,75]by injecting the jets at a radius R ∼900km,corresponding to an enclosed mass of M ∼1.4M ⊙.They investigated the dependence of nucleosynthesis outcome on ˙Edep for a range of ˙E dep ,51≡˙E dep /1051ergss −1=0.3−1500.The diversity of ˙E dep is consistent with the wide range of the observed isotropic equivalent γ-ray energies and timescales of GRBs ([1]and references therein).Variations of activities of the central engines,possibly corresponding to different rotational velocities or magnetic fields,may well produce the variation of ˙Edep .FIGURE5.Top:the ejected56Ni mass(red:explosive nucleosynthesis products,blue:the jet contribu-tion)as a function of the energy deposition rate.The background color shows the corresponding SNe(red: GRB-HNe,yellow:sub-luminous SNe,blue:faint SNe,green:GRBs060505and060614).Vertical lines divide the resulting SNe according to their brightness.Bottom:the dependence of abundance ratio[C/Fe] on the energy deposition rate.The background color shows the corresponding metal-poor stars(yellow: EMP,red:CEMP,blue:HMP stars).NUCLEOSYNTHESIS IN JET-INDUCED EXPLOSIONS Nucleosynthetic properties found in the above diversity are connected to the variation of the abundance patterns of extremely-metal-poor stars,such as the excess of C,Co,Zn relative to Fe.Such a connection are modeled in a unified manner with the jet-induced explosion model.We have computed hydrodynamics and nucleosynthesis for the explosions induced by relativistic jets.We have shown that(1)the explosions with large energy deposition rate,˙Edep,are observed as GRB-HNe and their yields can explain the abundances of normal EMP stars,and(2)the explosions with small˙E dep are observed as GRBs without bright SNe and can be responsible for the formation of the CEMP and the HMP stars.We thus propose that GRB-HNe and GRBs without bright SNe belong to a continuous series of BH-forming massive stellar deaths with the relativistic jets of different˙E dep.-10123-101234551015202530Z[X /F e ]FIGURE 6.A comparison of the abundance patterns of metal-poor stars and of our models.Top :typical EMP (red dots ,[11])and CEMP (blue triangles ,CS 22949–37,[16])stars and models with ˙E dep ,51=120(solid line )and =3.0(dashed line ).Bottom :HMP stars:HE 1327–2326,(red dots ,e.g.,[20]),and HE 0107–5240,(blue triangles ,[12,8])and models with ˙Edep ,51=1.5(solid line )and =0.5(dashed line ).Diversity of 56Ni MassThe top panel of Figure 5shows the dependence of the ejected M (56Ni )on the energy deposition rate ˙Edep .For lower ˙E dep ,smaller M (56Ni )is synthesized in explosive nucleosynthesis because of lower post-shock densities and temperatures (e.g.,[42,51]).If ˙E dep ,51∼>3,the jet injection is initiated near the bottom of the C+O layer,leading to the synthesis of M (56Ni )∼>10−3M ⊙.If ˙E dep ,51<3,on the other hand,the jet injection is delayed and initiated near the surface of the C+O core;then the ejected 56Ni is as small as M (56Ni )<10−3M ⊙.56Ni contained in the relativistic jets is only M (56Ni )∼10−6−10−4M ⊙because the total mass of the jets is M jet ∼10−4M ⊙in our model with Γjet =100and E dep =1.5×1052ergs.Thus the 56Ni production in the jets dominates over explosive nucleosynthesis in the stellar mantle only for ˙E dep ,51<1.5in the present model.For high energy deposition rates (˙E dep ,51∼>60),the explosions synthesize large M (56Ni )(∼>0.1M ⊙)being consistent with GRB-HNe.The remnant mass was M start rem ∼1.5M ⊙when the jet injection was started,but it grows as material is accreted from the equatorial plane.The final BH masses range from M BH =10.8M ⊙for ˙Edep ,51=60toM BH =5.5M ⊙for ˙E dep ,51=1500,which are consistent with the observed masses of stellar-mass BHs [5].The model with ˙Edep ,51=300synthesizes M (56Ni )∼0.4M ⊙and the final mass of BH left after the explosion is M BH =6.4M ⊙.For low energy deposition rates (˙Edep ,51<3),the ejected 56Ni masses (M (56Ni )<10−3M ⊙)are smaller than the upper limits for GRBs 060505and 060614.The final BH mass is larger for smaller ˙Edep .While the material ejected along the jet-direction involves those from the C+O core,the material along the equatorial plane fall back.If the explosion is viewed from the jet direction,we would observe GRB without SN re-brightening.This may be the situation for GRBs 060505and 060614.In particular,for ˙Edep ,51<1.5,56Ni cannot be synthesized explosively and the jet component of the Fe-peak elements dominates the total yields (Fig.6).The models eject very little M (56Ni )(∼10−6M ⊙).For intermediate energy deposition rates (3∼<˙E dep ,51<60),the explosions eject10−3M ⊙∼<M (56Ni )<0.1M ⊙and the final BH masses are 10.8M ⊙∼<M BH <15.1M ⊙.The resulting SN is faint (M (56Ni )<0.01M ⊙)or sub-luminous (0.01M ⊙∼<M (56Ni )<0.1M ⊙).Nearby GRBs with faint or sub-luminous SNe have not been observed.This may be because they do not occur intrinsically in our neighborhood or because the number of observed cases is still too small.In the latter case,further observations may detect GRBs with a faint or sub-luminous SN.Abundance Patterns of C-rich Metal-Poor StarsThe bottom panel of Figure 5shows the dependence of the abundance ratio [C/Fe]on ˙Edep .Lower ˙E dep yields larger M BH and thus larger [C/Fe],because the infall decreases the amount of inner core material (Fe)relative to that of outer material (C)(see also [42]).As in the case of M (56Ni ),[C/Fe]changes dramatically at ˙Edep ,51∼3.The abundance patterns of the EMP stars are good indicators of SN nucleosynthesis because the Galaxy was effectively unmixed at [Fe/H]<−3(e.g.,[76]).They are classified into three groups according to [C/Fe]:(1)[C/Fe]∼0,normal EMP stars (−4<[Fe/H]<−3,e.g.,[11]);(2)[C/Fe]∼>+1,Carbon-enhanced EMP (CEMP)stars (−4<[Fe/H]<−3,e.g.,CS 22949–37[16]);(3)[C/Fe]∼+4,hyper metal-poor (HMP)stars ([Fe/H]<−5,e.g.,HE 0107–5240[12,8];HE 1327–2326[20]).Figure 6shows that the general abundance patterns of the normal EMP stars,the CEMP star CS 22949–37,and the HMP stars HE 0107–5240and HE 1327–2326are reproduced by models with ˙Edep ,51=120,3.0,1.5,and 0.5,respectively.The model for the normal EMP stars ejects M (56Ni )∼0.2M ⊙,i.e.a factor of 2less than SN 1998bw.On the other hand,the models for the CEMP and the HMP stars eject M (56Ni )∼8×10−4M ⊙and 4×10−6M ⊙,respectively,which are always smaller than the upper limits for GRBs 060505and 060614.The N/C ratio in the models for CS 22949–37and HE 1327–2326is enhanced by partial mixing between the He and H layers during presupernova evolution [37].FIGURE7.The kinetic explosion energy E as a function of the main sequence mass M of the progenitors for several supernovae/hypernovae.SNe that are observed to show broad-line features are indicated.Hypernovae are the SNe with E51>10.FIGURE8.The ejected56Ni mass as a function of the main sequence mass M of the progenitors for several supernovae/hypernovae.DISCUSSIONThe large Zn and Co abundances and the small Mn and Cr abundances observed in very metal-poor stars can better be explained by introducing HNe.This would imply that HNe have made significant contributions to the early Galactic chemical evolution,In theoretical models,some element ratios,such as(K,Sc,Ti,V)/Fe,are too small, while some ratios such as Cr/Fe are too large compared with the observed abundance ratios[11].Underproduction of Sc and K may require significantly higher entropyenvironment for nucleosynthesis,e.g.,the“low density”progenitor models for K,Sc, and Ti[79,24].GRBs would have possible nucleosynthesis site,such as accretion disks around the black hole[65].Neutrino processes in the deepest layers of SN ejecta and a possible accretion disk around a black hole would open a new window for SN nucleosynthesis[65,21,22,80].GRB,Hypernovae,and Broad-LinesFigures7and8summarize the properties of core-collapse SNe as a function of the main-sequence mass M ms of the progenitor star[58].The broad-line SNe include both GRB-SNe and Non-GRB SNe.(1)GRB vs.Non-GRB:Three GRB-SNe are all similar Hypernovae(i.e.,E51∼>10. Thus E could be closely related to the formation of GRBs.SN1997ef seems to be a marginal case.E/M ej could be more important because SN1997ef has significantly smaller E/M ej than GRB-SNe.(2)Broad-Line features:The mass contained at v>30,000km s−1(or even higher boundary velocity)might be critical in forming the broad-line features,although further modeling is required to clarify this point[61].Black Holes vs.Neutron StarsThe discovery of XRF060218/SN2006aj and their properties extend the GRB-HN connection to XRFs and to the HN progenitor mass as low as∼20M⊙.The XRF060218 may be driven by a neutron star rather than a black hole.Thefinal fate of20-25M⊙stars show interesting variety.Even normal SN Ib2005bf is very different from previously known SNe/HNe[73,19].This mass range corresponds to the transition from the NS formation to the BH formation.The NSs from this mass range could be much more active than those from lower mass range because of possibly much larger NS masses(near the maximum mass)or possibly large magneticfield (magnetar).XRFs and GRBs from the mass range of20-25M⊙might form a different population.Hypernovae of Type II and Type Ib?Suppose that smaller losses of mass and angular momentum from low metallicity massive stars lead to the formation of more rapidly rotating NSs or BHs and thus more energetic explosions.Then we predict the existence of Type Ib and Type II HNe[30]. So far all observed HNe are of Type Ic.However,most of SNe Ic are suggested to have some He[9].If even the small amount of radioactive56Ni is mixed in the He layer, the He feature should be seen[40,56].For HNe,the upper mass limit of He has been estimated to be∼2M⊙[44]for the case of no He mixing.If He features would beA b s o l u t e m a g n i t u d e s Days since the explosion date-22-21-20-19-18-17M a g n i t u d e s Days FIGURE 9.(Left)Comparison between LCs of SNe 1998bw (open square [25]),2002ic (open circle [28]),and 2006gy (fulled triangle [66]).(Right)Comparison between R-and r-band LCs of SN 2006gy[63,66]and synthetic LCs for a model with M ej =53M ⊙,E 51=64,and M (56Ni )=15M ⊙and a PISNmodel with M ej =166M ⊙,E 51=65,and M (56Ni )=15M ⊙.seen in future HN observations,it would provide an important constraint on the models,especially,the fully mixed WR models [85,84,48].MOST LUMINOUS SUPERNOV A 2006GYRecently,several extremely luminous supernovae have been discovered,which includesSNe IIa 1997cy,2002ic,and SN Ic 1999as (Fig.9:left).The energy sources of theseSN light curves (LC)are closely related to SN nucleosynthesis.The post-maximumlight curves (LCs)of SNe IIa are powered by circumstellar interaction [14],althoughwhether the underlying SNe are Ia or Ic is under debate [7].SN 2006gy is the most luminous SN [63,66].It shows hydrogen emission featureslike SNe IIn and IIa to indicate circumstellar interaction.However,the X-ray luminosityis too low to explain the observed optical luminosity [66].This suggests that the LC ofSN 2006gy may be powered by the decays of 56Ni →56Co →56Fe,and the requiredlarge 56Ni mass,M (56Ni ),suggests that SN 2006gy could be a pair-instability supernova(PISN)[78,31]rather than a core-collapse [63,66].We have constructed the LC model of the PISN model as shown by the solid linewith 166M in Fig.9(right).Here we have calculated the evolution from the main-sequence with extensive mass loss to expose a C+O core.The star undergoes PISNwith M ej =166M ⊙,E 51=65,and M (56Ni )=15M ⊙.Here the explosion energy is not afree parameter (like a core-collapse model)but obtained from the nuclear energy releaseassociated with the production of M (56Ni ).Such a large M ej ,which is necessary toproduce large enough M (56Ni ),is too large for the LC.The model LC evolves muchslower than the observed LC of SN 2006gy (red points in Fig.9:right).In order to reproduce the LC of SN 2006gy,we artificially reduce the ejected massof the above exploding model down to M ej =53M ⊙,keeping E 51=64,and M (56Ni )=15M ⊙(the 53M line in Fig.9).In other words,the progenitor should have lost muchmore mass than the actual model,yet produced a large enough amount of 56Ni.These results imply whether SN2006gy is a core-collapse SN or a PISN is not clear yet.The PISN model can produce enough M(56Ni)but M ej might be too large.The core-collapse models currently available could produce too small M(56Ni).Also,the mass loss from such a massive star with solar metallicity would be too large to keep hydrogen-rich circumstellar matter as observed like SNe IIn and IIa[63]. Stellar merging in a close binary system or a dense star cluster might be necessary for the formation and evolution of very massive stars.Further study is clearly necessary to understand the evolutionary origin and nucleosynthesis of SN2006gy.REFERENCES1.Amati,L.,Della Valle,M.,Frontera,F.,et al.,A&A463(2007)9132.Argast,D.,Samland,M.,Gerhard,O.E.,&Thielemann,F.-K.,A&A356(2000)8733.Arnett,W.D.ApJ253(1982)7854.Arnett,W.D.,Supernovae and Nucleosynthesis(Princeton Univ.Press)(1996)5.Bailyn,C.D.,Jain,R.K.,Coppi,P.,&Orosz,J.A.,ApJ499(1998)3676.Beers,T.,&Christlieb,N.,ARA&A43(2005)5317.Benetti,S.,et al.,ApJ653(2006)L1298.Bessell,M.S.,&Christlieb,N.,in IAU Symp228,From Lithium to Uranium,ed.V.Hill et al.(Cambridge Univ.Press)(2005)2379.Branch,D.,Jeffery,D.J.,Timothy,R.Y.,&Baron.E.,PASP118(2006)79110.Campana,S.,et al.,Nature442(2006)100811.Cayrel,R.,et al.,A&A416(2004)111712.Christlieb,N.,et al.,Nature419(2002)90413.Della Valle,M.,et al.,2006,Nature444(2006)105014.Deng,J.,Kawabata,K.S.,Ohyama,Y.,Nomoto,K.,et al.,ApJ605(2004)L3715.Deng,J.,Tominaga,N.,Mazzali,P.A.,Maeda,K.,&Nomoto,K.,ApJ624(2005)89816.Depagne,E.,et al.,A&A,390(2002)18717.Fynbo,J.P.U.,et al.,ApJ609(2004)96218.Fynbo,J.P.U.,et al.,Nature444(2006)104719.Folatelli,G.,et al.,ApJ641(2006)103920.Frebel,A.,et al.,Nature434(2005)87121.Fröhlich,C.,Hauser,P.,Liebendörfer,M.,Martínez-Pinedo,G.,Thielemann,F.-K.,Bravo,E.,Zinner,N.T.,Hix,W.R.,Langanke,K.,Mezzacappa,A.,&Nomoto,K.,ApJ637(2006)41522.Fröhlich,C.,Martínez-Pinedo,G.,Liebendörfer,M.,et al.,Phys Rev Let96(2006)14250223.Fryer,C.L.(ed.)Stellar Collapse(Astrophysics and Space Science Library:Kluwer)(2004)24.Fryer,C.L.,Young,P.A.,&Hungerford,A.L.,ApJ650(2006)102825.Galama,T.,et al.,Nature395(1998)67026.Gal-Yam,A.,et al.,Nature444(2006)105327.Gehrels,N.,et al.,Nature444(2006)104428.Hamuy,M.,et al.,Nature424(2003)65129.Hamuy,M.,ApJ582(2003)90530.Hamuy,M.,Contreras,C.,Gonzalez,S.,Krzeminski,W.,IAU Circ.(2005)852031.Heger,A.,&Woosley,S.E.,ApJ567(2002)53232.Hill,V.,François,P.,&Primas,F.(ed.),IAU Symp228,From Lithium to Uranium:Elemental Tracersof Early Cosmic Evolution(Cambridge Univ.Press)(2005)33.Hillebrandt,W.,&Leibundgut,B.(ed.),From Twilight to Highlight:The Physics of Supernovae(Springer)(2003)34.Hjorth,J.,et al.,Nature423(2003)84735.Iwamoto,K.,Mazzali,P.A.,Nomoto,K.,et al.,Nature395(1998)67236.Iwamoto,K.,Nakamura,T.,Nomoto,K.,et al.,ApJ534(2000)66037.Iwamoto,N.,Umeda,H.,Tominaga,N.,Nomoto,K.,&Maeda,K.,Science309(2005)45138.Kawabata,K.,et al.,ApJ580(2002)L3939.Kobayashi,C.,Umeda,H.,Nomoto,K.,Tominaga,N.,&Ohkukbo,T.,ApJ653(2006)114540.Lucy,L.B.,ApJ383(1991)30841.Maeda,K.,Nakamura,T.,Nomoto,K.,Mazzali,P.A.,Patat,F.,&Hachisu,I.ApJ565(2002)40542.Maeda,K.&Nomoto,K.,ApJ598(2003)116343.Malesani,J.,et al.,ApJ609(2006)L544.Mazzali,P.A.,Deng,J.,Maeda,K.,Nomoto,K.,et al.,ApJ572(2002)L6145.Mazzali,P.A.,Kawabata,K.S.,Maeda,K.,Nomoto,K.,et al.,Science308(2005)128446.Mazzali,P.A.,et al.,ApJ645(2006a)132347.Mazzali,P.A.,Deng,J.,Nomoto,K.,et al.,Nature442(2006b)101848.Meynet,G.,&Maeder,A.,A&A464(2007)L1149.Modjaz,M.,et al.ApJ645(2006)L2150.Modjaz,M.,et al.,AJ(2007)submitted(astro-ph/0701246)51.Nagataki,S.,Mizuta,A.,&Sato,K.,ApJ647(2006)125552.Nakamura,T.,Prog.Theor.Phys.100(1998)92153.Nakamura,T.,Mazzali,P.A.,Nomoto,K.,Iwamoto,K.,ApJ550(2001)99154.Nomoto,K.,et al.,Nature371(1994)22755.Nomoto,K.,Shigeyama,T.,Kumagai,S.,Yamaoka,H.,&Suzuki,T.,in Supernovae,Les HoucheSession LIV(1994)ed.S.Bludmann et al.(North-Holland)48956.Nomoto,K.,Iwamoto,K.,&Suzuki,T.Phys.Rep.256(1995)17357.Nomoto,K.,Mazzali,P.A.,Nakamura,T.,et al.,in Supernovae and Gamma Ray Bursts,eds.M.Livioet al.(Cambridge Univ.Press)(2001)144(astro-ph/0003077)58.Nomoto,K.,et al.,in IAU Symp212,A massive Star Odyssey,from Main Sequence to Supernova,eds.V.D.Hucht,et al.(San Francisco:ASP)(2003)395(astro-ph/0209064)59.Nomoto,K.,et al.,in Stellar Collapse,ed.C.L.Fryer(Astrophysics and Space Science:Kluwer)(2004)277(astro-ph/0308136)60.Nomoto,K.,Tominaga,N.,Umeda,H.,&Kobayashi,C.,in IAU Symp228,From Lithium to Uranium,ed.V.Hill et al.(Cambridge Univ.Press)(2005)287(astro-ph/0603433)61.Nomoto,K.,Tominaga,N.,Umeda,H.,Kobayashi,C.,&Maeda,K.,Nuclear Phys A777(2006)424(astro-ph/0605725)62.Nomoto,K.,et al.,Nuovo Cinento121(2007)1207(astro-ph/0702472);the pptfile available from“program”at http://www.merate.mi.astro.it/docM/OAB/Research/SWIFT/sanservolo2006/63.Ofek,E.O.,et al.,ApJ659(2007)L1364.Pian,E.,et al.,Nature442(2006)101165.Pruet,J.,Woosley,S.E.,Buras,R.,Janka,H.-T.,&Hoffman,R.D.,ApJ623(2005)32566.Smith,N.,et al.,ApJ(2007)in press(astro-ph/0612617)67.Soderberg,A.M.,et al.,Nature442(2006)101468.Stanek,K.Z.,et al.,ApJ591(2003)L1769.Thompson,T.A.,&Duncan,R.C.,Mon.Not.Royal Astron.Soc.275(1995)25570.Thompson,T.A.,Chang,P.,&Quataert,E.,ApJ611(2004)38071.Thornton,K.,Gaudlitz,M.,Janka,H.-Th.,&Steinmetz,M.,ApJ500(1998)9572.Tominaga,N.,Deng,J.,Mazzali,P.A.,Maeda,K.,Nomoto,K.,et al.,ApJ612(2004)L10573.Tominaga,N.,Tanaka,M.,Nomoto,K.,et al.,ApJ633(2005)L9774.Tominaga,N.,Maeda,K.,Umeda,H.,Nomoto,K.,Tanaka,et al.,ApJ657(2007)L7775.Tominaga,N.,Umeda,H.,&Nomoto,K.,ApJ660(2007)51676.Tumlinson,J.,ApJ641(2006)177.Umeda,H.Nomoto,K.,&Nakamura,T.,in The First Stars(2000),ed.A.Weiss,T.Abel,&V.Hill(Berlin:Springer),150(astro-ph/9912248)78.Umeda,H.,&Nomoto,K.,ApJ565(2002)38579.Umeda,H.,&Nomoto,K.,ApJ619(2005)42780.Wanajo,S.,ApJ647(2006)132381.Wang,L.,Baade,D.,Höflich,P.,&Wheeler,J.C.,ApJ592(2003)45782.Woosley,S.E.,&Weaver,T.A.,ApJS101(1995)18183.Woosley,S.E.,&Bloom,J.S.,ARA&A44(2006)50784.Woosley,S.E.,&Heger,A.,ApJ637(2006)91485.Yoon,S.-C.,&Langer,N.,A&A443(2006)643。