Effect of titania (TiO2) interfacial coating on mechanical properties
NaTFSI界面修饰对平面TiO2基钙钛矿太阳能电池的影响

年教师科研提升资助计划( ZQN-706) 资助项目 Supported by Joint Funds of The National Natural Science Foundation of China( U1705256) ; National Natural Science Foundation of China(21771066,51972123,61804058) ; Promotion Program for Young and Middle-aged Teacher in Science and Technology Research of Huaqiao University( ZQN-706)
ZOU Yu, LI Zhao, CHEN Heng-hui, LIU Yi-chen, TONG An-ling, YAN Hui-ying, HE Ruo-wei, HUA Guo-xin, ZENG Wei-dong, SUN Wei-hai∗
( Engineering Research Center of Environment-friendly Functional Materials, Ministry of Education, Fujian Key Laboratory of Photoelectric Functional Materials,
Institute of Materials Physical Chemistry, College of Materials Science and Engineering, Huaqiao University, Xiamen 361021, China) ∗Corresponding Author, E-mail: sunweihai@ hqu. edu. cn
等离子体效应增强TiO2-SiO2-Ag复合薄膜的光吸收(英文)
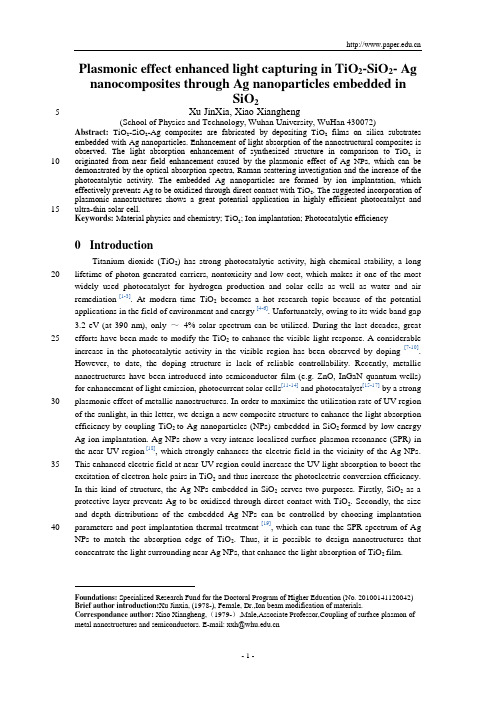
Plasmonic effect enhanced light capturing in TiO 2-SiO 2- Ag nanocomposites through Ag nanoparticles embedded inSiO 2Xu JinXia, Xiao Xiangheng5 (School of Physics and Technology, Wuhan University, WuHan 430072)Foundations: Specialized Research Fund for the Doctoral Program of Higher Education (No. 20100141120042) Brief author introduction:Xu Jinxia, (1978-), Female, Dr.,Ion beam modification of materials.Correspondance author: Xiao Xiangheng,(1979-),Male,Associate Professor,Coupling of surface plasmon of metal nanostructures and semiconductors. E-mail: xxh@Abstract: TiO 2-SiO 2-Ag composites are fabricated by depositing TiO 2 films on silica substrates embedded with Ag nanoparticles. Enhancement of light absorption of the nanostructural composites is observed. The light absorption enhancement of synthesized structure in comparison to TiO 2 is originated from near field enhancement caused by the plasmonic effect of Ag NPs, which can be 10demonstrated by the optical absorption spectra, Raman scattering investigation and the increase of the photocatalytic activity. The embedded Ag nanoparticles are formed by ion implantation, which effectively prevents Ag to be oxidized through direct contact with TiO 2. The suggested incorporation of plasmonic nanostructures shows a great potential application in highly efficient photocatalyst andultra-thin solar cell.15 Keywords: Material physics and chemistry; TiO 2; Ion implantation; Photocatalytic efficiency0 IntroductionTitanium dioxide (TiO 2) has strong photocatalytic activity, high chemical stability, a long lifetime of photon-generated carriers, nontoxicity and low cost, which makes it one of the most 20 widely used photocatalyst for hydrogen production and solar cells as well as water and air remediation [1-3]. At modern time TiO 2 becomes a hot research topic because of the potential applications in the field of environment and energy [4-6]. Unfortunately, owing to its wide band gap3.2 eV (at 390 nm), only ~ 4% solar spectrum can be utilized. During the last decades, great efforts have been made to modify the TiO 2 to enhance the visible light response. A considerable 25 increase in the photocatalytic activity in the visible region has been observed by doping [7-10]. However, to date, the doping structure is lack of reliable controllability. Recently, metallic nanostructures have been introduced into semiconductor film (e.g. ZnO, InGaN quantum wells) for enhancement of light emission, photocurrent solar cells [11-14] and photocatalyst [15-17] by a strong plasmonic effect of metallic nanostructures. In order to maximize the utilization rate of UV region 30 of the sunlight, in this letter, we design a new composite structure to enhance the light absorption efficiency by coupling TiO 2 to Ag nanoparticles (NPs) embedded in SiO 2 formed by low energy Ag ion implantation. Ag NPs show a very intense localized surface plasmon resonance (SPR) in the near-UV region [18], which strongly enhances the electric field in the vicinity of the Ag NPs. This enhanced electric field at near-UV region could increase the UV light absorption to boost the 35 excitation of electron-hole pairs in TiO 2 and thus increase the photoelectric conversion efficiency. In this kind of structure, the Ag NPs embedded in SiO 2 serves two purposes. Firstly, SiO 2 as a protective layer prevents Ag to be oxidized through direct contact with TiO 2. Secondly, the size and depth distributions of the embedded Ag NPs can be controlled by choosing implantation parameters and post-implantation thermal treatment [19], which can tune the SPR spectrum of Ag 40NPs to match the absorption edge of TiO 2. Thus, it is possible to design nanostructures that concentrate the light surrounding near Ag NPs, that enhance the light absorption of TiO 2 film.1 Experimental SectionHigh purity silica slides were implanted by Ag ions at 20, 40 and 60 kV to fluence of 5×1016 ions/cm 2 and at 40 kV to 1×1017 ions/cm 2 using a metal vapor vacuum arc ion source implanter, 45 respectively. The TiO 2-SiO 2-Ag nanostructural composites were obtained by depositing TiO 2 films (100 nm thick) on the surface of the as-implanted silica substrates using direct-current (DC) reactive magnetron sputtering system. For comparison, an un-implanted silica substrate was deposited with TiO 2 film under the same growth condition. Subsequently, all deposited samples were annealed at 500 °C in oxygen gas for 2 hours to obtain anatase phase TiO 2 film. The TiO 2 50covered silica substrates with embedded Ag NPs are named S1-S4 as shown in Table 1. The optical absorption spectra of all the samples were measured using a UV-vis-NIR dual-beam spectrometer (Shimadzu UV 2550) with wavelengths varied from 200 to 800 nm. Raman scattering spectra of all the samples were collected using a micro-Raman system (LabRAM HR800). An Ar laser (488.0 nm) was used as the excitation source, and the laser power was kept55 at 10 mW. The microstructure of the samples was investigated by using a JEOL JEM 2010 (HT) transmission electron microscopes (TEM) operated at 200 kV.Tab. 1 Ag ion implantation parameters for all samplessample Fluence of ion implantation(ions/cm2)Energy of ion implantation(kV)S1 5×1016 20S2 5×1016 40S3 1×1017 40 S4 5×1016 6060 2 Results and DiscussionThe photocatalytic efficiencies of TiO 2 and TiO 2-SiO 2-Ag nanostructural composites with an area of 4 cm 2 were evaluated by measuring the degradation rates of 5 mg/L methylene blue (MB) solution under UV-vis irradiation. A mercury lamp (OSRAM, 250 W with characteristic wavelength at 365 nm) was used as a light source. The TiO 2 and the TiO 2-SiO 2-Ag composites 65 films were placed into 40 mL MB solution with a concentration of 5 mg/L. Before irradiation, the samples were put into 40 mL MB solution for 30 minutes in the darkness to reach absorption equilibrium. The decolorization of MB solution was measured by an UV-vis spectrometer (Shimadzu UV 2550) at the wavelength of 664.0 nm. The absorption spectrum of the MB solution was measured at a time interval of 30 minutes and the total irradiation time was 4 hours. 70 Fig. 1 shows the optical absorption spectra of S1-S4 and the TiO 2 films. The absorption edge around 390 nm belongs to the intrinsic exciton absorption of TiO 2 [20]. The obvious absorption peaks at about 419-433 nm can be attributed to the SPR of Ag NPs formed by Ag ion implantation[21]. As seen the SPR of Ag NPs is close to the exciton edge (around 390 nm) of anatase TiO 2. Therefore, it is expected that an efficient energy transfer from the Ag NPs to TiO 2 can be occured. 75The position of Ag SPR absorption peak of the S2 is around 419 nm, which is blue shift comparing to that of the other three samples. The SPR peak of the S2 is the most close to the anatase TiO 2 exciton energy, therefore the strongest resonant coupling effect between Ag SPR and the excitons of the TiO 2 films maybe produce more effectively.80Fig. 1 The optical absorption spectra of S1-S4 and the pure TiO2 filmTo illustrate the strong near field induced by the SPR of Ag NPs, the Raman scattering spectra of S1-S4 and TiO2 are measured as presented in Fig. 2. The observed Raman bands at 144, 85199, 399, 516 and 640 cm-1 can be assigned to the Eg, Eg, B1g, A1g, or B1g and an Eg vibration modes of anatase phase, respectively, which are consistent with the characteristic patterns of pure anatase without any trace of rutile or brookite phase [22]. It is found that the Raman intensity for S1-S4 increase compared to that of TiO2, and the S2 shows the strongest Raman intensity. It is well known that Raman scattering intensity is proportional to the square of the electric field 90intensity [23], thus stronger Raman scattering attained from the TiO2-SiO2-Ag structure indicates that a stronger electric field is induced by Ag NPs embedded in SiO2 substrate. When the Ag NPs are irradiated by laser in spectral area of particle absorption band longer wavelength shoulder, a strong near field is produced due to the SPR, so Raman scattering is enhanced. As seen from Fig.2, the enhancement factors of Raman scattering of S1-S4 is different because of various coupling 95field efficiency. Thus, it is possible to conclude that the implantation energy and fluence have determined the Raman scattering enhancement factor.Fig. 2 The Raman scattering spectra of S1-S4 and the pure TiO2 filmTo understand the relationship between the size and depth distributions of Ag NPs in silica 100glass and the Raman scattering enhancement factor of the TiO2-SiO2-Ag nanocomposites, themicrostructural characterization of the S1-S4 were investigated by TEM as shown in Fig. 3. The TEM image of the S1 (Fig 3 (a)) shows that the size of Ag NPs appears to have a wide distribution.However, increasing the implantation energy to 40 kV as shown in Fig 3 (b), the Ag NPs in the S2 105are quite uniform in size (with an size of 20 nm) and distribute at nearly the same depth of 7 nm from the surface. Under high energy ion implantation, more heat will be induced in the sample in short time, which enhances the diffusion of Ag atoms. Therefore, the implanted Ag ions trend to aggregate to larger NPs around the projected range [24-26]. The near field induced by the SPR of Ag NPs is very strong due to the presence of the formed Ag NPs with bigger size and the near-field 110dipolar interactions between adjacent particles [27]. On the other hand, the dipolar interactions between adjacent particles with near the same size can result in a blue shift of SPR [28], thus the blue shift in the SPR peak of Ag NPs observed in the Fig. 1, which may produce a strongest resonant coupling effect between the SPR of Ag NPs and TiO2. It means the stronger near field can be induced. In this case, the S2 has the strongest Raman scattering enhancement factor. The 115size of Ag NPs in the S1 is smaller and the distribution is wider than that in the S2. It means that the near field induced by SPR of Ag NPs in the S1 is weaker than that in the S2. Further increasing the implantation energy to 60 kV as presented in Fig 3 (d), Ag NPs in the S4 reside deeper below the surface than that in the S2. Since the SP is an evanescent wave that exponentially decays with distance from the metal particles to the surface [29], the enhancement of 120Raman scattering decrease progressively with the increase of distance between Ag NPs with the TiO2 film, therefore Raman scattering intensity of the S4 has almost no enhancement. When the ion implantation fluence is increased to 1×1017 ions/cm2 with implantation energy of 40 kV (S3) as displayed in Figure 3 (c), large Ag NPs with size about 15 nm are formed near the surface and the small ones in deeper SiO2 matrix. The surface sputtering effect plays an important role for ion 125implantation at high fluence, the formed small Ag NPs near the surface are sputtered away by the subsequent implanted ions, as a result the large Ag NPs are populated near the surface of the S3[24].The Raman scattering enhancement factor is small with increasing the implantation fluence.Therefore, the Raman scattering enhancement demonstrates that the strong near field is actually induced by introducing Ag NPs, the increased field could locally concentrate the light surrounding the Ag NPs and thus enhance the absorption of light.130Fig. 3 Cross-sectional TEM images of the (a) S1, (b) S2, (c) S3, (d) S4.In order to study the enhancement of light absorption in TiO2-SiO2-Ag nanostructural 135composites, the photocatalysis activities of the S1-S4 are investigated by the UV degradation of MB solution at room temperature. For comparison, the TiO2 film is carried out under the same experimental conditions. As shown in Fig 4(a) inset one, the concentration of MB is decreased upon the irradiation time, and the TiO2 film can decompose 49% of MB after the UV irradiationfor 4 hours. However, the TiO 2-SiO 2-Ag nanostructural composite films obtained higherphotocatalytic efficiency than the pure TiO 2 film, and the S2 has the highest photocatalytic 140 efficiency than all other three samples, and degrade 72% MB. The enhancement ratio is as high as 47%. Meanwhile, the photodegradation of MB can be assumed to follow the classical Langmuir–Hinshelwood (L–H) kinetics [30], and its kinetics can be expressed as follows:0ln()A kt A = Where k is the apparent first-order reaction rate constant (min -1), 0A and A represent the145 absorbance before and after irradiation for time t , respectively. As displayed in Fig. 4(a), S2 shows the highest rate constant among all the samples, the k values of the S2 is about 2 times than that of the pure TiO 2. The kinetic rate constants follow the order S2>S3>S1>S4>TiO 2. This is consistent with the Raman scattering enhancement result.The near field enhancement in the TiO 2 layer due to the presence of the Ag NPs is also 150 simulated by Finite Difference Time Domain (FDTD) method as shown in Fig. 4(b). In our structure, we consider x as the light incident direction, the illuminating plane wave with a wavelength of 420 nm is y polarized, and an Ag NP with a diameter of 20 nm is embedded in SiO 2, and the distance to the surface of SiO 2 substrate is 7 nm. An amplitude enhancement to 3 can be observed. Theoretical and experimental results show that an enhancement of near field is induced 155by the SPR of Ag NPs. The SPR excitations cause a large increase in electromagnetic field in the vicinity of metal NPs, the localized amplification can increase the incident excitation field and boost the creation of hole-electron pairs, which results in the enhancement of the photocatalytic activity of TiO 2.160 Fig. 4 (a) The photodegradation of MB solution by S1-S4 and reference sample TiO 2 under UV light irradiation (inset one), and corresponding plots of 0ln()A Aversus the irradiation time, showing the linear fitting results; (b) amplitude enhancement of electric field inside a TiO 2 layer is simulated by FDTD method.3 Conclusion165 In conclusion, we have successfully demonstrated a plasmonic effect by simply incorporating Ag NPs with TiO 2 film. Optimum ion implantation conditions for Ag NPs synthesis in SiO 2 were experimentally estimated. The plasmonic effect occurring near interface of TiO 2 and silica glass has effectively enhanced the light trapping. Both the experimental data and the simulations show that the enhancement effect is attained from near field enhancement induced by the SPR of Ag 170 NPs. Our results have shown that the plasmonic effect has great potential in the application of increasing the UV light absorption in TiO 2 photocatalyst, and opening up opportunities for highly efficient ultra-thin film solar cells.References175[1] Wang D, Zou Y, Wen S and Fan D. A passivated codoping approach to tailor the band edges of TiO2 forefficient photocatalytic degradation of organic pollutants[J]. Appl. Phys. Lett. 2009, 95: 012106-1-3.[2] Han F, Kambala V S R, Srinivasan M, Rajarathnam D and Naidu R. Tailored titanium dioxide photocatalystsfor the degradation of organic dyes in wastewater treatment: A review[J]. Appl. Catal. A-Gen 2009, 359:25-40. 180[3] Yang J, You J, Chen C C, Hsu W C, Tan H R, Zhang X W, Hong Z and Yang Y. Plasmonic Polymer TandemSolar Cell[J]. ACS nano 2011, 5: 6210-6217.[4] Min B K, Heo J E, Youn N K, Joo O S, Lee H, Kim J H and Kim H S. Tuning of the photocatalytic1,4-dioxane degradation with surface Plasmon resonance of gold nanoparticles on titania[J]. Catal. Commun. 2009, 10:712 -715.185[5] Kumar M K, Krishnamoorthy S, Tan L K, Chiam S Y, Tripathy S and Gao H. Field Effects in PlasmonicPhotocatalyst by Precise SiO2 Thickness Control Using Atomic Layer Deposition[J]. ACS Catal. 2011, 1: 300-308.[6] Tong H, Quyang S, Bi Y, Umezawa N, Oshikiri M and Ye J. Nano- photocatalytic Materials: Possibilities andChallenges[J]. Adv. Mater. 2012, 24: 229-251.190[7] Anpo M. Preparation, Characterization, and Reactivities of Highly Functional Titanium Oxide-BasedPhotocatalysts Able to Operate under UV-Visible Light[J]. Bull. Chem. Soc. Jpn. 2004, 77:1427-1442.[8] Asahi R, Morikawa T, Ohwaki T, Aoki K and Taga Y. Visible-Light Photocatalysis in Nitrogen-DopedTitanium Oxides[J]. Science 2001, 293:269-271.[9] Ghicov A, Macak J M, Tsuchiya H, Kunze J, Haeublein V, Frey L and Schmuki P.Ion Implantation and 195Annealing for an Efficient N-Doping of TiO2 Nanotubes[J].Nano Lett.2006, 6 (5): 1080-1082.[10] Xu J H, Li J, Dai W L, Cao Y, Li H and Fan K. Simple fabrication of twist-like helix N,S-codoped titaniaphotocatalyst with visible-light response[J].Appl. Catal., B-Environ.2008, 79: 72-80.[11] Xiao X H, Ren F, Zhou X D, Peng T C, Wu W, Peng X N, Yu X F and Jiang C Z. Surface plasmon-enhancedlight emission using silver nanoparticles embedded in ZnO[J]. Appl. Phys. Lett.2010, 97:071909-1-3.200[12] Zhou X D, Xiao X H, Xu J X, Cai G X, Ren F, and Jiang C Z. Mechanism of the enhancement and quenchingof ZnO photoluminescence by ZnO-Ag coupling[J]. Europhys. Lett.2011, 93: 57009-p1-p6.[13] Zhang S G, Zhang X W, Yin Z G, Wang J X, Dong J J, Gao H L, Si F T, Sun S S and Tao Y. Localizedsurface plasmon-enhanced electroluminescence from ZnO-based heterojunction light-emitting diodes[J]. Appl.Phys. Lett.2011, 99: 181116-1-3.205[14] Okamoto K, Niki I, Shvartser A, Narukawa Y, Mukai T, and Scherer A. Surface-plasmon-enhanced lightemitters based on InGaN quantum wells[J]. Nature Mater.2004, 3: 601-605.[15] Awazu K, Fujimaki M, Rockstuhl C, Tominaga J, Murakami H, Ohki Y, Yoshida N, Watanabe T. APlasmonic Photocatalyst Consisting of Silver Nanoparticles Embedded in Titanium Dioxide[J]. J. Am. Chem.Soc.2008, 130: 1676 -1680.210[16] Oh J -H, Lee H, Kim D, Seong T Y. Effect of Ag nanoparticle size on the plasmonic photocatalytic propertiesof TiO2 thin films[J]. Surf. Coat. Technol. 2011, 206(1): 185-189.[17] Subrahmanyam A, Biju K P, Rajesh P, Jagadeesh Kumar K, Raveendra Kiran M. Surface modification ofsol gel TiO2 surface with sputtered metallic silver for Sun light photocatalytic activity: Initial studies[J]. Sol.Energy Mater. Sol. Cells 2012, 101: 241-248.215[18] Kerker M. The optics of colloidal silver: something old and something new[J]. J. Colloid Interface Sci.1985,105: 297-314.[19] Stepanov A L, Hole D E and Townsend P D. Modification of size distribution of ion implanted silvernanoparticles in sodium silicate glass using laser and thermal annealing[J]. Nucl. Instr. Meth. Phys. Res. B 1999, 149: 89-98.220[20] Linsebigler A L, Lu G Q and Yates Jr J T. Photocatalysis on TiO2 Surfaces: Principles, Mechanisms, andSelected Results[J]. Chem. Rev.1995, 95:735-758.[21] Ren F, Jiang C Z, Liu C, Fu D J and Shi Y. Interface influence on the surface plasmon resonance of Agnanocluster composite[J]. Solid State Commun. 2005, 135: 268-272.[22] Zhang W F, He Y L, Zhang M S, Yin Zand Chen Q. Raman scattering study on anatase TiO2 nanocrystals[J]. 225J. Phys. D: Appl. Phys. 2000, 33: 912-916.[23] Willets K A, Van Duyne R P. Localized Surface Plasmon Resonance Spectroscopy and Sensing[J]. Annu.Rev. Phys. Chem. 2007, 58: 267-297.[24] Ren F, Xiao X H, Cai G X, Wang J B, Jiang C Z. Engineering embedded metal nanoparticles with ion beamtechnology[J]. Appl. Phys. A.2009, 96: 317-325.230[25] Xiao X H, Ren F, Wang J B, Liu C, Jiang C Z. Formation of aligned silver nanoparticles by ionimplantation[J]. Mater. Lett.2007, 61: 4435-4437.[26] Ren F, Jiang C Z, Liu C, Wang J B and Oku T. Controlling the morphology of Ag nanoclusters by ionimplantation to different doses and subsequent annealing[J]. Phys. Rev. Lett. 2006, 97: 165501-1-4.[27] Biteen J S, Lewis N S, and Atwater H A. Spectral tuning of plasmon-enhanced silicon quantum dot 235luminescence[J]. Appl. Phys. Lett. 2006, 88:131109-1-3.[28] Maier S A and Atwater H A. Plasmonics Localization and guiding of electromagnetic energy inmetal/dielectric structures[J]. J. Appl. Phys. 2005, 98: 011101-1-10.[29] Chen C W, Wang C H, Wei C M, Chen Y F. Tunable emission based on the composite of Au nanoparticlesand CdSe quantum dots deposited on elastomeric film[J]. Appl. Phys. Lett. 2009, 94: 071906-1-3.240[30] Al-Ekabi H and Serpone N. kinetic studies in heterogeneous photocatalysis. 1. photocatalytic degradatlon ofchlorinated phenols in aerated aqueous solutions over TiO2 supported on a glass matrix[J]. J. Phys. Chem.1988, 92:5726-5731.等离子体效应增强TiO2-SiO2-Ag复合薄膜的光吸收徐进霞,肖湘衡245(武汉大学物理科学与技术学院,武汉 430072)摘要:利用离子注入方法将Ag离子注入到SiO2玻璃中,再利用反应磁控溅射沉积TiO2薄膜。
二氧化硅包覆二氧化钛
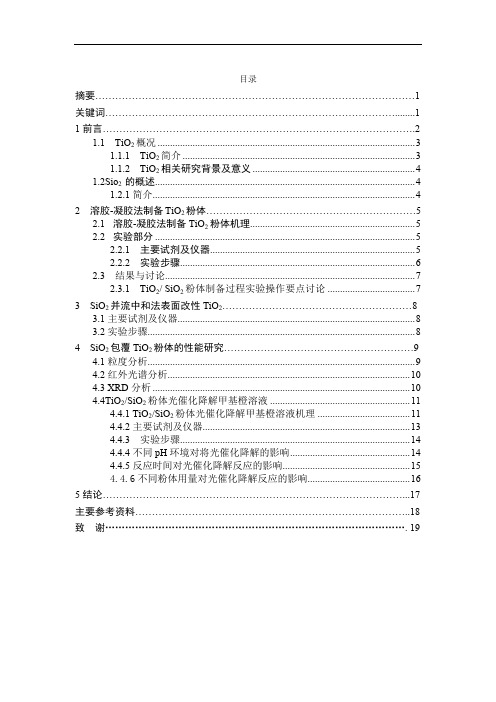
目录摘要 (1)关键词 (1)1前言 (2)1.1 TiO2概况 (3)1.1.1 TiO2简介 (3)1.1.2 TiO2相关研究背景及意义 (4)1.2Sio2 的概述 (4)1.2.1简介 (4)2 溶胶-凝胶法制备TiO2粉体 (5)2.1溶胶-凝胶法制备TiO2粉体机理 (5)2.2实验部分 (5)2.2.1 主要试剂及仪器 (5)2.2.2 实验步骤 (6)2.3 结果与讨论 (7)2.3.1 TiO2/ SiO2粉体制备过程实验操作要点讨论 (7)3 SiO2并流中和法表面改性TiO2 (8)3.1主要试剂及仪器 (8)3.2实验步骤 (8)4 SiO2包覆TiO2粉体的性能研究 (9)4.1粒度分析 (9)4.2红外光谱分析 (10)4.3 XRD分析 (10)4.4TiO2/SiO2粉体光催化降解甲基橙溶液 (11)4.4.1 TiO2/SiO2粉体光催化降解甲基橙溶液机理 (11)4.4.2主要试剂及仪器 (13)4.4.3 实验步骤 (14)4.4.4不同pH环境对将光催化降解的影响 (14)4.4.5反应时间对光催化降解反应的影响 (15)4.4.6不同粉体用量对光催化降解反应的影响 (16)5结论 (17)主要参考资料 (18)致谢 (19)湖南人文科技学院毕业论文(设计)SiO2包覆TiO2粉体的制备与性能研究李恩龙指导老师王书媚(湖南人文科技学院化学与材料科学系湖南娄底417000)摘要:以钛酸丁酯为先驱物,乙醇为溶剂,醋酸为螯合剂,采用溶胶-凝胶法制备超细TiO2粉体,以SiO2包覆材料用并流中和法对纳米TiO2粒子进行表面改性。
采用红外光谱分析、激光粒度分析、XRD、紫外光谱仪对改性前后粉体的晶型和晶粒大小进行了表征并对改性效果进行评价。
关键词:溶胶-凝胶法改性效果TiO2SiO2湖南人文科技学院毕业论文(设计) Study on Photocatalytic Properties ofTiO2/SiO 2PowdersLi EnlongTeacher Wang Shu-mei(Department of Chemistry and Material Science, Hunan Institute of Humanities,Science and Technology, Loudi, Hunan, 417000)Abstract: with butyl titanate as precursor, ethanol as solvent, acetic acid as chelating agent, was prepared by Sol - gel method of ultrafine titanium dioxide powder in Silicon dioxide coating material and stream and method of nano titanium dioxide particles surface modification. Using infrared spectrum analysis, laser particle size analysis, XRD, ultraviolet spectrometer of the modified powder crystal type and particle size were characterized and evaluated the effect of modified.Keywords : sol-gel modified effect Silicon dioxide Titania1前言纳米二氧化钛(TiO2)(有板钛型、锐钛型和金红石型3种晶体结构)是近年来发展较快的一种附加值很高的功能精细无机材料,因其具有粒径小,比表面积大,磁性强,光催化、吸收性能好,吸收紫外线能力强,表面活性大,热导性好,分散性好,以及良好的耐侯性、耐化学腐蚀性,抗紫外线能力强等特点,被广泛应用于感光材料、光催化剂、化妆品、食品包装材料、陶瓷添加剂、橡胶、塑料、皮革鞣制、高级轿车涂料等领域。
基于二氧化钛和活性碳纤维复合材料的光催化氧化亚砷

Photocatalytic Oxidation of Arsenite by a Composite of Titanium Dioxide and Activated Carbon FiberShuhua Yao1,2,Yongfeng Jia*1,Zhongliang Shi2and Shanlin Zhao31Institute of Applied Ecology,Chinese Academy of Sciences,Shenyang,China2School of Applied Chemistry,Shenyang University of Chemical Technology,Shenyang,China3Liaoning Shihua University,Fushun,ChinaReceived11June2010,accepted27August2010,DOI:10.1111/j.1751-1097.2010.00813.xABSTRACTPreoxidation process is usually needed in the treatment of arsenic-containing drinking water because arsenite(i.e.As[III]) is less easily removed by adsorption.Nano-scale titanium dioxide is an efficient photocatalyst for arsenite oxidation but its application in water treatment is limited due to the difficulty of separation or packed-bed application of the tiny particles. This study synthesized a composite photocatalyst by loading titanium dioxide onto activated carbonfiber(TiO2⁄ACF).The effects of calcination temperature,photocatalyst dosage,pH, initial concentration of As(III)and common anions on the oxidation of As(III)were studied.Photocatalytic oxidation of As(III)took place in minutes and followedfirst-order kinetics.0.80mg L)1of As(III)could be entirely oxidized to As(V) within30min in the presence of3.0g L)1photocatalyst and under UV-light irradiation.The oxidation of As(III)occurred in a wide range of pH as examined from2to10with the oxidation efficiency increasing markedly with pH.The presence of phosphate and silicate significantly decreased As(III)oxi-dation at pH7,while the effect of sulfate and chloride was small.INTRODUCTIONConsiderable documentation regarding the health threat of arsenic contamination in groundwater and drinking water is now available.The chronic toxicity of arsenic in drinking water is known to cause various types of cancer and black foot disease(1).In1993,the World Health Organization(WHO) proposed a new guideline for arsenic in drinking water of 10l g L)1.Recently,the US Environment Protection Agency (USEPA),the European Union and China,etc.adopted a new standard for arsenic in drinking water at10l g L)1,replacing the old standard of50l g L)1.An effective arsenic removal technology is thus highly desirable to provide safe drinking water to the affected people.Arsenic occurs in both organic and inorganic forms in natural waters,but organic arsenic is of less importance as it goes through biotransformation and detoxifies through methylation.Inorganic arsenic may occur in)3,)1,0,+3 and+5oxidation states.Arsenic of+3and+5oxidation states,i.e.As(III)and As(V),are the dominant aqueous species in the environment and represent a potential threat to the ecological systems and human health.Arsenite is more toxic, more mobile and less efficiently removed from drinking water than arsenate at natural pH.Arsenic removal procedures generally involve an oxidation pretreatment process followed by adsorption or coprecipitation using adsorbents or coagu-lants.According to published literature,the oxidation can be achieved by oxidants such as oxygen,ozone,hydrogen peroxide,manganese oxides,chlorine,ferrous,etc.(2–5).This may result in additional treatment cost and secondary con-tamination to the water.TiO2photocatalysis has been extensively studied as an efficient method of remediating polluted water and air(5–7). Although TiO2photocatalytic oxidations in most cases were applied to the destruction of organic pollutants(8–14), photocatalytic reactions with inorganic species have been also demonstrated,in particular,the photocatalytic oxidation of As(III)to As(V)in UV-irradiated TiO2suspension has been reported recently(15–19).Direct application of suspended TiO2powders to drinking water treatment may be problematic due to the difficulty of separation and recovery of the tiny particles.Supported TiO2 is one of the choices forfield application of the photocatalyst. Much research has been devoted to immobilize TiO2photo-catalyst on porous supporting matrices,such as silica, alumina,zeolite,granular activated carbon(20–23).Most of the substrate materials used are granule-shaped,which may significantly limit the efficiency of photocatalysis since most of the TiO2loaded in the pores is not available for photon irradiation.There were some reports recently on the preparation of loaded TiO2on cellulosefibers and cotton fibers(24,25).Activated carbonfiber is also a highly porous carbon material,but having much larger external surfaces than granular-activated carbon.This makes it an ideal candidate as supporting material for TiO2,but no study was reported so far about synthesis of ACF-supported TiO2 and its application to photocatalytic oxidation of arsenite in water.The objectives of this work were to prepare supported photocatalyst TiO2⁄ACF and to investigate its properties and As(III)oxidation characteristics.*Corresponding author email:yongfeng.jia@(Yongfeng Jia)Ó2010The Authors.Journal Compilation.The American Society of Photobiology0031-8655/10 Photochemistry and Photobiology,2010,86:1215–12211215MATERIALS AND METHODSMaterials.All the chemicals used in the study were of analytical grade. All the solutions in the study were prepared using deionized water. Arsenite stock solution(1000mg L)1)was prepared by dissolving dehydrated sodium arsenite(NaAsO2)in deionized water.Further working solutions were prepared from stock solution for each experimental run.Nonwoven viscose rayon-based carbonfiber cloth used in this study was supplied by Zichuan Carbon Fibers Co.Ltd.(China).It was repeatedly treated with boiling deionized water until constant pH,dried in vacuum oven at80°C and stored in a desiccator.Synthesis procedure.Anatase TiO2was prepared by a sol–gel method at low temperature using tetrabutyltitanate Ti(OBu)4as precursor.A solution was prepared as follows:Ti(OBu)4(0.02mol) was added to absolute alcohol(50mL)under vigorous stirring conditions and then triethylamine(0.01mol)was added as a stabilizer of the solution and stirred(200r min)1)for2–3min under the protection of nitrogen.A second solution was prepared as follows: hydrochloric acid(3.0mL)and water(0.72mL)were added to absolute alcohol(50mL)and mixed well by a magnetic stirrer(200r min)1). The two solutions were then mixed together and stirred vigorously for 30min under nitrogen gasflow.The ACF was impregnated with the formed transparent TiO2sol for1h.The titanium sol⁄ACF turned into titanium gel⁄ACF after aging for7days at25°C.Then TiO2⁄ACF catalyst was obtained by desiccation of the titanium gel⁄ACF at100°C for6h followed by calcination at500°C for2h in a nitrogen atmosphere.The heating rate was set at15°C min)1.The prepared samples were tailored to small pieces before being added to arsenite solution.Characterization.Specific surface area and pore volume were obtained by the cumulative adsorption of nitrogen at77K using a micromeritics2000instrument(ASAP2000;Micromeritics,USA).The morphologies of TiO2⁄ACF and original ACF were examined by a scanning electron microscope(SEM;Holland Philips,JSM-5800).The morphology of small TiO2particles on ACF was determined by transmission electron microscopy(TEM;JEM2000EX).Photocatalytic oxidation of As(III).The photocatalytic oxidation experiments were carried out using quartz tubes and ultraviolet mercury lamp(125W,365nm)at room temperature.Initial concen-tration of arsenite was4mg L)1in most experiments.The suspension of arsenite–TiO2⁄ACF was equilibrated in the dark for24h to allow adsorption equilibrium before it was illuminated by the ultraviolet mercury lamp.The pH was adjusted with either0.1mol L)1HCl or NaOH.Samples of the suspension were withdrawn after a time interval andfiltered through0.2l m membrane.Thefiltrates were analyzed for residual As(III)concentration.Dark controls were done for each series of experiments.To compare the photocatalytic activity of TiO2⁄ACF, the pure TiO2powder was also tested.The amount of TiO2powder chosen is1.0g L)1,which is adequate under our conditions without disturbing the UV-light entering the reactor.The TiO2⁄ACF sample was used repeatedly,before the beginning of the next cycle,the remaining solution is replaced by fresh solution.Analysis of arsenic concentration.Arsenic concentration was ana-lyzed on an atomicfluorescence spectrometer(AFS)coupled with a hydride generator.To detect As(III)selectively,the working solution was prepared in a pH4.4sodium citrate buffer(0.4M),with a mixture of0.8%KBH4and0.2%NaOH as reducing solution and0.1mol L)1 citric acid as carrier solution.Under this condition,only As(III)was converted to AsH3and detected on an AFS instrument.RESULTS AND DISCUSSIONCharacterization of TiO2⁄ACF photocatalystThe porous structure of the ACF was significantly altered after loading TiO2(see Table1).The specific surface area obtained using the Brunauer-Emmett-Teller(BET)method(S BET)was reduced from973.2to426.3m2g)1($56%)and total pore volume was reduced from0.50to0.34cm3g)1(32%).This indicates that the pores of the ACF were remarkably blocked by the loaded TiO2.The SEM micrographs of the photocatalyst calcined at 500°C and the original ACF are shown in Fig.1.It is clear that thefibers are10–20l m in diameter and have many unbroken axial grooves on the surface and the particles of TiO2 aggregated into clusters and werefixed on the surface of ACF.Furthermore,the TEM image in Fig.2confirms that the anchored TiO2granules were obviously sintered on calcina-tion.However,the size of the particles that formed the clusters is around40nm and very uniform.The surface TiO2particles would play an important role in the photocatalytic reaction since most of it would be photon accessible.Therefore,using fabric material as the support TiO2could maximize photon available catalytic surface compared to granular-activated carbon and zeolite,etc.Photocatalytic oxidation of arseniteIn order to evaluate the actual photocatalytic activity of the TiO2⁄ACF photocatalyst calcined at500°C for2h,compar-ison of four arsenite oxidation processes,namely,photolysis of TiO2,pure ACF,TiO2⁄ACF and adsorption of TiO2⁄ACF was carried out and experiments were conducted to assess the effect of catalyst on the overall oxidation rate for an initial arsenite concentration(4mg L)1);the results are shown in Fig.3.Experimental results show that the adsorption of arsenite on pure ACF gets saturated after120min under UV-light irradiation,the concentration of arsenite does not decrease any more with the prolonging of UV-light irradiation time. This indicates that the pure ACF has little photocatalytic activity(Fig.3a).Similarly,the TiO2⁄ACF shows little photocatalytic activity without irradiation of UV-light,and the saturated adsorption capacity of TiO2⁄ACF is higher than that of the pure ACF(Fig.3b).This is a result of the polarity of the ACF after the deposition of TiO2particles. The concentrations of arsenite decrease with UV-light irra-diation for the arsenite⁄TiO2system and arsenite⁄TiO2⁄ACF system,but the oxidation percentages of arsenite with TiO2⁄ACF are lower than those of TiO2(Fig.3c,d).By comparison of the amounts of arsenite oxidized with and without UV(Fig.3),it can be affirmed that the disappear-ance of arsenite is due to photocatalytic oxidation instead of only to adsorption.The photocatalytic activity of TiO2⁄ACF samples at dif-ferent calcination temperature is shown in Fig.4.It can be seen that the calcination temperature significantly affected the photocatalytic activity of TiO2⁄ACF.Arsenite oxidation rate increased notably with increasing calcination temperature from300to500°C,and decreased when temperature was further raised to600°C;these results were accordant with previous report(26).The phenomena mentioned above can be Table1.Surface area and pore volume of activated carbonfiber (ACF)and TiO2⁄ACF.SampleTiO2loaded(mg g)1)BET surfacearea(m2g)1)Total porevolume(cm3g)1)ACF0973.20.50TiO2⁄ACF158.4426.30.34ACF=activated carbonfiber.1216Shuhua Yao et al.attributed to the following fact:as the calcination temperature increases,the improvement in crystallinity of anatase-type structure of TiO 2is pronounced.However,when heat treat-ment temperature increases to 600°C,the anatase structure of TiO 2begins to transform rutile structure,so its photocatalytic activity decreases.Hence,the TiO 2⁄ACF used in the following experiments was calcined at 500°C.Photocatalyst dosage is an important parameter in the reaction of As(III)oxidation.There is no doubt that photo-catalytic activity would increase with the catalystdosage.Figure 2.TEM photograph of TiO 2particles from TiO 2⁄ACF sample.a bc dFigure 1.SEM photographs of the original ACF and TiO 2⁄ACF photocatalyst.(a)ACF,120·;(b)ACF,2500·;(c)TiO 2⁄ACF,1000·;(d)TiO 2⁄ACF,3000·.Photochemistry and Photobiology,2010,861217However,when the dosage exceeds the optimal amount,the suspension will be too thick to assure enough light for the photocatalysis.The effect of TiO2⁄ACF dosage(1.0–3.0g L)1)on As(III)oxidation rate is displayed in Fig.5. The catalytic oxidation activity of blank ACF is also shown for comparison.It can be seen that the blank ACF also showed some catalytic activity in the oxidation of As(III).This may be attributed to surface oxygen and⁄or nitrogen functional groups inherited from the precursor or generated during activation process.However,the catalytic activity of ACF was much lower than TiO2⁄ACF.As(III)oxidation rate in the presence of TiO2⁄ACF was found to increase markedly with catalyst dosage.At 3.0g L)1,the oxidation reaction was completed in approximately60min and this dosage was applied in the following experiments.The kinetics of As(III)oxidation reaction was also inves-tigated using the data from Fig.5by plotting lnc t against time t(see Fig.6),where c t represents the concentration of As(III)in solution at time t.Apparently,all the plots give linear relationships indicating that the reaction can be reasonably described byfirst-order kinetics.The coefficients of thefirst-order kinetics model are summarized in Table2.The regres-sion coefficient(R2)at all conditions was>0.98.Photocatalytic oxidation of As(III)by TiO2⁄ACF under UV-light irradiation was studied at various initial As(III) concentrations at solid⁄solution ratio of 3.0g L)1(see Fig.7).Photocatalytic oxidation of As(III)was fast in the beginning for all initial As(III)concentrations.Arsenite could be completely oxidized to arsenate within60min at initial As(III)concentration of0.80mg L)1,while at this time only$68%of As(III)was oxidized for8.0mg L)1 As(III)system.Catalytic oxidation rate of As(III)by ACF⁄TiO2was strongly dependent on solution pH(see Fig.8).As(III) oxidation rate increased markedly with increasing pH(2–10) especially from mildly acidic media to neutral⁄mildly alkaline media.This was probably caused by the following several factors controlling As(III)fiAs(V)redox reaction.Firstly,in semiconductor photocatalysis,photogenerated holes(h vb+),electrons(e cb)),hydroxyl radicals(•OH),super-oxide ions(O À2)may take part in redox reactions.It is thermodynamically favorable for As(III)to be oxidized to As(V)in the UV⁄TiO2system at all pH ranges(27).Thespecies h vb+,e cb),•OH and O À2can thus oxidize As(III)to As(IV),then As(IV)is further oxidized to As(V)(17,28). Under the conditions of this study,the oxidation could be proposed as the following mechanistic pathways:Table2.Coefficients of afirst-order kinetics modelfitting of As(III)oxidation.Solid solution ratio(g L)1) 1.0 2.0 3.0Rate constant k(min)1)7.45·10)3 1.79·10)2 2.97·10)2Squared correlationcoefficient R20.990.990.98 1218Shuhua Yao et al.TiO2À!hv TiO2ðh vbþþe cbÀÞð1ÞOHÀþh vbþ!OH ð2ÞO2þe cbÀ!O2 Àð3ÞAs(III)þOH !As(IV)þOHÀð4ÞAs(III)þO2 Àþ2Hþ!As(IV)þH2O2ð5ÞAs(IV)þO2!As(V)þO2 Àð6ÞFurthermore,the valance band holes and the photoreduced H2O2could also contribute to the oxidation in term of the following reactions(7):O2 Àþe cbÀþ2Hþ!H2O2ð7ÞO2 ÀþO2 Àþ2Hþ!H2O2þO2ð8ÞAs(III)þH2O2!As(V)þOHÀð9ÞAs(III)þh vbþ!As(IV)ð10ÞAs(IV)þO2!As(V)þO2 Àð11ÞAccording to the proposed mechanism(5,29–31),genera-tion of OH radicals and superoxide was improved in alkaline media.Therefore,it is favorable for the As(III)fiAs(V) oxidation reaction rate to increase with increasing pH. Aqueous arsenite and arsenate species also strongly depend on media pH.Hence,the standard reduction potentials of the As(V)⁄As(III)couple for various species are also the function of media pH(17,32).The mechanism of As(III)fiAs(V)oxidation reaction catalyzed by TiO2is proposed as following three steps:(1) adsorption of As(III)on the surface of TiO2;(2)oxidation of As(III)to As(V);(3)desorption of As(V)from the surface of TiO2.The adsorption⁄desorption profiles of As(III)and As(V) strongly depend on media pH.At acidic pH,TiO2shows stronger affinity for As(V)whereas at alkaline pH As(III)is more strongly adsorbed.Hence,steps(1)and(3)of the oxidation reaction are suppressed at acidic pH but promoted at alkaline pH.This is another important reason why the rate of photocatalytic oxidation of As(III)increases remarkably with increasing pH.Experiments were also carried out to investigate the effect of common anions on photocatalytic oxidation of As(III)by ACF⁄TiO2and the results are shown in Fig.9.Apparently, As(III)oxidation rate was significantly suppressed in the presence of SiO3and PO4ions,while the effect of SO4and ClPhotochemistry and Photobiology,2010,861219ions was small.Similar results were reported by Su and Puls (33).Competitive adsorption of these co-occurring anions inhibited As(III)adsorption onto the photocatalyst,as a result the oxidation reaction was suppressed.It is well known that silicate and phosphate strongly adsorb to metal oxide surfaces via inner-sphere complexation similar to the interaction mode of arsenite and arsenate ions with metal oxides.The adsorp-tion behavior of silicate is similar to arsenite since thefirst dissociation constant,pK1,of H2SiO3and H3AsO3is9.9and 9.2,respectively.On the other hand,the chemical similarity between arsenic and phosphorous explains the high interfering effect of phosphate in the As(III)adsorption onto the photocatalyst.The significant reduction in As(III)adsorption capacity in the presence of SiO3and PO4ions was due to the competition of the anions with As(III)for metal oxides sorption sites.As(III),silicate and phosphate are adsorbed on metal oxides through the formation of surface complexes with the surface hydroxyl groups(34).Sulfate ions can be adsorbed by metal oxides both specifically and nonspecifically via inner-and outer-sphere complexation.In addition,the sulfate binding affinity for metal oxides was much weaker than As(III)(35).Hence,the oxidation of As(III)is most signif-icantly affected by silicate and phosphate ions.In order to test the adhesion of TiO2and the ACF,the photo-oxidation experiments of arsenite had been repeated four times,and the results are shown in Fig.10.It showed the photocatalytic activity of TiO2⁄ACF remained above90%of its activity as-prepared after being used four times.Thus it is suggested that the deposited TiO2particles havefirmly attached to the ACF surface,and cannot be easily exfoliated from the ACF with mechanically stirred solutions for a long period.At the same time,it also proves that thefinal removal of arsenite from solution is caused by the photocatalytic oxidation other than the adsorption process that will lead to saturated adsorption of arsenite on the photocatalyst. CONCLUSIONSA supported photocatalyst was synthesized by loading TiO2 onto ACF using sol–gel method and its photocatalytic activity for As(III)oxidation was investigated.The oxidation of As(III)in the presence of TiO2⁄ACF was fast and the reaction followedfirst-order kinetics.The rate of photocatalytic As(III) oxidation was faster at higher pH.Silicate and phosphate ions were found to significantly suppress As(III)oxidation due to competitive adsorption with arsenite ions on the surface of TiO2.In addition,the supported samples can be used repeatedly and the high photocatalytic oxidation properties are maintained with a slight decline.Therefore,the supported photocatalyst of fabric form can be applied in water treatment units for preoxidation treatment of As(III). Acknowledgements—This work isfinancially supported by the Chinese Academy of Sciences(KZCX2-YW-446)and the National Natural Science Foundation of China(40925011).REFERENCES1.Smith,A.H.,C.Hopenhayn-Rich,M.N.Bates,H.M.Goeden,I.H.Picciotto,H.M.Duggan,R.Wood,M.J.Kosnett and M.T.Smith(1992)Cancer risks from arsenic in drinking-water.Environ.Health Perspect.97,259–268.2.Driehaus,W.,R.Seith and M.Jekel(1995)Oxidation of arseni-c(III)with manganese oxides in water treatment.Water Res.29, 297–305.3.Kim,M.J.and J.Nriangu(2000)Oxidation of arsenitein groundwater using ozone and oxygen.Sci.Tot.Environ.247, 71–79.4.Hug,S.J.and O.Leupin(2003)Iron-catalyzed oxidation ofarsenic(III)by oxygen and by hydrogen peroxide:pH-dependent formation of oxidants in the Fenton reaction.Environ.Sci.Technol.37,2734–2742.5.Pettine,M.,L.Campanella and lero(1999)Arseniteoxidation by H2O2in aqueous solutions.Geochim.Cosmochim.Acta63,2727–2735.6.Ollis,D.F.and H.Al-Ekabi,Ed.(1993)Photocatalytic Purifica-tion and Treatment of Water and Air.Elsevier,Amsterdam.7.Ryu,J.and W.Choi(2004)Effect of TiO2surface modificationson photocatalytic oxidation of arsenite:The role of superoxides.Environ.Sci.Technol.38,2928–2933.8.Zheng,S.,Q.Huang,J.Zhou and B.Wang(1997)A study on dyephotoremoval in TiO2suspension solution.J.Photochem.Photo-biol.A108,235–243.9.Herrmann,J.M.,J.Disdier,P.Pichat,S.Malato and J.Blanco(1998)TiO2-based solar photocatalytic detoxification of water containing organic pollutants.Case studies of2,4-dichlorphen-oxyaceticacid(2,4-D)and of benzofuran.Appl.Catal.B17,15–23.10.Arslan,I.,I.A.Balcioglu and D.W.Bahnemann(2000)Hetero-geneous photocatalytic treatment of simulated dyehouse effluents using novel TiO2-photocatalyst.Appl.Catal.B26,193–206. 11.Guillard,G.,S.Horikoshi,N.Watanabe,H.Hidaka andP.Pichat(2002)Photocatalytic degradation mechanism for heterocyclic derivatives of triazolidine and triazole.J.Photochem.Photobiol.A149,155–168.12.Kun,R.,K.Mogyor o si and I.De ka ny(2006)Synthesis andstructural and photocatalytic properties of TiO2⁄montmorillonite nanocomposites.Appl.Clay Sci.32,99–110.13.Zuo,G.M.,Z.X.Cheng,H.Chen,G.W.Li and T.Miao(2006)Study on photocatalytic degradation of several volatile organic compounds.J.Hazard.Mater.128,158–163.14.Me nesi,J.,L.Ko ro si,E.Bazso,V.Zo llmer,A.Richardt and I.De ka ny(2008)Photocatalytic oxidation of organic pollutants on titania-clay composites.Chemosphere70,538–542.15.Yang,H.,W.Y.Lin and K.Rajeshwar(1999)Homogeneous andheterogeneous photocatalytic reactions involving As(III)and As(V)species in aqueous media.J.Photochem.Photobiol.A123, 137–143.16.Bissen,M.,B.M.Vieillard,A.J.Schindelin and F.H.Frimmel(2001)TiO2-catalyzed photooxidation of arsenite to arsenate in aqueous samples.Chemosphere44,751–757.1220Shuhua Yao et al.17.Lee,H.and W.Choi(2002)Photocatalytic oxidation of arsenite inTiO2suspension,kinetics and mechanisms.Environ.Sci.Technol.36,3872–3878.18.Paritam,K.D.,S.O.Pehkoneno,S.Virenderk and R.Ajayk(2005)Photocatalytic oxidation of arsenic(III),evidence of hydroxyl radicals.Environ.Sci.Technol.39,1827–1834.19.Zhang,F.S.and H.Itoh(2006)Photo catalytic oxidation andremoval of arsenite from water using slag-iron oxide-TiO2 adsorbent.Chemosphere65,125–131.20.Huang,W.Q.,A.J.Duan,Z.Zhao,G.F.Wan,G.Y.Jiang,T.Dou,K.H.Chung and J.Liu(2008)Ti-modified alumina supports prepared by sol–gel method used for deep HDS catalysts.Catal.Today131,314–321.21.Tsumura,T.,N.Kojitari,H.Umemura,M.Toyoda and M.Inagaki(2002)Composites between photoactive anatase-type TiO2and adsorptive carbon.Appl.Surf.Sci.196,429–436.22.Hiromi,Y.and A.Masakazu(2003)Local structures andphotocatalytic reactivities of the titanium oxide and chromium oxide species incorporated within micro-and meso-porous zeolite materials:XAFS and photoluminescence studies.Solid Stale Mater.Sci.7,471–481.23.Fukahori,S.,H.Ichiura,T.Kitaoka and H.Tanaka(2003)Photocatalytic decomposition of bisphenol A in water using composite TiO x-zeolite sheets prepared by a papermaking tech-nique.Environ.Sci.Technol.37,1048–1051.24.Bozzi,A.,T.Yuranova,I.Guasaquillo,ub and J.Kiwi(2005)Self-cleaning of modified cotton textiles by TiO2at low temperatures under daylight irradiation.J.Photochem.Photobiol.A174,156–164.25.Uddin,M.J.,F.Cesano,F.Bonino,S.Bordiga,G.Spoto,D.Scarano and A.Zecchina(2007)Photoactive TiO2films on cellulosefibres:Synthesis and characterization.J.Photochem.Photobiol.A189,286–294.26.Yuan,R.S.,J.T.Zheng,R.B.Guan and Y.C.Zhao(2005)Surface characteristics and photocatalytic activity of TiO2loaded on activated carbonfibers.Colloids Surf.A254,131–136.27.Wardman,P.(1989)Reduction potential of one-electron couplesinvolving free radicals in aqueous solution.J.Phys.Chem.Ref.Data18,1637–1646.28.Kocar,B.D.and W.P.Inskeep(2003)Photochemical oxidationof As(III)in ferrioxalate solutions.Environ.Sci.Technol.37, 1581–1588.29.Haque,M.M.and M.Muneer(2007)Photodegradation of nor-floxacin in aqueous suspensions of titanium dioxide.J.Hazard.Mater.145,51–57.30.Buxton,G.V.,C.L.Greenstock,W.P.Helman and A.B.Ross(1988)Critical-review of rate constants for reactions of hydrated electrons,hydrogen-atoms and hydroxyl radicals in aqueous solution.J.Phys.Chem.Ref.Data17,513–886.31.Klaning,U.K., B.H.J.Bielski and K.Sehested(1989)Arsenic(IV)—A pulse-radiolysis study.Inorg.Chem.28,2717–2724.32.Bard, A.J.,R.Parsons and J.Jordan,Eds.(1985)StandardPotential in Aqueous Solution.Marcel Dekker,New York.33.Su,C.and R.W.Puls(2001)Arsenate and arsenite removal byzerovalent iron:Effects of phosphate,silicate,carbonate,borate, sulfate,chromate,molybdate,and nitrate,relative to chloride.Environ.Sci.Technol.35,4562–4568.34.Goldberg,S.(1985)Chemical modeling of anions competition ongoethite using the constant capacitance model.Soil Sci.Soc.Am.J.49,851–856.35.Meng,X.G.,S.B.Bang and G.P.Korfiatis(2000)Effects ofsilicate,sulfate,and carbonate on arsenic removal by ferric chlo-ride.Water Res.34,1255–1261.Photochemistry and Photobiology,2010,861221。
柠檬酸溶胶-凝胶法在制备多孔膜中的应用
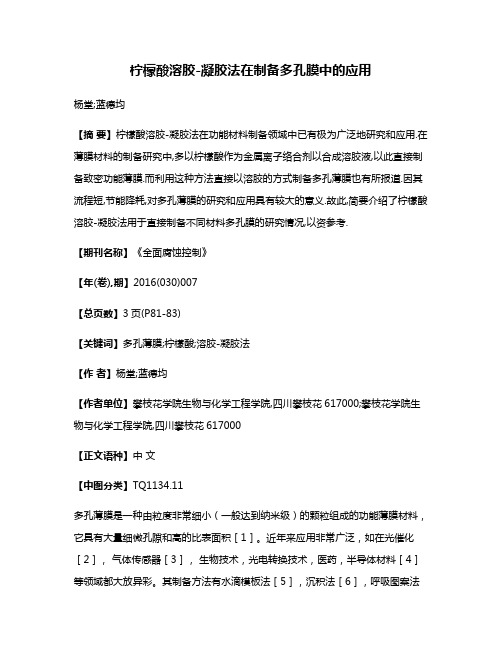
柠檬酸溶胶-凝胶法在制备多孔膜中的应用杨堂;蓝德均【摘要】柠檬酸溶胶-凝胶法在功能材料制备领域中已有极为广泛地研究和应用.在薄膜材料的制备研究中,多以柠檬酸作为金属离子络合剂以合成溶胶液,以此直接制备致密功能薄膜.而利用这种方法直接以溶胶的方式制备多孔薄膜也有所报道.因其流程短,节能降耗,对多孔薄膜的研究和应用具有较大的意义.故此,简要介绍了柠檬酸溶胶-凝胶法用于直接制备不同材料多孔膜的研究情况,以资参考.【期刊名称】《全面腐蚀控制》【年(卷),期】2016(030)007【总页数】3页(P81-83)【关键词】多孔薄膜;柠檬酸;溶胶-凝胶法【作者】杨堂;蓝德均【作者单位】攀枝花学院生物与化学工程学院,四川攀枝花617000;攀枝花学院生物与化学工程学院,四川攀枝花617000【正文语种】中文【中图分类】TQ1134.11多孔薄膜是一种由粒度非常细小(一般达到纳米级)的颗粒组成的功能薄膜材料,它具有大量细微孔隙和高的比表面积[1]。
近年来应用非常广泛,如在光催化[2],气体传感器[3],生物技术,光电转换技术,医药,半导体材料[4]等领域都大放异彩。
其制备方法有水滴模板法[5],沉积法[6],呼吸图案法[7],溶胶-凝胶法[8,9]等。
其中溶胶凝胶法因为成本低,工艺流程简单,且需要的环境和设备简单得到广泛应用。
柠檬酸是一种三元羟基酸,在溶胶凝胶法中常用作为金属离子络合剂,而以此制备薄膜的研究和应用也涉及光催化、光电转换,气体传感器等多个方面。
光催化在实验室的研究主要是薄膜材料对甲基橙的降解和对紫外光的吸收,以对甲基橙的降解作用的强弱来反映薄膜的光催化效果。
1.1 钡盐薄膜王晓惠[10]加入柠檬酸,采用溶胶凝胶法在450℃下制备了BiVO4薄膜电极,并且测试了薄膜的光催化性能(以可见光降解亚甲基蓝)。
实验结果表明,薄膜甲基蓝有一定的光催化降解效果(在太阳光照射下),但效果不如氖灯直接光照。
在氙灯光照下降解亚甲基蓝的效果最好,具有稳定的光催化性能;Yue Xu[11]采用溶胶凝胶+柠檬酸法得到BiOCl薄膜.实验表明柠檬酸的加入提高了薄膜的光催化活性;张海萍[12]制备Bil2TiO20薄膜,这种多孔薄膜对于光的敏感度很高。
离子掺杂对提高锐钛型TiO2高热稳定性的研究
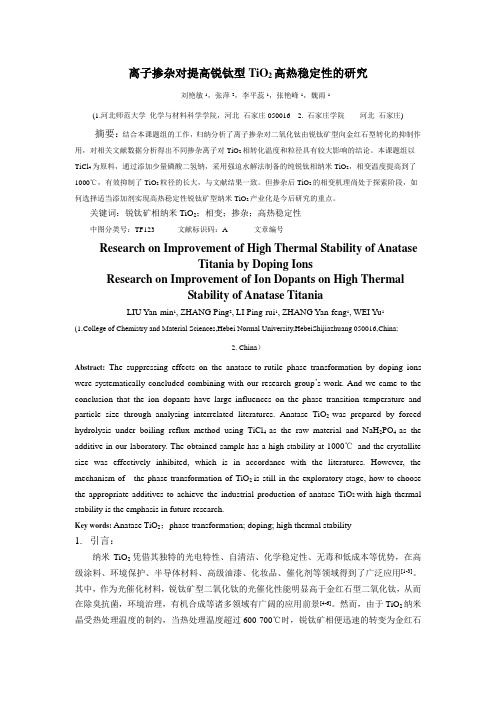
离子掺杂对提高锐钛型TiO2高热稳定性的研究刘艳敏1,张萍2,李平蕊1,张艳峰1,魏雨1(1.河北师范大学化学与材料科学学院,河北石家庄050016 2. 石家庄学院河北石家庄)摘要:结合本课题组的工作,归纳分析了离子掺杂对二氧化钛由锐钛矿型向金红石型转化的抑制作用,对相关文献数据分析得出不同掺杂离子对TiO2相转化温度和粒径具有较大影响的结论。
本课题组以TiCl4为原料,通过添加少量磷酸二氢钠,采用强迫水解法制备的纯锐钛相纳米TiO2,相变温度提高到了1000℃,有效抑制了TiO2粒径的长大,与文献结果一致。
但掺杂后TiO2的相变机理尚处于探索阶段,如何选择适当添加剂实现高热稳定性锐钛矿型纳米TiO2产业化是今后研究的重点。
关键词:锐钛矿相纳米TiO2;相变;掺杂;高热稳定性中图分类号:TF123 文献标识码:A 文章编号Research on Improvement of High Thermal Stability of AnataseTitania by Doping IonsResearch on Improvement of Ion Dopants on High ThermalStability of Anatase TitaniaLIU Yan-min1, ZHANG Ping2, LI Ping-rui1, ZHANG Yan-feng1, WEI Yu1(1.College of Chemistry and Material Sciences,Hebei Normal University,HebeiShijiazhuang 050016,China;2. China)Abstract:The suppressing effects on the anatase-to-rutile phase transformation by doping ions were systematically concluded combining with our research group’s work. And we came to the conclusion that the ion dopants have large influences on the phase transition temperature and particle size through analysing interrelated literatures. Anatase TiO2 was prepared by forced hydrolysis under boiling reflux method using TiCl4 as the raw material and NaH2PO4 as the additive in our laboratory. The obtained sample has a high stability at 1000℃and the crystallite size was effectively inhibited, which is in accordance with the literatures. However, the mechanism of the phase transformation of TiO2 is still in the exploratory stage, how to choose the appropriate additives to achieve the industrial production of anatase TiO2 with high thermal stability is the emphasis in future research.Key words:Anatase TiO2;phase transformation; doping; high thermal stability1.引言:纳米TiO2凭借其独特的光电特性、自清洁、化学稳定性、无毒和低成本等优势,在高级涂料、环境保护、半导体材料、高级油漆、化妆品、催化剂等领域得到了广泛应用[1-3]。
无机非金属材料工程专业英语

无机非金属材料工程专业英语一、无机非金属材料概论中文英文无机非金属材料inorganic non-metallic materials 定义definition分类classification组成composition结构structure性能properties制备方法preparation methods应用领域application fields陶瓷ceramics玻璃glass耐火材料refractories水泥cement石膏gypsum石棉asbestos碳素材料carbon materials石墨graphite碳纤维carbon fiber碳纳米管carbon nanotube钻石diamond全氟聚合物fluoropolymer聚四氟乙烯polytetrafluoroethylene (PTFE)聚偏氟乙烯polyvinylidene fluoride (PVDF)聚合物陶瓷polymer-derived ceramics (PDCs)氧化物陶瓷oxide ceramics氧化铝alumina (Al2O3)氧化锆zirconia (ZrO2)氧化镁magnesia (MgO)氧化钛titania (TiO2)非氧化物陶瓷non-oxide ceramics氮化硅silicon nitride (Si3N4)氮化铝aluminium nitride (AlN)碳化硅silicon carbide (SiC)碳化钨tungsten carbide (WC)碳化钛titanium carbide (TiC)二、物理化学中文英文物理化学physical chemistry物质matter结构structure组成composition性质properties变化规律laws of change分子运动论kinetic theory of molecules分子molecule原子atom离子ion气体gas液体liquid固体solid理想气体ideal gas真实气体real gas相平衡phase equilibrium相phase相图phase diagram相规则phase rule单元系unary system二元系binary system三元系ternary system溶液理论solution theory溶液solution溶剂solvent溶质solute浓度concentration摩尔分数mole fraction理想溶液ideal solution非理想溶液non-ideal solutionRaoult定律Raoult's lawHenry定律Henry's law三、无机材料科学基础中文英文无机材料科学基础fundamentals of inorganic materials science 无机材料inorganic materials结构structure性能properties结构-性能关系structure-property relationship晶体结构crystal structure晶体系统crystal system点阵类型lattice type空间群space group单胞参数lattice parameters基元胞primitive cell单位胞unit cell四、热工基础中文英文热工基础thermal engineering fundamentals热力学thermodynamics传热学heat transfer流体力学fluid mechanics热工学科thermal engineering disciplines 热力学第一定律first law of thermodynamics热力学第二定律second law of thermodynamics 热力系统thermodynamic system系统边界system boundary系统状态system state状态方程equation of state过程process循环cycle工作物质working substance理想气体ideal gas气体常数gas constant温度temperature压力pressure体积volume内能internal energy热容heat capacity比热容specific heat capacity焓enthalpy熵entropy自由能free energy吉布斯函数Gibbs function卡诺循环Carnot cycle热效率thermal efficiency 反向卡诺循环reversed Carnot cycle制冷系数coefficient of performance传导传热heat conduction傅里叶定律Fourier's law热导率thermal conductivity热阻thermal resistance稳态传热steady-state heat transfer非稳态传热transient heat transfer对流传热heat convection对流换热系数convection heat transfer coefficient 力对流forced convection自然对流natural convection努塞尔特数Nusselt number辐射传热heat radiation斯特藩-玻尔兹曼定律Stefan-Boltzmann law黑体blackbody发射率emissivity吸收率absorptivity反射率reflectivity透射率transmissivity灰体graybody视域因子view factor流体静力学fluid statics流体fluid密度density粘度viscosity表面张力surface tension液压hydrostatics帕斯卡定律Pascal's law流体运动方程equations of fluid motion质量守恒方程continuity equation动量守恒方程momentum equation能量守恒方程energy equation雷诺数Reynolds number理想流体ideal fluid实际流体real fluid层流laminar flow湍流turbulent flow边界层boundary layer阻力drag升力lift伯努利方程Bernoulli's equation皮托管Pitot tube五、无机非金属材料物理性能中文英文无机非金属材料物理性能physical properties of inorganic non-metallic materials 电学性能electrical properties磁学性能magnetic properties光学性能optical properties声学性能acoustic properties热学性能thermal properties电导率electrical conductivity电阻率electrical resistivity电容率electrical capacitance介电常数dielectric constant压电效应piezoelectric effect热电效应thermoelectric effect光电效应photoelectric effect半导体性质semiconductor properties铁电性质ferroelectric properties磁导率magnetic permeability磁化率magnetic susceptibility磁滞回线hysteresis loop铁磁性质ferromagnetic properties反铁磁性质antiferromagnetic properties顺磁性质paramagnetic properties抗磁性质diamagnetic properties光学常数optical constants折射率refractive index反射率reflectance吸收率absorbance透射率transmittance色散现象dispersion phenomenon双折射现象birefringence phenomenon声速sound velocity声阻抗acoustic impedance。
超临界合成Pt修饰的TiO2用于光催化CO2还原制备太阳能燃料

1. Introduction The increasing atmospheric concentration of greenhouse gases, especially CO2, is a pressing social issue at present [1]. Different processes to capture gas from large point sources such as the flue gases of coal, oil, natural gas and biomass pow‐ er plants have been patented in recent years [2,3]. The recov‐ ered CO2 can be either stored in natural caves or used as feed‐ stock to produce useful chemicals, especially fuel, which is the only CO2 conversion product that may substantially lower an‐ thropogenic CO2 emissions because of its high rate of consump‐ tion. However, because the CO2 molecule is very stable, only a few technologies for its conversion are available. In particular, effective photocatalytic conversion of CO2 to fuel has been demonstrated [4,5]. Thus, following this previous work, the present study focuses on the synthesis of catalysts for the pho‐ tocatalytic conversion of CO2 gas into fuel using solar energy (i.e., catalysts for solar fuel production from CO2), a process with an enormous future potential despite being in the early stages of development. The main drawbacks currently limiting photocatalytic CO2 reduction are low photoconversion speed and efficiency. These problems may be overcome through the design of highly active photocatalysts with favourable reactant adsorption, charge separation and transport, light harvesting and CO2 activation [6–14]. TiO2 particles show most of these features along with non‐toxicity, high photostability, chemical inertness, environ‐ mentally friendly nature and low cost. Thus, TiO2 is a promising material for use as a catalyst in CO2 photoreduction. The main weakness of TiO2 is that it only uses the ultraviolet (UV) region of the solar spectrum, which is less than 5% of the total solar energy. Moreover, after UV irradiation of TiO2 with an energy equal or larger than its band gap (3.2 eV), the result‐ ing photogenerated electron–hole pairs rapidly recombine.
- 1、下载文档前请自行甄别文档内容的完整性,平台不提供额外的编辑、内容补充、找答案等附加服务。
- 2、"仅部分预览"的文档,不可在线预览部分如存在完整性等问题,可反馈申请退款(可完整预览的文档不适用该条件!)。
- 3、如文档侵犯您的权益,请联系客服反馈,我们会尽快为您处理(人工客服工作时间:9:00-18:30)。
J O U R N A L O F M A T E R I A L S S C I E N C E L E T T E R S19(2000)699–701Effect of titania(TiO2)interfacial coating on mechanical properties of carbon–carbon compositesS.R.DHAKATE,V.K.PARASHAR,V.RAMAN,O.P.BAHLDivision of Engineering Materials,Carbon Technology Unit,National Physical Laboratory,Dr.K.S.Krishnan Marg,New Delhi110012,IndiaE-mail:dhakate@csnpl.ren.nic.inCarbon–carbon composites are promising materials for utilization in various strategic applications,such as space,defence,hydrogen gas turbine blades etc.[1,2]. The characteristics of the composites are governed by the nature of thefiber and matrix precursors used[3,4] for their fabrication and also onfiber–matrix interac-tions which form the interface.A strong interface is known to lead to a brittle tensile behavior,whereas a weak interface results infiber–matrix debonding and sliding.A non-linear stress–strain curve is obtained in cases wherefiber pull out takes place.Generally it is ob-served that toughness is achieved by the sliding mech-anism[5–8].Carbon–carbon composites developed using high strength carbonfiber with thermosetting phenolic or epoxy resin possess a strongfiber–matrix interaction.During pyrolysis the shrinkage stresses de-veloped lead to a microcraking in the composites[9]. On the application of external stresses,even at small stress levels,crack tips developed during pyrolysis initi-ate cracks which propagate continuously throughfibers without any deviation and damage thefiber structure, that degrades the mechanical properties of the compos-ites.To improve the mechanical performance of such carbon–carbon composites it is essential to modify the interface either by coating the carbonfiber surface or by modifying the matrix by incorporation of thermally stable compounds which reduce the interactions and thermal contraction betweenfiber and matrix.The ob-jective of the present investigation is to study the ef-fect of interfacial coating on the mechanical properties of carbon–carbon composites by employing titanium alkoxide as precursor material for coating the carbon fiber with titania.Titanium alkoxide which is employed in the present investigation as a source of titania is sensitive to mois-ture leading to the precipitation of titanium hydroxide which cannot be used for coating carbonfiber.In or-der to get hydrolyzed titanium solution it is essential to mask the titanium with complexing agents like acetyl acetone.Acetyl acetone when added to titanium alkox-ide in the molar ratio2:1gives a chelate complex which can be hydrolysed to get the sol.Titanium isopropaoxide,acetyl alcohol and ethanol were stirred for about3h to get the sol,which was coated onto the carbonfiber(T-300PAN based car-bonfiber having tensile strength=2.5GPa and tensile modulus=195GPa)by liquid infiltration at appropri-ate speed(coating speed such that everyfilament of the carbonfiber tow was coated by a desired coating thick-ness).Coatings were applied at different intervals of sol gel processing which helped to control the thickness of coating.The coated and the uncoatedfibers were em-ployed to develop polymer composites using phenolic resin as matrix precursor by match mold die technique keeping thefiber as45±2%.The match mold die con-sists of female mold and male plunger mold,made of die steel.On the basis offiber volume fraction,the required impregnated tows of the carbonfiber were stacked in the female mold.After stacking the carbonfiber tows, the male plunger wasfitted into the female mold with a stopper set for the desired thickness.The complete as-sembly was kept on the hot plate of the hydraulic press, the temperature of the hot plate increased and on reach-ing the desired temperature,the pressure was applied to get the composites of desired thickness.The composites developed using the coated carbonfibers are designated T-1,T-2and using the uncoated carbonfiber are desig-nated as T-0.The polymer composites were carbonized at1000◦C under nitrogen atmosphere.The compos-ites were characterized for mechanical properties us-ing three point bending on an Instron testing machine model4411.The coating on the carbonfiber surface and the interface developed after carbonization in uni-directional composites(the composites sample of size 4×4×3mm are taken from the unidirectional compos-ites andfixed with silver paste on the sample holder of the SEM)were observed by scanning electron micro-scope(model LEO440,manufactured by Leo electron microscopy limited,UK).Fig.1shows the SEM micrographs of carbonized composites,which gives the information about the coat-ing thickness and the interaction of the coating with thefiber and the matrix in the composites.The coating thickness on the carbonfiber was found to be around 150nm in T-1composites and around225nm in T-2 composites(Fig.1a and b).It is also seen from Fig.1a thatfiber and matrix are not in close contact which is contrary to the observation made with phenolic resin matrix composites(SEM micrographs of phenolic resin matrix composites,Fig.1c).This behavior is attributed to the TiO2layer developed around the carbonfiber after carbonization.It is observed that during carbonization the polymeric titanium network derived by the sol gel process shrinks in tensile mode(the interaction between polymeric titania and carbonfiber is weak,because the titania network shrank away from the carbonfiber dur-ing pyrolysis)and stresses developed during this phe-nomenon reduce the direct interaction of thefiber with0261–8028C 2000Kluwer Academic Publishers699Figure 1SEM micrographs of carbonized (a)T-1,(b)T-2,(c)T-0and (d)fracture behavior T-0.matrix and the above process is supported by negligi-ble difference in the coefficient of thermal expansion (CTE)of carbon fiber and the titania.Fig.2a shows the mechanical properties of the poly-mer and the carbonized composites.The value offlex-Figure 2Mechanical properties of composites:(a)flexural strength;(b)flexural modulus.ural strength in the case of T-0uncoated carbon fiber composites is higher as compared to T-1and T-2coated carbon fiber composites in polymer stage,T-0compos-ites are influenced by fiber matrix interactions and phe-nolic resin matrix makes strong interactions with T-300700Figure3Load–displacement curve of(T-0,T-1and T-2)carbonized composites.carbonfiber[10].The application of the coating(titania network)on thefiber reduces the interactions between fiber and matrix leading to lowerflexural strength in T-1and T-2composites as compared to T-0compos-ites.The same trend is noticed in theflexural modulus behavior.Theflexural strength of carbonized composites pre-sented in Fig.2a,shows that the coated titanium com-pound enhanced theflexural strength of the composites. During carbonization in the case of T-0composites due to the large difference of thermal expansion offiber and matrix the shrinkage stresses are developed which leads to microcraking in the matrix.On the application of the external load,the cracks once initiated in the ma-trix are not stopped at thefiber–matrix interface but propagate straight through thefiber and damage the fiber structure[9].Ultimately the composites fracture in tensile mode due to brittle behavior of composites (brittle fracture surface of T-0composites shown in Fig.1d)and fail at low load levels,as shown in the load–displacement curve(Fig.3,curve T0).Theflexural strengths of T-1composites are higher than T-0composites mentioned above.This enhance-ment is due to the interface developed during carboniza-tion of T-1.During heating the matrix shrinks and mi-crocracks are developed in the matrix phase[9]and on the application of the external stresses,the cracks de-veloped are not transferred to thefiber but are branched at the TiO2interphase[11](the TiO2interphase is hard material;instead of the crack passing through thefiber, crack branching occurs at the TiO2interphase)i.e.the fiber structure is not damaged and load is transferred through the interphase to thefiber.Even though the carbonfiber is brittle,the hard nature of the interphase material improves the fracture toughness of the com-posites and composites fractured at higher load level than T-0composites.The T-1composites fail in non catastrophic mode of fracture or tensile cum shear mode (semi brittle)[12](Fig.3,curve T1).The same trend is noticed in theflexural modulus behavior(Fig.2b).It is observed from Fig.2a and b that increasing the coat-ing thickness has negatively affected the mechanical properties,even though the same interphase is formed during heating in the case of T-2composites.This de-crease inflexural strength andflexural modulus may be due to the titania which behaves like a monolithic material when the coating thickness is increased.The catastrophic failure of these composites indicates that there may be an optimum coating thickness(concentra-tion)of TiO2interphase on carbonfiber where the me-chanical properties of composites improved to a great extent;below and above optimum coating thickness the mechanical properties are negatively affected.Further investigations have to be carried out to evaluate the opti-mum coating thickness(concentration)which can lead to an appreciable increase in mechanical properties. AcknowledgmentThe authors are grateful to Prof.A.K.Raychaudhari, Director NPL for his kind permission to publish these results.Thank also due to Dr Ram Kishore for his valu-able help in the characterization study(SEM). References1.J.D.B U C K L E Y and R.D.E D I E(eds.),“Carbon-carbon ma-terials and composites”,NASA Reference Publication1254(NASA Langley Research Centre,1992).2.E.F I T Z E R,Carbon25(1987)163.3.H.W E I S S H A U S,S.K E N I G and A.S I E G M A N N,ibid.29(1991)1203.4.F.D I L L O N,K.M.T H O M A S and H.M A R S H,ibid.31(1993)1337.5.E.F I T Z E R,K.H.G E I G L and W.H U T T N E R,ibid.18(1980)267.6.S.T A K A N O,T.U R U N O,T.K I N J O and C.P.T L O M A K,J.Mater.Sci.28(1993)5610.7.R.J.Z A L D I V A R,G.S.R E L L I C K and J.M.Y A N G,J.Mater.Res.8(1993)501.8.C.A H A R N and B.R A N D,Carbon34(1996)239.9.K.M.B E I N B O R N,M.M U L L E R and K.J.H U T T I N G E R,ibid.3(1995)1029.10.V.K.P A R A S H A R,S.R.D H A K A T E and O.P.B A H L,in Proceeding of23rd Biennial Conference on Carbon,Pennstate, USA,18–23July1997,p.586.11.E.Y A S U D A,Y.T A N A B E,L.M.M A N O C H A and S.K I M U R A,Carbon26(1988)225.12.L.M.M A N O C H A,O.P.B A H L and Y.K.S I N G H,ibid.27(1989)381.Received5Augustand accepted21October1999701。