Carbon nanotubes as a 1D template for the synthesis of air
锂离子电池中聚合物电解质的研究进展_步爱秀
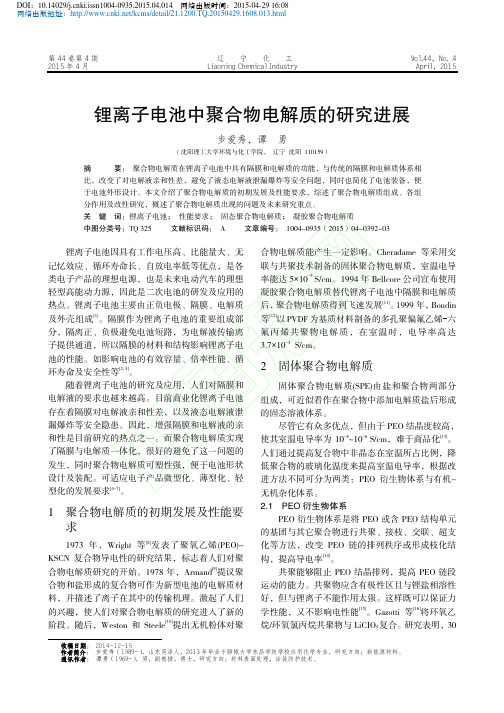
第44卷第4期 辽 宁 化 工 Vol.44,No. 4 2015年4月 Liaoning Chemical Industry April,2015收稿日期: 2014-12-15锂离子电池中聚合物电解质的研究进展步爱秀,谭 勇(沈阳理工大学环境与化工学院, 辽宁 沈阳 110159)摘 要: 聚合物电解质在锂离子电池中具有隔膜和电解质的功能,与传统的隔膜和电解质体系相比,改变了对电解液亲和性差,避免了液态电解液泄漏爆炸等安全问题,同时也简化了电池装备,便于电池外形设计。
本文介绍了聚合物电解质的初期发展及性能要求,综述了聚合物电解质组成、各组分作用及改性研究,概述了聚合物电解质出现的问题及未来研究重点。
关 键 词:锂离子电池; 性能要求; 固态聚合物电解质; 凝胶聚合物电解质中图分类号:TQ 325 文献标识码: A 文章编号: 1004-0935(2015)04-0392-03锂离子电池因具有工作电压高、比能量大、无记忆效应、循环寿命长、自放电率低等优点,是各类电子产品的理想电源,也是未来电动汽车的理想轻型高能动力源,因此是二次电池的研发及应用的热点。
锂离子电池主要由正负电极、隔膜、电解质及外壳组成[1]。
隔膜作为锂离子电池的重要组成部分,隔离正、负极避免电池短路,为电解液传输离子提供通道,所以隔膜的材料和结构影响锂离子电池的性能。
如影响电池的有效容量、倍率性能、循环寿命及安全性等[2, 3]。
随着锂离子电池的研究及应用,人们对隔膜和电解液的要求也越来越高。
目前商业化锂离子电池存在着隔膜对电解液亲和性差,以及液态电解液泄漏爆炸等安全隐患。
因此,增强隔膜和电解液的亲和性是目前研究的热点之一。
而聚合物电解质实现了隔膜与电解质一体化,很好的避免了这一问题的发生,同时聚合物电解质可塑性强,便于电池形状设计及装配。
可适应电子产品微型化、薄型化、轻型化的发展要求[4-7]。
1 聚合物电解质的初期发展及性能要求1973年,Wright 等[8]发表了聚氧乙烯(PEO)- KSCN 复合物导电性的研究结果,标志着人们对聚合物电解质研究的开始。
Carbon Nanotubes as Molecular Biosensors

Carbon Nanotubes as MolecularBiosensorsCarbon nanotubes (CNTs) are cylindrical structures made up of carbon atoms that are one-atom thick and can have a diameter of just a few nanometers. The unique physical and chemical properties of CNTs make them a promising candidate for a wide range of applications, including molecular biosensing.Molecular biosensors are devices that can detect and quantify the presence of specific biomolecules such as proteins, DNA, and RNA. These biomolecules are often indicative of disease states or environmental pollutants, and their detection can provide valuable insight into the underlying biological and chemical processes involved.To function as biosensors, CNTs are often modified with specific biomolecules, such as antibodies or aptamers, which bind to the target molecule of interest. The binding of the target molecule induces a change in the electrical or optical properties of the CNTs, which can be measured and used to detect the presence and concentration of the target molecule.One of the key advantages of CNT-based biosensors is their high sensitivity and selectivity. Due to their small size and high surface area, CNTs can detect biomolecules at very low concentrations, even down to the single-molecule level. Additionally, the surface chemistry of CNTs can be tailored to enhance their binding affinity for specific biomolecules, further improving their selectivity.Another advantage of CNT-based biosensors is their compatibility with various detection platforms. CNTs can be integrated with a range of transducers, such as field-effect transistors, optical sensors, or electrochemical sensors, to amplify and detect the binding-induced changes in electrical or optical signals.CNT-based biosensors have already shown promise in a range of applications, including early detection of cancer biomarkers, monitoring of bacterial infections, and detection of environmental pollutants such as heavy metals and pesticides.However, there are also some challenges and limitations to the use of CNTs as biosensors. One important consideration is their potential toxicity and biocompatibility. While CNTs are generally considered safe, there have been concerns about their potential accumulation and effects on living organisms. Therefore, extensive studies and regulations are necessary to ensure the safe use of CNTs in molecular biosensing applications.In conclusion, CNTs are emerging as a promising platform for molecular biosensing, with their high sensitivity, selectivity, and compatibility with various detection platforms. Further research and development in this field could lead to the development of CNT-based biosensors with improved performance and specificity, enabling their broader applications in disease diagnosis, environmental monitoring, and other areas.。
Properties and Uses of Carbon Nanotubes
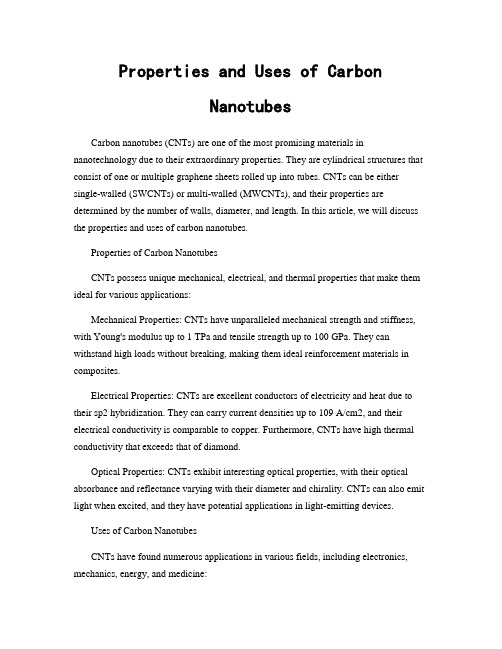
Properties and Uses of CarbonNanotubesCarbon nanotubes (CNTs) are one of the most promising materials in nanotechnology due to their extraordinary properties. They are cylindrical structures that consist of one or multiple graphene sheets rolled up into tubes. CNTs can be either single-walled (SWCNTs) or multi-walled (MWCNTs), and their properties are determined by the number of walls, diameter, and length. In this article, we will discuss the properties and uses of carbon nanotubes.Properties of Carbon NanotubesCNTs possess unique mechanical, electrical, and thermal properties that make them ideal for various applications:Mechanical Properties: CNTs have unparalleled mechanical strength and stiffness, with Young's modulus up to 1 TPa and tensile strength up to 100 GPa. They can withstand high loads without breaking, making them ideal reinforcement materials in composites.Electrical Properties: CNTs are excellent conductors of electricity and heat due to their sp2 hybridization. They can carry current densities up to 109 A/cm2, and their electrical conductivity is comparable to copper. Furthermore, CNTs have high thermal conductivity that exceeds that of diamond.Optical Properties: CNTs exhibit interesting optical properties, with their optical absorbance and reflectance varying with their diameter and chirality. CNTs can also emit light when excited, and they have potential applications in light-emitting devices.Uses of Carbon NanotubesCNTs have found numerous applications in various fields, including electronics, mechanics, energy, and medicine:Electronics: CNTs have been hailed as a replacement for silicon in the electronics industry due to their high charge-carrier mobility, low power consumption, and size compatibility. They can be used as field-effect transistors, sensors, memory devices, and interconnects.Mechanics: CNTs have been used as reinforcements in composites to improve their strength, stiffness, and toughness. They can also be used to develop lightweight materials, such as bicycle frames, tennis rackets, and airplanes.Energy: CNTs have been used as efficient catalysts, electrodes, and energy storage materials in energy applications. They can be used to produce hydrogen through the water-splitting reaction, to produce efficient and durable lithium-ion batteries, and to store hydrogen in fuel cells.Medicine: CNTs have been investigated for their biocompatibility and potential biomedical applications. They can be used as drug delivery vehicles, imaging agents, and tissue engineering scaffolds. However, their toxicity and environmental impact must be thoroughly investigated before their widespread use in medicine.ConclusionCarbon nanotubes possess unique properties that make them ideal for various applications in electronics, mechanics, energy, and medicine. Their mechanical strength, electrical and thermal conductivity, and optical properties have important implications for the development of new materials, devices, and systems. However, their commercialization and practical applications have been hindered by their high cost, scalability issues, and safety concerns. Further research and development are needed to overcome these challenges and fully exploit the potential of carbon nanotubes.。
CARBON NANOTUBE(S) AND METHOD FOR MAKING THE SAME
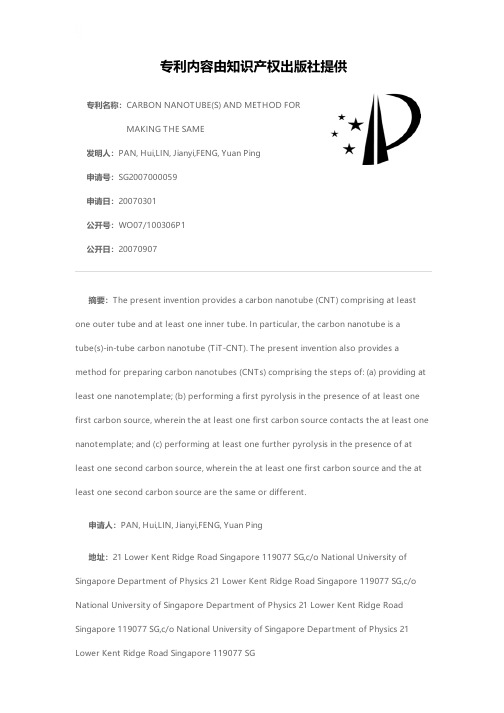
专利名称:CARBON NANOTUBE(S) AND METHOD FOR MAKING THE SAME发明人:PAN, Hui,LIN, Jianyi,FENG, Yuan Ping申请号:SG2007000059申请日:20070301公开号:WO07/100306P1公开日:20070907专利内容由知识产权出版社提供摘要:The present invention provides a carbon nanotube (CNT) comprising at least one outer tube and at least one inner tube. In particular, the carbon nanotube is atube(s)-in-tube carbon nanotube (TiT-CNT). The present invention also provides a method for preparing carbon nanotubes (CNTs) comprising the steps of: (a) providing at least one nanotemplate; (b) performing a first pyrolysis in the presence of at least one first carbon source, wherein the at least one first carbon source contacts the at least one nanotemplate; and (c) performing at least one further pyrolysis in the presence of at least one second carbon source, wherein the at least one first carbon source and the at least one second carbon source are the same or different.申请人:PAN, Hui,LIN, Jianyi,FENG, Yuan Ping地址:21 Lower Kent Ridge Road Singapore 119077 SG,c/o National University of Singapore Department of Physics 21 Lower Kent Ridge Road Singapore 119077 SG,c/o National University of Singapore Department of Physics 21 Lower Kent Ridge Road Singapore 119077 SG,c/o National University of Singapore Department of Physics 21 Lower Kent Ridge Road Singapore 119077 SG国籍:SG,SG,SG,SG代理机构:MATTEUCCI, Gianfranco 更多信息请下载全文后查看。
Chemical modification of carbon nanotubes

Chemical modification of carbonnanotubesCarbon nanotubes (CNTs) are a unique form of carbon allotrope, consisting of cylindrical tubes composed of graphite sheets rolled into a seamless tube. They possess excellent electronic and mechanical properties, and their small size and high surface area make them promising candidates for a wide range of applications including drug delivery, electronics, and energy storage. However, their hydrophobic nature and tendency to aggregate pose significant challenges in their use.One approach to addressing these issues is through chemical modification, which involves covalently attaching functional groups to the surface of the CNTs. This modification can alter their physical and chemical properties, such as solubility, reactivity, and biocompatibility. Chemical modification enables the tailoring of CNTs to specific applications, enhancing their functionality and improving their performance.Oxidation is the most commonly employed method of chemical modification of CNTs. This involves the introduction of oxygen functionalities, such as carboxyl groups (-COOH) and hydroxyl groups (-OH), onto the CNT surface. This creates a more polar surface, rendering the CNTs more hydrophilic and amenable to dispersion in aqueous or polar solvents. In addition, the increased reactivity of the oxidized CNTs enables further functionalization using a variety of organic molecules or polymers.In addition to oxidation, other methods of chemical modification have been developed. One such method is non-covalent functionalization, which involves the non-covalent attachment of molecules onto the CNT surface. This can be achieved through π-π bonding, van der Waals interactions, or electrostatic interactions. Non-covalent functionalization offers advantages such as preserving the structural integrity of the CNTs and providing a reversible modification that can be easily removed.Another method of chemical modification is the direct functionalization of CNTs with organic or inorganic molecules. This involves the covalent attachment of a specificmolecule or chemical group onto the CNT surface, resulting in a modified CNT with unique physical and chemical properties. Direct functionalization has been employed in a range of applications, such as drug delivery and biosensors.Chemical modification of CNTs has significant implications for their use in drug delivery. The introduction of functional groups can enhance their biocompatibility, enabling their use as drug carriers and targeted drug delivery vehicles. Additionally, incorporation of targeting ligands onto the surface of modified CNTs can improve their specificity and selectivity towards specific cell types or tissues.Overall, chemical modification offers a powerful strategy to tailor the properties of CNTs to specific applications. The versatility and functionality of modified CNTs make them promising candidates for a vast array of applications. While challenges remain, continued research and development in this field will undoubtedly contribute to unlocking the full potential of CNTs in a range of fields.。
The Physical Properties of Carbon Nanotubes
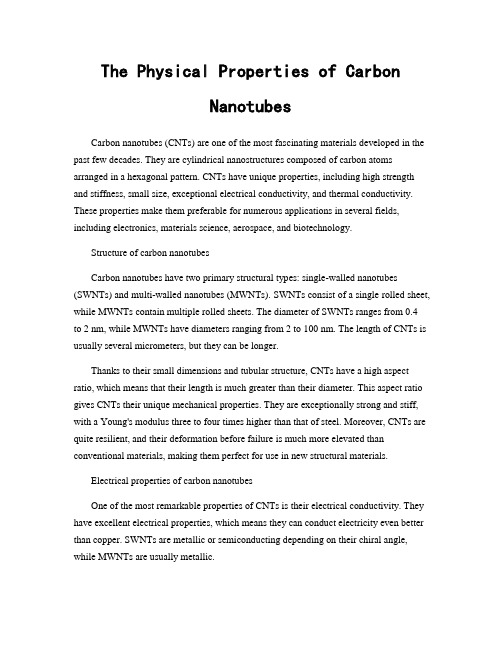
The Physical Properties of CarbonNanotubesCarbon nanotubes (CNTs) are one of the most fascinating materials developed in the past few decades. They are cylindrical nanostructures composed of carbon atoms arranged in a hexagonal pattern. CNTs have unique properties, including high strength and stiffness, small size, exceptional electrical conductivity, and thermal conductivity. These properties make them preferable for numerous applications in several fields, including electronics, materials science, aerospace, and biotechnology.Structure of carbon nanotubesCarbon nanotubes have two primary structural types: single-walled nanotubes (SWNTs) and multi-walled nanotubes (MWNTs). SWNTs consist of a single rolled sheet, while MWNTs contain multiple rolled sheets. The diameter of SWNTs ranges from 0.4to 2 nm, while MWNTs have diameters ranging from 2 to 100 nm. The length of CNTs is usually several micrometers, but they can be longer.Thanks to their small dimensions and tubular structure, CNTs have a high aspect ratio, which means that their length is much greater than their diameter. This aspect ratio gives CNTs their unique mechanical properties. They are exceptionally strong and stiff, with a Young's modulus three to four times higher than that of steel. Moreover, CNTs are quite resilient, and their deformation before failure is much more elevated than conventional materials, making them perfect for use in new structural materials.Electrical properties of carbon nanotubesOne of the most remarkable properties of CNTs is their electrical conductivity. They have excellent electrical properties, which means they can conduct electricity even better than copper. SWNTs are metallic or semiconducting depending on their chiral angle, while MWNTs are usually metallic.SWNTs have particular band structures, and their electrical properties depend heavily on their atomic structure. The electronic properties of CNTs make them ideal for use in electronic applications, such as field-effect transistors, diodes, and sensors. CNTs have the potential to improve the performance of transistors and other electronic devices significantly.Thermal properties of carbon nanotubesCNTs also have exceptional thermal conductivity, making them useful in thermal management materials. The thermal conductivity of CNTs is approximately seven times higher than that of copper. Moreover, CNTs are excellent heat conductors at the nanoscale, which gives them the potential to improve the efficiency of thermal management materials in electronic devices.Other physical properties of carbon nanotubesIn addition to their excellent mechanical, electrical, and thermal properties, CNTs also exhibit some other unique physical properties that make them advantageous for several applications. They are lightweight and can be dispersed in solvents, allowing them to be used in coatings, composites, and other materials.Furthermore, because of their nanoscale dimensions, CNTs have a high surface area-to-volume ratio, which makes them an effective adsorbent for gas and liquid molecules. This property makes CNTs promising candidates for gas storage and separation, as well as water purification.ConclusionCNTs are exceptional materials that have unique physical properties that lend themselves to several applications. They are lightweight, strong, stiff, and excellent electrical and thermal conductors, making them preferable for use in several fields, including electronics, materials science, and aerospace. Their physical properties make CNTs promising candidates for improving the performance of electronic devices, structural materials, and energy storage systems.。
流浪地球 太空电梯 英语描述

流浪地球太空电梯英语描述The movie "The Wandering Earth" is a Chinese science fiction film that has gained international attention forits epic portrayal of humanity's struggle to save Earth from an impending collision with Jupiter. The film follows the journey of a group of astronauts and scientists as they attempt to move the Earth out of its orbit and escape the solar system. One of the key technologies featured in the film is the space elevator, a concept that has long been a staple of science fiction but is now being seriously considered as a potential means of transporting materials and people into space.The concept of a space elevator has been around for over a century, but it wasn't until the 20th century that scientists and engineers began seriously considering its feasibility. The basic idea behind a space elevator is to construct a tall structure that extends from the surface of the Earth into space, with a counterweight at the other end to keep the structure taut. By using a combination ofadvanced materials and propulsion systems, it would be possible to transport payloads into space without the need for expensive and dangerous rocket launches.In "The Wandering Earth," the space elevator plays a crucial role in humanity's efforts to save the planet. As the Earth is moved out of its orbit, the space elevator becomes the primary means of transporting people and materials to the new world. This concept has captured the imagination of audiences around the world, as it represents a potential solution to the challenges of space travel and colonization.From a scientific perspective, the concept of a space elevator presents a number of significant challenges. One of the biggest hurdles is finding a material that is strong enough to support the immense weight of the structure while also being light enough to be practical. While carbon nanotubes have been proposed as a potential solution, the technology to produce them in the necessary quantities and at a reasonable cost is still a long way off. Additionally, the engineering challenges of constructing such a massivestructure are daunting, requiring precise coordination and control to ensure the elevator remains stable and safe.Despite these challenges, the concept of a space elevator has captured the imagination of scientists and engineers around the world. The potential benefits of such a system are immense, offering a cost-effective andefficient means of transporting materials and people into space. In addition to serving as a means of escape in the event of a planetary catastrophe, a space elevator could also enable the development of space-based industries and infrastructure, opening up new opportunities for scientific research and exploration.From a cultural perspective, the space elevator represents a powerful symbol of humanity's ability to overcome seemingly insurmountable challenges. In "The Wandering Earth," the space elevator becomes a beacon of hope for the characters, representing the possibility of a new beginning for humanity. This theme resonates with audiences around the world, tapping into a deep-seated desire for adventure and exploration. The concept of aspace elevator captures the imagination in a way that few other technologies can, offering a vision of the futurethat is both awe-inspiring and hopeful.In conclusion, the concept of a space elevator as depicted in "The Wandering Earth" offers a compellingvision of humanity's potential future in space. While the challenges of realizing such a system are significant, the potential benefits are immense, offering a cost-effective and efficient means of transporting materials and people into space. From a cultural perspective, the space elevator represents a powerful symbol of humanity's ability to overcome challenges and embrace the unknown. As we continue to explore the possibilities of space travel and colonization, the concept of a space elevator will undoubtedly remain a source of inspiration and wonder for generations to come.。
The properties of carbon nanotubes
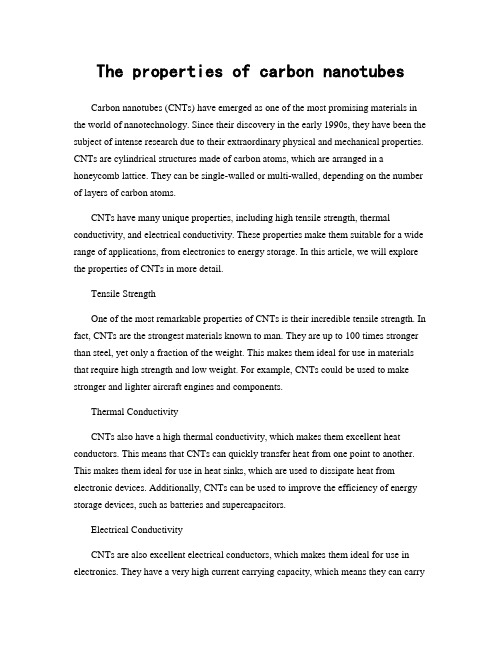
The properties of carbon nanotubesCarbon nanotubes (CNTs) have emerged as one of the most promising materials in the world of nanotechnology. Since their discovery in the early 1990s, they have been the subject of intense research due to their extraordinary physical and mechanical properties. CNTs are cylindrical structures made of carbon atoms, which are arranged in a honeycomb lattice. They can be single-walled or multi-walled, depending on the number of layers of carbon atoms.CNTs have many unique properties, including high tensile strength, thermal conductivity, and electrical conductivity. These properties make them suitable for a wide range of applications, from electronics to energy storage. In this article, we will explore the properties of CNTs in more detail.Tensile StrengthOne of the most remarkable properties of CNTs is their incredible tensile strength. In fact, CNTs are the strongest materials known to man. They are up to 100 times stronger than steel, yet only a fraction of the weight. This makes them ideal for use in materials that require high strength and low weight. For example, CNTs could be used to make stronger and lighter aircraft engines and components.Thermal ConductivityCNTs also have a high thermal conductivity, which makes them excellent heat conductors. This means that CNTs can quickly transfer heat from one point to another. This makes them ideal for use in heat sinks, which are used to dissipate heat from electronic devices. Additionally, CNTs can be used to improve the efficiency of energy storage devices, such as batteries and supercapacitors.Electrical ConductivityCNTs are also excellent electrical conductors, which makes them ideal for use in electronics. They have a very high current carrying capacity, which means they can carrya large amount of electricity without overheating. Additionally, they have a low resistance, which means that electrical signals can travel through them quickly and efficiently. CNTs could be used to make faster and more efficient computer chips, as well as more durable electronic components.Chemical StabilityCNTs are also very chemically stable, meaning they are resistant to chemical reactions. This is due to the strong covalent bonds between the carbon atoms in the honeycomb lattice. This property makes CNTs ideal for use in environments with harsh chemicals, such as in the oil and gas industry. They could be used to make stronger and more durable pipes and other components that are needed in these environments.ConclusionIn conclusion, CNTs have many unique and fascinating properties that make them ideal for a wide range of applications. From their incredible tensile strength to their high thermal and electrical conductivity, CNTs are proving to be one of the most promising materials in the world of nanotechnology. As research continues, it is likely that we will discover even more amazing properties of CNTs that could revolutionize the way we live and work.。
- 1、下载文档前请自行甄别文档内容的完整性,平台不提供额外的编辑、内容补充、找答案等附加服务。
- 2、"仅部分预览"的文档,不可在线预览部分如存在完整性等问题,可反馈申请退款(可完整预览的文档不适用该条件!)。
- 3、如文档侵犯您的权益,请联系客服反馈,我们会尽快为您处理(人工客服工作时间:9:00-18:30)。
Carbon nanotubes as a 1D template for the synthesis of air sensitive materials: About the confinement effectJ.P. Tessonnier, G. Winé, C. Estournès, C. Leuvrey, M.J. Ledouxand C. Pham-HuuLaboratoire des Matériaux, Surfaces et Procédés pour la Catalyse (UMR 7515 du CNRS), ECPM-ULP, 25 rue Becquerel, F-67087 Strasbourg Cedex 2, FranceInstitut de Physique et Chimie des Matériaux de Strasbourg (UMR 7504 du CNRS), ULP, 23 ruedu Loess, F-67037 Strasbourg Cedex 2, FranceAbstractCobalt ferrite and cobalt iron nanowires with an average diameter of 50 nm and lengths up to several micrometers were synthesized inside multi-walled carbon nanotubes (MWNTs) under mild reaction conditions, i.e. 100 °C and atmospheric pressure, using an aqueous nitrate precursor salt filling the tubes. The concept of a confinement effect inside carbon nanotubes has beenadvanced to explain the formation of CoFe2O4under such mild reaction conditions. Theformation of caps near the tube tips at the beginning of the nitrate decomposition meant that each nanotube was considered as a closed nanoreactor, in which the reaction conditions could be very different to the macroscopic conditions outside the tube. The subsequent reduction of theCoFe2O4 allowed to obtain CoFe nanowires cast in the carbon nanotubes. These nanowires exhibit a high resistance towards oxidation, whereas bulk CoFe is known to undergo oxidation at room temperature and atmospheric pressure. This phenomenon was attributed to oxygen diffusion problems due to the confinement effect of the carbon nanotubes.Keywords: Nanowires; Multi-walled carbon nanotubes; Confinement effect; Nanoreactor1. Introduction2. Experimental3. Results and discussion4. ConclusionReferences1. IntroductionCarbon nanostructures, nanotubes and nanofibers, the smallest organized form of carbon, have received an increasing scientific interest, from a fundamental point of view as well as for their physical properties, and for several of their potential applications ranging from living matter structure manipulation to nanometer-sized computer circuits [1], [2], [3] and [4], all this since their discovery at the beginning of the last decade by Iijima as a by-product in the arc-discharge synthesis [5]. The inner diameter of the multi-walled carbon nanotubes (MWNTs) can vary from few nanometers to several dozens depending on the nature of the synthesis method, i.e. arc-discharge, laser ablation or chemical vapor deposition [4], [6] and [7]. Both the single-walled carbon nanotubes (SWNTs) and the MWNTs exhibit an extremely high aspect ratio (length-to-diameter ratio) ranging from 30 to more than many thousands [8]. It was expected that the tubular morphology and the high aspect ratio of MWNTs could induce a confinement effect on the gas or the liquids trapped inside the tube leading to completely different physical behavior when compared to conventional bulk material. Several reports recently dealt with the behavior of water trapped inside MWNTs [9], [10], [11], [12], [13], [14] and [15]. The most detailed study was reported by Gogotsi and co-workers [14] and [15]. They reported that water and gas trapped inside the tubes rapidly reached the supercritical state under heating because of the confinement effect induced by the surrounding wall. Nanotubes can also be used to protect sensitive products against aggressive media. Dravid [16] has reported that nickel particles introduced inside the nanotubes were not damaged even after storage for several months in an aqua regia solution.In addition, introduction of foreign elements inside the carbon tubules can give rise to peculiar effects either on the properties of the filling element or of the filled carbon nanotube. Ajayan et al.[17] used the surface-tension effect to fill carbon nanotubes with vanadium oxide, which can be used in the catalytic oxidation process, at relatively high filling temperature, i.e. 1023 K. However, it should be stressed that the filling selectivity remained relatively low, filled and externally coated carbon nanotubes both being present in the final sample. Recently, Xu et al. [18] have reported the filling of single-walled carbon nanotubes with lanthanide halide by heating an intimate mixture of carbon nanotubes and halide above the melting point of this halide. These encapsulated material can be subsequently used in several potential domains including magnetism and catalysis fields.Metallic single elements or alloys of iron and cobalt made up of nanoclusters are extremely useful owing to their peculiar properties compared to the bulk metal or oxide counterparts either for magnetic or catalysis applications [19], [20], [21] and [22]. However, the use of metallic nanocluster materials was hampered by the high oxidation behavior of these materials in the presence of air.The aim of the present work is to report the use of multi-walled carbon nanotubes as a templatewith a confinement effect for the synthesis of CoFe2O4nanowires under mild conditions and theirsubsequent transformation into CoFe alloy nanowires. Thanks to the high aspect ratio of the MWNT template, the metallic alloy exhibits a relatively high resistance towards oxidation in air, allowing an easy handling and storage. This work is also motivated by the possible application of these metallic alloy nanowires directly as catalyst.2. ExperimentalPurified open MWNTs with mean outer and inner diameters of 100 and 60 nm, respectively, and lengths up to several micrometers (Applied Surface Science, OH, USA) were used as template.The CoFe2O4nanowires were synthesized as follows: the iron and cobalt precursors salts (14.47 gFe(NO3)3·9H2O and 4.94 g of Co(NO3)2·6H2O) were first dissolved in a volume of water and ethanol (60 ml, 90% distilled water and 10% ethanol) corresponding to the inner volume of the carbon tubule, previously determined by analysis of a statistical sample of microscopy images. Carbon nanotubes (10 g) were then added without further purification. The metal loading was set to be ca. 30%. The filling of the carbon nanotubes was possible thanks to capillary forces, the surface tension of the solution (<72 mN m−1) being lower than the critical surface tension reported in the literature for the carbon nanotubes (190 mN m−1). The filled material was then dried in air in a oven (Nabertherm model L3/11/P320) at 100 °C for 2 h in order to remove the excess of water.The calcination of the sample at 450 and 600 °C was performed in a Thermolyne 21100 furnaceequipped with a tubular quartz reactor, respectively, under flowing air and flowing helium(50 cm3 min−1, heating rate 10 °C min−1), during 2 h. The reduction of the CoFe2O4into CoFewas performed in the same furnace under flowing hydrogen (50 cm3 min−1) at 400 °C during 2 h (heating rate 10 °C min−1).The crystallinity of the sample was characterized by powder X-ray diffraction technique (XRD) using a non-monochromatic Co Kα source. The location of the sample with respect to the carbon nanotubes and the microstructure was followed by means of transmission electron microscopy (TEM) with a Topcon 002B microscope working at 200 kV accelerating voltage with a point-to-point resolution of 0.17 nm.3. Results and discussionThe drying of the filled material in air at 100 °C for 2 h induced the formation of a needle-likeCoFe2O4with a short-range crystallinity (Fig. 1A). Increasing the heating temperature from 100to 450 °C led to the modification of the nanowire microstructure, i.e. the hair-like CoFe2O4 was transformed into a more crystallized material (Fig. 1B). The nanowire microstructure again drastically changed when the heating temperature was increased up to 600 °C (Fig. 1C).Fig. 1. In situ TEM micrographs recorded as a function of the heating temperature ofthe CoFe2O4cast inside MWNT: (A) 100 °C; (B) 450 °C; and (C) 600 °C.The CoFe2O4particles of the sample after heat treatment at 600 °C became big enough to bedetected by the XRD technique. The mean particle size deduced from the Scherrer formula was ca. 35 nm, which was still relatively small owing to the reduction temperature. The details of the synthesis procedure have already been published elsewhere [23].The formation of the CoFe2O4 hair-like structure after heat treatment at 100 °C of the impregnated sample was attributed to the confinement effect of the surrounding MWNT. After 10 min of heating at 100 °C, part of the water was vaporized leading to the formation of a semi-permeableamorphous CoFe2O4solid cap at the tube tip, which in turn modifies the pressure inside the tube(Fig. 2A). The non-decomposed nitrate precursor remains trapped inside the tubes and continues to decompose under a higher local pressure leading, after 30 min at 100 °C, to the formation of a low ordered CoFe2O4 nanowire with a hair-like microstructure (Fig. 2B).Fig. 2. In situ TEM images of the permeable solid cap, i.e. amorphous CoFe 2O 4phase, formation from the nitrate solution under electron beam heating during 10 min(A) and 30 min (B). The length of the permeable solid cap formed as a function ofheating time was schematized by an arrow with increasing length.Such a pressure increase would be similar to that involved in hydrothermal synthesis of zeolites and probably enhances the rate of CoFe 2O 4 formation from its mother nitrate solution under mild synthesis conditions, i.e. 100 °C. Mössbauer spectrum of 57Fe nuclei was recorded at 4.2 K and confirmed the nature of the phase present in the MWNT tubule after the 30 min heating at 100 °C (Fig. 3).Fig. 3. Mössbauer spectrum recorded at 4.2 K on the nanowires filling the sample ofcarbon nanotubes after air treatment at 100 °C. Experimental points (○), irons ions in octahedral (...) and tetrahedral (—) sites and the resulting fit ().The formation of solid caps at the tube tips led to consider the inside of each nanotube like a closed nanoreactor. The non-decomposed nitrate precursor remaining trapped and blocked by the caps inside the tubes could see local synthesis conditions, different from those outside the tubes.The local pressure inside the tubes, i.e. a nanoscopic pressure, could be greater than the macroscopic pressure which remained unchanged outside the tubes. The strength of MWNTs could allow this pressure increase inside the nanotubes without being destroyed. It has recently been reported by Gogotsi et al. [15] that fluids pressurized around 30 MPa at room temperature could be trapped inside MWNTs with an outer diameter of 100 nm, without having destroyed the tubes. This confinement effect, in terms of increase in the reactant partial pressure inside the tubes, has already been advanced to explain kinetically the gain in catalytic performance when using silicon carbide nanotubes as catalyst support instead of the analogue grain-based material for supporting the phase active for the hydrogen sulfide selective oxidation into elemental sulfur [24].The TEM image of the CoFe alloy cast inside carbon nanotube after reduction of the parentCoFe2O4at 400 °C is presented in Fig. 4. The reduction temperature was deliberately kept lowerthan 600 °C in order to avoid any gazification of the surrounding carbon nanotubes by reaction with hydrogen, which could lead to the formation of methane and thus carburization of the CoFe alloy. Separate experiments carried out (not reported) have shown that the carburization starts at temperature of about 680 °C. The relatively low-temperature reduction allowed the complete retention of the 1D morphology of the material. A high-magnification TEM image (not shown) revealed that the alloy nanowire was made up of small particles with an average diameter of ca. 30–40 nm. Higher magnification in order to obtain further insight into the microstructure of the material was not possible due to the thickness of the material.Fig. 4. The CoFe2O4sample after heat treatment at 600 °C and after reduction inflowing hydrogen at 400 °C. The contrast generated inside the reduced sample wasattributed to the density change when going from CoFe2O4 to CoFe, which lead to theformation of voids inside the nanowire structure.The XRD pattern (Fig. 5) confirmed the complete reduction of the parent CoFe2O4 into its corresponding metallic alloy while the nanowire morphology was completely retained during the reduction process. The average metallic particle size deduced from the X-ray line broadening was around 36 nm, which was in good agreement with that observed by TEM. It is worth noting that the XRD pattern indicates the complete absence of any other phases, i.e. single oxide or carbide, in the samples reduced at temperature up to 400 °C which was not the case for high temperature encapsulation where a significant amount of carbide was observed due to the solid–solid reaction between the encapsulated material and the carbon material or through a gas-phase reaction during the preparation step [25], [26] and [27].Fig. 5. XRD pattern of the CoFe alloy reduced in flowing hydrogen at 400 °C for 2 hshowing the high selectivity of the reduction process.The oxidative resistance of the alloy was evaluated using an in situ high-temperature X-ray technique. The sample was heated in air from room temperature to 500 °C and a XRD pattern was recorded every 50 °C in order to check the phase transformation during heating. The results obtained are presented in Fig. 6. It is worth noting that the same CoFe alloy without carbon nanotubes surrounding was completely oxidized back to its corresponding parent oxide after exposure to air at room temperature. The observed results were in good agreement with those reported in the literature about the extremely high air sensitivity of the metallic alloy nanoclusters.Fig. 6. In situ high-temperature XRD patterns of the CoFe alloy during heating in airat different temperatures. The diffraction line corresponding to the Mo element wasoriginated from the sample holder. It is worth noting that the same sample withoutcarbon nanotube steadily combusted back to the CoFe2O4phase after air exposure atroom temperature.On the other hand, the carbon cast nanoclusters metallic alloy showed a relatively high resistancetowards air oxidation as no trace of CoFe2O4was observed even after heating up to 200 °C. Attemperatures higher than 200 °C, an oxidation process started to occur and a diffraction line corresponding to the CoFe2O4 phase was observed in the XRD pattern. At 350 °C, the complete oxidation occurred and the XRD pattern only showed a diffraction line corresponding to theCoFe2O4phase. The results observed clearly indicate that most ferromagnetic nanoclusters castedinside the multi-walled carbon nanotubes were well protected by the surrounding graphene planes. The high oxidative resistance of the CoFe cast samples could also be attributed to the high aspect ratio of the MWNT, which hindered oxygen diffusion inside the tube under atmospheric pressure and thus prevented the complete oxidation of the cast CoFe nanowire encountered with the naked sample.4. ConclusionIn summary, 1D ferromagnetic nanoclusters can be efficiently prepared using multi-walled carbon nanotubes as a controlling size template by a simple and reproducible method working at relatively low temperature. The as-synthesized sample exhibits a relatively high oxidative resistance as no oxidic peaks were detected even after heating in air up to 200 °C. Apparently, a combination of the high aspect ratio and the small free space between the surrounding carbon nanotube wall and the ferromagnetic alloy hindered oxygen diffusion inside the tube, thus avoiding the excessive oxidation of the sample as observed for its naked metallic counterpart. This example shows the exciting possibility of using such carbon nanomaterials as nanoscale reactors and opens new perspectives for preparing highly anisotropic nanomaterials for catalytic applications. Work is ongoing to check these 1D CoFe metallic alloy as catalyst and will be presented soon.ReferencesM.S. Dresselhaus, G. Dresselhaus and P.C. Eklund, Editors, Science of Fullerenes and Carbon Nanotubes, Academic Press, London (1996).In: T.W. Ebbesen, Editor, Carbon Nanotubes: Preparation and Properties, CRC Press, Boca Raton (1997).H. Dai, Acc. Chem. Res.35 (2002), p. 1045.P.M. Ajayan, Chem. Rev.99 (1999), p. 1787.J.M. Bonard, L. Forro, D. Ugarte, W.A. de Herr and A. Châtelain, ECC Res.1 (1998), p. 9 and references therein.K.T. Lau and D. Hui, Compos.: Part B Eng.33 (2002), p. 263 and references therein.E.T. Thostenson, Z. Ren and T.W. Chou, Compos. Sci. Technol.61 (2001), p. 1899.S. Iijima, Nature (London)354 (1991), p. 56.C. Laurent, E. Flahaut, A. Peigney and A. Rousset, New J. Chem. (1998), p. 1229 and references therein.Y.H. Hu and E. Ruckenstein, J. Catal.134 (1999), p. 298.M.A. Ermakova, D.Y. Ermakov, G.G. Kuvshinov and L.M. Plyasova, J. Catal.187 (1999), p. 77. V. Lordi and N. Yao, J. Mater. Res.15 (2000), p. 2770.K. Koga, G.T. Gao, H. Tanaka and X.C. Zeng, Nature412 (2001), p. 802.M.S.P. Sansom and P.C. Biggin, Nature414 (2001), p. 156.M.C. Gordillo and J. Marti, Chem. Phys. Lett.341 (2001), p. 250.R.E. Tuzun, D.W. Noid, B.G. Sumpter and R.C. Merkle, Nanotechnology7 (1996), p. 241.(a) J. Libera and Y. Gogotsi, Carbon39 (2001), p. 1307.(b) Y. Gogotsi, N. Naguib and J.A. Libera, Chem. Phys. Lett.365 (2002), p. 354.Y. Gogotsi, J.A. Libera, A.G. Yazicioglu and C.M. Megaridis In: A.M. Rao, Editor, Nanotubes and Related Materials, Materials Research Society Symposium Proceedings, vol. 633 Pittsburgh, Pennsylvania (2001), pp. A7.4.1–A7.4.6.V.P. Dravid, Nature374 (1995), p. 602.P.M. Ajayan, O. Stephan, Ph. Redlich and C. Colliex, Nature375 (1995), p. 564.C. Xu, J. Sloan, G. Brown, S. Bailey, V.C. Williams, S. Friedricks, K.S. Coleman, E. Flahaut, J.L. Hutchison, R.E. Dunin-Borkowski and M.L.H. Green, Chem. Commun. (2000), p. 2427.X. Li and S. Takahashi, J. Magn. Magn. Mater.214 (2000), p. 195.C. Pham-Huu, B. Madani, P. Leroi, S. Savin-Poncet, J.L. Bousquet, PCT/FR04/03380 (2003). G.H. Lee, S.H. Huh, J.W. Jeong and H.C. Ri, J. Magn. Magn. Mater.246 (2002), p. 404.A.E. Berkowitz and R.M. White, Mater. Sci. Eng. B3 (1989), p. 413.C. Pham-Huu, N. Keller, C. Estournès, G. Ehret and M.J. Ledoux, Chem. Commun. (2002), p. 1882.J.M. Nhut, R. Vieira, L. Pesant, J.P. Tessonnier, N. Keller, G. Ehret, C. Pham-Huu and M.J. Ledoux, Catal. Today76 (2002), p. 11.Z.H. Wang, C.J. Choi, B.K. Kim, J.C. Kim and Z.D. Zhang, Carbon41 (2003), p. 1751.X.L. Dong, Z.D. Zhang, Y.C. Chuang and S.R. Jin, Phys. Rev. B60 (1999), p. 3017.X.C. Sun, A. Gutierrez, M.J. Yacaman, X.L. Dong and S. Jin, Mater. Sci. Eng. A286 (2000), p. 157.Corresponding author. Tel.: +33 3 90 24 26 75; fax: +33 3 90 24 26 74.Member of ELCASS (European Laboratory for Catalysis and Surface Science).Original text : 。