Hydrothermal preparation of tobermorite from blast furnace slag for Cs+ and Sr2+ sorption
配制高锰酸钾溶液应用
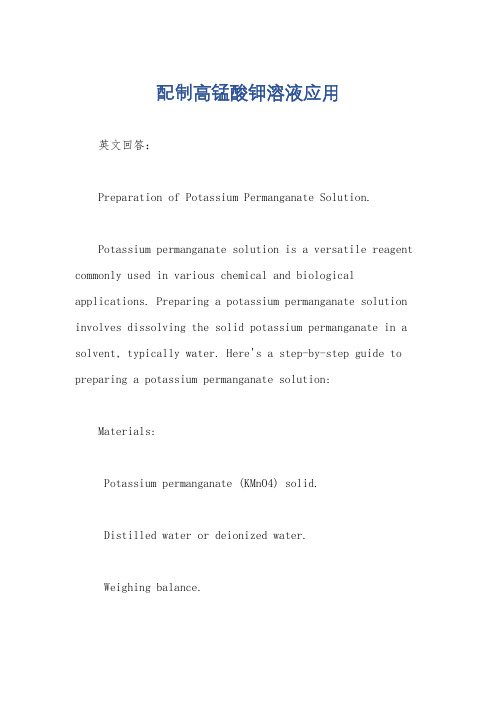
配制高锰酸钾溶液应用英文回答:Preparation of Potassium Permanganate Solution.Potassium permanganate solution is a versatile reagent commonly used in various chemical and biological applications. Preparing a potassium permanganate solution involves dissolving the solid potassium permanganate in a solvent, typically water. Here's a step-by-step guide to preparing a potassium permanganate solution:Materials:Potassium permanganate (KMnO4) solid.Distilled water or deionized water.Weighing balance.Measuring cylinder or graduated flask.Volumetric flask.Stirring rod or magnetic stirrer.Procedure:1. Calculate the amount of potassium permanganate:Determine the desired concentration of the potassium permanganate solution in terms of molarity (M). Calculate the mass of potassium permanganate required using the following formula:Mass (g) = Molarity (M) x Volume (mL) x Molecular weight (g/mol) of KMnO4。
Hydrothermal preparation of nanotubular particles of a nickel phyllosilicate
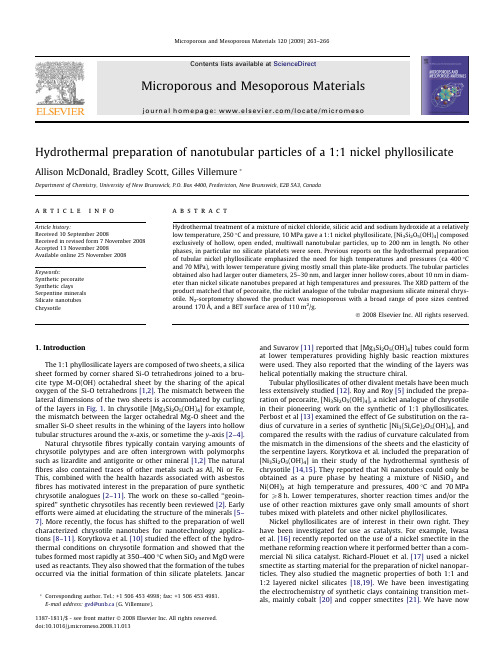
Hydrothermal preparation of nanotubular particles of a 1:1nickel phyllosilicateAllison McDonald,Bradley Scott,Gilles Villemure *Department of Chemistry,University of New Brunswick,P.O.Box 4400,Fredericton,New Brunswick,E2B 5A3,Canadaa r t i c l e i n f o Article history:Received 10September 2008Received in revised form 7November 2008Accepted 13November 2008Available online 25November 2008Keywords:Synthetic pecoraite Synthetic claysSerpentine minerals Silicate nanotubes Chrysotilea b s t r a c tHydrothermal treatment of a mixture of nickel chloride,silicic acid and sodium hydroxide at a relatively low temperature,250°C and pressure,10MPa gave a 1:1nickel phyllosilicate,[Ni 3Si 2O 5(OH)4]composed exclusively of hollow,open ended,multiwall nanotubular particles,up to 200nm in length.No other phases,in particular no silicate platelets were seen.Previous reports on the hydrothermal preparation of tubular nickel phyllosilicate emphasized the need for high temperatures and pressures (ca 400°C and 70MPa),with lower temperature giving mostly small thin plate-like products.The tubular particles obtained also had larger outer diameters,25–30nm,and larger inner hollow cores,about 10nm in diam-eter than nickel silicate nanotubes prepared at high temperatures and pressures.The XRD pattern of the product matched that of pecoraite,the nickel analogue of the tubular magnesium silicate mineral chrys-otile.N 2-sorptometry showed the product was mesoporous with a broad range of pore sizes centred around 170Å,and a BET surface area of 110m 2/g.Ó2008Elsevier Inc.All rights reserved.1.IntroductionThe 1:1phyllosilicate layers are composed of two sheets,a silica sheet formed by corner shared Si-O tetrahedrons joined to a bru-cite type M-O(OH)octahedral sheet by the sharing of the apical oxygen of the Si-O tetrahedrons [1,2].The mismatch between the lateral dimensions of the two sheets is accommodated by curling of the layers in Fig.1.In chrysotile [Mg 3Si 2O 5(OH)4]for example,the mismatch between the larger octahedral Mg-O sheet and the smaller Si-O sheet results in the whining of the layers into hollow tubular structures around the x -axis,or sometime the y -axis [2–4].Natural chrysotile fibres typically contain varying amounts of chrysotile polytypes and are often intergrown with polymorphs such as lizardite and antigorite or other mineral [1,2]The natural fibres also contained traces of other metals such as Al,Ni or Fe.This,combined with the health hazards associated with asbestos fibres has motivated interest in the preparation of pure synthetic chrysotile analogues [2–11].The work on these so-called ‘‘geoin-spired”synthetic chrysotiles has recently been reviewed [2].Early efforts were aimed at elucidating the structure of the minerals [5–7].More recently,the focus has shifted to the preparation of well characterized chrysotile nanotubes for nanotechnology applica-tions [8–11].Korytkova et al.[10]studied the effect of the hydro-thermal conditions on chrysotile formation and showed that the tubes formed most rapidly at 350–400°C when SiO 2and MgO were used as reactants.They also showed that the formation of the tubes occurred via the initial formation of thin silicate platelets.Jancarand Suvarov [11]reported that [Mg 3Si 2O 5(OH)4]tubes could form at lower temperatures providing highly basic reaction mixtures were used.They also reported that the winding of the layers was helical potentially making the structure chiral.Tubular phyllosilicates of other divalent metals have been much less extensively studied [12].Roy and Roy [5]included the prepa-ration of pecoraite,[Ni 3Si 2O 5(OH)4],a nickel analogue of chrysotile in their pioneering work on the synthetic of 1:1phyllosilicates.Perbost et al [13]examined the effect of Ge substitution on the ra-dius of curvature in a series of synthetic [Ni 3(Si,Ge)2O 5(OH)4],and compared the results with the radius of curvature calculated from the mismatch in the dimensions of the sheets and the elasticity of the serpentine layers.Korytkova et al.included the preparation of [Ni 3Si 2O 5(OH)4]in their study of the hydrothermal synthesis of chrysotile [14,15].They reported that Ni nanotubes could only be obtained as a pure phase by heating a mixture of NiSiO 3and Ni(OH)2at high temperature and pressures,400°C and 70MPa for P 8h.Lower temperatures,shorter reaction times and/or the use of other reaction mixtures gave only small amounts of short tubes mixed with platelets and other nickel phyllosilicates.Nickel phyllosilicates are of interest in their own right.They have been investigated for use as catalysts.For example,Iwasa et al.[16]recently reported on the use of a nickel smectite in the methane reforming reaction where it performed better than a com-mercial Ni silica catalyst.Richard-Plouet et al.[17]used a nickel smectite as starting material for the preparation of nickel nanopar-ticles.They also studied the magnetic properties of both 1:1and 1:2layered nickel silicates [18,19].We have been investigating the electrochemistry of synthetic clays containing transition met-als,mainly cobalt [20]and copper smectites [21].We have now1387-1811/$-see front matter Ó2008Elsevier Inc.All rights reserved.doi:10.1016/j.micromeso.2008.11.013*Corresponding author.Tel.:+15064534998;fax:+15064534981.E-mail address:gvd@unb.ca (G.Villemure).Microporous and Mesoporous Materials 120(2009)263–266Contents lists available at ScienceDirectMicroporous and Mesoporous Materialsjournal homepage:www.elsevi e r.c o m /l o c a t e /m i c r o m e soundertaken the hydrothermal synthesis of clays containing nickel. We report here the preparation of nanotubular particles of a1:1 nickel phyllosilicate.They were obtained at a lower temperature, and a much lower pressure than in previous reports.2.Experimental sectionAll chemicals were obtained from Aldrich(Milwaukee,WI)and used as received.The nickel clay was prepared by hydrothermal treatment of a mixture of nickel chloride,silicic acid and NaOH. Briefly,3.21g(13.5mmol)NiCl2Á6H2O was added to a suspension of0.70g(9.0mmol)silicic acid dispersed in200mL of distilled water under a nitrogen atmosphere.This was followed by drop wise addition of67.5mL of a1.0M NaOH solution over a period of10min to give a reaction mixture containing molar ratios Ni/ Si=1.5and OHÀ/Ni=5.The mixture was stirred for3days under N2,transferred to a Parr autoclave and purged several times with argon.The autoclave was then charged with500psi argon and heated to250°C for18h.At temperature,the pressure in the auto-clave was approximately10MPa.The green powder obtained was separated by centrifugation,washed with distilled water and dried in vacuum.The product was characterized by powder X-ray diffraction (XRD),transmission electron microscopy(TEM),electron diffrac-tion(ED),N2-sorptometry,thermo gravimetric analysis(TGA)and FTIR spectroscopy.The powder X-ray diffraction pattern was ob-tained on a Bruker D8X-ray diffractometer using CuK a radiation at40kV and20mA and a scanning rate of0.042h sÀ1.The trans-mission electron micrographs were taken on a JEOL2011scanning transmission electron microscope(STEM)operating at200keV. The micrographs were recorded digitally using a CCD camera(Ga-tan4kx4k Ultrascan).The clay was dispersed in ethanol,the sus-pension was sonicated and small drops($20l L)placed onto TEM copper grids previously coated with a thin Formvarfilm.Car-bon was evaporated onto the prepared grids.The electron diffrac-tion patterns were obtained by the fast Fourier transform(FFT)of the scanning mode TEM images of the sample.TEM/EDX elemental analysis of the product was done on the same instrument using an EDAX(Genesis)energy dispersive X-ray system.The nitrogen adsorption-desorption isotherm was taken on a Quantachrome Autosorb-1instrument using a static adsorption procedure.The powder was out gassed at120°C for20h before the measurements.The TGA was taken with Q50TGA from TA instruments.The sample was heated from room temperaturetoFig.2.TEM micrographs of the nickel1:1phyllosilicate,(A)general view,(B)side view of a tubular particle,(C)higher magnification view showing the hollow multiwall tubular structure and(D)end-on view of one of the tubes(Inset:electron diffractionpattern).Fig.1.Schematic representation of the two sheets,the octahedral M-O(OH)andtetrahedral Si-O sheets forming the layers of1:1phyllosilicates showing how themismatch between the lateral dimensions of the two sheets is compensated bycurling of the layers.Dimensions of the two sheets are from reference[13].264 A.McDonald et al./Microporous and Mesoporous Materials120(2009)263–266800°C at 5°C/min in flowing N 2(40mL/min).The FTIR spectra were recorded in total attenuated reflection mode (ATR-FTIR)using a flat ZnSe crystal plate on a NEXUS 470FT-TR thermo instrument equipped with Smart Performer of Thermo Nicolet Smart Accessories.3.Results and DiscussionFig.2shows TEM micrographs of the green powder obtained.The low magnification view in Fig.2A shows the clay consisted of short nanotubular particles,up to 200nm in length.No other phases,in particular no platelets were seen.At higher magnifica-tion in Figs.2B and 2C,the hollow,open-ended multiwall tubular structure of the particles was clearly observed.The outer diameters of the tubes ranged from 25–30nm.They were typically wider in the centres tapering off toward the ends,as might be expected for structures formed by the rolling of layers.The inner diameters were 10nm or more.These values for both the outer and inner diameters of the tubes are larger than those reported by Korytkova et al [15]for [Ni 3-Si 2O 5(OH)4]tubes prepared at high temperatures and pressures.They reported outer diameters of 10–20nm and inner diameters of only 2–3nm for nanotubes obtained at 400°C and 70MPa.The nickel phyllosilicate layers in the tube prepared here appeared not to have been as tightly rolled up as in tubes made at higher temperatures.The dimensions of the tubes prepared here are in fact more comparable to those reported for natural chrysotile fi-bres,outer diameters of 22–27nm,with 7nm hollow cores [22,23].The tubular particles obtained also have radius of curva-ture closer to what has be reported to be required to accommodate the mismatch between the dimensions of the Si-O tetrahedral and Ni-O octahedral sheets in 1:1nickel clays.Perbost et al.[13]esti-mated that nickel 1:1phyllosilicate particles should have a radius of curvature of about 9nm,based on the mismatch in the lateral dimensions of the two sheets and the elasticity of the serpentine layers.The spacing between the layers in the tube walls was just under 0.7nm in Fig.2C and a typical tube wall consisted of approxi-mately 15layers.Fig.2shows a head-on view of one tube.The electron diffraction pattern gave several rings,with the most in-tense reflections corresponding to lattice spacings of 4.56,3.61,2.51,1.52and 1.30Å.TEM/EDX analysis of several points of thesample gave consistent Si/Ni atomic ratios of 1.51±0.07,very close to the expected ratio for a trioctahedral 1:1nickel phyllosilicate.Fig.3shows the powder XRD pattern of the product.Prominent peaks at 7.31,3.64,2.64and 1.53Åand broader reflections at 4.52and 2.45Åwere observed.The pattern matched well that of the tubular nickel serpentine mineral pecoraite (JCPDS #49-1859),allowing these peaks to be indexed as the 002,110,004,200,202and 060reflections.The ATR FTIR spectrum of the powder was also consistent with that of pecoraite [24].A strong band at 958cm À1with shoulder at 1002cm À1and a less intense band at 1072cm À1assigned to Si-O stretching vibrations were observed.A broad band centred near 650cm À1maybe assigned to Si-O-Si bridging vibration overlapping with NiOH librations [24].Sharp bands at 3648and 3610cm À1were assigned to inner and inner surface OH stretching bands.Fig.4shows the N 2adsorption-desorption isotherm of the prod-uct.A type IV isotherm was obtained,indicating a mesoporous material [25].The hysteresis loop between the two branches did not close completely until the relative pressure P /P o had returned to 0.2in the desorption branch,indicating a broad distribution of pore sizes.This was confirmed by the BJH pore sized distribution obtained from the desorption branch (inset).A broad distribution of pore sizes ranging from 30Åto more than 350Åwas obtained with a maximum near 170Å.The cumulative pore volume was large,0.45cm 3/g.The BET surface area of the product,110m 2/g was similar to BET surface areas that have reported for chrysotile type materials [6].The TGA curve of the sample showed a very gradual small weight loss of less than 2%on heating the powder from room tem-perature up to about 400°C.This was followed by a steeper addi-tional weight loss of about 8%between 400°C and 650°C.This is consistent with the dehydroxylation of [Ni 3Si 2O 5(OH)4]to anhy-drous [Ni 3Si 2O 7]by the loss of two water molecules [9,26].4.ConclusionNanotubular particles of a 1:1nickel phyllosilicate were ob-tained as the sole product of the hydrothermal treatment of a mix-ture of nickel chloride,silicic acid and NaOH at relatively low temperature and pressure,250°C and 10MPa.In particular,no evi-dence of the presence of plate-like particles was seen in the prod-uct.Previous reports on the hydrothermal preparation oftubularFig.3.Powder XRD diffraction of the nickel nanotubularparticles.Fig. 4.Nitrogen adsorption-desorption isotherm of the synthetic 1:1nickel phyllosilicate.Inset:Pore size distribution calculated for the desorption branch using the BJH model.A.McDonald et al./Microporous and Mesoporous Materials 120(2009)263–266265nickel phyllosilicate emphasized the need for high temperatures and pressures(ca400°C and70MPa)with lower temperature giv-ing mostly small thin plate-like products with only small amounts of short tubes.The tubular particles obtained also had larger outer diameters and much larger hollow cores than nickel silicate tubes prepared at high temperature and pressure.AcknowledgmentsWe thank Dr.V.Reddy(UNB,Department of Geology)for the XRD measurements,Dr.L.Weaver(Microscopy and Microanalysis Facility)for help with the recording and interpretation of the TEM and ED and Dr ngmi(UNB,Department of Chemistry)for the TGA measurements.Financial support was provided by the Natural Science and Engineering Research Council of Canada and the Uni-versity of New Brunswick.References[1]F.J.Wicks,D.S.O’Hanley,Serpentine Minerals:Structure and Petrology in:S.W.Bailey(Ed.),Hydrous Phyllosilicates,Review in Mineralogy,vol.19, Mineralogical Society of America,Washington,DC,1988,pp.91(Chapter5).[2]N.Roveri,G.Falini,E.Foresti,I.G.Lesci,P.Sabatino,J.Mater.Res.21(2006)2711.[3]S.Piperno,I.Kaplan-Ashiri,S.R.Cohen,R.Popovitz-Biro,H.D.Wagner,R.Tenne,E.Foresti,I.G.Lesci,N.Roveri,Adv.Funct.Mater.17(2007)3332.[4]J.Xu,X.Li,W.Zhou,L.Ding,Z.Jin,Y.Li,J.Porous Mater.13(2006)275.[5]D.M.Roy,R.Roy,Am.Mineral.19(1954)956.[6]W.Noll,H.Kircher,W.Sybertz,Kolloid-Z.157(1958)1.[7]J.C-H.Yang,Am.Mineral.46(1961)748.[8]G.Fallini,E.Foresti,N.Roveri,mun.(2002)1512.[9]E.Foresiti,M.F.Hochella,H.Kornishi,I.G.Lesci,A.S.Madden,N.Roveri,H.Xu,Adv.Funct.Mater.15(2005)1009.[10]E.N.Korytkova,A.V.Maslov,L.N.Pivovarova,I.A.Drozdova,V.V.Gusarov,GlassPhys.Chem.30(2004)51.[11]B.Jancar,D.Suvorov,Nanotechnology17(2006)25.[12]S.W.Bailey,Structures and Compositions of the other Trioctahedral1:1Phyllosilicates in:S.W.Bailey(Ed.),Hydrous Phyllosilicates,Review in Mineralogy,vol.19,Mineralogical Society of America,Washington,DC,1988, pp.169(Chapter6).[13]R.Perbost,M.Amouric,J.Olives,Clay.Clay Min.51(2003)430.[14]E.N.Korytkova,L.N.Pivovarova,I.A.Drozdova,V.V.Gusarov,Glass Phys.Chem.31(2005)797.[15]E.N.Korytkova, A.V.Maslov,L.N.Pivovarova,Y.V.Polegotchenkova,V.F.Povinich,V.V.Gusarov,Inorg.Mater.41(2005)743.[16]N.Iwasa,M.Takizawa,M.Arai,Appl.Catal.A314(2006)32.[17]M.Richard-Plouet,M.Guillot,S.Vilminot,C.Leuvrey,C.Estournes,M.Kurmoo,Chem.Mater.19(2007)865.[18]M.Richard-Plouet,S.Vilminot,M.Guillot,New J.Chem.28(2004)1073.[19]M.Richard-Plouet,S.Vilminot,M.Guillot,M.Kurmoo,Chem.Mater.14(2002)3829.[20]Y.Xiang,G.Villemure,J.Phys.Chem.100(1996)7143.[21]J.Xiao,G.Villemure,Clay.Clay Min.46(1998)195.[22]K.Yada,Acta Crystallogr.A27(1971)659.[23]B.A.Cressey,E.J.W.Whittaker,J.W.Eric,Mineral.Mag.57(1993)729.[24]R.L.Frost,J.Reddy,M.J.Dicfos,J.Raman Spetrosc.39(2008)909.[25]P.A.Webb, C.Orr,Surface Area and Pore Structure by Gas Adsorption inAnalytical methods in Fine Particle Technology,Micromeritic Instrument Corporation,Norcross,GA1997,pp.53(Chapter3).[26]H.Suquet,Clay.Clay Min.37(1989)439.266 A.McDonald et al./Microporous and Mesoporous Materials120(2009)263–266。
HydrothermalChemistryReverseWeathering
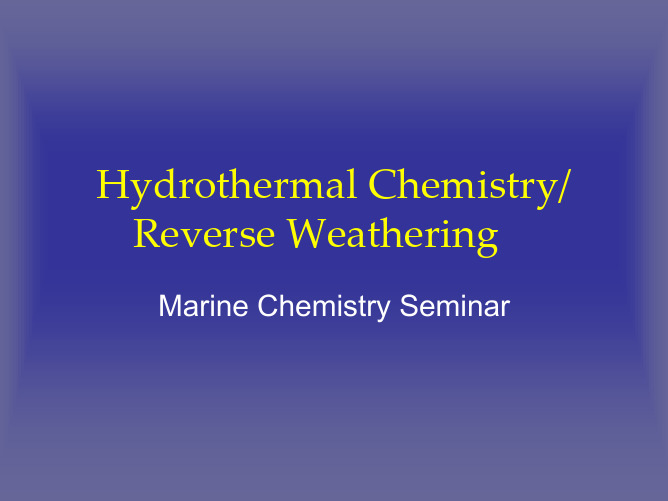
• Used heat flow budgets, heat flow anomalies, endmember compositions, and sediment porewater profiles
• Speculate as to depth of water/rock interactions (penetration of gabbros or dykes): not necessarily resolved, addressed in one of our other papers..
sediment thickness, and thermal conductivity • Chemical compositional changes: sediment
porewater profiles • Basement temperature and chemical flux can
• Low-temperature (3-13 °C) dilution trends are extrapolated out to high temperatures
• He derived heat flow measurements used to calculate elemental fluxes
– keep looking
• Model sediments are atypical
– Other sediments in other places might react with seawater to remove Mg2+
• Igneous rocks react with Mg2+ below the seafloor to form chlorite
水热法制备氢氧化钴及其超电容性能的研究

论文题目:水热法制备氢氧化钴及其超电容性能的研究姓名:院系专业:化学班级:学号:0指导老师:完成时间:目录摘要 (I)Abstract (II)一前言 (1)1.1 超级电容器的研究背景 (1)1.2 超级电容器的性能特点 (1)1.3 电化学电容器的储能原理 (2)1.4 超级电容器研究的电极材料及应用 (3)1.5 选题依据 (3)二实验部分 (5)2.1 试剂与仪器 (5)2.2 样品的制备 (5)2.3 电极的制备及电化学性能的测试 (6)三结果与讨论 (7)3.1 不同沉淀剂制备的氢氧化钴电化学性质研究 (7)3.1.1 循环伏安测试 (7)3.1.2 恒流充放电测试 (8)3.1.3 交流阻抗测试 (7)3.1.4 小结 (8)3.2 不同投料比的氢氧化钴电化学性质研究 (8)3.2.1 循环伏安测试 (8)3.2.2 恒流充放电测试 (9)3.2.3 交流阻抗测试 (11)3.2.4 小结 (11)四结论 (11)参考文献 (11)致谢 (19)摘要利用水热法,以硝酸钴为原料,氢氧化钠、醋酸钠、尿素为沉淀剂,制备了氢氧化钴。
通过循环伏安、恒电流充放电等测试表明,以醋酸钠为沉淀剂、反应温度为100℃时,氢氧化钴电极在1 mol·L-1氢氧化钾溶液中和-0.05~0.45通过电化学性能测试,得出醋酸钠与硝酸钴摩尔比为1:1时单电极比电容最大达184 F·g-1,电化学性能较好。
关键词:;超级电容器;电容性能AbstractUsing the hydrothermal method, using cobalt nitrate as raw materials, sodium hydroxide, sodium acetate, urea as precipitating agent, cobalt hydroxide was prepared. Shown by cyclic voltammetry, constant current charge-discharge test, with sodium acetate as precipitating agent, the reaction temperature is 100 ℃, the cobalt hydroxide electrode in 1 mol · L-1potassium hydroxide solution and -0.05~0.45 V (vs. feeding hydrogen oxidation ratio of the drill, the electrochemical performance tests, the molar ratio of sodium acetate and cobalt nitrate as 1:1 single electrode capacitance than the maximum up to 184 F · g-1, good electrochemical performance.Keywords: Hydrothermal; Super capacitor; Capacitance performance一前言1.1超级电容器的研究背景在环境污染严重,能源匮乏的今天,要求尽快改善人类生存环境的呼声日益高涨,因此,对无污染新能源的开发、利用及存储成为众多学者的研究热点之一。
水能 高中英语作文
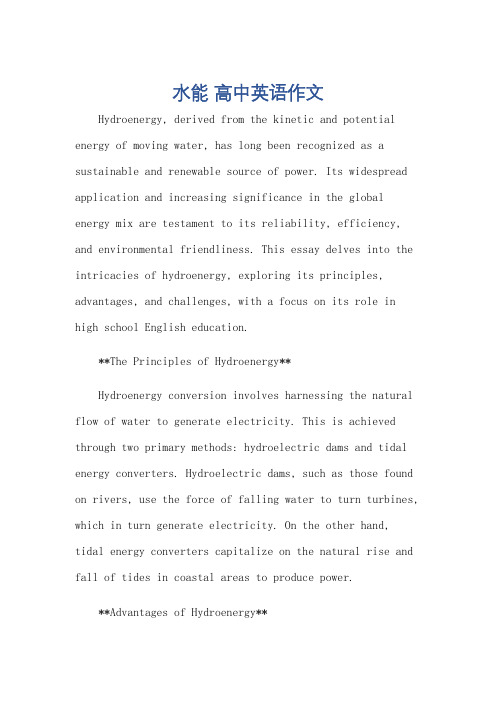
水能高中英语作文Hydroenergy, derived from the kinetic and potential energy of moving water, has long been recognized as a sustainable and renewable source of power. Its widespread application and increasing significance in the global energy mix are testament to its reliability, efficiency, and environmental friendliness. This essay delves into the intricacies of hydroenergy, exploring its principles, advantages, and challenges, with a focus on its role in high school English education.**The Principles of Hydroenergy**Hydroenergy conversion involves harnessing the natural flow of water to generate electricity. This is achieved through two primary methods: hydroelectric dams and tidal energy converters. Hydroelectric dams, such as those found on rivers, use the force of falling water to turn turbines, which in turn generate electricity. On the other hand,tidal energy converters capitalize on the natural rise and fall of tides in coastal areas to produce power.**Advantages of Hydroenergy**The appeal of hydroenergy lies in its numerous advantages. Firstly, it is a renewable resource, meaning it can be replenished naturally, ensuring a sustainable supply of energy. Secondly, hydroenergy has a low carbon footprint, resulting in reduced greenhouse gas emissions and a smaller impact on climate change. Furthermore, it is a reliable source of power, as water flows are relatively consistent and can be predicted with accuracy. Lastly, hydroenergy can be harnessed in both rural and urban settings, providing a versatile solution for meeting energy needs.**Challenges of Hydroenergy**Despite its numerous advantages, hydroenergy faces several challenges that limit its widespread application. One significant hurdle is the high initial capital investment required for dam construction and turbine installation. Additionally, the environmental impact of dams, including displacement of local communities and disruption of ecological systems, cannot be overlooked. Furthermore, the geographical limitations of tidal energy converters restrict their deployment to coastal areas.**The Role of Hydroenergy in High School English Education**Incorporating hydroenergy into high school English curricula offers students an engaging and relevant way to learn about renewable energy. Through reading and writing activities centered around hydroenergy, students can gain a deeper understanding of its principles, advantages, and challenges. This not only enhances their knowledge of science and technology but also cultivates their critical thinking and analytical skills.Moreover, discussing hydroenergy in an English classroom promotes cross-curricular learning, allowing students to apply their knowledge of language arts to real-world issues. Such integrated approaches foster a comprehensive understanding of complex topics, preparing students for the challenges of the 21st century.**Conclusion**In conclusion, hydroenergy represents a promising and sustainable solution for meeting our energy needs. Its potential to generate clean and renewable power isessential in the fight against climate change andenvironmental degradation. By integrating hydroenergy into high school English education, we can empower the next generation with the knowledge and skills necessary to shape a sustainable future.**水能的潜力:全球视角**水能,源自流动水的动能和势能,长期以来一直被视为一种可持续和可再生的能源。
虾加工副产物抗菌型水解液制备条件优化及抑菌作用

谷萝,宋茹. 虾加工副产物抗菌型水解液制备条件优化及抑菌作用[J]. 食品工业科技,2024,45(3):162−170. doi:10.13386/j.issn1002-0306.2023050066GU Luo, SONG Ru. Optimization of Hydrolysis Conditions and Antibacterial Activity of Hydrolysate from Shrimp Processing By-products[J]. Science and Technology of Food Industry, 2024, 45(3): 162−170. (in Chinese with English abstract). doi:10.13386/j.issn1002-0306.2023050066· 生物工程 ·虾加工副产物抗菌型水解液制备条件优化及抑菌作用谷 萝,宋 茹*(浙江海洋大学食品与药学学院,浙江舟山 316022)摘 要:目的:为了探究水产品加工副产物用于抗菌型水解液制备,并研究其抑菌作用,本文以南美白对虾优势腐败菌(Specific spoilage organisms of Penaeus vannamei ,PV-SSOs )为实验用菌,采用虾加工副产物制备抗菌型水解液(Antibacterial hydrolysate from shrimp processing by-products ,SPPH ),考察对PV-SSOs 的抑菌作用。
方法:以中华管鞭虾加工副产物为原料,分别采用5种蛋白酶水解,测定水解液对PV-SSOs 的抑菌作用。
以抑菌效果最强酶为实验用酶,研究加酶量、酶解时间、酶解温度和料液比对PV-SSOs 的抑菌效果影响,然后采用响应面法优化SPPH 酶解条件,高效液相色谱法分析SPPH 中肽段分子量分布,采用膜渗漏法测定SPPH 对PV-SSOs 的细胞膜渗透性影响,进一步通过扫描电子显微镜观察SPPH 作用后PV-SSOs 菌体微观结构变化。
【精品】外文翻译(中文)化学浴沉积法制备金属氧化物薄膜
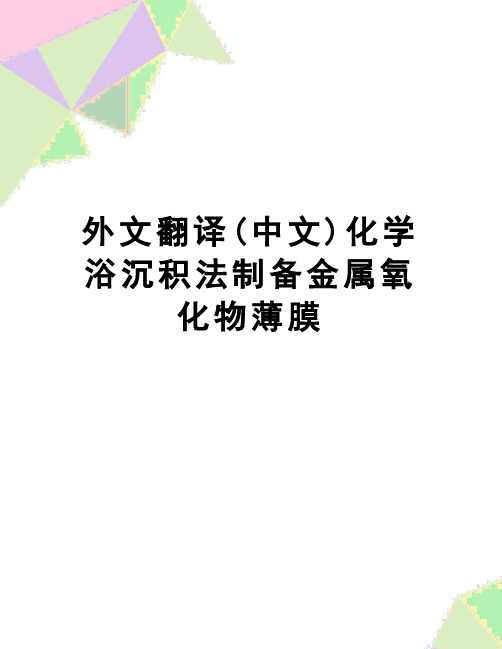
外文翻译(中文)化学浴沉积法制备金属氧化物薄膜化学浴沉积法制备金属氧化物薄膜R.S. Mane, C.D. Lokhande薄膜物理实验室,印度希瓦吉大学,Kolhapur416004,收到1999年7月22日,经修订的表格1999年12月28日收到;接受2000年1月3日。
------------------------------------------------------------------------------------------------------------------------- 摘要由化学方法制备金属氧化物薄膜的方法目前受到很大的关注,它相对因为这些是避免基体的氧化和侵蚀的低温程序,很多的基体,像是绝缘体、半导体或金属,能被利用。
这些是用改良的晶粒组织促进晶体较好的定方位的缓慢的过程。
根据沉积条件的不同,膜的生长可以采取离子对基材的材料凝结或从底物上的胶体粒子吸附的地方。
使用这些方法,II-VI,V-VI,III-VI的薄膜等已沉积出来。
太阳能选择性涂层,太阳能控制,光电导,固态及光电太阳能电池,光学成像,全息图记录,光大容量存储器等都是金属硫薄膜的一些应用。
在本综述中,我们有详细的介绍,化学浴金属硫系薄膜沉积法,它有高产优质薄膜的能力。
他们的制备参数,结构,光学,电学性能等进行了描述。
我们还讨论了化学浴沉积法制备薄膜的理论背景。
关键词:金属硫族化合物薄膜、薄固体、化学浴沉积-------------------------------------------------------------------------------------------------------------------------1 简介薄膜材料在不同的领域有很多应用。
他们有些是A.R.涂料、干扰滤波器、polarisers,狭带滤波器、日光电池,光导体, photoconductors,探测器,波导涂料,卫星的温度控制,光热太阳能涂层例如黑铬,镍,钴,等等。
蒽醌法双氧水生产英文工艺流程翻译
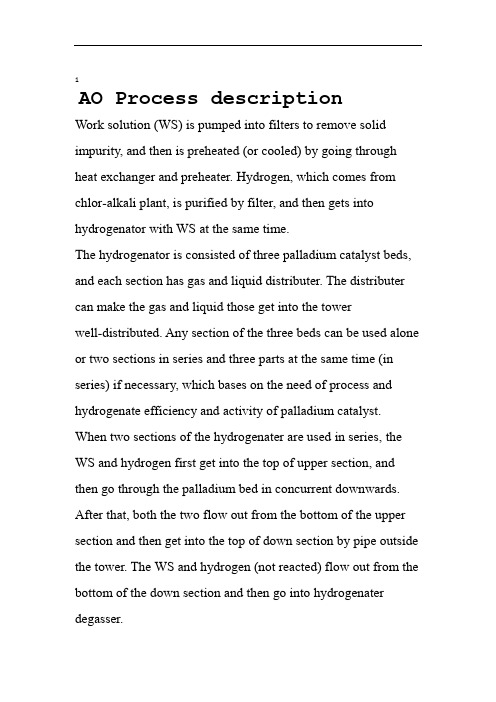
1AO Process descriptionWork solution (WS) is pumped into filters to remove solid impurity, and then is preheated (or cooled) by going through heat exchanger and preheater. Hydrogen, which comes from chlor-alkali plant, is purified by filter, and then gets into hydrogenator with WS at the same time.The hydrogenator is consisted of three palladium catalyst beds, and each section has gas and liquid distributer. The distributer can make the gas and liquid those get into the towerwell-distributed. Any section of the three beds can be used alone or two sections in series and three parts at the same time (in series) if necessary, which bases on the need of process and hydrogenate efficiency and activity of palladium catalyst. When two sections of the hydrogenater are used in series, the WS and hydrogen first get into the top of upper section, and then go through the palladium bed in concurrent downwards. After that, both the two flow out from the bottom of the upper section and then get into the top of down section by pipe outside the tower. The WS and hydrogen (not reacted) flow out from the bottom of the down section and then go into hydrogenater degasser.Hydrogenated WS (HWS) and the not reacted hydrogen, which together come out from the hydrogenator, go into hydrogenator degasser. The gas flows out from the top, and then gets into condenser. Condensate goes into receiver tank, and off gas is exhausted after controlling the flow by flow meter.Determined level of the degasser is controlled by auto valve. 10% HWS2first gets into alumina bed, and then together with other 90% HWS go through filters to remove solid impurity. After that, HWS going through heat exchanger and then gets into tank.Little hydrogen or other gases, which dissolve in the HWS, resolve in the tank. The gases then go into condenser, and are exhausted by evacuation water seal and flame arrester.Part of the hydrogenated WS, which flows out from the hydrogenator degasser, is pumped back into the hydrogenator. This can make the temperature of the tower well-distributed, and hydrogenated efficiency steady and operation safe. Hydrogenated WS (HWS) and phosphoric acid are pumped into HWS cooler, in which the temperature of HWS is cooled downto 50~55℃, and then both get into upper part of oxidizer. The HWS is stored in HWS tank, and the phosphoric acid is storedin phosphoric acid mixing tank. Phosphoric acid is pumped bymetering pump.There are three hollow sections of the oxidizer and the air is introduced into the tower from the bottom of middle and lower sections separately. The air is distributed into small bubble by gas distributor. Air flow based on oxidized efficiency and the concentration of oxygen in the off gas (normally is 6%-9%). Parts of or all the air, which is introduced into the middle and lower sections, flow into the bottom of the upper part of the oxidizer. The HWS and the air, which get into the bottom of the upper part, are concurrent upwards. HWS is partly oxidized and flows out from the top of the upper section and then gets into the bottom of the middle section. The HWS together with the fresh air are3concurrent upwards. The mixture of air and liquid, which flows out from the top of the upper section, gets into degasser to eliminate air. After that, the liquid gets into the bottom of the lower part and together with the fresh air are concurrent upwards. The mixture of air and liquid, which flows out from the top of the middle section, gets into degasser to eliminate air. Determinate level of the degasser is controlled by auto valve. Oxidized WS (OWS), after cooled by cooler, gets into tank, and then is pumped into the bottom of extractor. Off gas of theoxidizer gets into condenser, and then gas and condensate get into aromatic receiver drum. After that, the gas goes into off gas separator. Liquid is recovered into aromatic recover tank, and the off gas gets into handling unit after the pressure of the top of the tower is controlled by instrument. The temperature of the oxidizer is controlled by controlling flow of cooling water into U type pipe which is contained inside the tower.The oxidized WS, which contains H2O2, gets into the extractor from the bottom of the tower. It is dispersed by sieve plate and then floats upwards. Meanwhile, DMW with phosphoric acid is pumped to the top of the extractor from DMW mixing tank. The flow of the water is controlled by auto valve. The water flows downwards by downcome of the sieve plate, and the oxidized WS and water are countercurrent extraction.In this process, the water works as continuous phase and the oxidized WS as disperse phase. The concentration of H2O2 in DMW is getting higher as the water flows downwards and at last the water flows out from the bottom of the extractor (now the water is called extract). The extract gets into the top of4purification tower by potential difference. The concentration of H2O2 in the oxidized WS is getting lower as it floats upwards. Atlast, it flows out from the top of the extractor (now it is called raffinate).Purification column contains fillings and heavy aromatic. The extract, which gets into the purification column from the top of the column, is dispersed in the tower and flows downward. At the same time, heavy aromatic gets into the bottom of the purification column from the overhead aromatic tank by potential difference. The aromatic and the extract are countercurrent contact to eliminate organic impurity in H2O2. In this process, the heavy aromatic works as continuous phase while the extract as disperse phase. The H2O2, after purification, flows out from the bottom of the purification column and goes into crude H2O2 separator to eliminate aromatic that may be carried by H2O2. After that, the H2O2 goes into metering tank, in which it is blowed by air. And then, the H2O2 is pumped to packing or concentration procedure.The heavy aromatic, which flows out from the top of the purification column,enters into aromatic recovery tank. Attention, for safety consideration, before the aromatic is used for distillation or mixing of WS, it must be washed by DMW to eliminate H2O2 (the concentration of H2O2 must be below 0.15g/L). Raffinateflows out from the top of the extractor, and then goes into raffinate separator to eliminate water. After that, it goes through WS metering tank and then gets into the bottom of alkaline tower. The alkaline tower contains fillings and potassium carbonate solution (40% approximately) goes into the alkaline5tower form the top of the tower. In the alkaline tower, the H2O2 in the WS decomposes, and acid in the WS was neutralized. Besides, the water in the WS can also be further eliminated.The WS, which flows out from the top of the alkaline tower, goes into alkalisettler and then into alkali separator to eliminate potassium carbonate solution. After that, the WS enters into alumina bed which contains activated alumina. The alumina is used to recover anthraquinone degradation. And the potassium carbonate solution can also be absorbed by the alumina. The WS flows out from the top of the alumina bed and gets into WS tank. The WS is then pumped into hydrogenator and another circulation is started.2011-3-10。
- 1、下载文档前请自行甄别文档内容的完整性,平台不提供额外的编辑、内容补充、找答案等附加服务。
- 2、"仅部分预览"的文档,不可在线预览部分如存在完整性等问题,可反馈申请退款(可完整预览的文档不适用该条件!)。
- 3、如文档侵犯您的权益,请联系客服反馈,我们会尽快为您处理(人工客服工作时间:9:00-18:30)。
Journal of Hazardous Materials 266 (2014) 174–181Contents lists available at ScienceDirectJournal of HazardousMaterialsj o u r n a l h o m e p a g e :w w w.e l s e v i e r.c o m /l o c a t e /j h a z m atHydrothermal preparation of tobermorite from blast furnace slag for Cs +and Sr 2+sorptionTakuma Tsutsumi,Shunsuke Nishimoto,Yoshikazu Kameshima,Michihiro Miyake ∗Department of Material and Energy Science,Graduate School of Environmental and Life Science,Okayama University,3-1-1Tsushima-Naka,kita-ku,Okayama 700-8530,Japanh i g h l i g h t s•Al-substituted 11˚Atobermorite could be easily synthesized from blast furnace slag.•The samples consists of tobermorite and calcium silicate hydrate.•The samples exhibited high selective Cs +or Sr 2+removal in the presence of Na +.•The Cs +and Sr 2+removal reaction proceeded by ion-exchange.a r t i c l ei n f oArticle history:Received 2September 2013Received in revised form 16December 2013Accepted 17December 2013Available online 24 December 2013Keywords:Blast furnace slagHydrothermal treatmentAl-substituted 11˚A-tobermoriteCs +and Sr 2+sorptiona b s t r a c tAl-substituted 11˚A-tobermorite was formed by alkaline hydrothermal treatment of blast furnace slagwith sodium silicate added at 180◦C for 2–48h.Effects of the hydrothermal treatment time were charac-terized by XRD,SEM,and isothermal adsorption of N 2.Sorption characteristics of the obtained samples were examined for Cs +and Sr 2+.The sample obtained by hydrothermal treatment for 48h (HT-48h)consisted of calcium silicate hydrate (C–S–H),and Al-substituted 11˚A-tobermorite.The HT-48h showedthe highest performance for Cs +and Sr 2+selectivity in the presence of Na +.The interlayer Na +of Al-substituted 11˚A-tobermorite and surface Ca 2+played an important role in selective Cs +and Sr 2+.© 2014 Elsevier B.V. All rights reserved.1.IntroductionBast furnace (BF)slag is the major by-product of the iron and steel-making processes,and more than 25million tons is produced in Japan every year.Since the chemical composition of BF slag is composed mainly of CaO and SiO 2,it has been widely used for cement production,road construction,etc.However,as the amount of recycled BF slag has saturated these fields,the development of new value added products from such waste slag has been investi-gated in terms of sustainability [1–7].There have been a few reports on the hydrothermal synthesis ofAl-substituted 11˚A-tobermorite,Na x Ca 5Si 6−x Al x O 16(OH)2·4H 2O,from waste BF slag with a silica source added as a recycling method[5–7].Al-substituted 11˚A-tobermorite is a type of calcium silicatehydrate mineral comprising a double Ca–O sheet bound by silicate chains in parallel to the b -axis direction [8–12]and stacking in the∗Corresponding author.Tel.:+8186251890;fax:+81862518906.E-mail address:mmiyake@cc.okayama-u.ac.jp (M.Miyake).c -axis direction.It is known that Al-substituted 11˚Atobermorites possess the ability to remove heavy metal ions [13–20].Moreover,since Al-substituted 11˚Atobermorites bearing labile interlayer alkali metal ions exhibit reversible cation exchange properties,large less hydrated ions such as Cs +are known to be selectively incorporated in the interlayer space.This cation selectivity is use-ful for selective removal of radioactive species,e.g.,137Cs +and 90Sr 2+with long half-lives (about 30years)[21–26].Althoughprevious reports have successfully shown the Al-substituted 11˚A-tobermorite formation,the synthesis procedures and/or conditionshave not been optimized for the development of an efficient ion exchanger.Specifically,the hydrothermal treatment time is an important parameter that should be optimized,because it may have considerable influence on the crystallinity and porosity of the final product.Moreover,there are no reports on the Cs +and Sr 2+removal ability of the Al-substituted 11˚A-tobermorite materialssynthesized from the BF slag.Because of the outflow to the sea of radioactive materials from the Fukushima nuclear power plant destroyed by the Great East Japan Earthquake,further develop-ment of radioactive adsorbents,which are as cheap and efficient0304-3894/$–see front matter © 2014 Elsevier B.V. All rights reserved./10.1016/j.jhazmat.2013.12.024T.Tsutsumi et al./Journal of Hazardous Materials266 (2014) 174–181175as possible,has become an urgent issue.Therefore,in this study, we attempted the synthesis of Al-substituted tobermorite using blast BF slag by hydrothermal treatment at180◦C and investigated the effect of the hydrothermal treatment time on the Cs+and Sr2+ removal performances.2.Experimental2.1.Chemicals and materialsGranulated BF slag supplied by a steel corporation sample was used in this study.The other chemicals used in the tobermorite syn-thesis were NaOH(97.0%,Nacalai Tesque Inc.)and sodium silicate (Nacalai Tesque Inc.).2.2.SynthesisBF slag was ground with a ball mill for4h.Based on a previous report,that showed efficient Al-substituted11˚A-tobermorite for-mation,the molar ratio of the starting Ca–Si–Al system was set to be Ca/[SiO2+Al2O3]=0.80by adding sodium silicate as a silica source [27].The BF slag powder(3g)was well-mixed with NaOH(ca.3.0g) and sodium silicate(2.3g)in deionized water(15cm3).The result-ing slurry was sealed in a Teflon-lined stainless-steel autoclave and kept at180◦C for2–48h.After hydrothermal treatment,the prod-uct wasfiltered,washed several times with deionized water until the washing solution became neutral,dried at50◦C overnight,and then used in the sorption experiments.The samples are denoted by HT-x h(x:the hydrothermal treatment time).2.3.CharacterizationThe chemical composition of BF slag was analyzed by X-rayfluo-rescence(XRF;ZSX Primus II-R1,Rigaku Co.).The resulting samples were identified by X-ray diffractometry with monochromated Cu K␣radiation(XRD;RINT2100/PC,Rigaku Co.)and scanning electron microscopy(SEM;JSM-6380A,Jeol Co.).The amounts of crystalline Al-substituted11˚A-tobermorite in the samples were estimated by the internal standard method base on the integrated XRD inten-sities using an analytical curve.Reagent-grade NaCl(99.5%,Kanto Chemical Co.)powder was used as the internal standard,and pure cystalline Al-substituted11˚A-tobermorite powder synthesized by referring to the previous report[23]was used to determine the analytical curve.The specific surface areas of the resulting samples were evaluated by measuring the isothermal adsorption of N2at 77K based on the BET method(BELSORP18PLUS,BEL-Japan).2.4.Cs+and Sr2+uptake experimentsThe Cs+and Sr2+solutions for uptake experiments were pre-pared by dissolving CsCl(99.0%,Nacalai Tesque Inc.)and SrCl2 (extra pure,Nacalai Tesque Inc.)in deionized water.The sam-ples were subjected to the Cs+and Sr2+uptake experiments by a batch method under the following conditions:temperature,25◦C; solid/solution ratio,0.5g/100cm3;initial Cs+and Sr2+concentra-tions,0.1–8.0mmol/dm3;and reaction time,5min–24h.After the experiments,the solid and solution phases were separated by cen-trifugation,and an aliquot of the supernatant was collected for chemical analyses.An atomic absorption spectrophotometer(AA-6800,Shimadzu Co.)was used to determine the concentrations of Cs+and Sr2+in the solutions before and after the experiments.2.5.Cs+and Sr2+selectivity experimentsThe Cs+and Sr2+selectivity experiments were carried out under the following conditions,according to previousreportsFig.1.SEM image and XRD pattern of starting BF slag.[15,23,24,26]:temperature,25◦C;initial NaCl or CaCl2(95.0%, Kanto Chemical Co.)concentrations,0.02N(eq/dm3)contain-ing0.0002N CsCl or SrCl2(i.e.,solutions having molar ratio [Cs+]:[Na+],[Cs+]:[Ca2+],[Sr2+]:[Na+]and[Sr2+]:[Ca2+]equal to 1:100);solid/solution ratio,0.05g/50cm3;and reaction time,24h. The concentrations of Cs+and Sr2+in the solutions before and after the experiments were determined in the same manner used in the uptake experiment.2.6.Cs+removal experiment from artificial seawaterThe Cs+removal property of HT-48h from artificial seawa-ter was investigated.Artificial seawater was prepared by mixing NaCl(26.686g),MgCl2(97.0%,Nacalai Tesque Inc.,5.092g),CaCl2 (1.106g),and KCl(99.5%,Kanto Chemical Co.,0.762g)in1dm3 of deionized water[28].The Cs+removal experiment from arti-ficial seawater was carried out under the conditions as follows: temperature,25◦C;solid/solution ratio,0.5g/100cm3;initial Cs+ concentration,0.75mmol/dm3;and reaction time,24h.The con-centration of Cs+in the solutions before and after the experiments was determined in the same manner used in the uptake experi-ment.2.7.Cs+desorption experimentThe desorption behavior of Cs+in HT-48h,which was previ-ously treated with CsCl aqueous solution of8.0mmol/dm3for24h, was investigated.The Na+solutions for the desorption experiment were prepared by dissolving NaCl in deionized water.The samples were subjected to the Cs+desorption experiment by a batch method under the conditions as follows:temperature,25◦C;solid/solution ratio,0.5g/100cm3;initial Na+concentrations,0.1–8.0mmol/dm3; and reaction time,24h.The concentrations of Cs+in the solutions before and after the experiments were determined in the same manner used in the uptake experiment.3.Results and discussionThe XRF results show that the BF slag consisted of48.2mass% CaO,29.3mass%SiO2,14.2mass%Al2O3, 4.74mass%MgO,and 3.56mass%others(Table1).The XRD pattern and SEM image of the BF slag are shown in Fig.1.The XRD pattern showed a very broad176T.Tsutsumi et al./Journal of Hazardous Materials 266 (2014) 174–181Table 1Chemical composition (mass%)of the BF slag.CaOSiO 2A12O 3MgOSO 3TiO 2K 2OFe 2O 3Na 2OOthers BF slag48.229.314.24.741.470.550.420.300.200.62peak at 2Â=30◦due to the presence of the slag,while no crystalline phases were detected.SEM observation revealed that the BF slag consisted of smooth particles of 2–5m in diameter.Fig.2shows XRD patterns of the products obtained by the hydro-thermal treatment.In all samples,peaks identified as tobermorite phase were observed with the impurity phase based on the peak at about 2Â=28.9◦and 29.9◦identified as a calcium silicate hydrate (C–S–H)[29,30]and 2Â=29.4◦identified as a calcite.The basal spacing of the (002)diffraction peak from the tobermorite phasewas found to be 11.4–11.6˚A,and was in good agreement with the previously reported values of Al-substituted 11˚A-tobermorite [22,29].Thus,it was revealed that Al-substituted 11˚A-tobermoritewas mainly synthesized from the sodium silicate-added BF slag by hydrothermal treatment at 180◦C for 2–48h.Fig.3shows theamounts of Al-substituted 11˚A-tobermorite in the samples.TheAl-substituted 11˚A-tobermorite yield increased with increasingtreatment time,and the amount of Al-substituted 11˚A-tobermoritein HT-48h was estimated to be about 87mass%.Fig.4shows SEM images of the products obtained by the hydrothermal treat-ment.In the image of HT-2h,small particles considered to be the product were observed together with large smooth particles con-sidered to be the starting BF slag.The morphology of the products became porous for HT-3h,then lath-like with further hydrothermal treatment.The specific surface areas (SSAs)of HT-2h,HT-3h,and HT-48h are 72,92,and 51m 2/g,respectively.While SSA of HT-3h was larger than that of HT-2h,the SSAs of the samples became smaller with longer hydrothermaltreatment.Fig.2.XRD patterns of the products obtained by hydrothermaltreatment.Fig.3.Dependence of Al-substituted 11˚A-tobermorite yield on hydrothermal treat-ment time.On the basis of these results,models of the hydrothermal tober-morite formation process from the sodium silicate-added BF slagare proposed as shown in Fig.5.At around 2–3h of hydrothermal treatment,the BF slag changes to C–S–H,and some C–S–H phasesare changed to the Al-substituted 11˚A-tobermorite.Next,the crys-tallization of the Al-substituted 11˚A-tobermorite proceeded withincreasing hydrothermal treatment time.Table 2shows the SSAs values of the samples in this study together with those of some natural and synthetic sorbents [31–39].Although the SSAs of the samples were not as large as those of the typical sorbents,the SSAs of the samples were comparable to that of montmorillonite which is a layered compound.The uptake ability characterized by using CsCl and SrCl 2aque-ous solutions with initial Cs +and Sr 2+concentrations of 0.75and 1.14mmol/dm 3,respectively,are shown in Table 3.The uptake of Cs +and Sr 2+by the samples was determined after a reaction time of 24h,because the investigated sorption kinetics suggested that this time is sufficient to achieve equilibrium,as shown in Fig.6.That is,most of the Cs +in the solution was removed within only 5–30min.The starting BF slag exhibited no uptake of either Cs +or Sr 2+.On the other hand,the treated samples removed Cs +and Sr 2+,Table 2Specific surface areas of the samples and representative natural and synthetic sorbents.MaterialSSA (m 2/g)Reference This work (HT-2h,HT-3h,HT-48h)51–92–Zeolite A (synthetic)635[31]Zeolite X (synthetic)487[32]Zeolite Y (synthetic)700[33]Clinoptilolite (natural)450[33]NaX (synthetic)375[34]NaA (synthetic)280[34]Montmorillonite (natural)60[35]LTA-monolith (synthetic)14[36]Zeolite rocks 25–42[37]Zl (natural)34[38]Z2(natural)32[38]Gordes (natural)95[39]Bigadic (natural)54[39]T.Tsutsumi et al./Journal of Hazardous Materials 266 (2014) 174–181177T a b l e 3A m o u n t o f C s +o r S r 2+r e m o v e d a n d a m o u n t o f N a +a n d C a 2+r e l e a s e d i n u p t a k e e x p e r i m e n t s .S a m p l eC s +C s 2+/[N a ++l /2C a 2+]F i n a l p HS r 2+S r 2+/[2N a ++C a 2+]F i n a l p H (m m o l /d m 3)A m o u n t o f C s +r e m o v e d (m m o l /g )A m o u n t o f N a +r e l e a s e d (m m o l /g )A m o u n t o f C a 2+r e l e a s e d (m m o l /g )(m m o l /d m 3)A m o u n t o f S r 2+r e m o v e d (m m o l /g )A m o u n t o f N a +r e l e a s e d (m m o l /g )A m o u n t o f C a 2+r e l e a s e d (m m o l /g )B F s l a g 0.000.000–––9.30.000.000–––9.3H T -2h 0.560.112–––1010.730.146–––10.1H T -3h 0.700.1400.2280.0080.6010.21.090.2180.2600.0500.3810.0H T -6h 0.690.1380.1960.0260.739.70.870.1740.2180.0680.359.9H T -12h 0.620.1240.1060.0141.1010.10.970.1940.2300.0600.3710.1H T -24h 0.610.1220.0840.0201.3010.10.890.1780.2100.0680.369.8H T -48h0.590.1180.0420.0202.279.30.860.1720.2000.0680.3710.0W e i g h t o f s a m p l e =0.5g ,v o l u m e o f s o l u t i o n =100c m 3.I n i t i a l C s +a n d S r 2+c o n c e n t r a t i o n =0.73a n d 1.20(m m o l /d m 3),r e s p e c t i v e l y .R e a c t i o n t i m e =24h ,i n i t i a l p H =6.0,r e a c t i o n t e m p e r a t u r e =25◦C . =(i n i t i a l C s +o r S r 2+c o n c e n t r a t i o n )−(r e m a i n e d C s +o r S r 2+c o n c e n t r a t i o n ).Fig.4.SEM images of the products obtained by hydrothermal treatment.(a)HT-2h,(b)HT-3h,and (c)HT-48h.while releasing Na +and Ca 2+.Because the samples contain the Al-substituted 11˚A-tobermorite,C–S–H,and some amorphous phases,the Cs +and Sr 2+removal may be due to the ion-exchange andadsorption reactions.In particular,it is noted that a certain amount of Ca 2+and Na +was released if Cs +and Sr 2+were present,suggest-ing that the removal of Cs +and Sr 2+proceeded by ion-exchange.Moreover,the amount of the released Ca 2+in the case of Cs +was considerably smaller than that in the case of Sr 2+.This could be explained by the fact that Na +in the interlayer was preferentially exchanged with Cs +,as seen in previous reports [23].On the other hand,the interlayer Ca 2+or surface Ca 2+may be exchanged by Sr 2+.However,the ratios of Cs +/[Na ++1/2Ca 2+]and Sr 2+/[2Na ++Ca 2+]deviated from 1,and excess Na +tended to be released from the samples.Probably,the Na +release is due to the existence of excess Na +in the sample because the NaOH was added when the sam-ple was synthesized by the hydrothermal treatment,even though178T.Tsutsumi et al./Journal of Hazardous Materials266 (2014) 174–181Fig.5.Schematic illustration of the hydrothermal tobemrorite formation process from the sodium silicate-added BF slag.the sample was well washed with deionized water.Fig.7shows the uptake values as a bar chart.The uptake values were con-siderably increased when the hydrothermal treatment time was extended from2to3h,and HT-3h showed the highest uptake values for both Cs+and Sr2+.However,the uptake values gradu-ally decreased when the hydrothermal treatment time was further extended.The sorption isotherm was fabricated and analyzed for short-time treatment samples(HT-2h and HT-3h)and a long-time treatment sample(HT-48h).The sorption isotherms of the sam-ples are shown in Fig.8.At a low equilibrium concentration,the sorption of Cs+and Sr2+by the samples increased rapidly with the increase in the equilibrium concentrations,and then reached a plateau at high equilibrium concentrations,suggesting that the samples exhibited Cs+and Sr2+selectivity.The sorption behaviorswere analyzed by the Langmuir and Freundlich model.ThelinearFig.6.Amounts of Cs+removed by HT-3h(triangles)and HT-48h(squares),as a function of reaction time.Sample;0.5g,reaction solution;100cm3,reaction temper-ature;25◦C,initial Cs+concentration;0.73mmol/dm3,reaction time;5min–24h, initial pH:6.0.Fig.7.Dependence of amounts of Cs+and Sr2+uptake on the hydrothermal treatment time.Sample;0.5g,reaction solution;100cm3,reaction tempera-ture;25◦C,initial Cs+concentration;0.73mmol/dm3,initial Sr2+concentration;1.20mmol/dm3,reaction time;24h,initial pH:6.0.Fig.8.Sorption isotherms of(a)Cs+and(b)Sr2+by HT-2h(circles),HT-3h(tri-angles),and HT-48h(squares).Sample;0.5g,reaction solution;100cm3,reaction temperature;25◦C,initial Cs+or Sr2+concentration;0.1–8.0mmol/dm3,reaction time;24h,initial pH;6.0.T.Tsutsumi et al./Journal of Hazardous Materials266 (2014) 174–181179 Table4Langmuir and Freundlich parameters analyzed from Cs+and Sr2+sorption isotherms.Ion Sample Langmuir coefficient Freundlich coefficientq m(mmol/g)b(dm3/mmol)R2K(mmol1–1/n dm3/n/g)1/n R2Cs+HT-2h0.400 2.5000.99440.3650.4100.9004 HT-3h0.587 1.7030.99840.4330.3160.9360 HT-48h0.453 2.2070.99830.3670.3250.8970 Sr2+HT-2h0.524 1.9070.99580.3890.3390.9623 HT-3h0.601 1.6650.98670.3870.3730.9919 HT-48h0.334 2.9950.99840.2610.2700.9321 Weight of sample=0.5g,volume of solution=100cm3.Initial Cs+and Sr2+concentration=0.1–8.0mmol/dm3.Reaction time=24h,initial pH=6.0,reaction temperature=25◦C.form of the Langmuir isotherm model can be expressed as follows:C e q e =1(bq m)+1(q m)C ewhere C e and q e are the equilibrium concentrations of metal ions in the liquid and solid phases,respectively,and b and q m are Langmuir coefficients representing the equilibrium constant for sorbate–sorbent equilibrium and the monolayer capacity,respec-tively[40].Linear Langmuir plots were obtained by plotting C e/q e vs.C e,from which the sorption coefficients could be evaluated. The Freundlich isotherm model,which has also found widespread application in liquid–solid sorption system,can be represented asfollows:log q e=log K+1nlog C ewhere K and1/n are the Freundlich constants.This model is based on the assumption that the enthalpy of sorption becomes less negative as a function of the extent of sorption.Table4shows the Langmuir and Freundlich parameters for Cs+and Sr2+sorp-tion by the samples.Experimental steady-state data were found to correlate well with the Langmuir isotherm model,whereas the Freundlich model was shown to be comparatively less appro-priate.The calculated q m values for Cs+were0.40mmol/g for HT-2h,0.58mmol/g for HT-3h,and0.45mmol/g for HT-48,while those of Sr2+were0.46mmol/g for HT-2h,0.60mmol/g for HT-3h, and0.33mmol/g for HT-48.These values are comparable to the theoretical exchange capacity of Al-substituted11˚A-tobermorite, NaCa5Si5AlO16(OH)2·4H2O,i.e.,about0.66mmol/g for Cs+and Sr2+, which are estimated from its chemical composition assuming Na+ of1mol and Ca2+of1mol in the interlayer are exchangeable with Cs+and Sr2+,respectively.The q m values of HT-2h were smaller than that of HT-3h,because some unreactive BF slag probably remained in the HT-2h sample.Furthermore,the q m values of the short-time treatment sample(HT-3h)were larger than that of a long-time treatment sample(HT-48h).In the case of Sr2+sorp-tion,the q m value of a long-time treatment sample(HT-48h)was remarkably smaller than that of a short-time treatment sample (HT-3h).As mentioned above,it is probable that some surface Ca2+ and Na+of the sample contributed to the removal reaction.The number of exchangeable Ca2+ions could decrease from HT-3h to HT-48h,because some Ca2+is incorporated into the framework layer as shown in Fig.5.Thus,it was considered that the decrease in the total number of ion-exchangeable Ca2+ions by the long-time treatment caused the difference in the Cs+and Sr2+sorption behaviors.Accordingly,the Cs+and Sr2+selectivity experiments were carried out for HT-3h with the highest q m value and HT-48h with Al-substituted11˚A-tobermorite.Table4shows the selective ion-exchange properties for Cs+and Sr2+by the HT-3h and HT-48h.The properties are expressed in terms of the Cs+or Sr2+distribu-tion coefficient,K d(mg/cm3),which is defined as the ratio of the amount of Cs+or Sr2+sorbed per1g of sample to the amount of Cs+ remaining per1cm3of solution.In the Cs+selectivity experiments, the K d and percentage uptake values of HT-48h were larger than those of HT-3h,indicating that HT-48h can selectively uptake Cs+compared with HT-3h.Because the Na+in the interlayer of Al-substituted11˚A-tobermorite was preferentially exchanged with Cs+,as seen in previous reports[23],this difference could be attributed to the difference in the amount of Al-substituted 11˚A-tobermorite produced.In Sr2+selectivity experiments,both HT-3h and HT-48h exhibited high values in the presence of Na+,whereas they exhib-ited low values in the presence of Ca2+.Despite the difference in the hydrothermal treatment time,the HT-3h and HT-48h exhibited similar behavior,suggesting that the Sr2+selectivity of the Al-substituted11˚A-tobermorite was not very high.When monovalent Na+exists around the sample,bivalent Sr2+may be rel-atively exchangeable with the surface Ca2+together with Ca2+in the interlayer.However,it could be difficult for Sr2+to exchange with the surface Ca2+and Na+,when bivalent Ca2+exists around the sam-ple.Consequently,it was suggested that selective Sr2+removal in the presence of Na+could be mainly achieved by the ion-exchange reaction with surface Ca2+of the sample.The prepared samples with highly selective Cs+or Sr2+removal in the presence of Na+could be useful for the development of radioactive137Cs+and90Sr2+adsorbents from the sea where Na+ is the main cation.In particular,the development of radioactive 137Cs+sorbents from the sea has become an urgent issue in Japan. Therefore,the Cs+selectivity was evaluated in more detail.That is, the Cs+removal from artificial seawater was investigated by using HT-48h.In addition,the desorption behavior of Cs+in the sam-ple was investigated;the HT-48h sample,which was previously treated with CsCl aqueous solution with initial Cs+concentration of8.0mmol/dm3for24h,was dispersed in the NaCl aqueous solu-tion with various Na+concentrations for24h.As a result,it was found that percentage Cs+uptake value from artificial seawater reached47%(Table5),suggesting that the sample produced in this work functions as a Cs+sorbent.Fig.9shows the dependence of the amounts of Cs+desorption on the Na+concentration in the reaction solution.It was revealed that only about13%of Cs+was desorbed from the sample and the desorbed amounts did not depend on the Na+concentration.This result indicates the removal reaction of Cs+was not reversible in this experimental condition.Moreover, it is noted that the short-time hydrothermal treatment is sufficient for preparing Cs+or Sr2+sorbent with relatively high selectivity from the BF slag.Consequently,it was revealed that the cheap and efficient sorbents could be synthesized by the hydrothermal treatment.Further studies using the samples,such as the Cs+con-centration dependence of the selective removal properties from180T.Tsutsumi et al./Journal of Hazardous Materials266 (2014) 174–181Table5Cs+or Sr2+selectivity of HT-3h and HT-48h.Molar ratios[Cs+]:[Na+],[Cs+]:[Ca2+],[Sr2+]:[Na+],and[Sr2+]:[Ca2+]in the reaction solution are equal to1:100.Artificial seawater was prepared by mixing NaCl(26.686g),MgCl2(5.092g),CaCl2(1.106g),and KCl(0.762g)in1dm3of deionized water[28].Sample Solution condition Initial Cs+or Sr2+concentration(mmol/dm3) (mmol/dm3)Amount of Cs+or Sr2+removed(mmol/g)Uptake(%)K d(cm3/g)aHT-3h Na+Cs0.2610.1100.11042.2731 Ca+Cs0.1670.0570.05733.9514Na+Sr0.3640.1920.19252.71114Ca+Sr0.1780.0160.0169.1100 HT-48h Na+Cs0.2610.1500.15057.41349 Ca+Cs0.1670.0930.09355.91262Na+Sr0.3640.1950.19553.61159Ca+Sr0.1780.0120.0127.075Artificial seawater+Cs0.7620.3620.07247.5181Selectively uptake experiment:weight of sample=0.05g,volume of solution=50cm3,initial pH=6.0.Cs+removal from artificial seawater;weight of sample=0.5g,volume of solution=100cm3,initial pH=5.8.Reaction time=24h,reaction temperature=25◦C.=(initial Cs+or Sr2+concentration)-(remained Cs+or Sr2+concentration).a Kd=fraction of cation on exchanger1g of sample×1cm3of solutionfraction of cation in solution.Fig.9.Percentage of Cs+desorption from HT-48h in Na+aqueous solution.Sample; HT-48h of0.5g was previously treated with CsCl aqueous solution of8.0mmol/dm3 for24h,reaction solution;100cm3,reaction temperature;25◦C,initial Na+concen-tration;0.1–8.0mmol/dm3,reaction time;24h,initial pH;5.8.the artificial seawater,are in progress in order to develop practical applications.4.ConclusionAl-substituted11˚A-tobermorite was mainly synthesized from sodium silicate-added BF slag by hydrothermal treatment at180◦C for2–48h.It was suggested that most of BF slag was changed to C–S–H,the Al-substituted11˚A-tobermorite was formed at around3h of hydrothermal treatment,and the Al-substituted11˚A-tobermorite yield of the product increased with further treatment. The sorption behavior of the samples for Cs+and Sr2+could be explained by a Langmuir model.The HT-3h showed the high-est removal in both Cs+and Sr2+uptake experiments by a batch method,whereas HT-48h showed the highest performance in the Cs+and Sr2+selectivity experiments.These results are attributed to the difference in the amount of well-crystallized Al-substituted 11˚A-tobermorite in the sample.The Cs+selectivity for HT-48h was greater than that for HT-3h,and it was considered that the inter-layer Na+was selectively exchanged with Cs+.Both HT-48h and HT-3h exhibited Sr2+selectivity in the presence of Na+.Although Sr2+selectivity of the Al-substituted11˚A-tobermorite was not as high,most of such Sr2+may be exchanged with surface Ca2+.Con-sequently,Al-substituted11˚A-tobermorite-based materials were successfully synthesized from waste BF slag.Moreover it was sug-gested that the prepared samples were useful for the development of radioactive137Cs+and90Sr2+sorbents from the sea where Na+is the main cation.AcknowledgementThis work was partly supported by the Steel Foundation for Environmental Protection Technology.References[1]Y.Sugano,R.Sahara,T.Murakami,T.Narushima,Y.Iguchi,C.Ouchi,Hydro-thermal synthesis of zeolite a using blast furnace slag,ISIJ Int.45(2005) 937–945.[2]T.Kuroki,Y.Uchida,H.Takizawa,K.Morita,Effect of28GHz/2.45GHzmicrowave irradiation on the crystallization of blast furnace slag,ISIJ Int.47 (2007)592–595.[3]R.Anuwattana,K.J.Balkus Jr.,S.Asavapisit,P.Khummongkol,Conventionaland microwave hydrothermal synthesis of zeolite ZSM-5from the cupola slag, Microporous Mesoporous Mater.111(2008)260–266.[4]T.Wajima,K.Oya,A.Shibayama,K.Munakata,Preparation of adsorbent withhigh removal ability for phosphate ion from blast furnace slag using alkali fusion,Int.J.Soc.Mater.Eng.Resour.Jpn.18(2012)59–63.[5]A.Nakahira,H.Naganuma,T.Kubo,Y.Yamasaki,Synthesis of monolithic tober-morite from blast furnace slag and evaluation of its Pb removal ability,J.Ceram.Soc.Jpn.116(2008)500–504.[6]Z.Jing,F.Jin,T.Hashida,N.Yamasaki,E.H.Ishida,Influence of additions of coalfly ash and quartz on hydrothermal solidification of blast furnace slag,Cem.Concr.Res.38(2008)976–982.[7]Z.Jing,F.Jin,T.Hashida,N.Yamasaki,H.Ishida,Hydrothermal solidificationof blast furnace slag by formation of tobermorite,J.Mater.Sci.42(2007) 8236–8241.[8]J.D.C.McConnell.,The hydrated calcium silicates riversideite,tobermorite,andplombierite,Mineral.Mag.30(1954)293–305.[9]H.D.Megaw,C.H.Kelsey,Nature177(1956)390–391.[10]S.A.Hamid,The crystal structure of the11˚A natural tobermoriteCa2.25[Si3O7.5(OH)1.5]·1H2O,Z.Kristallogr.154(1981)189–198.[11]S.Merlino,E.Bonaccorsi,T.Armbruster,Tobermorite their real structure andorder–disorder(OD)character,Am.Mineral.84(1999)1613–1621.[12]S.Merlino,E.Bonaccorsi,T.Armbruster,The real structure of tobermorite11˚A:normal and anomalous forms,OD character and polytypic modifications,Eur.J.Mineral.13(2001)577–590.[13]S.Komarneni,D.M.Roy,New tobermorite cation exchangers,J.Mater.Sci.20(1985)2930–2936.[14]S.Komarneni,Heavy metal removal from aqueous solutions by tobermoritesand zeolites,Nucl.Chem.Waste Mange.5(1985)247–250.[15]N.J.Coleman,D.S.Brassington,Synthesis of Al-substituted11˚A tobermoritefrom newsprint recycling residue:a feasibility study,Mater.Res.Bull.38(2003) 485–497.[16]H.Katsumata,S.Kaneco,K.Inomata,K.Itoh,K.Funasaka,K.Masuyama,T.Suzuki,K.Ohta,Removal of heavy metals in rinsing wastewater from plating factory by adsorption with economical viable materials,J.Environ.Manage.69 (2003)187–191.[17]N.J.Coleman,Interaction of Cd(II)with waste-derived11˚A tobermorites,Sep.Purif.Technol.48(2006)62–70.[18]N.J.Coleman,D.S.Brassington,A.Raza,A.P.Mendham,Sorption of Co2+and Sr2+by waste-derived11˚A tobermorite,Waste Manage.26(2006)260–267.。