EFFECT+OF+CELL+WIDTH+ON+THE+DEVICE+PERFORMANCE+OF+AMORPHOUS+SILICON+SOLAR
研究生科研论文英文摘要常见问题分析及写作规范 泸州医学院
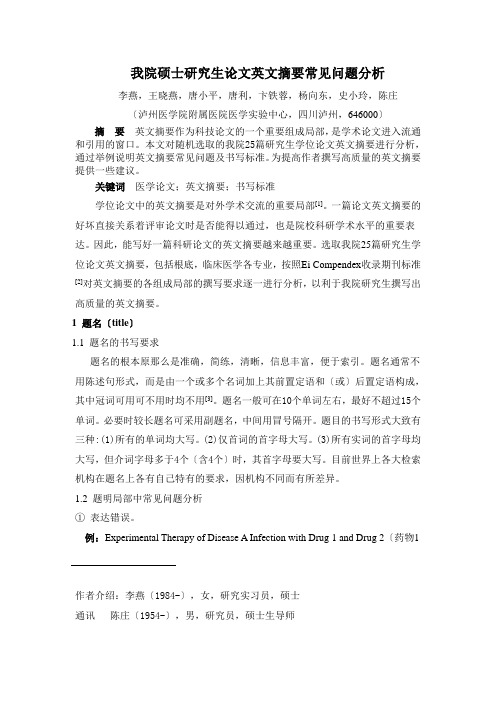
我院硕士研究生论文英文摘要常见问题分析李燕,王晓燕,唐小平,唐利,卞铁蓉,杨向东,史小玲,陈庄〔泸州医学院附属医院医学实验中心,四川泸州,646000〕摘要英文摘要作为科技论文的一个重要组成局部,是学术论文进入流通和引用的窗口。
本文对随机选取的我院25篇研究生学位论文英文摘要进行分析,通过举例说明英文摘要常见问题及书写标准。
为提高作者撰写高质量的英文摘要提供一些建议。
关键词医学论文;英文摘要;书写标准学位论文中的英文摘要是对外学术交流的重要局部[1]。
一篇论文英文摘要的好坏直接关系着评审论文时是否能得以通过,也是院校科研学术水平的重要表达。
因此,能写好一篇科研论文的英文摘要越来越重要。
选取我院25篇研究生学位论文英文摘要,包括根底,临床医学各专业,按照Ei Compendex收录期刊标准[2]对英文摘要的各组成局部的撰写要求逐一进行分析,以利于我院研究生撰写出高质量的英文摘要。
1 题名〔title〕1.1 题名的书写要求题名的根本原那么是准确,简练,清晰,信息丰富,便于索引。
题名通常不用陈述句形式,而是由一个或多个名词加上其前置定语和〔或〕后置定语构成,其中冠词可用可不用时均不用[3]。
题名一般可在10个单词左右,最好不超过15个单词。
必要时较长题名可采用副题名,中间用冒号隔开。
题目的书写形式大致有三种:(1)所有的单词均大写。
(2)仅首词的首字母大写。
(3)所有实词的首字母均大写,但介词字母多于4个〔含4个〕时,其首字母要大写。
目前世界上各大检索机构在题名上各有自己特有的要求,因机构不同而有所差异。
1.2题明局部中常见问题分析①表达错误。
例:Experimental Therapy of Disease A Infection with Drug 1 and Drug 2〔药物1作者介绍:李燕〔1984-〕,女,研究实习员,硕士通讯陈庄〔1954-〕,男,研究员,硕士生导师和药物2治疗疾病A的动物实验研究〕改:Experimental Therapy of Drug 1 and Drug 2 on Animal Disease A Model②指代不明,标点使用错误,值得注意的是英文中并无顿号。
底栖硅藻悬浮培养初探

Hans Journal of Agricultural Sciences 农业科学, 2021, 11(2), 104-110Published Online February 2021 in Hans. /journal/hjashttps:///10.12677/hjas.2021.112015底栖硅藻悬浮培养初探张凝,孙东红,颜纲,邹宁鲁东大学生命科学学院,山东烟台收稿日期:2021年1月8日;录用日期:2021年2月3日;发布日期:2021年2月18日摘要底栖硅藻是鲍鱼和海参的主要饵料,足量优质饵料是人工育苗生产中最为重要因素,底栖硅藻大部分采用固定化培养和静置培养,无法达到规模生产,因此其工业化大规模生产十分必要。
本文以舟形藻为研究材料,研究了不同硅浓度静置培养、不同硅浓度悬浮培养、光合反应器培养三种培养方式,打破传统的附着性生长培养状态,通过悬浮培养达到较高的培养密度。
通过其OD值和细胞密度比较分析,初步得出对舟形藻生长影响最佳培养条件和最佳硅浓度,结果表明,悬浮条件培养下,最有利于舟形藻培养,藻细胞密度最高可达2.45 × 106个/mL,为大规模悬浮培养优质舟形藻提供初步参考。
关键词底栖硅藻,培养条件,营养成分Preliminary Study on Suspension Culture of Benthic DiatomsNing Zhang, Donghong Sun, Gang Yan, Ning ZouCollege of Life Sciences, Ludong University, Yantai ShandongReceived: Jan. 8th, 2021; accepted: Feb. 3rd, 2021; published: Feb. 18th, 2021AbstractBenthic diatoms are the main food for abalone and sea cucumber. Sufficient and high-quality food is the most important factor in the production of artificial seedlings. Most benthic diatoms are cultured in immobilized culture and static culture, which cannot achieve large-scale production, therefore, its large-scale industrial production is very necessary. In this paper, three kinds of culture methods, I. E.Static culture with different silicon concentration, suspension culture with different silicon concen-张凝等tration and photosynthetic reactor culture are studied, which broke the traditional adherent growth culture state and achieved high culture density through suspension culture. The optimum culture conditions and the optimum silicon concentration were obtained by comparing the OD value and the cell density. The results showed that the suspension culture was the most favorable for the cultiva-tion of Navicular, and the maximum cell density was 2.45 × 106 cells/mL, which provided a prelimi-nary reference for large-scale suspension culture of high quality Navicular.KeywordsBenthic Diatom, Culture Condition, CompositionsThis work is licensed under the Creative Commons Attribution International License (CC BY 4.0)./licenses/by/4.0/1. 引言舟形藻(Navicula),属于硅藻门,羽纹纲,舟形藻目,舟形藻科,舟形藻属,其模式种为Navicula tripunctata (Müller) Bory [1]。
MTS法检测细胞增殖活性

Promega Corporation ·2800 Woods Hollow Road ·Madison, WI 53711-5399 USA1.Description (1)2.Product Components and Storage Conditions (4)3.Protocols (5)A.General Protocol (5)B.Example of a Protocol for Bioassay of IL-6 Using B9 Cells (5)4.General Considerations (6)A.Background Absorbance.....................................................................................6B.Optional Wavelengths to Record Data.............................................................7C.Lymphocyte Assays.............................................................................................8D.Reagent Optimization..........................................................................................8E.Cell Number Optimization (8)5.References (9)6.Related Products (9)1.DescriptionThe CellTiter 96®AQ ueous One Solution Cell Proliferation Assay (a)is a colorimetric method for determining the number of viable cells in proliferation or cytotoxicity assays. The CellTiter 96®AQ ueous One Solution Reagent contains a novel tetrazolium compound [3-(4,5-dimethylthiazol-2-yl)-5-(3-carboxymethoxyphenyl)-2-(4-sulfophenyl)-2H-tetrazolium, inner salt; MTS (a)] and an electron coupling reagent (phenazine ethosulfate; PES). PES has enhanced chemical stability, which allows it to be combined with MTS to form a stable solution. This convenient “One Solution” format is an improvement over the first version of the CellTiter 96®AQ ueous Assay, where phenazine methosulfate (PMS) is used as the electron coupling reagent, and the PMS Solution and MTS Solution are supplied separately.The MTS tetrazolium compound (Owen’s reagent) is bioreduced by cells into a colored formazan product that is soluble in tissue culture medium (Figure 1, 1).This conversion is presumably accomplished by NADPH or NADH produced by dehydrogenase enzymes in metabolically active cells (2). Assays are performed by adding a small amount of the CellTiter 96®AQ ueous One Solution Reagent directly to culture wells, incubating for 1–4 hours and then recording the absorbance at 490nm with a 96-well plate reader (3,4).CellTiter 96®AQ ueous One Solution Cell Proliferation AssayAll technical literature is available on the Internet at: /tbs Please visit the web site to verify that you are using the most current version of this Technical Bulletin. Please contact Promega Technical Services if you have questions on useofthissystem.E-mail:********************.1.Description (continued)The quantity of formazan product as measured by the absorbance at 490nm is directly proportional to the number of living cells in culture (Figure 2). Because the MTS formazan product is soluble in tissue culture medium, the CellTiter 96®AQ ueous One Solution Assay requires fewer steps than procedures that usetetrazolium compounds such as MTT or INT (5,6). The formazan product of MTT reduction is a crystalline precipitate that requires an additional step in the procedure to dissolve the crystals before recording absorbance readings at570nm (7).If you currently use a [3H]thymidine incorporation assay, addition of theCellTiter 96®AQ ueous One Solution Reagent can be substituted for the pulse of[3H]thymidine at the time point in the assay when the pulse of radioactivethymidine is usually added. Bioassay data comparing [3H]thymidineincorporation to the MTS-based CellTiter 96®AQ ueous Assay and the original MTT-based CellTiter 96®Assay demonstrate that tetrazolium reagents can be substituted for [3H]thymidine incorporation (4,7).Advantages of the CellTiter 96®AQ ueous One Solution Assay include:•Easy-to-Use:Add the CellTiter 96®AQ ueous One Solution Reagent to cells,incubate and read absorbance.•Convenient:Supplied as a single solution, filter-sterilized and ready for adding to assay plates.•Fast:Perform the assay in a 96-well plate with no washing or cell harvesting.Also eliminates solubilization steps normally required for MTT assays.•Non-Radioactive:Requires no scintillation cocktail or radioactive wastedisposal.Promega Corporation ·2800 Woods Hollow Road ·Madison, WI 53711-5399 USAFigure 1. Structures of MTS tetrazolium and its formazan product.N N N NSO 3OCH 2COOHMTS +SN CH 3CH 3N N N N H SO 3OCH 2COOH FormazanS NCH 3CH 31605M B 09_6A•Flexible:Plates can be read and returned to incubator for further color development.•Safe:Requires no volatile organic solvent to solubilize theformazanproduct (unlike MTT).Promega Corporation ·2800 Woods Hollow Road ·Madison, WI 53711-5399 USAFigure 2. Effect of cell number on absorbance at 490nm measured using theCellTiter 96®AQ ueous One Solution Assay. Various numbers of B9 hybridoma cells were added to the wells of a 96-well plate in RPMI containing 50μM 2-mercaptoethanol and supplemented with 5% FBS and 2ng/ml IL-6. The medium was allowed toequilibrate for 1 hour, then 20μl/well of CellTiter 96®AQ ueous One Solution Reagent was added. After 1 hour at 37°C in a humidified, 5% CO 2atmosphere, the absorbance at 490nm was recorded using an ELISA plate reader. Each point represents the mean ± SD of 4 replicates. The correlation coefficient of the line was 0.993, indicating alinear response between cell number and absorbance at 490nm. The backgroundabsorbance shown at zero cells/well was not subtracted from these data.2 × 1044 × 1046 × 1048 × 1041 × 1051.2 × 1051.4 × 1051.6 × 105A b s o r b a n c e (490n m )Cells/Well1606M A 10_9A2.Product Components and Storage ConditionsProduct Size Cat.# CellTiter 96®AQ ueous One SolutionCell Proliferation Assay200 assays G3582 For Laboratory Use. Includes:•4ml CellTiter 96®AQ ueous One Solution ReagentProduct Size Cat.# CellTiter 96®AQ ueous One SolutionCell Proliferation Assay1,000 assays G3580 For Laboratory Use. Includes:•20ml CellTiter 96®AQ ueous One Solution ReagentProduct Size Cat.# CellTiter 96®AQ ueous One SolutionCell Proliferation Assay5,000 assays G3581 For Laboratory Use. Includes:•100ml CellTiter 96®AQ ueous One Solution ReagentStorage Conditions:For long-term storage, store the CellTiter 96®AQ ueous One Solution Reagent at –20°C, protected from light. See the expiration date on the ProductInformation Label. For frequent use, solutions may be stored at 4°C, protected fromlight, for up to 6 weeks.Note:The performance of CellTiter 96®AQ ueous One Solution Reagent after 10 freeze-thaw cycles was demonstrated to be equal to that of freshly prepared solution.Safety:To the best of our knowledge, the chemical, physical and toxicologicalproperties of this product have not been thoroughly investigated; therefore, werecommend the use of gloves, lab coats and eye protection when working with these orany chemicals.Light-Sensitivity:The CellTiter 96®AQ ueous One Solution Reagent is light-sensitive andis supplied in an amber container. Discoloration may occur if solutions are exposed tolight outside of the container for several hours. This discoloration may cause slightlyhigher background 490nm absorbance readings, but it should not affect theperformance of the CellTiter 96®AQ ueous One Solution Assay.Promega Corporation·2800 Woods Hollow Road ·Madison, WI 53711-5399 USA3.ProtocolsMaterials to Be Supplied by the User•96-well plates suitable for tissue culture•repeating pipettes, digital pipettes or multichannel pipettes•96-well plate reader3.A.General Protocol1.Thaw the CellTiter 96®AQ ueous One Solution Reagent. It should takeapproximately 90 minutes at room temperature, or 10minutes in a waterbath at 37°C, to completely thaw the 20ml size.2.Pipet 20μl of CellTiter 96®AQ ueous One Solution Reagent into each well ofthe 96-well assay plate containing the samples in 100μl of culture medium.Note:We recommend repeating pipettes, digital pipettes or multichannelpipettes for convenient delivery of uniform volumes of CellTiter 96®AQ ueous One Solution Reagent to the 96-well plate.3.Incubate the plate at 37°C for 1–4 hours in a humidified, 5% CO2atmosphere.Note:To measure the amount of soluble formazan produced by cellularreduction of MTS, proceed immediately to Step 4. Alternatively, to measurethe absorbance later, add 25μl of 10% SDS to each well to stop the reaction.Store SDS-treated plates protected from light in a humidified chamber atroom temperature for up to 18 hours. Proceed to Step 4.4.Record the absorbance at 490nm using a 96-well plate reader.3.B.Example of a Protocol for Bioassay of IL-6 Using B9 Cells1.Maintain stock cultures of B9 cells in RPMI 1640 medium containing5%FBS, 50μM 2-mercaptoethanol (2-ME) supplemented with 5ng/mlhuman recombinant IL-6 . Subculture the stock cultures of cells to2×104cells/ml, and refeed with human recombinant IL-6 every 3 days orwhen a density of 2 × 105 cells/ml is reached.Note:B9 cells used for the bioassay should be from stock cultures 2 daysafter the last subculture (feeding with IL-6).2.Add 50μl/well of IL-6 samples or standards to be measured, diluted inRPMI 1640 medium containing 5% FBS and 50μM 2-ME. Start the titrationof the IL-6 standard at 4ng/ml in column 12, and perform serial twofolddilutions across the plate to column 2 (to 4pg/ml). (After the cellsuspension is added in Step 5 below, the final concentration of the titratedstandard will be 2ng/ml in column 12 to 2pg/ml in column 2.) Usecolumn1 for the negative control: RPMI 1640 medium (and supplements)without IL-6. Equilibrate the plate at 37°C in a humidified, 5% CO2atmosphere while harvesting the cells for assay.Promega Corporation·2800 Woods Hollow Road ·Madison, WI 53711-5399 USA3.B.Example of a Protocol for Bioassay of IL-6 Using B9 Cells (continued)3.Wash the B9 cells twice in RPMI 1640 containing 5% FBS and 50μM 2-MEby centrifugation at 300 × g for 5 minutes.4.Determine cell number and viability (by trypan blue exclusion), andresuspend the cells to a final concentration of 1 × 105 cells/ml in RPMI 1640supplemented with 5% FBS and 50μM 2-ME.5.Dispense 50μl of the cell suspension (5,000 cells) into all wells of the plateprepared in Step 2. The total volume in each well should be 100μl.6.Incubate the plate at 37°C for 48–72 hours in a humidified, 5% CO2atmosphere.7.Add 20μl per well of CellTiter 96®AQ ueous One Solution Reagent.8.Incubate the plate at 37°C for 1–4 hours in a humidified, 5% CO2atmosphere.Note:To measure the amount of soluble formazan produced by cellularreduction of MTS, proceed immediately to Step 9. Alternatively, to measurethe absorbance at a later time, add 25μl of 10% SDS to each well to stop thereaction. Store SDS-treated plates protected from light in a humidifiedchamber at room temperature for up to 18 hours. Proceed to Step9.9.Record the absorbance at 490nm using a 96-well plate reader.10.Plot the corrected absorbance at 490nm (Y axis) versus concentration ofgrowth factor (X axis). Determine the X-axis value corresponding to one-halfthe difference between the maximum (plateau) and minimum (no growthfactor control) absorbance values; this is the ED50value (ED50= theconcentration of growth factor necessary to give one-half the maximumresponse.)4.General Considerations4.A.Background AbsorbanceA small amount of spontaneous 490nm absorbance occurs in culture mediumincubated with CellTiter 96®AQ ueous One Solution Reagent. The type of culturemedium used, type of serum, pH and length of exposure to light are variablesthat may contribute to the background 490nm absorbance. Backgroundabsorbance is typically 0.2–0.3 absorbance units after 4 hours of culture.Background absorbance may result from chemical interference of certaincompounds with tetrazolium reduction reactions. Strong reducing substances,including ascorbic acid, or sulfhydryl-containing compounds, such asglutathione, coenzyme A and dithiothreitol, can reduce tetrazolium saltsnonenzymatically and lead to increased background absorbance values.Culture medium at elevated pH or extended exposure to direct light also maycause an accelerated spontaneous reduction of tetrazolium salts and result inincreased background absorbance values. If phenol red containing medium is Promega Corporation·2800 Woods Hollow Road ·Madison, WI 53711-5399 USAused, an immediate change in color may indicate a shift in pH caused by thetest compounds. Specific chemical interference of test compounds can beconfirmed by measuring absorbance values from control wells containingmedium without cells at various concentrations of test compound.Background 490nm absorbance may be corrected as follows: Prepare atriplicate set of control wells (without cells) containing the same volumes ofculture medium and CellTiter 96®AQ ueous One Solution Reagent as in theexperimental wells. Subtract the average 490nm absorbance from the “no cell”control wells from all other absorbance values to yield corrected absorbances.4.B.Optional Wavelengths to Record DataFigure 3 shows an absorbance spectrum of the formazan product resultingfrom reduction of MTS. We recommend recording data at the absorbance peakof 490nm; however, if your 96-well plate reader does not have a 490nm filter,data can be recorded at wavelengths of 450–540nm. Absorbance may berecorded at other wavelengths if necessary, but loss in sensitivity will result. Areference wavelength of 630–700nm may be used to subtract backgroundcontributed by excess cell debris, fingerprints and other nonspecific absorbance.Promega Corporation ·2800 Woods Hollow Road ·Madison, WI 53711-5399 USAFigure 3. Absorbance spectrum of MTS/formazan. The absorbance spectrum of the formazan product resulting from reduction of the MTS tetrazolium compoundshows an absorbance maximum at 490nm. The negative absorbance values (382nm)correspond to the disappearance of MTS as it is converted into formazan.A b s o r b a n c e Wavelength (nm)3.002.502.001.501.000.500.00-0.503004005006007002284M A 07_8A4.C.Lymphocyte AssaysLymphocytes may produce less formazan than other cell types (8). To achievesignificant absorbance changes with lymphocytes, increase the number ofcells to approximately 2.5–10 × 104cells/well and incubate the plate withCellTiter 96®AQ ueous One Solution Reagent for the entire 4-hour period.4.D.Reagent OptimizationThe concentrations of tetrazolium and electron transfer reagents have beenoptimized for general use with a wide variety of cell lines cultured in 96-wellplates containing 100μl of medium. If different volumes of culture medium areused, adjust the volume to maintain a ratio of 20μl CellTiter 96®AQ ueous OneSolution Reagent per 100μl culture medium. This reagent:medium ratio resultsin a final concentration of 317μg/ml MTS in the assay wells. Minor variationsin the optimum concentrations of tetrazolium and electron transfer reagentsoccur with different cell lines; however, assay sensitivity is seldomcompromised using the formulation in the CellTiter 96®AQ ueous One SolutionReagent. If reagent optimization is critical to your assay procedure, werecommend using the CellTiter 96®AQ ueous Non-Radioactive Cell ProliferationAssay (Cat.# G5421, G5430, G5440) or the CellTiter 96®AQ ueous MTS ReagentPowder products (Cat.# G1111, G1112) that supply the chemicals separately.4.E.Cell Number OptimizationCell proliferation assays require cells to grow over a period of time. Therefore,choose an initial number of cells per well that produces an assay signal nearthe low end of the linear range of the assay. This helps to ensure that the signalmeasured at the end of the assay will not exceed the linear range of the assay.This cell number can be determined by performing a cell titration as shown inFigure 2.Different cell types have different levels of metabolic activity. Factors that affectthe metabolic activity of cells may affect the relationship between cell numberand absorbance. Anchorage-dependent cells that undergo contact inhibitionmay show a change in metabolic activity per cell at high densities, resulting ina nonlinear relationship between cell number and absorbance. Factors thataffect the cytoplasmic volume or physiology of the cells will affect metabolicactivity.For most tumor cells, hybridomas and fibroblast cell lines, 5,000 cells per wellis recommended to initiate proliferation studies, although fewer than 1,000 cellscan usually be detected. The known exception to this is blood lymphocytes,which generally require 25,000–250,000 cells per well to obtain a sufficientabsorbance reading.Promega Corporation·2800 Woods Hollow Road ·Madison, WI 53711-5399 USA5.References1.Barltrop, J.A. et al.(1991) 5-(3-carboxymethoxyphenyl)-2-(4,5-dimenthylthiazoly)-3-(4-sulfophenyl)tetrazolium, inner salt (MTS) and related analogs of3-(4,5-dimethylthiazolyl)-2,5-diphenyltetrazolium bromide (MTT) reducing to purplewater-soluble formazans as cell-viability indicators. Bioorg. Med. Chem. Lett.1, 611-4.2.Berridge, M.V. and Tan, A.S. (1993) Characterization of the cellular reduction of3-(4,5-dimethylthiazol-2-yl)-2,5-diphenyltetrazolium bromide (MTT): Subcellularlocalization, substrate dependence, and involvement of mitochondrial electrontransport in MTT reduction. Arch. Biochem. Biophys.303, 474–82.3.Cory, A.H. et al.(1991) Use of an aqueous soluble tetrazolium/formazan assay for cellgrowth assays in culture. Cancer Commun.3, 207–12.4.Riss, T.L. and Moravec, R.A. (1992) Comparison of MTT, XTT, and a noveltetrazolium compound for MTS for in vitro proliferation and chemosensitivity assays.Mol. Biol. Cell (Suppl.)3, 184a.5.Mosmann, T. (1983) Rapid colorimetric assay for cellular growth and survival:Application to proliferation and cytotoxicity assays. J. Immunol. Methods65, 55–63.6.Bernabei, P.A. et al.(1989) In vitro chemosensitivity testing of leukemic cells:Development of a semiautomated colorimetric assay. Hematol. Oncol.7, 243–53.7.CellTiter 96®Non-Radioactive Cell Proliferation Assay Technical Bulletin#TB112,Promega Corporation.8.Chen, C.-H., Campbell, P.A. and Newman, L.S. (1990) MTT colorimetric assay detectsmitogen responses of spleen but not blood lymphocytes. Int. Arch. Allergy Appl.Immunol.93, 249–556.Related ProductsMTS/MTT-Based Cell Viability Assay SystemsProduct Size Cat.# CellTiter 96®AQ ueous Non-RadioactiveCell Proliferation Assay1,000 assays G54215,000 assays G543050,000 assays G5440 CellTiter 96®AQ ueous MTS Reagent Powder*250mg G11121g G1111 CellTiter 96®Non-RadioactiveCell Proliferation Assay1,000 assays G40005,000 assays G4100For Laboratory Use. *PMS is not supplied with MTS Reagent Powder and must beobtained separately.Promega Corporation·2800 Woods Hollow Road ·Madison, WI 53711-5399 USA6.Related Products (continued)Luminescent-Based Cell Viability Assay SystemProduct Size Cat.# CellTiter-Glo®Luminescent Cell Viability Assay10ml G757010 × 10ml G7571100ml G757210 × 100ml G7573Resazurin-Based Cell Viability Assay SystemProduct Size Cat.# CellTiter-Blue®Cell Viability Assay20ml G8080100ml G808110 × 100ml G8082Fluorescent-Based Cell Viability AssayProduct Size Cat.# CellTiter-Fluor™ Cell Viability Assay10ml G60805 × 10ml G60812 × 50ml G6082For Laboratory Use.Cytotoxicity Assay Systems (LDH)Product Size Cat.# CytoTox-ONE™ HomogeneousMembrane Integrity Assay200–800 assays G78901,000–4,000 assays G7891 CytoTox 96®Non-Radioactive Cytotoxicity Assay*1,000 assays G1780 CytoTox-Glo™ Cytotoxicity Assay*10ml G92905 × 10ml G92912 × 50ml G9292*For Laboratory Use.Promega Corporation·2800 Woods Hollow Road ·Madison, WI 53711-5399 USAApoptosis Assay SystemsProduct Size Cat.#Apo-ONE®Homogeneous Caspase-3/7 Assay1ml G779210ml G7790100ml G7791 Caspase-Glo®2 Assay*10ml G094050ml G0941 Caspase-Glo®6 Assay*10ml G097050ml G0971 Caspase-Glo®3/7 Assay* 2.5ml G809010ml G8091100ml G8092 Caspase-Glo®8 Assay* 2.5ml G820010ml G8201100ml G8202 Caspase-Glo®9 Assay* 2.5ml G821010ml G8211100ml G8212CaspACE™ Assay System, Colorimetric*50 assays G7351100 assays G7220 DeadEnd™ Fluorometric TUNEL System60 reactions G3250 DeadEnd™ Colorimetric TUNEL System40 reactions G713020 reactions G7360*For Laboratory Use.Apoptosis ReagentsProduct Size Cat.# CaspACE™ FITC-VAD-FMK In Situ Marker50μl G7461125μl G7462Anti-ACTIVE®Caspase-3 pAb50μl G7481Anti-Cytochrome c mAb100μg G7421Anti-pS473Akt pAb40μl G7441Anti-PARP p85 Fragment pAb50μl G7341 Caspase Inhibitor Z-VAD-FMK125μl G723250μl G7231 Caspase Inhibitor, Ac-DEVD-CHO100μl G5961 Promega Corporation·2800 Woods Hollow Road ·Madison, WI 53711-5399 USA6.Related Products (continued)Viability and Cytotoxicity AssayProductSize Cat.#MultiTox-Fluor Multiplex Cytotoxicity Assay 10ml G92005 × 10ml G9201(live/dead cell protease activity determination) 2 × 50ml G9202CytoTox-Fluor™ Cytotoxicity Assay 10ml G92605 × 10ml G9261(dead cell protease activity determination) 2 × 50ml G9262MultiTox-Glo Multiplex Cytotoxicity Assay 10ml G92705 × 10ml G9271(live/dead cell protease activity determination) 2 × 50ml G9272For Laboratory Use.Promega Corporation ·2800 Woods Hollow Road ·Madison, WI 53711-5399 USA(a)The MTS tetrazolium compound is the subject of U.S. Pat. No. 5,185,450 assigned to the University of South Florida and islicensed exclusively to Promega Corporation.© 1996, 1998, 1999, 2004, 2005, 2007 Promega Corporation. All Rights Reserved.Anti-ACTIVE, Apo-ONE, Caspase-Glo, CellTiter 96, CellTiter-Blue, CellTiter-Glo and CytoTox 96 are registered trademarks of Promega Corporation. CaspACE, CellTiter-Fluor, CytoTox-Fluor, CytoTox-Glo, CytoTox-ONE and DeadEnd are trademarks of Promega Corporation.Products may be covered by pending or issued patents or may have certain limitations. Please visit our Web site for more information.NEN is a registered trademark of NEN Life Science Products, Inc.All prices and specifications are subject to change without prior notice.Product claims are subject to change. Please contact Promega Technical Services or access the Promega online catalog for the most up-to-date information on Promega products.。
SIZE-DEPENDENT EFFECTS IN PROPERTIES OF

© 2009 Advanced Study Center Co. Ltd.Corresponding author: R.A. Andrievski, e-mail: ara@icp.ac.ruSIZE-DEPENDENT EFFECTS IN PROPERTIES OFNANOSTRUCTURED MATERIALSR.A. AndrievskiInstitute of Problems of Chemical Physics, Russian Academy of Sciences, Chernogolovka, Moscow Region,142432, Russia Received: February 17, 2009Abstract. Size-dependent effects in nanostructured (nanocrystalline, nanophase or nanocomposite)materials are of great importance both for fundamental considerations and modern technology.The effect of the nanoparticle/nanocrystallite size on surface energy, melting point, phase transfor-mations, and phase equilibriums is considered as applied to nanostructured materials. The role of size-dependent effects in phonon, electronic, superconducting, magnetic, and partly mechanical properties is also analyzed in detail. Special attention is paid to the contribution of other factors such as the grain boundary segregations, interface structure, residual stresses and pores, non-uniform distribution of grain sizes, and so on. The little explored and unresolved problems are pointed and discussed.1. INTRODUCTIONSize-dependent effects (SDE, i.e. the characteris-tic size influence of grains, particles, phase inclu-sions, pores, etc ., on the properties of materials and substances) have been studied in physics,chemistry, and materials science for a long time. It is quite enough to list the following well-known equations of Laplace, Thomson (Kelvin), Gibbs-Ostwald, Tolman, J. Thomson, Hall-Petch,Nabarro-Herring, and Coble, which connect the capillary pressure (P ), saturated vapor pressure (p ), saturated solubility (C ), surface energy of flat surface (σ0), conductivity (λ), hardness (H ) and creep rate (′ε) correspondingly with the pore/in-clusion radius (r ), thickness film (h ) and grains/crys-tallites size (L ):P r =20σ/, (1)p r p rRT =B =B =002exp /,σΩ (2)C r C rRT =B =B=002exp /,σΩ (3)σσξ0012r r =B =B =+//,(4)λλh f =B =B =+0510./. (5)H L H AL =B =+−005., (6)~/,ε1L m (7)where p o is the equilibrium pressure of saturated vapor on flat surface, C o is the equilibrium solubil-ity of subject with flat surface, Ω is an atomic (mo-lar) volume, R is the gas constant, T is tempera-ture, ξ is the Tolman constant, λo is conductivity of grain-coarse material (thick film), f = h /l o , l o is the mean free pass of carriers (f < 1), H o is the friction stress in the absence of grain boundaries (GB) and A is a constant. The factors m =2 and 3 correspond to the Nabarro-Herring diffusion creep and to theCoble diffusion creep correspondingly.The development of modern advancednanostructured materials (NM) manifests some new problems such as the identification adaptabil-ity of these equations (1-7) and other those in na-108R.A. Andrievskinometer interval; the role of quantum effects as well as an influence of other factors in the NM prop-erties or their stability and reproducibility. All these things are very important and actual to consider the SDE features taking in mind the update high rate in this field and not only fundamental consid-erations, but the strategy optimal development for nanotechnology. These questions have been ana-lyzed early by the present author [1-5] and other investigators (see, e.g., [6-19]). It seems to be nec-essary to tell apart the SDE in the isolated nanosubjects (clusters, nanoparticles, nanowires,and nanotubes) and consolidated ones (nanobulks,nanocomposites, and nanofilms/nanocoatings).Analyses of SDE in consolidated NM can be found in reviews [1-6], but the most publications were devoted to nanoparticles and clusters. Naturally,the SDE topic as a whole seems not to be cov-ered, because there are many new results scat-tered throughout the literature.Taking into account the comprehensive books [9,10,13,15,16], this review is mainly devoted to the SDE recent analysis in consolidated NM (papers mainly from 2001-2002), although some results for isolated nanosubjects will be also considered with the exception of catalytic and biological properties.Moreover, the comprehensive problem on the SDE in mechanical properties will be also discussed in limited scale as applied only to brittle high-melting point compounds (see Section 4 below).It is important to point that there are at least five principal features of size effects in consolidated NM [1-5]:- the crystalline size reduction to the nanometer scale results in a significant increase of the role of the interface defects such as GB, triple junc-tions (TJ), and elastically distorted layers;- interfacial properties on a nanometer scale can be different from those of conventional grain-coarse materials;- the reduced crystallite size can be overlapped by characteristic physical lengths such as the mean free path of carriers or the Frank-Read loop size for dislocations and so on;- size effects in NM can have quantum nature if the crystallite size is commensurable with the De Broyle wavelength l B =D /(2m *E )1/2 where D is the Planck constant, m * is the electron effective mass, E is the electron energy. Based on known values of m * and E, it is possible to predict that the quantum size effects can be exhibited in met-als only when the crystallite size is lower than ~ 1nm. For semiconductors (especially with narrow gap-zone, e.g., InSb) and semimetals (e.g., Bi),the l B value is significantly higher and can be of about 100 nm;- unlike conventional grain-coarse materials, in NM there are many factors for masking SDE such as residual stresses, pores, TJ and the presence of other defects, progressive accumulation of inter-face segregations, non-equilibrium phase ap-pearance, and so on.All these peculiarities may reveal in the pres-ence of certain specific points in the size depen-dencies and in the non-monotonous change of the properties determined by decreasing of the grain size. As a rule, such non-monotonous change is especially characteristic for structure-sensitive properties. For example, such specific features, i.e.twist points, and non-monotonous change of prop-erties have been revealed in the known hardness and magnetic coercivity studies (see, e.g., [1,2,4]).Such changes can be connected with different fac-tors such as other mechanisms of SDE, progres-sive accumulation of segregations on interfaces,and so on.2. THERMODYNAMIC PROPERTIES 2.1. Surface energyThe problem concerning surface energy is impor-tant both for fundamental sense and for many materials science and engineering applications such as the prognosis of phase diagrams, the es-timations of the fracture, milling, dilution, wetting,nucleation, coagulation, recrystallization charac-teristics, and so on. In this connection, the reveal-ing of surface energy change in nanometer inter-val is of special interest.Tolman’s equation (4), i.e. the effect of the drop radius on surface energy, has been widely dis-cussed (see, e.g., [7,8,11,14,20-23]). Omitting the details of these calculations, which are based on different physical and chemical approaches, let us to consider these results as a whole. Being ap-plied to isolated nanocrystals under calculations [11,14], the amendment g (r ) to the conventional σ0value fixed on the capillary pressure (1) is describedby the following expressiong r r r rr rB=B =B =−+−−211422182ln /ln /For many nanocrystals this amendment is very small and comes into particular prominence (about 10%) only at r < 5 nm.The radius effect on surface energy can be also described by equations:109Size-dependent effects in properties of nanostructured materialsσσr Kr at r r at r r =B =<>456,,, (9)where K is a constant, r o is critical radius (of 2-10nm) [21]. There are other theoretical calculations that have revealed about the similar values of criti-cal radius lower of which surface energy is de-creased (see, e.g., [20,22]). The experimental re-sults in this field are very limited, ambiguous, and often mutually contradictory. From one side, elec-trochemical measurements have revealed the σr values about 0.2 J/m 2 and 0.7 J/m 2 for Ag nanoparticles of r ~ 50 nm and r ~ 150 nm accord-ingly to [24]. These values seem to be not so pre-cise but are reasonable (it is appropriate to com-pare them with the value of σo = 1.14 J/m 2 and with the GB energy of ~0.27 J/m 2). From the other side,the experimental study of size-dependent evapo-ration of free-spherical Ag and PbS nanoparticles using relationship (2) results in values of 7.2 J/m 2and 2.45 J/m 2 respectively, these values are sig-nificantly higher as compared to that of the flat bulks [25]. May be, this is an effect of the evaporation conditions (see Section 2.2) or there is a system-atic error in the experimental results [25].As applied to nanocrystalline solids the analy-sis of size- or curvature-dependent interface, free energy results to the following relationshipσσr t r =B =B≈−01/, (10)where t is an interfacial layer (the semi-width of GB in consolidated nanometals) [7,8]. It is easy to see that at the reasonable values of 2t ~ 1nm and r = 5 nm the σ decreasing is of 10% that is in close agreement with estimations under Eq. (8).In Debye approximation, the Tolman constant ξin Eq. (4) can be expressed asξα=′−151.,h =B(11)where h ’ is the height of atomic monolayer, α is the ratio of root-mean-square amplitude of thermal vi-brations of atoms on surface and in volume [23]. If α > 1 and ξ > 0, then σr < σo and in the opposite case α < 1 and ξ < 0 then σr > σo . As it will be seen from here on, these two cases are important as applied to the behavior analysis of nanoparticles in matrices.It is known that distinct feature of nanostructure in NM is that, with the grain size confinement, the part of TJ is increased and, accordingly, the part of GB is decreased (see, e.g., [4]). The computer modeling with account of GB and TJ contributions reveals that Ni grain size reducing is accompaniedby decrease of the total excess enthalpy (i.e. the total GB energy) [26]; this result quantitatively agrees with experimental data for nano Se [27].The calculated interface GB energy in Cu-Ni bilayered nanofilm changes from 0.9 J/m 2 to about 0.7 J/m 2 when bilayer thickness decreases from ~1 nm to ~0.4 nm [28].From the foregoing data it has been evident that in spite of different theoretical approaches in cal-culations, they are evidence of the σ decrease in the confinement of isolated nanosubjects and nanostructures in NM. It seems to be reasonable to introduce the amendments into grain/crystalline boundary energy values at L lower than near 10nm. However, it is also evident that experimental studies in this field are necessary for further de-tailed continuation.The comprehensive description of the SDE role in the other surface phenomena such as wetting,nucleation, adsorption, and capillarity can be found in book [16]. It is worth to note that the observation by high-resolution transmission electron micro-scope (HRTEM) methods is in common practice (see, e.g., [19,29,30]).2.2. Melting pointIt is well known for a long time that small particles and thin films are characterized by the lower melt-ing points (T m ) as compared with their counterparts in bulk form as a result of the atom thermal vibra-tion amplitude increase in the surface layers. There are many relationships contacting T m and r for free-standing (supporting) metal (Au, Ag, Cu, Sn, etc .)clusters/nanoparticles as well as h for thin films (see, e.g., [4,10,13,15,16,19]). Generally, all pro-posed equations have the form of T m ~ 1/r (h ) type.Recent theoretical and experimental works tend to obtain more precise specifications: the size,shape, and stress effects on the T m nanograins on a substrate [31]; the role of fractal structure [32];the comparison of SDE in the case of spherical nanoparticles, nanofilms, and nanowires [9,33]; the effect of transition to amorphous state [34], kinetic study of the Cu film melting-dispergation [35], etc .Thus the consideration of size-dependent cohesive energy results in the T m variation for spherical nanoparticle, nanowire, and nanofilm of a material in the same characteristic size as 3:2:1 that is con-firmed by experimental values for In [33]. The mo-lecular dynamics simulation of the melting behav-ior for “model ” nanocrystalline Ag has exhibited two characteristic regions on the grain size decrease [34]. The first is above about 4 nm where the T m110R.A. Andrievskivalues decrease with grain size decreasing. The second one (r < 4 nm) is a size-independent re-gion where the T m values almost keep a constant.The dominant factor in this situation is supposed to be the nanocrystal T m shifts from grain to GB.The amorphous phase (r < 1.3 nm), as indicated from the radial distribution function and common neighbor analysis, is different from the GB by the sharp enhancement of local fivefold symmetry and is characterized by a much lower solid-to-liquid transformation temperature than that of Ag nanocrystal and GB. Melting and non-melting of solid surfaces including partly behavior of nanosystems are discussed in review [12].When the nanosolids are entirely coated by a high-melting point substance or embedded in such matrix, the nanosolid T m values can not only de-crease but increase too; this interesting phenom-ena is named by superheating or overheating. TheSME detailed analysis at melting and superheat-Fig. 1. Melting point of In nanoparticles embedded in Al matrix for two methods of preparation as a function of particle diameter: ball milling (n ) and spinning (l ). HRTEM images illustrated the different In/Al interface structures, replotted from [19].ing of bulks and nanosolids can be found in review [19]. The crucial role of interface in superheating is clearly demonstrated by the measurement re-sults of the ball-milled and the melt-spun Al-In samples T m values as a function of particle size of In (Fig. 1) [19].Fig. 1 clearly demonstrates that samples pre-pared by the two methods undergo completely dif-ferent melting behaviors which were investigated using differential scanning calorimetry (DSC), in-situ HRTEM, and in-situ X-ray diffraction (XRD). In the case of ball-milling procedure, there are irregu-lar In particles and the incoherent random inter-faces were formed between particles and the ma-trix, then the nanoparticles exhibit the SDE T m de-pression, as described previously. In the case of melt-spun samples, the In particles were found to be distributed both in the Al GB and within the Al grains. The particles within Al grains are truncated by octahedral shapes bounded by {111}/(100) fac-111Size-dependent effects in properties of nanostructured materials Compound Usual bulk phase Observed(metal, alloy)at RT and P =106 Pa nanocrystalline phaseZrO 2 Monoclinic Tetragonal BaTiO 3 Cubic Tetragonal PbTiO 3 Cubic Tetragonal Al 2O 3 α-Al 2O 3 γ-Al 2O 3 TiO 2 RutileAnataseY 2O 3Cubic (α-Y 2O 3) Monoclinic (γ-Y 2O 3)CdSe, CdSWurtzite Rock salt Co hcp * (α-Co) fcc * (β-Co) T hcp (α-Ti) bcc * (β-Ti) Fe-NiMartensiteAustenite (fcc)*hcp is hexagonal-closed-packed structure; fcc is face-centered-cubic structure; bcc is body-centered-cubic structure.Table 1. Structure of unusual phases observed in some NM [37].ets and can be considered as epitaxial coherent interfaces revealing the T m increase with decreas-ing of r . It is interesting to know whether other prop-erties, not only melting point, are also changing under the ball-milled and melt-spun nanoparicles in matrices. The superheating has been also ob-served in the systems of Pb-Al and Ag-Ni both in the Al and Ni matrixes as well as partly in layered Pb/Al films [19].On phenomenological level, superheating is explained by change of root-mean-square ampli-tude of thermal vibrations of atoms on surface and in volume (Eq. (11) [23]) and by modification of in-terfacial energy [19] or the coherent interface pa-rameter between inclusions and matrix [36].2.3. Phase transformationsApart of melting point SDE make an impact on other phase transformations in NM. Table 1 summarizes some data in this field [37].Observed solid-state transformations are con-sidered to be connected with an increased effec-tive internal pressure due to high surface/interfa-cial curvature or with the whole and surface en-ergy difference between allotropic phases [37]. The tetragonal-to-monoclinic (T → M) phase transfor-mation in the zirconia-yttria system is the most ex-plored subject. Fig. 2 shows experimental data on the T → M transformation temperature (T tr ) for dif-ferent YSZ powders and sintered pellets with par-ticles/grains in the nanometer interval [38].In Fig. 2 each line demarcated the tetragonal phase stability region (defined as “T ”) and that toleft of the line (defined as “T+M ”) where the T-phase starts to transform into the M-phase which was fixed in both the DSC and the dilatometer studies. It is evident from these results that the T tr value is de-creased with a reduction of crystallite/grain size.The curve extrapolation provide the opportunity to predict the particle critical size values at room tem-perature of the T-phase stability: 15, 30, 51, and 71 nm for 0YSZ, 0.5YSZ, 1.0YSZ, and 1.5YSZ powders, respectively. In the case of the 0.5YSZ,1.0YSZ, and 1.5YSZ sintered pellets, the grain criti-cal size values are 70, 100, and 155 nm, respec-tively. The observed difference in crystalline/grain size (about 2 times) can be attributed to the strain energy term and difference in the surface and in-terfacial energies.In the thermodynamic approach frame, the fol-lowing relationships between the T tr value and criti-cal crystalline/grain size (D c , L c ) have been pro-posed116///for powders ,D H T T c b tr b =−∆∆σ=B =B =B (12)1166////for pellets ,L H T T U cbtrbd=−+∆∆Σ∆∆Σ=B =B =B (13)where ∆H b and T b are the enthalpy and transfor-mation temperature for bulk (coarse-grained) sol-ids, ∆σ and ∆Σ are the differences in the surface/interfacial energies for T/M phases in powders and pellets, ∆U d is the strain energy involved in the transformation (this additional strain-energy term estimates only for consolidated pellets) [37,38].112R.A. AndrievskiFig. 2. Inverse critical grain/crystalline size versus transformation temperature (T → M) for different yttria-doped zirconia (a) powders and (b) pellets: 0YSZ – pure ZrO2, 0.5YSZ – 0.5 mol.%Y2O3, 1.0YSZ – 1.0mol.%Y2O3, 1.5YSZ – 1.5 mol.%Y2O3, replotted from [38].113Size-dependent effects in properties of nanostructured materials Fig. 3. The phase stability diagram for Nb/Zr multilayers as a function of the Nb volume fraction (f NB ) and the inverse of bilayer thickness (λ-1): # 1 is the stability region of hcp Zr/bcc Nb; # 2 is the stability region of bcc Zr/bcc Nb; # 3 is the stability region of hcp Zr/hcp Nb. The circles indicate experimental results and the line boundary calculated from the thermodynamic model, replotted from [41].The possibility to observe also the high-tem-perature phase at low temperature is the situation when the elementary crystal (germ) size of this phase is lower than crystallite size. Such situation tends to the transformation blocking and the high-temperature phase is fixing. This example is typi-cal for some martensite transformations in the Fe-Ni and Ti-Ni-Co micro- and nanocrystalline alloys [39]. The martensite volume part (M ) depends on the high-temperature initial phase crystallite size under the equationM M K Lm =−−005., (14)where M o and K m are some constants. The rela-tionship (14) coincides with that of Hall-Petch one (6). This agreement could be connected with the analogous nature of elastic strain fields at the crack and germ propagation.The availability of non-equilibrium phases in thin film is observed for a long time. Under film thick-ness lower than some critical value (h c ), non-com-mon phases can be fixed; that is connected with the Gibbs free energy excess effect due to the sur-face effect. One of the first relationships describ-ing this effect was proposed more than 50 years agoh F F c =−−σσ1221=B =B/, (15)where indexes 1 and 2 correspond to equilibrium and non-equilibrium phases, respectively, σi and F i are the total surface energy and the Gibbs free energy of these phases [40]. The h c rough estima-tion by Eq. (15) resulted in the quite reasonable values (h c ~ 10 nm).Basically, the idea [40] based on a classical ther-modynamic approach with some modifications is used in many modern studies especially with the development of nanostructured thin film multilayers such as Ti/Al(Nb, Zr) and TiN/AlN(NbN, ZrN), Fe/Cr, etc ., which can exhibit metastable structures in one or both layers. Fig. 3 shows the phase stability diagram for Nb/Zr multilayers with varying volume fractions and bilayer (λNb + λZr ) thickness [41].The phase stability region boundaries were cal-culated using a classical thermodynamic model taking into account the structural energy assess-114R.A. AndrievskiFig. 4. The Bi-Pb phase diagram. The dashed lines are the equilibrium bulk phase diagram and the solid lines are experimental results for nanoparticles of (a) 10 nm radii and (b) 5 nm radii, replotted from [42].115Size-dependent effects in properties of nanostructured materials Fig. 5. Pseudobinary TiN-TiB 2 phase diagram for equilibrium state (the solid lines) and for nanostructured films (L ~ 10 nm), replotted from [44].ment in the interfacial energy value. The equilib-rium phases (hcp Zr and bcc Nb) were observed only in the region #1; at that, the effect of the Nb volume fraction is not monotonous. The presence of the non-equilibrium bcc Zr and hcp Nb phases in sputtered films (regions #2 and #3, respectively)was confirmed experimentally by XRD and elec-tron diffraction.2.4. Phase equilibriumsBy now there are several examples of theoretical calculations and experimental studies of the phase diagram SDE in nanointerval: ZrO 2-Y 2O 3 [37], Pb-Bi [42], In-Sn [30], Au-Sn [30], carbon phase dia-gram [43], Al-Pb [18], Bi-Cd [18], TiB 2-TiN [44], TiB 2-B 4C [45], hydrogen systems [46,47], etc . Fig. 2 data have been used for the T/T+M line design in the ZrO 2-Y 2O 3 diagram as a function of particle size [37].The most detailed study was undertaken in the Pb-Bi diagram observing in-situ the melting behav-ior of isolated alloyed nanoparticles by hot stage TEM. Fig. 4 compares this diagram for particles of 10 nm radii and 5 nm radii as well as for bulks [42].As shown in Fig. 4, T m is a size-depend decreas-ing from the bulk value. The progressive narrow-ing of two-phase fields and an increase of solubil-ity are also observed. The theoretical calculations developed from thermodynamic first principles were in agreement with experimental results.The significant increase of solubility for nanosized subjects was observed in many cases including the hydrogen-metal (intermetallic) sys-tems [46,47], Pb-Fe and Pb-Sn systems [48,49],and systems-based high-melting point compounds [44,45]. Fig. 5 shows the TiN- TiB 2 phase diagram both in equilibrium state and film one (the grain size about of 10 nm) [44].The significant increase in solubility was fixed by the experimental XRD test [50] and the eutectic temperature decrease that was calculated in the regular solution approximation using the phase equilibrium at the eutectic temperature. The con-116R.A. Andrievskitribution made by the surface energy excess for solid state was written in per mole terms as ∆F S = 6V σgb /L where V is the molar volume and σgb is the interfacial (grain boundary) energy (see also [51]). The same approach was used for the SDE analysis in the case of the TiB 2-B 4C phase diagram [45]. Unfortunately,the σgb value information is very limited. It was supposed in the TiN-TiB 2 phase diagram calculations (Fig.5) that σgb ~ 3 J/m 2; in the case of the TiB 2-B 4C phase diagram the more reasonable σgb interval from 1J/m 2 to 3 J/m 2 was used[45].The solubility features in nanosystems are theoretically discussed in papers [52,53]. In many cases,the great role of the grain boundary segregations is pointed (see, e.g., [48]). However, as a whole, the question on the oversaturated solution formation nature remains insufficiently explored as applied to mechanical alloying, ion plating, severe plastic deformation, electrodeposition, and other preparation methods of NM.Another interesting feature in the SDE on phase equilibriums is that the spinodal decomposition and the triple point coordinates. It was theoretically shown that the grain segregations (thickness of about 1nm) increase the solid solution decomposition degree when the grain size does not exceed 10 nm [54].The spinodal decomposition regularities of supersaturated TiN-AlN system with the thermal stable nanostructure formation are very interesting and important for the development of new advanced NM. Ab initio calculations revealed the contribution role of strain and surface energy on the energetic balance for decomposition process [55].The calculation of SDE on the carbon diagram results in the following data for the triple point coordi-nates defined regions for solid nanodiamond, liquid that and amorphous nanocarbon [43] Particle diameter, (nm) 1 1.2 1.5 2 3 4 6 Singlecrystal Pressure (GPa) 15.2 16.5 16.115.615.214.814.513.5 T, K2210316035503820409041904300 4470These results are in qualitative agreement with some experimental/theoretical information [56]. The movement of triple point, defined equilibrium between solid state, liquid one, and vapor one, to the tem-perature decrease and pressure increase with the particle decrease was also described in book [9].In the most cited works dedicated to the SDE in phase equilibriums, the conditional thermodynamic approaches were used and the interface structure influence was not analyzed. In mean time, as it was shown at the Fig. 1, the impact of external interfaces on T m can be very great and opposite, so that interface contributions to the thermodynamic functions become significant [18,19]. The analysis of two-phase equilibrium in alloy nanoparticles has revealed the possibility of the eutectic point degeneration into intervals of composition where the alloy melts discontinuously and of the phase diagram topology dra-matic changes [57]. These observations tended to conclusion that the concept of equilibrium in nanostructures yet remains to be understood and needs in further detailed study [18,51].3. PHYSICAL PROPERTIES3.1. Phonon and electronic properties. SuperconductivityMeasurements of electronic heat capacity (γe ) of Cu-Nb (Pb) nanocomposites and studies of their phonon spectra revealed the decrease of the electronic state density at the Fermi level N (E F ) and the increase of low-frequency oscillations as compared with their coarse-grained (cg ) counterparts [58,59]. The γe value for Nb inclusions (L ~20 nm) in Cu 90Nb 10 nanocomposite was of 3.0 mJ/mol K 2 that is about three times lower than that in conventional Nb. The Debye temperature (ΘD ) value is also reduced with the inclusion decrease. These changes were connected with the weakening of interatomic interactions at the GB and assisted by the superconducting transition temperature (T C ) decrease.The size dependence of T C , the residual resistance ratio (RRR), the upper critical magnetic field (H C2)and the Ginzburg-Landau coherence length (ξGL ) for the nanocrystalline Nb films are shown in Table 2[60,61].It is clear from Table 2 that while the T C and RRR values decrease with size monotonically, the H C2 and ξGL values increase with size but there is non-monotonous change. The first change is supposed to be connected with the N (E F ) decrease rather than by phonon softening, i.e. the effect of the electron-phonon。
2008-Cytoprotective effect of fucoxanthin isolated from brown=FX=细胞保护
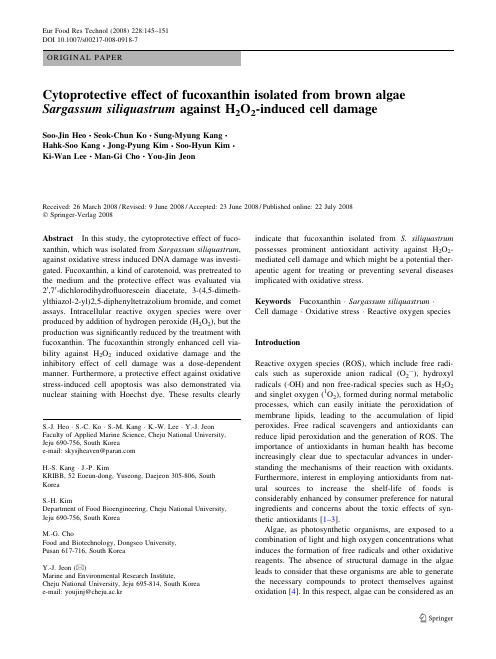
ORIGINAL PAPERCytoprotective effect of fucoxanthin isolated from brown algae Sargassum siliquastrum against H 2O 2-induced cell damageSoo-Jin Heo ÆSeok-Chun Ko ÆSung-Myung Kang ÆHahk-Soo Kang ÆJong-Pyung Kim ÆSoo-Hyun Kim ÆKi-Wan Lee ÆMan-Gi Cho ÆYou-Jin JeonReceived:26March 2008/Revised:9June 2008/Accepted:23June 2008/Published online:22July 2008ÓSpringer-Verlag 2008Abstract In this study,the cytoprotective effect of fuco-xanthin,which was isolated from Sargassum siliquastrum ,against oxidative stress induced DNA damage was investi-gated.Fucoxanthin,a kind of carotenoid,was pretreated to the medium and the protective effect was evaluated via 20,70-dichlorodihydrofluorescein diacetate,3-(4,5-dimeth-ylthiazol-2-yl)2,5-diphenyltetrazolium bromide,and comet assays.Intracellular reactive oxygen species were over produced by addition of hydrogen peroxide (H 2O 2),but the production was significantly reduced by the treatment with fucoxanthin.The fucoxanthin strongly enhanced cell via-bility against H 2O 2induced oxidative damage and the inhibitory effect of cell damage was a dose-dependent manner.Furthermore,a protective effect against oxidative stress-induced cell apoptosis was also demonstrated via nuclear staining with Hoechst dye.These results clearlyindicate that fucoxanthin isolated from S.siliquastrum possesses prominent antioxidant activity against H 2O 2-mediated cell damage and which might be a potential ther-apeutic agent for treating or preventing several diseases implicated with oxidative stress.Keywords Fucoxanthin ÁSargassum siliquastrum ÁCell damage ÁOxidative stress ÁReactive oxygen speciesIntroductionReactive oxygen species (ROS),which include free radi-cals such as superoxide anion radical (O 2-),hydroxyl radicals (ÁOH)and non free-radical species such as H 2O 2and singlet oxygen (1O 2),formed during normal metabolic processes,which can easily initiate the peroxidation of membrane lipids,leading to the accumulation of lipid peroxides.Free radical scavengers and antioxidants can reduce lipid peroxidation and the generation of ROS.The importance of antioxidants in human health has become increasingly clear due to spectacular advances in under-standing the mechanisms of their reaction with oxidants.Furthermore,interest in employing antioxidants from nat-ural sources to increase the shelf-life of foods is considerably enhanced by consumer preference for natural ingredients and concerns about the toxic effects of syn-thetic antioxidants [1–3].Algae,as photosynthetic organisms,are exposed to a combination of light and high oxygen concentrations what induces the formation of free radicals and other oxidative reagents.The absence of structural damage in the algae leads to consider that these organisms are able to generate the necessary compounds to protect themselves against oxidation [4].In this respect,algae can be considered as anS.-J.Heo ÁS.-C.Ko ÁS.-M.Kang ÁK.-W.Lee ÁY.-J.Jeon Faculty of Applied Marine Science,Cheju National University,Jeju 690-756,South Koreae-mail:skysjheaven@H.-S.Kang ÁJ.-P.KimKRIBB,52Eoeun-dong,Yuseong,Daejeon 305-806,South KoreaS.-H.KimDepartment of Food Bioengineering,Cheju National University,Jeju 690-756,South KoreaM.-G.ChoFood and Biotechnology,Dongseo University,Pusan 617-716,South KoreaY.-J.Jeon (&)Marine and Environmental Research Institute,Cheju National University,Jeju 695-814,South Korea e-mail:youjinj@cheju.ac.krEur Food Res Technol (2008)228:145–151DOI 10.1007/s00217-008-0918-7important source of antioxidant compounds that could be suitable for protecting our bodies against the ROS formed e.g.by our metabolism or induced by external factors(as pollution,stress,UV radiation,etc.).Fucoxanthin is found in edible brown algae and,along with b-carotene,is one of the most abundant carotenoids found in nature[5].There have recently been several reports that fucoxanthin showed biological activities such as radical scavenging,antiproliferative,antiobesity,anti-tumor,and antiangiogenic activities[6–10].Nonetheless, the protective effects of cell damages related to antioxidant activities of fucoxanthin have not yet been reported. Accordingly,the present study isolated the marine natural carotenoid fucoxanthin from Sargassum siliquastrum and evaluated its cytoprotective effects against H2O2-induced cell damage.Materials and methodsMaterialsThe marine alga S.siliquastrum was collected along the coast of Jeju Island,Korea,between October2005and March2006.The samples were washed three times with tap water to remove the salt,epiphytes,and sand attached to the surface,then carefully rinsed with fresh water,and maintained in a medical refrigerator at-20°C.Therefore, the frozen samples were lyophilized and homogenized with a grinder prior to extraction.General experimental proceduresOptical rotations were measured on a JASCO P-1020 polarimeter.The UV and FT-IR spectra were recorded on a Pharmacia Biotech Ultrospec3000UV/Visible spec-trometer and a SHIMAZU8400s FT-IR spectrometer, respectively.NMR spectra were recorded on a Varian INOVA400MHz NMR spectrometer.CD3OD was used as a solvent for the NMR experiments,and the solvent signals were used as an internal reference.ESI and HREI mass spectra acquired using a Finnigan Navigator30086and JMS-700MSTATION high resolution mass spectrometer system,respectively.The HPLC was carried out on a Waters HPLC system equipped with a Waters996photo-diode array detector and Millenium32software using C18 column(J’sphere ODS-H80,150mm920mm,4l m, YMC Co.).Extraction and isolationThe powdered S.siliquastrum(400g)was extracted with 80%aqueous MeOH,and was evaporated under vacuo.Then,the MeOH extract was partitioned with CHCl3.The chloroform extract(9g)was fractionated by silica column chromatography using stepwise elution with CHCl3–MeOH mixture(100:1?1:1)to afford separated active fractions.A combined active fraction was further subjected to a Sephadex LH-20column saturated with100%MeOH, and thenfinally purified by reversed-phase HPLC to give fucoxanthin(74.9mg).1H and13C NMR data were assigned in Table1and the structure was illustrated in Fig.1.Cell cultureCells of a monkey kidneyfibroblast line(Vero)were maintained at37°C in an incubator with humidified atmosphere of5%CO2.Cells were cultured in Dulbecco’s modified Eagle’s medium containing10%heat-inactivated fetal calf serum,streptomycin(100l g/mL),and penicillin (100U/mL).Intracellular ROS measurementFor detection of intracellular ROS,Vero cells were seeded in96-well plates at a concentration of19105cells/mL. After16h,the cells were treated with various concentra-tions of fucoxanthin,and incubated at37°C under a humidified atmosphere.After30min,H2O2was added at a concentration of1mM,and then cells were incubated for additional30min at37°C.Finally,20,70-dichlorodihy-drofluorescein diacetate(DCFH-DA;5l g/mL)was introduced to the cells,and20,70-dichlorofluorescein(DCF)fluorescence was detected at an excitation wavelength of 485nm and an emission wavelength of535nm,using a Perkin-Elmer LS-5B spectrofluorometer.The percentage of intracellular ROS scavenging activity was calculated in accordance with the following equation:Intracellular ROS scavenging activity%ðÞ¼1ÀC1=C0ðÞ½ Â100where C1is thefluorescence intensity of cells treated with H2O2and fucoxanthin,and C0is thefluorescence intensity of cells treated with H2O2and distilled water instead of fucoxanthin.Assessment of cell viabilityCell viability was then estimated via an3-(4,5-dimethyl-thiazol-2-yl)2,5-diphenyltetrazolium bromide(MTT) assay,which is a test of metabolic competence predicated upon the assessment of mitochondrial performance.It is colorimetric assay,which is dependent on the conversion of yellow tetrazolium bromide to its purple formazan derivative by mitochondrial succinate dehydrogenase inviable cells[11].The cells were seeded in96-well plate at a concentration of19105cells/mL.After16h,the cells were treated with fucoxanthin at difference concentrations. Then,10l L of H2O2(1mM)was added to the cell culture medium,and incubated for24h at37°C.MTT stock solution(50l L;2mg/mL)was then applied to the wells, to a total reaction volume of200l L.After4h of incu-bation,the plates were centrifuged for5min at8009g,and the supernatants were aspirated.The formazan crystals in each well were dissolved in150l L of dimethylsulfoxide (DMSO),and the absorbance was measured via ELISA at a wavelength of540nm.Relative cell viability was evalu-ated in accordance with the quantity of MTT converted to the insoluble formazan salt.The optical density of the formazan generated in the control cells was considered to represent100%viability.The data are expressed as mean percentages of the viable cells versus the respective control.Determination of DNA damage by comet assayComet assay was performed to determine the oxidative DNA damage[12].The cell suspension was mixed with 75l L of0.5%low melting agarose(LMA),and added to the slides precoated with1.0%normal melting agarose. After solidification of the agarose,slides were covered with another75l L of0.5%LMA and then immersed in lysis solution(2.5M NaCl,100mM EDTA,10mM Tris,andTable1NMR spectroscopic data for fucoxanthin in CDCl3Position13C1H(mult.J=Hz)Position13C1H(mult.J=Hz) 135.81035.2247.1 1.36(1H,dd,J=8.7,14.2)1.49(1H,dd,J=14.2)2045.4 1.41(1H,dd,J=10.4,14.9)2.00(1H,dd,J=2.9,14.9)364.3 3.80(1H,m)3068.0 5.37(1H,tt,J=8.8,12.0)441.6 1.77(1H,dd,J=8.7,14.2)2.29(1H,dd,J=2.9,17.8)4045.2 1.53(1H,dd,J=10.4,14.9)2.29(1H,dd,J=2.9,17.8)566.25072.7667.160117.5740.8 2.59,3.64(2H,d,J=20.4)70202.48170.580103.4 6.04(1H,s)9134.590132.510139.17.14(1H,d,J=12.8)100128.5 6.12(1H,d,J=11.6) 11123.4 6.58(1H,m)110125.7 6.71(1H,t,J=12.0) 12145.0 6.66(1H,t,J=12.8)120137.1 6.34(1H,d,J=11.6) 13135.4130138.114136.6 6.40(1H,d,J=11.6)140132.2 6.26(1H,d,J=11.6) 15129.4 6.67(1H,m)150132.5 6.71(1H,t,J=12.0,14.2) 1625.0 1.37(3H,s)16029.2 1.37(3H,s)1728.10.95(3H,s)17032.1 1.06(3H,s)1821.2 1.34(3H,s)18031.3 1.34(3H,s)1911.8 1.80(3H,s)19014.0 1.80(3H,s)2012.8 1.98(3H,s)20012.9 1.98(3H,s)30OAc,CH321.4 2.03(3H,s)30OAc,C=O197.9Data were obtained using400MHz for1H and100MHz for13C1%sodium lauryl sarcosine;1%Triton X-100and10% DMSO)for1h at4°C.The slides were next placed into an electrophoresis tank containing300mM NaOH and 10mM Na2EDTA(pH13.0)for40min for DNA unwinding.For electrophoresis of the DNA,an electric current of25V/300mA was applied for20min at4°C. The slides were washed three times with a neutralizing buffer(0.4M Tris,pH7.5)for5min at4°C,and then treated with ethanol for another5min before staining with 50l L of ethidium bromide(20l g/mL).Measurements were made by image analysis(Kinetic Imaging,Komet5.0, UK)andfluorescence microscope(LEICA DMLB,Ger-many),determining the percentage offluorescence in the tail(tail intensity;50cells from each of two replicate slides).Nuclear staining with Hoechst33342The nuclear morphology of the cells was evaluated using the cell-permeable DNA dye,Hoechst33342.Cells with homogeneously stained nuclei were considered viable, whereas the presence of chromatin condensation and/or fragmentation was indicative of apoptosis[13,14].The cells were placed in24-well plates at a concentration of 19105cells/mL.Sixteen hours after plating,the cells were treated with various concentration of fucoxanthin,and further incubated for1h prior to expose to H2O2(1mM). After24h,1.5l L of Hoechst33342(stock10mg/mL),a DNA-specificfluorescent dye,were added to each well, followed by10min of incubation at37°C.The stained cells were then observed under afluorescence microscope equipped with a CoolSNAP-Pro color digital camera,in order to examine the degree of nuclear condensation.Statistical analysisThe data are expressed as the mean±standard error.A statistical comparison was performed via the SPSS package for Windows(Version10).p-Values of\0.05were con-sidered to be significant.Results and discussionCells are protected from ROS-induced damage by a variety of endogenous ROS-scavenging enzymes,chemical com-pounds,and natural products.Recently,interest has been increased in the therapeutic potential of natural products to be used as natural antioxidants in the reduction of such freeradical-induced tissue injuries,thereby suggesting that many natural products may prove to possess therapeuti-cally useful antioxidant compounds [15–17].H 2O 2has been extensively used as an inducer of oxi-dative stress in in vitro models.It readily crosses the cellular membranes giving rise to the highly reactive hydroxyl radical,which has the ability to react with mac-romolecules,including DNA,proteins,and lipids,and ultimately damage to whole cell [18,19].Several studies have shown that oxidative stress is a major cause of cellular injuries in a variety of human diseases including cancer,neurodegenerative,and cardiovascular disorders [20–22].Recently,many researchers have made considerable efforts to search natural antioxidants.Many studies have revealed that seaweeds have potential to be used as a candidate for natural antioxidant.Potent antioxidative compounds have already been isolated from seaweeds and identified as phylopheophytin in Eisenia bicyclis (arame),fucoxanthine in Hijikia fusiformis (hijiki),phlorotannin in Ecklonia stolonifera ,bromophenols in Polysiphonia urceolata ,and sulfated polysaccharides [6,23–27].Among these antiox-idants,fucoxanthine,a kind of carotenoid,showed radical scavenging activity.In this study,we investigated the antioxidant effects of natural carotenoid fucoxanthin,after the administration of H 2O 2in cell lines.20,70-Dichlorodihydrofluorescein diacetate was used as a probe for ROS measurement.DCFH-DA crosses cell membranes and is hydrolyzed enzymatically by intracel-lular esterase to nonfluorescent DCFH.In the presence of ROS,DCFH is oxidized to highly fluorescent DCF.It is well known that H 2O 2is the principal ROS responsible for the oxidation of DCFH to DCF [28].In the presentstudy,Fig.5Photomicrographs of DNA damage and migration observed under fucoxanthin isolated from S.siliquastrum where the tail moments were decreased.a control,b cells treated with 50l M H 2O 2,c cells treated with 5l M compound +50l M H 2O 2,d cells treated with 50l M compound +50l M H 2O 2,e cells treated with 200l M compound +50l M H 2O 2we investigated scavenging activity of fucoxanthin on intracellular ROS and the results are illustrated in Fig.2.Fluorescence intensity of control cells was recorded as 3398while H 2O 2treated cells showed 7452.However,the addition of fucoxanthin to the cells mixed with H 2O 2reduced intracellular ROS accumulation to 6079,4867,and 3149at 5,50,and 100l M,respectively.Moreover,the scavenging activity of fucoxanthin on intracellular ROS was dose-dependently increased as 18.4,34.7,and 57.7%at 5,50,and 100l M,respectively.Hence these results indicate that fucoxanthin has efficient antioxidant proper-ties which can be developed into a potential bio-molecular candidate to inhibit ROS formation of cellular.To evaluate whether fucoxanthin protect from cellular damage induced by H 2O 2,cells were pretreated with fuco-xanthin for 24h in the absence or presence of oxidative stress.After then,cell viability was measured via MTT assay.As shown in Fig.3,H 2O 2treatment without fuco-xanthin decreased cell viability to 43.7%,while fucoxanthin prevented cells from H 2O 2-induced damage,restoring cell survival to 63.6,69.4,78.5,and 89.2%at the concentrations of 5,50,100,and 200l M,respectively.Active mitochon-dria of living cells can cleave MTT to produce formazan,the amount of which is directly correlated to the living cell number [29].The results of MTT assay showed that for-mazan content was reduced due to H 2O 2treatment,however,significantly increased with addition of fucoxan-thin.These results suggest that fucoxanthin has ability to protect vero cells from oxidative stress-related cellular injuries.The protective effect of fucoxanthin on DNA damages was also confirmed by comet assay and was presented as tail DNA percent.This assay can reflect different types of DNA damage,such as DNA single strand breakage,or incomplete DNA repairing and shows high sensitivity in detecting oxidative stress [30].As shown in Fig.4,H 2O 2treatment increased the tail moments in the cells versus the control cells.However,the increased tail moments caused by H 2O 2induced oxidative DNA damage were decreased in the cells treated with fucoxanthin.The inhibitioneffectFig.6Protective effect of fucoxanthin isolated from S.siliquastrum against H 2O 2-induced apoptosis in vero cells.Cellular morphological changes were observed under afluorescence microscope after Hoechst 33342staining.a untreated,b 500l M H 2O 2,c 5l M compound +500l M H 2O 2,d 50l Mcompound +500l M H 2O 2,e 200l Mcompound +500l M H 2O 2.Apoptotic bodies are indicated by arrowsof fucoxanthin on DNA damage was38.8,52.5,and63.4% at the concentrations of5,50,and200l M,respectively. The DNA migration profiles of tested cells when treated with different concentrations of fucoxanthin are presented in Fig.5.The DNA was completely damaged and the amount of tail DNA was significantly increased in the group treated with only H2O2(Fig.5b),compared to the control(Fig.5a).However,the amount of tail DNA was increasingly decreased with increased concentrations of fucoxanthin(Fig.5c–e).In a previous study,Kang et al.[23]reported that some natural compounds from brown seaweeds have an ability to increase catalase located at peroxisome in cell that converts H2O2into molecular oxygen and water.In this study,we showed that fuco-xanthin has prominent effect on the H2O2-induced DNA damage.The inhibition activities on the DNA damage and the decrease of the amounts of tail DNA might be related to the ability of fucoxanthin to increase catalase.The protective effect of fucoxanthin on apoptosis induced by H2O2was investigated by nuclear staining with Hoechst33342.Hoechst33342dye specifically stains DNA,and is widely used to detect the nuclear shrinkage such as chromatin condensation,nuclear fragmentation, and the appearance of apoptotic bodies,which are all indications of apoptosis[31].The microscopic photograph in Fig.6,the negative control,which contained no com-pound or H2O2,possessed intact nuclei(Fig.6a),and the positive control(H2O2treated cells)exhibited significant nuclear fragmentation,indicating apoptosis(Fig.6b). However,the addition of fucoxanthin with H2O2reduced the apoptotic bodies(Fig.6c–e),which suggested the ability of fucoxanthin to protect against nuclear fragmen-tation amidst oxidative stress.In conclusion,the results obtained in the present study show that fucoxanthin isolated from S.siliquastrum can effectively inhibit intracellular ROS formation,DNA damage,and apoptosis induced by H2O2.Moreover, fucoxanthin showed evidence in cell survival rate.These results revealed that fucoxanthin can be used not only as the easily accessible source of natural antioxidants but also as an ingredient of the functional food and cosmetic agents related to the prevention and control oxidative stress. Acknowledgements This research was supported by a grant(M-2007-03)from the Marine Bioprocess Research Center of the Marine Bio21Center,funded by Ministry of Marine Affairs and Fisheries, Republic of Korea.References1.Schwarz K,Bertelsen G,Nissen LR,Gardner PT,Heinonen MI,Hopia A,Huynh-Ba T,Lambelet P,McPhail D,Skibsted LH, Tijburg L(2001)Eur Food Res Technol212:319–3282.Farag RS,El-Baroty GS,Basuny AM(2003)Int J Food SciTechnol38:81–873.Heo SJ,Park EJ,Lee KW,Jeon YJ(2005)Bioresour Technol96:1613–16234.Jimenez-Escrig A,Jimenez-Jimenez I,Pulido R,Saura-Calixto F(2001)J Sci Food Agric81:530–5345.Berhard K,Moss GP,Toth GY,Weedon BLC(1976)Tetrahe-dron Lett17:115–1186.Yan X,Chuda Y,Suzuki M,Nagata T(1999)Biosci BiotechnolBiochem63:605–6077.Hosokawa M,Kudo M,Maeda H,Kohno H,Tanaka T,MiyashitaK(2004)Biochim Biophys Acta1675:113–1198.Maeda H,Hosokawa M,Sashima T,Funayama K,Miyashita K(2005)Biochem Biophys Res Commun332:392–3979.Kotake-Nara E,Asai A,Nagao A(2005)Cancer Lett220:75–8410.Sugawara T,Matsubara K,Akagi R,Mori M,Hirata T(2006)JAgric Food Chem54:9805–981011.Mosmann T(1983)J Immunol Methods65:55–6312.Singh NP(2000)Mutat Res455:111–12713.Gschwind M,Huber G(1995)J Neurochem65:292–30014.Lizard G,Fournel S,Genestier L,Dhedin N,Chaput C,FlacherM,Mutin M,Panaye G,Revillard JP(1995)Cytometry21:275–28315.Lee SE,Ju EM,Kim JH(2002)Exp Mol Med34:100–10616.Jung WK,Rajapakse N,Kim SK(2005)Eur Food Res Technol220:535–53917.Ahn GN,Kim KN,Cha SH,Song CB,Lee J,Heo MS,Yeo IK,Lee NH,Jee YH,Kim JS,Heo MS,Jeon YJ(2007)Eur Food Res Technol226:71–7918.Satoh T,Sakai N,Enokido Y,Uchiyama Y,Hatanaka H(1996)JBiochem120:540–54619.Halliwell B,Clement MV,Long LH(2000)FEBS Lett486:10–1320.Al-Enezi KS,Alkhalaf M,Benov LT(2006)Free Radic Biol Med40:1144–115121.Zhang L,Yu H,Sun Y,Lin X,Chen B,Tan C,Cao G,Wang Z(2007)Eur J Pharmacol564:18–2522.Strazzullo P,Puig JG(2007)Nutr Metab Cardiovasc Dis17:409–41423.Kang HS,Chung HY,Jung HA,Son BW,Choi JS(2003)ChemPharm Bull51:1012–101424.Cahyana AH,Shuto Y,Kinoshita Y(1992)Biosci BiotechnolBiochem56:1533–153525.Li K,Li XM,Ji NY,Wang BG(2007)Bioorg Med Chem15:6627–663126.Zhang Q,Li N,Liu W,Zhao Z,Li Z,Xu Z(2004)Carbohydr Res339:105–11127.Kim SH,Choi DS,Athucorala Y,Jeon YJ,Senevirathne M,RhaCK(2007)Int J Food Sci Nutr12:65–7328.LeBel CP,Ischiopoulos H,Bondy SC(1992)Chem Res Toxicol5:227–23129.Hu Z,Guan W,Wang W,Huang L,Xing H,Zhu Z(2007)CellBiol Int31:798–80430.Senthilmohan ST,Zhang J,Stanley RA(2003)Nutr Res23:1199–121031.Kerr JF,Gobe GC,Winterford CM,Harmon BV(1995)MethodsCell Biol46:1–27。
光伏电池栅线英语
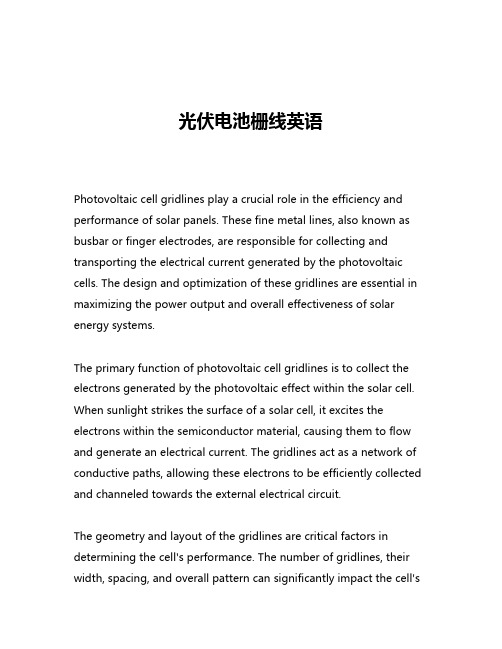
光伏电池栅线英语Photovoltaic cell gridlines play a crucial role in the efficiency and performance of solar panels. These fine metal lines, also known as busbar or finger electrodes, are responsible for collecting and transporting the electrical current generated by the photovoltaic cells. The design and optimization of these gridlines are essential in maximizing the power output and overall effectiveness of solar energy systems.The primary function of photovoltaic cell gridlines is to collect the electrons generated by the photovoltaic effect within the solar cell. When sunlight strikes the surface of a solar cell, it excites the electrons within the semiconductor material, causing them to flow and generate an electrical current. The gridlines act as a network of conductive paths, allowing these electrons to be efficiently collected and channeled towards the external electrical circuit.The geometry and layout of the gridlines are critical factors in determining the cell's performance. The number of gridlines, their width, spacing, and overall pattern can significantly impact the cell'sability to collect and transport the generated current. Optimizing these parameters is a delicate balance between maximizing the conductive area while minimizing the shadowing effect caused by the gridlines themselves.One of the key challenges in designing photovoltaic cell gridlines is to strike a balance between electrical conductivity and optical transparency. The gridlines need to be highly conductive to minimize resistive losses and ensure efficient current collection, but they should also minimize the amount of light they block from reaching the active semiconductor material. This trade-off is often referred to as the "finger shading" problem, where the gridlines can potentially reduce the overall solar cell efficiency if not designed properly.To address this challenge, researchers and engineers have developed various techniques and materials to improve the performance of photovoltaic cell gridlines. One approach is to use thin, narrow gridlines with a high aspect ratio, which can reduce the shadowing effect while maintaining good conductivity. This can be achieved through the use of advanced manufacturing techniques, such as screen printing, inkjet printing, or even laser-based patterning.Another strategy is to explore alternative grid materials and geometries. Instead of traditional metal-based gridlines, some researchers have investigated the use of transparent conductiveoxides (TCOs), such as indium tin oxide (ITO) or aluminum-doped zinc oxide (AZO). These materials can provide good electrical conductivity while allowing more light to pass through the cell, improving the overall efficiency.In addition to the gridline design, the integration of the gridlines with the rest of the solar cell structure is also crucial. The gridlines need to form a reliable and low-resistance contact with the semiconductor material, which can be achieved through careful surface preparation, metallization processes, and the use of appropriate adhesion layers or barrier materials.Furthermore, the durability and reliability of photovoltaic cell gridlines are essential considerations, as they need to withstand the harsh environmental conditions encountered during the lifetime of a solar panel. Factors such as thermal cycling, mechanical stress, humidity, and corrosion can all contribute to the degradation of the gridlines, leading to reduced performance and potential failures. Addressing these reliability challenges through material selection, encapsulation, and packaging design is an active area of research and development in the solar energy industry.As the demand for renewable energy continues to grow, the optimization of photovoltaic cell gridlines has become increasingly important. By improving the design, materials, and integration ofthese critical components, researchers and engineers can contribute to the overall advancement of solar energy technology, making it more efficient, cost-effective, and accessible to a wider range of applications and consumers.In conclusion, photovoltaic cell gridlines are a fundamental element in the performance and efficiency of solar panels. The continuous efforts to optimize their design, materials, and integration within the solar cell structure are crucial in driving the progress of the solar energy industry and contributing to a more sustainable energy future.。
仪器确认 附件3 紫外确认 中英文.doc

OMCL Network of the Council of Europe QUALITY ASSURANCE DOCUMENTPA/PH/OMCL (07) 11 DEF CORRQUALIFICATION OF EQUIPMENT仪器确认ANNEX 3: QUALIFICATION OF UV-VISIBLESPECTROPHOTOMETERSANNEX 3 OF THE OMCL NETWORK GUIDELINE“QUALIFICATION OF EQUIPMENT”OMCL网络指南“仪器确认”附件3QUALIFICATION OF UV-VISIBLE SPECTROPHOTOMETERS紫外-可见光分光光度仪的确认IntroductionThe present document is the third Annex of the core document “Qualification of Equipment”, and it should be used in combination with it when planning, performing and documenting the UV-Visible spectrophotometer qualification process.本文件是核心文件“仪器的确认”的第三个附件,在计划、实施和记录紫外-可见光分光光度计的确认时应与该核心文件结合使用。
The core document contains the Introduction and general forms for Level I and II of qualification, which are common to all type of instruments.核心文件包括了对第一级和第二级确认的介绍和一般表格,它们适用于所有类型的仪器。
For UV-Visible spectrometers, an example has been added to give instrument-specific proposals that may be used in combination with the general requirements presented in the core doc ument “Qualification of Equipment”, when drawing up a Level I checklist.对于紫外-可见光分光光度计,增加了一个例表给出仪器相关的方案,可以在规划第一级检查清单时,与核心文件里的通用要求“仪器的确认”结合使用。
整理液晶专业术语

液晶专业术语Backlight:背光。
CCFL(CCFT) (Cold Cathode Fluorescent 廿9^/7此6):冷阴极荧光灯。
Composite vide 复合视频。
Component vide 分量视频。
COB(Chip On Board):IC裸片通过邦定固定于印刷线路板上。
COF(Chip On Film):将IC封装于柔性线路板上。
COG(Chip On Glass):W IC 封装于玻璃上。
CRT(Cathode Radial Tube):阴极射线管。
DPI(Dot Per Inch):点每英寸。
Duty:占空比,高出点亮的阀值电压的部分在一个周期中所占的比率。
DVI(Digital Visual Interface):( VGA)数字接口。
ECB(Electrically Controlled Birefringence):电控双折射。
EL(Electro luminescence):电致发光。
EL层由高分子量薄片构成FSTN(Formulated STN):薄膜补偿型STN,用于黑白显示。
HTN(High Twisted Nematic):高扭曲向列的显示类型。
IC(Integrate Circuit):集成电路。
Inverter:逆变器。
ITO(Indium-Tin Oxide):氧化锢锡。
LCD(Liquid Crystal Display):液晶显示器。
LCM(Liquid Crystal Module):液晶模块。
LED(Light Emitting Diode):发光二极管。
LVDS(Low Voltage Differential Signaling):低压差分信号。
NTSC(National Television Systems Committee):NTSC 制式,全国电视系统委员会制式OSD(On Screen Display):在屏上显示。
PAL(Phase Alternating Line)PAL 制式(逐行倒相制式)。
- 1、下载文档前请自行甄别文档内容的完整性,平台不提供额外的编辑、内容补充、找答案等附加服务。
- 2、"仅部分预览"的文档,不可在线预览部分如存在完整性等问题,可反馈申请退款(可完整预览的文档不适用该条件!)。
- 3、如文档侵犯您的权益,请联系客服反馈,我们会尽快为您处理(人工客服工作时间:9:00-18:30)。
Height
Length Width Top contact based on Transmitting Conducting Oxides (T.C.O.) Semiconductor layers Bห้องสมุดไป่ตู้ttom contact
EFFECT OF CELL WIDTH ON THE DEVICE PERFORMANCE OF AMORPHOUS SILICON SOLAR CELLS
1
C. Monokroussos1, R. Rüther2, R. Gottschalg*,1, M. Kong1, D.G. Infield1 Centre for Renewable Energy Systems Technology, Department of Electronic and Electrical Engineering, Loughborough University, Loughborough, Leicestershire, LE11 3TU, UK, Tel.: +44-(0)1509-228148, email: R.Gottschalg@ 2 Labsolar, Universidade Federal de Santa Catarina, Caixa Postal 476, Florianopolis – SC 88040-900, Brazil Abstract: Thin film technologies are seen by many as the future of photovoltaics. Typically these devices have a top contact based on transparent conducting oxides (TCO) rather than a metal grid as with conventional crystalline silicon based devices. The resistance of these TCO layers is typically relatively high and thus effects of the distributed nature of the series resistance are more apparent. Furthermore, this distributed resistance will result in an increase of the effective series resistance of the device and hence it will influence the efficiency of the cell. This paper presents a modelling approach to include the effect of the distributed series resistance, based on the separation of current through the contacts and the device. To allow for the distributed series resistance, the current through the device is considered to consist of contributions from a number of sub-cells. Different cells were simulated, using different sheet resistances and different cell widths. It is shown that single junction cells below 20 mm width don’t lose more than 3.5% of their efficiency for sheet resistance values achieved in the production line. The effect of distributed series resistance is shown to be low for research samples but of importance for industrial samples. Keywords: a-Si, modelling, series resistance
1 INTRODUCTION Thin-film solar cells are seen by many as the future of photovoltaics. The reason is the combination of potentially low production cost and satisfactory efficiency. Thus the application of these technologies, although recently introduced into mass production, is expanding rapidly and may influence the market significantly. A key issue to be investigated is to increase the efficiency without escalating production costs. One possibility lies in optimising the cell geometry - in the case of thin film devices this can be achieved in two ways: optimising the layer structure, in order to optimise absorption and number of charge carriers reaching the junction, and ensuring that the interconnection effects are minimised. Latter has two main influences: wide cells will have, as explained below, a high series resistance due to the distributed nature of the resistance and the number of interconnects required for the cell, as these will create electrically dead areas. This paper is concerned with optimising the series resistance.*** Typically, thin-film cells have a top contact based on transparent conducting oxides (TCO) rather than a metal grid as with conventional crystalline silicon based devices. The resistivity of these TCO layers is relatively high. An effect of a distributed series resistance is observed for metal grids [1, 2]. A much more pronounced effect is observed for thin film photovoltaics [3]. This distributed resistance will result in an increase of the effective series resistance of the device with increasing width and hence it will influence the efficiency of the cell. This would suggest that an ideal cell should be as thin as possible. However, decreasing the width of cell will increase the number of interconnects which will add series resistance and also result in electrically inactive material of a width of 0.5 to 2 mm. Consequently, there * corresponding author
is an optimal width, which this project aims to investigate for a given class of devices. 2 MODEL DESCRIPTION In order to model the current through a photovoltaic device, one has to consider the different current directions as illustrated in Figure 1. In the following, the cell is assumed to be lying on a horizontal surface, with the top pointing to the sky. The current through the active semiconductor (p-n or p-i-n junction) of the cell is vertical, while the current through the contacting layers is horizontal. This will result in a variation of the resistance experienced by charge carriers, depending on the distance travelled within the TCO and the back contact. There is a significant difference in the conductivity of the back contact, usually aluminium or silver, and the TCO layer. The resistivity of TCO is typically 3.10-4Ωcm, while aluminium and silver present resistivities in the order of 10-6Ωcm. Hence the TCO is the dominant factor and the back contact resistance plays a less significant role. However, it should be noted that neglecting the back contact and concentrating purely on the TCO will overestimate the effect of distributed series resistance.