Analysis of cut-and-cover tunnels against large tectonic deformation
英语建筑专业单词表
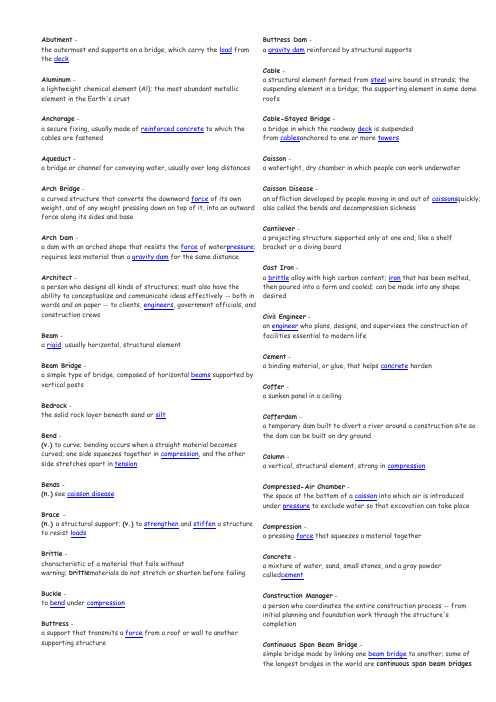
Abutment -the outermost end supports on a bridge, which carry the load from the deckAluminum -a lightweight chemical element (Al); the most abundant metallic element in the Earth's crustAnchorage -a secure fixing, usually made of reinforced concrete to which the cables are fastenedAqueduct -a bridge or channel for conveying water, usually over long distancesArch Bridge -a curved structure that converts the downward force of its own weight, and of any weight pressing down on top of it, into an outward force along its sides and baseArch Dam -a dam with an arched shape that resists the force of water pressure; requires less material than a gravity dam for the same distanceArchitect -a person who designs all kinds of structures; must also have the ability to conceptualize and communicate ideas effectively -- both in words and on paper -- to clients, engineers, government officials, and construction crewsBeam -a rigid, usually horizontal, structural elementBeam Bridge -a simple type of bridge, composed of horizontal beams supported by vertical postsBedrock -the solid rock layer beneath sand or siltBend -(v.) to curve; bending occurs when a straight material becomes curved; one side squeezes together in compression, and the other side stretches apart in tensionBends -(n.) see caisson diseaseBrace -(n.) a structural support; (v.) to strengthen and stiffen a structure to resist loadsBrittle -characteristic of a material that fails withoutwarning; brittle materials do not stretch or shorten before failingBuckle -to bend under compressionButtress -a support that transmits a force from a roof or wall to another supporting structure Buttress Dam -a gravity dam reinforced by structural supportsCable -a structural element formed from steel wire bound in strands; the suspending element in a bridge; the supporting element in some dome roofsCable-Stayed Bridge -a bridge in which the roadway deck is suspendedfrom cables anchored to one or more towersCaisson -a watertight, dry chamber in which people can work underwaterCaisson Disease -an affliction developed by people moving in and out of caissons quickly; also called the bends and decompression sicknessCantilever -a projecting structure supported only at one end, like a shelf bracket or a diving boardCast Iron -a brittle alloy with high carbon content; iron that has been melted, then poured into a form and cooled; can be made into any shape desiredCivil Engineer -an engineer who plans, designs, and supervises the construction of facilities essential to modern lifeCement -a binding material, or glue, that helps concrete hardenCoffer -a sunken panel in a ceilingCofferdam -a temporary dam built to divert a river around a construction site so the dam can be built on dry groundColumn -a vertical, structural element, strong in compressionCompressed-Air Chamber -the space at the bottom of a caisson into which air is introduced under pressure to exclude water so that excavation can take placeCompression -a pressing force that squeezes a material togetherConcrete -a mixture of water, sand, small stones, and a gray powdercalled cementConstruction Manager -a person who coordinates the entire construction process -- from initial planning and foundation work through the structure's completionContinuous Span Beam Bridge -simple bridge made by linking one beam bridge to another; some of the longest bridges in the world are continuous span beam bridgesCore -central region of a skyscraper; usually houses elevator and stairwellCut and Cover -a method of tunnel construction that involves digging a trench, building a tunnel, and then covering it with fillDeck -supported roadway on a bridgeDeform -to change shapeDiversion Channel -a bypass created to divert water around a dam so that construction can take placeDome -a curved roof enclosing a circular space; a three-dimensional archDownstream Face -the side of the dam that is not against the waterDynamite -a blasting explosive, based on nitroglycerin, but much safer to handle than nitroglycerin aloneElectrical Engineer -an engineer concerned with electrical devices and systems and with the use of electrical energyEmbankment Dam -a dam composed of a mound of earth and rock; the simplest typeof gravity damEngineering -a profession in which a knowledge of math and natural science is applied to develop ways to utilize the materials and forces of nature for the benefit of all human beingsEnvironmental Engineer -an engineer who designs and operates systems to provide safe drinking water and to prevent and control pollution in water, in the air, and on the landFire-Setting -an ancient tunneling technique in which rock is heated with fire and then doused with cold water, causing the rock to fractureForce -any action that tends to maintain or alter the position of a structureGeodesic Dome -a dome composed of short, straight pieces joined to form triangles; invented by Buckminster FullerGeotechnical Engineer -an engineer who evaluates and stabilizes foundations for buildings, roads, and other structuresGravity Dam -a dam constructed so that its great weight resists the force of water pressure Gunpowder -any of several low-explosive mixtures used as a blasting agent in mining and tunneling; the first such explosive was black powder, which consists of a mixture of potassium nitrate, sulfur, and charcoalJoint -a device connecting two or more adjacent parts of a structure;a roller joint allows adjacent parts to move controllably past one another; a rigid joint prevents adjacent parts from moving or rotating past one anotherLoad -weight distribution throughout a structure; loads caused by wind, earthquakes, and gravity, for example, affect how weight is distributed throughout a structureMasonry -a building material such as stone, clay, brick, or concreteMechanical Engineer -an engineer who applies the principles of mechanics and energy to the design of machines and devicesMonolithic Dome -a dome composed of a series of arches, joined together with a series of horizontal rings called parallelsMovable Bridge -a bridge in which the deck moves to clear a navigation channel;a swing bridge has a deck that rotates around a center point;a drawbridge has a deck that can be raised and lowered; a bascule bridge deck is raised with counterweights like a drawbridge; and the deck of a lift bridge is raised vertically like a massive elevatorNitroglycerin -an explosive compound made from a mixture of glycerol and concentrated nitric and sulfuric acids, and an important ingredient of most forms of dynamitePerimeter -the distance around the outside of a shapePier -a vertical supporting structure, such as a pillarPendentive -a triangular shape that adapts the circular ring of a dome to fit onto a flat supporting wallPile -a long, round pole of wood, concrete, or steel driven into the soilby pile driversPile Driver -a noisy machine that repeatedly drops a heavy weight on top ofa pile until the pile reaches solid soil or rock or cannot be pushed down any fartherPlastic -a synthetic material made from long chains of molecules; has the capability of being molded or shaped, usually by the application of heat and pressurePressure -a force applied or distributed over an areaReinforced Concrete -concrete with steel bars or mesh embedded in it forincreased strength in tension; in pre-tensioned concrete, the embedded steel bars or cables are stretched into tension before the concrete hardens; in post-tensioned concrete, the embedded steel bars or cables are stretched into tension after the concrete hardensRichter Scale -used to measure the magnitude of an earthquake; introduced in 1935 by the seismologists Beno Gutenberg and Charles Francis RichterRigid -(adj.) ability to resist deformation when subjected toa load;rigidity(n.) the measure of a structure's ability not to change shape when subjected to a loadRock Tunnel -a passage constructed through solid rockShear -a force that causes parts of a material to slide past one another in opposite directionsShear-Walls -solid concrete walls that resist shear forces; often used in buildings constructed in earthquake zonesSilt -sediment particles ranging from 0.004 to 0.06 mm (0.00016 to0.0024 inch) in diameterSoft-Ground Tunnels -a passage constructed through loose, unstable, or wet ground, requiring supports to keep the walls from collapsingSpan -(n.) the distance a bridge extends between two supports; (v.) to traverse a specific distanceSpillway -an overflow channel that allows dam operators to release lake water when it gets high enough to threaten the safety of a damSpire -an architectural or decorative feature of a skyscraper; the Council on Tall Buildings and Urban Habitat includes spires but not antennae when calculating the official height of a skyscraperStable -(adj.) ability to resist collapse and deformation; stability(n.)characteristic of a structure that is able to carry arealistic load without collapsing or deforming significantlySteel -an alloy of iron and carbon that is hard, strong, and malleableStiff -(adj.) ability to resist deformation; stiffness (n.) the measure of a structure's capacity to resist deformation Strong -(adj.) ability to carry a realistic load; strength (n.) the measure of a structure's ability to carry a realistic loadStructural Engineer -an engineer who investigates the behavior and design of all kinds of structures, including dams, domes, tunnels, bridges, and skyscrapers, to make sure they are safe and sound for human useSuspension Bridge -a bridge in which the roadway deck is suspended from cables that pass over two towers; the cables are anchored in housings at either end of the bridgeTailings Dam -a dam, usually made of earth and rock, used to contain mining wasteTensegrity -an array of tension cables and compression rods that supports a structure; invented by Buckminster Fuller student Kenneth SnellsonTension -a stretching force that pulls on a materialTension Ring -a support ring that resists the outward force pushing against the lower sides of a domeTorsion -an action that twists a materialTower -the vertical structure in a suspension bridge or cable-stayed bridge from which cables are hung; also used loosely as a synonym for the term skyscraperTruss -a rigid frame composed of short, straight pieces joined to form a series of triangles or other stable shapesTuned Mass Damper -a mechanical counterweight designed to reduce the effects of motion, such as the swaying of a skyscraper in the wind or in an earthquakeTunnel Boring Machine (TBM) -a mechanical device that tunnels through the groundTunnel Shield -a cylinder pushed ahead of tunneling equipment to provide advance support for the tunnel roof; used when tunneling in softor unstable groundUnstable -characteristic of a structure that collapses or deforms under a realistic loadUpstream Face -the side of a dam that is against the waterWrought Iron -an iron alloy that is less brittle than cast iron。
地质与岩土工程专业英语论文tb

岩土工程英语作业姓名:汤彪学号:013621814102班级:0133018141SHORT COMMUNICATIONS ANALYTICAL METHOD FOR ANALYSIS OFSLOPE STABILITYJINGGANG CAOs AND MUSHARRAF M. ZAMAN*tSchool of Civil Engineering and Environmental Science,University of Oklahoma, Norman, OK 73019, U.S.A.SUMMARYAn analytical method is presented for analysis of slopestability involving cohesive and non-cohesive soils.Earthquakeeffects are considered in an approximate manner in terms ofseismic coe$cient-dependent forces. Two kinds of failure surfaces areconsidered in this study: a planar failure surface, and acircular failure surface. The proposed method can be viewed asan extension of the method of slices, but it provides a moreaccurate etreatment of the forces because they are representedin an integral form. The factor of safety is obtained by usingthe minimization technique rather than by a trial and errorapproach used commonly.The factors of safety obtained by the analytical method arefound to be in good agreement with those determined by the localminimum factor-of-safety, Bishop's, and the method of slices. Theproposed method is straightforward, easy to use, and lesstime-consuming in locating the most critical slip surface andcalculating the minimum factor of safety for a given slope.Copyright ( 1999) John Wiley & Sons, Ltd.Key words: analytical method; slope stability; cohesive andnon-cohesive soils; dynamic effect; planar failure surface;circular failure surface; minimization technique;factor-of-safety.INTRODUCTIONOne of the earliest analyses which is still used in manyapplications involving earth pressure was proposed by Coulomb in1773. His solution approach for earth pressures against retainingwalls used plane sliding surfaces, which was extended to analysis of slopes in 1820 by Francais. By about 1840, experience with cuttings and embankments for railways and canals in England and France began to show that many failure surfaces in clay were not plane, but signi"cantly curved. In 1916, curved failure surfaces were again reported from the failure of quay structures in Sweden. In analyzing these failures, cylindrical surfaces were used and the sliding soil mass was divided into a number of vertical slices. The procedure is still sometimes referred to as the Swedish method of slices. By mid-1950s further attention was given to the methods of analysis usingcircular and non-circular sliding surfaces . In recent years, numerical methods have also been used in the slope stability analysis with the unprecedented development of computer hardware and software. Optimization techniques were used by Nguyen,10 and Chen and Shao. While finite element analyses have great potential for modelling field conditions realistically, they usually require signi"cant e!ort and cost that may not be justi"ed in some cases.The practice of dividing a sliding mass into a number of slices is still in use, and it forms the basis of many modern analyses.1,9 However, most of these methods use the sums of the terms for all slices which make the calculations involved in slope stability analysis a repetitive and laborious process.Locating the slip surface having the lowest factor of safety is an important part of analyzing a slope stability problem. A number of computer techniques have been developed to automate as much of this process as possible. Most computer programs use systematic changes in the position of the center of the circle and the length of the radius to find the critical circle.Unless there are geological controls that constrain the slip surface to a noncircular shape, it can be assumed with a reasonablecertainty that the slip surface is circular.9 Spencer (1969) found that consideration of circular slip surfaces was as critical as logarithmic spiral slip surfaces for all practical purposes. Celestino and Duncan (1981), and Spencer (1981) found that, in analyses where the slip surface was allowed to take any shape, the critical slip surface found by the search was essentially circular. Chen (1970), Baker and Garber (1977), and Chen and Liu maintained that the critical slip surface is actually a log spiral. Chen and Liu12 developed semi-analytical solutions using variational calculus, for slope stability analysis with a logspiral failure surface in the coordinate system. Earthquake e!ects were approximated in terms of inertiaforces (vertical and horizontal) defined by the corresponding seismic coe$cients. Although this is one of the comprehensive and useful methods, use of /-coordinate system makes the solution procedure attainable but very complicated. Also, the solutions are obtained via numerical means at the end. Chen and Liu12 have listed many constraints, stemming from physical considerations that need to be taken into account when using their approach in analyzing a slope stability problem.The circular slip surfaces are employed for analysis of clayey slopes, within the framework of an analytical approach, in this study. The proposed method is more straightforward and simpler than that developed by Chen and Liu. Earthquake effects are included in the analysis in an approximate manner within the general framework of static loading. It is acknowledged that earthquake effects might be better modeled by including accumulated displacements in the analysis. The planar slip surfaces are employed for analysis of sandy slopes. A closed-form expression for the factor of safety is developed, which is diferent from that developed by Das.STABILITY ANALYSIS CONDITIONS AND SOIL STRENGTHThere are two broad classes of soils. In coarse-grained cohesionless sands and gravels, the shear strength is directly proportional to the stress level:''tan f τσθ= (1)where fτ is the shear stress at failure, /σ the effectivenormal stress at failure, and /θ the effective angle of shearing resistance of soil.In fine-grained clays and silty clays, the strength depends on changes in pore water pressures or pore water volumes which take place during shearing. Under undrained conditions, the shear strength cu is largely independent of pressure, that is u θ=0. When drainage is permitted, however, both &cohesive' and &frictional' components ''(,)c θ are observed. In this case the shear strength is given by(2)Consideration of the shear strengths of soils under drained and undrained conditions, and of the conditions that will control drainage in the field are important to include in analysis of slopes. Drained conditions are analyzed in terms of effective stresses, using values of ''(,)c θ determined from drained tests, or from undrained tests with pore pressure measurement. Performing drained triaxial tests on clays is frequently impractical because the required testing time can be too long. Direct shear tests or CU tests with pore pressure measurement are often used because the testing time is relatively shorter.Stability analysis involves solution of a problem involving force and/or moment equilibrium.The equilibrium problem can be formulated in terms of (1) total unit weights and boundary water pressure; or (2) buoyant unit weights and seepage forces. The first alternative is a better choice, because it is morestraightforward. Although it is possible, in principle, to usebuoyant unit weights and seepage forces, that procedure is fraught with conceptual diffculties.PLANAR FAILURE SURFACEFailure surfaces in homogeneous or layered non-homogeneous sandy slopes are essentially planar. In some important applications, planar slides may develop. This may happen in slope, where permeable soils such as sandy soil and gravel or some permeable soils with some cohesion yet whose shear strength is principally provided by friction exist. For cohesionless sandy soils, the planar failure surface may happen in slopes where strong planar discontinuities develop, for example in the soil beneath the ground surface in natural hillsides or in man-made cuttings.ααβ图平面破坏Figure 1 shows a typical planar failure slope. From an equilibrium consideration of the slide body ABC by a vertical resolution of forces, the vertical forces across the base of the slide body must equal to weight w. Earthquake effects may be approximated by including a horizontal acceleration kg which produces a horizontal force k= acting through the centroid of the body and neglecting vertical inertia.1 For a slice of unit thickness in the strike direction, the resolved forces of normaland tangential components N and ¹ can be written as(cos sin )N W k αα=-(3)(sin cos )T W k αα=+(4) where is the inclination of the failure surface and w is given by02(tan tan )(tan )(cot cot )2LW x x dx H x dx H γβαγαγαβ=-+-=-⎰⎰ (5) where γ is the unit weight of soil, H the height of slope, cot ,cot ,L H l H βαβ== is the inclination of the slope. Since the length of the slide surface AB is /sin cH α, the resisting force produced by cohesion is cH /sin a. The friction force produced by N is (cos sin )tan W k ααφ-. The total resisting or anti-sliding force is thus given by(cos sin )tan /sin R W k cH ααφα=-+(6)For stability, the downslope slide force ¹ must not exceed the resisting force R of the body. The factor of safety, F s , in the slope can be defined in terms of effective force by ratio R /T, that is1tan 2tan tan (sin cos )sin()s k c F k H k αφαγααβα-=+++- (7) It can be observed from equation (7) that F s is a function of a. Thus the minimum value of F s can be found using Powell's minimization technique18 from equation (7). Das reported a similar expression for F s with k =0, developed directly from equation (2) by assuming that /s f d F ττ=, where f τ is the averageshear strength of the soil, and d τ the average shear stressdeveloped along the potential failure surface.For cohesionless soils where c =0, the safety factor can bereadily written from equation (7) as 1tan tan tan s k F k αφα-=+ (8) It is obvious that the minimum value of F s occurs when a=b, and the failure becomes independent of slope height. For such cases (c=0 and k=0), the factors of safety obtainedfrom the proposed method and from Das are identical.CIRCULAR FAILURE SURFACESlides in medium-stif clays are often deep-seated, and failure takes place along curved surfaces which can be closely approximated in two dimensions by circular surfaces. Figure 2 shows a potential circular sliding surface AB in two dimensions with centre O and radius r . The first step in the analysis is to evaluate the sliding' or disturbing moment M s about the centre of thecircle O . This should include the self-weight w of the sliding mass, and other terms such as crest loadings from stockpiles or railways, and water pressures acting externally to the slope.Earthquake effects is approximated by including a horizontal acceleration kg which produces a horiazontal force k d=acting through the centroid of each slice and neglecting vertical inertia. When the soil above AB is just on the point of sliding, the average shearing resistance which is required along AB for limiting equilibrium is given by equation (2). The slide mass is divided into vertical slices, and a typical slice DEFG is shown. The self-weight of the slice is dW hdx γ=. The method assumes that the resultant forces Xl and Xr on DE and FG , respectively, are equal and opposite, and parallel to the base of the slice EF . It is realized that these assumptions are necessary to keep theanalytical solution of the slope stability problem addressed in this paper achievable and some of these assumptions would lead to restrictions in terms of applications (e.g.earth pressure on retaining walls). However, analytical solutions have a special usefulness in engineering practice, particularly in terms of obtaining approximate solutions. More rigorous methods, e.g. finite element technique, can then be used to pursue a detail solution. Bishop's rigorous method5 introduces a furthernumerical procedure to permit specialcation of interslice shear forces Xl and Xr . Since Xl and Xr are internal forces, ()l r X X -∑ must be zero for the whole section. Resolving prerpendicularly and parallel to EF , one getssin cos T hdx k hdx γαγα=+(9)cos csin N hdx k hdx γαγα=-(10)22arcsin ,x a r a b rα-==+ (11)The force N can produce a maximum shearing resistance when failure occurs:sec (cos sin )tan R cdx hdx k αγααφ=+-(12)The equations of lines AC , CB , and AB Y are given by y22123tan ,,()y x y h y b r x a β===---(13)The sums of the disturbing and resisting moments for all slices can be written as013230(sin cos )()(sin cos )()(sin cos )()ls l lL s c M r h k dx r y y k dx r y y k dx r I kI γααγααγααγ=+=-++-+=+⎰⎰⎰ (14) []02300232sec (cos sin )tan sec ()(cos sin )tan ()(cos sin )tan tan ()lr l l lL c s M r c h k dx r c dx r y y k dx r y y k dx r c r I kI αγααφαγααφγααφϕγφ=+-=+--+--=+-⎰⎰⎰⎰ (15)22cot ,()L H l a r b H β==+-- (16)arcsinarcsin l a a r r ϕ-=+ (17) 1323022()sin ()sin 1(cot )sec 23Ll s L I y y dx y y dxH a b H rααββ=-+-⎡⎤=+-⎢⎥⎣⎦⎰⎰ (18) 13230222222222()cos ()cos tan tan 2()()()623(tan )arcsin (tan )arcsin 221()arcsin()4()()26L l s L I y y dx y y dxb r b r L a r L a r r r L a r a a H a b r r r l a b H r l ab l a H a r r ααββββ=-+-⎡⎤=-+---++⎣⎦-⎛⎫⎛⎫+-+- ⎪ ⎪⎝⎭⎝⎭-⎡⎤--+-+--⎣⎦⎰⎰ (19) The safety factor for this case is usually expressed as the ratio of the maximum available resisting moment to the disturbing moment, that istan ()()c s r s s s c c r I kI M F M I kI ϕγφγ+-==+ (20) When the slope inclination exceeds 543, all failures emerge at the toe of the slope, which is called t oe failure , as shown in Figure 2. However, when the slope height H is relatively large compared with the undrained shear strength or when a hard stratum is under the top of the slope of clayey soil with 03φ<, the slide emerges from the face of the slope, which is called Face failure , as shown in Figure 3. For Face failure , the safety factor F s is the same as ¹oe failure 1s using 0()Hh - instead of H .For flatter slopes, failure is deep-seated and extends to the hard stratum forming the base of the clay layer, which is called Base failure , as shown in Figure 4.1,3 Following the sameprocedure as that for ¹oe failure , one can get the safety factor for Base failure :()''''tan ()c s s s c c r I kI F I kI ϕγφγ+-=+ (21) where t is given by equation (17), and 's I and 'c I are given by()()()0100'0313230322201sin sin sin cot ()()(2)(33)12223l l l s l l I y y xdx y y xdx y y xdx H H bl H l l l l l a b bH H r r r β=-+-+-=+----+-+⎰⎰⎰ (22)()()()()()()[]22222203231030c 4612cot arcsin 2tan arcsin 21arcsin 2cot 412cos cos cos 1100a H a l ab l r r r H H a r r a rb r a H b r H r r Hl d y y d y y d y y I x l l x l l x l --+-+⎪⎭⎫ ⎝⎛⎪⎭⎫ ⎝⎛-+⎪⎭⎫ ⎝⎛-⎪⎭⎫ ⎝⎛----=⎰-+⎰-+⎰-='βββααα(23)其中,()221230,tan ,,y y x y H y b r x a β====---(24) ()220111cot ,cot ,22l a H l a H l a r b H ββ=-=+=+--(25)It can be observed from equations (21)~(25) that the factor of safety F s for a given slope is a function of the parameters a and b. Thus, the minimum value of F s can be found using the Powell's minimization technique.For a given single function f which depends on two independent variables, such as the problem under consideration here, minimization techniques are needed to find the value of these variables where f takes on a minimum value, and then to calculate the corresponding value of f. If one starts at a point P in an N-dimensional space, and proceed from there in some vector direction n, then any function of N variables f (P) can be minimized along the line n by one-dimensional methods. Different methods will difer only by how, at each stage, they choose the next direction n. Powell "rst discovered a direction set method which produces N mutually conjugate directions.Unfortunately, a problem of linear dependence was observed in Powell's algorithm. The modiffed Powell's method avoids a buildup of linear dependence.The closed-form slope stability equation (21) allows the application of an optimization technique to locate the center of the sliding circle (a, b). The minimum factor of safety Fs min then obtained by substituting the values of these parameters into equations (22)~(25) and the results into equation (21), for a base failure problem (Figure 4). While using the Powell's method, the key is to specify some initial values of a and b. Well-assumed initial values of a and b can result in a quick convergence. If the values of a and b are given inappropriately, it may result in a delayed convergence and certain values would not produce a convergent solution. Generally, a should be assumed within$¸, while b should be equal to or greater than H (Figure 4). Similarly, equations(16)~(20) could be used to compute the F s .min for toe failure (Figure 2) and face failure (Figure 3),except ()0H h - is usedinstead of H in the case of face failure .Besides the Powell method, other available minimization methods were also tried in this study such as downhill simplex method, conjugate gradient methods, and variable metric methods. These methods need more rigorous or closer initial values of a and b to the target values than the Powell method. A short computer program was developed using the Powell method to locate the center of the sliding circle (a , b ) and to find the minimum value of F s . This approach of slope stability analysis is straightforward and simple.RESULTS AND COMMENTSThe validity of the analytical method presented in the preceding sections was evaluated using two well-established methods of slope stability analysis. The local minimumfactor-of-safety (1993) method, with the state of the effective stresses in a slope determined by the finite element method with the Drucker-Prager non-linear stress-strain relationship, and Bishop's (1952) method were used to compare the overall factors of safety with respect to the slip surface determined by the proposed analytical method. Assuming k =0 for comparison with the results obtained from the local minimum factor-of-safety and Bishop's method, the results obtained from each of those three methods are listed in Table I.The cases are chosen from the toe failure in a hypothetical homogeneous dry soil slope having a unit weight of 18.5 kN/m3. Two slope configurations were analysed, one 1 : 1 slope and one 2 : 1 slope. Each slope height H was arbitrarily chosen as 8 m. To evaluate the sensitivity of strength parameters on slope stability, cohesion ranging from 5 to 30 kPa and friction angles ranging from 103 to 203 were used in the analyses (Table I). Anumber of critical combinations of c and were found to be unstable for the model slopes studied. The factors of safety obtained by the proposed method are in good agreement with those determined by the local minimum factor-of-safety and Bishop's methods, as shown in Table I.To examine the e!ect of dynamic forces, the analytical method is chosen to analyse a toe failure in a homogeneous clayey slope (Figure 2). The height of the slope H is 13.5 m; the slope inclination b is arctan 1/2; the unit weight of the soil c is 17.3 kN/m3; the friction angle is 17.3KN/m; and the cohesion c is 57.5 kPa. Using the conventional method of slices, Liu obtained theminimum safety factormin 2.09sF= Using the proposed method, one can get the minimum value of safety factor from equation (20) asmin 2.08sF= for k=0, which is very close to the value obtained from the slice method. When k"0)1, 0)15, or 0)2, one cangetmin 1.55,1.37sF=, and 1)23, respectively,which shows the dynamic e!ect on the slope stability to be significant.CONCLUDING REMARKSAn analytical method is presented for analysis of slope stability involving cohesive and noncohesive soils. Earthquake e!ects are considered in an approximate manner in terms of seismic coe$cient-dependent forces. Two kinds of failure surfaces are considered in this study: a planar failure surface, and a circular failure surface. Three failure conditions for circular failure surfacesnamely toe failure, face failure, and base failure are considered for clayey slopes resting on a hard stratum.The proposed method can be viewed as an extension of the method of slices, but it provides a more accurate treatment of the forces because they are represented in an integral form. The factor of safety is obtained by using theminimization technique rather than by a trial and error approach used commonly.The factors of safety obtained from the proposed method are in good agreement with those determined by the local minimum factor-of-safety method (finite element method-based approach), the Bishop method, and the method of slices. A comparison of these methods shows that the proposed analytical approach is more straightforward, less time-consuming, and simple to use. The analytical solutions presented here may be found useful for (a)validating results obtained from other approaches, (b) providinginitial estimates for slope stability, and (c) conducting parametric sensitivity analyses for various geometric and soil conditions.REFERENCES1. D. Brunsden and D. B. Prior. Slope Instability, Wiley, New York, 1984.2. B. F. Walker and R. Fell. Soil Slope Instability and Stabilization, Rotterdam, Sydney, 1987.3. C. Y. Liu. Soil Mechanics, China Railway Press, Beijing, P. R. China, 1990.448 SHORT COMMUNICATIONSCopyright ( 1999 John Wiley & Sons, Ltd. Int. J. Numer. Anal. Meth. Geomech., 23, 439}449 (1999)4. L. W. Abramson. Slope Stability and Stabilization Methods, Wiley, New York, 1996.5. A. W. Bishop. &The use of the slip circle in the stability analysis of slopes', Geotechnique, 5, 7}17 (1955).6. K. E. Petterson. &The early history of circular sliding surfaces', Geotechnique, 5, 275}296 (1956).7. G. Lefebvre, J. M. Duncan and E. L. Wilson.&Three-dimensional "nite element analysis of dams,' J. Soil Mech. Found,ASCE, 99(7), 495}507 (1973).8. Y. Kohgo and T. Yamashita, &Finite element analysis of "ll type dams*stability during construction by using the e!ective stress concept', Proc. Conf. Numer. Meth. in Geomech., ASCE, Vol. 98(7), 1998, pp. 653}665.9. J. M. Duncan. &State of the art: limit equilibrium and "nite-element analysis of slopes', J. Geotech. Engng. ASCE, 122(7), 577}596 (1996).10. V. U. Nguyen. &Determination of critical slope failuresurface', J. Geotech. Engng. ASCE, 111(2), 238}250 (1985).11. Z. Chen and C. Shao. &Evaluation of minimum factor of safety in slope stability analysis,' Can. Geotech. J., 20(1), 104}119 (1988).12. W. F. Chen and X. L. Liu. ¸imit Analysis in Soil Mechanics, Elsevier, New York, 1990.简要的分析斜坡稳定性的方法JINGGANG CAOs 和 MUSHARRAF M. ZAMAN诺曼底的俄克拉荷马大学土木环境工程学院摘要本文给出了解析法对边坡的稳定性分析,包括粘性和混凝土支撑。
应用地球化学元素丰度数据手册-原版
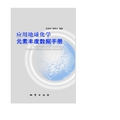
应用地球化学元素丰度数据手册迟清华鄢明才编著地质出版社·北京·1内容提要本书汇编了国内外不同研究者提出的火成岩、沉积岩、变质岩、土壤、水系沉积物、泛滥平原沉积物、浅海沉积物和大陆地壳的化学组成与元素丰度,同时列出了勘查地球化学和环境地球化学研究中常用的中国主要地球化学标准物质的标准值,所提供内容均为地球化学工作者所必须了解的各种重要地质介质的地球化学基础数据。
本书供从事地球化学、岩石学、勘查地球化学、生态环境与农业地球化学、地质样品分析测试、矿产勘查、基础地质等领域的研究者阅读,也可供地球科学其它领域的研究者使用。
图书在版编目(CIP)数据应用地球化学元素丰度数据手册/迟清华,鄢明才编著. -北京:地质出版社,2007.12ISBN 978-7-116-05536-0Ⅰ. 应… Ⅱ. ①迟…②鄢…Ⅲ. 地球化学丰度-化学元素-数据-手册Ⅳ. P595-62中国版本图书馆CIP数据核字(2007)第185917号责任编辑:王永奉陈军中责任校对:李玫出版发行:地质出版社社址邮编:北京市海淀区学院路31号,100083电话:(010)82324508(邮购部)网址:电子邮箱:zbs@传真:(010)82310759印刷:北京地大彩印厂开本:889mm×1194mm 1/16印张:10.25字数:260千字印数:1-3000册版次:2007年12月北京第1版•第1次印刷定价:28.00元书号:ISBN 978-7-116-05536-0(如对本书有建议或意见,敬请致电本社;如本社有印装问题,本社负责调换)2关于应用地球化学元素丰度数据手册(代序)地球化学元素丰度数据,即地壳五个圈内多种元素在各种介质、各种尺度内含量的统计数据。
它是应用地球化学研究解决资源与环境问题上重要的资料。
将这些数据资料汇编在一起将使研究人员节省不少查找文献的劳动与时间。
这本小册子就是按照这样的想法编汇的。
隧道英文-
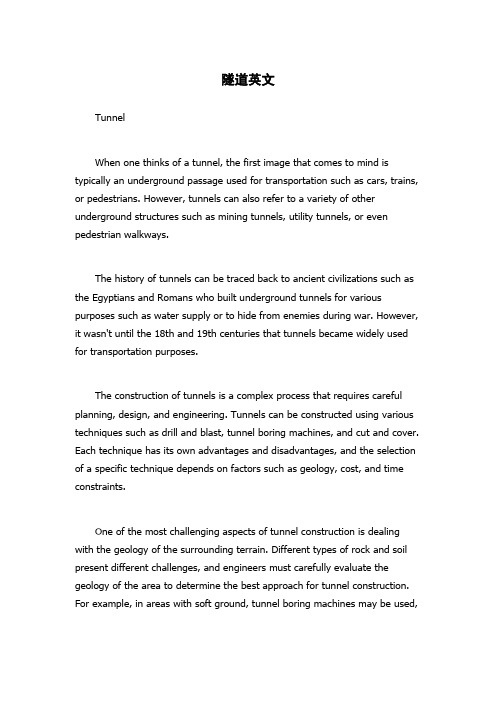
隧道英文TunnelWhen one thinks of a tunnel, the first image that comes to mind is typically an underground passage used for transportation such as cars, trains, or pedestrians. However, tunnels can also refer to a variety of other underground structures such as mining tunnels, utility tunnels, or even pedestrian walkways.The history of tunnels can be traced back to ancient civilizations such as the Egyptians and Romans who built underground tunnels for various purposes such as water supply or to hide from enemies during war. However, it wasn't until the 18th and 19th centuries that tunnels became widely used for transportation purposes.The construction of tunnels is a complex process that requires careful planning, design, and engineering. Tunnels can be constructed using various techniques such as drill and blast, tunnel boring machines, and cut and cover. Each technique has its own advantages and disadvantages, and the selection of a specific technique depends on factors such as geology, cost, and time constraints.One of the most challenging aspects of tunnel construction is dealing with the geology of the surrounding terrain. Different types of rock and soil present different challenges, and engineers must carefully evaluate the geology of the area to determine the best approach for tunnel construction. For example, in areas with soft ground, tunnel boring machines may be used,while in areas with hard rock, drill and blast methods may be more appropriate.Tunnels play a vital role in modern transportation infrastructure. They allow for faster and more efficient transportation of people and goods over long distances, and they can also help alleviate traffic congestion in urban areas. However, their construction can also have significant environmental impacts, such as disruption of natural habitats and water resources.In recent years, there has been increased interest in the construction of tunnels for alternative transportation options such as hyperloops and subways. These technologies promise even faster and more efficient transportation, and their development will likely lead to new innovations in tunnel construction techniques.In conclusion, tunnels are fascinating structures that have a long history and continue to be an important part of transportation infrastructure. While their construction can be challenging, the benefits they provide are numerous, and the future of tunnel technology is promising.。
超分子溶剂萃取

第42 卷第 5 期2023 年5 月Vol.42 No.5559~567分析测试学报FENXI CESHI XUEBAO(Journal of Instrumental Analysis)超分子溶剂萃取/超高效液相色谱-串联质谱法测定血浆中他克莫司含量谢以清1,2,吕悦广2,孟宪双2,雷海民1*,马强2*(1.北京中医药大学中药学院,北京102488;2.中国检验检疫科学研究院,北京100176)摘要:该文建立了血浆中免疫抑制剂他克莫司(TAC)的超分子溶剂(SUPRAS)萃取/超高效液相色谱-串联质谱分析方法。
通过单因素实验结合响应面设计对超分子溶剂组成、用量及涡旋萃取时间等关键因素进行优化后,血浆样本以正戊醇、四氢呋喃和水形成的超分子溶剂进行高效萃取。
萃取液经Waters ACQUITY UPLC BEH C18(50 mm × 2.1 mm,1.7 μm)色谱柱分离后,在电喷雾质谱正离子模式下,以多反应监测(MRM)模式对他克莫司进行测定,内标法定量。
结果表明,他克莫司在0.5 ~ 30 ng/mL质量浓度范围内的线性关系良好,相关系数(r)为0.998 6;方法检出限和定量下限分别为0.1、0.5 ng/mL;在低、中、高3个加标水平下,平均回收率(n = 3)为91.9% ~ 99.9%,相对标准偏差(RSD)为1.7% ~ 5.7%。
所建立的方法快速、灵敏、稳定,适用于血浆中他克莫司的准确测定。
关键词:他克莫司;免疫抑制剂;超分子溶剂;血浆;超高效液相色谱-串联质谱中图分类号:O657.7;R917文献标识码:A 文章编号:1004-4957(2023)05-0559-09Determination of Tacrolimus in Plasma by Supramolecular Solvent Extraction/Ultra-high Performance Liquid Chromatography-Tandem Mass SpectrometryXIE Yi-qing1,2,LÜ Yue-guang2,MENG Xian-shuang2,LEI Hai-min1*,MA Qiang2*(1.School of Chinese Materia Medica,Beijing University of Chinese Medicine,Beijing 102488,China;2.Chinese Academy of Inspection and Quarantine,Beijing 100176,China)Abstract:An analytical method for the determination of tacrolimus(TAC) in blood plasma was estab⁃lished by supramolecular solvent(SUPRAS)extraction combined with ultra-high performance liquid chromatography-tandem mass spectrometry.After optimizing the key factors such as the composition and amount of SUPRAS,and vortex extraction time through single factor experiment and response sur⁃face design,blood plasma samples were extracted efficiently with SUPRAS formed by pentanol,tetra⁃hydrofuran and water.The extract was separated on a Waters ACQUITY UPLC BEH C18column (50 mm × 2.1 mm,1.7 μm),analyzed by electrospray ionization mass spectrometry in positive ion mode under multiple reaction monitoring(MRM) mode,and quantified by internal standard method.Experimental results demonstrated that there was a good linear relationship for TAC in the concentration range of 0.5-30 ng/mL,with a correlation coefficient(r) of 0.998 6.The limit of detection(LOD)and quantitation(LOQ) were 0.1 ng/mL and 0.5 ng/mL,respectively.The average recoveries(n = 3)at low,medium and high spiked concentration levels ranged from 91.9% to 99.9%,with relative stan⁃dard deviations(RSDs) of 1.7%-5.7%.The proposed method is rapid,sensitive and stable,and it was suitable for the accurate determination of TAC in blood plasma.Key words:tacrolimus;immunosuppresive agent;supramolecular solvent;plasma;ultra-high performance liquid chromatography-tandem mass spectrometry免疫抑制剂是用于抑制机体免疫力的药物,多用于抑制肝肾移植术后的免疫反应,以及治疗变态反应性和自身免疫性疾病,如类风湿关节炎、红斑狼疮等[1-3]。
工程英汉汉英翻译术语库SDL

project manager quality control request(application) review risk river closure river diversion safety signature site site engineer specification staff Subcontractor submission supervise Supplier the International Chamber of Commerce unit price variation(change) World Bank Excavation and Support Open Excavation bench excavation concrete excavation fault excavation foundation excavation local excavation mass(bulk) excavation protective layer excavation rock excavation slope excavation soft ground excavation soil excavation structural excavation tooth excavation unclassified material excavation coefficient of nonuniformity cutoff trench dewatering(drainage) excavation pit
工程师 工程师代表 工程项目 国际工程 海外工程 国内工程 设备 外籍职员 专家 出口 土木工程承包商联合会 正式的 金结工程 进口 负责、主管 非正式的 土木工程师协会 仪器、器械 保险 劳务 布置 牵头公司、责任公司 责任、义务 总包 机械 人力资源 制造商 材料 办法、措施 采取有效措施 测量、计量 备忘录 进场 反对 支付 设备 观点、意见 资格预审 采购 利润 进度控制
抗生素生长促进剂对罗非鱼肠道菌群的效用

Arch Microbiol (2010) 192:985–994DOI 10.1007/s00203-010-0627-z123ORIGINAL PAPERE V ects of the antibiotic growth promoters X avomycin and X orfenicol on the autochthonous intestinal microbiota of hybrid tilapia (Oreochromis niloticus $£O. aureus #)Suxu He · Zhigang Zhou · Yuchun Liu · Yanan Cao · Kun Meng · Pengjun Shi · Bin Yao · Einar RingøReceived: 29 March 2010 / Revised: 24 August 2010 / Accepted: 1 September 2010 / Published online: 16 September 2010© Springer-Verlag 2010Abstract The 16S rDNA PCR-DGGE and rpo B quantita-tive PCR (RQ-PCR) techniques were used to evaluate the e V ects of dietary X avomycin and X orfenicol on the autoch-thonous intestinal microbiota of hybrid tilapia. The W sh were fed four diets: control, dietary X avomycin, X orfenicol and their combination. After 8weeks of feeding, 6 W sh from each cage were randomly chosen for the analysis. The total number of intestinal bacteria was determined by RQ-PCR. The results showed that dietary antibiotics signi W -cantly in X uenced the intestinal microbiota and dramatically reduced the intensity of total intestinal bacterial counts. The intensity of some phylotypes (EU563257, EU563262 and EU563255) were reduced to non-detectable levels by both dietary antibiotics, while supplementation of X orfenicol to the diet also reduced the intensity of the phylotypes EU563242 and EU563262, uncultured Mycobacterium sp.-like, uncultured Cyanobacterium -like and uncultured Cyanobacterium (EU563246). Dietary X avomycin only reduced the OTU intensity of one phylotype, identi W ed as amember of the phylum Fusobacteria . The antibiotic combi-nation only reduced the phylotypes EU563242 and EU563262. Based on our results, we conclude that the reduced e V ect of X orfenicol on intestinal microbiota was stronger than that of X avomycin, and when X avomycin and X orfenicol were added in combination, the e V ect of X orfeni-col overshadowed that of X avomycin.Keywords Hybrid tilapia · Dietary X avomycin · Florfenicol · RQ-PCR · DGGE · Intestinal bacteriaIntroductionAquaculture animals are colonized by trillions of microor-ganisms that have a symbiotic relationship with their host and are distributed in gill, body surface and gastrointestinal (GI) tract (Frenkiel and Mouëza 1995; Armstrong et al.2001; Izvekova et al. 2007). The majority of these microbes inhabits the GI tract and plays an important role in nutri-tional, physiological and pathological events (Denev et al.2009; Merri W eld et al. 2010; Nayak 2010). During the last decade, several studies have showed that the composition of W sh intestinal microbiota is highly variable and is a V ected by the developmental stages, diet and environmen-tal conditions (González et al. 1999; Ringø and Birkbeck 1999; Spanggaard et al. 2000; Huber et al. 2004).In China, antibiotics as growth promoters are widely used in aquafeeds, especially X avomycin and X orfenicol.Flavomycin is a glycolipid antibiotic produced by Strepto-myces species and inhibits peptidoglycan polymerases through impairment of the transglycolase activities of peni-cillin-binding proteins (Butaye et al. 2003). Flavomycin is known to change the equilibrium of gut microbiota and is active primarily against Gram-positive bacteria but also toCommunicated by Shuang-Jiang Liu.S. He · Z. Zhou (&) · Y. Liu · Y. Cao · K. Meng · P. Shi · B. Yao (&)Key Laboratory for Feed Biotechnology of the Ministry of Agriculture, Feed Research Institute, Chinese Academy of Agricultural Sciences, 100081 Beijing, People’s Republic of China e-mail: zhou_zg@ B. Yaoe-mail: yaobin@E. RingøNorwegian College of Fishery Science,Faculty of Biosciences, Fisheries and Economics, University of Tromsø, 9037 Tromsø, Norway抗生素土生的丆土著的986Arch Microbiol (2010) 192:985–994123some extent against certain Gram-negative bacteria, such as Pasteurella and Brucella (Huber and Nesemann 1968).Bacteria species such as Clostridium perfringens and many other clostridia species, several species of Enterococcus ,including E. gallinarum , E. casseli X avus , E. faecium ,E.mundtii and E. hirae , are reported to be of natural resis-tance to X avomycin (Devriese 1980; Dutta and Devriese 1980, 1982; Butaye et al. 1998, 2000a , b , 2001). Another important antibiotic is X orfenicol—a broad-spectrum bacte-riostatic antibiotic binding the 50S ribosomal subunit of susceptible pathogens (Plumb 2004). This antibiotic is reported to be e V ective against important W sh pathogens such as Yersinia ruckeri , Flavobacterium psychrophilum and Aeromonas salmonicida (Fukui et al. 1987; Samuelsen et al. 1998; Bruun et al. 2000), and Pasteurella multocida ,Mannheimia haemolytica , Actinobacillus pleuropneumo-niae and Streptococcus suis in vitro (Priebe and Schwarz 2003). However, the in X uence of antibiotics on intestinal microbiota has been determined by traditional culture-based method (Samuelsen et al. 1998; Bruun et al. 2000;Butaye et al. 2001). A large percentage of the intestinal microbiota cannot be cultured, resulting in limited under-standing of the impact of antibiotics on the autochthonous intestinal microbiota. Consequently, the objective of the present study was to obtain better knowledge and under-standing of the intestinal bacterial community of hybrid tilapia (Oreochromis niloticus $£O. aureus #) reared in cages and how supplementation of dietary X orfenicol and X avomycin, either singly or in combination, impacts the autochthonous intestinal microbiota.Materials and methods Experimental dietsBasal diets containing 26.0% protein and 3.0% lipid (Table 1) were formulated according to Li (2001). In the present study, four di V erent diets were used: basal diet (CK), diet supplemented with 20mg X orfenicol kg ¡1, diet supplemented with 20mg X avomycin kg ¡1 and a diet sup-plemented with 10mg X orfenicol kg ¡1 and 10mg X avomy-cin kg ¡1.Feeding trialThe culture experiment was conducted in a 4,000-m 2earthen pond at a local aquaculture farm, Jiaxing, Zhejiang,China. Juvenile hybrid tilapia (Oreochromis niloticus $£O. aureus #) was acclimated in a X oating net cage (4.0m £2.0m £1.5m) for 2weeks. Water depth of the pond was approximately 1.5m. After 2days of starvation,uniform W sh (50.89§0.27g) were randomly distributedinto 12 X oating net cages (1.1m £1.1m £1.1m). Each dietary group was fed in triplicate cages, and each cage contained 20 W sh. The W sh were hand-fed 3% of initial body weight three times a day (08:00, 11:30 and 17:30), and the feed ration was adjusted weekly to insure tilapias in each cage consume the diet pellets within 1h. Each cage was individually aerated, and one tenth of the experimental pond water was exchanged by fully aerated tap water each week. During the feeding period, rearing temperature was 27.0§3.0°C, while dissolved oxygen (DO)>5.0mg oxy-gen l ¡1, pH 7.8, NH 4+-N <0.50mg nitrogen l ¡1 and NO 2-N <0.05mg nitrogen l ¡1. The photoperiod was W xed at a natural condition from 5:00 to 19:00.Sampling of the autochthonous gut microbiotaSix W sh from each cage were randomly collected after 8-week feeding for gut bacterial analysis. Sampling of the autochthonous microbiota from the whole intestine was carried out after two days of starvation as previously described (Zhou et al. 2007). Brie X y, the digestive tracts were aseptically removed in their entirety, slit open with a sterile scalpel, and the contents and non-adherent bacteria were rinsed three times in phosphate-bu V ered saline (PBS; 0.1M, pH 7.2). The surface of each intestine wasTable 1Ingredients and chemical compositions of the experimental diets (%)aBasal diet: Cotton seed meal, Shandong, P.R. China (CP 40.0%),15.0; Rapeseed meal, Henan, P.R. China (CP 38.0%), 23.0; Single cell protein, Zhejiang, P.R. China (CP 73.8%), 1.0; Intestine casing meal,Zhejiang, P.R. China (CP 55.6%), 1.0; Malt sprouts, Zhejiang, P.R.China (CP 26.3%), 6.0; Wheat middings, Zhejiang, P.R. China (CP 16.7%), 13.0; Wheat X our, Shandong, P.R. China (CP 12.7%), 10.0;DDGS, Shandong, P.R. China (CP 26.6%), 6.0; Corn, Shandong, P.R.China (CP 9.5%), 3.0; Bentonite, Zhejiang, P.R. China, 6.0; Rice bran,Jiangsu, P.R. China (CP 14.2%), 12.0; Betaine, Shandong, P.R. China,0.1; Phospholipid oil, Jiangsu, P.R. China, 1.4; Calcium phosphate,Jiangsu, P.R. China, 1.6; Vc phosphate, Beijing, P.R. China, 0.02;Choline chloride, Shandong, P.R. China, 0.1; Antioxidantr, Shanghai,P.R. China, 0.03; Antimouldr, Shanghai, P.R. China, 0.1bSupplied by Zhejiang Yiwu Huatai Feed Company cSee the reference (Zhou et al. 2007)Ingredients CKFlavomycin Florfenicol Combinationof antibioticsBasal diet a 99.499.499.499.4Florfenicolb 0.00.00.0020.001Flavomycinb0.00.0020.00.001Mineral/vitamin mix c 0.60.60.60.6Chemical composition Crude protein 26.026.026.026.0Crude lipid 3.0 3.0 3.0 3.0Moisture 9.49.610.29.9Ash7.47.87.37.6Arch Microbiol (2010) 192:985–994987123homogenized using a glass homogenizer as described else-where (LeaMaster et al. 1997) and stored in 2-ml Eppen-dorf tubes at ¡20°C until analysis (Zhou et al. 2009a ).DGGE analysisPooled gut samples from six W sh in each cage (»200mg)were used to avoid erroneous conclusions due to individual variation in gut microbiota as described by Ringø et al.(1995), Spanggaard et al. (2000) and He et al. (2009). The total genomic DNA from the pooled gut samples were extracted using cetyltrimethylammonium bromide (CTAB;Gri Y ths et al. 2000) and lysozyme methods (Miller et al.1999) with some modi W cations. Brie X y, the gut samples were mixed with 500 l 5mg ml ¡1 lysozyme solution.After incubation at 37°C for 2h, 50 l 10mg ml ¡1 protein-ase K (Sigma, St. Louis, MO, USA) was added and mixed gently, followed by incubation at 55°C for 20min. Then 500 l CTAB lysis bu V er (100mM Tris–HCl, 100mM Na-EDTA, 1.5M NaCl, 1% CTAB, 2% SDS, pH 8.0) was added and incubated at 65°C for 2h. The DNA was recov-ered by precipitation with isopropanol and puri W ed as described by Liu et al. (2008). The V3 region of the 16S rRNA gene was ampli W ed with primers 338f (5Ј-ACTC CTACGGGAGGCAGCAG-3Ј) with a 40 base GC clamp at the 5Ј end and 518r (5Ј-ATTACCGCGGCTGCTGG-3Ј).The 50- l PCR reaction system contained 1£PCR bu V er (20mM Tris–HCl (pH 8.4) and 50mM KCl), 200 M dNTP, 500 nM each primer, 1.75mM MgCl 2, 670ng l ¡1bovine serum albumin, 1.25 U Platinum ® Taq DNA poly-merase (Invitrogen, USA) and 2 l puri W ed DNA. The PCR conditions were as follows: 5min of initial denaturation at 94°C, followed by 28 cycles of 30s of denaturation at 94°C, 30-s annealing at 65°C (decreasing 1°C per cycle until 56°C), and 30-s extension at 72°C and a W nal exten-sion at 72°C for 10min. PCR products were examined by 2% agarose gel electrophoresis. DGGE was performed with a D-Code universal mutation system (Bio-Rad, Her-cules, CA, USA). PCR products (»800ng) were loaded onto polyacrylamide gels in 0.5£TAE bu V er, with a gra-dient of 40–60% denaturant. Electrophoresis was per-formed at 60°C, 65V for 16h. After electrophoresis, gels were stained for 20min in distilled water containing ethi-dium bromide (0.5 g ml ¡1) and visualized under UV light. DGGE bands were excised from the gels, resus-pended in 100 l distilled water and kept at 4°C overnight.The supernatant was used as the template for a second round of PCR under the same conditions. The PCR prod-ucts were ligated into pGEM-T Easy vector (Promega,Madison, WI, USA) for sequencing (Invitrogen, Shanghai,China). Representative sequences were deposited in the NCBI database under accession numbers EU563242–EU563265.Total intestinal bacteria analysisEnumeration of total bacteria was conducted by real-time PCR according to Takahashi et al. (2006) and Silkie and Nelson (2009) with some modi W cations. Brie X y, several cultured bacteria were selected based on the predominant microbiota present in DGGE with an abundance index greater than 5%. In this study, Clostridium thermocellum B108 (Gram-positive bacteria) and Sphingomona s sp. B222(Gram-negative bacteria) were chosen as standards. Both bacterial species were cultured overnight in Luria–Bertani (LB) medium, and the total number of bacteria was counted using a hemocytometer. Thereafter, each bacterial strain was mixed equally at 0.5£108 cells ml ¡1, and total geno-mic DNA was extracted from 1ml of the combined mixture using CTAB (Gri Y ths et al. 2000) and lysozyme methods (Miller et al. 1999). To increase the concentration of puri-W ed intestinal DNA, the genomic DNA was pre-cooled at ¡70°C for »2h, freeze-dried (CHRIST, Osterode, Ger-many) overnight and solved in TE bu V er to the ideal con-centration of 50ng l ¡1.Serial dilutions of standards at 103, 104, 105 and 106CFU per template reaction were prepared for calibration.The RNA polymerase -subunit gene (rpo B; one copy in bacteria) was ampli W ed using the primers rpo B1698f (5Ј-A ACATCGGTTTGATCAAC-3Ј) and rpo B2041r (5Ј-CGT TGCATGTTGGTACCCAT-3Ј; Dahllöf et al. 2000). The reaction mixture (20 l) was prepared according to the manufacturer’s protocol: 7 l PCR-grade water, 1 l each primer (5 mol l ¡1), 10 l 2£real-time PCR master mix (SYBR Green; TOYOBO, Shanghai, China) and 1 l DNA template (50ng l ¡1). The PCR conditions consisted of ini-tial denaturation at 95°C for 5min, 40 cycles of denatur-ation at 95°C for 30s, annealing at 55°C for 30s and extension at 72°C for 30s, with a W nal extension step at 72°C for 5min.The concentration of each standard (CFU ml ¡1) was inputted into the LightCycler 2.0 software using the thresh-old cycle value (C T ) to construct a standard for absolute quanti W cation analysis. The number of bacteria present in unknown samples (12 samples) was calculated based on the standard curve. Each sample was analyzed in at least four replicates.Statistical analysisThe gel images were analyzed using the public domain NIH Image program to calculate relative abundance (RA, %;Simpson et al. 1999). Cluster analysis was performed based on the unweighted pair group method using the arithmetic mean algorithm (UPGMA) by the program NTSYS. In this study, pairwise similarity coe Y cient (C s) less than 0.60 is regarded as signi W cant di V erence; while 0.60·C s <0.85is988Arch Microbiol (2010) 192:985–994123marginal di V erence, and C s ¸0.85 is very similar accord-ing to Sun et al. (2004). The Shannon index of bacterial diversity, H , was calculated as Shannon and Weaver (1963)described.Results are presented as mean §SD. Data were subjected to one-way ANOVA to test the e V ect of dietary treatment. When signi W cant di V erences were detected (P <0.05), Duncan’s multiple range test was used to com-pare mean values among dietary treatments. All statistical analysis was carried out using the statistic software SPSS version 10.0.ResultsDGGE pro W les of intestinal microbiota in tilapiaBacterial DGGE pro W les of four di V erent treatments showed signi W cant di V erences (Fig.1, Tables 2, 4), and thedi V erence of the interior-group was more signi W cant than that of inter-group. There were 18.67§0.47, 15.00§0.82,14.33§0.47 and 14.66§0.47 OTUs in CK, X avomycin,X orfenicol and antibiotic combination groups, respectively (Table 2). The pairwise similarity coe Y cients (C s) matrix for the intestinal bacterial community of hybrid tilapia based on the DGGE W ngerprints is shown in Table 3. The bacterial community in X avomycin group was similar to that of CK with a C s value of 0.84. The bacterial commu-nity of CK was marginally di V erent to that of X orfenicol and the combination of X avomycin and X orfenicol.Identi W cation of dominant DGGE bandsA total of 19 representative OTUs were retrieved from the bacterial DGGE pro W les (Table 4). Proteobacteria (2 OTUs),Actinobacteria (2 OTUs), Cyanobacteria (3 OTUs), Fuso-bacterium (1 OTU) and Firmicutes (1 OTU) were the predominant autochthonous bacteria in hybrid tilapia intestine. The relative abundance (RA) results showed that OTU 3 (uncultured bacterium, EU418508), 7 (uncultured bacterium, EF532770), 8 (uncultured Cyanobacterium ,EF630240), 10 (uncultured prokaryote, AJ867878), 11(uncultured bacterium clone, DQ675149), 13 (Sphingo-monas sp., EU442226) and 18 (uncultured -Proteobacte-rium , EF697165) were not a V ected by dietary antibiotics. In contrast to these results, the intensities of OTU 4 (uncul-tured bacterium, AB206034), 12 (Streptomyces sp.,EU159565) and 19 (uncultured prokaryote, AJ867878) were reduced to non-detectable levels by dietary X avomycin and X orfenicol. One interesting observation was that OTU 17,an uncultured bacterium with 100% similarity to accession no. EF599665, was reduced to non-detectable levels by dietary X orfenicol and the antibiotic combination, but no di V erence in RA was observed between the control group (CK) and W sh that received X avomycin. Compared with CK, supplementation of dietary X orfenicol decreased the intensities of OTU 1 (uncultured bacterium, AJ504589), 2(uncultured Cyanobacterium , DQ158167), 5 (uncultured Cyanobacterium, EU751409) and 14 (uncultured Mycobac-terium sp., EF438322), but the intensities of OTU 9 (uncul-tured bacterium, EF669487) and 16 (uncultured bacterium,Fig.1DGGE pro W le generated from the V3-16S rDNA fragments of the bacteria from the intestinal wall of hybrid tilapia O. niloticus $£O. aureus #. CK1–3 represent samples taken from the intestine of tilapia fed control diet without antibiotic supplement; Fm1–3 are sam-ples from the intestine of tilapia fed diet supplemented with X avomy-cin; Fn1–3 are samples from the intestine of tilapia fed diet supplemented with X orfenicol; and FF1–3 are samples from the intes-tine of tilapia fed diet supplemented with X avomycin and X orfenicolTable 2E V ect of di V erent feeding regimes on the intestinal microbiota of hybrid tilapia O. niloticus $£O. aureus #Data (mean §SD) in the same row sharing a common superscript are not signi W cantly di V erent (Duncan’s multiple range test, P >0.05)FeedingCKFlavomycin Florfenicol Combination of antibiotics P value Bacterial counts(£107 CFU g ¡1 dry matter) 1.11§0.10a 0.47§0.05b 0.2§0.01c 0.43§0.08b <0.001OTUs 18.67§0.47a 15.00§0.82b 14.33§0.47b 14.66§0.47b <0.001H 2.64§0.02a 2.45§0.02b 2.36§0.01c 2.48§0.02b <0.001E H0.73§0.01a0.73§0.02a0.70§0.01a0.79§0.02b0.002Arch Microbiol (2010) 192:985–994989123AJ548786) were elevated. An interesting W nding was that the intensity of OTU 15 on the DGGE, identi W ed as a mem-ber of the phylum Fusobacteria , was reduced only by die-tary X avomycin. The combination of the two antibiotics signi W cantly decreased OTU 1 as a RA value of 1.37§0.12 was observed in the combined antibiotics group compared to the RA value of 2.60§0.24 in CK.Total intestinal bacterial countsThe total intestinal bacterial counts analyzed by RQ-PCR varied from 0.20§0.01 to 1.11§0.10CFU £107 g ¡1 as shown in Table 2. Supplementation of dietary X orfenicol and X avomycin signi W cantly reduced (P <0.05) the intesti-nal bacteria. Shannon diversity indexes (H )of the antibiot-ics treatments were signi W cantly lower (P <0.05) than that of CK. The value of H for X orfenicol group was higher than that of X avomycin and antibiotic combination (P <0.05),but the Shannon equitability indexes (E H ) were not a V ected by dietary antibiotics compared to CK (P >0.05).DiscussionIn the present study, the autochthonous intestinal microbi-ota was signi W cantly modulated by X avomycin. This result is consistent with previous reports that X avomycin reduced the incidence of the animal pathogens Salmonella and Clostridium in pre-slaughter broilers (Bolder et al. 1999)and modulated the ruminal gut microbiota (Edwards et al.2005). In the study of broiler chicks, Gunal et al. (2006)demonstrated that the counts of total bacteria and Gram-negative bacteria were signi W cantly decreased by X avomy-cin after 21 and 42days of feeding. Zhou et al. (2009b )observed that dietary X avomycin a V ected the autochtho-nous intestinal bacterial community in tilapia. In the present study, several intestinal phylotypes such as Streptomyces sp.-like bacterium, uncultured bacterium (EU563262),uncultured prokaryote-like bacterium and Fusobacteria bacterium (EU563264) were reduced by X avomycin. Fuso-bacterium species are Gram-negative bacteria (Garcia et al.1992) and have been reported in bovine rumen, pig, poultry and W sh (GU301238; Tan et al. 1996; Anderson et al. 2000;Edwards et al. 2005). F. necrophorum is generally regarded as an opportunistic pathogen (Brazier et al. 2002) but hasbeen reported to be inhibited by X avomycin in a study of sheep (Edwards et al. 2005). More recently, Jeong et al.(2009) showed that Fusobacterium spp. in human gut were susceptible to X avomycin. Previous studies reported that Fusobacterium spp. have a very high rate of deamination by converting excessive dietary amino acids to ammonia (Russell et al. 1991; Attwood et al. 1998), and suppression of Fusobacterium by X avomycin has been reported to have a favorable e V ect on nitrogen metabolism (Edwards et al.2005).The present study showed that supplementation of dietary X orfenicol reduced a number of autochthonous intestinal bacteria in tilapia compared to X avomycin. For example, the intensity of Streptomyces sp.-like bacterium,uncultured bacteria (EU563262 and EU563265) and uncul-tured prokaryote-like bacterium were reduced to non-detectable levels by dietary X orfenicol. On the other hand,some gut bacteria including uncultured Mycobacterium sp.-like bacterium, uncultured Cyanobacterium -like bacte-rium, uncultured Cyanobacterium (EU563246) and uncultured bacterium (EU563242) were partly decreased. Mycobacte-ria are obligate aerobic, acid-fast, Gram-positive, non-spore forming, non-motile and prevalent in soil and water (Frerichs 1993). In two recent studies (He et al. 2009; Zhou et al. 2009a ) using DGGE, Mycobacterium sp.-like bacteria were detected in the tilapia intestine. In the present study,X orfenicol a V ected the RA value of Mycobacterium sp.-like bacteria from 1.40§0.16 to 0.93§0.21; however, dietary X avomycin had no e V ect.Three species of Cyanobacteria were detected in the tila-pia intestine (Table 4). Cyanobacteria possess the capabil-ity to store abundant nutrients, and some species can convert gaseous nitrogen to ammonia via nitrogen W xation (Stewart 1967). Cyanobacteria are well known for their ability to produce a large number of diverse secondary metabolites (Vining 1992), which cause mortality, initiate or promote tumors or deteriorate the health of several culti-vated species (nile tilapia, cat W sh, white shrimp and rain-bow trout; Smith et al. 2008). Previous investigations have reported Cyanobacteria in the intestine of W lter-feeding W sh such as Atlantic menhaden (Brevoortia tyrannus ), silver carp (Hypophthalmichtyhs molitrix ) or tilapia (Oreochr-omis niloticus $£O. aureus #; Friedland et al. 2005;Kolmakov et al. 2006; He et al. 2009). In the present study,two species of Cyanobacteria were reduced by X orfenicol.Table 3Pairwise similarity coe Y cients (C s) matrix for the intestinal microbiota of hybrid tilapia O. niloticus $£O. aureus #CKFlavomycin FlorfenicolCombination of antibioticsCK 1.00Flavomycin 0.84MS 1.00Florfenicol0.79MS 0.95NS 1.00Combination of antibiotics0.79MS0.95NS1.00NS1.00NS very similar, MS marginal di V erence990Arch Microbiol (2010) 192:985–994123T a b l e 4R e p r e s e n t a t i v e s o f O T U s o r c l o n e s i s o l a t e d f r o m t h e i n t e s t i n e o f h y b r i d t i l a p i a u n d e r t h e e x p e r i m e n t a l f e e d i n g r e g i m e s a n d t h e i r r e l a t i v e a b u n d a n c eP h y l o g e n e t i c g r o u pB a n dA c c e s s i o n n o .R e l a t i v e a b u n d a n c e (R A , %)P v a l u e C l o s e s t r e l a t i v e (o b t a i n e d f r o m B L A S T s e a r c h )I d e n t i t y (%)I s o l a t e d f r o mC KF l a v o m y c i n F l o r f e n i c o l C o m b i n a t i o n o f a n t i b i o t i c s P r o t e o b a c t e r i a 13E U 5632632.77§0.653.07§0.344.00§0.433.73§0.820.213S p h i n g o m o n a s s p . (E U 442226)100D e e p t e r r e s t r i a l s u b s u r f a c e (B r o w n , u n p u b l i s h e d d a t a , N C B I )18E U 56326010.13§1.067.90§0.2910.43§1.349.27§0.420.082U n c u l t u r e d -p r o t e o b a c t e r i u m (E F 697165)99H u m a n g a s t r o i n t e s t i n a l r e s e c t i o n s p e c i m e n (F r a n k e t a l . 2007)A c t i n o b a c t e r i a 11E U 5632561.70§0.162.03§0.382.07§0.171.87§0.250.492U n c u l t u r e d a c t i n o b a c t e r i u m (D Q 675149)99L i m n o l o g y o f S t r a t i W e d L a k e s (A l l g a i e r a n d G r o s s a r t , u n p u b l i s h e d d a t a , N C B I )12E U 5632571.20§0.24a –b –b –b<0.001S t r e p t o m y c e s s p . 926 (E U 159565)96A c i d i c s o i l i n Y u n n a n , C h i n a (X u e t a l . u n p u b l i s h e d d a t a , N CB I )14E U 5632581.40§0.16a 1.57§0.21a 0.93§0.21b 1.53§0.21a0.04U n c u l t u r e d M y c o b a c t e r i u m s p . (E F 438322)98C o a l t a r c o n t a m i n a t e d s e d i m e n t (D e B r u y n e t a l . 2007)C y a n o b a c t e r i a 2E U 5632433.13§0.29a 3.73§0.49a 1.87§0.25b 3.40§0.45a<0.001U n c u l t u r e d c y a n o b a c t e r i u m (D Q 158167)98F r e s h w a t e r l a k e , G e r m a n y (C o r r e d o r e t a l . u n p u b l i s h e d d a t a , N C B I )5E U 5632460.93§0.05a 1.43§0.17a 0.57§0.05b 1.13§0.26a0.004U n c u l t u r e d c y a n o b a c t e r i u m (E U 751409)100S a n d s t o n e f o r m a t i o n s (K u r t z e t a l . u n p u b l i s h e d d a t a , N C B I )8E U 56325110.23§0.429.13§1.2711.77§1.2010.10§1.530.243U n c u l t u r e d c y a n o b a c t e r i u m (E F 630240)97S e a w a t e r (M o h a m e d e t a l . 2008)F u s o b a c t e r i u m 15E U 5632643.50§0.75a 1.97§0.39b 2.50§0.22a b 3.37§0.46a0.042F u s o b a c t e r i a b a c t e r i u m (D Q 837051)100H u m a n f e c e s (F i n e g o l d e t a l . 2003)F i r m i c u t e s 6E U 5632488.80§0.94b 11.63§1.05a 6.43§0.61c 9.30§0.51b0.002U n c u l t u r e d C l o s t r i d i u m s p . (D Q 168144)100E v e r g l a d e s w e t l a n d s (U z a n d O g r a m 2006)U n c l a s s i W e d b a c t e r i a1E U 5632422.60§0.24a 2.50§0.16a 1.60§0.14b1.37§0.12b <0.001U n c u l t u r e d b a c t e r i u m (A J 504589)99A c t i v a t e d s l u d g e (B r o w n a n d T u r n e r , u n p u b l i s h e d d a t a , N C B I )3E U 5632451.00§0.161.20§0.291.23§0.171.13§0.210.717U n c u l t u r e d b a c t e r i u m (E U 418508)100I n t e s t i n a l m i c r o X o r a o f C t e n o p h a r y n g o d o n i d e l l u s (H u a n g e t a l . u n p u b l i s h e d d a t a , N C B I )4E U 5632620.27§0.05a –b–b –b <0.001U n c u l t u r e d b a c t e r i u m (A B 206034)94A c t i v a t e d s l u d g e (O s a k a e t a l . 2006)。
gsas 精修

Talk Emphasis
• Solving relevant problems in the various sciences such as the geosciences may require an intimate and non-routine knowledge of possible analytical techniques and their use • You may have to obtain more information that you initially wante to know. • In this case:
Slide 7
Using diffraction methods to solve the problems of the world Lachlan M. D. Cranswick (l.m.d.cranswick@)
Applications of Le Bail fitting
Slide 9 Using diffraction methods to solve the problems of the world Lachlan M. D. Cranswick (l.m.d.cranswick@)
Invention of Le Bail fitting and Le Bail Extraction
"Beyond Classical Rietveld Analysis - using Le Bail fitting of X-ray Powder Diffraction data to help answer the questions of the world:
can the Earth's outer core contain Oxygen?"
- 1、下载文档前请自行甄别文档内容的完整性,平台不提供额外的编辑、内容补充、找答案等附加服务。
- 2、"仅部分预览"的文档,不可在线预览部分如存在完整性等问题,可反馈申请退款(可完整预览的文档不适用该条件!)。
- 3、如文档侵犯您的权益,请联系客服反馈,我们会尽快为您处理(人工客服工作时间:9:00-18:30)。
Bull Earthquake Eng(2010)8:283–307DOI10.1007/s10518-009-9135-4ORIGINAL RESEARCH PAPERAnalysis of cut-and-cover tunnels against large tectonic deformationIoannis Anastasopoulos·George GazetasReceived:11January2009/Accepted:9April2009/Published online:18June2009©Springer Science+Business Media B.V.2009Abstract Tunnels are believed to be rather“insensitive”to earthquakes.Although a number of case histories seem to favor such an argument,failures and collapses of under-ground structures in the earthquakes of Kobe(1995),Düzce–Bolu(1999),and Taiwan(1999) have shown that there are exceptions to this“rule”.Among them:the case of tunnels crossed by fault rupture.This paper presents the analysis and design of two highway cut-and-cover tunnels in Greece against large tectonic dislocation from a normal fault.The analysis, conducted withfinite elements,places particular emphasis on realistically modeling the tunnel-soil interface.Soil behavior is modeled thorough an elastoplastic constitutive model with isotropic strain softening,which has been extensively validated through successful pre-dictions of centrifuge model tests.A primary conclusion emerging from the paper is that the design of cut-and-cover structures against large tectonic deformation is quite feasible. It is shown that the rupture path is strongly affected by the presence of the tunnel,lead-ing to development of beneficial stress-relieving phenomena such as diversion,bifurcation, and diffusion.The tunnel may be subjected either to hogging deformation when the rupture emerges close to its hanging-wall edge,or to sagging deformation when the rupture is near its footwall edge.Paradoxically,the maximum stressing is not always attained with the max-imum imposed dislocation.Therefore,the design should be performed on the basis of design envelopes of the internal forces,with respect to the location of the fault rupture and the mag-nitude of dislocation.Although this study was prompted by the needs of a specific project, the method of analysis,the design concepts,and many of the conclusions are sufficiently general to merit wider application.Keywords Fault rupture·Seismic design·Soil-structure interaction·Cut-and-cover tunnel·Finite element·Constitutive relations·Calibration through experimental dataI.Anastasopoulos·G.Gazetas(B)School of Civil Engineering,National Technical University,Athens,Greecee-mail:gazetas@ath.forthnet.grI.Anastasopoulose-mail:ianast@civil.ntua.gr1231IntroductionTunnels and underground structures have long been considered to suffer little damage in earthquakes and to be safer than above-ground structures(Dowding and Rozen1978).Sev-eral case histories reported in the literature are in favor of such an argument.For example:(a) the Bay Area Rapid Transit(BART)immersed tunnel survived the1989Loma Prieta M s7.1 earthquake with practically no damage(PB1991);(b)the Osaka South Port immersed tun-nel in Japan also behaved exceptionally during the1995M JMA7.2Kobe earthquake;(c)a variety of Athens Metro(bored)tunnels and cut-and-cover stations sustained absolutely no damage in the1999M s5.9Athens(Greece)earthquake(Gazetas et al.2005).However,it should be mentioned that all three examples refer to structures that had been indeed designed against seismic loading.The BART tunnel,although built in the1960s,was one of thefirst underground structures designed for earthquake loading and equipped with special3-D joints (Kuesel1969;Douglas and Warshaw1971;Bickel and Tanner1982).The Osaka immersed tunnel,as well as all Athens Metro tunnels,built in the1990s,had also been designed against earthquake loading according to the latest standards of that time.Moreover,the above tunnels were subjected to substantially smaller seismic intensity levels than their seismic design.On the other hand,a number of case histories of tunnel damage,or even collapse,are also readily available in the literature.While a systematic review of damage to rock tunnels due to earthquake shaking can be found in Dowding and Rozen(1978),we briefly mention some characteristic examples from recent earthquakes.In the1995,M JMA7.2Kobe earthquake, damage to cut-and-cover box-section reinforced concrete tunnels was quite severe.While the most spectacular failure was the collapse of the Daikai Metro station(Iida et al.1996; Nakamura et al.1996),the Yamate line was also heavily damaged(Konagai et al.2001). Several mountain tunnels in central Taiwan sustained severe damage in the1999M w7.6 Chi-Chi earthquake.A systematic investigation by Wang et al.(2001)showed that49tunnels of57(in total)sustained damage from the earthquake.While most of the severely damaged tunnels were on the hanging wall,damage due to dislocation of the seismogenic Chelungpu fault was also reported.The collapse of a400m long section of the under construction18m diameter Bolu tunnel in the Düzce(1999)earthquake in Turkey,was arguably affected by the Kaynasli (Düzce–Bolu)fault rupture,although the intensity of shaking has also played a substantial role(O’Rourke et al.2001).Several earlier case histories of tunnel damage due to faulting can be found in the litera-ture.One of the earliest such case histories is the failure of the Wrights rail tunnel in the1906 M W7.7San Francisco earthquake.Built in the1870s,the tunnel was subjected to an offset of about1.8m of the strike-slip San Andreas fault(Prentice and Ponti1997).Tunnel damage was in the form of cave-ins at the location of fault crossing,accompanied by deformation and cracking over a length of400m.It was put back to service about a year later and abandoned in the1940s.A second well-documented case-history refers to the damage of the Inatori906m long tunnel in the1978M JMA7.0Izu-Oshima-Kinkai(Japan)earthquake:traversed by a strike-slip fault rupture with an offset of about1m,it was deformed substantially,requiring substantial repairs(Kawakami1984).Admittedly,the relative probability of a structure being damaged from tectonic dislocation is substantially lower compared to shaking.The latter,being the result of waves emitted from the seismogenic fault(due to its“slippage”)and propagating over large distances in the earth, affects the ground surface at a much larger area compared to the permanent offset of the fault; the latter is of importance only when the rupture extends to the surface(or near the surface). Even in such a case,the affected area will be an order of magnitude smaller compared to 123shaking:a narrow belt along the outcropped rupture,compared to an elliptical“circle”of diameter larger than the length of the fault.Therefore,it is understandable why earthquake engineering research and practice focused in understanding the response of soil-structure systems to ground oscillations,and developing suitable analysis and design methods.Much less effort has been devoted to the response of structures to imposed tectonic dislocation. This lack of insight almost unavoidably lead to over-conservatism:earlier seismic codes had in the past invariably demanded that buildings and important structures not be erected in the immediate vicinity of active faults.Especially in the case of long structures,such as tunnels and bridges,which cannot eas-ily avoid crossing active fault zones,such a strict prohibition is not only difficult to obey and unduly conservative,but sometimes even meaningless.Because,(a)field evidence from the recent earthquakes in Turkey(Kocaeli and Düzce)and Taiwan(Chi-Chi)has shown that several structures survived large tectonic dislocations almost unscathed(Youd et al. 2000;Erdik2001;Bray2001;Ural2001;Ulusay et al.2002;Pamuk et al.2005);(b)earlier studies had also suggested that structures can be designed to withstand large tectonic dis-placements(Duncan and Lefebvre1973;Niccum et al.1976;Youd1989;Berill1983);and (c)recent research efforts,combiningfield studies,centrifuge model testing,and numerical modeling(Anastasopoulos and Gazetas2007a,b;Bransby et al.2008a,b;Faccioli et al.2008; Anastasopoulos et al.2007,2008,2009)have culminated in the development of a validated analysis methodology for the design of structures against surface fault rupture.Moreover, earthquake fault ruptures do not follow precisely the surface of pre-existing faults,but follow planes of weakness within a rather broad shear zone.Thus,attempting to predict the exact location of a fault breakout at the surface is almost meaningless.The modern trend in some seismic codes is not to prohibit building in the vicinity of active faults,but to demand a case-specific tectonic-geotechnical-structural study to be performed. This paper presents one such case in Greece,dealing with the analysis of two highway cut-and-cover tunnels against large tectonic dislocation.2The“Kamena Vourla”bypass and seismotectonics of the areaThe under study cut-and-cover tunnels are a part of the“Kamena V ourla”bypass in central Greece,which is one of the key elements of the550-km highway connecting southern with northern Greece.The typical tunnel cross-section consists of two tubes,24.4m in width and 9.5m in height,and slab and wall thicknesses ranging from1.0to1.4m(Fig.1).Fig.1Typical twin tunnel cross section:geometry and basic dimensions(all dimensions in meters)123Fig.2The Kamena V ourla bypass and the surface trace of the homonymous fault zone As illustrated in Fig.2,the alignment of the highway passes through a narrow corridor between the Knemis mountain range and the town of Kamena V ourla,unavoidably crossing the almost in parallel running“Kamena V ourla”fault.The latter is a normal fault of NW–SE direction,forming part of a large fault system,which runs in parallel to the Gulf of Atalanti and the greater Northern Evoikos Gulf,comprising the predominant Atalanti fault,as well as a number of other significant faults(Pantosti et al.2001).Being a tectonic graben just to the west of the dextral strike-slip North Anatolian fault zone,the seismic activity of Northern Evoikos is quite intense,characterized by an average horizontal extensional velocity of the order of5.0mm/year(Papazachos and Kiratzi1996). The predominant Atalanti fault was activated in two seismic episodes in1894:M≈6.5and M≈7.0(Ambraseys and Jackson1990;Papazachos and Papazachou1997).The two earth-quakes produced surface fault ruptures of up to1.5m and major disturbances of the landscape, over a legth of40–60km according to Skouphos(1894),Richter(1958),Rondoyanni(1984), and Pantosti et al.(2001).Based on this data,in combination with the results of the conducted hazard and seismo-tectonic studies(OTM1997),it was decided that the cut-and-cover tunnels(1and2,see Fig.2) be designed to withstand normal tectonic dislocation of vertical offset AD=1m(“operating basis”value)and MD=2m(maximum possible value).3Soil conditionsWhile the broader area comprises Mesozoic(limestones,sandstones,dolomites and igne-ous rocks)and Tertiary layers(such as marls,clays,gravels,conglomerates,and marly limestones),the study area is clearly within quaternary sediments:alluvial and lacustrine deposits,and cones of debris.The geotechnical exploration included SPT measurements and sampling.Its mainfindings are depicted in Fig.3.The soil profile consists of alternating layers of silty sand to clayey sand,gravel with cones of debris,and low plasticity clay.Essentially,two soil layers were identified:one consisting of dense silty sand and cones of debris,with number of blows of the standard penetration test,N SPT,in the range of31–75;the other consisting of very dense silty to clayey gravel,with inter-layers of rock,and with N SPT consistently larger than50.123Fig.3Compilation of geotechnical data along the axis of the cut-and-cover tunnels:soil characterization and SPT blow countsFig.5Problem definition and model dimensions:cut-and-cover tunnel of width B=24m covered with H cover ranging from1to5m,subjected toα=60◦normal faulting of maximum vertical offset at bedrock h=2m.The left edge of the tunnel is positioned at distance s from the point where the dislocation would have outcropped in the freefield4Method of analysis and constitutive modellingThe problem investigated herein is schematically illustrated in Fig.5.We analyze a soil layer of depth H,at the base of which a normal fault of dip angleαruptures with offset(downward displacement)of vertical amplitude h.Based on the previously discussed geological and seismotectonic conditions,the depth of the soil deposit is taken equal to H=50m,while h max=2m andα=60◦.The analysis is conducted under plane-strain conditions,utilizing thefinite element(FE) code ABAQUS.While the soil is modeled with quadrilateral elements,elastic beam ele-ments are used for the tunnel structure(E=25GPa,assuming minor cracking of reinforced concrete).The latter is connected to the soil through special interface elements,allowing for detachment from the bearing soil and relative displacement(sliding).The total width of the model is B=4H,following the(repeatedly validated)earlier recommendation of Bray(1990),Bray et al.(1994a,b)and the quadrilateral elements are1m×1m to achieve a reasonably refined mesh[as documented in Anastasopoulos et al.(2007)].The analysis is performed in two steps.First,fault rupture propagation through soil is analyzed in the free field,ignoring the presence of the tunnel.Then,knowing the location of fault outcropping, the tunnel is positioned so that the unperturbed fault rupture outcrops at distance s from the hanging wall(left)edge of its base slab.With respect to s,three scenarios are investigated:(a)s=6m(i.e.,roughly one fourth of the width from the hanging wall edge of the tunnel);(b)s=12m(i.e.,at the middle);and(c)s=18m(i.e.,about one fourth of the width from the footwall edge).The offset is applied in adequately small consecutive steps.Soil behavior is modeled with an elastoplastic constitutive model having a Mohr–Cou-lomb failure criterion and isotropic strain softening,encoded in ABAQUS through a user subroutine(Anastasopoulos et al.2007).Strain softening is introduced by reducing the mobilised friction angleϕmob and the mobilised dilation angleψmob with the increase of plastic octahedral shear strain:ϕmob=ϕp−ϕp−ϕresγP fγP oct,for0≤γP oct<γP fϕres,forγP oct≥γP f(1)123ψmob=⎧⎨⎩ψp1−γP octγP f,for0≤γP oct<γP fψres,forγP oct≥γP f⎫⎬⎭(2)where:ϕp andϕres the ultimate mobilised friction angle and its residual value;ψp the ultimate dilation angle;γP f the plastic octahedral shear strain at the end of softening.Soil behaviour before yielding is modeled as linear elastic,with a secant modulus G S increasing with depth, in accordance with Fig.4.Model parameters are calibrated through direct shear test results, and an approximate scaling method is employed to take account of scale effects,as described in more detail in Anastasopoulos et al.(2007).Specifically,the following model parameters were used for the two(already discussed)idealized soil layers:(a)Soil layer1:ϕp=35◦,ϕres=29◦,ψp=8◦,ψres=0◦,c=5kPa,andν=0.30(b)Soil layer2:ϕp=41◦,ϕres=32◦,ψp=14◦,ψres=0◦,c=10kPa,andν=0.30 where c is the cohesion andνthe Poisson’s ratio.Model parameters for the backfill material (dense sand and gravel),were taken as follows:Backfill material:ϕp=46◦,ϕres=38◦,ψp=19◦,ψres=0◦,c=2kPa,and ν=0.30In all cases,the selection of model parameters was based on the aforementioned calibration procedure,but with some conservatism and attempting to maintain reasonable consistency with the design recommendations of the geotechnical studies.The friction coefficient at the soil-tunnel interface was assumed equal to0.8in all cases.The modeling methodology employed herein has been extensively validated through:(a) qualitative comparisons with the published experimental results of Horsfield(1977)and Cole and Lade(1984),and the case histories of Slemmons(1957),Brune and Allen(1967),and Taylor et al.(1985);(b)semi-quantitative comparisons with the observed performance of buildings in Gölcük,Turkey,affected by the Kocaeli1999earthquake major fault rupture (Anastasopoulos and Gazetas2007a,b);and most significantly(c)through quantitative blind Class“A”predictions(Lambe1973)of centrifuge model tests(Anastasopoulos et al.2007, 2008,2009).One such prediction(shown for thefirst time in this paper)is summarized in Figs.6and7. It refers to a B=25m rigid foundation with surcharge load q=84kPa,subjected to normal faulting at distance s=14.5m(notice that the hanging wall is now to the right),through an H=25m layer of Fontainebleau sand(Gaudin2002).Model test images and shear strain contours are compared to FE deformed mesh with shear strain contours(Fig.6).The observed rupture zones(denoted S1 and S2 )are compared to the ones that develop in the free-field case(S1and S2),i.e.,without the presence of the foundation.Initially,for h≈1.23m,a steep rupture zone S1 propagates half the way to the pared to S1(i.e.,its free-field equivalent),S1 is substantially diverted towards the hanging wall.The analysis does not predict quite such a steep initial rupture zone,but shows a less steep rupture about to emerge beneath the center of the foundation.At the same time,a second rupture starts forming at the footwall(left)edge of the foundation,propagating from top to bottom.Although this not par-ticularly clear in the model test image(Fig.6a),experimental shear strain contours(Fig.6b) agree fairly well with the numerical prediction.This second rupture starts becoming visible in the centrifuge test image for h≈1.52m:S2 makes its appearance just to the left of the foundation.It can be seen to correspond to S2of the free-field case,but strongly diverted(by about10m)towards the footwall.The numerical prediction(h=1.5m),is quite in line with the experiment.However,while in the centrifuge test shear strain tends to accumulate along S2 ,the analysis reveals stronger strain localization along S1 .Further increase of h to2.0m,123Fig.6Class“A”prediction of B=25m rigid foundation with surcharge load q=84kPa,subjected to normal faulting at distance s=14.5m:a centrifuge model test images;b experimental shear strain contours; compared to c FE predicted deformed mesh with superimposed shear stain contoursleads to deformation localization along S2 .Now,analysis and experiment are in very goodagreement.The comparison in terms of vertical displacement at the surface is satisfactoryfor all levels of imposed deformation(Fig.7).5Tunnel response to tectonic dislocationThe results are presented in terms of:(a)deformed mesh with superimposed plastic shearstrain contours;(b)tunnel displacement (which is the sum of rigid body motion and struc-tural distortion);(c)structural distortionδ(to better visualize the bending of tunnel slabsand walls);(d)normal contact stressesσv andσh at tunnel slabs and walls,respectively;and(e)bending moments M of tunnel structural elements.First,we focus on the tunnel withH cover=5m in combination with the“soft”soil profile,and elucidate the response of the soil-tunnel system for the three rupturing location scenarios:s=6,12,and18m.We theninvestigate the role of the overburden pressure(cover)by comparing the response of a tunnelwith H cover=1m.Finally,to unravel the effect of soil compliance we compare the behavior of the tunnel in the“soft”and in the“stiff”soil.5.1Tunnel subjected to faulting at s=6mThe response of the tunnel subjected to rupturing close to its hanging wall(left)edge is por-trayed in Fig.8in terms of deformed mesh with superimposed plastic shear strain contours. 123Fig.7Class“A”prediction of B=25m rigid foundation with surcharge load q=84kPa,subjected to normal faulting at distance s=14.5m:comparison of numerical with experimental vertical displacement at the ground surface.Imposed bedrock dislocation h=0.4–2.5mAt the beginning,for h=0.5m,a single rupture outcrops close to the left edge of the tunnel. The rupture can be seen to be substantially diverted towards the hanging wall(the dashed line represents the free-field rupture path),without however avoiding the tunnel.Increasing the imposed bedrock displacement to h=1.0m leads to a pronounced bifurcation of the rupture plane(i.e.,a change of mechanism).Now,a second rupture emerges almost at the middle of the bottom slab of the tunnel,while some diffusion can be observed in the same area. At the same time,an active-type wedge forms at the left(hanging wall side)of the tunnel, evidently due to the imposed extensional deformation(normal faulting).Further increase of h leads to accumulation of deformation along the already developed rupture planes,and to development of a second also active-type wedge at the footwall(right)edge of the tunnel (clearly seen for h=2m).Figure9depicts the evolution of tunnel displacement and distortion with increasing bed-rock offset h.The tunnel is subjected to horizontal and vertical displacements,rigid body rotation(Fig.9a),as well as distortion(Fig.9b).With the main rupture emerging next to the hanging wall(left)edge of tunnel,the structure is subjected to hogging deformation. Increasing h from0.5to2m leads to a substantial increase of the rotation of the tunnel.Para-doxically,however,the distortion of some tunnel members tends to decrease with increasing h.To explain it,consider the role of the previously discussed change of mechanism:the bifur-123Fig.8Tunnel with H cover=5m in the“soft”soil profile,subjected to normal faulting at distance s=6m: deformed mesh(deformation scale factor=2)with superimposed plastic strain contours for bedrock offset h=0.5–2.0m(the dashed line represents the freefield rupture path)cation of the rupture plane and the associated diffusion of the tectonic deformation towards the middle of the tunnel seem to act as a stress relief for the tunnel.The effect of this mecha-nism change is also evident in Fig.9c:for h≥0.7m the slope of y(vertical displacement) and D y(differential displacement,representing the rotation)increases remarkably,while x remains almost constant.Evidently,the mechanism change leads to transformation of the imposed deformation to rigid-body rotation rather than structural distortion. x is almost insensitive to h:since the tunnel is founded on the footwall,it is not subjected to substantial horizontal displacement.123Fig.9Tunnel with H cover=5m in the“soft”soil profile,subjected to normal faulting at distance s=6m: evolution of a tunnel displacement ;b distortionδ;and c horizontal displacement x,vertical displacementy and differential displacement D y with the increase of imposed bedrock offset hFig.10Tunnel with H cover=5m in the“soft”soil profile,subjected to normal faulting at distance s=6m: evolution with increasing imposed bedrock offset h of:a tunnel contact stressesσv andσh:b bending moments MFigure10illustrates the contact normal stressesσv andσh and bending moments M of tunnel structural elements(slabs and walls).As expected,the normal tractions on the top slab are insensitive to the imposed deformation(Fig.10a):the soilfill(cover)is displaced along with the tunnel.In stark contrast,bottom slab and side walls are subjected to significant stress changes,which result in tunnel distress.Observe that the horizontal stressσh acting on the side walls is decreased substantially with the increase of h:this is due to the already discussed(see also Fig.8)development of active type conditions.Notice,however,that at the top of the left and at the bottom of the right wallσh becomes larger than its initial value (before application of the dislocation,h=0).This is attributed to the rotation of the tunnel(see Fig.9a),which tends to compress the soil in these areas,locally generating larger stresses(closer to passive conditions).Notice also the substantial increase ofσv in the middle of the right side of the tunnel when h=2m:this is because the tunnel is now supported primarily in this location.At the bottom slab,the increase of h from0.5to2m leads only to a30%increase of the maximum observed M;from2.5to3.3MNm(Fig.10b).Evidently,the response of the system is highly non-linear,with a substantial part of the ultimate stressing being already attained when h≈0.5m:i.e.,roughly for the bedrock offset at which the primary rupture emerges underneath the tunnel.As soon as equilibrium is reached,any further imposed deformation does not seem to cause a substantial increase of the stressing(see also Fig.9).This response is qualitatively similar to that of slab foundations subjected to faulting(Anastasopoulos et al. 2009).Interestingly,the stressing of the top slab may even be reduced with increasing dislo-cation:increasing h from0.5to2m reduces M from4.2to3.9MNm.The same observation also holds for the left wall.The culprit for this highly nonlinear(resembling“softening”) behavior is the aforementioned mechanism change,which acts as a means of stress relief at least for some tunnel elements.5.2Tunnel subjected to faulting at s=12mWe now move the unperturbed fault rupture under the middle of the tunnel.As shown in Fig.11,for h=0.5m a single rupture is approaching the base of the tunnel,but without ever pared to the free-field path,it is diverted towards the hanging wall(to the left).As for s=6m(see Fig.8),the increase of h to1.0m leads to bifurcation of the rupture plane.However,due to the different geometry,the second rupture now emerges very close to the footwall(right)edge of the tunnel.Further increase of h leads to accumulation of deformation along the two rupture planes.Interestingly,a second smaller bifurcation can be observed at the tip of thefirst rupture,just underneath the bottom slab of the tunnel.Overall, the deformation patterns observed for the tunnel are qualitatively very similar to those for the raft foundation of Fig.6,which is of almost the same width(25m)but is subjected to lower surcharge load:84kPa,compared to the total load of the soil cover(5m×16kN/m3=80kPa) plus the self-weight of the tunnel(roughly some additional85kPa).The active-type wedges form almost with the same sequence as for s=6m.Fig.11Tunnel with H cover=5in the“soft”soil profile,subjected to normal faulting at distance s=12m:deformed mesh(deformation scale factor=2)with superimposed plastic strain contours for bedrock offset h=0.5–2.0m(the dashed line represents the freefield rupture path)The evolution of tunnel displacement and distortion with increasing h is illustrated inFig.12.Initially,for h≤0.5m,the main rupture approaches the tunnel close to its hangingwall(left)edge,generating hogging deformation.However,the increase of the imposed bed-rock offset,the bifurcation and the accumulation of additional deformation on the secondrupture(which emerges close to the footwall edge)lead to a rather dramatic mechanismchange:the structure is now subjected to sagging deformation.Again,the response is quali-tatively similar to that of raft foundations(Anastasopoulos et al.2009).In marked contrast tothe s=6m case,the increase of y and D y with h is now almost linear(Fig.12c).Interest-ingly,while the maximum y(for h=2m)is larger compared to the previous case(1.15minstead of0.75m for s=6m),D y is practically the same(1.85m,i.e.,a7.6%rotation). x is again rather insensitive to h,merely reaching0.13m for h=2m.Contact stressesσv andσh,and bending moments M are depicted in Fig.13.As in theprevious case,σv at the top slab is insensitive to the imposed deformation(Fig.13a).Unequiv-ocally,the bottom slab is subjected to substantial stress changes.For h=0.5m,a ratherlarge stress concentration(increase ofσv)is observed not far from the middle of the slab, accompanied by decompression at the two edges.In fact,σv close to the left edge becomesvanishingly small,indicating that the tunnel almost detaches from the bearing soil.Thisstress distribution is directly related to the aforementioned hogging deformation of the tun-nel.But for h=2m,the distribution ofσv changes radically.Stress concentration is nowobserved at the two edges,with decompression taking place at the middle of the tunnel:sagging deformation.As for s=6m,the horizontal stressesσh acting on the side walls tend to decrease(development of active conditions),with local passive-type stress increases due to tunnelrotation.The mechanism reversal(from hogging to sagging deformation)is responsible for thelarge changes of the bending moment M with increasing h(Fig.13b).At the bottom slab,with h varying from0.5to2m a different distribution of M develops:while for h=0.5mthe maximum M takes place at the middle of the right span(2.7MNm),for h=2m it ismaximized in the middle at the connection with the wall(3.7MNm).The same observationis valid for the top slab and the side walls:the change of mechanism creates a reversal of thestressing of all tunnel members(with the middle wall being the only exception).5.3Tunnel subjected to faulting at s=18mFinally,we further move the dislocation close to the footwall(right)edge of the tunnel.Snap-shots of deformed mesh with superimposed plastic shear strain contours are shown in Fig.14.Initially,for h=0.5m,a single rupture terminates at the base of the tunnel,slightly divertedtowards the hanging wall(to the left).As for s=12m,the increase of h to1.0m leads tobifurcation of the rupture plane,with the second rupture now emerging just at the footwall(right)edge of the tunnel.Further increase of h leads to accumulation of most of the additionaldeformation along the second rupture.As in the previous case,a second smaller bifurcationis observed at the tip of thefirst rupture.The active-type wedges form again with almost thesame sequence,but the one in the footwall side(right)isfinally overshadowed by the out-cropping rupture plane.At the footwall side wedge,a secondary antithetic rupture and a smallgravity graben can be clearly seen for h≥1.5m.This extensional feature is largely attributedto the lateral stiffness of the tunnel,which forces the extension to localize in this area.Figure15depicts the evolution of tunnel displacement and distortion with h.Now,thetunnel is always subjected to sagging deformation with the increase of h simply leadingto amplified distortion.Again,the response of the tunnel is qualitatively similar to that of。