无线电能传输
无线电能传输技术研究
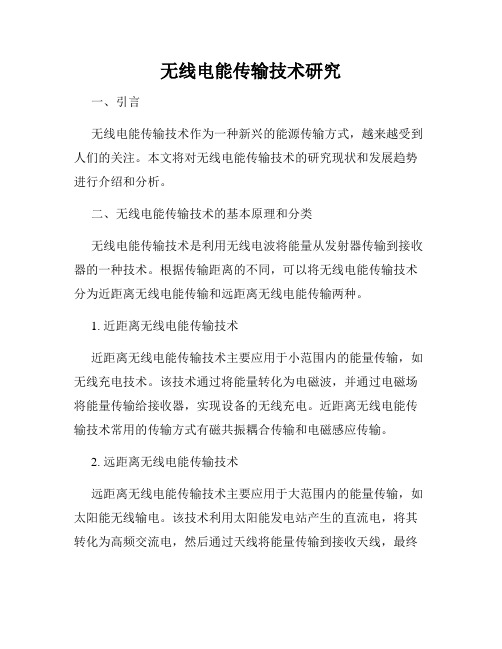
无线电能传输技术研究一、引言无线电能传输技术作为一种新兴的能源传输方式,越来越受到人们的关注。
本文将对无线电能传输技术的研究现状和发展趋势进行介绍和分析。
二、无线电能传输技术的基本原理和分类无线电能传输技术是利用无线电波将能量从发射器传输到接收器的一种技术。
根据传输距离的不同,可以将无线电能传输技术分为近距离无线电能传输和远距离无线电能传输两种。
1. 近距离无线电能传输技术近距离无线电能传输技术主要应用于小范围内的能量传输,如无线充电技术。
该技术通过将能量转化为电磁波,并通过电磁场将能量传输给接收器,实现设备的无线充电。
近距离无线电能传输技术常用的传输方式有磁共振耦合传输和电磁感应传输。
2. 远距离无线电能传输技术远距离无线电能传输技术主要应用于大范围内的能量传输,如太阳能无线输电。
该技术利用太阳能发电站产生的直流电,将其转化为高频交流电,然后通过天线将能量传输到接收天线,最终转化为直流电。
远距离无线电能传输技术常用的传输方式有微波传输和激光传输。
三、无线电能传输技术的应用领域无线电能传输技术具有广泛的应用领域,以下是一些常见的应用领域:1. 智能家居无线电能传输技术可以在家庭内实现智能家居设备的无线充电,提高家庭用电的便利性和安全性。
2. 移动通信无线电能传输技术可以为移动通信设备提供长时间的续航能力,减少用户频繁充电的困扰。
3. 无人机与机器人无线电能传输技术可以为无人机和机器人等设备提供能源支持,延长其工作时间和工作距离。
4. 新能源领域无线电能传输技术可以解决新能源发电和输电的难题,提高能源利用效率和节能减排效果。
四、无线电能传输技术的发展趋势无线电能传输技术在不断发展壮大的同时,也面临一些挑战和发展趋势。
1. 传输效率的提高目前无线电能传输技术的传输效率还不高,需要进一步提高能量传输的效率,降低能量在传输过程中的损耗。
2. 安全性的增强无线电能传输技术涉及到大量的能源传输和电磁波的产生与传输,需要加强对无线电波辐射对人体和环境的安全性研究和保障。
无线电能传输原理
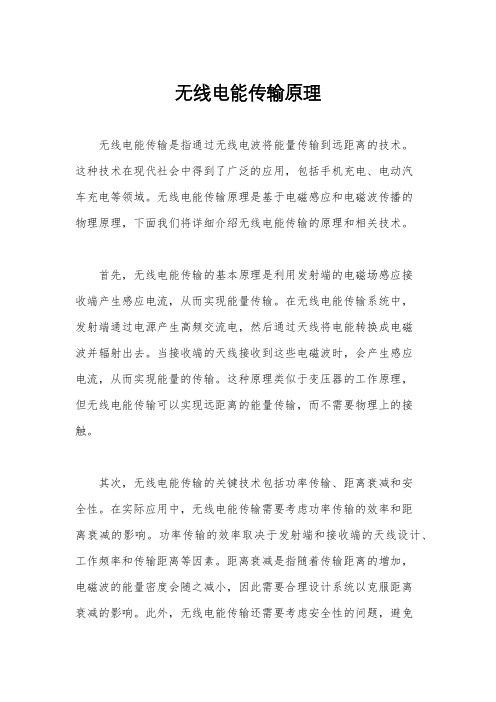
无线电能传输原理无线电能传输是指通过无线电波将能量传输到远距离的技术。
这种技术在现代社会中得到了广泛的应用,包括手机充电、电动汽车充电等领域。
无线电能传输原理是基于电磁感应和电磁波传播的物理原理,下面我们将详细介绍无线电能传输的原理和相关技术。
首先,无线电能传输的基本原理是利用发射端的电磁场感应接收端产生感应电流,从而实现能量传输。
在无线电能传输系统中,发射端通过电源产生高频交流电,然后通过天线将电能转换成电磁波并辐射出去。
当接收端的天线接收到这些电磁波时,会产生感应电流,从而实现能量的传输。
这种原理类似于变压器的工作原理,但无线电能传输可以实现远距离的能量传输,而不需要物理上的接触。
其次,无线电能传输的关键技术包括功率传输、距离衰减和安全性。
在实际应用中,无线电能传输需要考虑功率传输的效率和距离衰减的影响。
功率传输的效率取决于发射端和接收端的天线设计、工作频率和传输距离等因素。
距离衰减是指随着传输距离的增加,电磁波的能量密度会随之减小,因此需要合理设计系统以克服距离衰减的影响。
此外,无线电能传输还需要考虑安全性的问题,避免对人体和其他设备造成危害。
最后,无线电能传输技术的发展趋势是提高传输效率、扩大传输距离和提高安全性。
为了提高传输效率,研究人员正在开发新的天线设计和工作频率选择,以提高能量传输的效率。
同时,为了扩大传输距离,研究人员也在探索新的传输技术和材料,以克服距离衰减的影响。
此外,为了提高安全性,研究人员正在开发新的安全控制技术,以确保无线电能传输不会对人体和其他设备造成危害。
总的来说,无线电能传输是一种基于电磁感应和电磁波传播的技术,通过发射端产生的电磁波感应接收端产生感应电流,从而实现能量传输。
在实际应用中,无线电能传输需要考虑功率传输、距离衰减和安全性等关键技术,未来的发展趋势是提高传输效率、扩大传输距禿和提高安全性。
通过不断的研究和创新,无线电能传输技术将会在更多领域得到应用,并为人们的生活带来便利和舒适。
无线电能传输
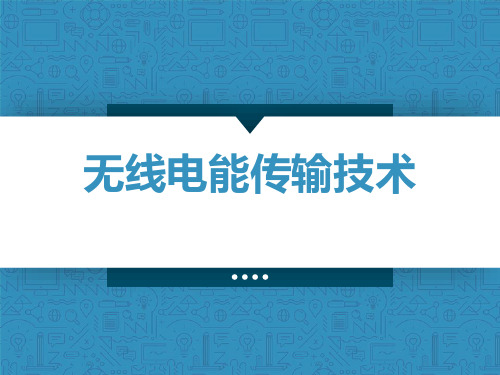
目 录
CONTENT
01 | 背景介绍
02 | 基本结构与工作原理
03 | 技术应用研究
04 | 发展趋势
PA R T O N E
0
1
背景介绍
背景介绍
• 在《三体》小说里,描绘了未来世界的 场景。在那个世界里也没有烦人的插座
4
和各类充电接口,在那个世界里汽车飞
机以及手机,全都是电动的,但是不需 要充电也不担心续航的问题。所有电器
发展趋势
23
预测 WPT 技术今后的发展趋势主要有以下几个方面:
• WPT 技术理论的系统化 • 智能化 WPT 系统 • WPT 系统的电磁环境安全 • WPT 技术行业标准。
谢谢观看
请老师同学批评指正
14
准直整形处理后发射,并通过自由空间到达接收端,且经过光学接收天
线接收聚焦到光电转换模块上完成激光–电能的转换。
PART THREE
0
3
技术应用研究
技术应用研究
16
MIT螺旋式无线电能传输样机
海尔的无尾电视
高通在宝马i8上搭载无线充电技术
苹果手机的无线快充
技术应用研究
17
• 磁 耦 合 谐 振 式 无 线 电 能 传 输 (magnetically-coupled resonant
19
技术应用研究
•
20
2011年,美国华盛顿大学、匹兹堡大学医学中心与英特尔宣布,利用磁耦合谐振无线电 能传输技术,共同试制出了植入式人工心脏使用的供电系统,该系统在一般的直径为数十 厘米谐振线圈的基础上进行了改进,在人工心脏上安装了直径4.3cm的接收线圈,并且将 其放入模拟人体组织环境的容器中,对能否从容器外部供电进行了实验研究。结果显示, 能够以80%的传输效率稳定施供电。如果把该技术与容量可为人工心脏供电约2个小时的 蓄电池组合使用,电源线就无需探出体外感染的风险会因此而骤降。而且,在蓄电池未耗
国内 无线电能传输技术
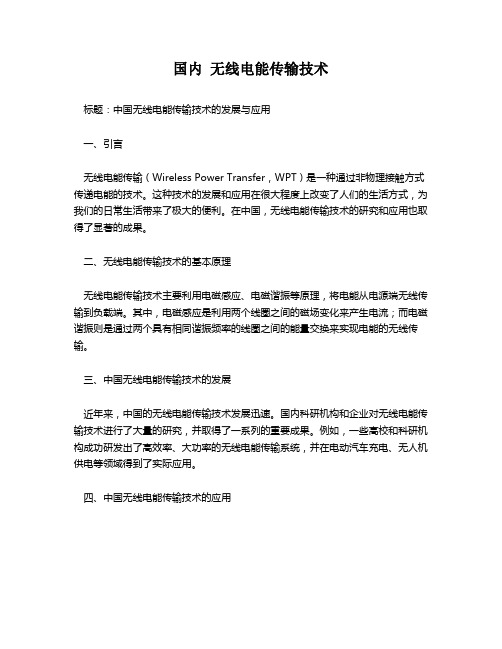
国内无线电能传输技术标题:中国无线电能传输技术的发展与应用一、引言无线电能传输(Wireless Power Transfer,WPT)是一种通过非物理接触方式传递电能的技术。
这种技术的发展和应用在很大程度上改变了人们的生活方式,为我们的日常生活带来了极大的便利。
在中国,无线电能传输技术的研究和应用也取得了显著的成果。
二、无线电能传输技术的基本原理无线电能传输技术主要利用电磁感应、电磁谐振等原理,将电能从电源端无线传输到负载端。
其中,电磁感应是利用两个线圈之间的磁场变化来产生电流;而电磁谐振则是通过两个具有相同谐振频率的线圈之间的能量交换来实现电能的无线传输。
三、中国无线电能传输技术的发展近年来,中国的无线电能传输技术发展迅速。
国内科研机构和企业对无线电能传输技术进行了大量的研究,并取得了一系列的重要成果。
例如,一些高校和科研机构成功研发出了高效率、大功率的无线电能传输系统,并在电动汽车充电、无人机供电等领域得到了实际应用。
四、中国无线电能传输技术的应用目前,中国的无线电能传输技术已经在多个领域得到了广泛应用。
在电动汽车充电方面,无线电能传输技术可以实现电动汽车的无接触充电,大大提高了充电的便利性。
在医疗设备供电方面,无线电能传输技术可以实现植入式医疗设备的无线供电,降低了手术风险。
此外,无线电能传输技术还在智能家居、消费电子等领域有着广阔的应用前景。
五、结论总体来看,中国的无线电能传输技术已经取得了显著的进步,并且在多个领域得到了广泛的应用。
未来,随着科技的进步和市场需求的变化,中国的无线电能传输技术将会得到进一步的发展,为人们的生活带来更多的便利。
无线电能传输原理
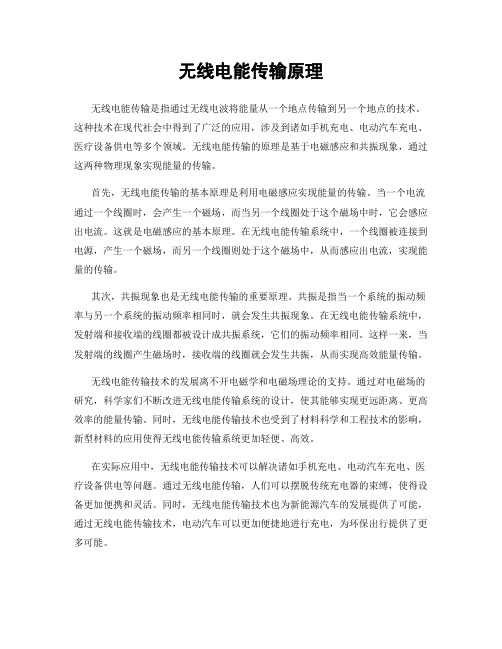
无线电能传输原理无线电能传输是指通过无线电波将能量从一个地点传输到另一个地点的技术。
这种技术在现代社会中得到了广泛的应用,涉及到诸如手机充电、电动汽车充电、医疗设备供电等多个领域。
无线电能传输的原理是基于电磁感应和共振现象,通过这两种物理现象实现能量的传输。
首先,无线电能传输的基本原理是利用电磁感应实现能量的传输。
当一个电流通过一个线圈时,会产生一个磁场,而当另一个线圈处于这个磁场中时,它会感应出电流。
这就是电磁感应的基本原理。
在无线电能传输系统中,一个线圈被连接到电源,产生一个磁场,而另一个线圈则处于这个磁场中,从而感应出电流,实现能量的传输。
其次,共振现象也是无线电能传输的重要原理。
共振是指当一个系统的振动频率与另一个系统的振动频率相同时,就会发生共振现象。
在无线电能传输系统中,发射端和接收端的线圈都被设计成共振系统,它们的振动频率相同。
这样一来,当发射端的线圈产生磁场时,接收端的线圈就会发生共振,从而实现高效能量传输。
无线电能传输技术的发展离不开电磁学和电磁场理论的支持。
通过对电磁场的研究,科学家们不断改进无线电能传输系统的设计,使其能够实现更远距离、更高效率的能量传输。
同时,无线电能传输技术也受到了材料科学和工程技术的影响,新型材料的应用使得无线电能传输系统更加轻便、高效。
在实际应用中,无线电能传输技术可以解决诸如手机充电、电动汽车充电、医疗设备供电等问题。
通过无线电能传输,人们可以摆脱传统充电器的束缚,使得设备更加便携和灵活。
同时,无线电能传输技术也为新能源汽车的发展提供了可能,通过无线电能传输技术,电动汽车可以更加便捷地进行充电,为环保出行提供了更多可能。
总的来说,无线电能传输技术是一种基于电磁感应和共振现象的能量传输技术,它的发展离不开电磁学、材料科学和工程技术的支持。
通过无线电能传输技术,人们可以实现更加便捷、高效的能量传输,为现代社会的发展提供了更多可能。
随着科学技术的不断进步,相信无线电能传输技术会在更多领域得到应用,为人们的生活带来更多便利。
国内 无线电能传输技术
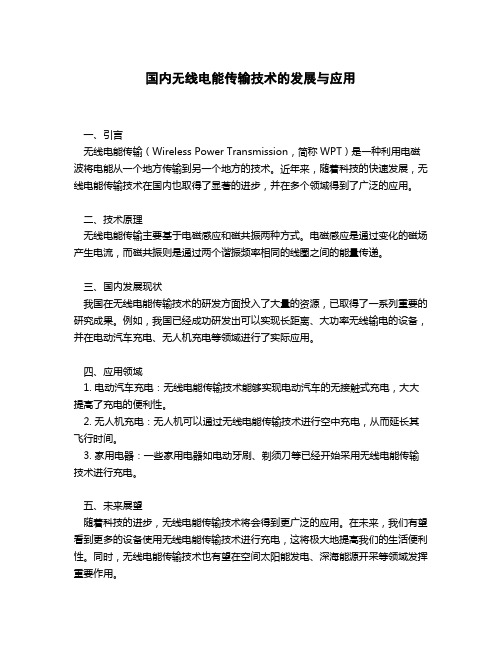
国内无线电能传输技术的发展与应用一、引言无线电能传输(Wireless Power Transmission,简称WPT)是一种利用电磁波将电能从一个地方传输到另一个地方的技术。
近年来,随着科技的快速发展,无线电能传输技术在国内也取得了显著的进步,并在多个领域得到了广泛的应用。
二、技术原理无线电能传输主要基于电磁感应和磁共振两种方式。
电磁感应是通过变化的磁场产生电流,而磁共振则是通过两个谐振频率相同的线圈之间的能量传递。
三、国内发展现状我国在无线电能传输技术的研发方面投入了大量的资源,已取得了一系列重要的研究成果。
例如,我国已经成功研发出可以实现长距离、大功率无线输电的设备,并在电动汽车充电、无人机充电等领域进行了实际应用。
四、应用领域1. 电动汽车充电:无线电能传输技术能够实现电动汽车的无接触式充电,大大提高了充电的便利性。
2. 无人机充电:无人机可以通过无线电能传输技术进行空中充电,从而延长其飞行时间。
3. 家用电器:一些家用电器如电动牙刷、剃须刀等已经开始采用无线电能传输技术进行充电。
五、未来展望随着科技的进步,无线电能传输技术将会得到更广泛的应用。
在未来,我们有望看到更多的设备使用无线电能传输技术进行充电,这将极大地提高我们的生活便利性。
同时,无线电能传输技术也有望在空间太阳能发电、深海能源开采等领域发挥重要作用。
六、结论总体来看,无线电能传输技术在我国的发展前景十分广阔。
然而,要实现这一技术的大规模应用,还需要我们在技术研发、标准制定等方面做出更大的努力。
我们期待无线电能传输技术能够在未来的日子里为我们的生活带来更多的便利。
无线电能传输技术研究
无线电能传输技术研究现如今,人们对于电能的需求是越来越高。
然而,传统有线电路方式传输电能存在众多的限制和缺陷,比如不能跨越大片区域,容易造成漏电等问题。
而无线电能传输技术的诞生,则在一定程度上缓解了这些问题,成为了一种备受关注的新型能源技术。
下面,本文将就无线电能传输技术进行深入探究。
一、无线电能传输技术的概念与分类无线电能传输技术,简称无线能量传输技术,是指使用电磁波进行无线传输能量的技术。
它可以将电能转化为无线电能,实现电能在空间上的传输,从而实现电能的遥控、无线供电等功能。
一般来讲,无线电能传输技术可以分为短距离和长距离两种。
1.短距离无线电能传输技术短距离无线电能传输技术,主要指定向传输和环向传输两种技术方式。
其中定向传输是指通过微波或激光束将电能传送到指定的接收器,而环向传输则是通过电磁波将电能传输到空间中的任意位置。
2.长距离无线电能传输技术长距离无线电能传输技术,则被称为远距离微波无线电能传输技术。
它通过在两个距离较远的位置分别设置发射器和接收器,利用微波来传送电能,实现了跨越大片区域的无线电能传输。
二、无线电能传输技术的应用与发展现状无线电能传输技术,优点很多,比如使用方便、可遥控、能够跨越一定距离、安全可靠等等,并且还可以应用到很多领域上。
比如在医疗方面,无线电能传输技术可以用于生产医疗器械,使其更加智能化;在农业方面,该技术可以应用于土壤水分监测、作物灌溉等方面。
除此之外,它还可以应用于智能家居、无人机等方面,为我们的生活带来了更加方便和高效。
目前,无线电能传输技术的发展还处于探索和研究阶段,还需要不断地努力和不断地完善。
近年来,各大科技企业都在积极探索该领域,并取得了一定的成果。
比如,日本的 NTT 通信公司就已经研发出了将电气能力进行转换成为无线电波并进行远距离传输的控制技术,相信随着科技的不断进步,该技术在未来会得到更加广泛和深入的应用。
三、无线电能传输技术的优缺点分析无线电能传输技术的优点非常明显,主要包括以下几个方面:1.避免了传统有线电路形成的耗能、漏电等负面影响。
无线电能传输技术
无线电能传输技术无线电能传输技术指的是传播能量时不使用电缆和线路的技术,也称为无线电能传导或无线电能外射。
无线电能传输技术可以实现远距离传输,它是无线网络技术的一个重要组成部分,广泛应用于军事和民用领域。
无线电能传输技术分为容量和时间两类。
容量传输要求能量在接收端可以稳定存在,而时间传输要求能量的持续时间足够短,能量的损耗较小。
无线电能传输技术也可以根据传输系统的架构来分类,准确性更高。
无线电能传输技术的发展受到了技术的不断进步的推动。
在过去的几十年里,无线电能传输技术经历了从单路径到多路径、从广播到半导体等技术改进,以及无线信号模式发展等,使传输效率和质量显著提高,技术飞速发展,利用率持续提高。
传统的无线电能传输技术主要是微波和红外线的传输,其中,微波传输技术主要利用发射天线发射微波信号,然后用接收天线接收微波信号并且转换成电能,从而达到长距离传输的效果。
红外线传输技术利用被称为发射光源的红外线发射器,发射一定强度的红外线,接收端利用接收光源接收红外线信号,并且转换成电能,从而达到传输目的。
随着科学技术的不断发展,无线电能传输技术发生了很大的改变,不仅在传输距离和传输效率上有了显著提高,而且在应用单位上也发生了变化。
如今,无线电能传输技术已经应用于电力行业、制造业和航空航天等,能够高效、安全地实现远距离能量传输,也成为电子产品和装备的重要基础技术。
从实际应用和研究上来说,无线电能传输技术主要有自动发射、智能发射、无线能量联网等,这些技术都是由传统的微波传输和红外线传输技术发展而来的,但在应用、研究过程中充分利用了新兴技术,使系统更加强大,传输效率更高。
无线电能传输技术在未来发展前景很广,已经成为现代社会的重要技术和应用领域。
未来,无线电能传输技术将发展到更高水平,能够更有效地实现远距离传输,应用于电力行业、制造业、军事领域等更为广泛,更可靠,更安全。
无线电能传输技术将在未来发挥越来越主要的角色,为电子产品和各类装备的发展提供更多的可能性,改变我们的生活。
无线电能传输技术及其应用研究
无线电能传输技术及其应用研究随着科技的不断进步和发展,越来越多的新技术不断涌现出来,其中无线电能传输技术是一项备受瞩目的技术。
它是利用电磁波在空气或其他介质中进行能量传递的一种技术,可以实现无线充电、远程供电等应用,具有广泛的应用前景。
本文将对无线电能传输技术及其应用进行研究和探讨。
一、无线电能传输技术简介无线电能传输技术是一种通过电磁波传输能量的技术。
在传统的有线电力传输方式中,电能是通过导线进行传输,这种方式存在着能量损耗大、安全隐患等问题。
而无线电能传输技术可以减少能量损耗,避免电线带来的安全隐患,具有更高的稳定性和可靠性。
无线电能传输技术主要分为两种:磁共振和电磁辐射。
磁共振是一种通过磁场共振转换能量的技术,它需要特殊的电路和设备来产生强磁场进行能量传输。
而电磁辐射则是通过电磁波在空气或介质中进行能量传输的技术,需要在发射端和接收端之间建立电磁场。
二、无线电能传输技术的应用领域无线电能传输技术具有广泛的应用前景,以下列举一些典型的应用领域:1.无线充电:无线充电是无线电能传输技术的一个重要应用领域。
通过将电磁场的能量传输到手机、电动汽车等设备中,实现对它们进行无线充电。
目前,无线充电技术已经广泛应用于移动设备、数码相机等电子产品中,正在逐步向其他领域拓展。
2.空间电力传输:空间电力传输是指将太阳能等可再生能源通过无线电能传输技术传输到地面或其他地方进行利用。
这种技术可以有效地解决能源短缺问题,同时也有助于保护环境。
目前,空间电力传输技术正在逐渐成熟。
3.医疗应用:无线电能传输技术在医疗领域也有广泛的应用。
例如,可以将无线电能传输到implantable medical device中,为患者提供持续的能量供应,避免患者不得不进行频繁的充电。
这对于一些身体残疾或严重疾病患者来说,具有非常重要的意义。
三、无线电能传输技术的优势无线电能传输技术相较于传统的有线传输技术具有多方面的优势,以下列举一些典型的优势:1.噪声较小:与传统的有线传输技术相比,无线电能传输技术传输过程中噪声会更小。
无线电能传输技术 (修改)
究和应用
3 在无线通信方面,国内在5G技术、物联网、智能家居等领域都取得了重要进展
4
5G技术的发展为无线通信带来了更高的传输速率和更低的延迟,推动了物联网、智能家居等领域的快 速发展
无线电能传输技术
1
同时,国内也在积极推进物联网的建设和应用,涉及到智能家居、智能交通、智能城市等多个领域.此 外,国内也在积极探索无线传输技术在医疗、工业等领域的应用
2
例如华中科技大学研究团队提出了一种基于磁共振的无线电能传输技术,可以将电能以高效、可靠的 方式传输到远离电源的设备中,具有较高的应用价值
3
同时,在工业界也存在着对无线电能传输技术的应用探索,例如近年来各大手机厂商开始采用无线充 电技术,可以将手机电池以无线电波的形式进行充电,解决用户使用手机时传输线缆带来的不便
无线电能传输技术
3. 工业自动化和智能制造的需求增长:工业自动化和智能制造领域对于无线传输技术的需求将会持续增 长。无线传输技术能够实现高效、可靠的数据传输,为工业自动化和智能制造提供更好的解决方案 4. 物联网和智能家居的广泛应用:物联网和智能家居领域对于无线传输技术的需求将会持续增长。无线 传输技术能够实现设备之间的无缝连接和数据传输,为人们的生活带来更多的便利和智能化 5. 安全性提高和互操作性增强:随着无线传输技术的广泛应用,安全性和提高互操作性将是未来发展的 重要方向。采用加密技术、建立安全的网络协议和加强用户身份验证等措施,能够提高无线传输的安全 性。同时,加强设备的互操作性测试,能够提高不同设备之间的兼容性和无缝连接能力 总之,无线传输技术在未来几年中具有广阔的发展前景,将在各个领域得到广泛应用。随着技术的不断 进步和应用场景的不断扩展,无线传输技术将会在更多领域发挥重要作用
- 1、下载文档前请自行甄别文档内容的完整性,平台不提供额外的编辑、内容补充、找答案等附加服务。
- 2、"仅部分预览"的文档,不可在线预览部分如存在完整性等问题,可反馈申请退款(可完整预览的文档不适用该条件!)。
- 3、如文档侵犯您的权益,请联系客服反馈,我们会尽快为您处理(人工客服工作时间:9:00-18:30)。
Frequency dependence of magnetic flux profile in the presence of metamaterials for wireless powertransferBoopalan GSchool of Electronics EngineeringVIT UniversityVellore, Tamil Nadu, Indiaboopalan@vit.ac.inSubramaniam C K School of Advance Sciences VIT University Vellore, Tamil Nadu, India subramaniam@vit.ac.inAbstract— We discuss the change in the magnetic flux profile by introducing a negative refractive index material (metamaterial) in between the source and receiver. The environment parameters, ε and μ , has a significant effect on the propagation of electromagnetic wave. The behavior of Transverse Magnetic (TM) wave when the medium in the path of propagation is changed to negative permittivity and permeability is simulated and discussed. The effect of size, shape and anisotrophy of the metamaterials, for near-field regions, on the magnetic flux density has been studied using finite element analysis. An enhancement in the magnetic flux density when a metamaterial is introduced in between the source and receiver was observed. The results show that the static and quasi-static behavior of the system is same.Keywords—metamaterials, quasi static, magnetic flux transverse magneticI.I NTRODUCTIONThe idea of charging on the go is an exciting option for various high power applications like Electric Vehicle. Wireless power charging can be done by radiative or non-radiative processes. Use of microwave and optical frequencies falls into the radiative category while non-radiative process refers to the near-field domain. This concept was put forward by Nikola Tesla when he invented an apparatus for transmitting electrical energy wirelessly [1]. Later, with the advent of microwave transmission technology in 1960’s researchers dreamed power transfer from satellite space station to earth [2]. For short distances inductive coupling is very convenient [3-4]. The enhancement in coupling efficiency is obtained by replacing coils with resonators [5-7]. The efficiency can further be improved by introducing a negative refractive index material between the source and the receiver [8-12]. The negative refractive index material or metamaterial has the unique property of enhancing the evanescent as well as non-evanescent waves [10].In this paper we present the magnetic flux density variations for quasi-static scenarios when a metamaterial is introduced in between the source and the receiver. The model used for simulation is a 2-dimensional one as we are interested only in the profile in that direction which is in the direction of propagation.II.T HEORYOur system consists of a source, receiver and a metamaterial as shown in fig. 1. The source is a circular loop of radius ‘a’ located in free space. The receiver is a point of interest ‘P’ where the magnetic flux density enhancement is observed. The metamaterial in between the source and the receiving point is a rectangular block which enhances the magnetic flux density at the point ‘P’. The transmitter is a single turn coil carrying current ‘I’ which in turn generates the magnetic field H in the surrounding medium. The magnetic field H at a distance ‘z’ from the center of the coil is given byI(1)The coil is fed with a current of ‘I’ amperes as given by the equation belowI . (2)Fig. 1. Schematic of Wireless Power transferyxzwhere, ‘J’ is the current density and ‘ds’ is the vector component perpendicular to the cross section of the circular coil. Then the applied potential V related to the current density J is given by the equationJ σVπ(3)where ‘σ’ is the conductivity of the coil and ‘a’ is the radiusof the coil.The direction of propagation is ‘z’, towards the point Pin fig. 1. To enhance the flux density the metamaterials of optimized dimension is placed in between the source and the receiver at a distance ‘z/2’ from the center of the transmitter coil as shown in figure 1. The metamaterial is one whose permittivity ε and permeability μ is negative. The materials can be classified on the values of εand μ as dielectric, plasmas, ferri-magnetic or metamaterial [11]. These parameters also constitute the propagation of electromagnetic waves in matter. Since the permittivity and permeability are negative for the metamaterial the refractive index also becomes negative as explained below. The refractive index is related to relative permittivity and relative permeability as mentioned belowε μ(4)If εr is -1 and μr is -1 then substituting e-iπ for -1 in the above equation gives refractive index as -1.We can solve the problem using FDTD (Finite Difference Time Domain) or the FEM (Finite Element Method). In both cases, the Maxwell equations are solved by dividing the solution region into nodes or elements considering suitable boundary conditions. We have used COMSOL Multiphysics, an FEM based tool for our simulation. The governing equation for quasi static situation is given byε (5)The magnetic flux density related to the magnetic potential and magnetic field intensity are given byμ (6)III.SIMULATIONA.ModelThe transmitter is a circular loop of radius 400mm excited with a current of 0.1 A. The coil is made up of copper of radius 1mm. From fig. 1 we can clearly see the structure of the system is symmetry and we are interested in the direction of propagation (z-direction). So it is sufficientto choose a 2D axisymmetry model for simulation. The metamaterial considered is anisotropic and characterised by uniaxial permittivity and permeability tensors as shown in equation belowε 000ε 000ε(7a)μ000μ00μ(7b)The distance between the source and the receiver is at a distance of 500mm. The magnetic flux density between the source and the receiving point of interest without the metamaterial is computed analytically and found to be be 3.87 × 10-8 Tesla. This scenario is simulated using COMSOL and found to be 3.74 × 10-8 Tesla.B.Results and DiscussionsThe propagation region can be subdivided into near field and far-field. In the far field region the efficiency is related to absorption and scattering by the environment. Also at high power electromagnetic interference becomes a critical issue. In reality propagation can occur by a simple radiative process. The radiative efficiency is reduced by losses due to absorption and scattering by the environment. At high intensities we will also have to deal with electromagnetic interference. In the near field, E/H ratio can be made negligible, thereby not causing any harm to biological and other objects in the vicinity. This in turn becomes a trade-off between the environmental benefits versus distance and efficiency of propagation. The propagation efficiency can be increased by introducing metamaterial in the medium between the source and receiver [8, 12].Fig. 2 shown below gives the magnetic flux density with when the transmitter coil is excited at a frequency ofFig. 2. Magnetic flux density (Tesla) with and without theNIM10 MHz without and with the metamaterial. It is clearly seen from the figure that there is an increase in the magnetic flux density when the metamaterial is introduced. Y. Urzhumov et al., [8] have also observed enhancement of transmission of TM polarized wave ie. magnetic field normal to propagation direction. Fig. 3 shows the relation between the size of the metamaterial and magnetic flux density. As the size of the material is increased radially in steps of 10mm we get a peak at a specific dimension. The same is observed when the material size is varied axially as seen in fig. 3. Similarly the distance between the material and receiver, size of the material, anisotrophy characteristics places a major role in enhancing the flux density [12]. Fig. 4 shows the magnetic flux density measured when the degree of anisotrophy in the direction of propagation εrzz /μrzz are varied from -1 to 1 in steps of 0.1. From the graph we can conclude that the magnetic flux density is high when εrzz and μrzz are -1.Y. Urzhumov et al., [8] suggests that the transverse wave propogation properties of a metamaterial slab of thickness ‘d’ and anisotropy ‘αTE ‘ are indistinguishable due to the invariance of the Maxwells equation, with respect to the coordinate transformation. This simply states that the metamaterial slab thickness can be reduced without effective change in performance. This is possible if the magnetic loss tangent is kept constant while increasing the negative real part of ‘μ‘.Fig. 5 shows the magnetic flux density is almost same from dc to 20 MHz. This gives an impression that the static and the quasi static behaviour of the system is same. This system can be used for High frequency RFID system (13.56 MHz) for enhancing the range and act as a power soure for charging the wireless devices in the Near-field regime. G. Shvet et al. [13], state that, for a single split ring resonator, SSR, of a metamaterial, the effective permeability approximately isμeff = 1 - F ω2 / ω2 – ωM 2 - i ωЃ andЄeff = 1 - (ωp 2 – ωE 2)/ ω2 – ωE 2 + i ωЃ (8)where F is the fractional area of a unit cell occupied by the SRR, ωM is the magnetic resonance frequency, and Ѓ, is the resistive loss coefficient, ωp is the characteristic ‘plasma’ frequency and ωE is the cut-wire resonance frequency. The filling factor F is typically kept small to avoid strong interaction between adjacent unit cells. Developing a metamaterial may require finding a resonant structure that has adjacent electric and magnetic resonances.For a periodic structure the effective permeability will reduce to [14]μ eff = μo [ 1 - (0.47)2 / { 1 – (1.05)2 / f 2 GHz } ] (9)where Єeff = 1.8 Єo . Here it is possible to show that a complex NIM structure can be equivalent to a uniform medium. The effective (Єeff - μeff ) of the medium will provide the width of the band around resonance. Here the losses are ignored, but from practical point it has a major impact. At the resonance, a very strong current is circulated in the loops and generates a considerable conduction loss.00.20.40.60.811.2100200M a g n e t i c f l u x d e n s i t y (×10-7) , T e s l aSize of the block increased in steps of 10mm in axial/radial direction, mmaxial radial00.20.40.60.811.2-2-112M a g n e t i c f l u x d e n s i t y (×10-7), T e s l aDegree of anisotrophy1.061.081.11.121.141.16-10102030M a g n e t i c f l u x d e n s i t y (×10-7), T e s l aFrequency, MHzFig. 3. Variation of magnetic flux density with block size(increased in steps of 10mm in axial/radial direction). Fig. 4. Degree of anisotrophy in z-direction varied from -1 to 1Fig. 5: Magnetic flux density variation from dc to 20 MHzThese are the issues that have to be resolved for practical purposes.IV.CONCLUSIONThere is an enhancement in the magnetic flux density when a metamaterial is introduced in between the source and receiver. This enhancement depends on the size, shape and anisotropy characteristics of the material. The above result implies that the static and quasi-static behavior of the system are same. Thus, the metamaterial can be used in between the source and receiver to enhance the range, which in turn increase the efficiency. It is also possible to further enhance the range if the source can be replaced with a resonator instead of the circular coil [6, 7]. This has immense possibility of application in high power energy transfer like electric vehicle wireless charging. An experimental setup is being fabricated and the results will be discussed in future. We are also looking at micro and nano-array architecture for the negative refractive index material. Preliminary results have been discussed elsewhere [15].A CKNOWLEDGMENTAuthors would like to thank the chancellor of VIT University for providing the encouragement and facilities to perform this work.R EFERENCES[1] N. Tesla, Apparatus for transmitting electrical energy, US patentnumber 1,119,732, December 1914.[2] W.C. Brown, “The history of power transmission by radio waves,”IEEE Trans. Microwave Theory Tech., vol. 32, pp.1230-1242, Sept.1984.[3] James O. McSpadden, John C. Mankins, “Space solar powerprograms and Microwave Wireless Power Transmission Technology”, IEEE Microwave Magazine, pp. 46-57, Dec. 2002.[4] A. Esser, H.-C. Skudelny, ”A new approach to Power supplies forRobots”, IEEE Trans. Industry Appl. Vol. 27, pp. 872-875, Oct. 1991. [5] J. Hirai, T.-W. Kim, A. Kawamura, “Wireless Transmission of Powerand Information for Cableless Linear Motor Drive”, IEEE Trans.Power Electron. vol. 15, pp. 21-27, Jan. 2000.[6] Andre Kurs, Aristeidis Karalis, Robert Moffatt, J.D. Joannopoulos,Peter Fisher, Marin Soljacic, “Wireless Power Transfer via StronglyCoupled Magnetic Resonances”, Science vol. 317, pp. 83-86, July 2007.[7] Aristeidis Karalis, J.D. Joannopoulos, Marin Soljacic, “Efficientwireless non-radiative mid-range energy transfer,” Annals of Physics,vol. 323, pp. 34-48, 2008.[8] Y. Urzhumov, D. R. Smith , “Metamaterial-enhanced couplingbetween magnetic dipoles for efficient wireless power transfer,”Physical Review B, vol. 83, 205114-1 to 205114-10, 2011.[9] B. Wang, K.H. Teo, T. Nishino, W. Yerazunius, J. Barnwell, J.Zhang, “Wireless Power Transfer with Metamaterials,” EuropeanConference on Antennas and Propagation (EuCAP 2011), CP03.3 (Rome, Italy), April 11-15, 2011.[10] J B Pendry, “Negative Refraction Makes a Perfect Lens”, PhysicsReview Letters, vol. 85, pp. 3966 – 3969, 2000.[11] C. Caloz, T. Itoh, “Electromagnetic metamaterials: Transmission linetheory and microwave applications”, Wiley-Interscience, 2006.[12] G. Boopalan and C.K. Subramaniam, “Study of Radiation FieldEffects in the presence of metamaterials”, presented at 7thInternational Conference on Materials for Advanced Technologies, ICMAT 2013, Singapore, pp. 176, 30th June to 5th July 2013.[13] G. Shvets and Y. Urzhumov, “Negative index meta-materials basedon two-dimensional metallic structures”, J. Opt. A: Pure Appl. Opt., vol. 8, pp. S122–S130, 2006.[14] H. Mosallaei and K. Sarabandi, A Novel Resonant Metallo-DielectricStructure for Design of High Performance Є - μ Meta-Materials, IEEE Antennas and Propagation Soc. International Symp., pp. 316-319, 2005.[15] G. Boopalan and C.K. Subramaniam, “A simulation study on theproperty of micro-arrayed negative refractive index material for the energy transfer”, Technical Proceedings of the 2013 NSTI Nanotechnology conference and Expo, NSTI-Nanotech 2013, pp.536-539, 12th May to 16th May 2013.。