胚胎干细胞遗传分析的工具
细胞生物学名词解释
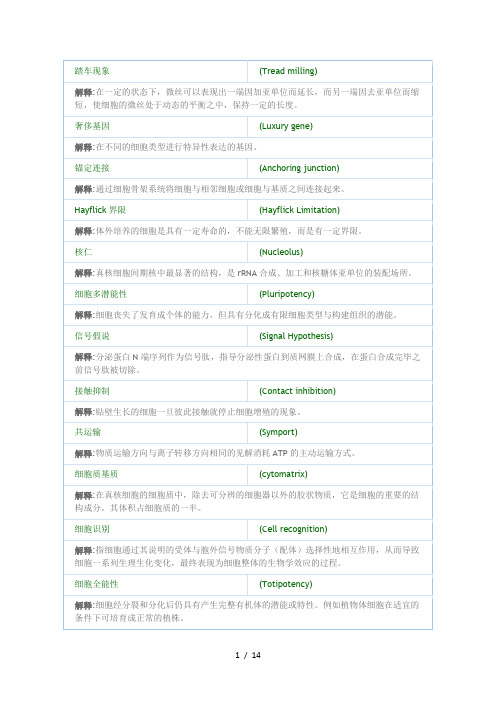
单克隆抗体
(Monoclonal Antibody)
解释:是单克隆的杂交瘤细胞群由亲本B细胞产生单一种类的抗体分子。这些单克隆抗体比来自血清的抗体具有特异性,产量高,易纯化,可长期保存的优点。
分子杂交
(Molecular Hybridization)
踏车现象
(Tread milling)
解释:在一定的状态下,微丝可以表现出一端因加亚单位而延长,而另一端因去亚单位而缩短,使细胞的微丝处于动态的平衡之中,保持一定的长度。
奢侈基因
(Luxury gene)
解释:在不同的细胞类型进行特异性表达的基因。
锚定连接
(Anchoring junction)
解释:通过细胞骨架系统将细胞与相邻细胞或细胞与基质之间连接起来。
细胞工程
(Cell Engineering)
解释:是细胞水平上的遗传工程,即应用细胞生物学和分子生物学方法对细胞进行遗传操作,如细胞培养,细胞融合,细胞诱变,细胞重构,遗传物质转移和生殖工程等。用来获得所期望的遗传组成的细胞或生物体。
整倍体
(Euploid)
解释:以单倍体数成倍增加的染色体数。
载体
(Vector)
生物采油
(OrganismOil-recovery)
解释:利用工程菌分解原油中的蜡来降低原油的黏度和提高油层的压力,从而增加石油的流出量。
器官培养
(Organ Culture)
解释:在体外维持器官、器官的一部分或器官原基的培养法。
受体细胞
(Receptor Cell)
解释:在基因工程中指承受重组体DNA并使目的基因得到复制或表达的细胞。
胚胎干细胞在干细胞研究中的应用
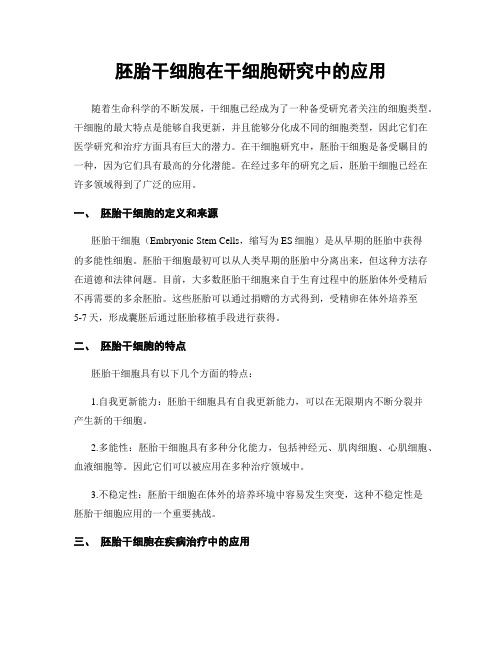
胚胎干细胞在干细胞研究中的应用随着生命科学的不断发展,干细胞已经成为了一种备受研究者关注的细胞类型。
干细胞的最大特点是能够自我更新,并且能够分化成不同的细胞类型,因此它们在医学研究和治疗方面具有巨大的潜力。
在干细胞研究中,胚胎干细胞是备受瞩目的一种,因为它们具有最高的分化潜能。
在经过多年的研究之后,胚胎干细胞已经在许多领域得到了广泛的应用。
一、胚胎干细胞的定义和来源胚胎干细胞(Embryonic Stem Cells,缩写为ES细胞)是从早期的胚胎中获得的多能性细胞。
胚胎干细胞最初可以从人类早期的胚胎中分离出来,但这种方法存在道德和法律问题。
目前,大多数胚胎干细胞来自于生育过程中的胚胎体外受精后不再需要的多余胚胎。
这些胚胎可以通过捐赠的方式得到,受精卵在体外培养至5-7天,形成囊胚后通过胚胎移植手段进行获得。
二、胚胎干细胞的特点胚胎干细胞具有以下几个方面的特点:1.自我更新能力:胚胎干细胞具有自我更新能力,可以在无限期内不断分裂并产生新的干细胞。
2.多能性:胚胎干细胞具有多种分化能力,包括神经元、肌肉细胞、心肌细胞、血液细胞等。
因此它们可以被应用在多种治疗领域中。
3.不稳定性:胚胎干细胞在体外的培养环境中容易发生突变,这种不稳定性是胚胎干细胞应用的一个重要挑战。
三、胚胎干细胞在疾病治疗中的应用1. 神经退行性疾病:神经退行性疾病是指中枢神经系统或外周神经系统中神经元死亡导致的各种疾病,如帕金森病。
胚胎干细胞可以通过分化成神经元来治疗这些疾病。
2. 心血管疾病:胚胎干细胞可以分化成具有心脏细胞功能的细胞,可用于心肌损伤的治疗。
3. 血液学疾病:胚胎干细胞可以分化成各种血细胞,包括红血球、白血球和血小板。
这些干细胞可以用于治疗血液学疾病,如再生障碍性贫血和白血病等。
四、胚胎干细胞在药物研究中的应用胚胎干细胞的应用不仅限于治疗领域,也可以应用在药物研究中。
在药物研究中,胚胎干细胞可以通过分化成具有药物靶标细胞的方式发挥作用。
遗传学——人胚胎干细胞表达生殖细胞分化相关基因的研究
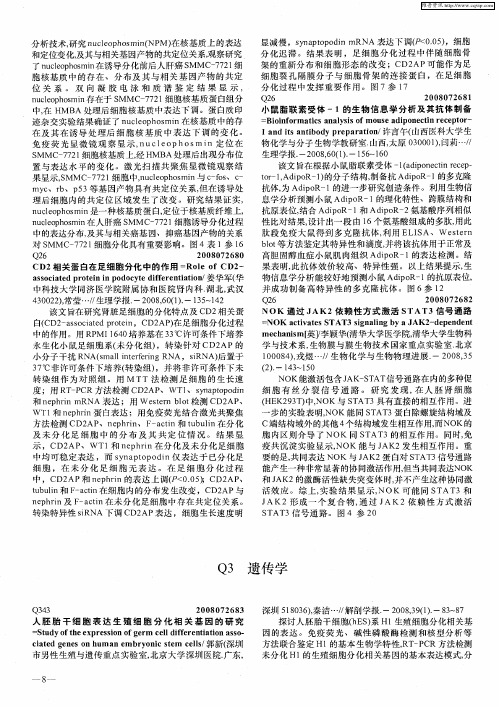
1 n s nio yp e aain 许言 午( 西医科 大 学 生 di t d rp rt / a ta b o 山 物化 学 与分 子 生物 学教 研 室 . 山西, 太原 0 0 0) 3 0 1, …/ 闫莉 / 生 理学报 . 0 8 01. 5~ 6 一2 0 , () 6 一16 10 该文 旨在根据小 鼠脂联索受体 一 (d o et cp 1 i nc nr e— ap i e
Q2 6 2 0 028 08 76 0
显 减慢 ,sn po o i mR A 表达 下 调( 0 5,细胞 y a tp dn N .) 0 分 化 迟 滞 。 结 果 表 明 ,足 细 胞 分 化 过程 中 伴 随 细 胞 骨 架 的重新分布 和细胞形态 的改变 ;C AP可能作为足 D2 细 胞 裂 孔 隔 膜 分 子 与 细 胞 骨 架 的连 接 蛋 白 ,在 足 细 胞 分 化 过 程 中 发挥 重 要 作 用 。 图 7参 1 7
性比对结果, 设计出一段 由 1 个氨基酸组成的多肽, 6 用此 肽段免疫大 鼠得到 多克隆抗体, 利用 E I A、We tr LS s n e bo等方法鉴定其特异性和滴度, l t p R 1 io 一 的表 达 检 测 。结 果 表 明, 抗体 效 价较 高 、特 异性 强 。 以上 结果 提 示 , 此 生 物信息学分析能较好地预测小鼠 A io ~ 的抗原表位, dp R 1
t 一 , io 一 ) o 1 pR 1的分子结构, r Ad 制备抗 A io 一 的多克隆 dp R 1 抗体, Ad o 一 的进一步研究创造条件 。利用 生物信 为 i R 1 p
息 学分 析 预 测 小 鼠 Adp R 1 io 一 的理 化 特性 、跨膜 结 构 和 抗原表位, 结合 A io 一 和 Ad o 一 dp R 1 i R 2氨基酸序列相似 p
生物学的研究工具(一)
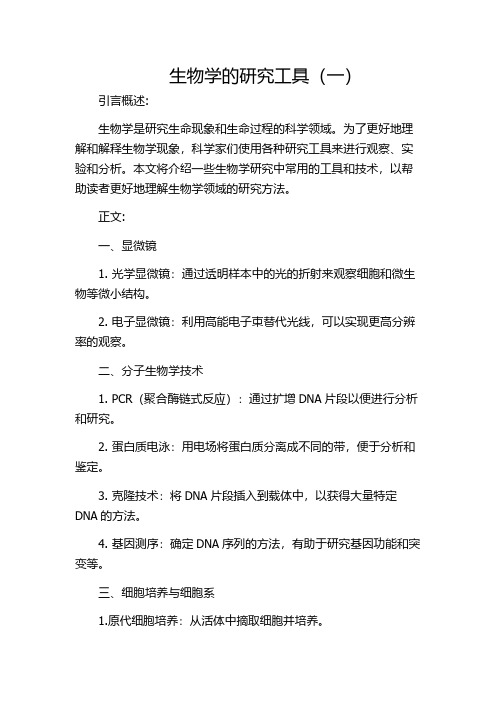
生物学的研究工具(一)引言概述:生物学是研究生命现象和生命过程的科学领域。
为了更好地理解和解释生物学现象,科学家们使用各种研究工具来进行观察、实验和分析。
本文将介绍一些生物学研究中常用的工具和技术,以帮助读者更好地理解生物学领域的研究方法。
正文:一、显微镜1. 光学显微镜:通过透明样本中的光的折射来观察细胞和微生物等微小结构。
2. 电子显微镜:利用高能电子束替代光线,可以实现更高分辨率的观察。
二、分子生物学技术1. PCR(聚合酶链式反应):通过扩增DNA片段以便进行分析和研究。
2. 蛋白质电泳:用电场将蛋白质分离成不同的带,便于分析和鉴定。
3. 克隆技术:将DNA片段插入到载体中,以获得大量特定DNA的方法。
4. 基因测序:确定DNA序列的方法,有助于研究基因功能和突变等。
三、细胞培养与细胞系1.原代细胞培养:从活体中摘取细胞并培养。
2. 细胞系:一种长时间培养的细胞株系,常用于体外实验和疾病模型研究。
四、动物实验模型1. 小鼠模型:常用于研究基因功能、药物治疗和疾病机制等。
2. 斑马鱼模型:有助于研究胚胎发育、遗传疾病和神经学等。
五、生物信息学1. 基因组学:研究整个基因组的结构和功能。
2. 蛋白质组学:研究特定物种或特定组织中蛋白质的组成和功能。
总结:生物学的研究工具涵盖了显微镜、分子生物学技术、细胞培养与细胞系、动物实验模型以及生物信息学等各方面。
这些工具和技术的应用使得生物学领域的研究更加丰富和深入。
随着科技的不断进步,生物学的研究工具也在不断发展和完善,为科学家们揭示生命奥秘提供了更大的可能性。
动物克隆 第三章胚胎干细胞分离培养
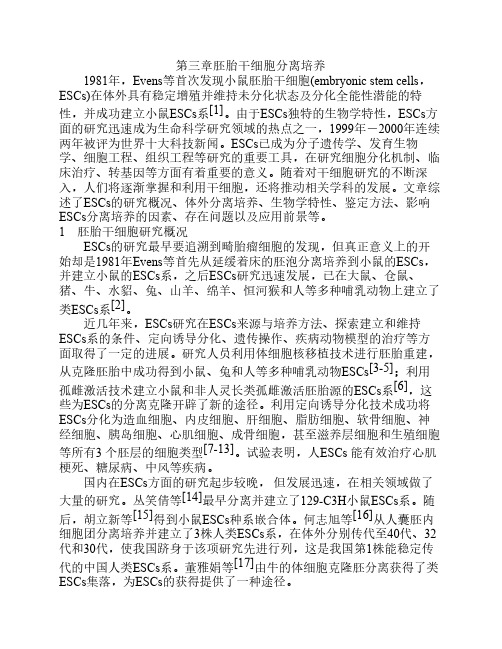
第三章胚胎干细胞分离培养1981年,Evens等首次发现小鼠胚胎干细胞(embryonic stem cells,ESCs)在体外具有稳定增殖并维持未分化状态及分化全能性潜能的特性,并成功建立小鼠ESCs系[1]。
由于ESCs独特的生物学特性,ESCs方面的研究迅速成为生命科学研究领域的热点之一,1999年-2000年连续两年被评为世界十大科技新闻。
ESCs已成为分子遗传学、发育生物学、细胞工程、组织工程等研究的重要工具,在研究细胞分化机制、临床治疗、转基因等方面有着重要的意义。
随着对干细胞研究的不断深入,人们将逐渐掌握和利用干细胞,还将推动相关学科的发展。
文章综述了ESCs的研究概况、体外分离培养、生物学特性、鉴定方法、影响ESCs分离培养的因素、存在问题以及应用前景等。
1 胚胎干细胞研究概况ESCs的研究最早要追溯到畸胎瘤细胞的发现,但真正意义上的开始却是1981年Evens等首先从延缓着床的胚泡分离培养到小鼠的ESCs,并建立小鼠的ESCs系,之后ESCs研究迅速发展,已在大鼠、仓鼠、猪、牛、水貂、兔、山羊、绵羊、恒河猴和人等多种哺乳动物上建立了类ESCs系[2]。
近几年来,ESCs研究在ESCs来源与培养方法、探索建立和维持ESCs系的条件、定向诱导分化、遗传操作、疾病动物模型的治疗等方面取得了一定的进展。
研究人员利用体细胞核移植技术进行胚胎重建,从克隆胚胎中成功得到小鼠、兔和人等多种哺乳动物ESCs[3-5];利用孤雌激活技术建立小鼠和非人灵长类孤雌激活胚胎源的ESCs系[6],这些为ESCs的分离克隆开辟了新的途径。
利用定向诱导分化技术成功将ESCs分化为造血细胞、内皮细胞、肝细胞、脂肪细胞、软骨细胞、神经细胞、胰岛细胞、心肌细胞、成骨细胞,甚至滋养层细胞和生殖细胞等所有3 个胚层的细胞类型[7-13]。
试验表明,人ESCs 能有效治疗心肌梗死、糖尿病、中风等疾病。
国内在ESCs方面的研究起步较晚,但发展迅速,在相关领域做了大量的研究。
胚胎干细胞的应用

胚胎干细胞的应用
胚胎干细胞是一种多功能干细胞,可以分化成多种组织类型,并
且在许多疾病中具有潜在的治疗效果。
以下是几个胚胎干细胞应用领域:
1. 疾病治疗:胚胎干细胞在再生医学和基因治疗方面的应用正
在迅速发展。
例如,研究人员正在探索用胚胎干细胞治疗多种遗传性
疾病的可能,包括肌营养不良症、先天性视网膜发育不全和脊髓性肌
萎缩症等。
2. 再生医学:胚胎干细胞可以分化成各种组织类型,包括皮肤、
神经、肌肉、骨骼和软骨等,因此被广泛用于再生医学领域,用于治疗肌肉损伤、皮肤疾病和骨骼疾病等。
3. 疾病预防:胚胎干细胞还可以用于预防某些遗传性疾病。
研究人员正在探索将胚胎干细胞注入人体胚胎中,以预防出生缺陷的方法。
4. 生物学研究:胚胎干细胞在生物多样性、基因组学和转录组学等领域具有广泛的应用价值。
通过研究胚胎干细胞的结构和功能,我
们可以更好地了解生命的基本原理和生物多样性。
胚胎干细胞具有广泛的应用前景,未来还有很多挑战和机遇需要
科学家们不断努力探索和研究。
小鼠胚胎干细胞的鉴定
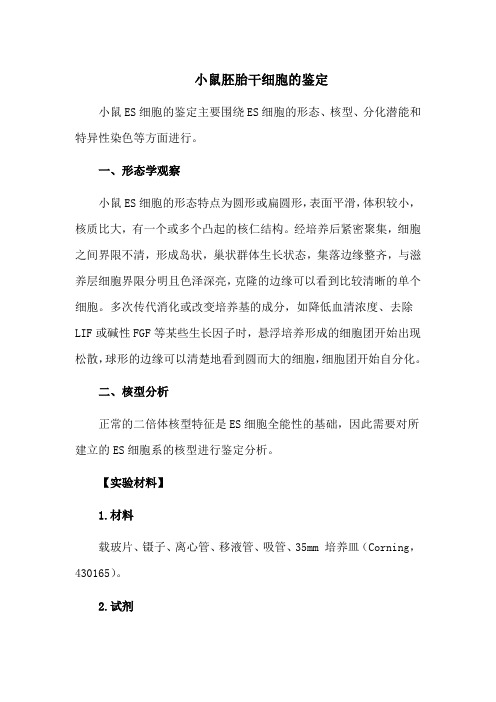
小鼠胚胎干细胞的鉴定小鼠ES细胞的鉴定主要围绕ES细胞的形态、核型、分化潜能和特异性染色等方面进行。
一、形态学观察小鼠ES细胞的形态特点为圆形或扁圆形,表面平滑,体积较小,核质比大,有一个或多个凸起的核仁结构。
经培养后紧密聚集,细胞之间界限不清,形成岛状,巢状群体生长状态,集落边缘整齐,与滋养层细胞界限分明且色泽深亮,克隆的边缘可以看到比较清晰的单个细胞。
多次传代消化或改变培养基的成分,如降低血清浓度、去除LIF或碱性FGF等某些生长因子时,悬浮培养形成的细胞团开始出现松散,球形的边缘可以清楚地看到圆而大的细胞,细胞团开始自分化。
二、核型分析正常的二倍体核型特征是ES细胞全能性的基础,因此需要对所建立的ES细胞系的核型进行鉴定分析。
【实验材料】1.材料载玻片、镊子、离心管、移液管、吸管、35mm 培养皿(Corning,430165)。
2.试剂Demecolcine(Sigma,D6165)、Giemsa(Sigma,G4507)、NaH2 PO4(Sigma,S6566)、Na2HPO4(Sigma,S5136)、甲醇、冰醋酸。
配制类试剂:①磷酸缓冲液:0.2mol/L NaH2PO4+0.2mol/L Na2 HPO4;②Giemsa染液:8ml磷酸缓冲液+2ml Giemsa贮存液,吹吸混匀;③固定液:甲醇∶冰醋酸=3∶1(现用现配)。
【实验步骤】1.将需要做核型分析的ES传代,通常用一个35mm皿的细胞做核型,在传代后25~40小时向培养皿中加入0.2µg/ml democolcin,继续培养1~2小时。
2.取出培养皿,用标准传代的消化方法将其消化成单细胞悬液,1200r/min离心10分钟,弃上清,注意:上清要吸得彻底些。
3.加入事先预热至37℃的0.56%KCl低渗液4ml,用滴管吹打成单细胞悬液(较剧烈),37℃低渗处理15~20分钟。
4.配10ml固定液,4℃冰箱预冷。
胚胎干细胞的鉴定方法

胚胎干细胞的鉴定方法
胚胎干细胞的鉴定方法通常包括以下几个方面:
1. 形态学特征:通过显微镜观察胚胎干细胞的形态,通常呈现出扁平、多边形、核大、核质比高的特点。
2. 表面标志物检测:使用流式细胞术或免疫荧光技术检测胚胎干细胞表面标志物的表达,如SSEA-1、SSEA-3、TRA-1-60 和TRA-1-81 等。
3. 多能性相关基因表达:通过RT-PCR、定量PCR 或基因芯片等技术检测胚胎干细胞中多能性相关基因的表达水平,如OCT4、SOX2、NANOG 和LIN28 等。
4. 分化能力检测:将胚胎干细胞诱导分化为特定的细胞类型,如神经元、心肌细胞等,并检测其分化能力和效率。
5. 核型分析:通过染色体核型分析技术,检测胚胎干细胞的染色体数目和结构是否正常。
6. 基因突变和遗传稳定性检测:使用基因测序或Southern 杂交等技术,检测胚胎干细胞是否存在基因突变和遗传稳定性问题。
- 1、下载文档前请自行甄别文档内容的完整性,平台不提供额外的编辑、内容补充、找答案等附加服务。
- 2、"仅部分预览"的文档,不可在线预览部分如存在完整性等问题,可反馈申请退款(可完整预览的文档不适用该条件!)。
- 3、如文档侵犯您的权益,请联系客服反馈,我们会尽快为您处理(人工客服工作时间:9:00-18:30)。
DOI 10.1007/s13238-012-2096-4 Protein & Cell Protein Cell 2012, 3(11): 806–810M INI-REVIEWHaploid embryonic stem cells: an ideal tool for mammalian genetic analysesLinyu Shi, Hui Yang, Jinsong LiGroup of Epigenetic Reprogramming, State Key Laboratory of Cell Biology, Institute of Biochemistry and Cell Biology, Chinese Academy of Sciences, Shanghai 200031, ChinaCorrespondence: jsli@Received September 3, 2010Accepted September 12, 2012ABSTRACTIdentification of the function of all genes in the mam-malian genome is critical in understanding basic mechanisms of biology. However, the diploidy of mammalian somatic cells has greatly hindered efforts to elucidate the gene function in numerous biological processes by mutagenesis-based genetic approaches. Recently, mouse haploid embryonic stem (haES) cells have been successfully isolated from parthenogenetic and androgenetic embryos, providing an ideal tool for genetic analyses. In these studies, mouse haES cells have already shown that they could be used in cell-based forward or reverse genetic screenings and in generating gene-targeting via homologous recombina-tion. In particular, haES cells from androgenetic em-bryos can be employed as novel, renewable form of fertilization agent for yielding live-born mice via injec-tion into oocytes, thus showing the possibility that ge-netic analysis can be extended from cellular level to organism level.KEYWORDS haploid embryonic stem cells, partheno-genetic embryos, androgenetic embryos, genetic screening, diploid, genomic imprintingINTRODUCTIONMost somatic cells in mammalian organisms are diploid, containing duplicate sets of chromosomes, a situation that is beneficial for their survival since if one copy of a gene is dis-rupted, the other copy can compensate for it. However, dip-loidy limits the identifying the function of a specific gene, be-haploid fungal spores of Saccharomyces cerevisiae have been largely utilized for mutational screens on a ge-nome-wide scale, as a recessive mutation should result in an unambiguous phenotype due to the absence of a second gene copy.In vertebrate, haploidy exists only in the postmeiotic germ-line, which is structurally specialized for fertilization. These cells cannot divide in vitro, thus preventing gene manipulation. Stable cultured haploid cell lines can be successfully derived from the embryos of some lower vertebrates, such as frogs (Freed and Mezger-Freed, 1970) and fish (Yi et al., 2009). Meanwhile, Zebrafish, as an important model organism for the study of early vertebrate development, its haploid em-bryos are amenable to insertional mutation and genetic screening (Wiellette et al., 2004). In mammalian organisms, the near-haploid human cell lines have been established from tumors (Kotecki et al., 1999; Sukov et al., 2010). Although these cells have been used for genetic screenings (Carette et al., 2009, 2011), their aneuploid karyotype and cancerous characteristics limits their application. Recently, three inde-pendent laboratories reported the isolation of mouse haES cells, with pilot genetic screenings and construction of a gene knockout (KO) mouse (Elling et al., 2011; Leeb and Wutz, 2011; Yang et al., 2012), representing an important break-through in the field.Here, we review recent progress in derivation of mouse haES cells, discuss their potential applications and outline future challenges.DERIVATION OF haES CELL LINESThere are two different kinds of haploid embryos according to their genetic origin, i.e., parthenogenetic and androgenetic806© Higher Education Press and Springer-Verlag Berlin Heidelberg 2012Haploid embryonic stem cells: an ideal tool for mammalian genetic analyses Protein & Cellniques (Paffoni et al., 2008), followed by the second polar body exclusion; the resulting embryos contain only one set of chromosome originated from oocyte. The other approach to make parthenogenetic haploid embryos is to remove the male pronucleus from the zygote. To generated androgenetic haploid embryos, nuclear transfer is employed to replace the chromosome-spindle-complex of a mature oocyte with a sperm head; the resulting embryos contain only one copy of paternal genome from sperm. The androgenetic haploid em-bryos can also be made by removal of the female pronucleus from the zygotes.The first mammalian ES cells were derived from mouse embryos more than thirty years ago (Evans and Kaufman, 1981). Shortly after that, the same group attempted to derive haES cells from mouse haploid embryos (Kaufman et al., 1983). They generated 4 ES cell lines, however, all of them displayed a diploid karyotype (Kaufman et al., 1983). These results indicated that haploid cells convert to diploid sponta-neously and haploidy might not be possible to be sustained in normal mammalian cells in vitro. Interestingly, haploid cells seem less compatible during differentiation and development, and haploid cell tends to become diploidy in vivo after trans-plantation of haploid embryos into pseudopregrant females (Ito et al., 1991; Kaufman, 1978; Latham et al., 2002).In 2011, two high-profile papers reported the generation of mouse haES cells from parthenogenetic embryos (Elling et al., 2011; Leeb and Wutz, 2011). Several months later, haES cells were generated from androgenetic embryos (Yang et al., 2012). These successes were made possible by haploid cell enrichment using fluorescence-activated cell sorting (FACS) (Elling et al., 2011; Leeb and Wutz, 2011). HaES cells showed a similar colony morphology as normal mouse diploid ES cells, expressed classical ES cell markers, and contrib-uted to various tissues (Elling et al., 2011; Leeb and Wutz, 2011) including the germline (Yang et al., 2012) upon injec-tion into diploid blastocysts. Furthermore, these cells sus-tained genome integrity and maintained stable haploidy in vitro by repeated cell sorting.APPLICATIONS OF haES CELLSThe motivation behind the generation of mammalian haES cells is to facilitate genetic studies. Recent reports (Elling et al., 2011; Leeb and Wutz, 2011; Yang et al., 2012) have shown preliminary applications of haES cells for genetic analyses without the limitation of normal diploid ES cells. Next we describe the applications of haES cells at both the cellular level and animal level (Fig. 1).Figure 1. The applications of haES cells. Mammalian haES cells show their great potential for genetic analyses. (A) At the cel-lular level, the haES cells have proven to be widely applicable in forward and reverse genetics; (B) the differentiated haploid cells could also be used for further genetic analyses. (C) At the organism level, AG-haES cells will be valuable tools in producing ge-netically modified animals for genome-wide screening or specific gene analysis.Protein & Cell Linyu Shi et al.At the cellular levelThe aim of forward and reverse genetic screenings is to dis-cover the function of genes. Forward genetic screening is an approach used to identify some of the genes responsible for the phenotype of interest, and reverse screening is used to analyze the phenotype by disrupting a specific gene.Transposon and retroviral insertional vectors were used to test the possibility of using haES cells for forward genetics (Elling et al., 2011; Leeb and Wutz, 2011). The authors adapted a similar loss-of-function screen strategy to select the relative drug-resistant ES cell clones. They identified some important genes after determining the insertion sites by PCR from ES cell clones selected. In addition, Elling et al. (2011) also described the application of mouse haES cells in reverse genetics. They designed an ingenious reversible gene trap vector for this purpose. The haES cells carrying the retroviral vector in the antisense orientation would not affect gene transcription, whereas transient Cre expression leading to convert the splice acceptor into the sense orientation would cause the truncation of the transcript. Thus, the phenotypic variation of the particular cell lines before and after Cre ex-pression can be observed.Furthermore, Yang et al. demonstrated that gene targeting via homologous recombination is feasible in haES cells, leading to successful generation of KO haES cells (Yang et al., 2012). Theoretically, KO haES cells could also be used for further gene screening by introduction of a cDNA library of expression plasmids or random insertional mutations, such as complementation screenings or synthetic lethal screenings (Grimm, 2004). Since mouse haES cells possess the ability to differentiate various cells of the three germ layers, an in-triguing application of haES cells is to screen the key genes involved in a specific cell fate determination during differen-tiation (Schimenti, 2011).At the organism levelAn important outcome of genetic screening analyses is the establishment of animal model, thus the function of a specific gene could be evaluated at organism level in vivo. Forward and reverse genetic screenings have been successfully ap-plied in parthenogenetic haES cells (Elling et al., 2011; Leeb and Wutz, 2011), however, the limitation of these haES cells is that successful conversion of the ES cells into live animals that would bear the ES cell-carried trait, has not been dem-onstrated (Elling et al., 2011; Leeb and Wutz, 2011). Yang et al. (2012) showed that AG-haES cells can be used as novel, renewable form of fertilization agent for yielding live-born mice (semicloned mice, SC mice) via injection into oocytes (intracytoplasmic AG-haES cell injection, ICAHCI), providing a new route to generate genetic-modified mouse model. They further obtained a F1 live-born pup after injection of the gene-targeted haES cells into oocytes and proved that this pup carried the targeted allele. Although this pup died shortly after birth, these results provide the proof of principle that genetically modified animals can be obtained via the ICAHCI technology using AG-haES cells that harbor a modified allele.One caveat of AG-haES cells is that all SC mice gener-ated via ICAHCI are females since the only the X chromo-some-bearing AG-haES cell lines can be generated, due to Y chromosome-bearing androgenetic embryos could not de-velop to the blastocyst stage (Latham et al., 2002, 2000). Nevertheless, there are still several advantages when AG-haES cells and ICAHCI technology are used for gener-ating genetic-modified mice. First, the biggest advantage of using AG-haES cells is the quick and reliable generation of F1 mice (SC mice). Therefore, it is not necessary to wait germline transmission from chimeras generated by traditional strategy using diploid ES cells, which is always the rate-limited step in both conventional and conditional gene knock-out experiments (Fig. 2). Second, for gene knock-in experiments, we can observe phenotype immediately in SC mice. In summary, the application of AG-haES cells will pro-vide a new and simple method in the production of genetic modified mice.An intriguing application of AG-haES cells is the genera-tion of gene-modified animals by injection them into oocyts in other species where conventional ES cells can be obtained but fail to support the production of chimeras, therefore can-not obtain gene-modified animals, such as the monkey (Tachibana et al., 2012). Moreover, for large animals, it is hard to have many chimeric animals and do lots of mating to get germline transmission by chance. In contrast, once a single F1 SC animal from AG-haES cells is obtained, it is certain to get a transmission into offspring with limited breeding as half of the progeny will inherit the genetic modi-fication.Another potential advantage is that the application of AG-haES cells is similar to artificial fertilization technologies, such as intracytoplasmic sperm injection (ICSI), which is commonly used in human. For human reproduction, although it is not clear now whether human AG-haES cells could be generated and they would be as good as sperm for the pur-poses of artificially fertilization, the ICAHCI technique might ultimately be used in assisted human reproduction for those affected by genetic disease.CHALLENGES OF haES CELLSMouse haES cells are amenable to efficient functional screening for genes involved in different biological processes; however, several technical hurdles need to be overcome in order to facilitate the potential applications of these cells.It is noteworthy that the mouse haES cells convert to dip-loid spontaneously in vivo and in vitro (Elling et al., 2011; Leeb and Wutz, 2011; Yang et al., 2012). The haploidy maintenance is dependent on periodic purification by cellHaploid embryonic stem cells: an ideal tool for mammalian genetic analyses Protein & CellFigure 2. The applications of AG-haES cells in the production of Knockout and Knock-in mice (provided by Bang-an Wang). There are several advantages of AG-haES cells and ICAHCI in making genetic modified mice, including: 1) quick and reli-able generation of F1 mice; 2) immediate phenotype in gene knock-in experiments.sorting every several passages. For this purpose, the cells are stained by nuclear dye, Hoechst 33342, and the cells with a haploid DNA content are sorted and enriched by exposure to ultraviolet (UV) light. However, this repeated UV light ex-posure may cause intracellular damage (Erba et al., 1988) and cell apoptosis (Zhang et al., 1999; Zhang and Kiechle, 1998). Furthermore, nuclear staining by Hoechst may impair the developmental competence of embryos (Li et al., 2004; Maside et al., 2011; Versieren et al., 2012). The intriguing questions are why and how haES cells become into diploid spontaneously; which genes or signaling are involved in this conversion; and whether it is possible to maintain haploidy in vitro without using FACS.Mouse haES cells are generated from parthenogenetic or androgenetic embryos, whose genetic materials are originated from maternal or paternal gametes, respectively. Meanwhile, since parental imprints established at the pri-mordial germ cell stage are propagated through fertilization and persist during embryonic development, it is interesting to examine parental imprinting state in these cells. As ex-pected, parental genomic imprints can be generally main-tained in AG-haES cells (Yang et al., 2012), while imprinting state is not mentioned in haES cells from parthenogeneitc embryos (Elling et al., 2011; Leeb and Wutz, 2011). The maintenance of normal imprints in AG-haES cells will likely determine that AG-haES cells can contribute to the germline after injection into diploid blastocyst (Yang et al., 2012) and can support the full-term development of embryos produced by injection into oocytes (Yang et al., 2012). Nevertheless, H19, a paternal imprinted gene, which should not be ex-pressed in sperm, thus not expressed in AG-haES cell, is upregulated in AG-haES cells (Yang et al., 2012). The loss of H19imprint in hAG-haES cells might account for the low birth rate of SC mouse and probably for growth-retarded fetuses by the following observations: 1) methylation varia-tion at the H19 imprinted gene is seen in AG-haES cells and the smaller SC pups born from ICAHCI are characterized by hypomethylation at H19; 2) growth-retarded pups were born at a higher frequency from ICAHCI using the cells of later passages that had severer loss of the H19methylation im-print; 3) the loss of H19 imprinting was observed in both KO AG-haES cells and the resulting retarded SC pup born after ICAHCI. Thus, a future task is to define the appropriate derivation and culture conditions that would enable the rela-tively stable maintenance of imprinting state in haploid ES cells.Protein & Cell Linyu Shi et al.CONCLUDING REMARKSThe generation of haES cells from mouse parthenogenetic and androgenetic embryos provides an ideal tool for genetic analyses. Remarkably, the sperm-like characteristics of AG-haES cells enable them artificially “fertilize” oocytes, making it easier to produce genetically modified mice. It is worthwhile to investigate how to stabilize the haploidy and imprinting state in mouse haES cells and whether haES cells could be derived from other species in future.ACKNOWLEDGEMENTSThis study was supported by grants from the Ministry of Science and Technology of China (No. 2009CB941101 to J.L.), and the ……Strategic Priority Research Program‟‟ of the Chinese Academy of Sciences (No. XDA01010403 to J.L.).REFERENCESCarette, J.E., Guimaraes, C.P., Varadarajan, M., Park, A.S., Wuethrich, I., Godarova, A., Kotecki, M., Cochran, B.H., Spooner, E., Ploegh, H.L., et al. (2009). Haploid genetic screens in human cells identify host factors used by pathogens.Science 326, 1231–1235.Carette, J.E., Guimaraes, C.P., Wuethrich, I., Blomen, V.A., Varadarajan, M., Sun, C., Bell, G., Yuan, B., Muellner, M.K., Nijman, S.M., et al. (2011). Global gene disruption in human cells to assign genes to phenotypes by deep sequencing. Nat Biotech 29, 542–546.Elling, U., Taubenschmid, J., Wirnsberger, G., O'Malley, R., Demers, S.P., Vanhaelen, Q., Shukalyuk, A.I., Schmauss, G., Schramek, D., Schnuetgen, F., et al. (2011). Forward and reverse genetics through derivation of haploid mouse embryonic stem cells. Cell Stem Cell 9, 563–574.Erba, E., Ubezio, P., Broggini, M., Ponti, M., and D'Incalci, M. (1988).DNA damage, cytotoxic effect and cell-cycle perturbation of Hoechst 33342 on L1210 cells in vitro. Cytometry 9, 1–6.Evans, M.J., and Kaufman, M.H. (1981). Establishment in culture of pluripotential cells from mouse embryos. Nature 292, 154–156. Freed, J.J., and Mezger-Freed, L. (1970). Stable haploid cultured cell lines from frog embryos. Proc Natl Acad Sci U S A 65, 337–344. Grimm, S. (2004). The art and design of genetic screens: mammalian culture cells. Nat Rev Genet 5, 179–189.Ito, M., Kaneko-Ishino, T., Ishino, F., Matsuhashi, M., Yokoyama, M., and Katsuki, M. (1991). Fate of haploid parthenogenetic cells in mouse chimeras during development. J Exp Zool 257, 178–183. Kaufman, M.H. (1978). Chromosome analysis of early postimplantation presumptive haploid parthenogenetic mouse embryos. J Embryol Exp Morphol 45, 85–91.Kaufman, M.H., Robertson, E.J., Handyside, A.H., and Evans, M.J.(1983). Establishment of pluripotential cell lines from haploid mouse embryos. J Embryol Exp Morphol 73, 249–261. Kotecki, M., Reddy, P.S., and Cochran, B.H. (1999). Isolation andcharacterization of a near-haploid human cell line. Exp Cell Res 252, 273–280.Latham, K.E., Akutsu, H., Patel, B., and Yanagimachi, R. (2002).Comparison of gene expression during preimplantation development between diploid and haploid mouse embryos. Biol Reprod 67, 386–392.Latham, K.E., Patel, B., Bautista, F.D., and Hawes, S.M. (2000).Effects of X chromosome number and parental origin on X-linked gene expression in preimplantation mouse embryos. Biol Reprod 63, 64–73.Leeb, M., and Wutz, A. (2011). Derivation of haploid embryonic stem cells from mouse embryos. Nature 479, 131–134.Li, G.P., White, K.L., and Bunch, T.D. (2004). Review of enucleation methods and procedures used in animal cloning: state of the art. Cloning Stem Cells 6, 5–13.Maside, C., Gil, M.A., Cuello, C., Sanchez-Osorio, J., Parrilla, I., Lucas, X., Caamano, J.N., Vazquez, J.M., Roca, J., and Martinez, E.A. (2011). Effects of Hoechst 33342 staining and ultraviolet irradiation on the developmental competence of in vitro-matured porcine oocytes. Theriogenology 76, 1667–1675. Paffoni, A., Brevini, T.A., Gandolfi, F., and Ragni, G. (2008).Parthenogenetic activation: biology and applications in the ART laboratory. Placenta 29 Suppl B, 121–125.Schimenti, J. (2011). Haploid embryonic stem cells and the dominance of recessive traits. Cell Stem Cell 9, 488–489. Sukov, W.R., Ketterling, R.P., Wei, S., Monaghan, K., Blunden, P., Mazzara, P., Raghavan, R., Oliviera, A.M., Wiktor, A.E., Keeney,G.L., et al. (2010). Nearly identical near-haploid karyotype in aperitoneal mesothelioma and a retroperitoneal malignant peripheral nerve sheath tumor. Cancer Genet Cytogenet 202, 123–128.Tachibana, M., Sparman, M., Ramsey, C., Ma, H., Lee, H.S., Penedo, M.C., and Mitalipov, S. (2012). Generation of chimeric rhesus monkeys. Cell 148, 285–295.Versieren, K., Heindryckx, B., Qian, C., Gerris, J., and De Sutter, P.(2012). Toxic effects of Hoechst staining and UV irradiation on preimplantation development of parthenogenetically activated mouse oocytes. Zygote, 1–9.Wiellette, E., Grinblat, Y., Austen, M., Hirsinger, E., Amsterdam, A., Walker, C., Westerfield, M., and Sive, H. (2004). Combined haploid and insertional mutation screen in the zebrafish.Genesis 40, 231–240.Yang, H., Shi, L., Wang, B.A., Liang, D., Zhong, C., Liu, W., Nie, Y., Liu, J., Zhao, J., Gao, X., et al. (2012). Generation of genetically modified mice by oocyte injection of androgenetic haploid embryonic stem cells. Cell 149, 605–617.Yi, M., Hong, N., and Hong, Y. (2009). Generation of medaka fish haploid embryonic stem cells. Science 326, 430–433.Zhang, X., Chen, J., Davis, B., and Kiechle, F. (1999). Hoechst 33342 induces apoptosis in HL-60 cells and inhibits topoisomerase I in vivo. Arch Pathol Lab Med 123, 921–927. Zhang, X., and Kiechle, F.L. (1998). Hoechst 33342 induces apoptosis and alters tata box binding protein/DNA complexes in nuclei from BC3H-1 myocytes. Biochem Biophys Res Commun 248, 18–21.。