The Optical Gravitational Lensing Experiment. BVI Maps of Dense Stellar Regions. I. The Sma
CXB4_U2_ADDITIONAL

2. Hubble Space Telescope
a space telescope carried into orbit by the Space Shuttle Discovery in April 1990
named after the American astronomer Edwin Hubble
5. American Astronomical Society
a US society of professional astronomers and other interested individuals, headquartered in Washington, DC
The basic objective of the AAS: to promote the advancement of astronomy and closely related branches of science
named after the open star cluster known in English as the Pleiades
the largest telescope in the world to use a single mirror as its primary mirror
4. Gravitational Lensing
one of the largest and most versatile space telescopes, and well-known as both a vital research tool and a public relations boon for astronomy.
the only telescope ever designed to be serviced in space by astronauts
一道80%的考生都会漏选的GRE阅读多选题

一道80%的考生都会漏选的GRE阅读多选题大家在做gre阅读的多选题的时候常常会有漏选的现象,下面小编就给大家实例讲解一下,告诉大家如何避免漏选的问题!一道80%的考生都会漏选的GRE阅读多选题阅读-正文Astronomers who study planet formation once believed that comets—because they remain mostly in the distant Oort cloud, where temperatures are close to absolute zero—must be pristine relics of the material that formed the outer planets.The conceptual shift away from seeing comets as pristine relics began in the 1970s, when laboratory simulations revealed there was sufficient ultraviolet radiation reaching comets to darken their surfaces and there were sufficient cosmic rays to alter chemical bonds or even molecular structure near the surface.Nevertheless, astronomers still believed that when a comet approached the Sun—where they could study it—the Sun’s intense heat would remove the corrupted surface layer, exposing the interior. About the same time, though, scientists realized comets might contain decaying radioactive isotopes that could have warmed cometary interiors to temperatures that caused the interiors to evolve.Consider each of the choices separately and select all that apply.Q:According to the passage, astronomers recognize which of the following as being liable to cause changes to comets?A. cosmic raysB. radioactive decayC. ultraviolet radiation易错点本题绝大部分同学都能通过定位到第二句,然后选出AC;然后他们会觉得B选项在第四句出现,属于非答案区间,所以不选。
Dark Matter
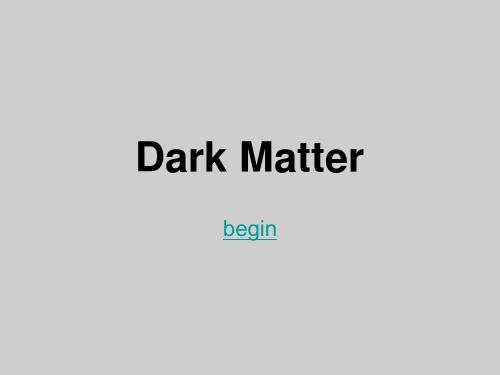
Mass / Energy Density
Scientists have measured the density of the universe by studying clusters of galaxies. We can call it either mass density or energy density. Einstein proved that mass and energy are the same with his equation E = mc2. He’s also the guy to blame for this curved space-time stuff. (learn more)
But . . .
But outer stars do not rotate correctly! If gravity causes galaxies to rotate, as we assume it does, then outer stars should behave much like the planets of our solar system. Inner planets rotate faster and outer planets rotate slower. This is called Keplerian motion. (learn more) In galaxies, however, both inner and outer stars rotate at about the same speed.
The Universe
Cosmologists study the birth and death of the universe. They also study its properties including its shape. Recent observation indicate that the universe is flat.
2022-2023学年江西省顶级名校高三下学期一模考试英语试题含解析

2023年高考英语模拟试卷注意事项:1.答卷前,考生务必将自己的姓名、准考证号填写在答题卡上。
2.回答选择题时,选出每小题答案后,用铅笔把答题卡上对应题目的答案标号涂黑,如需改动,用橡皮擦干净后,再选涂其它答案标号。
回答非选择题时,将答案写在答题卡上,写在本试卷上无效。
3.考试结束后,将本试卷和答题卡一并交回。
第一部分(共20小题,每小题1.5分,满分30分)1.I hope my teacher will take into _______ the fact that I was ill just before the exams when she marks my paper. A.idea B.considered C.account D.thought2.语音知识(共5小题;每小题l分,满分5分)从A、B、C、D四个选项中,找出其划线部分与所给单词的划线部分读音相同的选项。
并在答题卡上将该项涂黑。
3.Pandas are _____ to the mountains of central China and only about 1,000 remain in the wild.A. native B.sensitive C.relate D.familiar4.So absorbed ________ in her yoga exercises that she took no notice of the heavy rain outside.A.Mary was B.Mary has beenC.was Mary D.has Mary been5.______ property, we’re among the richest people in this city.A.In search of B.In spite of C.In place of D.In terms of6.We are to hold the sports meeting next weekend, ________ the air quality becomes better.A.which B.whenC.where D.while7.It is one thing to enjoy listening to good music, but it is quite ______ to perform skillfully yourself.A.another B.other C.the other D.others8.—What a shame! We misunderstood each other for such a long time.—Yes, I wish I _____ with you earlier.A.communicate B.had communicatedC.communicated D.would communicate9.During each NBA season, basketball fans cheer on their favorite teams to make _______ through.A.it B.themC.that D.those10.People tend to love agricultural products ________ without the use of fertilizers, pesticides or chemical additives. A.growing B.grownC.being grown D.having been grown11.It rained this morning, _____ actually didn’t bother me because I like walking in the rain.A.what B.whenC.where D.which12.The first snow didn’t fall until February in our province this year, ________ was unexpec ted.A.it B.which C.that D.what13.The disaster-stricken village was inaccessible ___________ by helicopter, and the storm added to the rescuers’ difficulty.A.instead of B.other than C.rather than D.regardless of14.—Did you enjoy your journey to Beijing last weekend?—. We had driven more than 3 hours before we found the right way.A.Absolutely B.No way C.Not at all D.With pleasure15.Regarding China-US differences on human rights issues, Hong said the two sides can enhance mutual understanding through dialogue ______ on equality and mutual respect.A.based B.to base C.basing D.base16.—Do you know when your mother ________ to pick you up?—At 11:40 am.A.had come B.is comingC.has come D.would come17.—Hi, Tom! I got a chance to be an exchange student in Harvard University.—_________! I had been expecting to study there.A.Lucky you B.Have funC.Take it easy D.Forget it18.The government officials met the workers and engineers working on the stadium, most____ were migrant workers.A.of which B.of who C.of whom D.of them19.—I’m sorry for breaking the cup.—Oh, ________. I’ve got plenty.A.help yourself B.forget itC.my pleasure D.pardon me20.—Got your driving license?—No. I too busy to have enough practice, so I didn’t take the driving test last week.A.was B.amC.have been D.had been第二部分阅读理解(满分40分)阅读下列短文,从每题所给的A、B、C、D四个选项中,选出最佳选项。
Weak Gravitational Lensing and its Cosmological Applications

2 4
4 5 7 8
Diagnostics of the lensing signal
Tests on simulated images . . . . . . . . . . . . . . . . . . . . . . . . . . . . . . . . . .
Cosmic shear and dark energy
20
20 21 23
The Future . . . . . . . . . . . . . . . . . . . . . . . . . . . . . . . . . . . . . . . .
Planned surveys . . . . . . . . . . . . . . . . . . . . . . . . . . . . . . . . . . . . . . . . Prospects for lensing cosmology . . . . . . . . . . . . . . . . . . . . . . . . . . . . . . .
2
Cosmology and Gravitational Lensing
3
is displaced from the bulk of the baryonic mass (1). Such measurements can provide important insights into the properties of dark matter. A number of applications of gravitational lensing have proven important for observational cosmology. When the lens is sufficiently strong, multiple images of the same source can be observed. If the source is variable, time delays between the variation of the images can be determined. An example of the applications of strong lensing is in the use of time delays to estimate the Hubble constant, provided a good model for the lens can be derived (see e.g. (2,3)). In this review, however, the focus will be on applications of weak gravitational lensing that can help us understand the properties of dark energy and dark matter on cosmological scales. Weak lensing refers to the shearing of distant galaxy images due to the differential deflection of neighboring light rays. The signal is small, typically inducing an ellipticity of order 1%. While this is negligible compared to the intrinsic shape of individual galaxies, it can be measured statistically using the coherence of the lensing shear over the sky. In the past two decades it has become possible to measure these subtle changes to study the distribution of dark matter in the universe. The first measurements used lensing by galaxy clusters; more recently cosmic shear measurements have been made in “blank fields”, without using any knowledge of foreground structures. A number of topics related to galaxy and cluster lensing are discussed in §5, but the main focus of this review is cosmic shear, i.e. lensing by large-scale structure in the universe. The reason for the recent popularity of cosmic shear is the fact that the signal is a direct measure of the projected matter power spectrum over a redshift range determined by the lensed sources (see e.g., (4, 5)). This straightforward interpretation of the signal is rather unique in the tools available for cosmology, and it potentially enables the determination of cosmological parameters with high precision. Lensing measurements are not only sensitive to the geometry (similar to distance measures such as type Ia supernovae or baryonic acoustic oscillations), but also provide measures of the growth of large-scale structure that test gravity on cosmological scales. These features make cosmic shear one of the most powerful probes of dark energy and modified gravity theories (6, 7), albeit an observationally challenging one. This review focuses on the methods and principal challenges in the cosmological applications of weak lensing. We review lensing theory but the focus is on measurements, current surveys and prospects for planned surveys in the coming decade. We refer the interested reader to reviews with a more detailed treatment of many other aspects of lensing (8, 9, 10, 11). In §2, we describe the key steps in the measurement and in §3 we discuss the interpretation of cosmological weak lensing. In §4 we review the primary systematic errors, as well as ways to deal with them. We highlight some of the current results in cosmological weak lensing in §5. In §6 we discuss lensing by galaxies and galaxy clusters. We conclude in §7 with a discussion of prospects
B1359+154 A Six Image Lens Produced by a z=1 Compact Group of Galaxies

a rXiv:as tr o-ph/1155v128Nov2B1359+154:A Six Image Lens Produced by a z ≃1Compact Group of Galaxies 1D.Rusin 2,C.S.Kochanek 3,M.Norbury 4E.E.Falco 3,C.D.Impey 5,J.Leh´a r 3,B.A.McLeod 3H.-W.Rix 6,C.R.Keeton 5,J.A.Mu˜n oz 7,C.Y.Peng 5ABSTRACT HST V and I-band observations show that the gravitational lens B1359+154consists of six images of a single z s =3.235radio source and its star-forming host galaxy,produced by a compact group of galaxies at z l ≃1.VLBA observations at 1.7GHz strongly support this conclusion,showing six compact cores with similar low-frequency radio spectra.B1359+154is the first example of galaxy-scale gravitational lensing in which more than four images are observed of the same background source.The configuration is due to the unique lensing mass distribution:three primary lens galaxies lying on the vertices of a triangle separated by 0.′′7≃4h −1kpc,inside the 1.′′7diameter Einstein ring defined by the radio images.The gravitational potential has additional extrema within this triangle,creating a pair of central images that supplement the “standard”four-image geometry of the outer components.Simple mass models consisting of three lens galaxies constrained by HST and VLBA astrometry naturally reproduce the observed image positions but must be finely-tuned to fit the flux densities.Subject headings:cosmology:gravitational lensing;galaxy groups1.IntroductionThe vast majority of the∼60known arcsecond-scale gravitational lens systems consist of two or four detectable images,consistent with the generic lensing properties of smooth,isolated and centrally steep mass distributions(e.g.Blandford&Kochanek1987).There are very few cases in which a“non-standard”number of images may have been observed.8APM08279+5255(Ibata et al.1999)and MG1131+0456(Chen&Hewitt1993)each contain a central component that could be an additional lensed image created by a sufficiently large galaxy core or shallow mass profile (Narasimha,Subramanian&Chitre1986;Rusin&Ma2000).Alternatively,APM08279+5255 may be a special class of imaging produced by an edge-on disk(Keeton&Kochanek1998), while the central component in MG1131+0456could be weak AGN emission associated with the lensing galaxy.In addition,MG2016+112(Lawrence et al.1984;Garrett et al.1996)exhibits a rather complicated image morphology consisting of four primary components,one of which has three subcomponents in VLBI maps.This may be a compound lens produced by two galaxies at different redshifts(Nair&Garrett1997).Benitez et al.(1999)suggest an alternative model,but it might not fully explain the VLBI observations.Complex interplay between mass distributions can lead to lens systems with more than four images of a source.For example,ellipsoidal mass distributions perturbed by shearfields may produce configurations with six or eight images arranged about the tangential critical curve (Keeton,Mao&Witt2000b).However,this requires that the relative magnitudes and orientations of the internal and external shear axes befinely tuned,and the resulting cross-sections are quite pound mass distributions have been shown to be far more efficient at producinga variety of complex geometries in whichfive or more images may be formed over a range of radii(Kochanek&Apostolakis1988).This has been observed in the cluster-lensing case,where substructure in the gravitational potential created by the resident galaxies can qualitatively alter the lensing properties that one would expect for a smooth halo mass distribution(e.g.Natarajan et al.1998;Meneghetti et al.2000).A dramatic example of this is CL0024+1654,which exhibits eight images of a single blue background galaxy(Kassiola,Kovner&Fort1992;Wallington, Kochanek&Koo1995;Colley,Tyson&Turner1996;Tyson,Kochanski&Dell’Antonio1998).Early-type galaxies preferentially participate in lensing(Kochanek et al.2000a),so a large fraction of lens galaxies should be members of small groups and clusters due to the morphology-density relation(Dressler1980;see also Keeton,Christlein&Zabludoff2000a). Indeed,many lens systems are known to be perturbed by nearby galaxies(e.g.B2319+051;Rusin et al.2000b),groups(e.g.PG1115+080;Schechter et al.1997),or clusters(e.g.QSO0957+561, Fischer et al.1997;RXJ0921+4529,Mu˜n oz et al.2000).A handful of systems are lensed by more than one primary galaxy(e.g.B1127+385,Koopmans et al.1999;B1608+656,Koopmans&Fassnacht1999),but in these cases standard image geometries are produced despite merged caustics.Several additional lenses are observed to have faint satellite galaxies as companions inside or near the Einstein radius(e.g.MG0414+0534,Schechter&Moore1993;B1030+074, Xanthopoulos et al.1998;B1152+199,Rusin et al.in preparation).While the likelihood offinding nearly equal mass galaxies close enough to have merged caustics is predicted to be only∼1% (Kochanek&Apostolakis1988),the probability offinding fainter satellites near the primary lens is not small because galaxy luminosity functions diverge for faint galaxies–all lenses should have faint neighbors,as discussed in the Appendix.While such systems typically produce regions of the source plane in which more than four images can form,the small size of the companion galaxies means that the cross-sections for creating non-standard image geometries are not significant.The gravitational lens system B1359+154(Myers et al.1999),discovered in the Cosmic Lens All-Sky Survey(CLASS;e.g.Myers et al.1995),has been suspected of containing more than four lensed images.Observations with the Very Large Array(VLA)and Multi-Element Radio-Linked Interferometer Network(MERLIN)show a total of six radio components(Myers et al.1999;Rusin et al.2000a).Four of these components(A–D)are arranged in a typical quad-lens configuration (maximum image separation of1.′′7),with two additional components(E and F)residing within the ring defined by the outer images.Preliminary radio spectral studies of B1359+154at high frequency(Myers et al.1999)suggested that E had a slightlyflatter spectrum than A–D,and therefore that the central components may be core-jet emission associated with a weak AGN in the lensing galaxy or galaxies,as in the case of B2045+265(Fassnacht et al.1999).When the spectra were extended down to5GHz,however,there appeared to be less disparity among the radio components(Rusin et al.2000a).Subsequent VLA observations at15GHz have failedto decisively confirm that E isflatter than A–D at high-frequency,or detect component F. Spectroscopy with the Keck II telescope determined the source redshift to be z s=3.235(Myers et al.1999).Adaptive optics observations of B1359+154conducted with the Canada-France Hawaii Telescope(CFHT)in the infrared K′band(Rusin et al.2000a)detected counterparts to radio components A–D,and discovered three extended emission peaks(K1–K3)bracketing the expected positions of E and F.K1–K3were identified as three possible lensing galaxies,comprising the core of a compact galaxy group.Evidence of an arc connecting A,B and C was also observed,along with a weaker emission feature associated with component E.The compound deflector system not only explained why attempts to model the outer four components using a single galaxy had failed (Myers et al.1999),but offered the means of creating additional extrema in the lensing potential. This opened the possibility that at least one of the central components is a lensed image.In this paper we present powerful new evidence from observations with the Hubble Space Telescope(HST)and Very Long Baseline Array(VLBA)that B1359+154consists of six images of a single background source,lensed by a compact group of galaxies at z l≃1.In§2we present VLBA observations of B1359+154and investigate the low-frequency radio spectra of the components.In§3we discuss and analyze HST V and I-band observations,which offer compelling evidence for the six-image hypothesis.In§4we use preliminary mass modeling to demonstrate thatB1359+154can be naturally explained as a true six-image lens system.Finally,in§5we discuss the prospects for obtaining improved constraints on the lensing mass distribution of B1359+154, and ultimately using the system to study the structure of small galaxy groups at high redshift.2.VLBA Imaging of B1359+154VLBA observations of B1359+154were obtained at1.7GHz on1999December10for an on-source integration time of2hr,and again on2000Aug28for8hr.These observations were performed by iterating between the target source for3min and the nearby phase-reference calibrator B1413+150for1.5min.The data for each of the two epochs were calibrated separately within the AIPS data reduction package,then mapped and model-fit using DIFMAP.Theflux densities of the model-fit components exhibited little variation(∼3%)between epochs,so the two data sets were combined to improve the signal-to-noise.The resulting naturally-weighted map of B1359+154is presented in Fig.1,and has an rms noise level of45µJy/beam.All six radio components seen in previous VLA and MERLIN observations are easily detected by the VLBA, and maps of the individual components are shown in ponents A,B,and C each exhibit a compact core with associated jet emission.Lensing-induced parity reversal is evident in the relative orientations of the ponents D,E and F are each unresolved.The data were modeled within DIFMAP using a total of9gaussian components(Table1):6compact cores(A1–F1),1extended jet(A2)and2compact subcomponents(B2,C2).The compact nature of radio components E and F strongly argue for their identification as additional lensed images,rather than weak core-jet emission associated with the lensing mass (Myers et al.1999).First,it would be rare for jet emission to remain unresolved at∼10mas resolution.All but one pair of radio components shown to be similarly compact in CLASS VLBA follow-up observations is a pair of lensed images,the only exception being the binary quasarB0827+525(Koopmans et al.2000).There is also no evidence in the VLBA data to suggest that any emission bridge might be connecting E and F.Second,it is unlikely that both E and F could mark the cores of independent AGN within the deflector.One would expect the positions of AGN to be correlated with brightness peaks of the lensing galaxies,and this is not the case in the CFHT data.Third,the structure of all six radio components are morphologically consistent with a single background source.Specifically,if the source consists of a core and weak jet,it is likely that extended emission would be visible in the more magnified lensed images while the fainter images show only the compact core.This is exactly what is observed in B1359+154:A–C are bright and exhibit weak subcomponents;D–F are faint and share identical unresolved morphologies.Next we construct low-frequency radio spectra of the components by combining the1.7GHz VLBAflux densities with those previously obtained at5GHz using MERLIN(Rusin et al.2000a) and at8.5GHz using the VLA(Myers et al.1999).One need not be concerned about combining data from different epochs as there is little evidence for any significant variability in the lensed source.The resulting radio spectra are plotted in Fig.3and exhibit striking similarities.Each ofAFEBDCFig.1.—VLBA1.7GHz naturally-weighted map of B1359+154.The data is restored with a beam of size13.9×12.5mas at PA=−3.34◦.The map rms noise level is45µJy/beam.Fig. 2.—VLBA1.7GHz naturally-weighted maps of B1359+154components.Top:A,B,C. Bottom:D,E,F.The beam is9.88×5.54mas at PA=1.11◦.Each box size is250×250mas. Contours are at3,6,9...×the map rms.ACBEDFFig.3.—Radio spectra of B1359+154components.Data from the1.7GHz VLBA,5GHz MERLIN (Rusin et al.2000a)and8.5GHz VLA(Myers et al.1999)observations.We assumeflux density uncertainties of5%to account for source variability and model-fitting errors.the six components has a spectrum that is ratherflat(α51.7≃−0.3,where Sν∝να)between1.7 and5GHz,then falls offsharply(α158.5≃−1.2)between5and8.5GHz.The1.7–5GHz spectral indices for all six components(Table1)agree within measurement errors.Even if the sharpness of the5GHz spectral break were somehow exacerbated by variability,it would still require that all six components vary in the same manner–something that would be impossible unless E and F are related to A–D.Therefore,the morphologies and low-frequency spectra of the B1359+154 radio components strongly suggest that they are six images of a single background source.3.HST Imaging of B1359+154Using the WFPC2instrument on HST we obtained images of B1359+154in the F555W (“V”,5200sec)and F814W(“I”,5000sec)bands on2000July10.Each observation was divided into four subexposures,at two dither positions,which were used to remove cosmic rays and bad pixels.Fig.4shows the resulting images.Optical counterparts to all six radio components are observed,along with extended emission from the three galaxies.The images were modeled asa combination of point sources and de Vaucouleurs models following the modeling proceduresof Leh´a r et al.(2000)for CASTLES observations of other lenses.Astrometric and photometric results are presented in Table2.The positions of images A–F derived from the HST data match the radio positions to an accuracy of0.′′02,consistent with our internal error estimates.All six optical counterparts of the radio components are blue,with an average color ofV–I=0.5mag and a dispersion in the colors of only0.1mag.Theflux ratios of the6radio components and their optical counterparts differ by less than a factor of two between1.7GHz and the V-band,so they must be six lensed images.Three of the counterparts(A,B and C)are clearly extended in both the V and I-band images,consistent with the presence of an Einstein ring in the Rusin et al.(2000a)infrared image.The rest frame wavelengths of the V and I-bandfilters at the source redshift of z s=3.235are1300˚A and1900˚A,respectively,so the host galaxy of the radio source must have a strong ultraviolet continuum to produce the arcs.While we lack images in bluerfilters,and the Myers et al.(1999)spectrum of the source is contaminated by AGN activity, the host galaxy appears to be a star-forming Ly-break galaxy.The emission lines from the source are relatively narrow(Lyαand N V are well resolved)and lines characteristic of both AGN(Civ) and star formation(Ciii])are seen in the spectrum.The optical counterparts(G,G′,G′′)of the three infrared sources K1–K3are extended and red.We have relabeled the sources using the standard CASTLES convention in which G is the most luminous lens and G′/G′′are of lower luminosity.All three are seen in the I-band image and essentially vanish in the V-band image,with a color of V–I>∼3mag.They are clearly three distinct galaxies which are well modeled by de Vaucouleurs profiles,rather than compact star-forming regions in a larger galaxy.With separations of only4h−1kpc(for z l≃1.0in anΩ0=0.3flat cosmological model),they are likely to be the core of a compact group.Unlike other lenses with secondary lens galaxies,the three galaxies have similar luminosities.Redshift estimates based onI=F814W G G''G'F E D C BA V=F555W G G''G'F E D CB A ResidualsG G''G'F EDCB A Residuals G G''G'F E DC BA Fig. 4.—HST I-band (left)and V-band (right)images of B1359+154.The top panels show the final images with the components labeled as in Table 2.The bottom panels show the residuals after subtracting counterparts to the six radio cores and the three lens galaxies.Crosses mark the centers of the subtracted components.their optical colors and the mass estimates derived from our models of the system(§4)and the fundamental plane(Kochanek et al.2000a)are mutually consistent.Wefind z l=1.35±0.16, 0.88±0.06and0.94±0.07for galaxies G,G′,and G′′,respectively.The average redshift isz l=1.05±0.20and the weighted average is z l=0.94±0.04,but for simplicity we will just assume z l=1for future estimates.The scatter of the individual estimates is consistent with the scatter found by Kochanek et al.(2000a)for lenses with known redshifts.Such a group of galaxies is unlikely to be a projection effect given the separations and the size of the overall lens sample. Based on the results in the Appendix we estimate that the a posteriori probability offinding such a chance projection in a sample of60lenses is∼10−3.We conclude that the lens is the coreof a compact galaxy group(see Hickson1997for a review of compact groups).With three lens galaxies of comparable luminosity and redshift inside their joint Einstein ring,the gravitational potential should have additional extrema inside the triangle formed by the galaxies.While images A–D resemble a standard four-image lens morphology,two additional images(E and F)lie in the region between the three primary lenses.Therefore,HST observations offer powerful evidence that B1359+154is thefirst example of a true six-image galaxy-scale gravitational lens system.We also cataloged the nearby galaxies in the HST images using the SExtractor package(Bertin &Arnouts1996).The software classified the optical objects,and computed total magnitudes using Kron-type automatic apertures(see Leh´a r et al.2000).Colors were computed usingfixed apertures scaled to the F814W size of each object.Table3lists all the galaxies detected by HST within20′′of the lens,after visually confirming the SExtractor classification.The galaxies were labeled G#in order of decreasing I-band brightness,or K#for the galaxies found in the Rusin et al.(2000a)infrared observations which were not detected in the I-band image.Table3also includes an estimate of the shear perturbation each neighboring galaxy can produce on the lens assuming they lie at the same redshift and have the same mass-to-light ratios(see Leh´a r et al. 2000).The sum of the shear contributions isγ=0.074atθγ=83◦,although the largest source of shear,galaxy G1,is probably a foreground galaxy.The contribution of all the other galaxies is γ=0.049atθγ=−60◦.4.Preliminary Mass ModelsConstructing any believable model for the lensing mass of B1359+154is a significant challenge.At a minimum the model must include mass distributions to represent each of the three lensing galaxies plus environmental contributions to the lensing potential,either in the form of external shear or a separate group halo.Since even the simplest models require a large number of free parameters,the best hope for producing a realistic mass model may ultimately rely on constraints from deep HST imaging of the extended arc emission(Kochanek,Keeton&McLeod 2000b).For now our primary objective is to demonstrate that a six-image lens system can be naturally produced by the observed lensing galaxies.All models are constrained with12coordinates and5flux density ratios of the lensed radiocomponents,as derived from the VLBA data listed in Table1.Only the core components A1–F1 are used at this time.We set afit tolerance of1mas for the image positions and assume a10% uncertainty on theflux densities.The galaxy positions are constrained according to the astrometric error bars given in Table2.The goodness-of-fit parameter is thereforeχ2tot=χ2pos+χ2flx+χ2gal, which includes contributions to thefit from the positions of the radio components,(χ2pos),their flux(χ2flx),and the positions of the lens galaxies(χ2gal).The redshift of the group is set at z l=1, and aflat cosmology withΩo=0.3is assumed for all calculations.The models are optimized using the lensmodel software package(Keeton2000).94.1.A Demonstration of PrincipleSmooth gravitational potentials must produce an odd number of lensed images(Burke1981). However,images can be trapped and demagnified in the singular or near-singular cores of lensing galaxies(Narasimha et al.1986).We can label the possible image configurations by A/B,where A is the true number of images and B is the observed number of images.Since A is always odd,B1359+154must be either a7/6or9/6system.A single lens can generate a7/6image configuration,but it should consist of6images near the Einstein radius of the system(see Keeton et al.2000b).The central location of images E and F and the comparable luminosities of the three galaxies(1.0:0.4:0.4for G:G′:G′′)strongly suggest that all three lenses are important and that we are looking at a9/6lens system.We begin by considering a compound mass distribution consisting of three singular isothermal spheres(SIS)in an external shearfield.This model is unlikely tofit the data very well as it ignores galaxy ellipticity,but we use it to demonstrate the principles of compound deflectors in the context of B1359+154.From the expected caustic structure alone one can argue that9/6 systems are possible.Each SIS deflector has an associated radial caustic on the source plane,at which an inward-crossing source produces a pair of images at the corresponding galaxy center:one observable and one trapped in the core.The asymmetry of the potential will also lead to at least one tangential caustic,at which an inward-crossing source produces a pair of observable images at the corresponding critical curve.Therefore,a9/6system is formed when a source sits within one tangential and three radial caustics.Because three positive parity images will be trappedin the galaxy cores,four of the observed images must have negative parity(saddle points in the time delay surface)to ensure that the parities of all nine images sum to1(Burke1981;Blandford &Narayan1986).Images A–D are arranged in a typical quad configuration,so we expect their parities to alternate in the usual way:A(+),B(–),C(+),D(–).This means that both E and F must correspond to saddle points(–),and we model the system under this assumption.The critical curves,caustics and time delay surface for the best-fit3SIS model are plotted inFig.5.Because the separation of the galaxies is small compared to their Einstein radii,a single tangential critical curve encloses all three galaxies(Fig.5a).This maps to the highly distorted tangential(astroid)caustic on the source plane(Fig.5b).The large circular features in Fig.5b correspond to the radial caustics associated with each SIS.These not only significantly overlap each other,but also overlap much of the astroid caustic creating a substantial9/6region in which the source resides.The cross-section of the model is dominated by3/2,5/3,7/5and9/6 geometries,but it can also produce7/4and11/8configurations.The11/8region is due to the tangential caustic folding over on itself and creating a swallowtail catastrophe.The probability of observing7/4,7/5,9/6and11/8systems will be greatly enhanced through magnification bias. Despite the complexity of the caustic structure,the topology of the virtual time delay surface (Fig.5c)is quite generic(Schneider1985;Blandford&Narayan1986).Images A–D have the standard topology for four-image lenses.Images A–C are associated with a lemniscate critical contour encompassing two minima(A and C)and the saddle point(B).Image D is a saddle point associated with a lima¸c on contour which would usually encompass images A–C in the large loop and the core of the lens galaxy in the smaller loop.In this case,however,the smaller loop contains all three lens galaxies.Images E and F lie at the saddle points of two new lemniscate critical contours with maximum-saddle-maximum topologies,where the maxima sit on the galaxy cores.The3SIS model has a best-fit ofχ2=1570for NDF=10,and its parameters are listed in Table4.The model poorlyfits the positions of images A(χ2pos,A=323),B(χ2pos,B=659)and F (χ2pos,F=299).Theflux density of image A is also significantly lower than observed(predicted2.4 mJy,χ2flx,A=80).Given the simplicity of the model,the errors in the image positions are of little concern and should be greatly improved by adding ellipticity or adjusting the radial mass profiles of the galaxies.The mismatch of theflux densities is a more generic ponents B and C are clearly a merging pair of images associated with the tangential critical curve,and in most lens systems would be the brightest images.To reproduce the observedflux ordering inB1359+154,the critical curve must pass closer to A than either B or C,so that A will be the most magnified.10The3SIS model is unable to accomplish this,as A sits quite far from the tangential critical curve(Fig.5a).Accounting for the relativefluxes will therefore require models with more free parameters.4.2.More Realistic ModelsWe now attempt to improve thefits to B1359+154by investigating more realistic lens models. The mass distributions of lensing galaxies are ellipsoidal rather than spherical,so we consider models with3singular isothermal ellipsoids(SIE)in an external shearfield.The SIE is described3/23/23/25/39/65/37/5(b)Fig.5.—Best-fit 3SIS model.(a)Critical curves.Observed (model-predicted)image and galaxy positions are marked as crosses (squares).Note that A is far from the tangential critical curve,and thus cannot be highly magnified in this model.(b)Caustic curves.Prominent imaging regions are marked as A/B,where A is the total number of images and B is the observable number of images.The source sits at the intersection of the dotted lines,near a swallowtail catastrophe.(c)Contours of constant time delay,in steps of 3h −1days outward from A (thin lines),except for the critical contours (bold lines)passing through the saddle points in this surface (B,D,E and F).Images trapped in the galaxy cores correspond to local maxima.by the scaled surface mass densityκ(x,y)=b/2[(1−ǫ)x2+(1+ǫ)y2]1/2−b/2(b)3/23/23/25/39/67/45/3Fig.6.—Best-fit 3SIE model.Plots are the same as in Fig.5.Note that the critical curve (a)passes closer to A than either B or C,ensuring that A is the brightest image.The high magnification of A is due to the position of the unlensed source,which lies just outside a swallowtail cusp (b).We now turn to the truncated mass distributions.The3PJS model has afit statistic ofχ2tot=538,a significant improvement over the3SIS model but with the same number of free parameters(NDF=4).Theflux density of A is11.2mJy compared to the observed value of22.6 mJy,but overall theflux densities arefit rather well(χ2flx=46).Thefit statistic is dominated by offsets from the galaxy positions(χ2gal=290).The3PJE/FIX model also does better than its SIE counterpart,offering afit ofχ2tot=100for NDF=7.This is dominated by thefit to theflux density of A(predicted8.8mJy,χ2flx,A=37).The3PJE model offers a best-fit ofχ2tot=65,but again,A is not the brightest image(predicted11.3mJy,χ2flx,A=25).It is interesting to note that while the3SIE model more accurately reproduces theflux density of A,the predicted galaxy position angles in the3PJE model(69◦,18◦,62◦for G,G′and G′′,respectively)are much closer to those of the observed surface brightness distributions(48◦,29◦,63◦,respectively).However, the external shears in the3PJE and3PJE/FIX models(γ≃0.25)are significantly larger than those in the3SIE and3SIE/FIX models(γ≃0.15).To test whether the large external shears predicted by each of our lens models may be due to a dark matter halo associated with the galaxy group,we also investigated models with four distinct mass distributions.The external shear was set to zero in these trials.Although one might expect the three primary lenses to define the group center,we were unable tofind reasonable models in which the group halo resides within the Einstein ring.Problems arise because a fourth mass distribution that is even moderately concentrated significantly disturbs the caustic structure of the system,unless it is centered on one of the lensing galaxies.This makes it very difficult to account for the image properties without creating additional lensed components.Not surprisingly, wefind that in the vast majority of our trials the group halo is pushed out of the Einstein ring toward the east,where it becomes a large external shear contributor.We can check whether the mass ratios of the galaxies,as estimated from their lens model critical radii b,are consistent with the observed luminosity ratios.In standard lens models and observations of lenses,b∝L1/2(see Keeton,Kochanek&Falco1998),so we predict critical radius ratios of1.0:0.62±0.2:0.63±0.2for G:G′:G′′based on the I-band luminosities in Table2.The model ratios–1.0:0.59:0.86(3SIS),1.0:0.62:0.75(3SIE/FIX),1.0:0.80:0.82(3SIE),1.0:0.85:0.55 (3PJS),1.0:0.68:0.60(3PJE/FIX),and1.0:0.57:0.68(3PJE)–are remarkably consistent with these predictions.This provides additional evidence that all three lensing galaxies are important to the model and indicates that the B-band(for z l≃1)mass-to-light ratios of the three lenses must be very similar.The compatible galaxy colors and the consistency of our fundamental plane redshift estimates further reinforce this argument.5.DiscussionHST and VLBA observations demonstrate that the gravitational lens B1359+154consists of six images of a single background radio source.This is thefirst example of a galaxy-scale lens system with more than four images.The unique configuration is produced by the complex。
2018版高考一轮总复习英语模拟演练:1-5-1a5Unit1 Greatscientists含解析
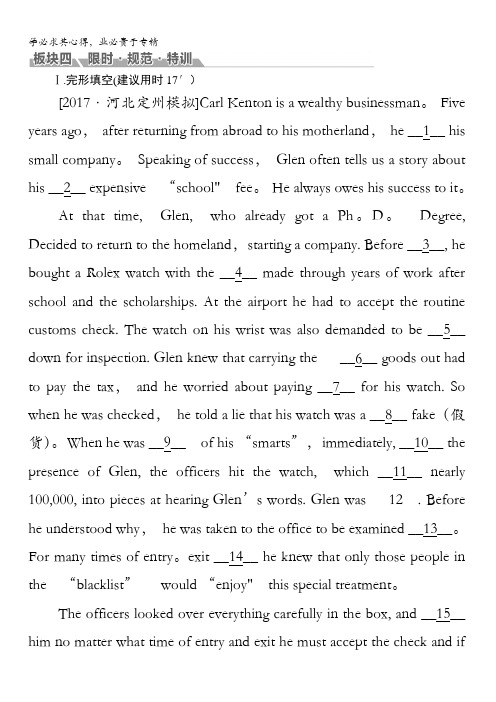
Ⅰ.完形填空(建议用时17′)[2017·河北定州模拟]Carl Kenton is a wealthy businessman。
Five years ago,after returning from abroad to his motherland,he __1__ his small company。
Speaking of success,Glen often tells us a story about his __2__ expensive “school" fee。
He always owes his success to it。
At that time, Glen, who already got a Ph。
D。
Degree, Decided to return to the homeland,starting a company. Before __3__, he bought a Rolex watch with the __4__ made through years of work after school and the scholarships. At the airport he had to accept the routine customs check. The watch on his wrist was also demanded to be __5__ down for inspection. Glen knew that carrying the __6__ goods out had to pay the tax,and he worried about paying __7__ for his watch. So when he was checked,he told a lie that his watch was a __8__ fake(假货)。
光学:阿瑟·阿什金人物简介
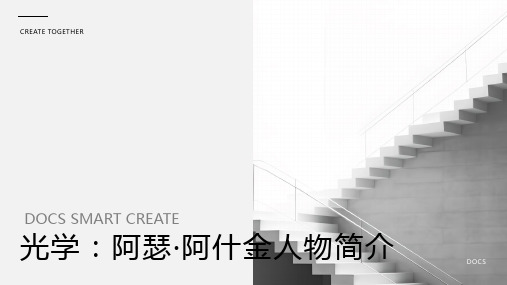
据
03
1956年提出光学衍射技术的原理
• 光学衍射技术是光学领域的重要技术之一
• 阿瑟·阿什金的原理为光学衍射技术的发展提供了理论依
据
03
阿瑟·阿什金的学术地位与影响
阿瑟·阿什金在光学领域的学术地位
阿瑟·阿什金被认为是现代光学领域的奠基人之一
• 激光技术、光纤通信技术、生物医学、环境监测等领域
• 为人类科技进步做出了巨大贡献
阿瑟·阿什金获得了许多荣誉和奖项
• 1977年获得诺贝尔物理学奖
• 1984年获得美国国家科学奖章
• 1985年获得沃尔夫奖
• 1989年获得洛林克拉克奖
02
阿瑟·阿什金的光学研究成果
阿瑟·阿什金在激光技术方面的贡献
术等领域的成果
光学产业领域
• 为光学产业的发展提供了新
• 为光学产业的发展做出了巨
的思路和技术支持
大贡献
04
阿瑟·阿什金的个人品质与精神
阿瑟·阿什金的勤奋与毅力
阿瑟·阿什金是一位非常勤奋的科学家
阿瑟·阿什金是一位具有毅力的科学家
• 他在光学领域的研究工作非常刻苦
• 他在面临困难和挑战时,从不放弃
阿瑟·阿什金的团队合作精神
阿瑟·阿什金的团队合作精神对他的学术成就产生了重要影响
• 他的团队合作精神为光学领域的发展提供了有力的人才支持
阿瑟·阿什金是一位具有团队合作精神的科学家
• 他在进行研究工作时,非常注重与他人的合作
• 与他的团队成员共同取得了许多重要的学术成果
CREATE TOGETHER
• 光纤传感技术是光纤通信技术的重要应用领域
- 1、下载文档前请自行甄别文档内容的完整性,平台不提供额外的编辑、内容补充、找答案等附加服务。
- 2、"仅部分预览"的文档,不可在线预览部分如存在完整性等问题,可反馈申请退款(可完整预览的文档不适用该条件!)。
- 3、如文档侵犯您的权益,请联系客服反馈,我们会尽快为您处理(人工客服工作时间:9:00-18:30)。
a rXiv:as tr o-ph/986313v21J ul19981The Optical Gravitational Lensing Experiment.BVI Maps of Dense Stellar Regions.I.The Small Magellanic Cloud ∗A.U d a l s k i 1,M.S z y m a ´n s k i 1,M.K u b i a k 1,G.P i e t r z y ´n s k i 1,P.W o ´z n i a k 2,and K.˙Z e b r u ´n 11Warsaw University Observatory,Al.Ujazdowskie 4,00-478Warszawa,Poland e-mail:(udalski,msz,mk,pietrzyn,zebrun)@.pl 2Princeton University Observatory,Princeton,NJ 08544-1001,USA e-mail:wozniak@ ABSTRACT We present three color,BVI maps of the Small Magellanic Cloud.The maps contain precise photometric and astrometric data for about 2.2million stars from the central regions of the SMC bar covering ≈2.4square degrees on the sky.Mean brightness of stars is derived from observations collected in the course of the OGLE-II microlensing search from about 130,30and 15measurements in the I ,V and B -bands,respectively.Accuracy of the zero points of photometry is about 0.01mag,and astrometry 0.15arcsec (with possible systematic error up to 0.7arcsec).Color-magnitude diagrams of observed fields are also presented.The maps of the SMC are the first from the series of similar maps covering other OGLE fields:LMC,Galactic bulge and Galactic disk.The data are very well suited for many projects,particularly for the SMC which has been neglected photometrically for years.Because of potentially great impact on many astrophysical fields we decided tomake the SMC data available to the astronomical community from the OGLE Internet archive.Key words:Magellanic Clouds –Surveys –Catalogs –Techniques:photometrics 1IntroductionThe Optical Gravitational Lensing Experiment (OGLE)is a long term ob-serving project which started in 1992as the search for microlensing events2in our Galaxy with the ultimate goal of providing information about the dark unseen matter(Udalski et al.1992).In1993thefirst ever observed microlensing event toward the Galactic bulge was detected.In total about 20microlensing events were found during thefirst phase of the project which ended in1995(Udalski et al.1994,Wo´z niak and Szyma´n ski1998).Starting from January1997the OGLE project entered its second phase –OGLE-II.With a new,dedicated1.3-m Warsaw telescope located at the Las Campanas Observatory,the observing capabilities of the OGLE project increased by a factor of30(Udalski,Kubiak and Szyma´n ski1997).New targets,namely the Large and Small Magellanic Clouds,newfields in the Galactic bulge and Galactic disk have been added to the list of regularly observed regions of the sky.After thefirst year of observations large databases of the observed targets have been created andfirst microlensing events have been detected(Udalski and Szyma´n ski1998).The OGLE project photometric observations are collected in the standard BVI-bands which makes them very well suited not only for microlensing but also for many side projects.For instance, photometry of the LMC and SMC has already been used for a new distance determination to both Magellanic Clouds(Udalski et al.1998,Udalski1998).In this paper,first of the series,we present the BVI maps of dense stellar regions in the SMC observed in the course of the OGLE-II project.The maps provide BVI-band photometry of all stellar objects detected in observed fields and in the case of the SMC contain well calibrated photometry and astrometry of about2.2million stars from the central regions(≈2.4square degree)of the SMC bar.The data are used to construct color-magnitude diagrams(CMDs)of observedfields revealing many subtle features of stellar populations in the SMC.Presented maps can be a very useful tool for many astronomical projects. For instance,a unique catalog of clusters in the SMC with accurate color-magnitude diagrams follows this paper(Pietrzy´n ski et al.1998).The SMC observations with the modern,precise techniques are rare and those which can be found in the literature concentrate on particular objects.The maps contain a full variety of objects includingfield stars and clusters located in different parts of the SMC and therefore are ideal for comparisons of stellar populations in different regions of the SMC.Bearing in mind potential impact of the OGLE photometric data on our understanding of the SMC and other OGLE objects,we decided to make these data available to the astronomical community.At the end of this paper we provide information how to obtain the SMC maps.In the next papers3 of this series the LMC and Galactic bulge maps will be gradually released.In subsequent Sections we describe observations,reduction and calibra-tion techniques used to construct the maps.Then results of accuracy and completeness tests are presented.Finally,we show CMDs of all ourfields in the SMC.2ObservationsAll observations presented in this paper were carried out with the1.3-m Warsaw telescope at the Las Campanas Observatory,Chile,which is oper-ated by the Carnegie Institution of Washington,during the second phase of the OGLE experiment.The telescope was equipped with the”first genera-tion”camera with the SITe2048×2048CCD detector.The pixel size was 24µm resulting in0.417arcsec/pixel scale.The observations of the SMC were performed in the”slow”reading mode of the CCD detector with the gain3.8e−/ADU and readout noise≈5.4e−.Details of the instrumentation setup can be found in Udalski,Kubiak and Szyma´n ski(1997).Observations of the SMC started on June26,1997.As the microlens-ing search is planned to last for a few years,observations of selectedfields will be continued during next few seasons.In this paper we present data collected before the end of thefirst SMC season–Mar.4,1998.Obser-vations were carried out in the driftscan mode.In this mode the CCD detector columns are aligned along the drift direction and the detector is read continuously with the rate synchronized with the drift rate of the star. As a result much larger areas can be observed with a single chip than in the normal,still frame mode.Also the dead time for detector reading is avoided.The images are rectangular with the width equal to the width of the CCD detector and the length proportional to the scanning time.For technical reasons–handling of large datafiles–OGLE driftscans are limited to8192lines which produces images of2048×8192pixel size(≈34MB raw image).The OGLE driftscans are made with the drift along the meridian (i.e.,declination)direction.In this way any region of the sky is accessible for observations including the Magellanic Clouds located at high southern declinations.The drift of stars is forced by tracking the telescope in both axes:normal tracking in RA and additional tracking in declination.The declination tracking rate determines the effective exposure time which in the case of the SMC is125sec,174sec and237sec for the I,V and B-bands, respectively.Very sensitive CCD detector allows to obtain good photometry4down to V≈21.5mag even with such relatively short exposure times.Observations are made in three bands:B,V and I.Because of microlens-ing observing strategy,the vast majority of observations is collected in the I-band with some additional measurements in the B and V-bands.Standard set of glass Schottfilters is used,closely approximating the standard BVI system.Because of large stellar density in the OGLE-II main targets,the obser-vations are limited to very good seeing conditions only,in order to ensure good photometry.Typical median seeing of presented data set is about 1.25arcsec in the I-band with the best images reaching0.8arcsec.It should be noted that the active driftscans with open loop(no guiding)tracking in two axes give always slightly worse seeing because of inaccuracies in tracking etc.Observations of the SMC are usually stopped when the seeing exceeds 1.8arcsec.11driftscanfields were selected in the SMC.They cover practically all dense regions of the SMC bar.Fig.1presents the picture of the SMC from the Digitized Sky Survey CD-ROMs with contours of ourfields.Coordinates of the center of eachfield are given in Table1.Each of thefields covers ≈14.2×57arcmin on the sky(≈0.22square degree).Fields are shifted in declination to follow the location of the center of the SMC bar on the sky. Neighboringfields overlap by about1arcmin for calibration of photometry and astrometry purposes.In the next observing seasons additionalfields will be added to those listed in Table1to cover practically entire SMC.During thefirst observing season about100–150observations in the I-band were collected,depending on thefield.For twofields,SMC SC6a few additional measurements were collected earlier,in January 1997.Number of B and V observations is smaller–about15and30per field,respectively.It is though,large enough to derive precise photometry of objects in these bands.3Photometric Reductions3.1The OGLE Data PipelineWhen a new frame is collected it is automatically intercepted by the OGLE photometric data reduction pipeline.All reductions are performed almost in real time at the telescope.In thefirst step preliminary reductions are performed–debiasing,and flat-fielding based on regularly collected twilight skyflat-field and bias im-5T a b l e1Equatorial coordinates of the SMCfieldsSMCSC20h40m53s−73◦17′30′′SMCSC40h46m59s−73◦07′30′′SMCSC60h53m01s−72◦58′40′′SMCSC80h58m58s−72◦39′30′′SMCSC101h04m51s−72◦24′45′′SMCages.It should be noted that the driftscan modeflat-fields are one-dimensio-nal line which is usually an average of a few thousand lines(depending on the length of the calibration driftscanflat-field image)and thus determined very precisely.After the frame is de-biased andflat-fielded it is sent to the second stage reduction procedures which derive photometry of stars on the image.The photometry is calculated with the modified version of the DoPhot pho-tometry program(Schechter,Saha and Mateo1993).Because of significant variations of the Point Spread Function(PSF)across the image caused by some inaccuracies of the telescope tracking and variable seeing in different moments of scanning,photometry reductions are performed on the64sub-frames of512×512pixel size on which the PSF can be assumed as constant in thefirst approximation.The DoPhot program is run in the so called fixed position mode,that is an input list of positions of objects in a given field,generated in advance,is provided to the program.Coordinates from this list are transformed tofit the coordinates of the current frame andfixed for PSF photometryfitting.This mode is much faster and more accurate than the regular mode,in particular when the seeing of the frame is worse.The input list of coordinates comes from the so called template image which is one of the best images of the entire data set for a givenfield and6filter taken at the possibly best seeing conditions.Positions of objects from the template images are obtained from photometric reductions with a special procedure based on regular andfixed mode reductions with the DoPhot program.Photometry of each subframe of the template image is tied based on photometry in overlapping regions between subframes(for template re-duction somewhat larger subframes are cut from the template image to reach 100pixel overlap).Photometry of the template image is further treated as the reference instrumental photometry for the entire data set.Template coordinate lists for the B and V-bands are generated in similar way with the exception that positions of objects in these frames arefittedfirst with those of the I-band template image.In this way position of the star is always the same in the list of each band photometry which makes further data handling much easier.When reductions of all subframes of a given image are done,photometry of each subframe is tied to that of the reference template subframe and results of photometry are stored in compact binary format in diskfiles.The process of reductions:dividing to subframes,reductions,correction of the zero points etc.is performed fully automatically.The amount of CPU of the system is sufficient to perform photometric reductions of all frames collected during the night(50–70driftscans)within24hours.3.2Transformation to the Standard SystemSeveral Landolt(1992)fields were observed during about30photometric nights to derive transformation of our instrumental magnitudes in the tem-plate photometric system to the standard BVI system.Based on a few hundreds of measurements of standard stars the following mean transforma-tion was derived:B=b−0.041×(B−V)+const BV=v−0.002×(V−I)+const VI=i+0.029×(V−I)+const I(1) B−V=0.959×(b−v)+const B−VV−I=0.969×(v−i)+const V−Iwhere lower case letters b,v,i denote the aperture magnitudes normalized to1sec exposure time.The transformation coefficients are the mean for the entire season and were determined on a few nights when number of measurements of standard stars was large.The zero points and extinction7 coefficients(when impossible,mean extinction values were used)were de-termined individually for each night.Residuals betweenfitted magnitudes of standard stars and observed ones did not exceed0.02–0.03mag.As can be seen the transformation coefficients are close to zero for magni-tude or one for color transformations,thus indicating that our instrumental system very well approximates the standard one and transformation errors are small.As thefirst step to convert our instrumental system to the stan-dard one,aperture corrections to the template magnitudes were derived.A special procedure was written,based on the DoPhot program,which re-duces each subframe of a given image in analogous way as during normal reductions,selects bright stars well separated from neighboring stars which could contaminate their photometry and calculates the aperture magnitude for every selected star removing all remaining objects from the frame.About 10–50aperture stars were usually used in each of the64subframes of a given image.The median of aperture corrections derived from these stars was adopted as the aperture correction of a given subframe.Next,the”to-tal correction”for each subframe of a given image was calculated.This is defined as the sum of its aperture correction,the transformation zero point, extinction correction and normalization to1sec exposure time.Then”total corrections”determined from about25,10and6nights for the I,V and B-bands,respectively,were averaged producing an array of64mean”total corrections”for a givenfield and band.Typical mean error of thefinal”total correction”values was less than0.01mag.3.3Final Databases of Results and BVI Maps of the SMC ”Total corrections”,determined as described in the previous Section,were used to convert the template photometric system of eachfield andfilter to the instrumental system very close to the standard one(the difference involves only color terms of Eqs.(1)).At this stage databases of photomet-ric results were created and the appropriate”total corrections”were simply added to the template photometric system magnitudes when databases were filled up.The databases used are very similar to those developed for the OGLE-I phase of the project(Szyma´n ski and Udalski1993)with some slight improvements and modifications.They contain all individual photometric measurements in the system close to the standard one(no color terms) and all basic information about each frame:heliocentric Julian date,expo-sure time,mean seeing and its standard deviation,frame grade etc.Entire database for a givenfield andfilter consists of two parts–that of stars8detected on the template frame and that of”new”objects.In this paper we limit ourselves to the template objects only.The databases of the SMC contain approximately2.25million objects.The vast majority of them are objects classified by the DoPhot program as stellar.T a b l e2Number of stellar objects in the SMC mapsSMCSC2107326SMCSC4198201SMCSC6326367SMCSC8211115SMCSC10140589SMCWhen the databases of photometric results were created for eachfield andfilter for our SMCfields,thefinal BVI-band maps of the SMC were constructed.First,measurements of each star were averaged with5σrejec-tion alghoritm in each band.Then color term transformation corrections were calculated andfinally the standard system magnitudes were derived. Only magnitudes derived from more than40,10and5good measurements in the I,V and B-bands,respectively,were listed in thefinal maps of the SMC.Good measurement was defined as the one with the stellar type object returned by the DoPhot program and the error returned by DoPhot not exceeding1.6median of the errors from the entire set of measurements of a given star.Final number of objects included in the SMC maps is given in Table2.Table3presents sample data of thefinal map for the SMC10observations,number of rejected observations and standard deviation of the mean magnitude for B,V and I-bands,respectively.Such maps for all our fields are available to the astronomical community(see Section7).4AstrometryTo convert positions of stars to the equatorial system the pixel coordinate system of the I-band template image for eachfield was transformed to the equatorial system using the Digital Sky Survey(DSS)images.The procedure consists of the following steps.First,FITS image,some-what larger than the area of a givenfield,is extracted from the DSS CD-ROMs.Then,the”autofind”program is run on that frame.Itfinds all objects brighter than selected threshold and calculates a centroid of each detected object.Now,the main transformation program is run.First,it con-verts the(x,y)coordinates of each star from the DSS image to RA and DEC based on prescription and procedures provided with the DSS distribution. Then(RA,DEC)of each star are transformed with simple trigonometric for-mulae to(x′,y′)coordinates of the Cartesian system on the plane tangent to the celestial sphere at the center of a givenfield.Finally,transforma-tion between(x′,y′)coordinates and pixel coordinate system of the I-band template image follows.The transformation between those two systems of coordinates is relatively simple and third order polynomials are sufficient to achieve good accuracy.The transformation is calculated in a few iterations with decreasing critical radius within which objects are treated as identical. Thefinal critical radius is0.75arcsec.About3000–8000stars in the SMC fields were used for transformation depending on stellar density of thefield. 5Data Tests5.1PhotometryTo asses quality of photometry a few tests were performed.First,we com-pared photometry of the same stars located in the overlapping regions be-tweenfields.As the overlapping regions extend along the length of the image we compared the magnitude differences of stars on both frames as a function of line number.Figs.2,3and4present plots”magnitude differ-ence D line number”forfields located in the west,center and east regions of the SMC(SMC5–6,and SMC11 and I.Similar plots for otherfields look practically identical.As can be seen the photometry in overlapping regions of neighboringfields is in very good agreement.The mean difference is always below0.01mag indicating that our calibration procedures were correct.In the second test we compared our photometry with other reliable mea-surements from the literature.Unfortunately good quality observations of the SMC with modern techniques are rare.We limited our comparison mostly to CCD measurements,as only those can be reliable in crowded fields of the SMC.We found the following past observations of the SMC:•CCD photometry of NGC346was published by Massey,Parker and Garmany(1989).Only part of this cluster is located in the SMCSC7field)was obtained by Walker (Caloi et al.1993).Photometry of Walker is in good agreement with more recent photometry of Vallenari,Ortolani and Chiosi(1994).The mean difference between the OGLE and Walker photometry is∆V=−0.002±0.020mag and∆(B−V)=0.040±0.014mag(9stars).•CCD photometry of thefield around HV1876(SMCSC11field)vari-able star(Jensen,Clausen and Gim´e nez1988).The mean difference between the OGLE and Jensen,Clausen and Gim´e nez(1988)pho-tometry of proposed standard stars is∆V=−0.007±0.011mag and ∆(B−V)=0.015±0.006mag.•Photoelectric photometry sequence around NGC419(SMC12•Finally we compared the I-band light curves of two”stable”variable stars from the NGC330region(SMCSC1,SMC SC10and represent the least and most dense regions of the SMC bar.Selected subframes cover the central,most dense part of eachfield.To each subframe a number of artificial stars in randomly selected posi-tions was added using a special software based on DoPhot procedures.In order not to change crowding of the frame we added only296stars in each test with magnitude distribution as shown in column1of Table4.Then we checked how many of them were recovered with our standard reduction pipeline treating the input and output star as identical if its difference of position and magnitude was smaller than0.8arcsec and±0.5mag,respec-tively.One hundred such tests were performed for each subframe.The rate of recovered objects is listed in Table4.It is clear that down to B≈20.0,V≈20.5and I≈20.0detection completeness is very high and then it fades gradually reaching≈50%at B≈21.2,V≈21.5and I≈21.0for the least densefields.Thisfigures might be assumed as limiting magnitudes of our survey.As expected com-pleteness is somewhat lower for the most densefield SMC13T a b l e4Completeness of the SMC maps1SC10V SC6SC1SC10estimate that only small percentage of objects was omitted.5.3AstrometryAccuracy of our astrometric solutions was checked by comparison of coor-dinates of detected objects located in overlapping regions betweenfields. First,transformation betweenfields was derived and common objects were identified.Then,equatorial coordinates of each identified object were cal-culated based on transformations for bothfields and the difference between the two.Finally,the mean difference and its standard error were calculated from the entire sample of identified pairs of stars(typically a few hundred objects).The mean difference of coordinates betweenfields was typically about0.1–0.15arcsec with the standard deviation of about0.1–0.2arcsec. This error is representative of internal error of our determination of equato-rial coordinates.To check possible systematic errors we comparedfields with astrometric solutions based on different plates from the DSS.We repeated the above pro-14cedure comparing coordinates offields determined from different plates of the DSS.In this case the mean difference was much larger,sometimes reach-ing0.7arcsec.This number might be assumed as the possible systematic error of the DSS coordinates system and thus of our equatorial coordinates. 6Color-Magnitude DiagramsBased on our three color maps of the SMC,we constructed composite color-magnitude diagrams for each of ourfields.Figs.6–16present the V−(B−V) and V−(V−I)CMDs of all ourfields in the SMC.Only10–30%of stars were included in these Figures for clarity.The CMDs of the SMCfields reveal in great detail main features known from previous studies:the main sequence,red giant branch with nicely pro-nounced red clump of giants,its vertical extension(similar to that observed in the LMC,cf.Zaritsky and Lin1997,Beaulieu and Sackett1998,Udalski et al.1998)and asymptotic branch clump.Also many otherfine features become recognizable due to large statistics of stars in these diagrams.It is obvious that our maps provide a unique tool for analysis of the wide variety of topics concerning the SMC and stars in general.7ConclusionsWe release in this paper the three color,BVI-band maps of the SMC.The data contain photometry for about2.2million stars from the central parts of the SMC bar.The data are precisely calibrated,the mean magnitudes are determined from tens/hundreds of individual measurements.Our tests show that accuracy of the zero point of our photometry should be about 0.01mag.Precise equatorial coordinates for all objects are determined from the DSS astrometric solution with internal accuracy of about0.15arcsec,and possible systematic errors of the DSS astrometric system up to0.7arcsec. Large number of photometric measurements used for determination of mean magnitudes makes our unique data a huge set of secondary photometric standards in the SMC.In the next paper of this series similar data for the LMC barfields observed during the OGLE-II project will be presented.Release of similar maps of the Galactic bulge is planned in the future.15 The three color maps are available to the astronomical community fromthe OGLE archive .pl/˜ftp/ogle and its mirror/˜ogle(or ftp:///ogle/ogle2/maps/smc). Also I-band FITS template images of ourfields are included.Due to largevolume of data–about400MB,which is not always suitable for networktransfers we also distribute the entire set of data on CD-ROMs for a small feecovering handling costs.Please contact via e-mail:cfpa@.pl.Usage of the data is allowed under the condition of proper acknowledgmentto the OGLE project.We provide these data in the most original form to avoid any addi-tional biases.For instance we do not mask bright stars which often producemany artifacts,but such masking could potentially remove some interestinginformation on objects located close to bright stars.We do not remove ob-jects which are located in overlapping areas between the neighboringfields.Cross-identification of these objects can be easily done based on providedequatorial coordinates.Acknowledgements.We would like to thank Prof.Bohdan Paczy´n skifor many encouraging discussions and help at all stages of the OGLE project.The paper was partly supported by the Polish KBN grant2P03D00814to A.Udalski.Partial support for the OGLE project was provided with the NSFgrant AST-9530478to B.Paczy´n ski.We acknowledge usage of The DigitizedSky Survey which was produced at the Space Telescope Science Institutebased on photographic data obtained using The UK Schmidt Telescope,operated by the Royal Observatory Edinburgh.REFERENCESBeaulieu,J.P.,and Sackett,P.D.1998,Astron.J.,in press,(astro-ph/9710156).Caloi,V.,Cassatella,A.,Castellani,V.,and Walker,A.1993,Astron.Astrophys.,271, 109.Jensen,K.S.,Clausen,J.V.,and Gim´e nez,A.1988,Astron.Astrophys.Suppl.Ser.,74, 331.Landolt,A.U.1992,Astron.J.,104,372.Massey,P.,Parker,J.W.,and Garmany,C.D.1989,Astron.J.,98,1305.Pietrzy´n ski,G.,Udalski,A.,Szyma´n ski,M.,Kubiak,M.,Wo´z niak,P.,and˙Zebru´n,K.1998,Acta Astron.,48,175.Schechter,P.L.,Saha,K.,and Mateo,M.1993,P.A.S.P.,105,1342.Sebo,K.M.,and Wood,P.R.1994,Astron.J.,108,932.Szyma´n ski,M.,and Udalski,A.1993,Acta Astron.,43,91.Udalski,A.1998,Acta Astron.,48,113.Udalski,A.,Kubiak,M.,and Szyma´n ski,M.1997,Acta Astron.,47,319.Udalski,A.,and Szyma´n ski,M.1998,IAU Circ.,#6901.16Udalski,A.,Szyma´n ski,M.,Ka l u˙z ny,J.,Kubiak,M.and Mateo,M.1992,Acta Astron., 42,253.Udalski,A.,Szyma´n ski,M.,Stanek,K.Z.,Ka l u˙z ny,J.,Kubiak,M.,Mateo,M.,Krzemi´n ski, W.,Paczy´n ski,B.,and Venkat,R.1994,Acta Astron.,44,165.Udalski,A.,Szyma´n ski,M.,Kubiak,M.,Pietrzy´n ski,G.,Wo´z niak,P.,and˙Zebru´n,K.1998,Acta Astron.,48,1,(astro-ph/9803035).Vallenari,A.,Ortolani,S.,and Chiosi,C.1994,Astron.Astrophys.Suppl.Ser.,108,571. Wenderoth, E., Alvarado,F.,Alcaino,F.,and Liller,W.1994,Astron.Astrophys.Suppl.Ser., 106,253.Wo´z niak,P.,and Szyma´n ski,M.1998,Acta Astron.,48,269.Zaritsky,D.,and Lin,D.B.C.1997,Astron.J.,114,2545.。