Aboveground and belowground effects of single-tree removals in new Zealand rain forest
增温改变高寒草甸植物群落异速生长关系
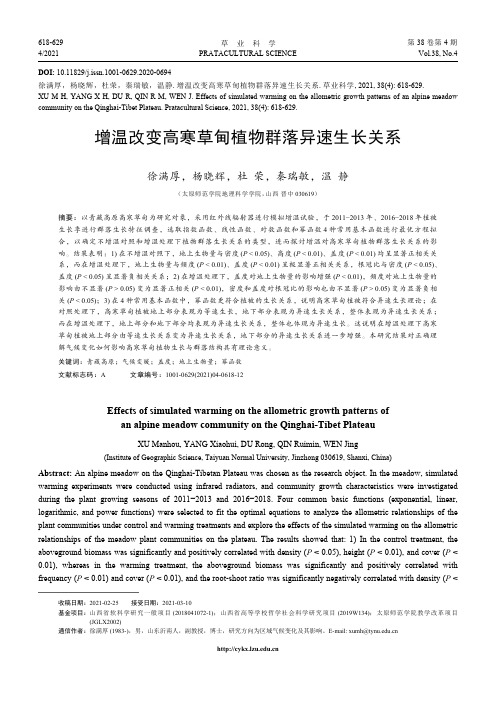
618-629草 业 科 学第 38 卷第 4 期4/2021PRATACULTURAL SCIENCE Vol.38, No.4DOI: 10.11829/j.issn.1001-0629.2020-0694徐满厚,杨晓辉,杜荣,秦瑞敏,温静. 增温改变高寒草甸植物群落异速生长关系. 草业科学, 2021, 38(4): 618-629.XU M H, YANG X H, DU R, QIN R M, WEN J. Effects of simulated warming on the allometric growth patterns of an alpine meadow community on the Qinghai-Tibet Plateau. Pratacultural Science, 2021, 38(4): 618-629.增温改变高寒草甸植物群落异速生长关系徐满厚,杨晓辉,杜 荣,秦瑞敏,温 静(太原师范学院地理科学学院,山西 晋中 030619)摘要:以青藏高原高寒草甸为研究对象,采用红外线辐射器进行模拟增温试验,于2011−2013年、2016−2018年植被生长季进行群落生长特征调查,选取指数函数、线性函数、对数函数和幂函数4种常用基本函数进行最优方程拟合,以确定不增温对照和增温处理下植物群落生长关系的类型,进而探讨增温对高寒草甸植物群落生长关系的影响。
结果表明:1)在不增温对照下,地上生物量与密度(P < 0.05)、高度(P < 0.01)、盖度(P < 0.01)均呈显著正相关关系,而在增温处理下,地上生物量与频度(P < 0.01)、盖度(P < 0.01)呈极显著正相关关系,根冠比与密度(P < 0.05)、盖度(P < 0.05)呈显著负相关关系;2)在增温处理下,盖度对地上生物量的影响增强(P < 0.01),频度对地上生物量的影响由不显著(P > 0.05)变为显著正相关(P < 0.01),密度和盖度对根冠比的影响也由不显著(P > 0.05)变为显著负相关(P < 0.05);3)在4种常用基本函数中,幂函数更符合植被的生长关系,说明高寒草甸植被符合异速生长理论;在对照处理下,高寒草甸植被地上部分表现为等速生长,地下部分表现为异速生长关系,整体表现为异速生长关系;而在增温处理下,地上部分和地下部分均表现为异速生长关系,整体也体现为异速生长。
Above- and belowground biomass allocation

ROOTS
23.21±28.95 32.23±15.31 14.49±14.56 39.70±22.82 29.21±21.63 61.35±36.89 33.36±16.13 21.96±10.38
SOIL
7516.08±646.94 5667.31±2290.95 6081.07±923.85 19636.66±10789.00 5363.39±808.46 7768.04±1314.22 8672.09±5460.13 7488.88±1258.82
7228.69±1034.88
4619.57±121.56 11349.16±6094.98
K-WF-F5
K-WF-F6 AVERAGE
32.18±19.73
13.18±2.08 19.32±9.29
14.79±6.82
16.63±2.56 12.95±3ห้องสมุดไป่ตู้14
46.97±25.59
29.81±1.29 32.18±9.34
Carpesium abrotanoides L. Enneapogon borealis Honda Corispermum hyssopifolium L. Enneapogon borealis Honda Tribulus terrestris L. Inula britannica L.var. chinensis (Rupr.) Reg. Scutellaria baicalensis Georgi Cleistogenes squarrosa Lespedeza bicolor Turcz Glycyrrhizae Tribulus terrestris L. Scutellaria baicalensis Georgi Corispermum hyssopifolium L. Scutellaria baicalensis Georgi Salsola ruthenica lljin Corispermum hyssopifolium L. Cyperus rotundus L. Potentillae Chinensis Inula britannica L.var. chinensis (Rupr.) Reg. Cyperus rotundus L. Potentillae Chinensis Chloris virgata Swartz Calamagrostis epigeios (L.) Roth Kochia scoparia (Linn.) Schrad. var. sieversiana (Pall.) Ulbr. ex Aschers. et Graebn Phragmites australis (Cav.) Trin. ex steud. Chloris virgata Swartz Cyperus rotundus L. Phragmites australis (Cav.) Trin. ex steud. Corispermum hyssopifolium L. Pennisetum centrasiaticum Tzvel. Salsola ruthenica lljin Corispermum hyssopifolium L.
8个山毛榉引进品种秋季叶色变化研究

引用格式:马俊青,卢绍辉.8个山毛樺引进品种秋季叶色变化研究[几湖南农业科学,2020(12):45・4&DOI:10.16498/ki.hnnykx.2020.012.0138个山毛棒引进品种秋季叶色变化研究马俊青,卢绍辉(河南省林业科学研究院,河南郑州450008)摘要:以加拿大引种的8个山毛樺品种为材料.通过分析其叶片町溶性糖、可溶性蛋H的含量以及秋季叶色变化期叶片的L:、八X值,来挑选适宜在河南地区栽植的山毛榕品种"结果表明:山毛样叶片可溶性蛋白含量与叶色参数J值正相关,8个]11毛樺引进品种中(i Purple Fountain"t(Dawyck Gold Beech”"Red Obelisk"4'Roseomarginata”,这4个品种秋季出现彩叶的吋间较长,叶色较为明丽,可作为彩叶山毛棒的优良品种进一步培育各关键词:山毛桦;秋季叶色;可溶性蛋白;可溶性糖;济源中图分类号:Q949.736.3文献标识码:A文章编号:1006-060X(2020)12・0045・04 Study on Leaf Color Change of8Beech(Fagus sylvatica)Varieties in AutumnMA Jun-qing,LU Shao-hui(Henan Academy oj Forestry Sciences,Zhengzhou450008,PRC)Abstract:Tn order to select beech varieties adaptive to the Henan region,we measured soluble sugar,soluble protein and L,a and b* values in the autumn leaves of8European beech(Fagus sylvatica)varieties introduced from Canada.The results show that there is a positive conelation between leaf soluble protein content and a value(the leaf color parameter).Among the eight beech varieties,four varieties,i.e.,'Purple Fountain\'Dawyck Gold Beech c Red Obelisk1and'Roseomarginata5present a longer color-leaf stage with bright and colorful leaves,which can be further cultivated as good color-leaf b eech varieties.Key words:beech(Fagus sylvatica)',autumn leaf color;soluble protein;soluble sugar;Jiyuan欧洲山毛棒(Fagus sylvatica),为壳斗科(Fagaceae)水青冈属(Fagus L.)植物,是一种高大的落叶乔木,广泛分布于亚洲、欧洲与北美洲等地区,用途非常广泛叫欧洲山毛棒质地细腻,姿态美观,秋色叶褐色带红,可作为大型庭园、园林、绿地及公共场所或周边树种,也可用作行道树。
白桦养护方案
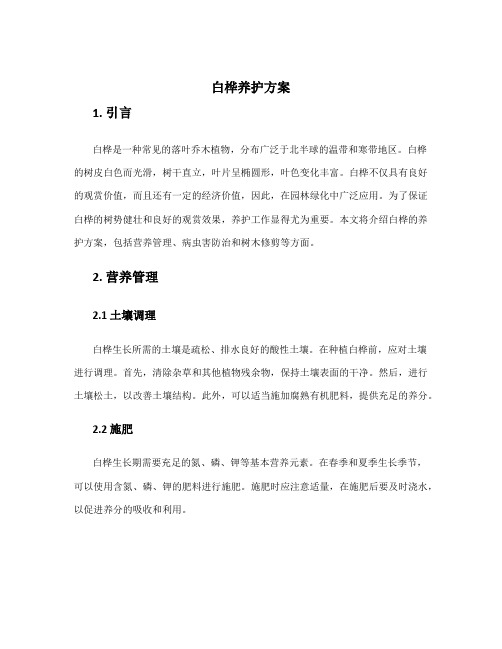
白桦养护方案1. 引言白桦是一种常见的落叶乔木植物,分布广泛于北半球的温带和寒带地区。
白桦的树皮白色而光滑,树干直立,叶片呈椭圆形,叶色变化丰富。
白桦不仅具有良好的观赏价值,而且还有一定的经济价值,因此,在园林绿化中广泛应用。
为了保证白桦的树势健壮和良好的观赏效果,养护工作显得尤为重要。
本文将介绍白桦的养护方案,包括营养管理、病虫害防治和树木修剪等方面。
2. 营养管理2.1 土壤调理白桦生长所需的土壤是疏松、排水良好的酸性土壤。
在种植白桦前,应对土壤进行调理。
首先,清除杂草和其他植物残余物,保持土壤表面的干净。
然后,进行土壤松土,以改善土壤结构。
此外,可以适当施加腐熟有机肥料,提供充足的养分。
2.2 施肥白桦生长期需要充足的氮、磷、钾等基本营养元素。
在春季和夏季生长季节,可以使用含氮、磷、钾的肥料进行施肥。
施肥时应注意适量,在施肥后要及时浇水,以促进养分的吸收和利用。
3. 病虫害防治3.1 病害防治白桦常见的病害有白粉病、锈病等。
对于白粉病,可以使用草铵膦等药剂进行喷洒防治。
对于锈病,可选用氧髓霉素等药剂进行喷洒。
在病害防治过程中,要注意药剂的使用方法和浓度,遵循使用说明,以防止对植物造成伤害。
3.2 虫害防治白桦常见的虫害有桦树毛虫、桦树长角象等。
对于桦树毛虫,可以使用氯氟环螨等药剂进行喷洒防治。
对于桦树长角象,可选用气雾剂进行喷洒。
在虫害防治过程中,要及时发现虫害并采取措施,避免虫害扩散。
4. 树木修剪4.1 去除枯枝白桦生长期间,常会出现一些枯枝。
这些枯枝不仅影响树木的美观,还可能给树木带来病害。
因此,及时去除枯枝是必要的。
修剪枯枝时,应使用锋利的修枝剪,避免对树木造成伤害。
4.2 维持树形白桦生长速度较快,容易形成枝繁叶茂的树冠。
为了维持树木的均衡和整齐的树形,需要进行定期修剪。
修剪时应注意控制修剪的强度,以免过度修剪影响树木的生长和营养吸收。
5. 结语白桦作为常见的园林绿化用树种,其养护工作对于保证树木的健康和观赏效果至关重要。
植物地上部分与地下部分的联系
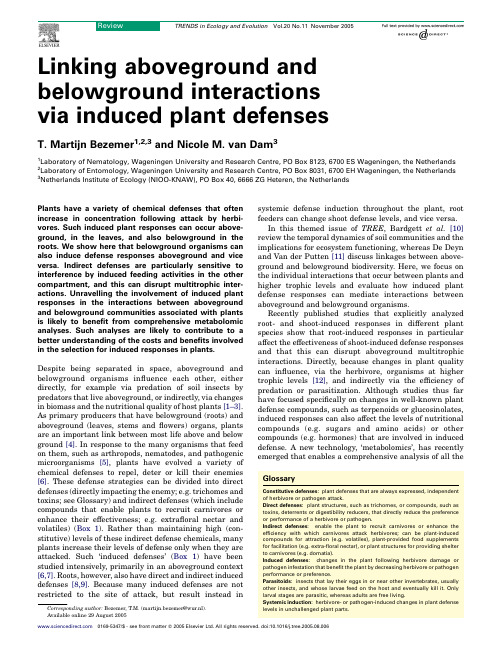
Linking aboveground and belowground interactions via induced plant defensesT.Martijn Bezemer 1,2,3and Nicole M.van Dam 31Laboratory of Nematology,Wageningen University and Research Centre,PO Box 8123,6700ES Wageningen,the Netherlands 2Laboratory of Entomology,Wageningen University and Research Centre,PO Box 8031,6700EH Wageningen,the Netherlands 3Netherlands Institute of Ecology (NIOO-KNAW),PO Box 40,6666ZG Heteren,the NetherlandsPlants have a variety of chemical defenses that often increase in concentration following attack by herbi-vores.Such induced plant responses can occur above-ground,in the leaves,and also belowground in the roots.We show here that belowground organisms can also induce defense responses aboveground and vice versa.Indirect defenses are particularly sensitive to interference by induced feeding activities in the other compartment,and this can disrupt multitrophic inter-actions.Unravelling the involvement of induced plant responses in the interactions between aboveground and belowground communities associated with plants is likely to benefit from comprehensive metabolomic analyses.Such analyses are likely to contribute to a better understanding of the costs and benefits involved in the selection for induced responses in plants.Despite being separated in space,aboveground and belowground organisms influence each other,either directly,for example via predation of soil insects by predators that live aboveground,or indirectly,via changes in biomass and the nutritional quality of host plants [1–3].As primary producers that have belowground (roots)and aboveground (leaves,stems and flowers)organs,plants are an important link between most life above and below ground [4].In response to the many organisms that feed on them,such as arthropods,nematodes,and pathogenic microorganisms [5],plants have evolved a variety of chemical defenses to repel,deter or kill their enemies [6].These defense strategies can be divided into direct defenses (directly impacting the enemy;e.g.trichomes and toxins;see Glossary)and indirect defenses (which include compounds that enable plants to recruit carnivores or enhance their effectiveness;e.g.extrafloral nectar and volatiles)(Box 1).Rather than maintaining high (con-stitutive)levels of these indirect defense chemicals,many plants increase their levels of defense only when they are attacked.Such ‘induced defenses’(Box 1)have been studied intensively,primarily in an aboveground context [6,7].Roots,however,also have direct and indirect induced defenses [8,9].Because many induced defenses are not restricted to the site of attack,but result instead insystemic defense induction throughout the plant,root feeders can change shoot defense levels,and vice versa.In this themed issue of TREE ,Bardgett et al.[10]review the temporal dynamics of soil communities and the implications for ecosystem functioning,whereas De Deyn and Van der Putten [11]discuss linkages between above-ground and belowground biodiversity.Here,we focus on the individual interactions that occur between plants and higher trophic levels and evaluate how induced plant defense responses can mediate interactions between aboveground and belowground organisms.Recently published studies that explicitly analyzed root-and shoot-induced responses in different plant species show that root-induced responses in particular affect the effectiveness of shoot-induced defense responses and that this can disrupt aboveground multitrophic interactions.Directly,because changes in plant quality can influence,via the herbivore,organisms at higher trophic levels [12],and indirectly via the efficiency of predation or parasitization.Although studies thus far have focused specifically on changes in well-known plant defense compounds,such as terpenoids or glucosinolates,induced responses can also affect the levels of nutritional compounds (e.g.sugars and amino acids)or other compounds (e.g.hormones)that are involved in induced defense.A new technology,‘metabolomics’,has recently emerged that enables a comprehensive analysis of allthe Corresponding author:Bezemer,T.M.(martijn.bezemer@wur.nl).Available online 29August 2005TRENDS in Ecology and Evolution Vol.20No.11November 2005 0169-5347/$-see front matter Q 2005Elsevier Ltd.All rights reserved.doi:10.1016/j.tree.2005.08.006metabolites of a plant [13](Box 2).Future aboveground–belowground studies should take advantage of this tech-nology,which could significantly improve our under-standing of the role of induced defenses in the complex multitrophic interactions associated with plants.Effects of belowground organisms on aboveground plant defenseWhen exposed to belowground organisms,plants can show several direct (e.g.production of toxins)and indirect (e.g.release of volatiles)defense responses in the foliage that can affect aboveground herbivores and disrupt multitrophic interactions.Direct defenseA range of belowground organisms that are directly associated with plant roots (e.g.insects,nematodes,root pathogens and mycorrhizal fungi)influence the concen-tration of plant defense compounds,such as terpenoids,glucosinolates or phenolics,in aboveground plant tissues [14–21].Because these compounds frequently have a negative effect on aboveground herbivores,the action of belowground organisms can influence the performance of aboveground organisms via changes in plant defense levels.Increases and decreases in aboveground defenseas a result of belowground herbivory have been reported,suggesting that there is a range of interactions between aboveground and belowground herbivores.We argue here that these differences in responses of the plant to root-associated soil organisms are related to the differences in their feeding habits.Several studies have shown that root chewing by belowground insects causes an increase in plant defense compounds in aboveground plant parts that is similar to that observed for foliar-feeding chewing insect larvae [14,15,19,20].Studies that use artificial root damage or hormone applications as a substitute for root feeding show similar induction patterns [14,17,18].Root and shoot chewers thus elicit similar induction pathways.Interest-ingly,the distribution of defense compounds between leaves in response to damage by root chewers can differ from that observed after foliar feeding [14].Such spatial changes in plant defense can have major implications for aboveground organisms feeding from the plant and,thus,for plant fitness (Box 3).Plant responses to root-feeding nematodes are more variable,and decreases and increases in concentrations of aboveground defense compounds have both been observed [16,22].The response depends not only on the suscepti-bility of the plant to nematodes,but also on the type of feeding behaviour [16,23].For example,endoparasitic sedentary root-knot and cyst nematode species,such as Meloidogyne and Heterodera spp.,establish a feeding cell,which elicits hormonal regulatory responses in the host plant;endoparasitic migratory and ectoparasitic plant-feeding nematodes,such as Pratylenchus and Tylenchorynchus ,do not form a cell and are likely to influence the host plant to a lesser extent [23,24].TRENDS in Ecology and Evolution Vol.20No.11November 2005618Plants that are infested by bacterial or fungal root patho-gens can also increase their defenses against shoot pathogens,even though the shoots are not yet infected, so-called‘systemic acquired resistance’(SAR)[20].The presence of non-pathogenic soil bacteria,however,can cause the faster accumulation of aboveground defenses once the shoots become infested with pathogenic bacteria or fungi. The enhanced responsiveness of induced responses above-ground by non-pathogenic root bacteria has been called ‘induced systemic resistance’(ISR)[25].Induction of SAR by pathogenic root-bacteria involves the salicylic(SA)signal-ing pathway,whereas induction of ISR by non-pathogenic soil bacteria is SA independent and requires functional jasmonic acid(JA)and ethylene(ET)pathways[20]. SAR and ISR act independently and in an additive way: plants in which there is simultaneous activation of both pathways experience enhanced levels of shoot protection against pathogens compared with plants with only SAR or ISR[20].Mycorrhizal fungi also form associations with plant roots.There are various types of such fungi(i.e.ectomy-corrhiza and arbuscular mycorrhiza)and,although they are commonly considered beneficial,they can also have negative or neutral effects on the growth of the host plant [26,27].Even closely related arbuscular mycorrhizal species can cause different effects within the host plant.A comparison of infection of roots of Medicago truncatula by two different arbuscular mycorrhizal species(Glomus spp.),for example,showed that,within the plant,several hundred genes are up-regulated specifically by only one of the two mycorrhizal species[28].Arbuscular mycorrhizal infection can cause an increase in aboveground defense compounds[29],whereas ectomycorrhizal infection can cause a decrease[30].Other studies,using different plant species,have shown that plant defense does not change following infection with arbuscular mycorrhiza or ecto-mycorrhiza[21,31].The variety of the effects of different types and species of mycorrhiza on plants could explain the variation in aboveground direct defenses(from a decrease,to none,to an increase in direct defense)in response to mycorrhizal infection[32].Although earthworms and other decomposers are not directly associated with plant roots,they can influence aboveground plant defense levels and shoot herbivore performance[31,33,34].A decline in foliar iridoid gluco-sides was observed,for example,in the presence of earth-worms[31]and probably occurs as a result of differences in the availability of nutrients to the plant.Via decompo-sition,earthworms can increase nitrogen availability in the soil,resulting in the plant investing more in growth and less in direct defense compounds.A recent study has shown that earthworms can increase lipoxygenase(lox) gene expression in the leaves[34].Lox is thefirst enzyme in the pathway that results in the signaling hormone JA, which is responsible for systemic defense induction[35]. Thus,the increase in lox expression caused by earthworm Figure II.Extrafloral nectar gland with nectar droplet(arrow)on a cotton leaf. The production of extrafloral nectar is an indirect defense response of the plant.TRENDS in Ecology and Evolution Vol.20No.11November2005619 activity belowground can influence aboveground plant defense responses directly.Indirect defenseRecent studies have shown that soil organisms also influence aboveground indirect defense responses,such as the emission of volatiles.Indirect defenses enable the plant to attract carnivores or enhance the effectiveness with which those carnivores attack herbivores.Brassica rapa turnips that are infested with rootfly larvae,for example,emit a specific shoot-produced volatile blend that attracts parasitoids of rootflies[36].Similar induction processes have been found in maize[9].We can thus assume that parasitoids that live aboveground as adults, but attack soil-dwelling hosts,have evolved the ability to detect shoot-emitted plant cues that indicate the presence of their hosts.However,predators and parasitoids of aboveground herbivores can also be influenced by below-ground organisms.Extrafloral nectar production is an indirect defense response that is found in many plant species.By producing extrafloral nectar in response to foliar herbivory,plants attract ants that subsequently feed on the insect herbivore[37].Gossypium herbaceum cotton plants exposed to root-feeding insects also show increased production of extrafloral nectar in the leaves(Box3). Other studies have shown that plants exposed to root herbivory by chewing herbivores experience increased visits from parasitoids of their aboveground herbivores [38,39].Although these studies did not explicitly measure induced responses,such as volatiles,the results indicate that aboveground volatile production is likely to increase after root herbivory given that parasitoids are known to use plant-emitted volatiles to locate their hosts.Similar effects on aboveground indirect defenses were found for symbiotic root interactions.The volatile blends released by plants with arbuscular mycorrhizal fungi were more attractive to aphid parasitoids than were the blends from plants without mycorrhiza[40].The herbivore (aphids),however,performed significantly worse on plants with mycorrhizal fungi.Parasitoids were thus attracted to the plants with the fewest hosts.The so-called‘cry for help’response of the plant is thought to be a reliable defense response of plants against aboveground herbi-vores[41],but these results suggest that belowground organisms confuse this system,because carnivores are attracted to the least-infested plants.An important question is how these indirect defense responses have evolved under natural conditions in which plants con-stantly interact with different types of organisms below-ground(Box4).Effects of aboveground organisms on belowground plant defenseSimilar to belowground organisms,foliar-feeding organ-isms can influence belowground direct and indirect plant defense responses.Although the effects of aboveground organisms on plant defense levels in roots are less well studied,the data suggest that the influence of above-ground organisms on direct belowground plant defense is less severe,but that the composition of the soil community can be altered by changes in indirect root defenses induced by aboveground herbivores.Direct defenseAboveground herbivory can influence the concentration of defense compounds in the roots.Foliar feeding by caterpillars,for example,caused a decrease in alkaloid concentrations in ragwort roots;these alkaloids are known to reduce the growth of pathogenic root fungi [18].One study reported an increase in hydroxamic acids in root exudates of rice,but only after repetitive defoli-ation[42].This result is of relevance,however,because hydroxamic acids are used by the plant to defend itself against herbivores and pathogens.Other studies using foliar-feeding insects[14],shoot hormone applications[17] or moderate artificial defoliation[43]showed no signifi-cant change in the levels of defense compounds in roots. The lack of induction of root defenses by shoot feeders might be due to the source–sink relationships within a plant,with plants increasing the amount of carbon allocated to root tissues after foliar damage[2,4],and thus investing more in root growth than in root defense.There are also several studies providing evidence, without identifying the defense compounds involved, that aboveground-induced responses influence root-associated organisms.Foliar application of JA to grape vine reduced the number of root-feeding grape phylloxera to about half the numbers on control plants[44].Appli-cation of SA to leaves of okra plants also halved the numbers of root-knot nematode that established on treated compared with control plants[45].However, shoot induction of SA pathways tripled the root infestation rate by endoparasitic migratory nematodes in barley[46].In addition to changes in internal defense levels, aboveground feeding,particularly by foliar-feeding insects,might also alter the quantity and quality of root exudates.Root exudates contain various organic sub-stances,such as sugars,amino acids and plant defense compounds[47],and attract root-feeding insects,bacteria, fungi and nematodes.Given that these exudates are released into the soil,they also influence soil organisms that live in the vicinity of the roots,but are not directly associated with them,such as decomposers[5].Root exudation can increase in response to foliar herbivory or defoliation[42,47,48],thus having a detrimental effect on the roots themselves(if root herbivores are attracted).Moreover,after severe defoliation,the concen-tration of defense compounds in root exudates can increase,possibly as a result of passive leakage from the roots[42,47].Indirect defenseRoot-feeding organisms are exposed to different types of predators and parasites,such as carnivorous nematodes, mites,fungi and insects[5,49].Carnivorous nematodes of insects(i.e.entomopathogenic nematodes),as well as insect parasitoids,are attracted to root exudates and volatile organic compounds(VOC)that are excreted by plant roots when they are damaged by root-chewing herbivores[8,9,36].Similar results have been found for predatory mites that are attracted to volatiles that areTRENDS in Ecology and Evolution Vol.20No.11November2005 620emitted by mite-infested tulip bulbs[50].Because above-ground herbivory can change the quantity and compo-sition of root exudates,it is likely that the attraction of entomopathogenic nematodes and other predators can also be altered by aboveground foliar feeders.We are not aware of any published work on such aboveground–belowground interactions affecting belowground induced indirect defense.Such interactions,however,could have far-reaching consequences for belowground multitrophic interactions.For example,they could result in a‘mis-match’,whereby predators are attracted to plants where their prey are not present;alternatively,root-feeding insects and other organisms that are not directly associated with the root,such as collembola or mites, can also be attracted by root exudates[47].Different types of organism can thus be attracted to roots of plants that sustain aboveground damage,resulting in more complex soil food webs associated with damaged than with undamaged plants.Because the stability of soil food webs is often related to the diversity of organisms that the food web consists of[51],aboveground organisms could affect the stability of belowground food webs.TRENDS in Ecology & EvolutionRoot-associated soil organisms Root-associatedsoil organisms Root-associated soilorganismsSoilSystemicresponseShoot-inducedresponsesRoot-inducedresponsesRoot-inducedresponsesinducedresponsesSystemicresponseSystemicresponseNon-root-associatedsoil organismsNon-root-associatedsoil organismsTRENDS in Ecology and Evolution Vol.20No.11November2005621Several studies have shown that adult parasitoids of root-feeding insects are also attracted to plants that are damaged by aboveground herbivores as a result of shoot-produced VOC that shoot-damaged plants emit[36,52]. These parasitoids live aboveground as adults but their larvae develop in soil-dwelling larvae or pupae.Thus, although belowground indirect plant defense efficacy appears to be affected by feeding activities of aboveground organisms,further empirical studies are needed to con-firm these patterns.Additional aboveground–belowground effects of plant defense compoundsPlant defense compounds can also influence aboveground–belowground interactions in ways that,strictly speaking, are not plant defense responses.For example,if foliar herbivory increases levels of defense compounds in leaves, these compounds can remain in dead leaf material and thus influence the decomposition behaviour and perform-ance of belowground decomposers[3,53].Moreover,com-positional changes in leaf VOCs or root exudates might trigger neighbouring plants to induce their defenses [54,55].This‘eaves-dropping’can result in either an increase in direct resistance against herbivores in neigh-bouring plants[54],or in enhanced attractiveness of these plants to aboveground parasitoids or predatory mites [55,56].Predators might also be attracted to the‘wrong’host plant(i.e.the undamaged one),and thus above-ground–belowground interactions via plant–plant com-munication could disrupt the reliability of indirect defense responses of the plant.Alternatively,this type of com-munication could lead to a collective odour plume and, thus,to a more effective attraction of small predators or parasitoids.Given that damaged plants appear to produce more VOC than do undamaged ones[57],differences in the odour gradient within the plume would lead the natural enemy to the correct plant.Conclusions and future directionsThere is increasing evidence that induced plant defense responses have a significant role in interactions between aboveground and belowground organisms.Both direct and indirect plant defenses are affected;for example,the interactions between root-and shoot-induced defenses can reduce the effectiveness of multitrophic relationships that are mediated by indirect defense responses.Based on this evidence,we identified three areas for future research on aboveground–belowground inter-actions.First,a substantial research effort has been expended on identifying which herbivores switch on par-ticular signaling pathways and how this affects defense compounds that are often part of well-characterized meta-bolic pathways.However,plants contain thousands of metabolites;many of these are important for organisms feeding on the plant,and can change following herbivory. Until recently,it was difficult to study these metabolites simultaneously;however,novel analytical and statistical techniques now enable researchers to study comprehen-sively the chemical phenotype(i.e.metabolome)of a plant (Box2).Such metabolomics studies have been predomi-nantly descriptive,but this technique offers unprecedented possibilities for understanding the interactions between plants and aboveground and belowground organisms. Recently,for the legume Lotus japonicus,the level of hundreds of known and unknown metabolites was described in nodules,roots,leaves andflowers[58]. Similar studies at the transcriptional level are underway for symbioses with mycorrhizal fungi[28].Future studies should address how metabolites in aboveground and belowground plant organs change after aboveground or belowground herbivory,and what the consequences are of changes in these metabolites for other associated organ-isms.There is great potential for studies that link complex changes in induction patterns to changes in multitrophic complexes,as well as to differences in whole-plantfitness.Second,the spatial and temporal aspects of induced defenses of plants to aboveground and belowground organisms need to be considered in more detail.The spatial distribution of defense compounds,for example among leaves,might differ depending on the compartment (aboveground or belowground)in which the induction is triggered.Additionally,there are temporal aspects of aboveground and belowground induced responses that must be considered when evaluating the ecological and evolutionary aspects of these interactions(Box4).Third,studies should go beyond individual interactions between single species pairs of aboveground and below-ground plant feeders and analyse the effects of soil and shoot multitrophic communities associated with plants. Indirect defense responses,both aboveground and below-ground,could become disrupted,with consequences for multitrophic interactions.Future work should address the question of whether the composition of indirect plant defense compounds differs following aboveground and belowground damage.For example,how do volatile blends that are released aboveground change when plants are damaged belowground,and what are the consequences for herbivores,their natural enemies,and the plant?These ‘side’effects might be ecologically important and,conse-quently,could be a significant selective force.Thus,the comprehensive analysis of metabolomic changes in roots and shoots in a multitrophic context is likely to contribute to a better understanding of the costs and benefits involved in the selection for induced responses in plants.In nature,plants are exposed almost constantly to aboveground and belowground herbivores. Given that such herbivores can influence each other via changes in the shared host plant,a better understanding of those changes will also help to disentangle the rela-tionships that occur between plants and their enemies.In agricultural systems,such knowledge could enhance the effectiveness of biological control programmes and might explain why some biological control programmes are suc-cessful whereas others fail.AcknowledgementsWe thank Richard Bardgett and Wim van der Putten for their encouragement to write this article,Wim van der Putten,Marcel Dicke, Jeffrey Harvey and Susanne Wurst for helpful comments,and Roel Wagenaar for providing Figure I in Box3.T.M.B.is supported by a fellowship from the Research School for Production Ecology and Resource Conservation,Wageningen University,the Netherlands,and N.M.v.D.by a VIDI grant,no.864–02–001,of the Netherlands Organization forTRENDS in Ecology and Evolution Vol.20No.11November2005 622Scientific Research.Publication3595NIOO-KNAW Netherlands Insti-tute of Ecology.References1Wardle,D.A.et al.(2004)Ecological linkages between aboveground and belowground biota.Science304,1629–16332Bardgett,R.D.et al.(1998)Linking above-ground and below-ground interactions:how plant responses to foliar herbivory influence soil organisms.Soil Biol.Biochem.30,1867–18783Bardgett,R.D.and Wardle,D.A.(2003)Herbivore-mediated linkages between aboveground and belowground communities.Ecology84, 2258–22684Wardle, D.A.(2002)Communities and Ecosystems.Linking the Aboveground and Belowground Components,Princeton University Press5van der Putten,W.H.et al.(2001)Linking above-and belowground multitrophic interactions of plants,herbivores,pathogens and their antagonists.Trends Ecol.Evol.16,547–5546Karban,R.and Baldwin,I.T.(1997)Induced Responses to Herbivory, University of Chicago Press7Agrawal, A.A.et al.(1999)Induced Plant Defenses Against Pathogens and Herbivores:Biochemistry,Ecology,and Agriculture, APS Press8van Tol,R.W.H.M.et al.(2001)Plants protect their roots by alerting the enemies of grubs.Ecol.Lett.4,292–2949Rasmann,S.et al.(2005)Recruitment of entomopathogenic nema-todes by insect-damaged maize roots.Nature434,732–73710Bardgett,R.D.et al.(2005)Linking aboveground and belowground ecology:a temporal approach.Trends Ecol.Evol..doi:10.1016/j.tree.2005.08.00511De Deyn,G.B.and Van der Putten,W.H.(2005)Linking aboveground and belowground diversity.Trends Ecol.Evol.doi:10.1016/j.tree.2005.08.00912Bezemer,T.M.et al.(2005)Soil community composition drives aboveground plant–herbivore–parasitoid interactions.Ecol.Lett.8, 652–66113Bino,R.J.et al.(2004)Potential of metabolomics as a functional genomics tool.Trends Plant Sci.9,418–42514Bezemer,T.M.et al.(2004)Above-and below-ground terpenoid aldehyde induction in cotton,Gossypium herbaceum,following root and leaf injury.J.Chem.Ecol.30,53–6715Bezemer,T.M.et al.(2003)Interactions between above-and below-ground insect herbivores as mediated by the plant defense system.Oikos101,555–56216van Dam,N.M.et al.(2005)Root herbivory reduces growth and survival of the shoot feeding specialist Pieris rapae on Brassica nigra.Entomol.Exp.Appl.115,161–17017van Dam,N.M.et al.(2004)Interactions between aboveground and belowground induction of glucosinolates in two wild Brassica species.New Phytol.161,801–81018Hol,W.G.H.et al.(2004)Root damage and aboveground herbivory change concentration and composition of pyrrolizidine alkaloids of Senecio jacobaea.Basic Appl.Ecol.5,253–26019Birch,A.N.E.et al.(1992)Glucosinolate responses of swede,kale, forage and oilseed rape to root damage by turnip rootfly(Delia floralis)larvae.J.Sci.Food Agric.60,1–920Pieterse, C.M.J.et al.(2002)Signalling in rhizobacteria-induced systemic resistance in Arabidopsis thaliana.Plant Biol.4,535–544 21Manninen,A.M.et al.(1998)Susceptibility of ectomycorrhizal and nonmycorrhizal Scots pine(Pinus sylvestris)seedlings to a generalist insect herbivore,Lygus rugulipennis,at two nitrogen availability levels.New Phytol.140,55–6322van Dam,N.M.et al.(2003)Interactions between aboveground and belowground induced responses against phytophages.Basic Appl.Ecol.4,63–7723Mateille,T.(1994)Biology of the plant nematode relationship: physiological changes and the defense mechanism of plants.Nema-tologica40,276–31124Zinov’eva,S.V.et al.(2004)Biochemical aspects of plant interactions with phytoparasitic nematodes:a review.Appl.Biochem.Microbiol.40,111–11925Conrath,U.et al.(2002)Priming in plant–pathogen interactions.Trends Plant Sci.7,210–21626Klironomos,J.N.(2003)Variation in plant response to native and exotic arbuscular mycorrhizal fungi.Ecology84,2292–230127Gange,A.C.and Brown,V.K.(2001)All mycorrhizas are not equal.Trends Ecol.Evol.16,671–67228Hohnjec,N.et al.(2005)Overlaps in the transcriptional profiles of Medicago truncatula roots inoculated with two different Glomus fungi provide insights into the genetic program activated during arbuscular mycorrhiza.Plant Physiol.137,1283–130129Gange,A.C.and West,H.M.(1994)Interactions between arbuscular mycorrhizal fungi and foliar-feeding insects in Plantago lanceolata L.New Phytol.128,79–8730Rieske,L.K.et al.(2003)Foliar chemistry and gypsy moth,Lymantria dispar(L.),herbivory on pure American chestnut,Castanea dentata (Fam:Fagaceae),and a disease-resistant hybrid.Environ.Entomol.32,359–36531Wurst,S.et al.(2004)Combined effects of earthworms and vesicular-arbuscular mycorrhizas on plant and aphid performance.New Phytol.163,169–17632Strack,D.et al.(2003)Arbuscular mycorrhiza:biological,chemical, and molecular aspects.J.Chem.Ecol.29,1955–197933Wurst,S.et al.(2004)Earthworms and litter distribution affect plant-defensive chemistry.J.Chem.Ecol.30,691–70134Blouin,M.et al.(2005)Belowground organism activities affect plant aboveground phenotype,inducing plant tolerance to parasites.Ecol.Lett.8,202–20835Stratmann,J.W.(2003)Long distance run in the wound response–jasmonic acid is pulling ahead.Trends Plant Sci.8,247–25036Neveu,N.et al.(2002)Systemic release of herbivore-induced plant volatiles by turnips infested by concealed root-feeding larvae Delia radicum L.J.Chem.Ecol.28,1717–173237Wa¨ckers,F.L.and Bezemer,T.M.(2003)Root herbivory induces an above-ground indirect defence.Ecol.Lett.6,9–1238Masters,G.J.et al.(2001)Host-plant mediated effects of root herbivory on insect seed predators and their parasitoids.Oecologia 127,246–25039Poveda,K.et al.(2003)Effects of below-and above-ground herbivores on plant growth,flower visitation and seed set.Oecologia135, 601–60540Guerrieri,E.et al.(2004)Do interactions between plant roots and the rhizosphere affect parasitoid behaviour?Ecol.Entomol.29,753–756 41Vet,L.E.M.and Dicke,M.(1992)Ecology of infochemical use by natural enemies in a tritrophic context.Annu.Rev.Entomol.37, 141–17242Collantes,H.G.et al.(1999)Defoliation affects chemical defenses in all plant parts of rye seedlings.J.Chem.Ecol.25,491–49943Collantes,H.G.et al.(1998)Changes in growth and chemical defences upon defoliation in maize.Phytochemistry49,1921–192344Omer, A.D.et al.(2000)Jasmonic acid induced resistance in grapevines to a root and leaf feeder.J.Econ.Entomol.93,840–845 45Nandi, B.et al.(2003)Salicylic acid-induced suppression of Meloidogyne incognita infestation of okra and cowpea.Nematology 5,747–75246Sonnemann,I.et al.(2002)Does induced resistance in plants affect the belowground community?Appl.Soil Ecol.21,179–18547Grayston,S.J.et al.(1997)Rhizosphere carbonflow in trees,in comparison with annual plants:the importance of root exudation and its impact on microbial activity and nutrient availability.App.Soil Ecol.5,29–5648Holland,J.N.et al.(1996)Herbivore-induced changes in plant carbon allocation:assessment of below-ground Cfluxes using Carbon-14.Oecologia107,87–9449Strong, D.R.et al.(1996)Entomopathogenic nematodes:natural enemies of root-feeding caterpillars on bush lupine.Oecologia108, 167–17350Aratchige,N.S.et al.(2004)Below-ground plant parts emit herbivore-induced volatiles:olfactory responses of a predatory mite to tulip bulbs infested by rust mites.Exp.Appl.Acarol.33,21–3051De Ruiter,P.C.et al.(1995)Energetics,patterns of interaction strengths,and stability in real ecosystems.Science269,1257–1260 52Brown,P.E.and Anderson,M.(1999)Factors affecting ovipositor probing in Trybliographa rapae,a parasitoid of the cabbage rootfly.Entomol.Exp.Appl.93,217–225TRENDS in Ecology and Evolution Vol.20No.11November2005623 。
放牧干扰下高寒草甸物种和生活型丰富度与地上及地下生物量的关系

第 38卷第 8期 2018年 4月
生 态 学 报
ACTA ECOL0GICA SINICA
Vo1.38.No.8 Apr.,2018
D O I: 10.5846/stxb20170王贵珍 ,刘丽 ,杜国祯 ,花立 民.放牧干扰下高寒草甸物种和 生活型 丰富度与地 上及地下 生物量 的关 系.生 态学报 ,2018,38(8):
摘要 :明晰放牧干扰下高寒草甸植物 丰富度 与生物量的相关关系 ,为草地植物不 同生长 时期生物量 的预测 提供依据 。设 置 6个 放牧强度样地 ,连续 3a放牧 ,2014年进行 3个季节 (6月 、8月、l0月 )的植物 丰富度和地上 、地下 生物量调查 ,对 比分 析放牧 干 扰下物种和生活型 丰富度(生活型 的种类 )分别与地上 、地下生物量 的相关关 系。结果表 明 :(1)物种和生活 型丰富度与地上 生 物量 均受放牧强度 的显著影 响 ,物种丰 富度仅在 8月与放牧强度显著负相关 ,生活型丰富度在 10月 随放牧强度单 峰变化 ,地 上 生物量在不 同季节均与放牧 强度 显著负相关 ,而地下 生物 量与放牧 强度无 关。 (2)物种丰 富度与地上 和地 下生物量 均受季 节 的显著影响 。物种 丰富度和地上生物量仅在低强度放 牧区随季 节呈单峰 变化 ,地下 生物量在 中等强度 放 牧 区随季节 呈单峰 变 化 ;生活型丰 富度与 季节无关 。(3)放牧干扰前物种和生活 型丰 富度 与地上 和地下生物量均显著 正相关 。3a放牧后仅 在 8月 , 物种 丰富度只与地上生物量显著正相关 ,生活型丰富度与地上 和地下生物量均显著 正相关 。(4)对于不 同放 牧强度 ,物种丰 富 度仅 在低 强度放牧 区与地上生物量显 著正 相关 ,而生活型 丰富度在所有放牧强度 区均 与地上生 物量显著 正相关 。综上所述 ,放 牧干扰扰 乱了高寒草甸丰富度与生物量之 间的关系 ,尤其影 响了物 种丰 富度 与地下生物量 之间 的相关 关系 。生活 型丰富度与 地上生物 量之间的显著关系不受放牧强度 干扰 .使生活型丰 富度在预测生物量方 面表现 出优势 。 关键词 :高寒草甸 ;放牧干扰 ;物种 丰富度 ;生活 型丰 富度 ;生物量
遮阴对大豆农艺性状的影响
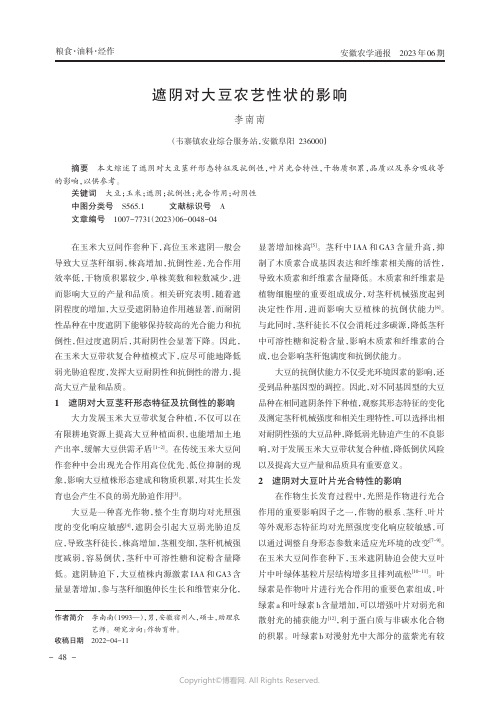
安徽农学通报2023年06期粮食·油料·经作遮阴对大豆农艺性状的影响李南南(韦寨镇农业综合服务站,安徽阜阳236000)摘要本文综述了遮阴对大豆茎秆形态特征及抗倒性,叶片光合特性,干物质积累,品质以及养分吸收等的影响,以供参考。
关键词大豆;玉米;遮阴;抗倒性;光合作用;耐阴性中图分类号S565.1文献标识号A文章编号1007-7731(2023)06-0048-04在玉米大豆间作套种下,高位玉米遮阴一般会导致大豆茎秆细弱,株高增加,抗倒性差,光合作用效率低,干物质积累较少,单株荚数和粒数减少,进而影响大豆的产量和品质。
相关研究表明,随着遮阴程度的增加,大豆受遮阴胁迫作用越显著,而耐阴性品种在中度遮阴下能够保持较高的光合能力和抗倒性,但过度遮阴后,其耐阴性会显著下降。
因此,在玉米大豆带状复合种植模式下,应尽可能地降低弱光胁迫程度,发挥大豆耐阴性和抗倒性的潜力,提高大豆产量和品质。
1遮阴对大豆茎秆形态特征及抗倒性的影响大力发展玉米大豆带状复合种植,不仅可以在有限耕地资源上提高大豆种植面积,也能增加土地产出率,缓解大豆供需矛盾[1-2]。
在传统玉米大豆间作套种中会出现光合作用高位优先、低位抑制的现象,影响大豆植株形态建成和物质积累,对其生长发育也会产生不良的弱光胁迫作用[3]。
大豆是一种喜光作物,整个生育期均对光照强度的变化响应敏感[4],遮阴会引起大豆弱光胁迫反应,导致茎秆徒长,株高增加,茎粗变细,茎秆机械强度减弱,容易倒伏,茎秆中可溶性糖和淀粉含量降低。
遮阴胁迫下,大豆植株内源激素IAA和GA3含量显著增加,参与茎秆细胞伸长生长和维管束分化,显著增加株高[5]。
茎秆中IAA和GA3含量升高,抑制了木质素合成基因表达和纤维素相关酶的活性,导致木质素和纤维素含量降低。
木质素和纤维素是植物细胞壁的重要组成成分,对茎秆机械强度起到决定性作用,进而影响大豆植株的抗倒伏能力[6]。
与此同时,茎秆徒长不仅会消耗过多碳源,降低茎秆中可溶性糖和淀粉含量,影响木质素和纤维素的合成,也会影响茎秆饱满度和抗倒伏能力。
大气增温对杉木幼树叶片及细根生理特征的影响

第40卷第21期2020年11月生态学报ACTAECOLOGICASINICAVol.40,No.21Nov.,2020基金项目:国家自然科学基金项目(31500408);国家重大基础研究计划课题(2014CB954003)收稿日期:2019⁃03⁃30;㊀㊀网络出版日期:2020⁃09⁃01∗通讯作者Correspondingauthor.E⁃mail:xdc104@163.comDOI:10.5846/stxb201903300614叶旺敏,熊德成,杨智杰,张秋芳,刘小飞,高艳丽,胥超,杨玉盛.大气增温对杉木幼树叶片及细根生理特征的影响.生态学报,2020,40(21):7681⁃7689.YeWM,XiongDC,YangZJ,ZhangQF,LiuXF,GaoYL,XuC,YangYS.EffectsofatmosphericwarmingonphysiologicalcharacteristicsofleavesandfinerootsofCunninghamialanceolatasaplings.ActaEcologicaSinica,2020,40(21):7681⁃7689.大气增温对杉木幼树叶片及细根生理特征的影响叶旺敏1,2,熊德成1,2,∗,杨智杰1,2,张秋芳1,2,刘小飞1,2,高艳丽1,2,胥㊀超1,2,杨玉盛1,21福建师范大学地理科学学院,福州㊀3500072湿润亚热带山地生态国家重点实验室培育基地,福州㊀350007摘要:为揭示亚热带森林对未来全球变暖的生理响应特征,本研究以杉木为研究对象,利用开顶式增温方式模拟气候变暖,研究其对叶片和细根丙二醛含量㊁活性氧代谢㊁渗透调节物质含量以及抗氧化酶活性的影响㊂研究结果显示:(1)增温显著增加杉木叶片和细根的丙二醛含量,且叶片丙二醛含量显著高于细根,说明增温加剧了杉木叶片和细根氧化损伤,且叶片氧化损伤程度高于细根;(2)增温后,杉木叶片脯氨酸和可溶性蛋白含量降低,细根脯氨酸和可溶性蛋白含量则增加;(3)增温显著提高了杉木叶片过氧化物酶活性,对杉木细根抗氧化酶活性无显著影响;(4)增温后,杉木叶片和细根活性氧含量未发生显著变化,杉木叶片活性氧含量显著高于细根㊂综合分析表明,尽管增温增加了杉木叶片和细根的氧化损伤,但杉木可以通过提高抗氧化保护酶活性(叶片)和积累较多的渗透调节物质(细根)来维持体内活性氧代谢平衡㊂可见,杉木地上和地下部分器官间的相互合作与协调使杉木能有效地适应高温环境㊂关键词:大气增温;杉木;渗透调节物质;抗氧化酶活性;膜酯过氧化EffectsofatmosphericwarmingonphysiologicalcharacteristicsofleavesandfinerootsofCunninghamialanceolatasaplingsYEWangmin1,2,XIONGDecheng1,2,∗,YANGZhijie1,2,ZHANGQiufang1,2,LIUXiaofei1,2,GAOYanli1,2,XUChao1,2,YANGYusheng1,21SchoolofGeographicalSciences,FujianNormalUniversity,Fuzhou350007,China2CultivationBaseofStateKeyLaboratoryofHumidSubtropicalMountainEcology,Fuzhou350007,ChinaAbstract:Inordertorevealtheresponseofphysiologicalcharacteristicsofsubtropicalforeststoglobalwarminginthefuture,theCunninghamialanceolata(C.lanceolata)wastakenastheresearchobject,theopen⁃topheatingmethodwasusedtosimulateclimatewarmingtostudythecontentsofmalondialdehyde(MDA),activeoxygenmetabolism,andpenetrationinleavesandfineroots.Theresultsshowedthat(1)warmingsignificantlyincreasedthecontentsofMDAintheleavesandfineroots.AlsothecontentofMDAinleaveswashigherthanthatoffineroots,Theseindicatedthatoxidativestressenhancedtheoxidativedamageofleavesandfineroots,andthedegreeofoxidativedamageofleaveswashigherthanfineroots;(2)warmingincreasedthecontentsofprolineandsolubleproteininfineroots,butdecreasedthecontentsofprolineandsolubleproteininleaves;(3)warmingincreasedtheperoxidaseactivityoftheleavessignificantly,buthadnosignificanteffectontheantioxidantenzymeactivityofthefineroots;(4)theactiveoxygencontentsofleavesandfinerootshadnosignificantchangeafterwarming,buttheactiveoxygencontentofleaveswasobviouslyhigherthanthatoffineroots.2867㊀生㊀态㊀学㊀报㊀㊀㊀40卷㊀Comprehensiveanalysisshowedthatalthoughwarmingincreasedtheoxidativedamageofleavesandfineroots,C.lanceolatasaplingscouldmaintaintheirownactiveoxygenmetabolismbalancebyincreasingtheantioxidantenzymesactivityinleavesandaccumulatingmoreosmoticadjustmentsubstancesinfineroots.ItcanbeseenthatthecooperationandcoordinationbetweentheabovegroundandbelowgroundorganswerehelpfulforC.lanceolatasaplingstoadapttothehightemperatureenvironmenteffectively.KeyWords:atmosphericwarming;Cunninghamialanceolata;osmoticadjustmentsubstances;antioxidantenzymeactivities;membraneesterperoxidation.自工业革命以来,由于CO2等温室气体的大量排放,导致全球气候变暖日渐加剧㊂据IPCC第五次报告指出,预计到21世纪末,全球大气温度可能升高0.3 4.8ħ[1]㊂森林是地球陆地生态系统的重要组分,具有较高的生物生产力和生物多样性[2],研究森林生态系统对全球气候变暖响应模式已成为当代生态学研究的热点问题之一㊂以往研究结果显示,由于高纬度及高寒地区植物所处环境温度普遍低于最适生长温度值,适当增温可以增加其净光合速率,加快物候生长节律并延长生长季,以促进植物地上地下生物量的积累[3⁃4]㊂而就亚热带地区而言,由于本身温度较高,该地区森林所处的环境温度可能已接近阈值,在未来全球变暖的趋势下,可能对亚热带植物的生长及生理学特性造成剧烈影响,进而影响森林生产力[5⁃6],但有关亚热带森林对气候变暖的响应报道仍十分有限㊂抗氧化特征和渗透调节是植物对外界环境适应的综合体现㊂正常环境下,植物细胞进行有氧代谢生成的活性氧类物质与抗氧化防御机制(酶促抗氧化剂和非酶促抗氧化剂)对其的清除能力处在氧化还原的动态平衡[7]㊂但当外界环境胁迫程度超过植物抗氧化防御系统清除能力,便会引起活性氧的大量积累,导致植物细胞脂质㊁蛋白质㊁核酸㊁光合色素及酶的氧化损伤,影响植物正常代谢功能[8⁃9]㊂目前,关于植物在温度升高下生理响应和适应策略的研究已有很多,但多数研究都局限于植物单个器官或组织上㊂植物不同器官组织结构不同,所处的微环境亦有所差异,因此,对逆境响应机制可能不同,甚至相反㊂例如:Roessner等[10]研究发现,大麦(Hordeumvulgare)同一品种不同器官间生理代谢差异大于品种间差异㊂Skliros等[11]认为,植物不同器官能够合成各种特异代谢化合物,它们之间相互协作,共同抵御外界环境胁迫㊂白羽扇豆(Lupinusalbus)茎对其耐旱策略的贡献最大[12],而细根则是杠柳(Periplocasepium)适应干旱环境的重要器官[13]㊂由此可见,仅对植物单一器官进行研究,很难全面地揭示植物适应高温环境的策略,有必要对高温条件下植物体不同器官同时进行研究,以更好地理解植物适应增温的机制和策略㊂杉木(Cunninghamialanceolata)作为亚热带地区主要造林树种之一,广泛分布于我国南方16个省区,面积占世界人工林面积的6%,我国人工林面积的19%㊁蓄积量的25%,在我国人工林生产中占有重要地位[14]㊂为此,本文以杉木为研究对象,通过在福建三明森林生态系统与全球变化研究站 陈大观测点内开展开顶式(OTC)模拟增温实验,以探究增温对杉木地上部分(叶片)和地下部分(细根)的渗透调节物质㊁保护酶活性及丙二醛含量的影响,以深入认识亚热带经济树种生理代谢特征对未来全球变暖的响应㊂1㊀材料与方法1.1㊀样地基本概况本试验地点位于福建三明森林生态系统与全球变化研究站 陈大观测点(26.19ʎN,117.36ʎE),该地区平均海拔300m,年均气温19.1ħ,年均降水量1749mm,年均蒸发量1580mm,相对湿度81%,属中亚热带季风气候,土壤为黑云母花岗岩发育的红壤㊂1.2㊀研究方法实验设置增温(W)和对照(CT)两种处理,每种处理共6个重复,共计12个小区㊂每个小区面积2.5mˑ2.5m,深0.5m㊂小区内土壤均取自观测站附近杉木人工林(0 40cm),填土过程主要是将取回的土壤去除根系和沙石,充分混合均匀后分别填入各小区内,以此尽可能的消除土壤的异质性㊂增温处理采用开顶式(OTC)大气增温,于2014年10月份在各个实验小区周边建立增温室,增温室采用不锈钢管架设而成,顶部半敞开,底部面积16m2,顶部露天面积约10m2,高度4.8m,框架搭建完成后采用进口塑料薄膜覆盖,依次相连建立6个大气增温室㊂于各个实验小区地表下10cm处布设一个土壤温㊁湿度传感器用以监测土壤温度及水分变化,大气温度监测主要采用大气温度记录仪(MicroLite5032P⁃RH),降雨量采用气象站监测数据㊂于2015年1月,将5棵一年生三代杉木幼苗种植于各小区,用号码牌标记各个树苗,并每半个月对杉木树高㊁地径㊁冠幅等指标进行观测㊂于2016年11月,在对照(CT)和OTC增温(W),选取杉木幼树健康且向阳叶片进行随机采集,阳生叶片取样主要参照等Huang等[15]的方法㊂细根取样采用土芯法,于每个实验小区各取6个土钻,土钻直径3.5cm,取样深度为10cm,尽量保证在小区中心和边缘均有取样点以做到随机取样,将所取叶片和细根用液氮保存并迅速带回实验室进行测定㊂表1㊀不同处理杉木初始生长特征参数Table1㊀InitialgrowthcharacteristicparametersofdifferenttreatmentsofCunninghamialanceolata处理Treatment树高Height/cm地径Grounddiameter/mm冠幅Crownwidth/cm根长Rootlength/cm对照Control52.70ʃ2.24.78ʃ0.3424.89ʃ1.4532.54ʃ0.89增温Warming53.22ʃ1.74.81ʃ0.2625.83ʃ2.0334.33ʃ1.211.3㊀测定项目与方法1.3.1㊀叶片和细根活性氧类物质的测定超氧阴离子自由基的测定采用羟氨氧化法,利用紫外分光光度计于550nm下测定吸光度,具体操作参照柯德森等[16]㊂过氧化氢含量的测定参照Prochazkova等[17]方法,1.3.2㊀叶片和细根抗氧化酶的测定超氧化物歧化酶活性的测定采用黄嘌呤氧化酶法,每毫克蛋白抑制1mL反应液的50%时对应的SOD量作为1个酶活性单位(U),具体操作参照Giannopolitis和Ries[18]方法㊂过氧化物酶活性采用愈创木酚法测定,于波长420nm处测定吸光值,具体操作参照Kochhar等[19]方法㊂过氧化氢酶活性参照Trevor等[20]方法进行测定㊂抗坏血酸含量和抗坏血酸过氧化物酶活性采用2,4-二硝基苯肼法,具体操作参照Nakano和Asada[21]方法㊂1.3.3㊀叶片和细根丙二醛㊁渗透调节物质含量的测定丙二醛含量采用硫代巴比妥酸(TBA)法测定,根据532nm下的吸光值减去600nm下最小吸光值从而计算丙二醛含量[22]㊂可溶性蛋白浓度采用考马斯亮蓝法进行测定[23]㊂脯氨酸(Pro)含量的测定:采用酸性茚三酮显色法,具体操作参照Walter和Lindsley[24]方法㊂1.4㊀数据分析利用Excel2013对所有数据进行处理计算,使用统计软件SPSS20.0中单因素方差分析,对增温和对照处理中杉木叶片和细根活性氧类物质㊁抗氧化酶活性以及渗透调节物质进行显著性分析,并比较同种处理叶片和细根生理的特征差异,显著性水平设定为P=0.05㊂采用Origin9.0完成制图㊂2㊀结果与方法2.1㊀空气温度及土壤温湿度由图1可以看出,OTC处理使大气温度(4 11月)平均增加1.12ħ,土壤温度平均增加0.26ħ,土壤含水量相对比例降低为12.10%㊂3867㊀21期㊀㊀㊀叶旺敏㊀等:大气增温对杉木幼树叶片及细根生理特征的影响㊀图1㊀大气温度,土壤温度及土壤水分变化Fig.1㊀Airtemperature,soiltemperatureandmoistureCT:对照Control;W:增温处理Warmingtreatment2.2㊀增温对杉木幼树生长特征的影响增温对杉木生长特征均无显著影响(图2)㊂对照处理中杉木幼树树高和地径分别为297.3cm㊁54.7mm;增温处理中杉木幼树树高和地径分别为处理分别为310.9cm㊁51mm㊂图2㊀增温和对照处理杉木生长特征Fig.2㊀ThegrowthcharacteristicofCunninghamialanceolate误差线上的不同大写字母表示两种处理杉木树高和地径差异显著(P<0.05)2.3㊀增温对杉木幼树叶片和细根活性氧类物质的影响活性氧类物质是植物进行有氧代谢的必然产物,包括超氧阴离子自由基㊁羟自由基和过氧化氢等,具有超强的氧化能力㊂由图3可以看出,增温处理均未对杉木叶片㊁细根超氧阴离子自由基和过氧化氢浓度产生显著影响(P>0.05)㊂另外,无论增温还是对照处理下,杉木幼树叶片超氧阴离子自由基和过氧化氢浓度均显著4867㊀生㊀态㊀学㊀报㊀㊀㊀40卷㊀高于细根(P<0.05)㊂图3㊀增温对杉木幼树叶片、细根活性氧类物质的影响Fig.3㊀EffectsofwarmingonleavesandfinerootactiveoxygenicspeciesofCunninghamialanceolatasaplings误差线上的不同大写字母表示同种处理杉木叶片和细根差异显著(P<0.05);误差线上不同小写字母表示杉木叶片和细根在不同处理差异显著(P<0.05)2.4㊀增温对杉木幼树叶片和细根抗氧化酶活性的影响超氧化物歧化酶㊁过氧化氢酶㊁过氧化物酶㊁抗坏血酸过氧化物酶是植物体内重要的4种保护酶,抗坏血酸则能直接清除植物体内活性氧㊂由图4可知,增温较对照相比,杉木叶片过氧化氢酶㊁抗坏血酸过氧化物酶活性和抗坏血酸含量分别提高了13.9%(P>0.05)㊁9.2%(P>0.05)和4.2%(P>0.05),过氧化物酶显著提高42.4%(P<0.05),但超氧化物歧化酶活性相比于对照则降低9.2%(P>0.05);细根超氧化物歧化酶和抗坏血酸过氧化物歧化酶活性较对照均有增加的趋势,分别增加9.2%(P>0.05)和8.9%(P>0.05),增温后杉木细根过氧化氢酶和过氧化物酶活性分别降低了20.7%(P>0.05)和15.3(P>0.05),细根抗坏血酸含量无显著差异(P>0.05)㊂同种处理下,杉木细根抗坏血酸过氧化物酶活性和抗坏血酸含量均高于叶片(P>0.05),过氧化氢酶显著高于叶片(P<0.05),细根过氧化物酶活性则显著低于叶片(P<0.05),另外,对照处理中,杉木叶片和细根超氧化物歧化酶活性无显著差异㊂增温后,细根超氧化物歧化酶活性显著大于叶片(P<0.05)㊂2.5㊀增温对杉木幼树叶片㊁细根渗透调节物质及丙二醛含量的影响可溶性蛋白和脯氨酸含量是植物体内重要的两种渗透调节物质,由图5看出,增温后杉木叶片可溶性蛋白和脯氨酸含量均降低,分别降低5.5%(P>0.05)和10.3%(P>0.05),细根可溶性蛋白和脯氨酸含量则分别增加了9.2%(P>0.05)和9.2%(P>0.05);增温和对照处理下杉木叶片可溶性蛋白和脯氨酸均显著高于叶片;本研究中,增温显著增加杉木叶片和细根丙二醛含量(P<0.05),其中叶片提高了19.7%,细根提高25.5%(P<0.05);增温和对照处理下杉木叶片丙二醛含量显著高于细根(P<0.05),说明杉木叶片氧化损伤程度高于细根㊂3㊀讨论3.1㊀增温对杉木叶片㊁细根活性氧代谢和丙二醛含量的影响植物进行有氧代谢时体内通常会积累大量活性氧类物质,该类物质会引起植物细胞膜酯和蛋白的氧化损伤,破坏植物细胞膜的结构及功能的稳定性[25⁃26]㊂丙二醛是植物细胞膜脂过氧化作用的最终产物,用以表征细胞膜受损程度以及植物抗逆性强弱[27]㊂本研究发现,增温处理并未导致杉木叶片和细根超氧阴离子自由基和过氧化氢浓度发生显著变化(图3),但杉木叶片和细根丙二醛含量均显著增加(图5)㊂可能原因在于夏季高温胁迫降低了杉木叶片光合能力,使蛋白质合成受限及抗氧化酶活性降低,加剧叶片氧化损伤,进而影响光合碳向地下部分输送过程,导致可供杉木细根代谢生长的能源物质降低,亦使细根膜脂过氧化加剧㊂而至11月份,高温胁迫程度降低,杉木可以通过自身物质合成以及诱导激活抗氧化防御系统,使体内活性氧类物5867㊀21期㊀㊀㊀叶旺敏㊀等:大气增温对杉木幼树叶片及细根生理特征的影响㊀图4㊀增温对杉木幼树叶片、细根抗氧化酶活性的影响Fig.4㊀EffectsofwarmingonantioxidantenzymeactivitiesinleavesandfinerootsofCunninghamialanceolatasaplings质维持在原有水平㊂本研究还发现无论增温还是对照处理下,杉木叶片超氧阴离子自由基和过氧化氢浓度均显著高于细根(图3),且叶片丙二醛含量亦显著高于细根(图5),这与肖群英等[28]和周瑞莲等[29]研究结果相似㊂可见,杉木叶片氧化损伤程度高于细根㊂在光照辐射下,杉木叶片光合和呼吸强度较高产生的活性氧类物质较多是导致其丙二醛含量显著高于细根的主要原因㊂同时也表明细根是杉木适应高温环境的重要器官,器官间的生理整合作用与协调使其能有效地适应的高温环境㊂3.2㊀增温对杉木叶片㊁细根渗透调节物质的影响渗透调节被认为是植物对逆境胁迫的一种重要生理适应策略[30],可溶性蛋白和脯氨酸是植物体内主要渗透调节物质㊂在遭受高温胁迫时,植物体内通常积累较多的脯氨酸和可溶性蛋白,以平衡细胞渗透势,维持细胞正常膨压,从而适应外界条件的变化[31⁃32]㊂本研究中,增温较对照相比,杉木细根脯氨酸和可溶性蛋白均有所提高(图5),这可能是由于增温引起土壤含水量降低,杉木细根通过合成较多的脯氨酸和可溶性蛋白,来维持正常的渗透调节功能[33]㊂另外,增温对杉木叶片脯氨酸和可溶性蛋白的积累却存在一定的抑制作用(图5),一方面可能因为叶片直接与空气接触,大气增温引起的叶温增幅较大,使其蛋白水解酶活性增加㊂另一方面则可能是由于杉木叶片在经历过夏季高温胁迫后,叶片体内碳水化合物的供应受阻,影响了谷氨酸的合成,进一步抑制脯氨酸的积累[34]㊂另外,本研究发现,无论增温还是对照下,杉木叶片可溶性蛋白和脯氨酸含量均显著高于细根(图5),可见杉木叶片渗透调节能力高于细根㊂6867㊀生㊀态㊀学㊀报㊀㊀㊀40卷㊀图5㊀增温对杉木幼树叶片、细根渗透调节物质和丙二醛含量的影响Fig.5㊀EffectsofwarmingonleafandfinerootsosmoticadjustmentsubstancesubstancesandmalondialdehydecontentinCunninghamialanceolatasaplings3.3㊀增温对杉木叶片㊁细根抗氧化酶活性的影响植物体内抗氧化系统由酶促和非酶促抗氧化剂共同组成[35]㊂超氧化物歧化酶通常被认为是植物抗氧化系统的第一道防线,超氧阴离子先由超氧化物歧化酶歧化为过氧化氢和氧气,再由过氧化氢酶㊁过氧化物酶及抗坏血酸过氧化物酶等联合作用将过氧化氢转化为水和氧气,另外抗坏血酸则能直接清除植物体内活性氧[36]㊂吴永波和叶波[37]发现构树幼苗在高温胁迫下,叶片超氧化物歧化酶㊁过氧化物酶及过氧化氢酶活性均显著提高,但过度高温胁迫则破坏抗氧化酶活性,抑制酶表达㊂郭盈添[38]等亦发现金露梅幼苗叶片能通过增强过氧化物酶㊁过氧化氢酶和抗坏血酸过氧化物酶活性以清除因高温胁迫积累的活性氧类物质,但随高温胁迫时间延长,各项抗氧化酶活性呈下降趋势㊂本研究中,增温后杉木叶片过氧化物酶活性显著提高(图4),叶片过氧化氢酶㊁抗坏血酸过氧化物酶活性和抗坏血酸含量(图4)也均有不同程度的增加趋势,超氧化物歧化酶活性则略微降低(图4),表明植物叶片不同种类抗氧化酶活性对温度的敏感性存在差异㊂增温后杉木叶片过氧化物酶活性显著提高是导致叶片活性氧类物质无显著变化的主导因素㊂细根是植物吸收养分和水分的主要器官,也是植物根系中最敏感的部分[39]㊂本研究中,增温后杉木细根超氧化物歧化酶和抗坏血酸过氧化物酶活性和抗坏血酸含量均有增加趋势(图4),过氧化氢酶和过氧化物酶活性则降低(图4),但总体而言变化并不显著㊂这可能是由于地上增温方式并未对根区环境造成明显影响,这与李伟成等[40]模拟高温对髯毛箬竹根系保护酶系统的研究结果一致㊂增温后杉木细根超氧化物歧化酶和抗坏血酸过氧化物酶活性提高,与较高的脯氨酸含量共同作用,增加了细根抗氧化能力,使细根活性氧物质维持在原有水平㊂由此可见,杉木叶片和细根清除活性氧自由基的方式存在一定差异,这可能是由于叶片和细根功能和代谢不同所致㊂另外,本研究中,无论增温还是对照处理,杉木细根过氧化氢酶㊁抗坏血酸过氧化物酶活性和抗坏血酸含量均高于叶片,其中过氧化氢酶达显著水平(图4),仅过氧化物酶显著低于叶片(图4)㊂由此可推断,杉木细根活性氧和丙二醛含量显著低于叶片的原因可能在于较叶片相比细根超氧化物歧化酶和过氧化氢酶显著高于叶片,亦说明了细根在抗氧化能力可能更优于叶片㊂温度几乎影响植物所有的代谢过程,植物对增温的响应是植物各器官之间通过代谢和信号事件进行复杂7867㊀21期㊀㊀㊀叶旺敏㊀等:大气增温对杉木幼树叶片及细根生理特征的影响㊀8867㊀生㊀态㊀学㊀报㊀㊀㊀40卷㊀的互动交流的结果[41]㊂若只考虑叶片在增温后的表现,显然无法深入解释杉木整体生理代谢过程对未来全球变暖的响应机制㊂对杉木地上(叶片)和地下部分(细根)同时进行研究,可以更好的揭示杉木适应增温的机制和策略㊂在增温条件下,杉木细根灵活高效地调节保护酶系统的能力,是杉木适应高温环境的重要的生理生态机制,这与Dichio等[42]研究结果类似㊂4㊀结论综上所述,研究发现增温后杉木叶片和细根丙二醛含量显著升高,但二者活性氧物质均无显著变化,这可能是由于夏季高温胁迫加剧杉木叶片和细根的氧化损伤,而随着高温胁迫程度降低,杉木叶片和细根可以通过诱导激活自身的抗氧化系统以及增强其渗透调节能力使其活性氧物质维持在稳定水平㊂另外,本研究还发现,无论增温还是对照处理下,杉木叶片活性氧类物质均显著高于细根,即叶片氧化损伤高于细根,可能归因于细根超氧化物歧化酶和过氧化氢酶活性显著高于叶片㊂虽然在本文中,增温后杉木可以通过自身物质合成和诱导较高的抗氧化酶活性来平衡体内活性氧含量,但夏季增温引起的极端高温环境可能对杉木产生氧化损伤㊂因此,今后的研究还应重点关注增温对不同季节杉木活性氧代谢及抗氧化系统的影响㊂参考文献(References):[1]㊀IPCC.ClimateChange2013:ThePhysicalScienceBasis,ContributionofWorkingGroupItoFifthAssessmentReportoftheIntergovernmentalPanelonClimateChange.Cambridge:UniversityPress,2013.[2]㊀刘国华,傅伯杰.全球气候变化对森林生态系统的影响.自然资源学报,2001,1(1):71⁃78.[3]㊀FuG,ShenZX,SunW,ZhongZM,ZhangXZ,ZhouYT.Ameta⁃analysisoftheeffectsofexperimentalwarmingonplantphysiologyandgrowthontheTibetanPlateau.JournalofPlantGrowthRegulation,2015,34(1):57⁃65.[4]㊀XuMH,XueX.Analysisontheeffectsofclimatewarmingongrowthandphenologyofalpineplants.JournalofAridLandResources&Environment,2013,27:137⁃141.[5]㊀DusengeME,WayDA.Warmingputsthesqueezeonphotosynthesis⁃lessonsfromtropicaltrees.JournalofExperimentalBotany,2017,68(9):2073⁃2077.[6]㊀CavaleriMA,ReedSC,SmithWK,WoodTE.Urgentneedforwarmingexperimentsintropicalforests.GlobalChangeBiology,2015,21(6):2111⁃2121.[7]㊀DandapatJ,ChainyGBN,RaoKJ.Lipidperoxidationandantioxidantdefencestatusduringlarvaldevelopmentandmetamorphosisofgiantprawn,Macrobrachiumrosenbergii.ComparativeBiochemistryandPhysiologyPartCToxicology&Pharmacology,2003,135(3):0⁃233.[8]㊀GossettDR,MillhollonEP,LucasMC,BanksSW,MarneyMM.TheeffectsofNaClonantioxidantenzymeactivitiesincallustissueofsalt⁃tolerantandsalt⁃sensitivecottoncultivars(Gossypiumhirsutum,L.).PlantCellReports,1994,13(9):498⁃503.[9]㊀ZhaoC,LiuQ.EffectsofsoilwarmingandnitrogenfertilizationonleafphysiologyofPinustabulaeformisseedlings.ActaPhysiologiaePlantarum,2012,34(5):1837⁃1846.[10]㊀RoessnerU,PattersonJH,ForbesMG,FincherGB,LangridgeP,BasicA.AnInvestigationofBoronToxicityinBarleyUsingMetabolomics.PlantPhysiology,2006,142(3):1087⁃1101.[11]㊀SklirosD,KalloniatiC,KaraliasG,SkaracisGN,RennenbergH,FlemetakisE.Globalmetabolomicsanalysisrevealsdistinctivetolerancemechanismsindifferentplantorgansoflentil(Lensculinaris)uponsalinitystress.Plant&Soil,2018,429(1⁃2):451⁃468.[12]㊀PinheiroC,PassarinhoJA,RicardoCP.EffectofdroughtandrewateringonthemetabolismofLupinusalbusorgans.JournalofPlantPhysiology,2004,161(11):1203⁃1210.[13]㊀安玉艳,郝文芳,龚春梅,韩蕊莲,梁宗锁.干旱⁃复水处理对杠柳幼苗光合作用及活性氧代谢的影响.应用生态学报,2010,21(12):3047⁃3055.[14]㊀ChenGS,YangZJ,GaoR,XieJS,GuoJF,HuangZQ,YangYS.CarbonstorageinachronosequenceofChinesefirplantationsinsouthernChina.ForestEcologyandManagement,2013,300(4):68⁃76.[15]㊀HuangW,ZhangSB,HuH.Sunleavesup⁃regulatethephotorespiratorypathwaytomaintainahighrateofCO2assimilationintobacco.FrontiersinPlantScience,2014,5(688):688.[16]㊀柯德森,王爱国,孙谷畴,董良峰.活性氧对外源IAA诱导的ACC合酶活性的影响.植物学报,2002,44(5):551⁃556[17]㊀ProchazkovaD,SairamRK,SrivastavaGC,SinghDV.Oxidativestressandantioxidantactivityasthebasisofsenescenceinmaizeleaves.PlantScience,2001,161(4):765⁃771.[18]㊀GiannopolitisCN,RiesSK.SuperoxideDismutases:I.OccurrenceinHigherPlants.PlantPhysiology,1977,59(2):309⁃14.[19]㊀KochharS,KochharVK,KhandujaSD.ChangesinthepatternofisoperoxidasesinthedormantcanesofThompsonSeedlessgrapes.AmericanJournalofEnology&Viticulture,1979,30(4):275⁃277.[20]㊀TrevorE,KrausR,AustinF.Paclobutrazolprotectswheatseedlingsfromheatandparaquatinjury.isdetoxificationofactiveoxygeninvolved.Plant&CellPhysiology,1994,35(1):45⁃52.[21]㊀NakanoY,AsadaK.Hydrogenperoxideisscavengedbyascorbate⁃specificperoxidaseinspinachchloroplasts.Plant&CellPhysiology,1981,22(5):867⁃880.[22]㊀DhindsaRS,Plumb⁃DhindsaP,ThorpeTA.LeafSenescence:Correlatedwithincreasedlevelsofmembranepermeabilityandlipidperoxidation,anddecreasedlevelsofsuperoxidedismutaseandcatalase.JournalofExperimentalBotany,1981,32(126):93⁃101.[23]㊀BradfordMM.Arapidandsensitivemethodforthequantitationofmicrogramquantitiesofproteinutilizingtheprincipleofprotein⁃dyebinding.AnalyticalBiochemistry,1976,72(S1⁃2):248⁃254.[24]㊀TrollW,LindsleyJ.Aphotometricmethodforthedeterminationofproline.JournalofBiologicalChemistry,1955,215(2):655.[25]㊀ApelK,HirtH.Reactiveoxygenspecies:metabolism,oxidativestress,andsignaltransduction.AnnualReviewofPlantBiology,2004,55(1):373⁃399.[26]㊀MøllerI,JensenPE,HanssonA.Oxidativemodificationstocellularcomponentsinplants.AnnualReviewofPlantBiology,2007,58(1):459⁃481.[27]㊀ZhouXB,ZhangYM,YinBF.Divergenceinphysiologicalresponsesbetweencyanobacterialandlichencruststoagradientofsimulatednitrogendeposition.Plant&Soil,2016,399(1⁃2):121⁃134.[28]㊀肖群英,尹春英,濮晓珍,乔敏峰,刘庆.川西亚高山季节性冻土期针叶林主要树种叶片和细根的生态生理特征.植物生态学报,2014,38(4):343⁃354.[29]㊀周瑞莲,王相文,左进城,杨润亚,黄清荣,刘怡.海岸不同生态断带植物根叶抗逆生理变化与其Na+含量的关系.生态学报,2015,35(13):4518⁃4526.[30]㊀YangY,YaoY,XuG,LiC.Growthandphysiologicalresponsestodroughtandelevatedultraviolet⁃BintwocontrastingpopulationsofHippophaerhamnoides.PhysiologiaPlantarum,2005,124(4):431⁃440.[31]㊀杨岚,师帅,王红娟,向增旭.水杨酸对高温胁迫下铁皮石斛幼苗耐热性的影响.西北植物学报,2013,33(3):534⁃540.[32]㊀宋海凤,李绍才,孙海龙,刘雅静,陈艳华.根施不同浓度多效唑对紫穗槐生长特性和相关生理指标的影响.植物生理学报,2015(9):1495⁃1501.[33]㊀崔豫川,张文辉,李志萍.干旱和复水对栓皮栎幼苗生长和生理特性的影响.林业科学,2014,50(7):66⁃73.[34]㊀曹仪植,吕忠恕.水分胁迫下植物体内游离脯氨酸的累积及ABA在其中的作用.植物生理学报,1985(1):11⁃18.[35]㊀AhmadP,SarwatM,SharmaS.Reactiveoxygenspecies,antioxidantsandsignalinginplants.JournalofPlantBiology,2008,51(3):167⁃173.[36]㊀WangK,ZhangX,ErvinE.Antioxidativeresponsesinrootsandshootsofcreepingbentgrassunderhightemperature:Effectsofnitrogenandcytokinin.JournalofPlantPhysiology,2012,169(5):0⁃500.[37]㊀吴永波,叶波.高温干旱复合胁迫对构树幼苗抗氧化酶活性和活性氧代谢的影响.生态学报,2016,36(2):403⁃410.[38]㊀郭盈添,范琨,白果,石杰霞,董开茂,关雪莲,郑健.金露梅幼苗对高温胁迫的生理生化响应.西北植物学报,2014,34(9):1815⁃1820.[39]㊀许辰森,熊德成,邓飞,史顺增,钟波元,冯建新,陈云玉,陈光水,杨玉盛.杉木幼苗和伴生植物细根对土壤增温的生理生态响应.生态学报,2017,37(4):1232⁃1243.[40]㊀李伟成,盛海燕,高贵宾,温星,田新立.高温干旱对髯毛箬竹的叶和细根的生理生态影响.环境科学研究,2017,30(12):1908⁃1918.[41]㊀BaghourM,ChekrounKB,SáezJMR,RomeroL.Root⁃zonetemperatureaffectsthephytoextractionofironincontaminatedsoil.JournalofPlantNutrition,2016,39(1):51⁃58.[42]㊀DichioM,XiloyannisC,SofoA,MotanaroG.Osmoticregulationinleavesandrootsofolivetreesduringawaterdeficitandrewatering.TreePhysiology,2006,26(2):179⁃185.9867㊀21期㊀㊀㊀叶旺敏㊀等:大气增温对杉木幼树叶片及细根生理特征的影响㊀。
- 1、下载文档前请自行甄别文档内容的完整性,平台不提供额外的编辑、内容补充、找答案等附加服务。
- 2、"仅部分预览"的文档,不可在线预览部分如存在完整性等问题,可反馈申请退款(可完整预览的文档不适用该条件!)。
- 3、如文档侵犯您的权益,请联系客服反馈,我们会尽快为您处理(人工客服工作时间:9:00-18:30)。
Ecology,89(5),2008,pp.1232–1245Ó2008by the Ecological Society of AmericaABOVEGROUND AND BELOWGROUND EFFECTS OF SINGLE-TREE REMOVALS IN NEW ZEALAND RAIN FORESTD AVID A.W ARDLE,1,2,5S USAN K.W ISER,2R OBERT B.A LLEN,2J AMES E.D OHERTY,3K AREN I.B ONNER,2AND W ENDY M.W ILLIAMSON41Department of Forest Ecology and Management,Swedish University of Agricultural Sciences,SE90183,Umea˚,Sweden2Landcare Research,P.O.Box40,Lincoln7640,New Zealand3T¯u hoe Tuawhenua Trust,P.O.Box4,Murupara,Bay of Plenty,New Zealand4ESR,Christchurch Science Centre,P.O.Box29181,Christchurch8030,New ZealandAbstract.There has been considerable recent interest in how human-induced species loss affects community and ecosystem properties.These effects are particularly apparent when acommercially valuable species is harvested from an ecosystem,such as occurs through single-tree harvesting or selective logging of desired timber species in natural forests.In New Zealandmixed-species rain forests,single-tree harvesting of the emergent gymnosperm Dacrydiumcupressinum,or rimu,has been widespread.This harvesting has been contentious in partbecause of possible ecological impacts of Dacrydium removal on the remainder of the forest,but many of these effects remain unexplored.We identified an area where an unintended40-year‘‘removal experiment’’had been set up that involved selective extraction of individualDacrydium trees.We measured aboveground and belowground variables at set distances fromboth individual live trees and stumps of trees harvested40years ago.Live trees had effectsboth above and below ground by affecting diversity and cover of several components of thevegetation(usually negatively),promoting soil C sequestration,enhancing ratios of soil C:Pand N:P,and affecting community structure of soil microflora.These effects extended to8mfrom the tree base and were likely caused by poor-quality litter and humus produced by thetrees.Measurements for the stumps revealed strong legacy effects of prior presence of trees onsome properties(e.g.,cover by understory herbs and ferns,soil C sequestration,soil C:P andN:P ratios),but not others(e.g.,soil fungal biomass,soil N concentration).These resultssuggest that the legacy of prior presence of Dacrydium may remain for several decades orcenturies,and certainly well over40years.They also demonstrate that,while large Dacrydiumindividuals(and their removal)may have important effects in their immediate proximity,within a forest,these effects should only be important in localized patches containing highdensities of large trees.Finally,this study emphasizes that deliberate extraction of a particulartree species from a forest can exert influences both above and below ground if the removedspecies has a different functional role than that of the other plant species present.Key words:Dacrydium cupressinum;microbial community;New Zealand;removal experiment;rimu;selective logging;single-tree harvesting;species effects.I NTRODUCTIONThere has been considerable recent interest in predicting how human-induced losses of species and functional groups influence community and ecosystem properties(Hooper et al.2005).The ecological impacts of species loss can be especially apparent when particular plant or animal species are deliberately harvested from the ecosystem(e.g.,Bodmer et al. 1997,Cardillo et al.2005).Single-tree(or selective) harvesting in natural forests that contain several tree species represents such a situation;here,individuals of one or more tree species with desired timber properties are selectively removed from the forest with the remainder of theflora being left.In a mixed-species forest,different tree species may have vastly different effects on both aboveground and belowground proper-ties(Binkley and Giardina1998,Finzi et al.1998, Binkley and Menyialo2005),and in such forests, individual tree effects may be important drivers of spatial heterogeneity of both soil properties and understory vegetation(Boettcher and Kalisz1990, Saetre and Ba a th2000).It follows that selective removal of some tree species using logging,while leaving other tree species,can have important effects on the remainder of the ecosystem(Pollmann2002,Verburg and van Eijk-Bos2003,Villela et al.2006).What is less clear is how long these effects persist following harvesting.The vast majority of experiments investigating eco-logical effects of human-induced loss of biodiversity have involved randomly assembled communities(Bal-vanera et al.2006,Cardinale et al.2006).However,in reality,human activity does not result in species beingManuscript received20September2007;accepted25 September2007.Corresponding Editor:J.Belnap.5E-mail:david.wardle@svek.slu.se1232lost at random from the community(Wardle1999, Solan et al.2004,Zavaleta and Hulvey2004),and some taxa with specific attributes or traits may be lost from a community more rapidly than others(Duncan and Young2000,Petchey and Gaston2002).The ecological impacts of nonrandom species losses in real communi-ties are therefore arguably often better investigated by ‘‘removal experiments’’in which the effects of biodiver-sity loss are studied following the experimental removal of various components of those communities(e.g., Wardle et al.1999,Symstad and Tilman2001,Wardle and Zackrisson2005,Polley et al.2006;for a review see Dıaz et al.2003).It has been recognized that forests from which particular tree species have been removed for timber(e.g.,through selective logging)may serve as long-term replicated‘‘removal experiments’’for investi-gating the ecological impacts of nonrandom loss of species through human activity(Dıaz et al.2003), although few studies have specifically utilized such experiments in this way.In New Zealand,about25%of the land area is covered by indigenous forest,and this includes signifi-cant areas of mixed podocarp(gymnosperms in the Podocarpaceae)–hardwood forest.One of the most widespread and conspicuous tree species in the lowland or lower montane mixed forests of New Zealand is Dacrydium cupressinum(Podocarpaceae),or rimu, which commonly reaches.35m(and up to60m)in height,and often grows as a long-lived emergent tree above the forest canopy(Franklin1968,Six Dijkstra et al.1985,Norton et al.1988;see Plate1).This species hashighly desirable timber properties and,as such,was widely logged throughout New Zealand until the1980s. There are some areas where single-tree harvesting of Dacrydium has been performed from the1950s to the present day,with coexisting tree species(notably hardwood)being left relatively intact.This selective harvesting has been a contentious political issue in New Zealand for over three decades(James and Norton 2002),in part because of possible effects of Dacrydium removal on the composition and functioning of the remainder of the forest.These effects remain unex-plored.In the present study,we identified an area in which an unintended‘‘removal experiment’’had been performed;40years ago in a mixed podocarp–hardwood forest from which Dacrydium had been selectively logged.Specifically,we sought to answer this question:do long-lived large trees of Dacrydium(a gymnosperm)have a legacy(on the order of decades) above and below ground when the residual canopy is dominated by hardwood(angiosperm)species?To do this,we assessed aboveground and belowground prop-erties and aboveground–belowground relationships at set distances from both live trees and from stumps of Dacrydium trees that had been removed40years ago. Further,we sought to better understand the long-term influence of selective logging on individual tree effects, and in a more general sense to generate insights about the ecological impacts of human-induced losses of key biotic components from natural forest ecosystems.M ETHODSStudy system and tree selectionThe study area is in the central North Island of New Zealand;part of the area is in the land administered by the T¯u hoe Tuawhenua Trust,and the remainder is in Te Urewera National Park.It is located at388370S,1768520 E,the mean annual rainfall is1600–2400mm,equally distributed throughout the year,and the mean January and July air temperatures are148C and48C,respectively. The soil present is Urewera steepland soil,which is a type of podzolic orthic pumice soil known in the USDA soil taxonomy as a Vitrand or Vitricryands(Hewitt 1998).The underlying parent material is greywacke(a fine-grained sandstone)overlain by rhyolitic ash.The dominant(canopy-forming)hardwood tree species is Beilschmiedia tawa,with Dacrydium,Prumnopitys ferru-ginea,and Metrosideros robusta as emergents.Many of the Dacrydium trees that occur are.500years old and have a diameter at breast height(dbh,about1.4m high) of.1m.This species produces very poor litter that decomposes slowly,especially in comparison with Beilschmiedia(data in Wardle et al.2002).The understory species consist of smaller hardwoods(Meli-P LATE 1.An emergent Dacrydium cupressinum tree and associated forest in Te Urewera National Park,North Island, New Zealand.Photo credit:S.K.Wiser.May20081233RAIN FOREST SINGLE-TREE REMOVAL EFFECTScytus ramiflorus,Pseudowintera spp.),tree ferns(Dick-sonia squarrosa and Cyathea smithii),ground ferns (Blechnum discolor,Asplenium bulbiferum,Leptopteris hymenophylloides),and other herbaceous plants(Micro-laena avenacea,Uncinia uncinata).The area specifically considered in our study is a strip of land;1km long3300m wide.On one side of this strip(1km3150m)all Dacrydium trees of millable size (dbh.30cm)were logged between1956and1970 (mean time since harvest¼40years).On the other side of the strip(1km3150m),no logging was ever performed and all Dacrydium trees remain intact.In the absence of logging,the density of Dacrydium stems with a dbh.30cm is14.4stems/ha.At these densities, Dacrydium trees(or stumps)were usually far enough apart to be considered independent.Total tree basal area(dbh.30cm)is43.2m2/ha,and Dacrydium stems comprise35%of this basal area(R.Scott,unpublished data).Of the total basal area,3%consists of stems,10 cm dbh,42%of stems10–30cm dbh,28%of stems30–50dbh,13%of stems50–80cm dbh,and14%of stems .80cm dbh.During19–27November2004,we randomly selected 20live Dacrydium trees and20stumps of Dacrydium individuals that had been logged40years earlier.We selected only live trees with dbh.80cm and only stumps that had comparable diameters at ground level to the live trees we used.We treated each live tree or tree stump as a discrete replicate,which we maintain is defensible,given the narrow dimensions of the land used relative to its length.Plot demarcation and vegetation measurementsAll vegetation measurements were performed over19–27November2004.For each live tree or stump,the diameter at ground level was recorded,and for each live tree,the dbh was also noted.Three plots were then set up at increasing distances from the base of each tree or stump,so that each plot was24m2.Thefirst plot was arc shaped and extended from0to4m from the base of the tree or tree stump.The left-and right-hand boundaries of the plot were both segments of radial lines extending from the center of the stump or tree,with the angle between the two lines being calculated so that the plot area was24m2.This angle varied slightly according to the diameter of tree or stump base,but was on average818.The second plot was also arc shaped and extended from4to8m from the base of the tree or stump,and with its left-and right-hand boundaries being determined in the same way as for thefirst plot,so as to yield a total plot area of24m2.Here,the angle between left and right boundaries was,on average,408. The third plot extended from12to16m from the base of the tree or stump,and had dimensions of436m;it was rectangular rather than arc shaped because the degree of curvature at that distance from the tree was minor relative to the dimensions of the plot.The direction of plots from the tree or stump base was chosen at random,except that bulldozer tracks made during tree harvesting were avoided.On each plot,vascular species present were recorded in six height tiers(.25m,12–25m,5–12m,2–5m,0.3–2m,and,0.3m).Foliar cover over the plot area for each species in each tier was estimated using a modified Braun-Blanquet cover scale(1¼few plants,cover,1%; 2¼1–5%cover;3¼6–25%;4¼26–50%;5¼51–75%;6¼.75%;Allen,1992).Species were classified intofive groups,namely woody canopy species(primarily Beilschmiedia),tree ferns,understory woody plants, understory ferns,and understory herbs.Total cover for each group was calculated by summing the midpoints of the cover class recorded for each species in that group across all the tiers in which it occurred,as described by Wiser et al.(2002).As a consequence,the total cover of any given group can exceed100%.Belowground measurementsWhen the vegetation was measured,soil samples were collected midway between the innermost and outermost edges of each of the vegetation plots,at2,6,and14m from the base of the tree or tree stump.For each plot, three intact cores of soil with surface litter removed (each5cm diameter35cm depth)were taken,1m apart from each other.At this depth the soil is primarily humus with some incorporation of mineral soil,with an organic matter content of67%on average.The three samples were bulked for each plot and the wet mass determined;the dry mass(dried at808C for24h)was then determined for a20-g subsample for determination of soil bulk density(dry mass per unit volume).The soil sample from each plot was sievedfield-moist to pass through a4-mm mesh and a subsample was used for determination of the following soil chemical proper-ties:soil pH,total soil C using a Leco FP-2000Analyzer (Laboratory Equipment Corporation,St.Joseph,Mich-igan,USA,),total N(Kjeldahl method),P(as described by Jackson1958),mineral N(ammonium and nitrate) using automated colorimetric procedures(Technicon Instruments1977)following extraction with2mol/L KCl,and bicarbonate extractable C using the Olsen method(Jackson1958).Because the bulk density of each soil sample was known,total C,N,and P per unit area stored in the soil to5cm depth were also calculated. Microbial basal respiration(BR)and substrate-induced respiration(SIR),a relative measure of active microbial biomass,were determined for each sample as described by Wardle(1993).Briefly,a15-g(dry mass equivalent)subsample of soil was amended to150% gravimetric moisture content(dry mass basis),either by gradual drying or rewetting with afine mist,placing it in a130-mL airtight vessel,and incubating at228C. Evolution of CO2between1and4h was then determined by injecting1-mL subsamples of headspace gas into an infrared gas analyzer(ADC2250Gas Analyzer,ADC BioScientific,Hoddesdon,UK)used as a measure of BR.Measurement of SIR(Anderson andDAVID A.WARDLE ET AL.1234Ecology,Vol.89,No.5Domsch1978)was performed in the same way,but with amendment of the sample with150-mg anhydrous D(þ)-Glucose at the start of the incubation(Wardle1993). The microbial metabolic quotient(q CO2),the ratio of BR to SIR,was used as a measure of relative microbial-C-use efficiency;this ratio declines as efficiency increases (Anderson and Domsch1985).The ratio of SIR to organic C was used as an indicator of soil C quality because when soil C is more favorable for microbes,a higher proportion of it is assimilated in microbial tissue (Insam and Domsch1988).Microbial community composition for each sample was assessed by measuring the composition of microbial phospholipids fatty acids(PLFAs)using the method of Bligh and Dyer(1959),as modified by White et al. (1979)and Bardgett et al.(1996);different PLFAs are derived from different subsets of the soil microflora.For each soil,the abundance of each of the individual fatty acids extracted was expressed as relative nmoles per gram of dry soil using standard nomenclature(Tunlid et al.1989).The PLFAs that were used to represent bacteria were i-15:0,a-15:0,15:0,16.0,i-16.0,16:1x9,i-17:0,cyc-17:0,17:0,18:1x9c,18:1x9t,and cyc-19:0,and the PLFA used to represent fungi was18:2x6(Parekh and Bardgett2002).The PLFAs used for calculation of total branched PLFAs(indicative of gram-positive bacteria)were i-15:0,a-15:0,i-16:0,and i-17:0,while those used for determining total cyclic PLFAs were cyc-17:0and cyc-19:0.The ratio of total bacterial PLFAs to fungal plus bacterial PLFAs was used as an estimate of the relative importance of the bacterial and fungal energy channels(Bardgett et al.1996,Parekh and Bardgett2002).To assess plant litter decomposition,two nylon-mesh litter bags were positioned at each of three distances(2, 6,and14m)from the edge of each tree or stump,which was in the center of the plots used for vegetation measurement.Each litter bag measured10310cm with mesh size of1mm and contained3g(dry-mass basis)of air-dried fresh litter of Beilschmiedia tawa(N and P concentrations¼1.34%and0.067%,respectively).Litter bags were positioned at the interface of the humus and litter layers during19–27November2004,and all bags were harvested approximately one year later during7–8 December2005.Following harvest,the litter in each bag was cleaned and the remaining dry mass(dried at808C for24h)was determined.Data analysisAll response variables,except for those summarizing community structure,were analyzed using split-plot analysis of variance(ANOVA)with each tree or stump serving as the main plot and with the three distances from each tree or stump serving as the subplots.For the soil abiotic variables,split-plot multivariate ANOVA (MANOVA)was also used for the entire data set because of the large number of variables measured (other multivariate methods were used for the plant and microbial community data).In all cases,the effects tested for were tree vs.stump(S),distance from tree or stump(D),and the S3D interaction.When any of these effects was significant at P,0.05,differences among means were compared using the least significant difference test at P,0.05.Variables were transformed as necessary to satisfy assumptions of all parametric data analyses performed in this study.For analysis of both the vegetation and PLFA community structure data we used the constrained-ordination split-plot analogue as implemented in the software package CANOCO(Microcomputer Power, Ithaca,New York,USA;Baar and ter Braak1996,ter Braak and S milauer2002).This was chosen because it is the only ordination approach available for performing the analogue of a split-plot ANOVA.To do this,we used redundancy analysis,a linear ordination method, because a preliminary analysis by the nonlinear de-trended correspondence analysis method resulted in short gradient lengths(i.e.,,3.0standard deviations;ter Braak and S milauer2002).We then used Monte Carlo permutation tests with plots permuted as appropriate to test for the statistical significance of S,D,and S3D effects on vegetation and PLFA community structure,as described by ter Braak and S milauer(2002).To examine the relationship between vegetation composition and PLFA composition across plots,we analyzed both data sets using the unconstrained linear ordination technique,principal components analysis (PCA),and determined Spearman rank correlation coefficients between vegetation,and PLFA ordination plot scores for the two main axes.This was done using only the stump data,only the live tree data,and all stump and live tree data combined.R ESULTSAboveground responsesFor the20live Dacrydium trees,dbh was136.6632.3 cm(mean6SD).The mean diameter at ground level of these trees was208.2650.1cm,while that of the20 stumps was240.3652.2cm.The live trees and tree stumps were therefore of comparable dimensions,as the cut stumps remain largely intact and have diminished little since being harvested.The cover of understory herbs,understory ferns,and tree ferns significantly increased with increasing distance from trees and stumps,according to ANOVA(Table1,Fig.1);the same pattern occurred for understory woody plants in relation to live trees,but this effect was not statistically significant,according to ANOVA.Meanwhile Beilsch-miedia cover was least at the greatest distance from trees and stumps.Further,the cover of both tree ferns and understory woody plants was greater for stumps than for live trees(Fig.1).There was no interactive effect between distance from trees or stumps,and trees vs. stumps,for the cover of any plant group(Table1). When the overall species composition of the vegeta-tion was analyzed,both distance from tree or stump andMay20081235RAIN FOREST SINGLE-TREE REMOVAL EFFECTStree vs.stump had significant effects (Table 1,Fig.2).There was no interactive effect,however,between these two factors.The ordination revealed that some plant species were particularly important in separating the plots from each other (Fig.2).Thus,the tree fern Cyathea smithii had increased cover in plots that were farthest from the live trees and stumps.The understory woody plant Pseudowintera colorata and tree fern Dicksonia squarrosa were most closely associated with stumps,while the understory fern Polystichum richardii was associated most closely with live trees.The woody understory plant Melicytus ramiflorus and the understo-ry ferns Leptopteris hymenophylloides and Asplenium bulbiferum tended to be associated with live trees and with plots at greater distances from trees or stumps (12–16m vs.0–4m or 4–8m).Vegetation community composition in the ordination analysis was related to spatial variability in several soil properties,notably soil C concentration and ratios of C:N and N:P,which increased toward the stumps,and soil pH and bulk density,which increased away from them (Fig.2).Species richness of all vascular plants,and of understory herbs and understory ferns,increased significantly with increasing distance from trees or stumps (Table 1,Fig.1).Further,in the case of understory ferns,species richness was greater for live trees than for stumps (Table 1,Fig.1).Species richness of understory woody plants showed no significant relationship with stumps or with live trees.There was no interactive effect between distance from trees or stumps or trees vs.stumps for the species richness of any plant group (Table 1).Belowground responsesThe MANOVA performed over the full data set of abiotic soil properties revealed a highly significant overall interaction between trees vs.stumps and distance (Table 2).Univariate ANOVAs on these variables showed that distance and trees vs.stumps both significantly influenced soil variables,contributing to this overall interactive effect (Table 2).Concentrations of total soil C and N both declined at increasing distances from trees and stumps,and soil N concentra-tions were also greater for stumps than for trees (Table 2,Fig.3).In contrast,concentrations of total soil P and labile forms of N (i.e.,mineral N)and P (i.e.,Olsen P)were unaffected by trees or stumps.Mass ratios of C:N,C:P,and N:P were all significantly greater close to the stump or tree than farther away from it (Table 2,Fig.3).Bulk density of soil increased with increasing distance from the tree or stump,and the amounts of C and N sequestered in the soil per unit area (i.e.,soil content)declined.Soil pH increased at increasing distances from trees or stumps.The ratio of labile mineral N to total N and labile Olsen P to total P were unaffected by distance from tree or stump,although the ratio of mineral N to total N was marginally significantly greater (P ¼0.038)for live trees than for stumps.For the univariate ANOVAs,interactive effects between distance and stump vs.tree were significant only for mineral N and the soil C:N ratio,despite being highly significant for the MANOVA (Table 2).Two measures of soil biological activity,the soil BR and litter decomposition rate,declined significantly (though not strongly)with increasing distance from trees and stumps (Table 3,Fig.4).For BR this effect was greater for stumps than for live trees,though this effect was not statistically significant.Measures of the total microbial biomass (SIR and total PLFAs)were unre-sponsive to trees or stumps,but the ratio of SIR to organic C was significantly greater for stumps than for trees (Table 3,Fig.4).Further,there was evidence for effects of trees and stumps on microbial community composition as revealed using PLFA analysis.Fungal PLFA quantities were greater for live trees than for stumps (Fig.4)and declined with increasing distances from the tree (Table 3).T ABLE 1.Effect of stump vs.live tree,distance from stump or tree,and their interaction on vegetation biological properties as revealed by split-plot two-way analysis of variance (or redundancy analysis for the plant community compositional data).Response variableStump vs.tree (S)Distance from stump or tree (D)S 3D interaction F (df ¼1,38)P F (df ¼2,76)P F (df ¼2,76)P Multivariate analysesPlant community composition5.620.002 3.500.0020.830.824Univariate analysesBeilschmiedia tawa cover à0.210.652 5.320.0070.110.897Cover of tree ferns à11.510.00214.60,0.001 1.030.363Cover of understory herbs (excluding ferns)à0.070.788 3.840.0260.440.646Cover of understory ferns à0.730.3989.10,0.001 1.570.214Cover of understory woody plants à8.620.006 2.560.084 1.370.261Vascular plant species richness2.150.15112.29,0.0010.320.724Understory herb species richness (excluding ferns)0.210.6503.550.0340.580.564Understory fern species richness6.540.01512.71,0.001 1.630.202Understory woody plant species richness0.400.5282.530.0870.230.793Derived from the redundancy analysis presented in Fig.2.àData were log-transformed before analysis.DAVID A.WARDLE ET AL.1236Ecology,Vol.89,No.5The ratio of fungal to bacterial PLFAs showed a broadly similar response,though not as strongly.Total branched PLFAs also declined with increasing distance from trees or stumps,while cyclic PLFAs were unresponsive.Trees and stumps,however,had no detectable effect on the diversity of microbial PLFAs.Interactive effects between stump vs.tree and distance were significant only for the BR:SIR ratio (Table 3).The structure of the soil microbial community,determined through redundancy analysis of the PLFA data,showed significant overall responses to the distance from live trees or stumps,but not to trees vs.stumpsIG .1.Measures of plant cover and species richness at three distances from the center of live Dacrydium cupressinum trees and tree stumps.Within each panel,bars topped by the same lowercase letter do not differ significantly at P ¼0.05(least significant difference test)derived from split-plot ANOVA (Table 1).SEM is standard error of the mean pooled across distance and tree vs.stump.May 20081237RAIN FOREST SINGLE-TREE REMOVAL EFFECTS(Table 3,Fig.5).There was also a marginally nonsignif-icant interactive effect between these two factors (Table 3).Both bacterial PLFAs (e.g.,15:0,i16:0,16:0,cyc17:0)and the dominant fungal PLFA (18:2x 6)served as important discriminators among plots in the analysis (Fig.5).Microbial community composition in the ordination analysis was related to spatial variability in several soil properties,including C concentration,ratios of C:N and N:P,pH,and bulk density (Fig.5).Correlation analyses between PCA scores for plant community structure and PCA scores for microbial community structure (PLFA data)provided little evidence of relationships between the plant and micro-bial data across plots.Correlation coefficients amongpairs of PCA axes were never statistically significant when data for all plots or data for live-tree plots only were used (data not shown),and for stump plots the only significant relationship was between the second PCA axis for plants and the second PCA axis for microbes (r ¼0.293,P ¼0.023).D ISCUSSIONAboveground responsesOur study provided evidence that live Dacrydium trees have important effects on the rest of the vegetation;cover,and species richness of understory herbs and ferns,and cover of tree ferns were all lower closer totheF IG .2.Regression biplot derived from a redundancy analysis of plant species with respect to the model:stumps vs.trees;distance (0–4,4–8,12–16m).The biplot displays 8%of the variance in abundances (cover scores)and 43%of the variance in the fitted abundances.Eigenvalues of the first two axes are 0.051and 0.031,respectively;the sum of all canonical eigenvalues is 0.087.Only species with .20%frequency across all vegetation subplots are shown.C,N,and P are carbon,nitrogen,and phosphorus.Species codes are as follows:woody vascular plants,ARISER ¼Aristotelia serrata ,BEITAW ¼Beilschmiedia tawa ,BRAREP ¼Brachyglottis repanda ,CARSER ¼Carpodetus serratus ,COPTEF ¼Coprosma tenuifolia ,ELADEN ¼Elaeocarpus dentatus ,METPER ¼Metrosideros perforata ,MELRAM ¼Melicytus ramiflorus ,MYRAUS ¼Myrsine australis ,PARSON ¼Parsonsia spp .,PSEAXI ¼Pseudopanax axillaris ,PSECOL ¼Pseudowintera colorata ,PRUFER ¼Prumnopitys ferruginea ,and SCHDIG ¼Schefflera digitata ;understory herbs,COLHAS ¼Collospermum hastatum ,MICAVE ¼Microlaena avinacea ,and UNCUNC ¼Uncinia uncinata ;understory ferns,ASPBUL ¼Asplenium bulbiferum ,ASPFLA ¼Asplenium flaccidum ,ASPPOL ¼Asplenium polyodon ,BLEDIS ¼Blechnum discolor ;LEPHYM ¼Leptopteris hymenophylloides,MICPUS ¼Microsorum pustulatum ,and POLRIC ¼Polystichum richardii ;tree ferns,CYASMI ¼Cyathea smithii and DICSQU ¼Dicksonia squarrosa .DAVID A.WARDLE ET AL.1238Ecology,Vol.89,No.5。