济南大学毕业设计外文资料翻译参考格式(理工科类)2014
毕业论文的参考文献的中文格式与翻译
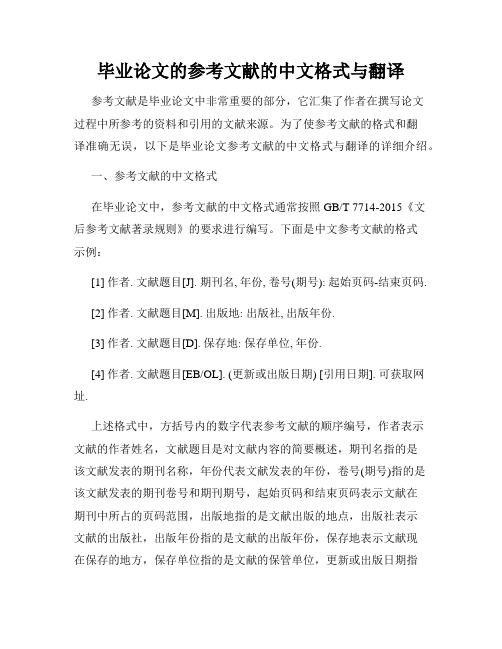
毕业论文的参考文献的中文格式与翻译参考文献是毕业论文中非常重要的部分,它汇集了作者在撰写论文过程中所参考的资料和引用的文献来源。
为了使参考文献的格式和翻译准确无误,以下是毕业论文参考文献的中文格式与翻译的详细介绍。
一、参考文献的中文格式在毕业论文中,参考文献的中文格式通常按照GB/T 7714-2015《文后参考文献著录规则》的要求进行编写。
下面是中文参考文献的格式示例:[1] 作者. 文献题目[J]. 期刊名, 年份, 卷号(期号): 起始页码-结束页码.[2] 作者. 文献题目[M]. 出版地: 出版社, 出版年份.[3] 作者. 文献题目[D]. 保存地: 保存单位, 年份.[4] 作者. 文献题目[EB/OL]. (更新或出版日期) [引用日期]. 可获取网址.上述格式中,方括号内的数字代表参考文献的顺序编号,作者表示文献的作者姓名,文献题目是对文献内容的简要概述,期刊名指的是该文献发表的期刊名称,年份代表文献发表的年份,卷号(期号)指的是该文献发表的期刊卷号和期刊期号,起始页码和结束页码表示文献在期刊中所占的页码范围,出版地指的是文献出版的地点,出版社表示文献的出版社,出版年份指的是文献的出版年份,保存地表示文献现在保存的地方,保存单位指的是文献的保管单位,更新或出版日期指的是网上资源的更新或出版日期,引用日期是获取网上资源时的日期,网址指的是网上资源的网址。
二、参考文献的翻译当我们在毕业论文中引用外文文献时,需要对文献题目进行翻译以便读者能够理解。
下面是一些常见的翻译方法和示例:1. 直译法:将外文文献的标题逐字逐句地直接翻译成中文。
示例:[1] Smith J. How to Write a Research Paper[J]. Journal of Academic Writing, 2018, 5(2): 123-135.翻译为:[1] 史密斯 J. 如何写一篇研究论文[J]. 学术写作杂志, 2018,5(2): 123-135.2. 意译法:根据文献的内容和语境将外文文献的标题进行意义上的翻译。
毕业论文 外文翻译格式
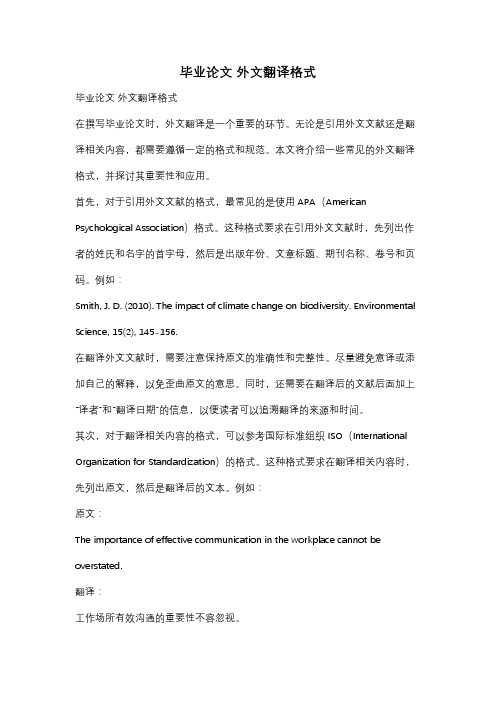
毕业论文外文翻译格式毕业论文外文翻译格式在撰写毕业论文时,外文翻译是一个重要的环节。
无论是引用外文文献还是翻译相关内容,都需要遵循一定的格式和规范。
本文将介绍一些常见的外文翻译格式,并探讨其重要性和应用。
首先,对于引用外文文献的格式,最常见的是使用APA(American Psychological Association)格式。
这种格式要求在引用外文文献时,先列出作者的姓氏和名字的首字母,然后是出版年份、文章标题、期刊名称、卷号和页码。
例如:Smith, J. D. (2010). The impact of climate change on biodiversity. Environmental Science, 15(2), 145-156.在翻译外文文献时,需要注意保持原文的准确性和完整性。
尽量避免意译或添加自己的解释,以免歪曲原文的意思。
同时,还需要在翻译后的文献后面加上“译者”和“翻译日期”的信息,以便读者可以追溯翻译的来源和时间。
其次,对于翻译相关内容的格式,可以参考国际标准组织ISO(International Organization for Standardization)的格式。
这种格式要求在翻译相关内容时,先列出原文,然后是翻译后的文本。
例如:原文:The importance of effective communication in the workplace cannot be overstated.翻译:工作场所有效沟通的重要性不容忽视。
在翻译相关内容时,需要注意保持原文的意思和语气。
尽量使用准确的词汇和语法结构,以便读者能够理解和接受翻译后的内容。
同时,还需要在翻译后的文本后面加上“翻译者”和“翻译日期”的信息,以便读者可以追溯翻译的来源和时间。
此外,对于长篇外文文献的翻译,可以考虑将其分成若干章节,并在每个章节前面加上章节标题。
这样可以使读者更容易理解和阅读翻译后的内容。
英文文献及中文翻译撰写格式

关于毕业设计说明书(论文)英文文献及中文翻译撰写格式为提高我校毕业生毕业设计说明书(毕业论文)的撰写质量,做到毕业设计说明书(毕业论文)在内容和格式上的统一和规范,特规定如下:一、装订顺序论文(设计说明书)英文文献及中文翻译内容一般应由3个部分组成,严格按以下顺序装订。
1、封面2、中文翻译3、英文文献(原文)二、书写格式要求1、毕业设计(论文)英文文献及中文翻译分毕业设计说明书英文文献及中文翻译和毕业论文英文文献及中文翻译两种,所有出现相关字样之处请根据具体情况选择“毕业设计说明书” 或“毕业论文”字样。
2、毕业设计说明书(毕业论文)英文文献及中文翻译中的中文翻译用Word 软件编辑,英文文献用原文,一律打印在A4幅面白纸上,单面打印。
3、毕业设计说明书(毕业论文)英文文献及中文翻译的上边距:30mm;下边距:25mm;左边距:3Omm;右边距:2Omm;行间距1.5倍行距。
4、中文翻译页眉的文字为“中北大学2019届毕业设计说明书” 或“中北大学××××届毕业论文”,用小四号黑体字,页眉线的上边距为25mm;页脚的下边距为18mm。
5、中文翻译正文用小四号宋体,每章的大标题用小三号黑体,加粗,留出上下间距为:段前0.5行,段后0.5行;二级标题用小四号黑体,加粗;其余小标题用小四号黑体,不加粗。
6、文中的图、表、附注、公式一律采用阿拉伯数字分章编号。
如图1.2,表2.3,附注3.2或式4.3。
7、图表应认真设计和绘制,不得徒手勾画。
表格与插图中的文字一律用5号宋体。
每一插图和表格应有明确简短的图表名,图名置于图之下,表名置于表之上,图表号与图表名之间空一格。
插图和表格应安排在正文中第一次提及该图表的文字的下方。
当插图或表格不能安排在该页时,应安排在该页的下一页。
图表居中放置,表尽量采用三线表。
每个表应尽量放在一页内,如有困难,要加“续表X.X”字样,并有标题栏。
外文翻译格式以及封面
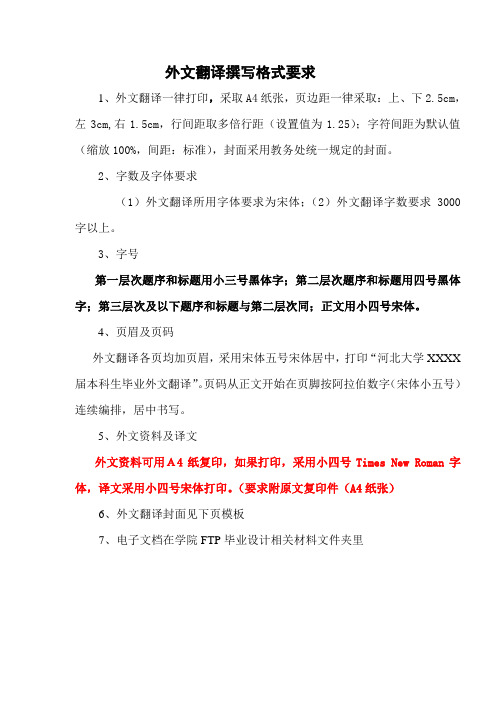
外文翻译撰写格式要求
1、外文翻译一律打印,采取A4纸张,页边距一律采取:上、下2.5cm,左3cm,右1.5cm,行间距取多倍行距(设置值为1.25);字符间距为默认值(缩放100%,间距:标准),封面采用教务处统一规定的封面。
2、字数及字体要求
(1)外文翻译所用字体要求为宋体;(2)外文翻译字数要求3000字以上。
3、字号
第一层次题序和标题用小三号黑体字;第二层次题序和标题用四号黑体字;第三层次及以下题序和标题与第二层次同;正文用小四号宋体。
4、页眉及页码
外文翻译各页均加页眉,采用宋体五号宋体居中,打印“河北大学XXXX 届本科生毕业外文翻译”。
页码从正文开始在页脚按阿拉伯数字(宋体小五号)连续编排,居中书写。
5、外文资料及译文
外文资料可用A4纸复印,如果打印,采用小四号Times New Roman字体,译文采用小四号宋体打印。
(要求附原文复印件(A4纸张)
6、外文翻译封面见下页模板
7、电子文档在学院FTP毕业设计相关材料文件夹里
本科生外文文献翻译
外文题目:Hardware-in-the-Loop Simulation System in the Development of Temperature Controller of Plastic Extruder 中文题目
: 硬件在环仿真系统在塑料挤出机温度控制器的发展中的应用
学 院 电子信息工程学院 学科门类 工学 专 业 自动化 学 号 2010448022 姓 名 李珊
装
订
线
指导教师郝雷。
毕业论文正文、文献综述和外文翻译文本编辑排版格式要求
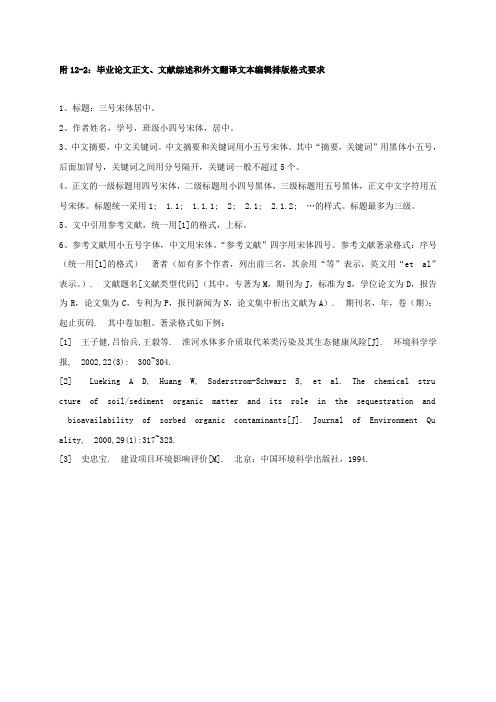
附12-2:毕业论文正文、文献综述和外文翻译文本编辑排版格式要求1、标题:三号宋体居中。
2、作者姓名,学号,班级小四号宋体,居中。
3、中文摘要,中文关键词。
中文摘要和关键词用小五号宋体。
其中“摘要,关键词”用黑体小五号,后面加冒号,关键词之间用分号隔开,关键词一般不超过5个。
4、正文的一级标题用四号宋体,二级标题用小四号黑体,三级标题用五号黑体,正文中文字符用五号宋体。
标题统一采用1; 1.1; 1.1.1; 2; 2.1; 2.1.2; …的样式。
标题最多为三级。
5、文中引用参考文献,统一用[1]的格式,上标。
6、参考文献用小五号字体,中文用宋体。
“参考文献”四字用宋体四号。
参考文献著录格式:序号(统一用[1]的格式)著者(如有多个作者,列出前三名,其余用“等”表示,英文用“et al”表示。
). 文献题名[文献类型代码](其中,专著为M,期刊为J,标准为S,学位论文为D,报告为R,论文集为C,专利为P,报刊新闻为N,论文集中析出文献为A). 期刊名,年,卷(期):起止页码. 其中卷加粗。
著录格式如下例:[1] 王子健,吕怡兵,王毅等. 淮河水体多介质取代苯类污染及其生态健康风险[J]. 环境科学学报, 2002,22(3): 300~304.[2] Lueking A D, Huang W, Soderstrom-Schwarz S, et al. The chemical structure of soil /sediment organic matter and its role in the sequestration and bioavailability of sorb ed organic contaminants[J]. Journal of Environment Quality, 2000,29(1):317~323. [3] 史忠宝. 建设项目环境影响评价[M]. 北京:中国环境科学出版社,1994.。
外文翻译标准格式

毕业设计(论文)
译文及原稿译文题目:[单击此处添加译文题目]
原稿题目:[单击此处添加原稿题目]
原稿出处:[单击此处添加原稿出处]
[单击此处添加译文题目]
[单击此处添加译文正文]
以下是说明文字,正式成文后请删除。
1、模板的使用方法
(1)请不要删除任何具有格式的模板文字。
(2)按照提示直接单击或单击后选择“编辑”→“选择性粘贴”—“无格式文本”就可添加相应内容。
2、在“原稿出处”中对原稿的来源进行说明,包括作者姓名、出处、出版信息等。
期刊类文献书写方法:作者(不超过3人,多者用“等”或“et al”表示).题名[J].刊名,出版年,卷号(期号):起止页码.
论文集类文献书写方法:著者.题名[C].编者.论文集名.出版地:出版社,出版年份:起止页码.
有一篇译文,按如下顺序列出:
译文题目一
原稿题目一
原稿出处一
3、检索和利用外文资料的能力是现阶段毕业设计(论文)环节中所要求掌握的基本能力之一。
外文翻译是在查阅外文文献时,将篇幅适当、内容与选题相关的外文资料翻译成中文。
通过翻译外文资料,可更深层次地了解国外相关领域的现状,同时也是对翻译能力的锻炼,并为考察和检验学生外语能力提供依据。
4、进行外文翻译应注意以下几点:
(1)翻译的外文文献内容应与论文选题相关;一般应选自国外学术期刊、学术会议的文章及其他相关材料。
(2)篇幅、深度和难度适当,可以由1篇文章组成。
5、外文翻译字符应达到2000字符。
[单击此处添加外文原稿题目] [单击此处添加外文原稿正文]。
外文翻译格式说明
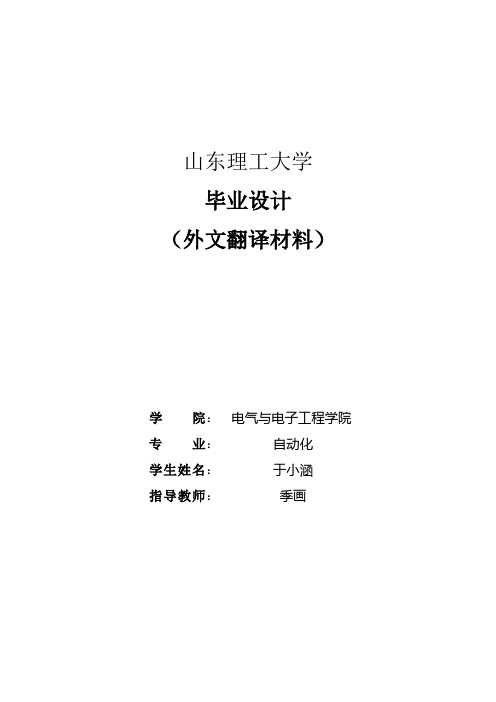
山东理工大学毕业设计(外文翻译材料)学院:专业:学生姓名:指导教师:电气与电子工程学院自动化于小涵季画外文翻译材料格式要求:1.页边距:上3.8磅;下3.8磅;左3.2,右3.2;页眉距边界2.8,页脚距边界32.原文题目:Arial,小三;间距:段前18磅,段后12磅,间距21磅3.原文正文:Times New Roman,小四;间距:段前0磅,段后6磅,间距21磅4.译文题目:黑体,小三;间距:同原文题目5.译文正文:宋体,小四;间距同原文正文6.页眉页脚:原文页眉处写:外文翻译(原文),宋体,五号。
译文页眉处写:外文翻译(译文),宋体,五号。
原文译文的页脚统一编页码(不要单独编页码)。
Plant Model Generation for PLC SimulationHyeong-Tae ParkAbstract:This paper reports an automated procedure for constructing a plant model for PLC simulation. Since PLC programs contain only the control logic without information on the plant model, it is necessary to build the corresponding plant model to perform the simulation. Conventionally, a plant model for PLC simulation has been constructed manually, which requires much effort and indepth knowledge of the simulation. As a remedy for this problem, we propose an automated procedure for generating a plant model from the symbol table of a PLC program. To do so, we propose a naming rule for PLC symbols so that the symbol names include sufficient information on the plant model. By analysing such symbol names, we extract a plant model automatically. The proposed methodology has been implemented and test runs performed.Keywords: agile manufacturing; CAD/CAM; CAPP; simulation1. IntroductionTo survive and prosper in the modern manufacturing era, manufacturers need to continuously improve their products, as well as their production systems. A modern manufacturing line is a highly integrated system composed of automated workstations, such as robots with tool-changing capabilities, a hardware handling system and storage system, and a computer control system that controls the operations of the- 1 -entire system.Since the implementation of a manufacturing line requires heavy investment, proper verification of a line’s operational status sho uld be performed to ensure that the highly automated manufacturing system will successfully achieve the intended benefits. Simulation technology is considered to be an essential tool in the design and analysis of complex systems that cannot be easily described by analytical or mathematical models . Simulation is useful for calculating utilisation statistics, finding bottlenecks, pointing out scheduling errors, and even for creating manufacturing schedules. Traditionally, various simulation languages, including ARENA and AutoMod, have been used for the simulation of manufacturing systems. These simulation languages have been widely accepted both by industry and by academia; however, they remain as analysis tools for the rough design stage of a production line, because their simulation models are not sufficiently realistic to be utilised for a detailed design or for implementation purposes. For example, real production lines are usually controlled by PLC (Programmable Logic Controller) programs (Rullan 1997), but conventional simulation languages roughly describe the control logic with independent entity flows (job flows) between processes.- 2 -Production systems typically consist of simultaneously operating machines, which are controlled by PLCs, currently the most suitable and widely employed industrial control technology. A PLC emulates the behaviour of an electric ladder diagram. As they are sequential machines, to emulate the workings of parallel circuits that respond instantaneously, PLCs use an input/output symbol table and a scanning cycle. When a program is being run in a PLC it is continuously executing a scanning cycle. The program scan solves the Boolean logic related to the information in the input table with that in the output and internal relay tables. In addition, the information in the output and internal relay tables is updated during the program scan. In a PLC, this Boolean logic is typically represented using a graphical language known as a ladder diagram (IEC 2003).Since the abstraction levels of conventional simulators and PLC programs are quite different, the control logic of conventional simulators cannot be reused for the generation of PLC programs. Usually, electrical engineers manually write PLC programs by referring to the rough control logic of conventional simulators, as shown in Figure 1. Since PLC programming is a very tedious and error-prone job, it is essential to verify the PLC programs offline to reduce the stabilisation time of a production system.Previous approaches to a PLC program can be categorised into two groups:- 3 -(1) verification of a given PLC programand (2) generation of a dependable PLC program. In the first group, various software tools have been developed for the verification of PLC based systems via the use of timed automata, such as UPPAAL2k, KRONOS, Supremica and HyTech, mainly for programs written in a statement list language, also termed Boolean (Manesis and Akantziotis 2005). Such software tools verify PLC programs to a certain extent; however, they remain limited. Since they mainly focus on the checking of theoretical attributes (safety, liveness, and reachability), it is not easy for users to determine whether the PLC programs actually achieve the intended control objectives. In the second group, many researchers have focused on the automatic generation of PLC programs from various formalisms including state diagrams, Petri nets, and IDEF0. These formalisms can help the design process of control logics; however, it is still difficult to find hidden errors, which is the most difficult part of verifying a control program.Figure 2. The concept of PLC simulation.To overcome the aforementioned problems, it is necessary to utilise simulation techniques for PLC program verification. By simulating PLC programs, it is possible to analyse the control logic in various ways and recognise hidden errors more intuitively (David 1998). Although PLC simulation can be a very powerful tool for the detailed verification of a production system, the accompanying construction of a plant model is a major obstacle (the counterpart model of a control program). Since PLC programs only contain the control information, without device models, it is necessary to build a corresponding plant model to perform simulation, as shown in- 4 -Figure 2. However, constructing a plant model requires an excessive amount of time and effort. Sometimes, the plant model construction requires much more time than the PLC programming. This serves as the motivation for exploring the possibility of finding an automatic procedure for generating a plant model from a given PLC program.Figure3.Symbol table of a PLC program.Although the objective of a PLC program is not to describe a plant model (device models), the symbol table of a PLC program can provide a glimpse of the plant model. As shown in Figure 3, symbols in a PLC program usually contain some information related to the plant. For example, ‘EXLINE_MB_AGV_P1’ means that the symbol is a signal that is related to the control of an ‘AGV’ (Auto Guided Vehicl e) belonging to the ‘MB’ station of ‘EXLINE’ line. The above scenario reveals the key idea of the present study. If we can develop a proper naming rule for PLC symbols, then it might be possible to extract a plant model by analysing the symbol names.This paper has two major objectives: (1) to propose a proper naming rule for PLC symbols and (2) to develop a procedure for generating a plant model by analysing the symbol names. The application area of the proposed methodology includes all types of automated manufacturing systems controlled by PLC programs, such as automotive production lines, FMSs (flexible manufacturing systems), and ASRSs (automatic storage and retrieval systems). The overall structure of the paper is as follows. Section 2 addresses the specifications of a plant model for PLC simulation. Section 3 describes a naming rule for PLC symbols, which enables the automatic generation of a plant model. Finally, concluding remarks are given in Section 4.2. Plant model for PLC simulationBefore explaining the specification of a plant model enabling PLC simulation, we want to address the importance of the PLC simulation. Chuang et al. (1999) proposed a procedure for the development of an industrial automated production system that- 5 -- 6 -consists of nine steps, as follows: (1) define the process to be controlled; (2) make a sketch of the process operation; (3) create a written sequence of the process; (4) on the sketch, add the sensors needed to carry out the control sequence; (5) add the manual controls needed for the process setup or for operational checks; (6) consider the safety of the operating personnel and make additions and adjustments as needed;(7) add the master stop switches required for a safe shutdown; (8) create a ladder logic diagram that will be used as a basis for thePLC program; and (9) consider the possible points where the process sequence may go astray. The most time-consuming task for the control logic designers is the eighth step, which is usually done by the repetitive method of code writing, testing, and debugging until the control objectives are achieved (Manesis and Akantziotis 2005). This is the reason why conventional PLC programming is often inefficient and prone to human error. As the configurations of production lines and their control programs become more complicated, there is a strong need for a more efficient PLC simulation environment. It is hoped that this paper will take positive steps in this direction.A PLC can be considered as a dedicated computer system having input and output signals. To run a PLC, the corresponding plant model (the counterpart system) is required to interact with the input and output of the PLC. The behaviour of the plant model should be the same as that of the actual system to achieve PLC verification. Since a production line consists of various devices, including robots, transporters, jigs, solenoids, proximity sensors, and light sensors (Groover 2006), we can consider a plant model as a set of device models. To build such a device model, this paper em ploys Zeigler’s DEVS (Discrete Event Systems Specifications) formalism (Zeigler 1984, Kim 1994), which supports the specification of discrete event models in a hierarchical, modular manner. The semantics of the formalism are highly compatible with object-oriented specifications for simulation models. We use the atomic model of the DEVS formalism to represent the behavior of a device model. Formally, an atomic model M is specified by a 7-tuple:M =〈X, S,Y, sin δ,ext δ,λ ,t a 〉X input events setS sequential states setY output events setsin δ S→S: internal transition functionext δQ*X→S: external transition functionQ={(s, e)∣s ∈S, 0≤e ≤t a (s)}: total state of MλS →Y: output function- 7 -t a S →Real: time advance functionThe four elements in the 7-tuple, namely sin δ,ext δ,λand t a , are called the characteristic functions of an atomic model. The atomic model of the DEVS formalism can be considered as a timed-FSA (finite state automata), and it is suitable for describing the behaviour of a device model. Once the device models (plant model) are obtained, it becomes possible to perform the PLC simulation. Currently, device models should be construed manually, which takes much time and effort. To cope with the problem, the objective of the paper is to propose an automated generation procedure for device models.Before explaining the automatic generation procedure of a plant model, let us take a look at the manual procedure to construct device models. To construct a device model, first it is necessary to identify the set of tasks that are assigned to the device. The activation of each task is normally triggered by an external signal from PLC programs. Once the set of tasks is identified for a device, it is then possible to extract the state transition diagram, which defines an atomic model of the DEVS formalism. Figure 4(a) shows a simple example of an AGV (Automatic Guided Vehicle) with two tasks, T1 (movement from p1 to p2) and T2 (movement from p2 to p1). As the two tasks should be triggered by external events, the shell part of the AGV must have two input ports, termed here as Signal_1 and Signal_2, as shown in Figure 4(b).From the set of tasks, it is possible to instantiate the state transition diagram. For this example, there are four states, P1, DoT1, P2 and DoT2. While P1 and P2 take external events from the input ports (Signal_1, Signal_2) for state transitions, DoT1 and DoT2 take internal events that are the end events of the two tasks (T1 and T2). The DEVS atomic model of the virtual device, corresponding to the AGV, can be described as follows:- 8 -Shell of a virtual device:M=〈X,S,Y,sin δ,ext δ,λ ,t a 〉}2_,1_{Signal Signal =X S={P1,DOT1,P2,DOT2} Y={T1Done,T2Done}sin δ(DOT1)=P2 s i nδ(DOT2)=P1 ext δ(P1,Signal_1)=DOT1 ext δ(P2,Signal_2)=DOT2λ(DOT1)=T1Done λ(DOT2)=T2Donet a (DOT1)=Time_1 t a (DOT2)=Time_2Once a plant model has been constructed, it is possible to perform the PLC simulation, which enables the intuitive verification of a PLC program. Figure 5 shows the connections between a PLC program and a plant model. The plant model includes all device models of a production system, and the PLC program contains the control logic for the plant model. To integrate the plant model and the PLC program, it is necessary to define the mapping between the plant model and the PLC program, which is described by I/O mapping. To enable the visual verification of a PLC program, it is necessary to import 3D graphic models, which are controlled by the logical device models (the state transition diagrams). Since 3D graphic models are not always necessary, they are optional for PLC simulation. As mentioned already, the objective of this paper is to extract device models from the symbol names of PLC programs. To do so, it is necessary to develop a proper naming rule for PLC symbols. The naming rule will be addressed in the next section.3. Symbol naming for plant model generationAlthough IEC 61131-3 provides various standard specifications for a PLC, the naming rules of PLC symbols have rarely been brought into focus. Since there have been no standard rules for the naming of PLC symbols, it has been fully dependent on individual PLC programmers.To generate device models from PLC symbols, it is necessary to make PLC symbols that include enough information concerning the plant model. To achieve this objective, we interviewed many PLC programmers and analysed various conventional rules. As a result, we came up with a naming structure consisting of five fields: (1) line name, (2) process number, (3) device name, (4) input or output, and (5) task name (or state name). Figure 6 shows the naming structure for PLC symbols.If the PLC symbols are named according to the proposed naming structure, then it becomes possible to extract device models (atomic models of DEVS formalism) by simply analysing the symbol names. There are two types of symbols (signals), input or output, which are specified by the fourth field. The purpose of the output signal is to trigger a task that is specified by the fifth field. Thus, it is possible to identify the set of tasks of a device by analysing the output symbols. As mentioned already, once the set of tasks is identified for a device, it is then possible to extract the state transition diagram for the device model, which defines an atomic model of the DEVS formalism. While an output signal (symbol) is issued by a PLC to trigger a task, an input signal (symbol) is issued by a device to report the completion of the task to theδand internal transition functions PLC. This means that external transition functionsextδof a device model can be automatically extracted from the output and input sinsymbols, respectively. We demonstrate the generation procedure of a plant modelfrom PLC symbols using an example, as shown in Figure 7.- 9 -- 10 -In the example cell, we assume a part is loaded manually on the AGV by a worker. When the AGV senses the existence of a part, it moves to transfer the part to the machine. After the transfer, the machine performs machining to convert the part into a finished product. In this case, the plant model consists of two device models: an AGV model and a machine model. The PLC program to control the simple manufacturing cell is shown in Figure 8(a), and its symbol table is shown in Figure 8(b).As shown in Figure 8(b), the AGV model has two output symbols and two input symbols. From the output symbols (EX_OP_AGV_O_GOP1, EX_OP_AGV_O_GOP2), we can intuitively recognise that the AGV has two tasks- 11 -(movement from P2 to P1, and movement from P1 to P2). By using the output symbols, we can extract the state transition diagram, as well as the external transitionfunctions, as shown in Figure 9(a).As mentioned already, an output symbol triggers a task of a device model, and an input symbol is made by the device to notify the completion of the task. Since the execution of a task is performed internally by the device, the internal transition functions of a device model can easily be extracted from the input symbols(EX_OP_AGV_I_DONEGOP1, EX_OP_AGV_I_DONEGOP2). In this way, the device model of the machine can be extracted from the related symbols(EX_OP_MC_, etc.), as shown in Figure 9(b). The procedure for the construction of a device model can be described as follows.- 12 -(1) Identify all corresponding pairs between output symbols and input symbols. While an output symbol triggers a task, the corresponding input symbol reports the completion of the task. For example, EX_OP_AGV_O_GOP1 corresponds toEX_OP_AGV_I_DONEGOP1. (2) Define the states of a device mode using the last naming fields of input/output symbols. In the case of the AGV, we can define four states, GoP1, DoneGoP1, GoP2, and DoneGoP2. (3) Define external (internal) transition functions using output (input) symbols. Once a plant model has been obtained, it becomes possible to perform the PLC simulation by defining the I/O mapping relations between the plant model and the PLC symbols. Through the PLC simulation, we can efficiently check whether the PLC program achieves the control objectives or not.The proposed methodology was implemented in C++language, and test runs were made on a personal computer, as shown in Figure 10. The PLC program shown in Figure 8(a) was written using GX IEC developer version 7.0 provided byMitsubishi Electric Corporation. The GX IEC developer can export a symbol table in the form of an Excel file, as shown in Figure 8(b). The exported symbol table becomes the input for the generation of a plant model. Figure 10 shows that the generated device models by analysing the exported symbol table.4. Discussion and conclusionsThrough PLC simulation, it is possible to analyse control logic in various ways and recognise hidden errors more intuitively. Although PLC simulation can be a very powerful tool for the detailed verification of a production system, the accompanying construction of a plant model requires too much time and effort. To remedy this problem, we have proposed an automated procedure to generate a plant model from the symbol table of a PLC program. To do so, we have also proposed a naming rule for PLC symbols so that the symbol names include sufficient information on the plant model. By analysing the symbol names, a plant model can be extracted automatically. Since a plant consists of various manufacturing devices, we can consider a plant model as a set of device models. To represent such a device model, the proposed method employs Zeigler’s DEVS formalism. We use the atomic model of the DEVS formalism to describe the logical behavior of a device model. In other words, it is necessary to extract the device models from the symbol table in the form of an atomic model of the DEVS formalism. Although the proposed methodology only deals with the local verification of PLC programs, it is also possible to extend the methodologyto include the verification of mechanical aspects of the plant .- 13 -工厂模型生成PLC仿真Hyeong-Tae Park摘要 :本文介绍一个自动程序可编程序控制器(PLC)生成工厂模型仿真。
外文译文(格式要求)
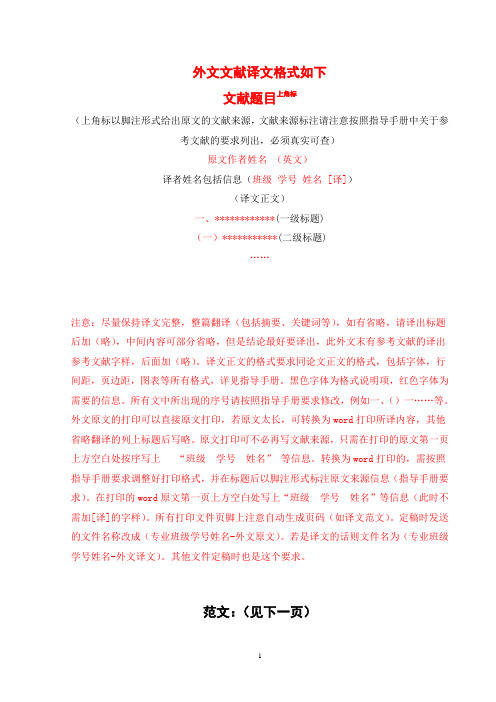
外文文献译文格式如下文献题目上角标(上角标以脚注形式给出原文的文献来源,文献来源标注请注意按照指导手册中关于参考文献的要求列出,必须真实可查)原文作者姓名(英文)译者姓名包括信息(班级学号姓名 [译])(译文正文)一、************(一级标题)(一)***********(二级标题)……注意:尽量保持译文完整,整篇翻译(包括摘要、关键词等),如有省略,请译出标题后加(略),中间内容可部分省略,但是结论最好要译出,此外文末有参考文献的译出参考文献字样,后面加(略)。
译文正文的格式要求同论文正文的格式,包括字体,行间距,页边距,图表等所有格式,详见指导手册。
黑色字体为格式说明项,红色字体为需要的信息。
所有文中所出现的序号请按照指导手册要求修改,例如一、()一……等。
外文原文的打印可以直接原文打印,若原文太长,可转换为word打印所译内容,其他省略翻译的列上标题后写略。
原文打印可不必再写文献来源,只需在打印的原文第一页上方空白处按序写上“班级学号姓名”等信息。
转换为word打印的,需按照指导手册要求调整好打印格式,并在标题后以脚注形式标注原文来源信息(指导手册要求)。
在打印的word原文第一页上方空白处写上“班级学号姓名”等信息(此时不需加[译]的字样)。
所有打印文件页脚上注意自动生成页码(如译文范文)。
定稿时发送的文件名称改成(专业班级学号姓名-外文原文)。
若是译文的话则文件名为(专业班级学号姓名-外文译文)。
其他文件定稿时也是这个要求。
范文:(见下一页)韩国**对经济影响的分析①原作者名可直接用英文经济学 061*班 2006*****6 张三[译][摘要] 本文研究的目的*******的影响。
在探讨*****影响各行业的产出、就业、收入、增值和进口中应用了投入产出模型。
*******************。
根据研究的结果,得出结论*********的作用。
[关键词] ***** 投入-产出模型 **效应 **效应一、引言在当今经济全球化***************。
- 1、下载文档前请自行甄别文档内容的完整性,平台不提供额外的编辑、内容补充、找答案等附加服务。
- 2、"仅部分预览"的文档,不可在线预览部分如存在完整性等问题,可反馈申请退款(可完整预览的文档不适用该条件!)。
- 3、如文档侵犯您的权益,请联系客服反馈,我们会尽快为您处理(人工客服工作时间:9:00-18:30)。
济南大学毕业设计外文资料翻译 - 1 - Powder Technology ,2007,178:114–118)
Regulating characteristics of loop seal in a 65 t/h oil shale-fired circulating fluidized bed boiler
Xiangxin Han, Zhigang Cui, Xiumin Jiang⁎, Jianguo Liu Institute of Thermal Energy Engineering, School of Mechanical Engineering, Shanghai Jiao Tong University, Shanghai, 200240, PR China
摘 要 本文对65t/h油页岩燃烧流化床锅炉的环封调节特性的研究是为了引导一个工业冷却试验。环封的起始特性、空气供给度的影响和密闭液态空气是需要调查的。与其他的校正模型比,保持流化空气速率恒定和调节供应空气流量的联合监控模式可使环封获得更好的调节质量,也为循环流化床锅炉稳定运行提供更可靠的保证。为了防止循环材料在循环底部的沉积和结渣,流化空气流量和空气供应度最好为分别为循环材料的最小流化速度的2-3倍和1.2-1.5倍。这些实验结果可以为调节65t/h循环流化床锅炉的热条件和设计一个新的环封作为一个参考。 关键词 油页岩,循环流化床,环封,调节特性曲线
1.引言 油页岩的燃烧技术主要包括粉燃料炉、气泡流化床和循环流化床。因为它非常低的污染排放量和对低级的化石燃料的良好适应性,油页岩循环流化床技术已经被广泛认为是在所有油页岩的利用率模式中最干净、最经济的途径。 作为一个循环流化床锅炉循环回路的重要组成部分,固体循环回收系统控制固体循环率。一般来说,有两种类型的阀门可用于固体循环系统:一类是是机械阀,另一类是是非机械阀。典型机械阀门有旋转形,螺丝形,蝶形,和滑动形阀门,机械的移动部件驱动并控制燃料的流动率。在高温高压条件下,由于密封和机械等问题,这类阀门不能轻易使用。在高温和一定压力条件下,通常用非机械阀门(环形、L-型、J-型和V-型阀门)。在建筑中由于移动部件的缺乏使得非机械性型阀门更畅销,便宜和简单。因此,从1996年中国华电开始对65t/h油页岩循环流化床锅炉成功应用环流而被投入商业运营,也被认为是最早的大型石油页岩循环流化床锅炉应用在生产生活中的粒子。 为了扩大石油页岩CFB锅炉,作者曾研究65t/h油页岩循环流化床流动结构和燃烧特性的提升途径。本文的主要目的是研究在65t/h密封油页岩循环流化床锅炉的调节特性。 济南大学毕业设计外文资料翻译 - 2 - 2.实验
2.1 实验装置 图1:说明了65t/h石油页岩循环流化床锅炉的原理图。炉高17米,在下游横截面面积为23平方米,在上游逐渐减少到10.47平方米并在空气分配器中实现高流化速度,可以有效地产生怜板状油页岩颗粒。顺着热气体的流动的反方向,锅炉包含一个带水冷却膜的火炉、过热器、蒸发管束,在中等温度的两个气旋,省煤器和空气预热器。65t/h循环流化床锅炉通过使用气旋、灰漏斗、储水管、环封和循环回收管道构成固体回收系统。主蒸汽的压力和温度5.29 MPa和450°C,分别;炉温度可保持在850°C左右主要通过调节空气比、循环灰比例等。江等人已经描述了循环流化床锅炉特性的更多细节。
图1:65 t / h石油页岩CFB锅炉的原理图。所有尺寸都是毫米。(1)燃料加料器,(2) 济南大学毕业设计外文资料翻译
- 3 - 熔炉,(3)过热器,(4)蒸发管束,(5)气旋,(6)灰漏斗,(7)废气加热器,(8)环封,(9)空气预热器。T1--T6:温度测量计的位置。
图2:图2显示了65t/h循环流化床锅炉的环封的结构和尺寸,它的厚度为500毫米。在环封中有三个隔间:一个隔间是紧挨在储水管下面并被称为材料循环的“供应室”;另一个是紧挨在回收管上面的材料循环的“恢复室”;“回收室”是第三室,在供应和恢复室之间。供应和回收室之间的隔间是环封中材料循环的通路。控制循环的空气来自一个单独的在供应室和回收室之的下气箱,由罗茨鼓风机控制。供应室负责“送风”;其他空气通过循环室后形成“流化空气”。流化空气在回收室使循环材料完全流化,防止沉淀到回收室底部。在供应室提供空气可以疏松循环材料并为其通过供应室和回收室之间的通道提供动力,也可以防止循环材料沉淀到供应室底部。
图2.环封结构的示意图。所有尺寸均为毫米。(1)供应室,(2)储水管,(3)回收室,(4)恢复室。
2.2 实验材料 在这项研究中实验材料是65 t / h循环流化床锅炉的循环材料。表1给出了它的 济南大学毕业设计外文资料翻译 - 4 - 物理性质,表明循环材料的颗粒大小不一。粒度范围宽的细颗粒会帮助改善粗糙固体流化特性。即使在一个非常低的流化速度,回收室也会有一个高循环率。因此,循环材料的较宽的粒径范围有利于稳定地操作65t/h锅炉的环封。
2.3 实验方法 在这个实验中,在每个测试之前料斗中必须充满循环材料,因为环封中没有立管流化操作。立管只是用作环封回路中的材料的接收器。空气通过环封的时间需要记录下来。在指定的时间间隔后,从立管底部收集环密封材料并进行称重,然后可以计算循环材料率。
表1:实验中循环材料应用的比率 范围 质量百分数(%) <0.1mm 19.41 0.1-0.25mm 35.09 0.25-0.5mm 32.08 0.5-1mm 7.09 1-2mm 1.69 2-3mm 1.27 >3mm 3.37 相同直径的粒子的直径 0.237 粒子的表面浓度 1450 填充层的体积密度 707.4 最小流化速度 0.177 最终速度 0.961 临界流化态的空隙率 0.57 弧度 0.6
图3:供应室和回收室空气分配器的阻力特性 济南大学毕业设计外文资料翻译
- 5 - 3结果和讨论 3.1 空气分配器的阻力特性 图3说明了没有循环材料的供应室和回收室的空气分配器的阻力特征会陷入循环,表明上两室的空气分配器的空气阻力特性的是相似的。
3.2 环封的起始特性 类似于其他非机械阀门,环封有一个关于最小通风的实验。只有当空气流量达到最低值时,环封开始运作,固体颗粒开始流动。发生这种情况后,材料循环速度会随空气流量的增加而增加。研究者还发现,相比于仅在空气供应处的应用,空气的最小流量对空气流动处和空气供给处的供应是不足的,原因是供给处的空气进入回收室时还需要通过供应和回收室之间的通路有一定的消耗。图4显示了在没有注入新的流化空气时材料循环率与液化空气的关系。根据图4,流化空气在单独作用时最小流量应在80Nm3/h左右。
3.3 流化空气对材料循环率的影响 流化空气是在环封过程中影响循环运输材料、调节和控制循环材料率的一个重要因素。前面的实验表明,固体颗粒在通过回收室旁边的通路(处于回收和恢复室之间)时存在一个停滞区域,空气流动速率非常低。停滞区域随着空气流动速率的上升而逐渐减少。在回收室中,当空气流动速率高到足以使流化所有的固体颗粒时,停滞区将会消失。在环封中为防止固体颗粒的沉积和结渣,空气流动速率的选定至少应等于循环材料的最小流化速度。基于对65t/h锅炉环封的操作经验,这时适于操作的空气流动速率应是2−3倍的最小循环材料流化速度。 图5显示了不同的空气供应率下材料循环率与空气流动速率的关系。根据图5,在供应空气率为57.9 Nm3/h时,材料循环率将随空气流动速率的增加呈线性增加。然而,当空气供应率为83.4Nm3/h、空气流动速率为0Nm3/h时,材料循环率已明显超过40t/h,随后并急剧增加,与此同时随着空气流动速率的逐渐增加。在实验结果的基础上,回收室将获得大量的空气供应量,使液化固体中没有流化空气注入,从而很小速度的流化空气可以使液化回收室的低停滞区的材料循环速度大大加快,这将不利于锅炉的稳定运行。 济南大学毕业设计外文资料翻译
- 6 - 图4:材料循环率与空气流动速率的比较 图5:在不同空气提供率下材料循环率和空气流动速率的比较 3.4 供给空气对材料循环率的影响 供给空气有两个作用:(1)帮助循环材料从储水管向下移动;(2)为循环材料通过供应室和回收室之间的通路提供能量。由于储水管中固体颗粒的阻力很大,只有很少一部分供应空气可以进入储水管,而主要的供应空气将通过回收室进入储水管。图6表明在空气流动速率一直保持恒定材料循环率与供应空气流量的关系。从图6中,可以看到,供应空气不仅可以调节和控制材料循环率,也可以稳定地运输固体颗粒并帮助其循环。为了避免材料的沉积和结渣,空气供应率在实际操作中应选择最小流化材料循环率的1.2-1.5倍。 根据上述分析,无论是调节特性还是保持循环流化床锅炉的稳定运行,保持为恒 济南大学毕业设计外文资料翻译 - 7 - 定和调节提供空气流量的综合调节模式优越于保持供应空气速率恒定和调节空气流化速度的联合监管模式和单一的控制流化空气模式,调节质量还是循环流化床锅炉的稳定操作。
4.结论 在本文中,一个工业冷实验是为了研究出65t/h油页岩循环流化床锅炉的最优调节循环的模式。通过实验结果可以得出以下结论: (1)保持空气流动速率恒定和调节空气供应率的综合监管模式,可使材料循环率随空气供应率的增加成线性增加,这有利于对循环流化床锅炉的稳定操作。 (2)保持空气供应率恒定和调节空气流动速率的综合监管模式,很容易使循环材料率在更高的空气供应率时呈非线性变化。特别是,当空气流动速率很低时,它的一个细微的变化将导致材料循环率大幅度增加,致使循环流化床锅炉不能稳定工作。 (3)无论在调节特性还是维持循环流化床锅炉的稳定运行,保持流化空气速率恒定和调节空气供应量的综合监管模式是优越于保持空气供应率恒定和调节空气流动速率的联合监管模式和单一的调节空气流动速率的模式。
表6:在不同流化空气速率时材料循环率和供应空气速率的比较 (4)为了避免固体颗粒沉积和结渣在环封的底部,建议空气流动速率和空气供应率分别为最小材料循环流化速度的2-3和1.2-1.5倍。