Nature407-491单分子探测文献
近100分的Nature顶级子刊重磅综述揭秘这个研究热点!快收藏!
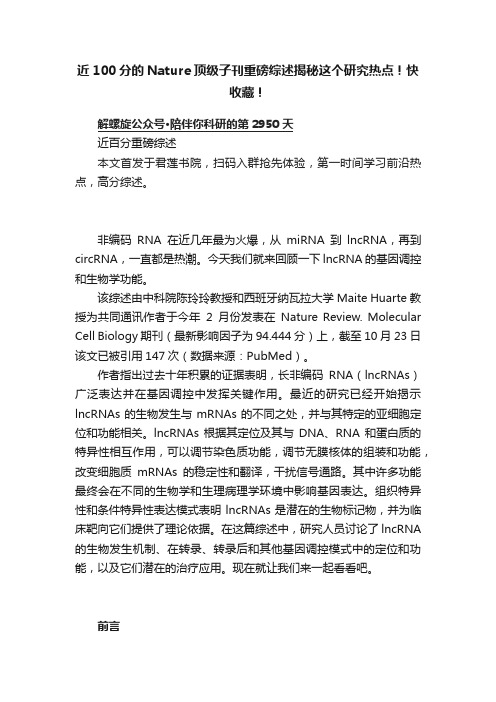
近100分的Nature顶级子刊重磅综述揭秘这个研究热点!快收藏!近百分重磅综述本文首发于君莲书院,扫码入群抢先体验,第一时间学习前沿热点,高分综述。
非编码RNA在近几年最为火爆,从miRNA到lncRNA,再到circRNA,一直都是热潮。
今天我们就来回顾一下lncRNA的基因调控和生物学功能。
该综述由中科院陈玲玲教授和西班牙纳瓦拉大学Maite Huarte教授为共同通讯作者于今年2月份发表在Nature Review. Molecular Cell Biology期刊(最新影响因子为94.444分)上,截至10月23日该文已被引用147次(数据来源:PubMed)。
作者指出过去十年积累的证据表明,长非编码RNA(lncRNAs)广泛表达并在基因调控中发挥关键作用。
最近的研究已经开始揭示lncRNAs的生物发生与mRNAs的不同之处,并与其特定的亚细胞定位和功能相关。
lncRNAs根据其定位及其与DNA、RNA和蛋白质的特异性相互作用,可以调节染色质功能,调节无膜核体的组装和功能,改变细胞质mRNAs的稳定性和翻译,干扰信号通路。
其中许多功能最终会在不同的生物学和生理病理学环境中影响基因表达。
组织特异性和条件特异性表达模式表明lncRNAs是潜在的生物标记物,并为临床靶向它们提供了理论依据。
在这篇综述中,研究人员讨论了lncRNA 的生物发生机制、在转录、转录后和其他基因调控模式中的定位和功能,以及它们潜在的治疗应用。
现在就让我们来一起看看吧。
前言基因组被广泛转录并产生数千个lncRNAs,它们被定义为长度超过200个核苷酸的RNA,不能翻译成功能性蛋白质。
这一宽泛的定义涵盖了一个巨大且高度异质的转录物集合,这些转录物在其生物成因和基因组起源上存在差异。
来自人类基因编码的统计数据表明,人类基因组包含16000多个lncRNAs,但其他估计超过100000个人类lncRNAs。
这些主要包括RNA聚合酶II(Pol II)转录的lncRNAs,也包括其他RNA聚合酶转录的lncRNAs;基因间区的lncRNAs (lincRNAs)以及与其他基因重叠的正反义转录本。
年终盘点:2016年Nature杂志重磅级突破性研究成果
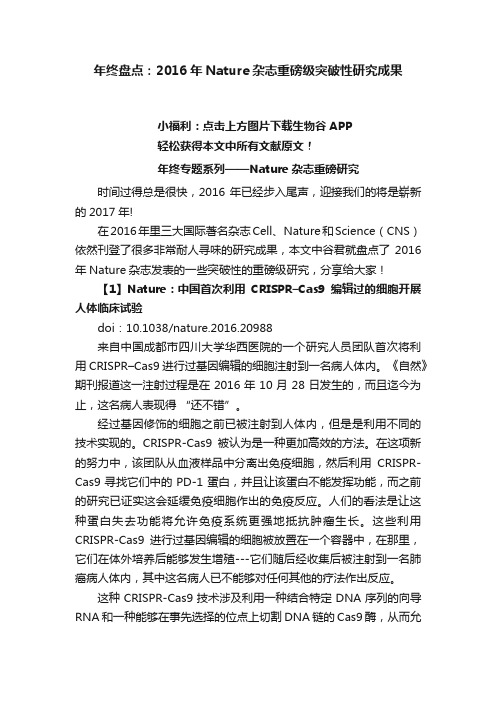
年终盘点:2016年Nature杂志重磅级突破性研究成果小福利:点击上方图片下载生物谷APP轻松获得本文中所有文献原文!年终专题系列——Nature杂志重磅研究时间过得总是很快,2016年已经步入尾声,迎接我们的将是崭新的2017年!在2016年里三大国际著名杂志Cell、Nature和Science(CNS)依然刊登了很多非常耐人寻味的研究成果,本文中谷君就盘点了2016年Nature杂志发表的一些突破性的重磅级研究,分享给大家!【1】Nature:中国首次利用CRISPR–Cas9编辑过的细胞开展人体临床试验doi:10.1038/nature.2016.20988来自中国成都市四川大学华西医院的一个研究人员团队首次将利用CRISPR–Cas9进行过基因编辑的细胞注射到一名病人体内。
《自然》期刊报道这一注射过程是在2016年10月28日发生的,而且迄今为止,这名病人表现得“还不错”。
经过基因修饰的细胞之前已被注射到人体内,但是是利用不同的技术实现的。
CRISPR-Cas9被认为是一种更加高效的方法。
在这项新的努力中,该团队从血液样品中分离出免疫细胞,然后利用CRISPR-Cas9寻找它们中的PD-1蛋白,并且让该蛋白不能发挥功能,而之前的研究已证实这会延缓免疫细胞作出的免疫反应。
人们的看法是让这种蛋白失去功能将允许免疫系统更强地抵抗肿瘤生长。
这些利用CRISPR-Cas9进行过基因编辑的细胞被放置在一个容器中,在那里,它们在体外培养后能够发生增殖---它们随后经收集后被注射到一名肺癌病人体内,其中这名病人已不能够对任何其他的疗法作出反应。
这种CRISPR-Cas9技术涉及利用一种结合特定DNA序列的向导RNA和一种能够在事先选择的位点上切割DNA链的Cas9酶,从而允许移除DNA链,或者加入新的DNA片段。
【2】Nature:实验性疫苗与免疫刺激剂组合使用有望治疗HIV doi:10.1038/nature20583在一项新的研究中,来自美国贝斯以色列女执事医疗中心、沃尔特里德陆军研究院、Janssen疫苗与预防公司(Janssen Vaccines & Prevention B.V.)和吉利德科学公司的研究人员证实将一种实验性疫苗与一种先天性免疫刺激剂结合在一起可能有助导致HIV感染者体内的病毒缓解。
纳米孔单分子测序技术
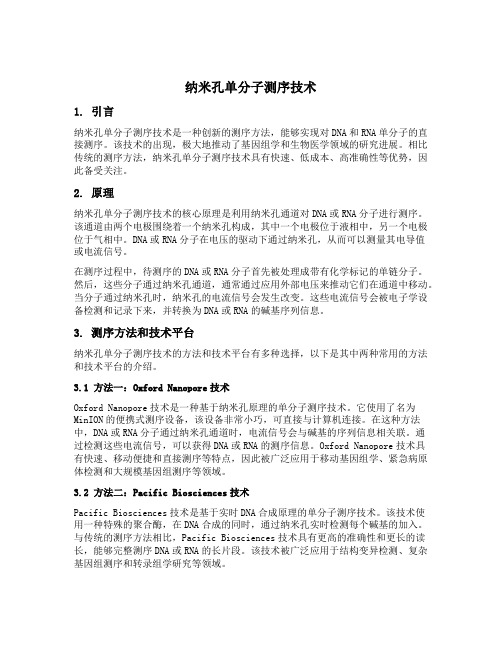
纳米孔单分子测序技术1. 引言纳米孔单分子测序技术是一种创新的测序方法,能够实现对DNA和RNA单分子的直接测序。
该技术的出现,极大地推动了基因组学和生物医学领域的研究进展。
相比传统的测序方法,纳米孔单分子测序技术具有快速、低成本、高准确性等优势,因此备受关注。
2. 原理纳米孔单分子测序技术的核心原理是利用纳米孔通道对DNA或RNA分子进行测序。
该通道由两个电极围绕着一个纳米孔构成,其中一个电极位于液相中,另一个电极位于气相中。
DNA或RNA分子在电压的驱动下通过纳米孔,从而可以测量其电导值或电流信号。
在测序过程中,待测序的DNA或RNA分子首先被处理成带有化学标记的单链分子。
然后,这些分子通过纳米孔通道,通常通过应用外部电压来推动它们在通道中移动。
当分子通过纳米孔时,纳米孔的电流信号会发生改变。
这些电流信号会被电子学设备检测和记录下来,并转换为DNA或RNA的碱基序列信息。
3. 测序方法和技术平台纳米孔单分子测序技术的方法和技术平台有多种选择,以下是其中两种常用的方法和技术平台的介绍。
3.1 方法一:Oxford Nanopore技术Oxford Nanopore技术是一种基于纳米孔原理的单分子测序技术。
它使用了名为MinION的便携式测序设备,该设备非常小巧,可直接与计算机连接。
在这种方法中,DNA或RNA分子通过纳米孔通道时,电流信号会与碱基的序列信息相关联。
通过检测这些电流信号,可以获得DNA或RNA的测序信息。
Oxford Nanopore技术具有快速、移动便捷和直接测序等特点,因此被广泛应用于移动基因组学、紧急病原体检测和大规模基因组测序等领域。
3.2 方法二:Pacific Biosciences技术Pacific Biosciences技术是基于实时DNA合成原理的单分子测序技术。
该技术使用一种特殊的聚合酶,在DNA合成的同时,通过纳米孔实时检测每个碱基的加入。
与传统的测序方法相比,Pacific Biosciences技术具有更高的准确性和更长的读长,能够完整测序DNA或RNA的长片段。
单分子荧光共振能量转移技术
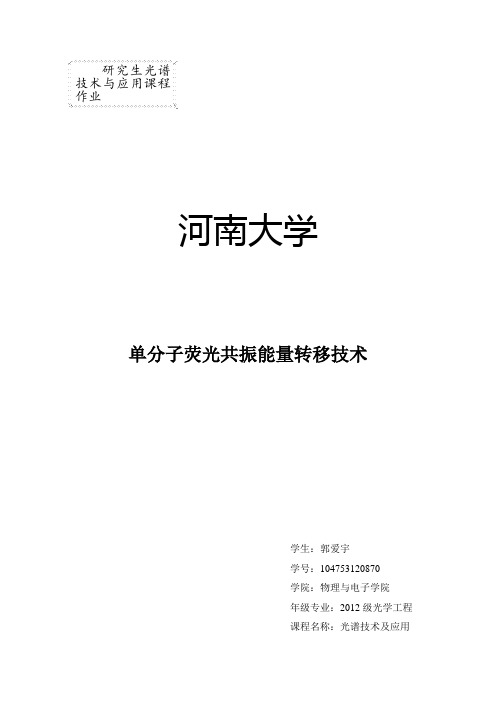
研究生光谱技术与应用课程作业河南大学单分子荧光共振能量转移技术学生:郭爱宇学号:************学院:物理与电子学院年级专业:2012级光学工程课程名称:光谱技术及应用指导老师:郭立俊教授单分子荧光共振能量转移技术摘要:单分子荧光共振能量转移技术(single molecule fluorescence resonance energy transfer, smFRET) 通过检测单个分子内的荧光供体及受体间荧光能量转移的效率,来研究分子构象的变化。
在单分子探测技术发展之前,大多数的分子实验是探测分子的综合平均效应(ensemble averages),这一平均效应掩盖了许多特殊的信息。
单分子探测可以对体系中的单个分子进行研究,得到某一分子特性的分布状况,也可研究生物分子的动力学反应。
介绍了近来单分子荧光共振能量转移技术的进展。
关键词:单分子;荧光共振能量转移;荧光基团1 引言光谱技术是研究生物分子最常用的方法之一。
在单分子光谱(single molecule spectroscopy, SMS)探测技术发展以前,大多数的实验是探测分子的综合平均效应,得到的是由大量对象组成的一个整体所表现出的平均响应和平均值,这一平均效应掩盖了许多特殊的信息。
而单分子探测可对体系中的单个分子进行研究,通过与时间相关过程的探测,能实时了解生物大分子构象变化的信息。
2002年美国第46届生物物理年会表明单分子仍是生物物理学目前和今后重点发展的研究领域。
主要的技术手段包括生物大分子荧光光谱,单分子荧光能量转移谱、与原子力显微镜结合进行单分子水平的分子间相互作用力的测量,以及可进行单分子操作的激光光钳,高时间分辨率的单分子轨迹追踪等[1]。
由此可见,单分子荧光技术具有重要的地位。
标记在生物大分子上单个荧光基团的各种特性变化能够提供有关分子间相互作用、酶活性、反应动力学、构象动力学、分子运动自由度(molecular freedom of motion)及在化学和静电环境下活性改变的信息。
单分子(单链)磁体的研究

LT LS [LS-Co(III)LS-Fe(II)]
HT HS [HSCo(II)LS-Fe(III)]
65.5 K 3.1× 10-10
33.3 K 3.3×10-8
机理 5f-3d
Nature Chem. 2012,4,1011-1017
-1
-12.0
-7
-12.2
-12.3 0.30
-12.4
0.55
0.5
0.6
0.7
0.8
0.9
1.0
1.1
1.2
T /K
-1
D
-5
Linear Fit
-6
-7
S
-8
n=9.7
-9
-10
-3 -4 -5 -6 -7 -8 -9 -10 -11 0.5 0.6 0.7 0.8 0.9 1.0 1.1 1.2 1.3 1.4
任旻 主题报告 20130922
• 多功能:铁电 介电 自旋交叉。。。 • 机理:高能垒 弛豫 构效关系。。。 • 调控:光 电 溶剂 配体 取代基 。。。
多功能
JASC: /10.1021/ja407570n
2: HS J = −0.96(3) K and g = 2.02(5). 3: LS J1= −1.09(9) K, J2 = +0.04(1) K, and g = 2.00(5).
单分子(单链)磁体的研究进展
JASC: /10.1021/ja407570n Nature Chem. 2012,4,921-926 Nature Chem. 2012,4,1011-1017 Nature Chem. 2013,5,577-581 JACS: /10.1021/ja403154z Angew. Chem. Int. Ed. 2013, 52, 9130 –9134 Inorg. Chem. 2012, 51, 8538−8544 Nature Chem. 2013,5,673-678 Chem. Eur. J. 2013, 19, 12982 – 12990 JACS: /10.1021/ja405284t J. Am. Chem. Soc. 2013, 135, 13242−13245
作为单分子磁体的分子自旋电子研究进展
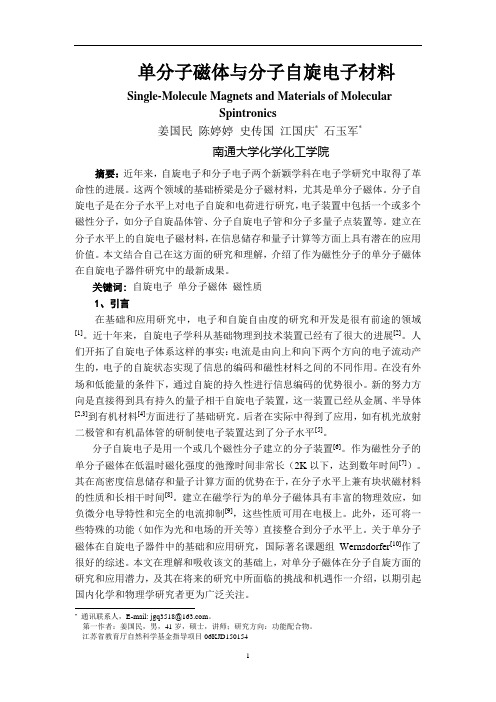
单分子磁体与分子自旋电子材料Single-Molecule Magnets and Materials of MolecularSpintronics姜国民陈婷婷史传国江国庆*石玉军*南通大学化学化工学院摘要:近年来,自旋电子和分子电子两个新颖学科在电子学研究中取得了革命性的进展。
这两个领域的基础桥梁是分子磁材料,尤其是单分子磁体。
分子自旋电子是在分子水平上对电子自旋和电荷进行研究,电子装置中包括一个或多个磁性分子,如分子自旋晶体管、分子自旋电子管和分子多量子点装置等。
建立在分子水平上的自旋电子磁材料,在信息储存和量子计算等方面上具有潜在的应用价值。
本文结合自己在这方面的研究和理解,介绍了作为磁性分子的单分子磁体在自旋电子器件研究中的最新成果。
关键词:自旋电子单分子磁体磁性质1、引言在基础和应用研究中,电子和自旋自由度的研究和开发是很有前途的领域[1]。
近十年来,自旋电子学科从基础物理到技术装置已经有了很大的进展[2]。
人们开拓了自旋电子体系这样的事实:电流是由向上和向下两个方向的电子流动产生的,电子的自旋状态实现了信息的编码和磁性材料之间的不同作用。
在没有外场和低能量的条件下,通过自旋的持久性进行信息编码的优势很小。
新的努力方向是直接得到具有持久的量子相干自旋电子装置,这一装置已经从金属、半导体[2,3]到有机材料[4]方面进行了基础研究。
后者在实际中得到了应用,如有机光放射二极管和有机晶体管的研制使电子装置达到了分子水平[5]。
分子自旋电子是用一个或几个磁性分子建立的分子装置[6]。
作为磁性分子的单分子磁体在低温时磁化强度的弛豫时间非常长(2K以下,达到数年时间[7])。
其在高密度信息储存和量子计算方面的优势在于,在分子水平上兼有块状磁材料的性质和长相干时间[8]。
建立在磁学行为的单分子磁体具有丰富的物理效应,如负微分电导特性和完全的电流抑制[9],这些性质可用在电极上。
此外,还可将一些特殊的功能(如作为光和电场的开关等)直接整合到分子水平上。
【国家自然科学基金】_傅里叶变换红外光谱(ftir)_基金支持热词逐年推荐_【万方软件创新助手】_20140803
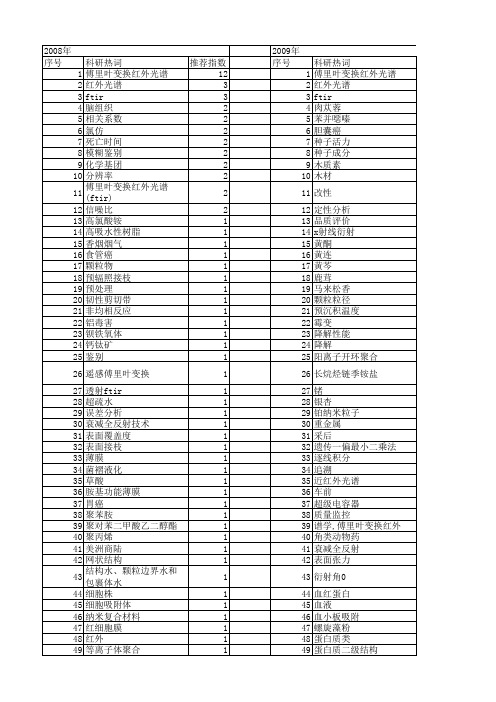
1 1 1 1 1 1 1 1 1 1 1 1 1 1 1 1 1 1 1 1 1 1 1 1 1 1 1 1 1 1 1 1 1 1 1 1 1 1 1 1 1 1 1 1 1 1 1 1 1 1 1 1 1 1
53 54 55 56 57 58 59 60 61 62 63 64 65 66 67 68 69 70 71 72 73 74 75 76 77 78 79 80 81 82 83 84 85 86 87 88 89 90 91 92 93 94 95 96 97 98 99 100 101 102 103 104 105 106
科研热词 推荐指数 傅里叶变换红外光谱 12 红外光谱 3 ftir 3 脑组织 2 相关系数 2 氯仿 2 死亡时间 2 模糊鉴别 2 化学基团 2 分辨率 2 傅里叶变换红外光谱(ftir) 2 信噪比 2 高氯酸铵 1 高吸水性树脂 1 香烟烟气 1 食管癌 1 颗粒物 1 预辐照接枝 1 预处理 1 韧性剪切带 1 非均相反应 1 铝毒害 1 钡铁氧体 1 钙钛矿 1 鉴别 1 遥感傅里叶变换 1 透射ftir 1 超疏水 1 误差分析 1 衰减全反射技术 1 表面覆盖度 1 表面接枝 1 薄膜 1 菌褶液化 1 草酸 1 胺基功能薄膜 1 胃癌 1 聚苯胺 1 聚对苯二甲酸乙二醇酯 1 聚丙烯 1 美洲商陆 1 网状结构 1 结构水、颗粒边界水和包裹体水 1 细胞株 1 细胞吸附体 1 纳米复合材料 1 红细胞膜 1 红外 1 等离子体聚合 1 磁性材料 1 硼碳氮薄膜 1 硫酸掺杂的含钛高炉渣 1
107 108 109 110 111 112 113 114 115 116 117 118 119 120 121 122 123 124 125 126 127 128 129 130 131 132 133 134 135 136 137 138
单分子荧光技术及其应用研究

单分子荧光技术及其应用研究单分子荧光(Single-molecule fluorescence,SMF)技术是一种新兴的生物学、化学及材料学交叉学科的分析技术。
相较于传统分析技术,这种技术能够在单个分子水平上对样品进行分析,减少了分析误差,并且能够捕捉到“隐藏”在平均测量值中的单个分子信号。
这种技术可以用于研究生物分子的结构与功能、材料表征、化学反应动力学等领域,在这些领域中发挥了重要的作用。
一、单分子荧光技术的原理单分子荧光技术是利用荧光分子在激发光的作用下发射出可见光的性质,研究单个分子的分子特性,在不同激发条件下记录荧光信号。
单分子荧光技术的原理可以简单概括为:在低浓度下,荧光分子发出的光信号可以被探测到,同一荧光分子时刻只能发出一个光子,每个光子可以探测到,这样就实现了单分子的探测。
二、单分子荧光技术的应用1. 生物分子结构与功能研究单分子荧光技术在生物分子研究领域中扮演者重要的角色,可以研究生物分子如核酸、蛋白质、糖类分子等的结构和功能。
在这些研究中,研究者通常会加入荧光染料,将其与生物分子偶联,然后通过单分子荧光技术来观察这些分子的结构和功能。
例如,在研究蛋白质时,研究者可以通过单分子荧光技术来观察蛋白质分子的结构、构象变化及相互作用等。
此外,单分子荧光技术还常用于植物生长激素研究等领域。
2. 化学反应动力学研究单分子荧光技术在化学反应动力学研究中也有广泛的应用。
化学反应通常在小尺度和短时间内进行,无法被传统的测量技术观察到,而单分子荧光技术可以通过检测单个分子的荧光信号来研究化学反应过程及动力学性质。
例如,可以用荧光探针标记分子,然后在某种环境下模拟特定的反应条件,利用单分子荧光技术来观察反应速率、反应产物以及反应机理等。
此外,单分子荧光技术还可应用于研究材料的表征。
3. 荧光探针研究单分子荧光技术可以将荧光探针和基于荧光的传感器带入到生物内部,对生物组织进行标记和监测。
例如,可以使用荧光探针来标记癌细胞,在单分子水平上研究其生长、分化和死亡的机制,并用于诊断或治疗癌症等疾病。
- 1、下载文档前请自行甄别文档内容的完整性,平台不提供额外的编辑、内容补充、找答案等附加服务。
- 2、"仅部分预览"的文档,不可在线预览部分如存在完整性等问题,可反馈申请退款(可完整预览的文档不适用该条件!)。
- 3、如文档侵犯您的权益,请联系客服反馈,我们会尽快为您处理(人工客服工作时间:9:00-18:30)。
letters to nature................................................................. Single photons on demand from a single molecule at room temperature B.Lounis*²&W.E.Moerner**Department of Chemistry,Stanford University,Stanford,California94305-5080,USA²CPMOH,UniversiteÂBordeaux I,33405Talence,France .............................................................................................................................................. The generation of non-classical states of light1is of fundamental scienti®c and technological interest.For example,`squeezed' states2enable measurements to be performed at lower noise levels than possible using classical light.Deterministic(or trig-gered)single-photon sources exhibit non-classical behaviour in that they emit,with a high degree of certainty,just one photon at a user-speci®ed time.(In contrast,a classical source such as an attenuated pulsed laser emits photons according to Poisson statistics.)A deterministic source of single photons could®nd applications in quantum information processing3,quantum cryptography4and certain quantum computation problems5. Here we realize a controllable source of single photons using optical pumping of a single molecule in a solid.Triggered single photons are produced at a high rate,whereas the probability of simultaneous emission of two photons is nearly zeroÐa useful property for secure quantum cryptography.Our approach is characterized by simplicity,room temperature operation and improved performance compared to other triggered sources of single photons.Various schemes have been proposed to create a single-photon source,for example,involving single atoms in cavities6±8,or highly nonlinear cavities9.Until now,such sources have only been demon-strated in two experiments at cryogenic temperatures.The®rst such demonstration utilized a``turnstile''effect10based on a Coulomb blockade for electrons and holes in a mesoscopic double-barrier p±n junction11.A dilution refrigerator operating at50mK was essential to minimize the thermal energy background.Owing to the sample geometry,the detection ef®ciency was limited to about 10-4,which made it dif®cult to measure the second-order intensity correlation of the emitted photons,an important diagnostic of the non-classical nature of the light.A second approach is based on the controlled excitation of single molecules in a solid12.A rapid adiabatic passage method is used to prepare the molecule in its¯uorescent state.Very narrow optical absorption lines are needed for this method,which requires liquid helium temperatures(2K).The detection ef®ciency was limited to about3´10-3,mainly because®ltering out the pumping laser excitation also eliminates single-molecule¯uorescence near the laser frequency.Our source of single photons on demand is based on a simple concept,the pulsed optical excitation of a single highly¯uorescent molecule as illustrated in Fig.1a.A short pulse of green laser light pumps the four-level scheme of the molecule from the ground singlet state to a vibronically excited level of the®rst electronic excited singlet state.After fast(picoseconds)relaxation to the lowest electronic excited state,the molecule subsequently emits a single photon.Because the molecule can be pumped at high intensity,the probability of preparing the emitting state can approach unity.This scheme has the further advantage of spectrally separating the laser excitation and the¯uorescence emission.Fluorescence microscopy at ambient conditions of single emissive dye molecules13,14embedded in polymers,droplets and gels or dispersed on surfaces has revealed various previously unknown effects that are normally obscured by averaging in conventional ensemble measurements.Digital photobleaching15is an example; however,it imposes an upper limit on the number of photons detectable from a molecule.The photobleaching quantum ef®ciency of most single molecules has been reported to range from about10-4to about10-7in various host matrices,which is a severe limitation for quantum optical experiments16±18.However,abcFigure1Scheme of the experimental measurements with images of single molecules.a,Pumping scheme of the molecules to their¯uorescent state.b,Chemical structures ofterrylene(upper)and p-terphenyl(lower).The sample is a sublimed crystal¯ake(a fewmicrometres in thickness)of p-terphenyl doped with terrylene at low concentrations ofabout10-11moles of terrylene per mole of p-terphenyl.Terrylene¯uoresces at around579nm in the p-terphenyl crystal,and has a¯uorescence quantum yield close to unity.c,Confocal¯uorescence image(10m m´10m m)of single terrylene moleculesembedded in crystalline p-terphenyl with continuous-wave excitation at532nm of about1.5m W,with signal-to-background ratio greater than5.d,Schematic representation ofthe scanning confocal microscope set-up used to characterize the single-moleculeemission.The laser excitation was provided by a continuous-wave532nm frequency-doubled Nd-YAG laser for the antibunching measurement.For the pulsed excitation weused an actively mode-locked picosecond Nd-YAG laser from Lightwave Electronics(model131,pulse width35ps,repetition rate100MHz,average power220mW).A pulsepicker was used to reduce the repetition rate to6.25MHz.The pulsed green light wasproduced by single pass second-harmonic generation in a periodically poled lithiumniobate crystal28(a maximum average green power of0.2mW was obtained for anaverage near infrared power of10mW).The excitation beam was directed onto thesample by the dichroic mirror of an inverted microscope and was focused on the sampleplane by a1.4NA(numerical aperture)oil-immersion objective.The¯uorescence photonswere collected by this objective,and®ltered from the residual excitation light by aholographic notch®lter and long pass glass®lter.The emitted¯uorescence was sentthrough a50/50beam splitter onto two(start and stop)Si avalanche photon-countingphotodiodes.The histogram of the delays between detected photons was measured usinga time-to-amplitude converter and a pulse-height analyser.The stop signal was delayedfor negative times.Time delay (ns)CountsFigure2Characterization of the average time structure of the source.The arrival times of¯uorescence photons are recorded with one detector using a time-to-amplitude convertertriggered by the laser pulse.The average power of the pulsed laser is6m W and theacquisition time is60s.Circles represent the histogram of arrival times of detectedphotons after the pump pulse at t=0.The line is a single exponential decay®t(decay timeof3.8ns).Inset,wide time range.highly stable single terrylene molecules have recently been detected in p-terphenyl molecular crystals at room temperature 19,20(for structures see Fig.1b).In this organic crystal,the ¯uorescent molecules are protected by the host from exposure to diffusing quenchers (such as oxygen),and pro®t from the ability to emit host phonons to prevent thermally induced damage.We ®nd that for thick crystals (,10m m),this system indeed has extremely high photostability,allowing hours of continuous illumination without photobleaching.Figure 1c shows a scanning confocal microscope image of the ¯uorescence from single terrylene molecules embedded in a thin,sublimed platelet of crystalline p-terphenyl.The isolated peaks (,400nm full-width at half-maximum)represent the emission of single molecules.Saturation studies that we performed on this system show that maximum emission rates as high as 2.5MHz are achievable,and typical saturation intensities are close to 1MW cm -2.The high saturation intensities are the result of the orientation of the molecular transition dipole moment which is nearly perpendicular to the plane of the sample and hence to the laser polarization.The light emitted by a single molecule excited by a continuous-wave laser consists of single photons separated by random time intervals which depend on the excited state lifetime and the pumping rate.Because a single molecule can never emit two photons at once,the distribution of photon pairs separated by a time t rises from zero at t =0.This phenomenon,called photon antibunching,has previously been observed for single molecules at low 21and room temperature ing the standard Hanbury-Brown and Twiss coincidence set-up as shown in Fig.1d,we observed strong antibunching correlations (data not shown),an unequivocal signature of the single-molecule nature of the peaks shown in Fig.1c.To use the non-classical ¯uorescence properties of the single molecule directly,we trigger the single-photon emission by pump-ing with a periodic,pulsed laser source (pulse width t p =35ps,repetition rate n =6.25MHz).Single photons are then generated at predetermined times,within the accuracy of the emission lifetime of a few nanoseconds.We used the standard time-correlated single-photon counting technique to measure the average time structure of our source (see Fig.2).The inset shows that the photons are emitted at times separated by the laser repetition time (160ns).As expected,the main ®gure shows that the excited state population rises in a very short time,demonstrating the rapid pumping of the molecule to its emitting state.The distribution then decays exponentially with a time constant of t f =3.8ns,consistent with the lifetime of terrylene in p-terphenyl as deduced from the homogeneous width of approximately 40MHz at low temperature 23.We now discuss the probabilities p (m )that m photons will be emitted by the molecule after each pulse.Since the pump pulse width is very short compared to the ¯uorescence lifetime,the probability that two photons will be emitted per pulse,p (2),is very small (see below).Thus the detected count rate from the molecule,S ,is directly proportional to the probability p (1)that a single photon is emitted after each laser pulse:S =h n p (1)where h is the detection ef®ciency.By consideration of the losses in ®lters and optics as well as the collection ef®ciency for a molecular dipole oriented nearly perpendicular to the plane of the microscope stage 24,we ®nd h <6%.To estimate the maximum value of p (1),we performed a power saturation study of the detected count rate.In Fig.3we show the measured S as a function of the average excitation power of the pulsed laser.The signal shows power saturation behaviour that is well ®tted by the saturation law of a two-level system from rate equations,S =S `(I /I s )(1+I /I s )-1[1-exp{-(1+I /I s )t p /t f }]=S `p (1)where I and I s are respectively the excitation and the saturation intensities.From the ®t we obtain a saturation count rate S `=34366kHz and I s =1.260.1MW cm -2(in agreement with continuous-wave measurements,not shown).The maximum measured count rate in our experiment reached 310kHz and was limited only by the available laser power.The maximum achieved p (1)can be determined in two ways:using our maximum pumping intensity and I s determined above,p (1)=88%;using the maximum observed S and h ,p (1)=83%,in good agreement.A small value of double-photon emission,p (2),is essential to the usefulness of a single-photon source.For example,quantum key distribution using polarization states of photons requires immunity from a beam-splitter attack 25,where a large value of p (2)allows an intruder to obtain information without being detected.Two-photon emission requires that the molecule must ®rst be excited,then a photon must be emitted,and then the molecule must beletters to natureAverage power (µW)D e t e c t e d c o u n t r a t e (k H z )Figure 3Determination of p (1).The ®gure shows the intensity dependence of the emission rate from the triggered single photon source (dots)with a two-level model ®t to the saturation behaviour (solid line).The maximum measured count rate is 310kHz,and the expected saturation count rate from the ®t is S `=343kHz,with I s =1.2MW cm -2.C o i n c i d e n c e s0200400600Delay (ns)–160–800801601020a bFigure 4Second-order intensity correlation function of the emitted photons.The traces show the histogram of delays between detected photons under pulsed excitation with 6m W average power measured with the start±stop apparatus of Fig.1d.a ,A single molecule is excited and the ratio of the area of the central peak to the lateral ones is 0.27.The area of the central peak is proportional to (B 2+2SB ),where B is the average rate detected from the background.In addition to the background±background events,a larger contribution arises from the signal±background and background±signal events for the start±stop coincidences.Similarly,the area of each lateral peak is proportional to (B +S )2.The ratio of the area of the central peak to the lateral peak is in good quantitative agreement with the ratio expected from the measured average count rate for the signal and the background (S /B <6).b ,When the laser spot is positioned away from any molecule,all peaks have the same area,as expected for a poissonian source.The data were accumulated for a ,120s and b ,300s.excited a second time,all within35ps.We have estimated the value of p(2)and®nd it to be less than8´10-4at our maximum intensity.To directly demonstrate the non-classical sub-poissonian statis-tics of our source,we measured the intensity correlation function of the emitted light with the coincidence set-up of Fig.1d.For both the single-molecule(Fig.4a)and background(Fig.4b)cases,the histograms show the expected peak pattern,given the time pattern of the photon emission in the inset of Fig.2.For poissonian light, such as that from an attenuated pulsed laser or the¯uorescence from the background excited by the laser pulses,the central peak is identical in intensity and shape to the lateral ones(Fig.4b).In the case of a single molecule,the central peak should vanish altogether as no more than one single photon can be emitted by the molecule. The ratio of the central peak's area to the area of the lateral peaks is the signature of the sub-poissonian statistics of the light emitted by our source.The residual peak at zero delay in Fig.4a arises from coincidence events involving background photons excited during each laser pulse.In our experiment,the background signal shows a lifetime of about4ns,which indicates that it arises from weak ¯uorescence from out-of-focus terrylene molecules,not from Raman scattering.It is convenient to compare the probability distribution p(m)for our source to that expected from a Poisson distribution by means of the Mandel parameter Q s=(j2-n av)/n av,where j2is the variance of the distribution and n av is the average number of photons26.At the highest pumping power,the probabilities of our source are p(0)=0.14,p(1)=0.86and p(m.1)<0.This distribution is radically different from that for a pulsed coherent source with the same n av=0.86:p coh(0)=0.42,p coh(1)=0.36,p coh(2)=0.16,¼.The Mandel parameter of our source is Q s=-0.86,not far from-1,the value expected for a perfect single-photon emitter,and far from0, the value for a poissonian source.The Mandel parameter Q d of the detected photon counts is naturally affected by the light detection ef®ing Q d=Q s(h/2),we®nd Q d<-3%.The parameters of our source(repetition rate and single-photon generation probability)are limited only by the laser system used; nevertheless,the current performance already surpasses that of previous work.This high performance combined with the simpli-city of our source may make it suitable for a variety of quantum optical experiments and for other applications where triggered single photons are needed.Photons are emitted into a range of solid angles,which can limit the detection ef®ciency;however, optical solutions to this problem can be envisaged,that is,one can imagine that the single molecule could be coupled to a single cavity mode to reduce losses,change the emission pattern,or modify the emission lifetime and thus increase the emission rate.To reduce the background(from out-of-focus molecules or Raman scattering),reduced terrylene doping,pumping with z-axis polarized light,or use of a crystalline system with a more favourable orientation of the single absorber can be utilized.With further development,single molecules in solids may soon provide compact and reliable sources of single photons.MReceived27January;accepted15August2000.1.Meystre,P.&Sargent,M.III Elements of Quantum Optics(Springer,Berlin,1991).2.Drummond,P.D.Quantum Squeezing(Springer,Berlin,1999).3.Special issue on quantum information.Phys.World11(3),(1998).4.Bennett,C.H.,Brassard,G.&Ekert,A.K.Quantum cryptography.Sci.Am.267(4),50±57(1992).5.Turchette,Q.A.,Hood,C.J.,Lange,W.,Mabuchi,H.&Kimble,H.J.Measurement of conditionalphase shifts for quantum logic.Phys.Rev.Lett.75,4710±4713(1995).6.Parkins,A.S.,Marte,P.,Zoller,P.,Carnal,O.&Kimble,H.J.Quantum-state mapping betweenmultilevel atoms and cavity light®elds.Phys.Rev.A51,1578±1596(1995).7.Cirac,J.I.,Zoller,P.,Kimble,H.J.&Mabuchi,H.Quantum state transfer and entanglementdistribution among distant nodes in a quantum network.Phys.Rev.Lett.78,3221±3224(1997). 8.Kuhn,A.,Hennrich,M.,Bondo,T.&Rempe,G.Controlled generation of single photons from astrongly coupled atom-cavity system.Appl.Phys.B69,373±377(1999).9.Imamoglu,A.,Schmidt,H.,Woods,G.&Deutsch,M.Strongly interacting photons in a nonlinearcavity.Phys.Rev.Lett.79,1467±1470(1997).10.Imamoglu,A.&Yamamoto,Y.Turnstile device for heralded single photons:coulomb blockade ofelectron and hole tunneling in quantum con®ned p-i-n heterojunctions.Phys.Rev.Lett.72,210±213 (1994).11.Kim,J.,Benson,O.,Kan,H.&Yamamoto,Y.A Single-photon turnstile device.Nature397,500±503(1999).12.Brunel,C.,Lounis,B.,Tamarat,P.&Orrit,M.Triggered source of single photons based on controlledsingle molecule¯uorescence.Phys.Rev.Lett.83,2722±2725(1999).13.Xie,X.S.&Trautman,J.K.Optical studies of single molecules at room temperature.Annu.Rev.Phys.Chem.49,441±480(1998).14.Moerner,W.E.&Orrit,M.Illuminating single molecules in condensed matter.Science283,1670±1676(1999).15.Ambrose,W.P.,Goodwin,P.M.,Martin,J.C.&Keller,R.A.Single-molecule detection andphotochemistry on a surface using near-®eld optical-excitation.Phys.Rev.Lett.72,160±163 (1994).16.Ambrose,W.P.et al.Fluorescence photon antibunching from single molecules on a surface.Chem.Phys.Lett.269,365±370(1997).17.De Martini,F.,Di Giuseppe,G.&Marrocco,M.Phys.Rev.Lett.76,900±903(1996).18.Kiston,S.C.,Jonsson,P.,Rarity,J.G.&Tapster,P.R.Intensity¯uctuation spectroscopy of smallnumbers of dye molecules in a microcavity.Phys.Rev.A58,620±627(1998).19.Fleury,L.,Sick,B.,Zumofen,G.,Hecht,B.&Wild,U.P.High photo-stability of single molecules in anorganic crystal at room temperature observed by scanning confocal optical microscopy.Mol.Phys.95, 1333±1338(1998).20.Kulzer,F.,Koberling,F.,Christ,T.,Mews,A.&Basche,T.T errylene in p-terphenyl:single moleculeexperiments at room temperature.Chem.Phys.247,23±34(1999).21.BascheÂ,T.,Moerner,W.E.,Orrit,M.&Talon,H.Photon antibunching in the¯uorescence of a singledye molecule trapped in a solid.Phys.Rev.Lett.69,1516±1519(1992).22.Fleury,L.,Segura,J.-M.,Zumofen,G.,Hecht,B.&Wild,U.P.Nonclassical photon statistics in singlemolecule¯uorescence at room temperature.Phys.Rev.Lett.84,1148±1151(2000).23.Kummer,S.,BascheÂ,T.&BraÈuchle,C.Terrylene in p-terphenyl:a novel single crystalline system forsingle molecule spectroscopy at low temperatures.Chem.Phys.Lett.229,309±316(1994).24.Plakhotnik,T.,Moerner,W.E.,Palm,V.&Wild,U.P.Single molecule spectroscopy:maximumemission rate and saturation mun.114,83±88(1995).25.Buttler,W.T.et al.Quantum key distribution over1km.Phys.Rev.Lett.81,3283±3286(1998).26.Short,H.&Mandel,L.Observation of sub-poissonian photon statistics.Phys.Rev.Lett.51,384±387(1983).27.Mandel,L.Sub-poissonian photon statistics in resonance¯uorescence.Opt.Lett.4,205±207(1979).ler,G.D.et al.42%-ef®cient single-pass cw second-harmonic generation in periodically poledlithium niobate.Opt.Lett.22,1834±1836(1997).AcknowledgementsWe thank Lightwave Electronics for use of the mode-locked Nd-YAG laser,M.Fejer and M.Fayer for providing non-linear crystals,and the Stanford Synchrotron Radiation Laboratory for providing the pulse height analyser.B.L.thanks D.Wright for assistance and NATO for Fellowship support.Correspondence and requests for materials should be addressed to W.E.M.(e-mail:moerner@).letters to nature................................................................. Logical computation using algorithmic self-assemblyof DNA triple-crossover molecules Chengde Mao*,Thomas Bean²,John H.Reif²&Nadrian C.Seeman**Department of Chemistry,New York University,New York,10003,USA&²Department of Computer Science,Duke University,Durham,North Carolina27707,USA .............................................................................................................................................. Recent work1±3has demonstrated the self-assembly of designed periodic two-dimensional arrays composed of DNA tiles,in which the intermolecular contacts are directed by`sticky'ends.In a mathematical context,aperiodic mosaics may be formed by the self-assembly of`Wang'tiles4,a process that emulates the opera-tion of a Turing machine.Macroscopic self-assembly has been used to perform computations5;there is also a logical equivalence between DNA sticky ends and Wang tile edges6,7.This suggests that the self-assembly of DNA-based tiles could be used to per-form DNA-based computation8.Algorithmic aperiodic self-assembly requires greater®delity than periodic self-assembly,。