09_ITG524_Heidelberg_Brueck Backhaul Requirements for Centralized and
Improved activity and thermostability of Bacillus pumilus lipase by directed

Journal of Biotechnology 164 (2013) 123–129Contents lists available at SciVerse ScienceDirectJournal ofBiotechnologyj o u r n a l h o m e p a g e :w w w.e l s e v i e r.c o m /l o c a t e /j b i o t ecImproved activity and thermostability of Bacillus pumilus lipase by directed evolutionNagihan Akbulut a ,∗,Merve Tuzlako˘g lu Öztürk a ,Tjaard Pijning b ,Saliha ˙I s ¸sever Öztürk a ,Füsun Gümüs ¸el a ,1a Department of Molecular Biology and Genetics,Gebze Institute of Technology (GIT),41400Kocaeli,TürkiyebLaboratory of Biophysical Chemistry,Groningen Biomolecular Sciences and Biotechnology Institute (GBB),University of Groningen,Nijenborgh 7,9747AG Groningen,The Netherlandsa r t i c l ei n f oArticle history:Received 11September 2012Received in revised form 20December 2012Accepted 21December 2012Available online 11 January 2013Keywords:Bacillus pumilus lipase BiocatalystDirected evolution DNA shuffling Thermostability3D homology modela b s t r a c tTo improve enzymatic activity of Bacillus pumilus lipases,DNA shuffling was applied to two lipase genes from local B.pumilus ing a high-throughput activity assay,the mutant with highest activity was selected.This chimeric mutant (L3-3),carrying two crossover positions and three point mutations,has a specific activity 6.4and 8.2times higher than the two parent enzymes.The mutant also is more tolerant to various detergents and organic solvents,and has a 9times longer half-life at 50◦C.Homology modeling of mutant L3-3,based on the highly homologous B.subtilis lipase A,shows that the increased thermostability is likely due to structural rigidification and reduced surface hydrophobicity.Increased specific activity may result from the location of mutations close to the active site.Together,our results show that it is possible to evolve,by DNA shuffling,B.pumilus lipase variants with improved applicability as biocatalysts,even if the two parent enzymes are highly similar.© 2013 Elsevier B.V. All rights reserved.1.IntroductionLipases (triacylglycerol acylhydrolases,EC 3.1.1.3)catalyze the hydrolysis and synthesis of esters of glycerol and long-chain fatty acids.Microbial lipases are of commercial interest for chemical,food,pharmaceutical,detergent and other industrial applications (Jaeger and Eggert,2002;Pandey et al.,1999;Sharma et al.,2001).Among them,Bacillus pumilus lipases have been classi-fied as members of subfamily I.4,sharing sequence identities of 74–77%(Arpigny and Jaeger,1999;Jaeger et al.,1999);also the well-characterized Bacillus subtilis lipases belong to this family.The subfamily I.4lipases are the smallest lipases known,having a minimal ␣/hydrolase fold (van Pouderoyen et al.,2001)and a solvent-exposed substrate-binding site.In order to use lipases as biocatalysts in industrial applications,it is often desirable to improve properties such as activity (in aque-ous or organic solvent environments),thermostability,substrate specificity and enantioselectivity (Arnold and Volkov,1999;Reetz,∗Corresponding author.Tel.:+902626052540;fax:+902626052505.E-mail addresses:nakbulut@.tr ,nagihanakbulut@ (N.Akbulut).1During this work,Prof.Dr.Füsun Gümüs ¸el passed away;we remember her with respect.2004).Directed evolution is a powerful approach to achieve such improvements.Rapid generation of molecular diversity is essential,and one of the best methods to achieve this is homologous recombi-nation through DNA shuffling (Crameri et al.,1998).When coupled with high-throughput screening,DNA shuffling and other directed evolution methods have often resulted in remarkable improve-ments of activity,thermostability or enantioselectivity (Crameri et al.,1998;Reetz,2004;Schmidt-Dannert and Arnold,1999).The lipase A from B.subtilis has been the subject of several such stud-ies (Acharya et al.,2004;Ahmad et al.,2008;Augustyniak et al.,2012;Dröge et al.,2006;Kamal et al.,2011).In contrast,a directed evolution approach for B.pumilus lipase has only been reported by Huang et al.(2008)who used error-prone PCR to evolve mutants with increased activity.We applied the DNA shuffling method,coupled with a high-throughput screening assay,to improve the activity of lipases of subfamily I.4produced by local isolates of B.pumilus .The lipase mutant (L3-3)showing the highest activity was sequenced and purified,and biochemically characterized.Obtained after a single round of DNA shuffling from two parents sharing 89%identity,this chimeric mutant has two cross-over positions and carries three point mutations.Its activity was 6.4and 8.2times higher than that of the two parent enzymes.Surprisingly,L3-3also displayed a remarkable increase in thermostability,with a 9times longer half-life (T 1/2)at 50◦C.Taking advantage of the high sequence similarity0168-1656/$–see front matter © 2013 Elsevier B.V. All rights reserved./10.1016/j.jbiotec.2012.12.016124N.Akbulut et al./Journal of Biotechnology164 (2013) 123–129with B.subtilis lipase A,a3D homology model was constructed for L3-3,and the role of sequence differences between the mutant and the parents on enzyme activity and thermostability is discussed.2.Materials and methods2.1.Isolation and identificationBacterial strains L5and L21had been isolated by traditional bacteriological methods from hot springs at Balıkesir and Bursa, Türkiye(Tuzlako˘g lu et al.,2003).Characterization of the strains was done using biochemical tests,microscopical observations (Sneath,1984)and16S rRNA gene sequencing(˙I s¸severÖztürk et al., 2008).Database homology searches were performed with BLAST (/Blast.cgi).2.2.Cloning and expression of parent lipase genesChromosomal DNA was isolated from B.pumilus strains L5and L21(Tuzlako˘g lu et al.,2003;˙I s¸severÖztürk et al.,2008)and used to amplify by PCR the lipase-encoding genes,using a pair of degen-erate primers(forward:21F,reverse:22R).After30amplification cycles,a0.65-kb PCR product was recovered from an agarose gel. Cloning was carried out with InsTAclone TM PCR Cloning Kit(Fer-mentas).The purified PCR products were ligated in pTZ57R/T,and E.coli JM109cells were transformed with this ligation product.The resulting plasmids were named pTZ-L5and pTZ-L21.For expres-sion studies,Hind III–Eco RI fragments from the plasmids pTZ-L5and pTZ-L21were subcloned into the expression vector pUC19previ-ously digested with the same enzymes,separately.E.coli JM109 cells carrying recombinant vectors were grown for24h in the pres-ence of ampicillin(100g/ml)and gene expression was induced with afinal concentration of0.1mM IPTG.2.3.DNA shuffling library constructionA library of random fragments was constructed using modi-fied DNA shuffling methods(Lorimer and Pastan,1995;Stemmer, 1994;Zhao and Arnold,1997).Two0.65kb DNA fragments contain-ing lipase genes from L5and L21were amplified by using primers 21F and22R.Fragments of0.65kb were purified from1%agarose gel.Parent DNA fragments were digested with bovine pancreas DNase I in the presence of Mn2+.A mixture of50l(containing 1.5g of each parent DNA)and5l10×digestion buffer(50mM Tris(tris(hydroxymethyl)aminomethane)–HCl,10mM MnCl2)was equilibrated at25◦C for5min;0.45U of DNase I(diluted in1×digestion buffer)was added.Digestion was performed at25◦C and terminated after11min by heating at90◦C for10min.The digested fragments were separated by1.5%agarose gel electrophoresis;frag-ments of<70bp were isolated and purified from the gel.PCR without primers.The reaction volume(50l)contained 20l purified fragments,0.4mM dNTP mix,2.5U Pfu DNA poly-merase,1×Pfu DNA polymerase reaction buffer.The following PCR protocol was applied:3min at96◦C,40cycles of1min at94◦C, 1min at55◦C,1min+5s/cycle at72◦C,10min at72◦C.PCR with primers.The reaction mixture containing reassembled DNA-fragments(1l)along with primers21F and22R was used to amplify the full-length genes,using the same PCR cycling program as described in PCR without primers.PCR conditions(50lfinal vol-ume):80pmol of each primer,1×Taq polymerase reaction buffer, 0.2mM dNTP mix(Roche)and2.5U Taq/Pfu(1:1)polymerase mix-ture.The purified PCR product was digested with Hind III–Eco RI and ligated into plasmid pUC19,which had been digested with corre-sponding restriction enzymes to create the recombination library. Freshly prepared E.coli JM109cells were transformed with the resulting DNA mixture.Cells were plated on LB-agar containing1.5%agar and1%ampicillin,and incubated overnight.2.4.Enzyme expression and library screeningActive transformants were assessed by a three-step screening protocol.First,transformant colonies were replicated on tributyrin-agar plates containing0.15%Gum Arabic and1.5%tributyrin in LB-agar(Liebeton et al.,2000),supplemented with1%ampicillin.After incubation for16h,enzyme secretion into the medium was induced by incubation for6h at4◦C.Transformants showing lipase activity (resulting in clear halos surrounding the colonies)were selected.In the second step,selected variants were inoculated into the individual wells of96-well plates containing250l LB with1% ampicillin.After overnight growth(37◦C),lipase activity in the cul-ture supernatant was assayed quantitatively using p NP-palmitate as substrate,according to the method of Eom et al.(2005)with slight modifications;absorbance at405nm was measured with a Fluostar Omega Microplate Reader(BMG Labtech).Measured activ-ities were normalized for culture density;variants showing a higher normalized activity than parent strains were selected.Selected variants were further confirmed and analyzed in a third step by growing them in shakeflask cultures at37◦C.Ten milliliters of LB medium containing1%ampicillin were inoculated with0.1ml pre-culture;gene expression was induced with0.1mM IPTG.Nor-malized lipase activity was assayed according to the method of Winkler and Stuckmann(1979).2.5.DNA sequencing,purification and characterization of parents and mutant lipaseThe plasmid DNA of parents L5and L21and of the trans-formant with highest activity was isolated and sequenced (see Supplementary Material,Section1.3).The expressed lipase enzymes were subjected to a single-step purification;their purity was determined from an SDS-PAGE gel.Purification details and characterization of the purified enzymes by determination of temperature and pH profiles and stability,the effect of various detergents,organic solvents,metal ions and inhibitors on activity, and analysis of substrate specificity are described in Supplementary Material(Section1.4).2.6.Modeling studiesAfter analysis of the B.pumilus L3-3mutant sequence by the FFAS03server(Jaroszewski et al.,2011)the structure with the high-est sequence identity(78%),B.subtilis lipase A(PDB ID:1I6W(van Pouderoyen et al.,2001)),was used as a template in the“One-to-one threading”protocol of the Phyre2server(Kelley and Sternberg, 2009)to obtain3D models of mutant L3-3and parents L5and L21.Differences and mutation positions of the models were eval-uated in PyMOL(Schrödinger,LLC,version1.2r1)by looking at interaction possibilities and clash problems.Secondary structure assignment was calculated with DSSP(Kabsch and Sander,1983); hydrogen-bonding was assessed within PyMOL.Structuralfigures were prepared with PyMOL.3.Results3.1.Isolation and identification of lipase-producing strainsCharacterization of the bacterial strains previously isolated from hot springs near Balıkesir and Bursa(Türkiye)showed that they are Gram-positive,rod shaped,aerobic,catalase-positive and sporeN.Akbulut et al./Journal of Biotechnology164 (2013) 123–129125Fig.1.Schematic representation of the sequences of the two Bacillus pumilus parents(L5,light gray,and L21,dark gray)and mutant L3-3.Dark gray and light gray colors in the L3-3mutant indicate from which parent the L3-3mutant derived its sequence.Because of local homology at the DNA level,the crossover positions in L3-3cannot be determined exactly;thefirst crossover position is between residues20/21and23/24,and the second crossover position is between residues149/150and168/169,as indicated by the shaded parts.Chimeric differences between the two parents are indicated with black triangles;the3point mutations in the L3-3mutant are indicated with black bars.A more detailed alignment is given in Supplementary Material Fig.S2.forming.Biochemical tests and16S rDNA gene analysis identified the strains as B.pumilus,and they were designated as L5and L21.3.2.Cloning,sequencing and expression of the parent lipasesThe0.65kbp lipase open reading frames(ORFs)of the two B.pumilus strains L5and L21were amplified from the chro-mosomal DNA(Supplementary Material Fig.S1).Cloning into pTZ57R/T and subsequent DNA isolation and sequencing con-firmed the presence of ORFs of645bp,encoding precursor lipases of215amino acid residues.DNA translation showed that the encoded enzymes contain a34-residue signal peptide(SignalP 4.0,http://www.cbs.dtu.dk/services/SignalP);after cleavage,the mature enzymes thus contain181amino acid residues.Sequence analysis revealed that the L5and L21parent lipases share89%iden-tity with each other(at the protein level),and78%identity with B. subtilis lipase A(Supplementary Material Fig.S2).The parent lipase gene sequences have been deposited in GenBank with accession numbers JX163855(L5)and JX163856(L21).The L5and L21lipase genes were successfully subcloned into a pUC19vector,as confirmed by digestion of the recombinant plasmid and identification of the645bp DNA fragments.Trans-formation of E.coli JM109cells with the recombinant plasmids resulted in active expression of the lipases,as was confirmed by lipase activity assays.3.3.DNA shuffling and screening of the libraryA random B.pumilus lipase library was generated by DNA shuf-fling,using<70bp fragments obtained from the two B.pumilus lipase parent genes L5and L21.Reassembled products ran as sin-gle bands with the correct size on agarose gels(Supplementary Material Fig.S1).These were used to transform E.coli JM109cells; 5500transformants(55%)expressed a functional lipase,forming clear halos due to the hydrolysis of tributyrin.The350transfor-mants with highest activity(as judged by eye)were selected for the second screening step.From these,the16transformants showing a higher activity than the parent strains were selected for a third screening step,in which more favorable conditions for bacterial growth were applied.The transformant showing the highest nor-malized activity was further characterized by comparison with the two parent lipases.Sequencing revealed that the lipase expressed by this transformant(L3-3)is a chimeric mutant with2crossover positions,resulting in a large middle fragment originating from the L5parent,and shorter N-and C-terminal fragments derived from the L21parent(Fig.1and Supplementary Material Fig.S2).There are11“chimeric differences”(residues that differ between the two parent enzymes)in the middle fragment and3such differences in the terminal fragments.In addition,L3-3carries3point muta-tions(G14S,A15G and V109S);they do not stem from either parent, nor are they present in B.subtilis lipase A.Consequently,L3-3is Table1Specific activity and half-life of the partially purified parent(L5,L21)and mutant (L3-3)enzymes.The specific activity is given before(raw)and after correction for purity(40,25and60%for L5,L21and L3-3,respectively).L5L21L3-3Raw specific activity(U/mg)1150±3558±411,012±4 Corrected specific activity(U/mg)2878±82238±1618,332±7T1/2,50◦C(min) 4.20±0.12 4.40±0.0338.5±0.7 different from parent L5at6positions,and different from parent L21at14positions.3.4.Purification and characterization of parent and mutant B. pumilus lipasesResults of the purification of the two parent B.pumilus lipases and mutant L3-3are summarized in Supplementary Material Table S1.Typically,thefinal yield of enzyme was about50%of the ini-tial activity,with a9-fold increase in specific activity compared to the culture lysate supernatant.On SDS-PAGE,the purified enzymes were observed at about19kDa(Supplementary Material Fig.S3), with purities of about40,25and60%for L5,L21and L3-3,respec-tively.We did not succeed in purifying the enzymes further.For both parent and mutant lipases,the optimum temperature was37◦C(Supplementary Material Fig.S4),but,after correction for the differences in purity,the specific activity of mutant L3-3 was about6.4and8.2times higher than that of the parents L5 and L21,respectively(Table1).At higher temperatures,activity decreased fast to near-zero values at55◦C,but the L3-3mutant clearly retained more activity than the parent enzymes(Fig.2).TheFig.2.Relative residual activity of parents(L5,L21)and L3-3after pre-incubation at different temperatures for30min.126N.Akbulut et al./Journal of Biotechnology 164 (2013) 123–129Fig.3.3D homology model of the B.pumilus L3-3mutant,generated with Phyre2(Kelley and Sternberg,2009)based on the crystal structure of B.subtilis lipase A (van Pouderoyen et al.,2001).The N-and C-terminal polypeptide segments derived from parent L21,containing the 3chimeric differences (M12,A20and V169)are shown in blue;the middle segment derived from parent L5is shown in gray.The three point mutations G14S,A15G and V109S are shown with yellow carbon atoms.The catalytic residue S77in the active site is also shown.(For interpretation of the references to color in figure legend,the reader is referred to the web version of the article.)half-life (at 50◦C)of mutant L3-3was 9times longer than that of the parent enzymes (Table 1).The pH-activity profiles of both parents and mutant L3-3were very similar (Supplementary Material Fig.S5a ),with an optimum pH of 8.0.The residual activity profiles after 1week of incubation at 4◦C were also similar,with 80–100%activity retained between pH 6.5and 10.0(Supplementary Material Fig.S5b ).Metal ions (10mM)in general had modest effects (Supplementary Material Fig.S6,left panel );relative activi-ties were in the range of 50–163%.The most prominent effect was observed for CuCl 2,which showed an increased activity for L3-3while the parent lipases were inhibited.In addition,CoCl 2and FeCl 2increased activity of L3-3significantly.The presence of CaCl 2slightly inhibited the mutant,while the presence of EDTA (ethylene diamine tetraacetic acid)(1or 10mM)hardly affected activity;PMSF (phenylmethylsulfonyl fluoride)strongly inhibited activity of both parent enzymes and the mutant (Supplementary Material Fig.S6,right panel ).All tested detergents,except for Na-deoxycholate,inhibited the activity of parent and mutant lipases at the highest tested concen-tration;CTAB (cetyl trimethylammonium bromide)(1%)and SDS (sodium dodecyl sulfate)(1%)almost completely inactivated the enzymes (Supplementary Material Fig.S7a ).However,in several cases mutant L3-3retained a significantly higher activity than the parents,or was even stimulated.Most of the tested organic solvents had a slightly inhibiting effect on the activity of parent enzymes at 10%concentration;this effect was stronger at higher concentration (30%)(Supplementary Material Fig.S7b ).Notably,the L3-3mutant showed a tolerance to all tested organic solvents at 10%concentration except isoamyl alcohol.Analysis of the substrate specificity of parent and mutant lipases revealed only small variations (Supplementary Material Fig.S8);mutant L3-3showed a slightly higher activity toward long chain triacylglycerol fatty acids than the parent enzymes.3.5.Structural observationsThe 3D models generated for the B.pumilus lipase L3-3mutant (Fig.3)and its L5and L21parents showed high Phyre confidence values.Of the 40sequence differences between the B.subtilis lipase A and the B.pumilus L3-3mutant (Supplementary Material Fig.S2),almost half are homologous substitutions.For 35of these,the sidechains are at the surface and exposed to the solvent;the remaining differences are located in the hydrophobic core,and comprise at most one methylene or methyl group.About half (19)of the differ-ences occur in non-regular secondary structure elements such as loops and 310helices.For the G14S mutation,a different side chain rotamer was chosen to avoid a close contact with the side chain of N18.For all other changed residues,no severe clash problems were observed.The three chimeric differences of L3-3with parent L5and the three point mutations in L3-3are described below.The chimeric differences (M12,A20and V169).Residue 12is located at the tip of a 6-residue loop (residues 10–15)connect-ing strand 3and helix 1/␣A (Fig.4a).Its side chain is exposed to the solvent,and the chimeric change from isoleucine to methionine may increase hydrophobic and van der Waals interactions with thesubstrate.Residue 20,at the start of helix ␣A,is located about 16˚Afrom the active site,and has a solvent-exposed side chain.Changing a phenylalanine to alanine at this position will considerably reduce the hydrophobicity at the surface,and the tendency to aggregate at higher temperatures.Residue 169is in helix ␣F;its side chain is located in the hydrophobic interior of the enzyme (Fig.3),far from the active site.The change from isoleucine to valine at this posi-tion (one methyl group)may slightly change local packing in the enzyme’s interior.The point mutations (G14S ,A15G and V109S ).Residue 14is located in the 3-1/␣A loop (residues 10–15),adjacent to the sub-strate binding cleft (Fig.4a).The introduction of the serine side chain has no effect on the main chain torsion angles (ϕ=−86◦, =−171◦),but it increases the local surface polarity.In addition,it provides the possibility of the formation of two additional hydro-gen bonds within the loop.The -turn hydrogen bond interaction between the main chain oxygen atom of G11and the main chain nitrogen atom of S14is preserved.Mutation of residue 15intro-duces a third glycine residue in the 3-1/␣A loop (Fig.4a).In the parent B.pumilus enzymes (like in B.subtilis LipA),the alanine side chain at position 15points into the solvent,forming a hydropho-bic surface patch together with the side chain of Y17,at the rim of the substrate binding cleft.The absence of the methyl group in mutant L3-3mutant reduces local surface hydrophobicity.Residue 109is positioned at the surface,just after 310helix 4(Fig.4b).In both parent B.pumilus lipases L5and L21residue 109is a valine,and its mutation to serine reduces the local surface hydrophobic-ity.In mutant L3-3,this methyl group is absent,and local surface hydrophobicity is reduced.Moreover,the serine hydroxyl group isN.Akbulut et al./Journal of Biotechnology164 (2013) 123–129127Fig.4.Stereofigures of the L3-3mutant homology model.Point mutations are shown with yellow carbon atoms.Hydrogen bond interactions are shown as blue dashed lines.(a)Point mutations G14S and A15G located in the3-1/␣A loop(residues10–15)that connects strand1and helix␣A.The S14O␥atom has hydrogen bonding interactions with the N␦2atom of N18from helix␣A and the main chain nitrogen atom of G11.The-turn hydrogen bond interaction between G11O and G14N is also shown.The3-1/␣A loop is on one side of the substrate binding cleft,where the leaving group of a substrate would be bound.Some residues lining this part of the active site(I157,L160)are also shown in stick representation as well as the nucleophilic serine(S77).The main chain nitrogen atom of residue M12that forms part of the oxyanion hole is indicated with an asterisk(*).(b)The V109S point mutation;its side chain makes direct hydrogen bonds to the N␦2atom of N48,the N-terminal residue of helix ␣B,and to the main chain oxygen of A81(in helix␣C).The long␣B helix can make only one other direct hydrogen bond,between S56and D91.(For interpretation of the references to color infigure legend,the reader is referred to the web version of the article.)able to form two hydrogen bonds,similar to the equivalent thre-onine in B.subtilis lipase A.It can make one hydrogen bond to the main chain oxygen atom of residue A81(in helix␣C),and a second hydrogen bond to the N␦2atom of N48,the N-terminal residue of helix␣B.4.Discussion4.1.Activity and thermostability of mutant L3-3Mutant L3-3was selected from the shuffling library by screening for lipase activity.Our approach did not account for differences in lipase expression levels in the library and therefore may be biased.Nevertheless,our selection strategy resulted in a mutant with significantly improved specific activity.This mutant(L3-3) shows a6.4-and8.2-fold increase in specific activity,respectively when compared to its parent enzymes L5and L21.The usefulness of(mutant)lipases in industrial applications also depends on the effects of metal ions,detergents and organic solvents.For exam-ple,enzyme activity and stability in the presence of detergents is a requirement for laundry applications(Gaur et al.,2008).Further-more,tolerance to organic solvents facilitates the use of enzymes as biocatalysts in non-aqueous media,e.g.when it is necessary to dissolve or recover substrates or products in an organic phase, to decrease unwanted substrate or product inhibition(Hun et al., 2003),or when the product itself is an organic compound(e.g. methanol in the production of biodiesel)(Li et al.,2012).Our results indicate that for many of the compounds tested,mutant L3-3retains a comparable or higher relative activity than the parent enzymes,and therefore has improved characteristics as a possible biocatalyst.With respect to substrate specificity,L3-3remains a true lipase,with the highest activity observed for long chain tria-cylglycerol fatty acids,like the parent enzymes L5and L21.The significant stimulating effect of the presence of(10mM) FeCl2,CoCl2or CuCl2on L3-3is remarkable,since many lipases are inhibited by these metal salts(Gaur et al.,2008;Nthangeni et al.,2001;Sharma et al.,2001).The minimal effect of the metal-chelating agent EDTA on the activity of the parent enzymes and L3-3suggests that no metal binding sites exist,in agreement with previous studies.The strong inhibition by PMSF confirms that the enzymes under study are of the serine hydrolase class.Secondly, the tolerance of L3-3to detergents is comparable to or slightly bet-ter than that of the parent enzymes.Notably,the higher retained activity of L3-3in the presence of0.1%SDS compared to the parent enzymes,indicates that it is more resistant to unfolding.Thirdly,128N.Akbulut et al./Journal of Biotechnology164 (2013) 123–129the higher retained activity of L3-3for most organic solvents when compared to the parents indicates an increased tolerance to such compounds.Surprisingly,although we screened for activity as the desired property to be increased,L3-3also displays a remarkable increase in thermostability.Its half-life(T1/2)at50◦C of38.5min is a9.2-and8.8-fold improvement with respect to the parent enzymes. This is also reflected by a higher resistance to thermal inactivation: 70%of the initial activity of L3-3is retained after a30min incuba-tion at50◦C,a2.5-and3.7-fold increase compared to the parent enzymes(Fig.2).The fact that we obtained a mutant with both increased thermostability and activity indicates that it is possible to improve these properties at the same time.A similar case has been reported for Candida antarctica lipase B(Suen et al.,2004);there-fore a‘dual’screening approach involving both thermostability and activity may be generally beneficial for the directed evolution of lipase enzymes.In addition,further improvement of thermosta-bility and activity may be obtained by increasing the number of DNA-shuffling cycles.4.2.Structural implicationsIt has been proposed that several sequence/structural features contribute to the greater stability of thermophilic proteins(Kumar et al.,2000).These features include packing(of the core struc-ture),polar surface area,helical content/propensity,salt bridge and other hydrogen bond interactions,proline substitutions,insertions or deletions,loop stabilization and protein oligomerization.In the case of the B.pumilus L3-3mutant,the basis of enhanced thermosta-bility and activity(with respect to the parent enzymes)must lie, in one way or another,in the chimeric differences and point muta-tions.To study their structural effects,we constructed3D homology models for the B.pumilus L5and L21parent lipases and mutant L3-3(Figs.3and4),based on the crystal structure of B.subtilis lipase A(van Pouderoyen et al.,2001).Given the high sequence identity (78%)between the B.pumilus lipases and the B.subtili lipase A,and the nature and distribution of the about40differences between them,the homology models can be regarded as fairly reliable with an estimated root mean square deviation for backbone atoms of 0.6˚A(Chothia and Lesk,1986).Even in regions where differences are concentrated(mostly in loops and310helices),the main chain need hardly be affected because most of the differences are at the surface.In only one case(residue S14in L3-3)the model was man-ually adjusted to a more favorable side chain rotamer.Three of the six differences between mutant L3-3and parent L5occur in the3-1/␣A loop(residues10–15,Fig.4a),which forms one‘wall’of a narrow hydrophobic substrate binding cleft (Dröge et al.,2006).Both the chimeric I12M difference and A15G point mutation result in a reduced surface hydrophobicity,which may contribute to an increase in thermostability of L3-3.A similar proposal has been made for the A15S mutation in B.subtilis lipase A mutants3-3A9,4D3and6B(Ahmad et al.,2008;Kamal et al., 2011).The third difference,G14S,is thefirst reported mutation at this position for a family I.4lipase.The introduction of the serine side chain may increase thermostability by facilitating an addi-tional intra-loop hydrogen bond interaction with residue G11.This interaction would stabilize the conformation of the3-1/␣A loop, counteracting theflexibility-increasing effect of the introduction of a third glycine in this loop(mutation A15G).Together,the ther-mostability enhancing effects of mutations in the3-1/␣A loop may be attributed to a combination of reduced surface hydropho-bicity and stabilization of loop conformation.The observed enhanced activity of L3-3on p NP-palmitate as a substrate most likely stems from the G14S and I12M mutations in the substrate binding cleft.In the complexes of B.subtilis lipase A with different phosphonate inhibitors(Dröge et al.,2006)(PDB IDs:1R4Z,1R50),the IPG moiety of the inhibitor binds in a nar-row hydrophobic groove between residues I12-G13-G14(in the 3-1/␣A loop)and H156-I157.The importance of residues in the 3-1/␣A loop of B.subtilis lipase A has been shown previously in a loop-grafting study(Boersma et al.,2008),where enantioselectiv-ity toward IPG esters could be inversed by replacing this loop with loops originating from other␣/-hydrolases.A superposition(not shown)of L3-3with B.subtilis lipase A reveals that in L3-3the p NP moiety of the substrate would occupy the same space as the IPG moiety in B.subtilis lipase A.The side chain of a serine residue at position14would point into the binding groove and could interact with the substrate.The increased polarity of the environment of the scissile bond may favorably affect the hydrolysis of the cova-lent tetrahedral reaction intermediate.This reaction intermediate is stabilized by two peptide NH groups(the oxyanion hole)formed by residues12and78(Jaeger et al.,1999).Thus,the I12M and G14S mutations may affect both substrate affinity and reaction kinetics, apparently leading to a more active enzyme.On the other hand, substrate specificity is hardly affected(Supplementary Material Fig. S8).In contrast to the above mentioned mutations,point mutation V109S and the chimeric differences F20A and I169V,located at distances between12and16˚A from the active site serine,likely will not affect the activity of L3-3,at least not through short range effects.Instead,the F20A and V109S mutations appear to increase thermostability by reducing surface hydrophobicity,or by stabi-lizing interactions that are absent in the parent enzymes.Like in B.subtilis lipase A,two hydrogen bonds can link S109(T109in lipase A)to N48on helix␣B and to A81on helix␣C,thereby anchoring this part of the long109–123loop to the core secondary structure elements.The S109-N48hydrogen bond interaction also fixes the N-terminal end of the long␣B helix(Fig.4b),which has only one other hydrogen bond interaction(S56-D91).Likely, the stabilizing interactions due to the V109S mutation result in a more rigid enzyme structure,in agreement with the observa-tion that the L3-3mutant is more resistant to unfolding by SDS. Together with the removal of a solvent-exposed non-polar phenyl or methyl group of residues20and109,respectively,this results in enhanced thermostability.Finally,the I169V difference may affect thermostability by slightly changing the interior hydrophobic pack-ing of the enzyme.5.ConclusionsTo the best of our knowledge,our study describes thefirst application of DNA-shuffling to lipases from B.pumilus.From a single round of DNA-shuffling with two B.pumilus parent lipases, we have obtained a chimeric mutant(L3-3)with an up to8-fold increased specific activity and a9-fold increased half-life(at50◦C). The increased tolerance of L3-3to various detergents and organic solvents further enhances its application possibilities as a biocat-alyst.Based on a reliable homology model,we conclude that the observed enhancement of thermostability of L3-3is likely the con-sequence of(a)rigidification of enzyme structure by strengthening (hydrogen bonding)interactions between structural elements,and (b)the removal of hydrophobic patches on the enzyme surface (A15G,F20A,V109S).The same factors have been proposed to account for increased thermostability of evolved B.subtilis lipase A mutants(Ahmad et al.,2008;Kamal et al.,2011).The effect on enzyme activity is likely due to the fact that three of the six differences between mutant L3-3and parent L5(I12M,G14S,A15G) are in a loop adjacent to the substrate-binding site.These mutations may affect substrate binding and increase the reaction rate for the hydrolysis of the covalent reaction intermediate,but do not alter the substrate specificity.Although synergistic effects of mutations。
fulltext5
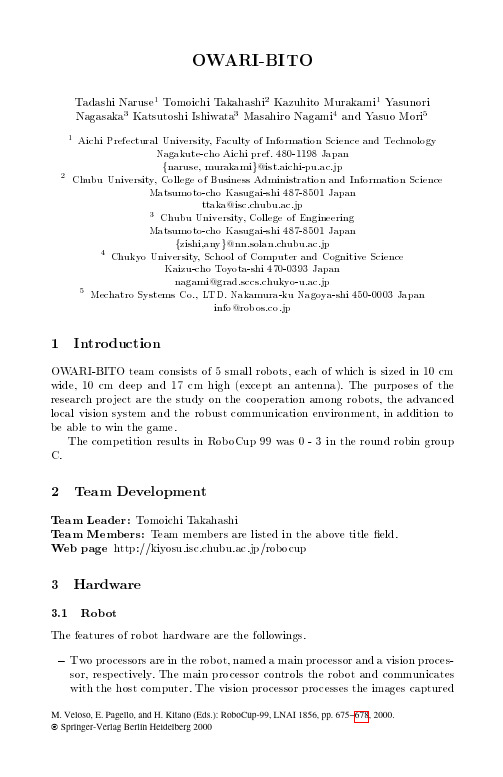
3 Hardware
3.1 Robot
The features of robot hardware are the followings.
{
Two processors are in the robot, named a main processor and a vision processor, respectively. The main processor controls the robot and communicates with the host computer. The vision processor processes the images captured
2 Team Development
Team Leader: Web page Team Members:
Tomoichi Takahashi Team members are listed in the above title eld. http://kiyosu.isc.chubu.ac.jp/robocup
3
ttaka@isc.chubu.ac.jp Chubu University, College of Engineering
Matsumoto-cho Kasugai-shi 487-8501 Japan
4
fzishi,anyg@nn.solan.chubu.ac.jp
Chukyo University, School of Computer and Cognitive Science Kaizu-cho Toyota-shi 470-0393 Japan
The position data are sent to the strategy process.
python pychorus副歌检测原理 -回复
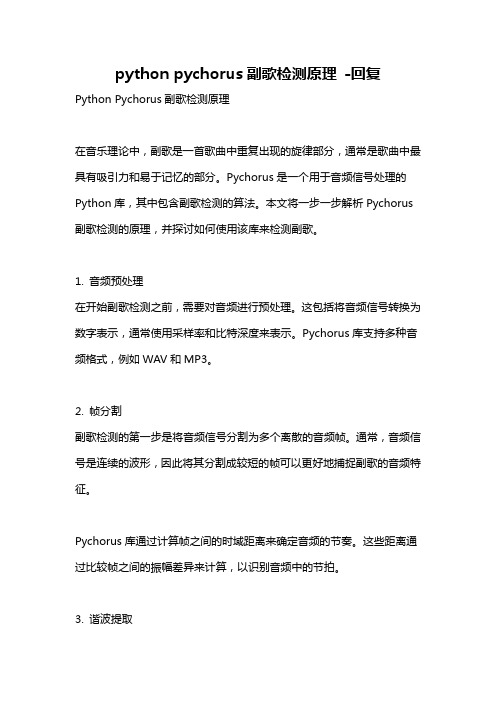
python pychorus副歌检测原理-回复Python Pychorus副歌检测原理在音乐理论中,副歌是一首歌曲中重复出现的旋律部分,通常是歌曲中最具有吸引力和易于记忆的部分。
Pychorus是一个用于音频信号处理的Python库,其中包含副歌检测的算法。
本文将一步一步解析Pychorus 副歌检测的原理,并探讨如何使用该库来检测副歌。
1. 音频预处理在开始副歌检测之前,需要对音频进行预处理。
这包括将音频信号转换为数字表示,通常使用采样率和比特深度来表示。
Pychorus库支持多种音频格式,例如WAV和MP3。
2. 帧分割副歌检测的第一步是将音频信号分割为多个离散的音频帧。
通常,音频信号是连续的波形,因此将其分割成较短的帧可以更好地捕捉副歌的音频特征。
Pychorus库通过计算帧之间的时域距离来确定音频的节奏。
这些距离通过比较帧之间的振幅差异来计算,以识别音频中的节拍。
3. 谐波提取一旦音频被分割为帧,Pychorus库通过计算傅里叶变换来提取每个帧的频域特征。
为了抽取更有代表性的特征,通常只关注低频和中频范围。
通过提取帧的频域特征,可以计算每个帧的谐波能量。
这些谐波能量值表示了音频信号中不同频率的能量分布情况。
副歌通常包含较高的谐波能量值,并且这些能量值在副歌的每个重复部分中是相似的。
4. 相似性度量一旦提取了每个帧的谐波能量,就可以通过计算帧之间的相似性度量来检测副歌。
Pychorus库使用动态规划算法来比较帧之间的相似性。
动态规划算法使用一个矩阵来存储每个帧之间的相似性度量。
该矩阵的每个元素表示两个帧之间的相似性程度。
通过计算前一帧和当前帧之间的相似性度量,并将其与最大相似性度量值相加,可以得到当前帧的最大相似性度量值。
重复此过程,直到计算到最后一个帧为止。
5. 副歌检测副歌检测是通过检查相似性度量值的变化来进行的。
通常,副歌部分会在连续的帧中产生较高的相似性度量值。
Pychorus库使用一个阈值来判断一个帧是否属于副歌。
Host Key White Paper
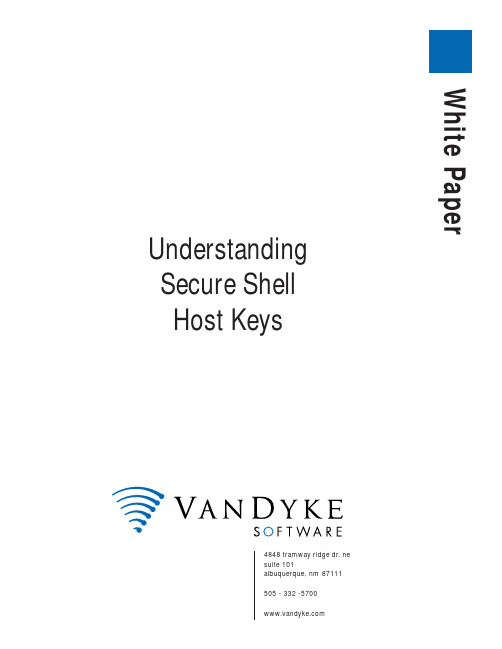
White PaperUnderstandingSecure ShellHost Keys4848 tramway ridge dr. nesuite 101albuquerque, nm 87111505 - 332 -5700Understanding Host KeysThink about the last time you faxed personal or company information to someone for the first time. Did you wonder if the number you were sending this information to was the right one? Unlike a phone call, where no personal information is exchanged until you have identified who you are speaking to, when you send a fax you might wonder where your information is ending up. When using the public network (internet), verifying that the server being connected to is the “right number” is taken for granted far too often. In this white paper, we will talk about the importance of knowing that the server you (or one of your end users) is connecting to is the “right number” and how Secure Shell server host keys are used to verify a server’s identity.This paper assumes the reader has a general familiarity with the Secure Shell protocol. For more information, refer to our Secure Shell Overview white paper which can be read online or downloaded from our web site:/solutions/ssh_overview/index.html.Here is a brief excerpt from that white paper’s introduction:Secure Shell (SSH) provides an open protocol for securing network communicationswhich is less complex and expensive than hardware-based VPN solutions. Secure Shell client/server solutions provide command shell, file transfer, and data tunneling servicesfor TCP/IP applications. SSH connections provide highly secure authentication,encryption, and data integrity to combat password theft and other security threats.IntroductionUsers and administrators turn to Secure Shell for many reasons. Some need to replace Telnet or FTP. Others are looking to move away from simple passwords to public-key, Kerberos, or keyboard-interactive authentication. Still others are looking for a low cost alternative to VPNs. Whatever the reason for using Secure Shell, every user and administrator of Secure Shell needs to understand host keys. Understanding what host keys are, how they work, and the security they provide can reduce confusion and possible frustration about administering and using this authentication tool for both administrators and end users.Failure to understand host keys can cause many problems. Some users may be frightened or confused by messages when a new or changed host key is encountered. This can result in additional and unnecessary support costs. Even worse, improper handling of host keys can lead to a compromised system.What vulnerabilities do host keys help address?Man-in-the middle attacks: When you connect to a remote host, if you cannot reliably verify that the host key is from the host you intend to connect to, you risk the possibility that an adversary has placed a server pretending to be the Secure Shell server between you and the final destination. The server you’re actually connecting to is a “man-in-the-middle”. This man-in-the-middle is able to see both the username and password information you transmit as you attempt to authenticate. Once intercepted, a man-in-the-middle can use this information to establish a connection with the remote server and see all traffic between you and the remote server.Depending on your network topology, the risk of man-in-the-middle attack can vary widely. If users and administrators take some very basic steps, the risk of a man-in-the-middle attack can be significantly reduced.How web servers prevent man-in-the-middle attacks: This uncertainty about the authenticity of a remote host is not a unique problem. Most of us have connected to a secure web server at one time or another. We look down at the bottom corner of the browser and we see a little padlock. It gives us a warm fuzzy feeling that we know we have connected to a secure web server. So, how do secure web servers solve this problem? A secure web server uses a certificate issued by a trusted third-party Certificate Authority (CA) such as VeriSign® and the client is responsible for checking that certificate upon connecting. In Internet Explorer and other browsers, you can review a list of certificate authorities that are deemed to be trusted. When you initially connect to the web server, the browser checks the certificate it receives from the remote server to see that it has been signed by one of the known trusted authorities. It also checks to see if the certificate has been revoked or expired. If a certificate has been revoked or expired, a dialogue will pop up indicating there is a problem with the certificate. You can also get a pop-up dialog if the certificate doesn’t match the host you are connecting to.In general, Secure Shell servers don't have the same type of key infrastructure that web servers depend on. Most Secure Shell servers rely on host keys that are created automatically by the server after installation. And these host keys can't easily be verified the first time a client connects.What is the purpose of the host key?A host key is the server’s public key. The host key is used by the client to decrypt an authentication message sent from the server when connecting. The basic purpose of the host key is to ensure that when you connect to a remote host, it is actually the host that you intended to connect to. Unfortunately, this presents something of a catch-22, as described in the following illustrations. If you've never connected to the host before, how do you know the host you are connecting to is the right one?In Secure Shell, host keys can be used for host-based authentication, but this paper will not be addressing host-based authentication since it is not widely used.Creating host keysCreating a host key for a Secure Shell server is usually done only once. The server software creates the host key automatically during installation and configuration. Less commonly, an administrator can elect to manually generate a host key and select the encryption algorithm and key length. For those of you familiar with Secure Shell's public-key authentication, the choice of algorithms (DSA or RSA) and the key length (usually between 1024 and 2048 bits long) is identical to the options for those user authentication keys.A host key consists of two components, a private and a public component. The public component is sent to the client when the client connects. The private component should be protected so that only the administrator and the Secure Shell server have access to it. This cannot be overemphasized. If an adversary acquires a copy of the private host key, it can be used to impersonate your server with complete impunity.Accepting a new host keyThe illustrations below describe how the client application and the host perform a host key exchange when connecting for the first time.Once the connection is established, the key exchange takes place before any personal data is sent by the client application.If the host key does not match an existing key in the client application’s host key database, a challenge message is generated by the client application. An example text message and dialog box are shown below.The host key database does not contain an entry for the hostnamemyserver, which resolved to 192.168.0.29, port 22.It is recommended you verify your host key before accepting.Server's host key fingerprint (MD5 hash):14:09:26:bc:13:24:31:5c:f7:6c:39:94:f7:4d:52:14If you trust this host, enter “y” to add the key to the host key database and connect. If you do not trust this host, enter “n” to abandon theconnection.Accept and save? (y/n)If you’re using a Windows client, you may see a dialog such as:Many users, not understanding exactly what the text or dialog means, simply accept the new host key. Accepting the key is problematic if the user does not know for certain that the host key it has just accepted is actually from the server that the client believes it is connecting to.Before accepting the new host keys, the user should use a secure method to verify that the host key corresponds to the actual server. When a Secure Shell server host key is created, a unique fingerprint is also generated. This fingerprint is a human-readable cryptographic hash that can be used to verify the authenticity of the key being presented by the server to the client. Methods for verifying host keys, including using the host key’s fingerprint, are discussed below.Handling a changed host keyAfter a host key has been accepted and saved, the user should not see this message again. However, if the host key presented by the server on a subsequent connection is different from the one saved on the user’s local system, a second message will be displayed. Here is an example: The host key sent by the server is different from the host key stored in the host key database for myserver (192.168.0.1), port 22. This may mean that a hostile party has "hijacked" your connection and you are notconnected to the server you specified.It is recommended you verify your host key before accepting.Server's host key fingerprint (MD5 hash):14:09:26:bc:13:24:31:5c:f7:6c:39:94:f7:4d:52:14If you trust this host, enter “y” to add the key to the host key database and connect. If you do not trust this host, enter “n” to abandon theconnection.Accept and save? (y/n)As you can see from the text of the message, a user seeing words such as “hijacked” might get very nervous. There are several scenarios that could cause this situation. The first is that theserver has been compromised or you are experiencing a man-in-the-middle attack. However, this is not usually the case. Here are two more likely scenarios.1.It’s possible that the administrator changed the host key.2.The machine the user is connecting to actually has more than one Secure Shell serverrunning and the client is not keeping track of the different host keys for the differentservers running on the same machine.As with a new host key, before accepting the changed host key, the user should use a secure method to verify the host key being presented corresponds to the actual server. Here are a few methods to address this question of host authenticity.Known hostsAfter the client connects to the server for the first time and accepts and saves the host key, it is stored in a local database. One solution for eliminating the need to manually verify the host key is for the administrator to pre-populate the database of known hosts on each of the client machines. Where host keys are stored and the exact format they are stored in is usually client specific. For example, in SecureCRT or SecureFX, host keys are stored in the user's application data area and can be viewed, imported, or deleted using the Global Options dialog. For VanDyke's Linux and UNIX servers, they are stored in the user's home directory under ~/.vshell/known_hosts.In addition, most clients also look in a common location. For example, under Linux or UNIX, a common set of known host keys can be found in /usr/local/etc/known_hosts .Verifying host keysCalling the system administrator and verifying the host key over the phone is a simple solution to making sure the host key is correct and that the client is not vulnerable to a man-in-the-middle attack. However, in many situations this is not a practical solution. There may be too many servers. There may be too many clients. Or, the administrator may not be available when the user first connects.There are a number of other methods that can be used to distribute host keys or fingerprints: •An ISP or network administrator might distribute host key fingerprints on a secure web page that all customers or users have access to.•The host key fingerprint can be sent by e-mail to end users so they have it readily available to compare to the fingerprint displayed in the challenge message.•For enterprises that already use a system such as SMS to push files out to client systems, host keys could also be distributed through this system.•Organizations using Kerberos could take advantage of Secure Shell's GSSAPI key exchange which doesn't require hosts keys and instead leverages Kerberos hostverification.Recently, an IETF draft has been released that specifies a method of checking host key fingerprints using secure DNS (DNSSEC). Secure Shell solutions implementing this new mechanism are not yet widely available.The need for policyWith any security solution, there is a need for policy. As part of a company's security awareness training, users should be educated about the value of host keys and made aware of a company's procedure for checking host keys. Clearly communicating this policy can alleviate a lot of the fear, uncertainty, and doubt that accompany users’ decision-making when a new host key is presented.Administrators should understand that changing host keys could have a ripple effect. In the case where a host key must be changed, the change should, if at all possible, be communicated to users of the service in advance of making it. By doing so, much of the help desk grief can be eliminated.SummaryTurning off Telnet and FTP and moving to Secure Shell makes sense and protects both passwords and data from being sent in the clear. In addition to encryption, Secure Shell provides a way to authenticate both the end user and host. This document has illustrated how host keys play an integral role in establishing this trusted connection. In order to maintain the security and integrity of the system, users and administrators need to understand host keys and the correct method for verifying them.。
A Versatile Zero Background T-Vector System for Gene
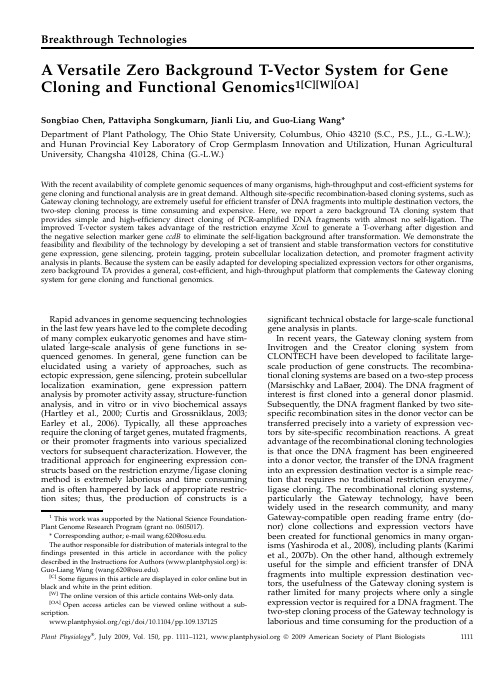
Breakthrough TechnologiesA Versatile Zero Background T-Vector System for Gene Cloning and Functional Genomics1[C][W][OA]Songbiao Chen,Pattavipha Songkumarn,Jianli Liu,and Guo-Liang Wang*Department of Plant Pathology,The Ohio State University,Columbus,Ohio43210(S.C.,P.S.,J.L.,G.-L.W.); and Hunan Provincial Key Laboratory of Crop Germplasm Innovation and Utilization,Hunan Agricultural University,Changsha410128,China(G.-L.W.)With the recent availability of complete genomic sequences of many organisms,high-throughput and cost-efficient systems for gene cloning and functional analysis are in great demand.Although site-specific recombination-based cloning systems,such as Gateway cloning technology,are extremely useful for efficient transfer of DNA fragments into multiple destination vectors,the two-step cloning process is time consuming and expensive.Here,we report a zero background TA cloning system that provides simple and high-efficiency direct cloning of PCR-amplified DNA fragments with almost no self-ligation.The improved T-vector system takes advantage of the restriction enzyme Xcm I to generate a T-overhang after digestion and the negative selection marker gene ccdB to eliminate the self-ligation background after transformation.We demonstrate the feasibility andflexibility of the technology by developing a set of transient and stable transformation vectors for constitutive gene expression,gene silencing,protein tagging,protein subcellular localization detection,and promoter fragment activity analysis in plants.Because the system can be easily adapted for developing specialized expression vectors for other organisms, zero background TA provides a general,cost-efficient,and high-throughput platform that complements the Gateway cloning system for gene cloning and functional genomics.Rapid advances in genome sequencing technologies in the last few years have led to the complete decoding of many complex eukaryotic genomes and have stim-ulated large-scale analysis of gene functions in se-quenced genomes.In general,gene function can be elucidated using a variety of approaches,such as ectopic expression,gene silencing,protein subcellular localization examination,gene expression pattern analysis by promoter activity assay,structure-function analysis,and in vitro or in vivo biochemical assays (Hartley et al.,2000;Curtis and Grossniklaus,2003; Earley et al.,2006).Typically,all these approaches require the cloning of target genes,mutated fragments, or their promoter fragments into various specialized vectors for subsequent characterization.However,the traditional approach for engineering expression con-structs based on the restriction enzyme/ligase cloning method is extremely laborious and time consuming and is often hampered by lack of appropriate restric-tion sites;thus,the production of constructs is a significant technical obstacle for large-scale functional gene analysis in plants.In recent years,the Gateway cloning system from Invitrogen and the Creator cloning system from CLONTECH have been developed to facilitate large-scale production of gene constructs.The recombina-tional cloning systems are based on a two-step process (Marsischky and LaBaer,2004).The DNA fragment of interest isfirst cloned into a general donor plasmid. Subsequently,the DNA fragmentflanked by two site-specific recombination sites in the donor vector can be transferred precisely into a variety of expression vec-tors by site-specific recombination reactions.A great advantage of the recombinational cloning technologies is that once the DNA fragment has been engineered into a donor vector,the transfer of the DNA fragment into an expression destination vector is a simple reac-tion that requires no traditional restriction enzyme/ ligase cloning.The recombinational cloning systems, particularly the Gateway technology,have been widely used in the research community,and many Gateway-compatible open reading frame entry(do-nor)clone collections and expression vectors have been created for functional genomics in many organ-isms(Yashiroda et al.,2008),including plants(Karimi et al.,2007b).On the other hand,although extremely useful for the simple and efficient transfer of DNA fragments into multiple expression destination vec-tors,the usefulness of the Gateway cloning system is rather limited for many projects where only a single expression vector is required for a DNA fragment.The two-step cloning process of the Gateway technology is laborious and time consuming for the production of a1This work was supported by the National Science Foundation-Plant Genome Research Program(grant no.0605017).*Corresponding author;e-mail wang.620@.The author responsible for distribution of materials integral to the findings presented in this article in accordance with the policy described in the Instructions for Authors()is: Guo-Liang Wang(wang.620@).[C]Somefigures in this article are displayed in color online but in black and white in the print edition.[W]The online version of this article contains Web-only data.[OA]Open access articles can be viewed online without a sub-scription./cgi/doi/10.1104/pp.109.137125single expression vector.This is particularly true when a large number of plasmids must be cloned.Although a one-step recombinational cloning method was de-scribed to eliminate the production of an entry clone (Fu et al.,2008),the approach is rather limited in scope because long primers containing the specific attach-ment site(att)and two-step PCR are required(Fu et al., 2008).TA cloning is routinely used for cloning of PCR-amplified fragments.This technique exploits the ter-minal transferase activity of some DNA polymerases that add a3#-A overhang to each end of the PCR product.PCR products can be easily cloned into a linearized vector with3#-T overhangs compatible with 3#-A overhangs.Because it is difficult to generate a high-quality TA cloning vector in individual laborato-ries,many TA cloning kits are available in the market. Many of them use blue/white screening for recombi-nants,and the DNA fragments can only be cloned into the TA vector provided in the kit.To meet the need for high-throughput cloning of DNA fragments into di-verse expression vectors,we have developed a signif-icantly improved TA cloning vector system by taking advantage of the negative selection gene marker ccdB to eliminate the self-ligation background after trans-formation.We refer to this new method as the zero background TA cloning system(ZeBaTA).Numerous cloning tests in our laboratory have shown that ZeBaTA provides very high cloning efficiency with almost no self-ligation.Moreover,the ZeBaTA technology can be flexibly adapted for developing specialized expression vectors allowing single-step assembly of PCR-ampli-fied genes or fragments.We demonstrate the feasibility andflexibility of the technology by developing a set of 12transient and12stable transformation vectors for constitutive gene expression,gene silencing,protein tagging,protein subcellular localization,and promoter fragment activity analysis for rice(Oryza sativa)and Arabidopsis(Arabidopsis thaliana).Our results suggest that ZeBaTA technology can also be easily used to develop expression vectors for other organisms(e.g. Escherichia coli,yeast[Saccharomyces cerevisiae],insect, and mammal),thereby providing a novel and general high-throughput platform for functional genomics of target genes.RESULTSConstruction of the ZeBaTA SystemTwo different strategies were used to produce T-vectors,i.e.adding a single thymidine at the3# blunt ends of a linearized vector(Holton and Graham, 1991;Marchuk et al.,1991)and generating single3#-T overhangs of a linearized vector by restriction endo-nuclease digestion(Kovalic et al.,1991;Mead et al., 1991;Ichihara and Kurosawa,1993;Chen et al.,2006a). Although the former has been used to produce com-mercial cloning kits like the pGEM-T system,we selected the restriction endonuclease digestion-mediated strategy to develop a TA cloning vector system because this approach is easy to use for indi-vidual laboratories.Previous publications have de-scribed the use of restriction enzyme Xcm I(Kovalic et al.,1991;Mead et al.,1991)or Ahd I/Eam1105I (Ichihara and Kurosawa,1993;Chen et al.,2006a)to produce intermediate T-vectors.We chose Xcm I as the digestion enzyme to develop the ZeBaTA cloning system because it had a better digestion efficiency than AhdI.Figure1.Construction of the ZeBaTA system.A,Schematic represen-tation of direct cloning of PCR product using the ZeBaTA vector system. The linker of the vector(in gray)is removed after Xcm I digestion yielding a linearized T-vector.B,TA cloning tests of the ZeBaTA system.(1)Self-ligation of Xcm I-digested pGXT using T4DNA ligase from Promega.(2)Ligation of Xcm I-digested pGXT with the PCR product of the rice blast fungus M.oryzae gene MGG_07986.5using T4DNA ligase from Promega.(3)Ligation of Xcm I-digested pGXT with the PCR product of MGG_07986.5using T4DNA ligase from USB Corporation. C,Samples of restriction digestion analysis of the randomly selected colonies derived from ligation of Xcm I-digested pGXT with the PCR product of MGG_07986.5using T4DNA ligase from Promega.pGXT contains two Bam HI recognition sites outside the two Xcm I recognition sites(Supplemental Fig.S1),and MGG_07986.5contains one internal Bam HI site.All samples(lanes1–20)digested by Bam HI released two bands as expected.M,1-kb DNA ladder.Chen et al.The schematic illustration of the improved T-vector system for PCR-amplified gene/fragment cloning is shown in Figure 1A.A pair of Xcm I recognition sites,CCAATACT/TGTATGG,was introduced in the vec-tors,which allowed the generation of a single thymi-dine residue at both 3#ends of the vector when digested with Xcm I.To eliminate the potential self-ligation due to incomplete Xcm I digestion of the vec-tor,the ccdB gene (Bernard and Couturier,1992;Miki et al.,1992),which inhibits growth of E .coli strains by expressing a protein to interfere with its DNA gyrase,was introduced between the two Xcm I sites.Hence,any self-ligation transformants containing the ccdB gene will be eliminated.To test the cloning efficiency of the T-vector system,an intermediate vector pGXT was generated based on the backbone of the pGEM-T easy vector.After Xcm I digestion,ligation reactions of the resulting T-vector alone and T-vector with the PCR-amplified product of the rice blast fungus Mag-naporthe oryzae gene MGG_07986.5were set up follow-ing the standard protocol of the Promega pGEM-T easy vector system.Transformation tests showed that ligation of the T-vector with the MGG_07986.5frag-ments yielded a large number of colonies,whereas ligation of the T-vector alone yielded only a few colonies (Fig.1B).Restriction digestion screening con-firmed that the plasmids yielded from ligation of theT-vector with the PCR product were true recombinants (Fig.1C).To establish a general guide for consistently successful cloning,several factors,such as Xcm I over-digestion for generating a T-vector,insert-to-vector molar ratios,and different T4DNA ligases,were tested to determine their effect on cloning efficiency.Surprisingly,we observed that T4DNA ligases could have a significant impact on cloning efficiency.Liga-tions using Promega T4DNA ligase,the same product used by the pGEM-T easy vector system,consistently gave very high cloning efficiency with almost no self-ligation background.However,regular T4DNA li-gases from USB Corporation usually gave very low ligation efficiency for this TA cloning system (Fig.1B).Although the ligation efficiencies were a little higher at insert-to-vector molar ratios of 4:1to 8:1with the T-vector generated by standard digestion,ligations from vectors with 10-or 20-fold overdigestion and ligations with insert-to-vector molar ratios of 1:1,4:1,8:1,and 12:1all yielded good cloning efficiency when Promega T4DNA ligase was used (data not shown).Set of Expression ZeBaTA Vectors for PlantsUsing ZeBaTA,we developed a set of transient and stable expression vectors for different applications in both dicot and monocot plants.The backbone ofallFigure 2.Site-specific mutagenesis of the maize ubiqutin-1promoter (A)and the backbone of the binary vector pCAMBIA1300(B)in which three Xcm I recognition sites were deleted.The nucleotides represented in lowercase italic letters are the positions where deletions or mutations were made.Kan,Kanamycin resistance gene;LB,T-DNA left border;RB,T-DNA right border.C,Comparison of the levels of GUS expression mediated by the original and modified maize ubiquitin-1promoter in transiently transfected rice protoplasts.GUS activities are represented as a ratio of relative GUS/LUC.The experiment was repeated three times with similar results.1,Protoplast sample transfected with pUbiGUS;2,protoplast sample transfected with pXUN-GUS.A Zero Background Vector Systemtransient expression vectors is derived from pBlue-script II KS (),a high-copy-number cloning vector that can facilitate the isolation of a large amount of plasmid DNA for transient ex-pression.The backbone of all stable expression vectors is derived from pCAMBIA1300(),an Agrobacterium tumefaciens binary vector widely used for transformation in both dicot and monocot plants.Two different promoters,a cauliflower mosaic virus 35S promoter (Odell et al.,1985)and a maize (Zea mays )ubiquitin-1promoter (Christensen et al.,1992)were used to drive expression of genes of interest in dicots and monocots,respectively.The 35S promoter is more efficient in dicots,whereas the maizeubiquitin-1Figure 3.ZeBaTA-based expression vectors for gene overexpression/silencing,protein tagging,protein subcellular localization,and promoter analysis in plants.A,Schematic structures of the transient expression vectors generated by Xcm I digestion.B,Schematic structures of the Agrobacterium -mediated stable transformation vectors generated by Xcm I digestion.LB,T-DNA left border;RB,T-DNA right border.Chen et al.promoter is more efficient in monocots(Christensen et al.,1992).The original maize ubiquitin-1promoter and pCAMBIA1300vector,however,contain one and three Xcm I recognition sites,respectively(Fig.2,A and B).To facilitate the construction of the ZeBaTA-based expression vectors,the Xcm I recognition sites of the maize ubiquitin-1promoter and pCAMBIA1300vec-tor were eliminated by site-specific deletion or site-specific mutation(Fig.2,A and C).The designed expression vectors were all engineered with the cas-sette of the Xcm I-ccdB-Xcm I fragment(Supplemental Fig.S1).Figure3,A and B,illustrates the structural maps of the12transient and12stable transformation T-vectors.All vectors have been tested for cloning at least one time,and the results showed that these ZeBaTA expression vectors,including those binary vectors that are relatively large in size(.10kb), consistently yielded high cloning efficiency(Supple-mental Fig.S2).Because the maize ubiquitin-1promoter used in this system was modified to block its original Xcm I recog-nition site by deleting a single base(Fig.2A)at the position of nucleotide2480,a gus gene(Jefferson et al., 1987)was amplified by PCR and then cloned into the Xcm I-digested pXUN vector to produce an expression construct to test the expression activity of the modified maize ubiquitin-1promoter.The derived constructpXUN-GUS and a control construct pUbi-GUS(Chen et al.,2006b),of which a gus gene is driven by the original maize ubiquitin-1promoter,were tested tran-siently in the transfected rice protoplasts.Transient expression assays showed that the levels of GUS activity in rice protoplasts transfected with these two constructs were similar(Fig.2C),indicating that the deletion of nucleotide2480does not affect the activity of the maize ubiquitin-1promoter.Testing of Tagged Protein ExpressionEpitope tagging is a widely used method for the rapid and effective characterization,purification,and in vivo localization of the protein products of cloned genes.To facilitate gene cloning for epitope tagging in plants,a total of12epitope-tagging vectors(Fig.3,A and B)were constructed using ZeBaTA.These vectors contain a35S promoter or a maize ubiquitin-1pro-moter,allowing direct cloning of genes of interest into expression vectors to express a translational fusion of target protein with three commonly used epitope tags in plants(i.e.FLAG,HA,or Myc;Earley et al.,2006). To determine the feasibility of this epitope-tagging system,a gfp gene was cloned into the pXUN-HA vector to fuse with the HA tag.The resulting expres-sion construct pXUN-HA-GFP was transiently ex-pressed in rice protoplasts.As shown in Figure4,A and B,protoplasts transfected with pXUN-HA-GFP showed strong GFPfluorescence,and HA-tagged GFP protein was detected in protein extracts of trans-fected protoplasts but not in the nontransfected con-trol,demonstrating the potential application of this system for functional study of target proteins in plants.Gene Silencing by Hairpin RNAi or Artificial MicroRNA In plants,a typical and efficient approach to induce gene silencing is to use an inverted-repeat construct to express hairpin RNA(hpRNA;Waterhouse et al.,1998; Smith et al.,2000).However,a major limitation of the hpRNA interference(hpRNAi)approach for high-throughput gene functional analysis is the cumber-some cloning procedure for generating hpRNAi constructs(Helliwell and Waterhouse,2003).The gen-eration of a hpRNAi construct using conventional restriction enzyme digestion and DNA ligation meth-ods usually requires several cloning steps.Although Gateway cloning technology has been adapted to gen-erate hpRNAi constructs(Helliwell and Waterhouse, 2003;Miki and Shimamoto,2004),it still requires two cloning steps.With the ZeBaTA system,hpRNAi con-structs can be made by a single-step cloning procedure (Fig.5A).Instead of making an inverted-repeat cas-sette by DNA recombination techniques,we designed a new approach to assemble the hpRNAi cassette by overlapping PCR.Briefly,a target fragment with an additional3#-terminal sequence complementary to both the5#-and3#-terminal ends of a designed spacer fragment is amplified as afirst step.The overlapping fragments are then fused together in a subsequent PCR reaction,and the resulting inverted-repeat is cloned directly into a ZeBaTA expression vector(Fig.5A).To test the feasibility of this approach,an RNAiconstruct Figure4.Transient expression and protein-tagging detection of the ZeBaTA vectors in rice protoplasts.A,Fluorescence microscopy of the expression of HA-tagged GFP in rice protoplasts.B,Detection of HA-tagged GFP by western ne1,Nontransfected control protoplast sample;lanes2to4,independent protoplast samples transfected with pXUN-HA-GFP.[See online article for color version of thisfigure.]A Zero Background Vector Systemwas generated by overlapping PCR in which the sense and antisense 217-bp fragments of the Arabidopsis phytoene desaturase gene (PDS )were separated by a 420-bp stuffer fragment derived from the gus gene.The resulting fragment was cloned into the pCXSN vector (Fig.3B)to generate the expression construct pCXSN-atPDS-RNAi.The RNAi construct was introduced into Arabidopsis by the floral-dip method.Over 80%of transgenic plants had a clear albino phenotype (Fig.6A),a typical visible phenotype caused by silencing of the PDS gene (Guo et al.,2003;Miki and Shimamoto,2004).Recently,the artificial microRNA (amiRNA)ap-proach has been introduced for highly specific gene silencing in both dicot and monocot plants (Niu et al.,2006;Schwab et al.,2006;Ossowski et al.,2008;Warthmann et al.,2008).Typically,the amiRNA is generated by site-directed mutagenesis on precursors of endogenous miRNAs to exchange the natural miRNA sequences with those of amiRNAs using overlapping PCR (Ossowski et al.,2008).The same ZeBaTA-based vector system developed for ectopic gene expression and hpRNAi can also be used for making amiRNA expression constructs by simpleTAFigure 5.Schematic illustration of the construction of hpRNAi or amiRNA constructs by single-step cloning.A,Generation of hpRNAi constructs by overlapping PCR approach.The target gene fragment and the stuffer sequence fragment are amplified in the first-round PCR.Primers P2,P3,and P4introduce complementary adapters (indicated by vertically lined boxes)to the amplified fragments.The two amplified fragments are fused together as an inverted-repeat cassette in the second-round PCR by using single P1primer.The resulting fragment is then directly cloned into the plant expression T-vector.B,Generation of amiRNA constructs by overlapping PCR approach.C,Generation of amiRNA constructs for rice genes by single-step PCR.The expression vectors pXUN-osaMIR528and pCXUN-osaMIR528were preassembled with 5#and 3#stemloop backbone sequences of a rice miRNA precursor Osa-MIR-528(Warthmann et al.,2008).Thus,making amiRNA constructs for rice target genes only requires an amiRNA-amiRNA*fragment generated from single-step PCR.The nucleotides represented in lowercase letters are the positions where mutations were made to introduce two Xcm I recognition sites.Chen et al.cloning (Fig.5B),thus bypassing the time-consuming two-step procedure for the regular restriction enzyme digestion-mediated cloning or the Gateway cloning (Ossowski et al.,2008).We further developed a ZeBaTA-amiRNA system to simplify the generation of rice amiRNA constructs because our lab is focusing on rice functional genomics.The new ZeBaTA-amiRNA vector was designed based on the stemloop backbone derived from Osa-MIR528,an endogenous rice miRNA precursor that has been used to efficiently express amiRNAs for highly specific silencing of targeted genes in rice (Warthmann et al.,2008).By site-directed muta-genesis of a single base on the 5#and 3#stemloop backbones of Osa-MIR528,respectively,a cassette of 5#Osa-MIR528stemloop backbone-Xcm I-ccdB -Xcm I-3#Osa-MIR528was assembled and cloned into the expres-sion vectors where the expression of amiRNA is under the control of the maize ubiquitin-1promoter.Figure 5C illustrates the structural maps of the Osa-MIR528-based vectors pXUN-osaMIR528and pCXUN-osaMIR528.The vectors allow for high-throughput generationof rice amiRNA constructs by cloning the amiRNA-amiRNA*fragment generated from a single-step PCR into the ZeBaTA vector with the preassembled Osa-MIR528stemloop backbone (Fig.5C;Supplemental Fig.S3),thus avoiding the time-consuming overlapping PCR.The modified vector was evaluated by expression of the amiRNA for silencing of the OsPDS gene.The two constructs pCXUN-amiPDS and pCXUN528-PDS,which contain original or modified Osa-MIR528stem-loop backbone with amiRNA sequence targeting OsPDS ,respectively ,were introduced into rice cv Nip-ponbare by Agrobacterium -mediated transformation.Consistent with a previous study (Warthmann et al.,2008),70.1%of the primary transgenic lines transformed with pCXUN-amiPDS had a bleaching PDS silencing phenotype (Fig.6B;Table I).Similarly,77.1%of the primary transgenic lines transformed with pCXUN528-PDS had the same albino phenotype,suggesting that the mutagenesis on the Osa-MIR528stemloop backbone does not affect the biogenesis of the amiRNA for silenc-ing of the PDS gene.Protein Subcellular Localization/Colocalization and Promoter Activity AssayTo investigate the subcellular localization or colo-calization of particular proteins,a set of ZeBaTA vectors (i.e.pXDG,pXDR,pCXDG,and pCXDR)was devised for transient or stable expression of protein fusions with GFP or red fluorescent protein.The vectors contain a 35S promoter-driven gfp or DsRed cassette that has been used to visualize protein local-ization in both dicot and monocot plants (Goodin et al.,2002;Chen et al.,2006b).As shown in Figure 3,A and B,PCR products of genes of interest can be simply engineered into the vectors to fuse with the gfp or DsRed gene.To confirm whether the vectors can be used for detecting protein localization in plant cells,the rice Spin1gene encoding a putative RNA-binding protein previously shown to be nuclear targeted(Vega-Sa´nchez et al.,2008)was cloned into vectors pXDG and pXDR to fuse in-frame with gfp and DsRed ,respectively.Transient expression of the constructs pXDG-Spin1and pXDR-Spin1in rice protoplasts dem-onstrated that the GFP-and DsRed-SPIN1fusion proteins were targeted to the nuclear region as pre-dicted (Fig.7A).For promoter activity assays,two reporters,gus and gfp ,were used for constructing pXGUS-P/pCXGUS-P and pXGFP-P/pCXGFP-P ,respectively.The linear T-vectors of pXGUS-P/pCXGUS-P orpXGFP-P/Figure 6.Silencing of the PDS gene in Arabidopsis and rice by the ZeBaTA-based hpRNAi or amiRNA approaches.A,Arabidopsis plants transformed with the hpRNAi construct pCXSN-atPDS-RNAi showing the PDS silencing albino phenotype.(1)Control plant;(2and 3)two examples of transgenic Arabidopsis plants.B,Rice plants transformed with the amiRNA vectors showing the albino phenotype.(1)Control plant;(2)example of pCXUN-amiPDS-transformed plants;and (3)example of pCXUN528-PDS-transformed plants.C,RT-PCR analysis of PDS suppression transgenic rice plants.Five independent primary plants (1,2,3,4,and 5)transformed with pCXUN-amiPDS and five independent primary plants (6,7,8,9,and 10)transformed with pCXUN528-PDS were selected for the analysis.CK,Wild-type Nip-ponbare plant used as the control.Table I.PDS silencing frequency of transgenic rice mediated by the ZeBaTA-amiRNA systemamiRNA VectorTotal Independent TransformantsAlbino PhenotypeEfficiency%pCXUN-amiPDS 553970.1pCXUN528-PDS 352777.1A Zero Background Vector SystempCXGFP-P (Fig.3,A and B)allow direct cloning of PCR-amplified promoter fragments located in front of the reporter genes.As proof of concept,the 35S pro-moter was cloned into pCXGUS-P to drive expression of the reporter gene gus .Arabidopsis plants stably transformed with the construct pCX-35S-GUS showed constitutive GUS expression in the whole plants (Fig.7B),confirming the feasibility of the system for assay-ing promoter activity.DISCUSSIONWith the rapid development of the next-generation sequencing technology,more plant genomes will be sequenced in the near future.How to rapidly deter-mine the function of the identified genes on a large scale is a daunting challenge.The ability to efficiently make constructs to transiently and stably express specific genes in cells,tissues,or whole plants is a fundamental aspect and bottle neck of plant functional genomics research.Traditionally,the cloning vectorsfor plant research carry a multicloning site (MCS)within their target gene expression cassettes.The restriction sites in the MCS are rather limited,making cloning of most target genes difficult.Although TA cloning vectors have been widely used for cloning of PCR-amplified fragments,the system has not yet been incorporated in the cloning vectors for transient and stable expression of target genes because of the tech-nical challenge of generating low-background TA cloning vectors.The Gateway system has been a popular choice for generating various constructs be-cause it allows the gene of interest to be easily cloned into specifically designed plasmids without DNA re-striction digestions.The two-step cloning and expen-sive reagents,however,make the Gateway system impractical for large-scale cloning in most individual laboratories when the entry clone collections are not available.The ZeBaTA system described here over-comes the limitations of both the TA and Gateway cloning systems.After two Xcm I recognition sites have been introduced into the MCS,any PCR fragments with a T-overhang can be easily cloned into a ZeBaTA vector.With the introduction of the negative selection marker gene ccdB between the two Xcm I sites,any self-ligation transformants are ing this tech-nology,we constructed a set of 12transient and 12stable transformation vectors for plant gene expres-sion studies and tested the vectors in rice or Arabi-dopsis in our laboratories.These vectors can be used in a wide range of functional genomics projects in plants and will be distributed to the research community upon request.Under certain conditions,cloning with T-vectors generated by digestion with Ahd I or Xcm I gave low efficiency and the T residue of the insert-vector junc-tion in the recombinant clones is often missing (Mead et al.,1991;Chen et al.,2006a).Chen et al.(2006a)speculated that this may be due to the presence of unknown factors that,during digestion and prepara-tion of the T-vectors,influence the stability of 3#-T overhangs.In this study,we found that the main factor affecting successful cloning is the use of an appropri-ate T4DNA ligase.We tested the Promega T4DNA ligase,which is included in the pGEM-T easy vector system,and the T4DNA ligase from USB Corporation.The ligations using Promega T4DNA ligase consis-tently gave a very high cloning efficiency;most of the ligations using USB Corporation T4DNA ligases yielded low efficiency.Many of the recombinant plas-mids from the latter ligations missed a T residue in the insert-vector junction,consistent with observations by Mead et al.(1991)and Chen et al.(2006a).The T residue is missing mainly because regular commercial T4DNA ligases contain exonuclease activities that can remove the 3#-T tails from the vector,as reported in the technical manual of the pGEM-T and pGEM-T easy vector systems (/tbs/tm042/tm042.pdf);removal of the 3#-T tails from the vector results in very low cloning efficiency.When the Promega T4DNA ligase was used for ligation,weFigure 7.Protein subcellular localization and promoter activity anal-ysis using the ZeBaTA vectors.A,Fluorescence microscopy of the coexpression of GFP and DsRed,or GFP-SPIN1and DsRed-SPIN1fusions in rice protoplasts.Scale bar =20m m.The RNA binding nuclearprotein SPIN1was used as a tester (Vega-Sa´nchez et al.,2008).B,GUS staining of Arabidopsis transformed with pCX-35S-GUS,where the 35S promoter was cloned into the vector pCXGUS-P to test the system.CK,Plant transformed with control vector pCAMBIA1300();pCX-35S-GUS-1and pCX-35S-GUS-2,two independent primary transgenic plants.Chen et al.。
Analytic Moment-based Gaussian Process Filtering
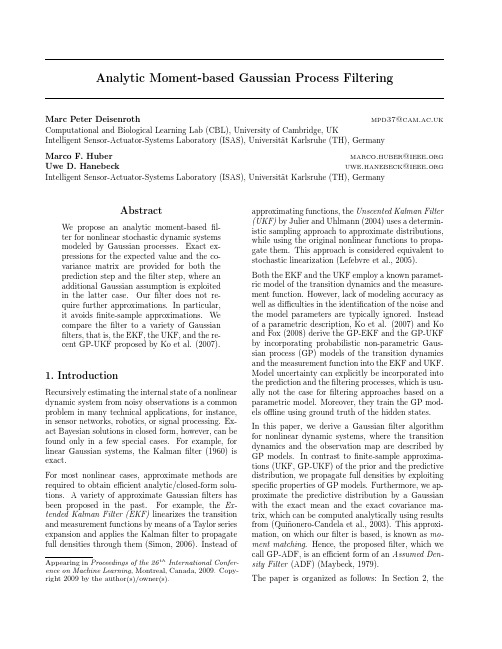
Prediction Step When we predict, we determine the distribution p(xk |y1:k−1 ) of the hidden state xk , where the result of the previous filter result p(xk−1 |y1:k−1 ) serves as the prior. Bayes’ law yields p(xk |y1:k−1 ) = p(xk |xk−1 )p(xk−1 |y1:k−1 ) dxk−1 (3) by averaging over xk−1 . Often, the involved integral and the multiplication cannot be solved analytically and require approximate methods. Filter Update The filter update determines the distribution p(xk |y1:k ) of the hidden state xk based on collected observations from all previous and the current time steps. Bayes’ law yields the filter update p(xk |y1:k ) = p(yk |xk )p(xk |y1:k−1 ) . p(yk |y1:k−1 ) (4)
f( · ) x k −1 xk xk+1
Table 1. Classification of Gaussian filter methods. samples full density f, g : known f, g : unknown UKF GP-UKF EKF GP-ADF
Introduction to Maize GDB database
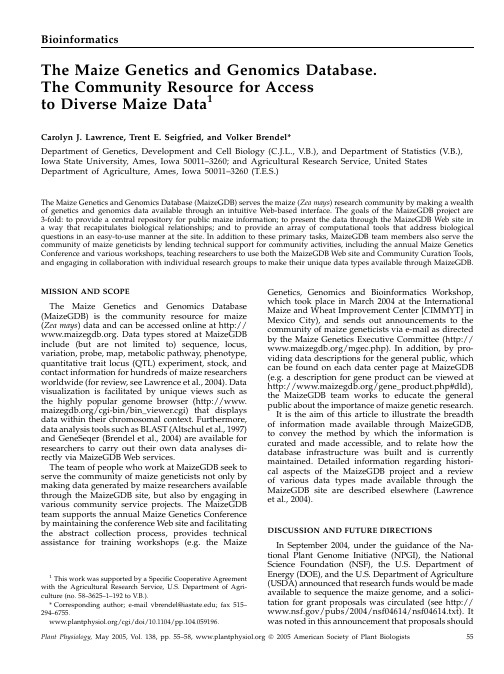
BioinformaticsThe Maize Genetics and Genomics Database.The Community Resource for Accessto Diverse Maize Data1Carolyn wrence,Trent E.Seigfried,and Volker Brendel*Department of Genetics,Development and Cell Biology(C.J.L.,V.B.),and Department of Statistics(V.B.), Iowa State University,Ames,Iowa50011–3260;and Agricultural Research Service,United States Department of Agriculture,Ames,Iowa50011–3260(T.E.S.)The Maize Genetics and Genomics Database(MaizeGDB)serves the maize(Zea mays)research community by making a wealth of genetics and genomics data available through an intuitive Web-based interface.The goals of the MaizeGDB project are 3-fold:to provide a central repository for public maize information;to present the data through the MaizeGDB Web site in a way that recapitulates biological relationships;and to provide an array of computational tools that address biological questions in an easy-to-use manner at the site.In addition to these primary tasks,MaizeGDB team members also serve the community of maize geneticists by lending technical support for community activities,including the annual Maize Genetics Conference and various workshops,teaching researchers to use both the MaizeGDB Web site and Community Curation Tools, and engaging in collaboration with individual research groups to make their unique data types available through MaizeGDB.MISSION AND SCOPEThe Maize Genetics and Genomics Database (MaizeGDB)is the community resource for maize (Zea mays)data and can be accessed online at http:// .Data types stored at MaizeGDB include(but are not limited to)sequence,locus, variation,probe,map,metabolic pathway,phenotype, quantitative trait locus(QTL)experiment,stock,and contact information for hundreds of maize researchers worldwide(for review,see Lawrence et al.,2004).Data visualization is facilitated by unique views such as the highly popular genome browser(http://www. /cgi-bin/bin_viewer.cgi)that displays data within their chromosomal context.Furthermore, data analysis tools such as BLAST(Altschul et al.,1997) and GeneSeqer(Brendel et al.,2004)are available for researchers to carry out their own data analyses di-rectly via MaizeGDB Web services.The team of people who work at MaizeGDB seek to serve the community of maize geneticists not only by making data generated by maize researchers available through the MaizeGDB site,but also by engaging in various community service projects.The MaizeGDB team supports the annual Maize Genetics Conference by maintaining the conference Web site and facilitating the abstract collection process,provides technical assistance for training workshops(e.g.the Maize Genetics,Genomics and Bioinformatics Workshop, which took place in March2004at the International Maize and Wheat Improvement Center[CIMMYT]in Mexico City),and sends out announcements to the community of maize geneticists via e-mail as directed by the Maize Genetics Executive Committee(http:// /mgec.php).In addition,by pro-viding data descriptions for the general public,which can be found on each data center page at MaizeGDB (e.g.a description for gene product can be viewed at /gene_product.php#dld), the MaizeGDB team works to educate the general public about the importance of maize genetic research. It is the aim of this article to illustrate the breadth of information made available through MaizeGDB, to convey the method by which the information is curated and made accessible,and to relate how the database infrastructure was built and is currently maintained.Detailed information regarding histori-cal aspects of the MaizeGDB project and a review of various data types made available through the MaizeGDB site are described elsewhere(Lawrence et al.,2004).DISCUSSION AND FUTURE DIRECTIONSIn September2004,under the guidance of the Na-tional Plant Genome Initiative(NPGI),the National Science Foundation(NSF),the U.S.Department of Energy(DOE),and the U.S.Department of Agriculture (USDA)announced that research funds would be made available to sequence the maize genome,and a solici-tation for grant proposals was circulated(see http:// /pubs/2004/nsf04614/nsf04614.txt).It was noted in this announcement that proposals should1This work was supported by a Specific Cooperative Agreement with the Agricultural Research Service,U.S.Department of Agri-culture(no.58–3625–1–192to V.B.).*Corresponding author;e-mail vbrendel@;fax515–294–6755./cgi/doi/10.1104/pp.104.059196.utilize existing,previously funded resources,one of the resources listed explicitly being the maize com-munity genome database.Currently,MaizeGDB provides a portal to maize genome sequencing information (which can be viewed at /genome).Displayed on that page are documents outlining details concerning the maize genome sequencing endeavor,links to maize sequence repositories,information outlining the di-versity of sequencing strategies currently employed,and a list of relevant publications germane to the maize genome sequencing project.As the maize ge-nome gets sequenced,MaizeGDB will adapt to pro-vide a sequence-centered portal to all maize data similar to the one provided by The Arabidopsis Infor-mation Resource (TAIR;;Rhee et al.,2003).Presently,the number of people working at MaizeGDB is quite small when compared to the per-sonnel associated with other database projects like TAIR (),Gramene (),and the Solanaceae Genomics Network (SGN;).In order to serve as a central site for making large numbers of sequences,contigs,assemblies,and,even-tually,a fully sequenced maize genome available at MaizeGDB,it is necessary that partnerships be forged between MaizeGDB and other databases,sequencing projects,and large-scale generators of maize data.In an initial effort to engage in such a collaboration,a pipeline for getting sequence data into MaizeGDB has been developed wherein all available maize se-quences are downloaded from GenBank (/Genbank)by staff working at the Plant Genome Database (PlantGDB;http://www.;Dong et al.,2004).Once the sequences have been cleaned,analyzed,and assembled into contigs at PlantGDB,they are delivered to MaizeGDB for long-term storage and display.By creating intuitive links to PlantGDB and facilitating database interoper-ability between MaizeGDB and PlantGDB,the weight of the burden to deliver high-quality sequence-based products to the community of maize geneticists is shared among mutually benefiting parties.Such col-laborations are useful and are facilitated by initiatives like the Plant Ontology Consortium (;Bruskiewich et al.,2002),a group working to create controlled vocabularies for describ-ing plant structures and growth and developmental stages to facilitate database interoperability among resources serving plant biologists working on a broad range of species.Forging new,useful partnerships with other databases and helping to develop methods to facilitate database interoperability are the highest priority tasks facing the MaizeGDB team in the coming year.Over the course of the past year,cytological map images generated by the Cytogenetic Map of Maize project (;Koumbaris and Bass,2003)were added to the database,and displays for cytological map data were developed (e.g./cgi-bin/displayfishrecord.cgi?id 512098&map 5892372).Community and Professional Curation Tools were developed for most data types,and the MaizeGDB Editorial Board began selecting noteworthy maize primary literature for rigorous pro-fessional data curation (see /editorial_board.php).During the coming months,work will focus on associating Plant Ontology ()terms of type PlantStructureFigure 1.The name of a probe can be used at MaizeGDB to locate SNP data available at CerealsDB.The researcher first searches ‘‘all records’’from the top of any MaizeGDB page (A;step 1,circled in red)using ‘‘p-csu571’’as her query;she locates the probe record.From the probe page (A),the researcher follows a link (step 2,circled in red)to view the MaizeGDB sequence display page.From the sequence display page (B),she follows a link to CerealsDB (step 3,circled in red)to check for SNP contigs that include the sequence.Data describing a putative SNP contig (C)are presented directly via the CerealsDB site (/maize_snips/snip_1563.htm).The sequence of interest (EST AA051884)is listed at the bottom of the group of sequences shown.Lawrence et al.and Trait (an ontology currently under development)with records,and the raw data generated by QTL experiments will be made available.A Community Curation Tool module for QTL data is slated for development in the very near future.MATERIALS AND METHODSWeb Interfaces Allow Access to Data within a Biological ContextThe data stored at MaizeGDB are made available through a series of interconnected Web pages.Researchers can also contact the MaizeGDB team at mgdb@ to request access to Web-based read-only SQL tools allowing direct queries on the curation copy of the database.These pages are coded in HTML,and most are automatically generated by PHP and Perl scripts.Through the Web interface (accessible at ),each data display page shows detailed information on a specific biological entity (e.g.a locus),as well as basic information about data associated with it (e.g.maps,variations,probes,and citations are among data types associated with loci),and links to related off-site resources (e.g.locus pages link directly to Gramene;Ware et al.,2002).Access to individual data displays is made possible through a number of different mechanisms,including a text search tool (available at the top right corner of each page)and a genome browser (located at the bottom left of the site’s main page).These tools and inter-connected pages allow researchers to easily navigate from point to point as they investigate research topics of interest.The interface design attempts to maximize the information available,requiring only a minimal amount of input from the researcher.MaizeGDB’s method of data delivery is aimed at making information available within the framework of its scientific meaning.Data displays place specific pieces of data within a biological context.For example,if you arrive ata map page by way of a locus page,the locus that was last visited is high-lighted within the map display.Not only does using the biological relatedness of data types in conjunction with following a researcher’s clickstream enable the interface to reflect real biological relationships,it also aids researchers by causing the site to seem to follow their actual train of thought.The following usage case demonstrates the interrelatedness of different types of biological information,reveals MaizeGDB’s method of recapitulating those interrela-tionships,and highlights the placement of links to off-site resources that can help researchers gain access to related information that is not stored at MaizeGDB.An intrepid researcher visits MaizeGDB in the hopes of finding informa-tion that would help her to design multiplex PCR primers to genotype F 2plants.She is working to determine the transmission of different variants of chromosome 10,and wishes to develop a protocol for diagnosing which chromosome 10variants are present in any given plant growing in a half-acre field.Because the researcher knows that the expressed sequence tag (EST)probe p-csu571labels bands that migrate differentially on Southern blots between the two backgrounds of interest (Mroczek,2003),she decides to start by investigating sequence data for that EST.In Step 1,by searching ‘‘all records’’from the top of any MaizeGDB page (see Fig.1A)using ‘‘p-csu571’’as her query,she locates the probe record for p-csu571(/cgi-bin/displayestrecord.cgi?id 5118621)and discovers that the sequence AA051884is associated with p-csu571.In Step 2,she follows the sequence link to view the MaizeGDB copy of that sequence record.In Step 3,in the right bar on the sequence record page (/cgi-bin/displayseqrecord.cgi?id 51531734;Fig.1B),a link to ‘‘Find SNPs for AA051884at CerealsDB’’is displayed.By clicking that link to automatically search CerealsDB (/discover.htm;Barker et al.,2003)for single-nucleotide polymorphisms (SNPs)that include AA051884(gi 1531734),she identifies a putative SNP cluster (snip_1563;Fig.1C)de-monstrating that,among sequences similar to AA051884,multiple polymor-phisms exist.This information will allow her to design a multiplex PCR experiment protocol that could enable her to genotype plants in the field without resorting to performing hundreds of time-consuming Southern blots.Most of the continued development of the MaizeGDB interface is guided by members of the maize (Zea mays )genetics research community:Commu-nity members have sent hundreds of suggestions and requests concerning methods to find and display data.To aid in encouraging community feedback,a highly utilized context-sensitive feedback tool appears at the bottom of every page.The needs of researchers serve as the major impetus for interface development,and addressing those needs directly allows for tools to be developed that are both timely and germane to the needs of maize geneticists.An example of a research need that guided interface development comes from Bill Sheridan,a maize geneticist working in the Department of Biology at the University of North Dakota.Dr.Sheridan contacted the MaizeGDB team seeking an easy method to summarize which simple sequence repeats (SSRs)detected bacterial artificial chromosomes that were also associated with genetically mapped markers.Dr.Sheridan worked with the MaizeGDB team to design a table-generating tool that provides approximate map loca-tions for markers,the SSRs for those markers,and bacterial artificial chromo-somes detected by the SSRs (see the links to each of the 10maize chromosomes beneath the heading ‘‘Mapped &Anchored SSRs’’at /ssr.php).Dr.Sheridan was able to use this tool for his research and was pleased that his input guided the development of such a useful tool.This sort of interface development to address specific research needs typifies the method by which members of the MaizeGDB team work alongside research-ers to help them gain access to complex relationships documented in the database.In summary,MaizeGDB’s interface was initially designed to provide a context for interpreting maize data,and continued interface development is driven by specific input from and collaborative design with members of the maize research community at large.Data Curation:Driven by the Community of Maize GeneticistsAt present,the MaizeGDB team does not have any individual member dedicated solely to data curation.Instead,all team members curate data as the need arises and in accordance with his or her particular knowledge base.Most data are added to the curation copy of the database (see below for a description of how each copy of the database is utilized)in bulk and are contributed by community members directly.Feedback from researchers often guides in-dividual data additions and edits.By describing which data to associate with existing records or by explaining why mistakenly associatedinformationFigure 2.Researchers can add annotation to records at MaizeGDB.After having logged in to the site,the researcher’s username appears in the upper right corner of the window,and links appear on pages allowing the researcher to add annotations (top red circle).Once an annotation has been added,it appears toward the bottom of the page (bottom red circle)along with the contributor’s name and the date the annotation was submitted.The Maize Genetics and Genomics Databaseshould be updated,the community of maize geneticists contributes directly to curating the data stored at MaizeGDB.Moreover,community members can add annotations to records at MaizeGDB by logging in through the annotation link at the top of any MaizeGDB page.Once logged in,researchers can add notes like the one shown in Figure2(/cgi-bin/ displaybacrecord.cgi?id5424644)by clicking the link to‘‘Add your own annotation to this record’’shown at the top of virtually all data displays.For researchers interested in contributing data directly to the database,a set of Java-based Community Curation Tools has been developed and is available for general use.Data types accessible through these tools include clone library, gel pattern,gene product,linkage group,locus,map,map scores,panel of stocks,person,phenotype,primer/enzyme,probe,recombination data, reference,species,stock,term,and variation.By creating data records,re-searchers become Community Curators who own the records they create and retain the ability to edit owned records directly.To ensure that records entered by community members are complete, a curation level system has been implemented.Newly entered records are considered‘‘submitted’’and are checked by a professional curator.Once checked,the records are marked‘‘approved’’or‘‘failed,’’and only‘‘ap-proved’’records become publicly accessible through the Web interface.Each time a community member edits a record he or she owns,the record is reassigned the‘‘submitted’’curation level and must be reapproved to regain accessibility through the Web interface.Workshops demonstrating the use of the Community Curation Tools were taught at Iowa State University(ISU)in August2004and at the Plant and Animal Genome Conference in San Diego,January2005.To schedule an on-site training session for your research group,contact the MaizeGDB team at mgdb@.Professional curators have access to a set of Java-based Professional Curation Tools that were originally created to interact with the Maize Genetics Cooperation Stock Center(MGCSC)MySQL copy of the database and that subsequently were adapted to interact with the ISU Oracle-based curation database.Whereas the Community Curation Tools were designed specifically to allow researchers from the community of maize geneticists to gain limited and controlled access to the database,the Professional Curation Tools allow less restricted access to data,enabling professional curators to create and edit records in an efficient and authoritative manner.Standard Operating Procedures,Accessibility,and Machine ArchitectureThree copies of the MaizeGDB database exist at ISU:a production copy, a curation(staging)copy,and a test copy.Each copy is housed on a separate machine.The production copy of the database is accessible through http:// .This copy is not edited and is accessible by the public through the Web interface.The curation copy of the database is accessible by both community and professional curators via curation tools:It is the copy of the database to which new data are added and within which existing data are edited when the need to do so arises.The curation copy of the database is dumped in a compressed form tofile each pressed daily dumps of the curation database are formatted for Oracle and can be accessed at http:// /;oracle.A typical dumpfile is currently approxi-mately750MB in size(approximately2GB when uncompressed).Dumps from the curation database are housed on a different machine than the curation database itself.Individuals can request copies of the curation database(or individual tables contained therein;see http://www.maizegdb. org/MaizeGDBSchema.pdf for access to the current MaizeGDB schema)for-matted for Oracle,MySQL,or Microsoft Access by e-mailing the MaizeGDB team at mgdb@.On thefirst Tuesday of each month,a duplicate of the curation copy of the database replaces the production copy.Scheduled replacements are announced at the bottom right of the main page(http:// ).The test copy of the database serves as a testing ground for tool development and improvements to both Community and Professional Curation Tools and is also used as a training site for community curators to gain familiarity with the functionality of the Community Curation Tools before using them to access the curation copy of the database.The servers that support MaizeGDB run Oracle9i,which is licensed every 2years.The machines that house the various copies of the database are Dell PowerEdge servers(Round Rock,TX)with232.0GHz Xeon processors,4 GB of RAM,5373GB Ultra32010K RPM drives with Red Hat Advanced Server2.1(Raleigh,NC)installed.All servers are nearly identically config-ured.In addition to the copies of the database housed at ISU,a MySQL copy exists at the MGCSC in Urbana/Champaign,Illinois,enabling the staff of the MGCSC to keep track of data associated with maize stocks directly(a service described in detail in Scholl et al.,2003).The MGCSC copy of the database is accessible through the Professional Curation Tools and is synchronized with the ISU curation database at regular intervals. ACKNOWLEDGMENTSWe are indebted to Darwin A.Campbell for his work as the MaizeGDB database administrator;Qunfeng Dong for his work as the database manager at PlantGDB,the source for all sequence data made available through MaizeGDB;and Marty Sachs,Director of the MGCSC,for his work curating stock and associated data types.We also thank Michael Brekke,systems support specialist;Sanford B.Baran,contract Web developer and creator of the Community Curation Tools;and Jason Carter,information technology specialist for the MGCSC and creator of the Professional Curation Tools that enable the MGCSC and MaizeGDB to maintain data synchronization. MaizeGDB would not have been possible without the legacy work of Ed Coe and Mary Polacco on the original MaizeDB resource.We are grateful for their continued interest and contributions.Received December31,2004;returned for revision January28,2005;accepted February20,2005.LITERATURE CITEDAltschul SF,Madden TL,Schaffer AA,Zhang J,Zhang Z,Miller W, Lipman DJ(1997)Gapped BLAST and PSI-BLAST:a new generation of protein database search programs.Nucleic Acids Res25:3389–3402 Barker G,Batley J,O’Sullivan H,Edwards KJ,Edwards D(2003)Re-dundancy based detection of sequence polymorphisms in expressed sequence tag data using autoSNP.Bioinformatics19:421–422Brendel V,Xing L,Zhu W(2004)Gene structure prediction from consensus spliced alignment of multiple ESTs matching the same genomic locus.Bioinformatics20:1157–1169Bruskiewich R,Coe EH,Jaiswal P,McCouch S,Polacco M,Stein L, Vincent L,Ware D(2002)The Plant Ontology TM Consortium and plant p Funct Genomics3:137–142Dong Q,Schlueter SD,Brendel V(2004)PlantGDB,plant genome database and analysis tools.Nucleic Acids Res32:D354–D359 Koumbaris GL,Bass HW(2003)A new single-locus cytogenetic mapping system for maize(Zea mays L.):overcoming FISH detection limits with marker-selected sorghum(S.propinquum L.)BAC clones.Plant J35: 647–659Lawrence CJ,Dong Q,Polacco ML,Seigfried TE,Brendel V(2004) MaizeGDB,the community database for maize genetics and genomics.Nucleic Acids Res32:D393–D397Mroczek RJ(2003)Molecular,genetic,and cytogenetic analysis of the structure and organization of the abnormal chromosome10of maize.PhD dissertation.University of Georgia,Athens,GARhee S,Beavis W,Berardini T,Chen G,Dixon D,Doyle A,Garcia-Hernandez M,Huala E,Lander G,Montoya M,et al(2003)The Arabidopsis Information Resource(TAIR):a model organism data-base providing a centralized,curated gateway to Arabidopsis biology, research materials and community.Nucleic Acids Res31:224–228 Scholl R,Sachs MM,Ware D(2003)Maintaining collections of mutants for plant functional genomics.In E Grotewold,ed,Plant Functional Genomics,Vol236.Humana Press,Totowa,NJ,pp311–326Ware DH,Jaiswal P,Ni J,Yap IV,Pan X,Clark KY,Teytelman L, Schmidt SC,Zhao W,Chang K,et al(2002)Gramene,a tool for grass genomics.Plant Physiol130:1606–1613Lawrence et al.。
read checksum error for16384b block

read checksum error for16384b block Checksum errors are a common occurrence when dealing with data transmission or storage. These errors can occur due to various factors including hardware malfunctions, software bugs, or even environmental interference. One specific example of a checksum error is when it is encountered for a 16384-byte block.In order to understand the root cause of this issue, let's first delve into what a checksum is and how it is calculated. A checksum is a value that is calculated from a set of data, such as a file or a block of memory. Its purpose is to ensure data integrity by evaluating the error rate during transmission or storage. When the data is received or read back, the checksum is recalculated, and any discrepancies between the original and the recalculated checksum indicate the presence of errors.A 16384-byte block is a sizable chunk of data, and checksum errors can have significant implications in terms of data corruption. To address this issue, we will explore the steps involved in diagnosing the cause of the checksum error and potential solutions to rectify it.Step 1: Identify the Source of the ErrorThe first step in tackling the checksum error is to identify the source of the problem. Since checksum errors can be caused by various factors, it is essential to investigate all possible contributors. One common cause of such errors is faulty hardware or transmission channels. Thus, checking and verifying the integrity of the hardware components involved, such as disk drives, network cables, or memory modules, is a critical initial step.Step 2: Check for External FactorsWhile hardware-related issues are often responsible for checksum errors, external factors can also play a role. Environmental factors, such as electromagnetic interference or power fluctuations, can introduce errors during data transmission. It is important to assess the environment where the error occurred and take proper precautions to mitigate potential sources of interference.Step 3: Review Software and FirmwareSoftware bugs or firmware inconsistencies are another potential cause of checksum errors. It is necessary to eliminate any software-related issues by reviewing the relevant programming codes and ensuring that all components are updated to the latest versions. This includes operating systems, device drivers, and anyother relevant software or firmware that might impact data transfer or storage.Step 4: Recalculate the ChecksumAt this stage, if the previous steps have not resolved the issue, it is imperative to recalculate the checksum of the 16384-byte block. This will help determine if the error is specific to the block or extends to other portions of the data. By recalculating the checksum and comparing it with the corrupted data, it is possible to pinpoint the exact location and nature of the error, which can be useful in troubleshooting and finding a suitable solution.Step 5: Implement Error Correction TechniquesAfter precisely identifying the nature of the checksum error, appropriate error correction techniques can be employed to rectify the problem. These techniques vary depending on the specific circumstances surrounding the error. For example, if the error is caused by faulty hardware, repairing or replacing the defective components may be necessary. In cases where the error is due to software or firmware issues, applying patches or updates might suffice.Step 6: Retest and MonitorOnce the potential solutions have been implemented, it is crucial to retest the system and monitor it for any recurring issues. This step is essential to ensure that the problem has been fully resolved and that the checksum error no longer occurs. System monitoring allows for the detection of any new errors that may arise, enabling prompt action to minimize their impact.In conclusion, encountering a checksum error for a 16384-byte block can be a perplexing issue with various potential causes. However, by following a systematic approach encompassing identification, elimination of external factors, review of software and firmware, checksum recalculation, implementation of error correction techniques, and diligent retesting, it is feasible to diagnose and resolve the problem. Data integrity is paramount in any system, and mitigating checksum errors ensures the reliability and accuracy of the transmitted and stored information.。
- 1、下载文档前请自行甄别文档内容的完整性,平台不提供额外的编辑、内容补充、找答案等附加服务。
- 2、"仅部分预览"的文档,不可在线预览部分如存在完整性等问题,可反馈申请退款(可完整预览的文档不适用该条件!)。
- 3、如文档侵犯您的权益,请联系客服反馈,我们会尽快为您处理(人工客服工作时间:9:00-18:30)。
Backhaul Requirements for Centralized and Distributed Cooperation TechniquesDr. Stefan Brück Qualcomm CDMA Technologies8th July 2010OverviewThe LTE Architecture The X2 Interface in LTE Release 8An introduction to the X2AP protocolCategories of Downlink Coordinated Multipoint (CoMP) Two Implementation ExamplesCentralized Joint Transmission Decentralized Coordinated Scheduling/BeamformingX2AP Protocol ExtensionsExamples for the considered CoMP schemes*)Bandwidth and Delay Requirement Analysis for Downlink CoMP Conclusions2*) S. Brueck, L. Zhao, J. Giese, M. A. Awais, “Centralized Scheduling for Joint-Transmission Coordinated Multi-Point in LTE-Advanced” and J. Giese, M. A. Awais, “Performance Upper Bounds for Coordinated Beam Selection in LTE-Advanced” in Proceedings of the ITG/IEE Workshop on Smart Antennas, Bremen (WSA'10), Germany, 23. – 24. February 20102The LTE ArchitectureMME/S-GW MME/S-GWThe core network is packetswitched only The E-UTRAN consists of one node only S1 interface between S-GW/MME and eNBEPCS1 S1 S1S1 S1 S1Logical many-to-many interface Supports procedures to establish, maintain and release E-UTRAN Radio Access Bearers Supports transfer of NAS signalling messages between UE and EPCE-UTRANeNB X2 eNBX2 X2eNBX2 interface between eNBsLogical point-to-point interface Seamless mobility Interference management Load management3EPC = Evolved Packet Core3X2 Protocol StructureRadio Network Layer Control Plane X2-AP User Plane User Plane PDUsTransport Network User PlaneTransport Network LayerTransport Network User PlaneSignaling TransportGTP-U SCTP IP Data link layer Physical layer UDPData TransportIP Data link layer Physical layerClear separation between radio network and transport network layersThe radio network layers defines interaction between eNBs The transport network layer provides services for user plane and signaling transport44X2 Application Protocol (X2AP)The X2AP is responsible for providing signaling transport between eNBsSpecification: 3GPP TS 36.423X2AP functions are executed by so called Elementary Procedures Release 8 defines eleven EPs related to different X2AP functions In Release 9 six additional EPs have been added In Release 8/9 limited load management functionality is supportedeICIC is currently under specification for Release 10Function Mobility Management Elementary Procedure(s) a) Handover Preparation b) SN Status Transfer c) UE Context Release d) Handover Cancel a) Load Indication b) Resource Status Reporting Initiation c) Resource Status Reporting Error Indication Reset X2 Setup a) eNB Configuration Update b) Cell Activation Mobility Settings Change a) Radio Link Failure Indication b) Handover Report a) eNB Configuration Update b) Cell ActivationLoad ManagementRelease 8Reporting of General Error Situations Resetting the X2 Setting up the X2 eNB Configuration Update Mobility Parameters Management Mobility Robustness Optimisation Energy Saving5Release 95Coordinated Multipoint in 3GPPNetwork coordination became popular by publications of Bell Labs (Network MIMO)M. Karakayali, G. Foschini and R. Valenzuela, “Network Coordination for spectrally efficient Communications in Cellular Systems”, August 2006, IEEE Wireless Communications Magazine3GPP considered coordination techniques under the name Coordinated Multipoint (CoMP) for Release 103GPP TR 36.814, v9.0.0, March 2010 3GPP Conclusion: CoMP will not be part of the Release 10 specification3GPP Downlink CoMP terminologyJoint Processing Coordinated Multipoint: User data to be transmitted to one terminal is available in multiple sectors of the network. A subclass of joint processing is joint transmission, where the data channel to one terminal is simultaneously transmitted from multiple sectors. Coordinated Scheduling/Beamforming: User data is only available in one sector, the socalled serving cell, but user scheduling and beamforming decisions are made with coordination among the sectors.66Example 1: Centralized Joint TransmissionMaster CellA central master cell manages all resources of the cooperating cellsTransmission Point 1 = Serving Cell = Master CellEach UE reports channel state information (CSI) to its serving cell The serving cell forwards the CSI to the master over the X2 interfaceTransmission Point 1 = Serving Cell Transmission Point 2 = Slave CellThe master distributes scheduling decisions to the transmitting cells over X2 Advantage:Allows optimal scheduling since the master knows the CSI of all UEs Only two X2 usages are requiredDisadvantageCSI needs to be sent over X2 for each active UE77Example 2: Decentralized Coordinated SchedulingGoal: Avoidance of interference in the spatial domain Idea: Identify worst interferer and avoid “collision”Prevent interferers from using “most destructive” precoding matrices Interfering cell can serve UEs on “non-destructive” beamsTypical Coordination Approach1) Active UEs send information about “most destructive” interfering precoding matrices to their serving cellsRESTRICTION REQUEST message in slide 92) The serving cell forwards the received messages to the interfering cells over X2Option: The message is only forwarded if the requesting user is scheduled in order to reduce the number of X2 messages3) Optional: Cells negotiate their beams to improve a utility metricREQUEST GRANT/REJECT messages in slide 988Example of a Coordination Time Line*)UE 1CSI feedbackCell 1Cell 2CSI feedback RESTRICTION REQUESTUE 2UE 3CSI feedbackCell 3RESTRICTION REQUEST Do individual scheduling; check if result contradicts RR Do individual scheduling; check if result contradicts RR REQUEST GRANT/REJECT REQUEST GRANT/REJECT if REQUEST GRANT received from cell 1 conflicts with RG sent to cell 3: compare coordination gain with cell 3 to gain with cell 1 send GRANT REJECT to cell with lower coordination gain (here cell 1) if gain with cell 3 > gain with cell 1, send GRANT REJECT Data transmission Data transmission Data transmission Do individual scheduling; check if result contradicts RRUplinkDownlinkCell-to-cell*) Based on J. Giese, M. A. Awais, “Performance Upper Bounds for Coordinated Beam Selection in LTE-Advanced”, in Proceedings of the ITG/IEE Workshop on Smart Antennas, Bremen (WSA'10), Germany, 23. – 24. February 201099X2 Bandwidth ConsiderationsCentralized Joint Transmission CoMPEstimate of Signaling Load per active User (Slave → Master) Information Content Required Bits Two Cell IDs (ECGI) 56 bits ACK/NACK report 2 x 1 bit (dual stream transmission) 9 bits (subband CQI) CQI/PMI/RI reports (assuming implicit ≤ 11 bits (wideband CQI) (per measured periodic reporting as in LTE Rel8) radio link) Σ ≤ 58 bits + 11 bits/measured radio linkDecentralized Coordinated Scheduling/BeamformingEstimate of Signaling Load for Restriction REQUEST Information Content Required Bits Two Cell IDs (ECGI) 56 bits UE average throughput 16 bits SINR Increase by precoding matrix 16 bits restriction (silencing) Σ ≤ 86 bitsIf coordination takes place each TTI = 1ms, one RESTRICTION REQUEST message requires a bandwidth of 86 kbits/s1010The figures show the number of sent andreceived X2 messages/cell for a coordinated scheduling approach*) With probability of 95%, less or equal than three messages are received per cell The number of received RESTRICTION REQUEST messages scales with theFrequency of X2 Messages 11number of scheduled users in this example Receiving three messages/cell requires a bandwidth of 3 * 86 kbits/s = 258 kbits/sA three sector eNB would therefore require a X2 bandwidth of 3 * 258 kbits/s = 774 kbits/s This assumes 10 UEs/cell and RESTRICTION REQUEST messages targeting 900 kHz and sent at for geometries < -3dB 11*) Based on J. Giese, M. A. Awais, “Performance Upper Bounds for Coordinated Beam Selection in LTE-Advanced”, in Proceedings of the ITG/IEE Workshop on Smart Antennas, Bremen (WSA'10), Germany, 23. –24. February 2010Impact of X2 DelayThe figure shows the throughput loss for a centralized non-coherentJT CoMP approach*)The X2 delay increases the time till the CSI/CQI is available in the MACschedulerConsequently, the CSI information alters and the data rates cannot be12adapted well to the channelThe figure shows the impact of the one-way X2 delayV = 3 km/hRelease 8 CQI delay = 6msThe X2 delay comprises ofa)Interface propagation delay b)eNB Tx/Rx processing delay12*) S. Brueck, L. Zhao, J. Giese, M. A. Awais, “Centralized Scheduling for Joint-Transmission Coordinated Multi-Point in LTE-Advanced”,in Proceedings of the ITG/IEE Workshop on Smart Antennas, Bremen (WSA'10), Germany, 23. –24. February 2010ConclusionsDownlink coordination techniques require extensions of the X2 application protocol (3GPP TS 36.423)In case of centralized coordination, the required X2 bandwidth scales with the number of users in RRC_CONNECTED modeThis number can be in the order of tens to hundredsThe required X2 bandwidth has a large varianceDecentralized coordination techniques allow for a scaling with the number of 13 scheduled usersThis allows reducing the X2 bandwidth significantlyThe drawback is a loss in performance compared to optimal schemesCoordination techniques are delay sensitiveX2 delay comprises of interface propagation delay and eNB processing delay A X2 delay of 5ms caused a loss in spectral efficiency of 20% for the investigated scheme13Thank you!!14Contact: Dr. Stefan BrückTel: 0911 ********sbrueck@14。