Characteristic of retained austenite decomposition during tempering and its effect on impact toughne
DH36钢中的动态应变时效特性

[8]Nemat -Nasser S ,Li Jiangyu.Electromechanical response of ionic polymer -metal composites [J ].Journal of Applie Physics ,2000,87(7):3321-3331.[9]Bonomo C ,Fortuna L ,Giannone P ,et al.A method to charac -terize the deformation of an IPMC sensing membrane [J ].Sen -sors and Actuators A ,2005,123/124:146-154.[10]Shahinpoor M ,Kim K J.Ionic polymer -metal composites :I.fundamentals [J ].Smart Materials and Structures ,2001,10:819-833.[11]金宁,王帮峰,卞侃,等.面粗化工艺对IPMC 的制备及性能影响[J ].功能材料,2008,39(11):1933-1936.DH36钢是一种熔点高、韧性好和可焊接性优良的新型高强度船用钢,其力学行为近些年来已引起研究者的极大兴趣。
其中,DH36钢塑性流动中存在的异于静态“时效”和“PLC 锯齿屈服动态应变时效”的“第3种动态应变时效”现象[1-2]是DH36钢的一个显著特性。
数十年来,研究者对静态应变时效和PLC 锯齿屈服的动态应变时效现象和机理进行了大量的描述和解释,Kocks [3]、Reed-Hill [4]、Kaufman [5]和Cho [6]等人认为,这两种时效现象是由于金属材料在塑性变形的过程中,运动位错和溶质原子相互作用的效果。
钱匡武[7-10]等人指出动态应变时效是金属和合金中移动着的溶质原子(如钢中的C 、N )和运动中的位错发生交互作用时所表现的一种强化现象,而时效对金属疲劳、断裂等现象有很大影响。
UK 22

To harden a steel, rapid cooling (quenching) must normally take place from a temperature above the critical temperature so that the iron carbide is retained in solution.
1/2
Retain: v.t 1.保留;保持;保有;维持 retain an appearance of youth 保有年轻的外貌 retain one‘s presence of mind 镇定自若 2.留住;挡住 retaining wall (防止沙土崩溃的)护堤壁,撑壁 retaining works 拦水工程;蓄水工程
This change is accompanied by a contraction on heating through the critical range and,vice versa, by an expansion on cooling. vice versa:〔拉丁语〕 反过eedle-like crystalline structure called martensite
Martensite is steel in its hardest form. It is very hard, brittle and full of stress.
2/3
1/2
Austenite (gamma iron) crystal structure
Austenite is normally unstable at room temperature. Under certain conditions it is possible to obtain austenite at room 2/2 temperature.
TMSEM__ANALYSIS OF HEAT TREATMENT FOR MARTENSITIC STAINLE

ANALYSIS OF HEAT TREATMENT FOR MARTENSITIC STAINLESSSTEEL USED IN CRDMWei Qiao yuan, Li Yue zhongChina Nuclear Power Engineering Co., Ltd.; TianAn 5#Building; Shen zhen, Guang dong,518172, China Keywords: CRDM; Martensitic Stainless Steel; Heat Treatment; Tempering TemperatureAbstractThe influence of heat treatment on the properties of martensitic stainless steel used in the Control Rod Drive Machine (CRDM) of Pressurized Water Reactor was analyzed. The mechanical properties and magnetic properties of martensitic stainless steel are significantly impacted by the heat treatment, especially the tempering temperature. Taking martensitic stainless steel X12Cr13 and X3CrNiMo13-4 for examples, by comparing different heat treatment rules, the results indicate that the heat treatment can change the ratio of austenite structure contained in the martensitic stainless steel, consequently change the mechanical properties and magnetic properties of the material. The increased ratio of austenite leads to better toughness, lower strength, and lower magnetic properties. It proves that the optimal tempering temperature for martensitic stainless steel X3CrNiMo13-4 is between 560℃ and 600℃ to obtain excellent toughness, and the required magnetic properties can be achieved by taking the tempering temperature close to the lower limit.0 IntroductionPressurized Water Reactor (PWR) is a widely used nuclear reactor type in the world. Control Rod Drive Machine (CRDM) of a PWR plant is used to drive the control rod assemblies stepping in the core. Magnetic lifting type CRDM is used the most widely in PWR and electromagnetic force is used to move Latches. In this kind of CRDM martensitic stainless steel has to be used because of its excellent magnetic properties. Therefore the mechanical properties and magnetic properties of martensitic stainless steel are very important to the function of CRDM and the safety of plant. In CPR1000 nuclear reactor, the pole, armature and drive rod are made up of X12Cr13. In EPR nuclear reactor the latch housing is made up of X3CrNiMo13-4. The mechanical properties and magnetic properties of martensitic stainless steel are significantly impacted by the heat treatment, especially the tempering temperature. This paper takes martensitic stainless steel X12Cr13 and X3CrNiMo13-4 for examples to analyze the influence of heat treatment on the properties of martensitic stainless steel.Energy Materials 2014The Chinese Society for Metals (CSM) and The Minerals, Metals & Materials Society (TMS)TMS, 20141 Heat TreatmentTempering is a heat treatment process in which the quenched steel is heated to a certain temperature, and the temperature is preserved for a planned duration, and then the steel colds down in a proper way. Quenching shall be tracked by tempering which determines the microstructure and performance of steel for use.The purpose of tempering is to reduce or eliminate residual stress and to ensure the completeness of corresponding organization transformation. Meanwhile is can stabilize the size and properties of work piece, as well as to improve the toughness and plastic of the material. In order to meet different functional requirements, different tempering temperatures are selected to obtain appropriate combinations of hardness, strength, ductility and toughness.Martensitic transformation is accomplished by austenitizing heat treatment and quenching. Then the martensitic stainless steel is tempered to form part of finely dispersed stable austenite organization to improve the toughness of the material. During the tempering heat treatment, microstructure of the martensitic stainless steel and performance determined by such microstructure can be adjusted in a wide range.2 Industrial Preparation of Martensitic Stainless Steel2.1 Martensitic Stainless Steel X12Cr13 Used in CPR1000 CRDMThe pole, armature, and drive rod of CPR1000 CRDM are made up of martensitic stainless steel X12Cr13. The manufactures have to choose a proper heat treatment to obtain the properties required in the technical specification. The heat treatment of lift pole, movable gripper pole, and movable gripper armature of LingAo plant, NingDe plant, and YangJiang plant is listed in table 1.Table 1: Comparison of the heat treatment in CPR1000 plantsPlant PartsHeat treatmentInitialTemperatureHeatingRateTemperingtemperatureDuration Cold-down MethodLingAo LP* 100℃64℃/h810℃ 2 hours cold to 650℃ in the furnace and then cold in air to room temperature.MP* 50℃84℃/hMA* 50℃84℃/hNingDe LP* 50℃76℃/hMP* 80℃71℃/hMA* 60℃91℃/hYangJiang LP* 120℃95℃/hMP* 80℃62℃/hMA* 80℃62℃/h*LP-Lift Pole; MP- Movable Gripper Pole; MA-Movable Gripper ArmatureComparing the heat treatments between different plants and different parts, the initial temperature and heating rate are slightly different, but the tempering temperature (810),℃duration (2 hours), and cold-down method (cold to 650 in the furnace and then cold in air℃to room temperature) are all the same. The tempering temperature, duration, and cold-downmethod are the primary factors affecting the properties of martensitic stainless steel.2.2 Martensitic Stainless Steel X3CrNiMo13-4 Used in EPR CRDMIn order to reduce the resistance around the magnetic circuit, the Latch Housing of EPR CRDM is made of martensitic stainless steel X3CrNiMo13-4 (KTA 3201.1) which has been in operation for more than 17 years in German Konvoi plant.The tempering temperature specified by KTA 3201.1 is 560~600℃. The tempering duration is specified at least 8 hours.3 Effect of Heat Treatment on Properties3.1 Effect on Microscopic Structures and Mechanical PropertiesThere are 3 phase transformations during tempering [3]:z carbide precipitation;z recovery of the microstructure (reduction of dislocation density);z formation of stable austenitic organizationThe austenitic organization formed during tempering process must be stable otherwise unstable residual austenite organization will change during the service process and impact the properties of material. If the heat treatment applied by the manufacture of martensitic stainless steel X3CrNiMo13-4 is inappropriate, or the heat treatment of dissimilar welds of EPR CRDM housing is inappropriate, this kind of unstable residual austenite could be produced [3].Influence of tempering temperature on the content of austenite in martensitic stainless steel X3CrNiMo13-4 and characteristic of the material are shown in Figure 1 [4].Figure 1[4]. Influence of Tempering Temperature onMaterial Properties of X3CrNiMo13-4As it can be seen from figure 1 that [4]:up to a tempering temperature of about 450 °C, yieldand tensile strength show a slight increase as a result of carbide precipitation, while impact energy is slowly declining. From 450 °C to 600 °C, a significant drop in strength takes place while the impact toughness is increasing apparently due to a softening of the martensite as well as a coarsening of the precipitations.The toughness shows a maximum value at about 600 °C which is a result of the formation of up to 15 - 20% finely dispersed stable austenite during tempering which does not transform to martensite. Higher tempering temperatures will result in the formation of unstable austenite that transforms to martensite when cooled down which is the cause for a remarkable increases in the materials strength and in turn a significant loss of toughness, and could result in a possible susceptibility to stress corrosion cracking. The heat treatment shall be specified to avoid the formation of unstable austenite.3.2 Effect on Magnetic PropertiesThe magnetic properties of X3CrNiMo13-4 are inversely proportional to the content of stable austenite in microstructure.The influence of the tempering temperature on the magnetization is shown in Figure 2 [5]. Compare to the curve of ratio of austenite in Figure 1, the more austenite contained in the martensitic stainless steel, the lower magnetization of the materiel.Figure 2[5]. Influence of Tempering Temperature on TheMagnetization Behavior of X3CrNiMo13-4Studies have shown saturation polarization and coercive field strength of materials tempered at 530 °C, 560 °C and 600 °C for 8 h [5]. The magnetization curve after tempering at 560 °C is only slightly lower compared to the one of material tempered at 530 °C, but tempering at 600 °C results in a remarkable lower magnetization.Therefore martensitic stainless steel X3CrNiMo13-4 could be tempered between the temperatures of 560°C to 600°C to obtain the highest level of toughness. Well according to the experience of German Konvoi Plant, tempering treatment for the pressure housing material was chosen at the lower bound of the temperature range that is 560 °C, to receive therequired magnetization properties.3.3 Other Factors Could Influence the Magnetic PropertiesMagnetic parameters can be divided into two categories: structure insensitive parameters and structure sensitive parameters. Structure insensitive parameters are only related to composition and crystal structure of materials, and have nothing to do with its microstructure, including saturation magnetization,the Curie temperature,magnetic susceptibility and so on. Structure sensitive parameters are related to the shape of the magnetization curve and magnetic hysteresis loop. That means structure sensitive parameters not only depend on the chemical composition and crystal structure, but also closely relate to the size, shape, orientation of grains and inclusions and stress distribution within the material. The structure sensitive parameters, such as permeability, remanence, and coercive force, change along with different material manufacturing process [5].Structure sensitive parameters are remarkably affected by the second phase precipitation and its shape, size and distribution. So the alloy elements also have a certain influence on the magnetic properties of martensitic stainless steel. The chemical composition of martensitic stainless steel must be strictly controlled to achieve the specified magnetic properties. At the same time, the tempering temperature can also affect the separation and distribution of alloy elements.Magnetic properties are also affected by grain size and inner stress. Smaller grains lead to smaller permeability and bigger coercive force. Because smaller grain size means more grain boundaries which hindrance the movement of magnetic domains. Inner stress will also hinder the movement of magnetic domains which lead to the permeability decline and the coercive force increase. This tendency will aggravate with the increase of grain deformation caused by the inner stress. Both of the grain size and inner stress of martensitic stainless steel under final state are seriously affected by tempering temperature. If the tempering temperature is too high, individual grains increased aberrance. If the tempering temperature is too low, the inner stress eliminated insufficiently which also affect the magnetic properties of materials.4 ConclusionsThe influence of heat treatment on the mechanical properties and magnetic properties of martensitic stainless steel used in CRDM was analyzed, especially the effects of tempering temperature.(1) Tempering temperature changes the mechanical properties and magnetic properties of martensitic stainless steel by changing the ratio of austenite contained in the material. (2) The increased ratio of austenite structure leads to better toughness, lower strength, and lower magnetic properties.(3) Tempering heat treatment must be set carefully on the basis of experience. According to the engineering practice, the optimal temper temperature for martensitic stainless steel X3CrNiMo13-4 is between 560℃ and 600℃ to obtain excellent toughness, and the required magnetic properties can be achieved by taking the tempering temperature close to the lower limit.Reference[1]“Data Sheet on Soft Martensitic Stainless steel X3CrNiMo13-4(1.4313)” (Report,Edelstahlwerke Südwestfahlen GmbH, 2005).[2]Niederau, “State of Development of Soft Martensitic Stainless Chromium-Nickel Steel”(Paper presented at the 119th General Session of the Material Committee of VDEh, Düsseldorf, Germany, 15 December 1977), 129-135.[3]Sun Han, Joining Dissimilar Stainless Steel for Pressure Vessel Components TechnicalResearch Centre of Finland(Finland: VTT Tiedotteita-meddelanden Research Notes, 1550), 24-25.[4]Niederau, “Entwicklungsstand nichtrostender weichmartensitischer Chrom-Nickel-Stähleunter besonderer berücksichtigung des stahles X3CrNiMo13-4,” Stahl und Eisen, 98 (8) (1978), 385-392.[5]Heimann Strom, “Eigenschaften und Gefüge einiger weichmartensitischer nichtrostenderChrom-Nichel-(Molybdän-) Stähle,” Thyssen Edelstahl Technische Berichte, 8 (2) (1982), 115-120.。
HT101
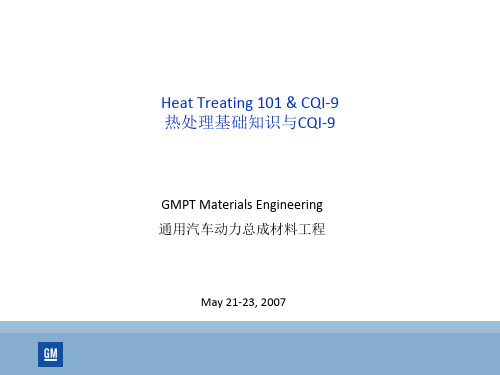
GMPT Materials Engineering Group
9
Heat Treating 101 & CQI-9
Heat Treating of Metallic Materials/金属材料热处理
Principal of heat treating/热处理基本原理: During heating: Formation of Austenite/加热阶段:奧氏体形成 Austenite will be formed from original microstructure of ferrite and pearlite or tempered martensite. /奧氏体由铁素体, 珠光体或回火马氏体转变而成 During cooling: Decomposition of Austenite: CCT diagram /冷却阶段:奧氏体分解-连续冷却转变曲线
GMPT Materials Engineering Group 6
Crystal Structure of phase/晶体结构 Bcc/体心立方晶体 Bcc/体心立方晶体 Fcc/面心立方晶体 Complex orthorhombic / 斜方晶体 Hexagonal /六边形
bct
bct
Heat Treating 101 & CQI-9
3.
CQI-9 (Heat Treat System Assessment)/热 处理系统评估 • What is CQI-9/CQI-9内容 Why is CQI-9 needed/为什么需要CQI-9 • What are CQI-9 requirements/要求 • What heat treatment processes are covered/所涉及的热处理工艺 • What approach should be used in implementing CQI-9/CQI-9实施方法
一种低合金超高强度钢组织与性能的研究

第43卷 第5期 2008年5月钢铁Iron and SteelVo l.43,N o.5M ay 2008一种低合金超高强度钢组织与性能的研究厉 勇1, 王春旭1, 田志凌1, 刘宪民1, 张景海2(1.钢铁研究总院结构材料研究所,北京100081; 2.宝山钢铁股份公司特殊钢分公司,上海200940)摘 要:通过拉伸、冲击和断裂韧性等力学试验方法,光学显微镜、SEM 、T EM 和X RD 等分析测试方法以及热力学平衡计算方法,对一种发动机壳体用低合金超高强度钢(30Cr3)的组织和性能进行了研究。
结果表明:试验钢30Cr 3的室温平衡转变组织为 -F e 和碳化物(包括M C 、M 23C 6、M 7C 3);30Cr 3钢具有很好的淬透性,正火处理后,主要得到马氏体组织;在回火过程中, -碳化物在马氏体板条内弥散析出。
在试验条件下,30Cr 3钢的抗拉强度和屈服强度随着回火温度的升高而逐渐提高,在250 回火时,抗拉强度达到最大值1870M Pa;而随着回火温度的提高,冲击韧性和断裂韧性逐渐降低。
关键词:超高强度钢;组织;回火;力学性能中图分类号:T G 142.7 文献标识码:A 文章编号:0449-749X (2008)05-0075-05Investigation on Microstructure and Mechanical Propertiesof a Low Alloyed Ultra -High Strength SteelLI Yong 1, WAN G Chun -x u 1, T IA N Zh-i ling 1, LIU Xian -min 1, ZH A NG Jing -hai2(1.Institut e fo r Str uctura l M ater ials,Centr al Ir on and Steel Research Inst itute,Beijing 100081,China;2.Special Steel Br anch Special Steel Research &Dev elopment Center,Bao shanIro n &Steel Co.,L td.,Shanghai 200940,China)Abstract:With T hermo -Calc soft war e the equilibr ium phases of a low alloy ed ultr ahigh strengt h steel 30Cr3for en -g ine case w ere studied.By mechanica l test,transmission elect ron micro sco pic(T EM ),scanning electro n micr oscopic (SEM )and XRD o bser vatio n the perfor mance of 30Cr3steel was investigated.T he results sho wed that at roo m temper atur e the st ruct ur e components of 30Cr3steel after equilibrium phase t ransfo rmation ar e -Fe and carbides (M C 、M 23C 6、M 7C 3).30Cr 3steel has g oo d hardenability aft er no rmalization,of which microstr uctur e is mainly mar -tensite.D ur ing temper ing -carbide is pr ecipitated in martensite laths.T he tensio n str eng th and y ield strength o f 30Cr 3steel fir st rise up with a max.T ension strength of 1870M Pa at 250 ,then decline w ith temper ing tempera -tur e;the impact energ y and fracture to ug hness decline w ith increase of tempering temper ature.Key words:ultra -hig h st rength steel;micro st ruct ur e;tempering ;mechanical pr operty作者简介:厉 勇(1980-),男,博士生; E -mail :liysea@; 修订日期:2007-11-20低合金超高强度钢以经济的价格以及高强度水平而得到广泛的应用,这类钢的合金元素总量一般在5%(质量分数)左右,淬火后在200 ~300 的温度范围进行回火处理,靠马氏体相变和回火析出 -碳化物达到超高强度。
S32001双相型不锈钢高温力学性能试验

第50卷第6期2022年6月同济大学学报(自然科学版)
JOURNALOFTONGJIUNIVERSITY(NATURALSCIENCE)
Vol.50No.6
Jun.2022
论文拓展介绍
S32001双相型不锈钢高温力学性能试验楼国彪1,2,杨未1,陈武龙1,陶宇超1,王美南3(1.同济大学土木工程学院,上海200092;2.同济大学土木工程防灾国家实验室,上海200092;3.远大可建科技有限公司,湖南长沙430121)
摘要:对S32001双相型不锈钢进行了高温稳态拉伸试验研究,得到了高温下初始弹性模量、名义屈服强度、抗拉强度、断后伸长率等主要力学性能指标及其变化规律;利用试验数据研究了Rasmussen模型和Gardner模型的适用性,并基于Rasmussen模型提出了S32001不锈钢硬化指数的计算公式,
建立了高温下不锈钢材料本构关系表达式;对比分析了S32001不锈钢与其他种类不锈钢及Q235B结构钢的高温力
学性能。研究表明,S32001不锈钢的屈服强度和极限强度随温度升高下降,600℃时低于常温时的50%,但高温下材料强度明显高于S30408不锈钢,具有更加优越的抗火性能。该研究结果可用于结构受火性能研究和抗火设计。
关键词:双相型不锈钢;力学性能;本构关系;高温中图分类号:TU511.3文献标志码:A
ExperimentalInvestigationonMechanicalPropertiesofS32001DuplexStainlessSteelatElevatedTemperatures
LOUGuobiao1,2,YANGWei1,CHENWulong1,TAO
Yuchao1,WANGMeinan3
(1.CollegeofCivilEngineering,TongjiUniversity,Shanghai200092,China;2.StateKeyLaboratoryforDisasterReductioninCivilEngineering,TongjiUniversity,Shanghai200092,China;3.BroadSustainableBuilding,Changsha430121,Hunan,China)
不同温度下bcc-Fe中螺位错滑移及其与^(12)[111]位错环相互作用行为
![不同温度下bcc-Fe中螺位错滑移及其与^(12)[111]位错环相互作用行为](https://img.taocdn.com/s3/m/d2877531a200a6c30c22590102020740bf1ecd4c.png)
11¯1不同温度下bcc-Fe 中螺位错滑移及其与½[]位错环相互作用行为*王瑾† 贺新福 曹晗 贾丽霞 豆艳坤 杨文(中国原子能科学研究院, 反应堆工程技术研究部, 北京 102413)(2020 年10 月8日收到; 2020 年11 月9日收到修改稿)11¯1¯211¯110¯211采用分子动力学方法模拟研究了不同温度下bcc-Fe 中螺位错滑移行为和螺位错与½[ ]位错环相互作用机制. 结果表明, 螺位错在低温2 K 剪切应力下主要沿( )面滑移; 随温度逐渐升高到823 K, 它容易发生交滑移, 该交滑移在( )和( )面之间交替进行, 因此随温度升高, 临界剪切应力逐渐降低. 当螺位错滑移靠近位错环时, 不同温度下螺位错与位错环相互作用机制不同: 低温2 K 时, 螺位错与位错环之间存在斥力作用, 当螺位错滑移靠近位错环过程中, 螺位错发生交滑移, 切应力比无位错环时有所降低; 中温300 K 和600 K 时, 螺位错与位错环间斥力对螺位错的滑移影响减弱, 螺位错会滑移通过位错环并与之形成螺旋结构, 阻碍螺位错继续滑移, 切应力有所升高; 高温823 K 时, 螺位错因热激活更易发生交滑移, 位错环也会滑移, 两者在整个剪切过程中不接触, 剪切应力最低.关键词:bcc-Fe, 螺位错, 位错环, 分子动力学PACS :87.10.Tf, 87.15.A– DOI: 10.7498/aps.70.202016591 引 言在聚变反应堆中, 核结构材料将遭受恶劣的工作环境(高温、高压和高通量的中子辐照), 由此材料会发生级联碰撞和He 辐照损伤, 经长时间服役,出现肿胀、硬化和脆化等性能恶化[1−3]. 低活化铁素体/马氏体钢(reduced activation ferritic/mar-tensitic steels, RAFM)作为典型的体心立方(bcc)结构Fe 基材料, 因具有抗高热负荷性能, 抗高能粒子辐照性能和较好力学性能成为未来聚变堆候选结构材料. 然而目前RAFM 钢的发展瓶颈为辐照脆化和高温强度不足. 辐照硬化脆化主要归因于位错环、富 Cr 析出物、He 泡等辐照缺陷阻碍位错运动[4−25]. Hardie 等[4]发现 Fe-Cr 合金在辐照下会产生富Cr 析出相, 随后, 文献[5−7]采用分子¯11111¯1动力学方法对富Cr 析出物与位错的相互作用机制及硬化机理进行了研究, 模拟发现富Cr 析出物会阻碍位错运动, 引起剪切应力增大, 且不同析出物尺寸、Cr 含量和切过位置均会对硬化效果产生不同影响. 与之类似, He 泡[8−10]和位错环[11−15]也会阻碍位错滑移进而产生硬化. Terentyev 等[11]发现½[ ]位错环与刃位错之间存在排斥作用, 而当位错环上富集Cr 时则由排斥转为吸引. Rong 等[12]发现½[111]位错环迁移能很低, 容易被刃位错拖拽并随之一起滑移. ½[ ]位错环与刃位错的相互作用与温度(300—900 K)相关[13]: 当温度较高时, 位错环被刃位错拖拽一起运动; 当温度较低时, 位错环会被刃位错线拖拽一段距离后从位错上分离出来. 可见, 温度会影响位错环与刃位错的相互作用机制. 近年来, Liu 和 Biner [14]模拟研究了100—300 K 下½[111]位错环对螺位错滑移行为* 国家自然科学基金(批准号: U1867217)和国家重点研发计划(批准号: 2018YFE0308104)资助的课题.† 通信作者. E-mail: wangjin118114@© 2021 中国物理学会 Chinese Physical Society⟨111⟩11¯1的影响, 结果表明, 当½[111]位错环较小时, 环会发生旋转, 先被螺位错吸收随后又释放出位错环; 当½[111]位错环较大时, 先形成[100]位错片段, 最后½[111]位错环完全转变成[100]位错环.然而高温下½ 位错环对螺位错滑移行为的研究还相对较少. 相对于刃位错而言, 螺位错因较低可移动性, 对材料的塑性变形行为具有更为重要的影响[24,25]. 因此为了充分地理解螺位错在bcc-Fe 结构材料中所扮演的角色, 本文模拟研究了不同温度下螺位错的滑移行为和½[ ]位错环对螺位错滑移的影响, 并详细探讨了温度对位错环和螺位错之间相互作用的影响机制.2 原子模型与方法11¯111¯1112¯11011¯1¯11011¯111¯111¯1为了探究螺位错滑移行为及其与½[ ]位错环相互作用机制, 构建了如图1所示的两种初始构型. 图1(a)是螺位错模型, 图1(b)是含½[ ]位错环的螺位错模型. 这两个模型中x , y , z 轴取向分别为: [ ], [ ], [ ]. 模型尺寸是40.56 nm ×30.28 nm × 14.34 nm (晶格常数a 0 = 2.8553 Å),共包含1528242个原子. 螺位错滑移面法向为[ ], 位错线方向为 [ ], 柏氏矢量方向为 [ ].图1(b)中½[ ]位错环位于螺位错滑移面上, 位错环直径1.5 nm, 距离螺位错12 nm. 建立好模型后, 对上述模型进行能量最小化处理得到稳定构型, 随后进行模拟.采用分子动力学程序Lammps [26]进行模拟,Fe 原子间相互作用势函数的选取来自于文献[27].在整个模拟过程中, x 和y 方向采用自由边界条件,z 方向采用周期性边界条件[15]. 首先, 对初始构型进行能量最小化处理, 得到稳定构型; 随后, 采用NPT 系综, 对模型在一定温度2, 300, 600和823 K 下进行弛豫, 保持温度稳定; 最后, 采用NVT 系综, 对弛豫后的构型进行剪切变形, 沿着z 方向施加应变率为3.0 × 108 s –1, 时间步长为1 fs.⟨111⟩在模拟分析中采用开源可视化工具OVITO (open visualization tool) [28], 用公共近邻分析法CNA (common neighbor analysis)分析原子结构的转变, 位错提取算法DXA (dislocation extraction algorithm)分析不同柏氏矢量的位错. Fe 原子均用蓝色球形原子表示, 错排原子用白色球形原子表示, ½ 位错用绿色线条表示.3 模拟结果与讨论3.1 切应力-应变曲线11¯1图2为不同温度下螺位错及其与½[ ]位错环模型切应力-应变曲线, 图3(a)—(f)分别为2 K 下螺位错在剪切过程中不同应变量下z 截面构型图, 为了便于分析, 模型中均删除bcc 结构的Fe 原子, 仅剩余错排原子(包括界面原子、间隙原子和位错结构原子). 由图2可知, 当温度为2 K 时, 螺位错切应力-应变曲线包含4个阶段, 分别为I, II, III 和IV. 阶段I 为弹性变形阶段, 该阶段内螺位错未发生移动, 模型仅发生弹性变形, 应力Screw dislocation[112]½[111]dislocation loop- [111]- [110]-(a)(b)11¯1图 1 (a)螺位错模型和(b)含½[ ]位错环的螺位错模型11¯1Fig. 1. (a) Model of screw dislocation; (b) model of screw dislocation with ½[ ] dislocation loop./G P a11¯1图 2 不同温度下螺位错及其与½[ ]位错环模型切应力-应变曲线11¯1Fig. 2. Shear stress-strain (t -e ) curves of screw dislocationmodel with and without ½[ ] loop under different tem-peratures.¯21111¯1随应变量增加而线性增加, 应变区间和应力区间分别为e = 0—0.03和t = 0—0.98 GPa, 当e = 0时, 构型如图3(a)所示, 螺位错未滑移, 稳定存在于基体中, 图3(a)右上角为位错结构放大图. 阶段II 为位错滑移启动阶段, 该阶段内应力仍随应变量增大而增大, 但由于位错已准备启动滑移, 因此曲线斜率比阶段I 稍有降低, 应变区间和应力区间分别为e = 0.03—0.048和t = 0.98—1.45 GPa,当e = 0.03时, 螺位错结构发生变化, 准备启动滑移(图3(b)); 当e = 0.048时, 螺位错以扭结对形核[29]并开始滑移(图3(c)). 阶段III 为稳定滑移阶段, 如图3(d)和图3(e)所示, 位错以扭结对形式沿着( )面[15]稳定滑移, 应变区间e = 0.048—0.068, 该阶段内应力出现平台, 平台应力值为1.45 GPa, 不随应变量增大发生变化, 位错划过后在基体内残留下空位, 这些空位以聚集的错排原子显示(图3(e)), 是与文献[30]结论相似. 阶段IV 为位错划出模型阶段, 当e = 0.075时, 位错已经划出模型, 基体中仅剩下残余空位, 模型构型如图3(f)所示, 图3(f)右上角为临近右边界模型x 截面局部放大图, 可见残余空位线性排列, 该阶段剪切应力引起弹性变形, 应力随应变量增加线性增加, 应变区间和应力区间分别为e = 0.068—0.075和t = 1.45—1.52 GPa. 随着温度升高和½[ ]位错环插入, 切应力-应变曲线与单根螺位错模型存在明显不同(见图2), 这表明温度和位错环的存在会显著影响螺位错滑移行为, 下文3.2节和3.3节将详细阐明该滑移行为的差异.3.2 温度对螺位错滑移行为的影响¯211¯110由图2可知, 随温度升高, 螺位错切应力-应变曲线与2 K 时明显不同, 均不存在应力平台阶段. 图4(a)和图4(d), 图4(b)和图4(e), 图4(c)和图4(f)分别为300, 600和823 K 下螺位错在应变量e = 0.03和0.045下的z 截面构型图. 当e =0.03时, 螺位错因温度升高均已发生了滑移, 如图4(a)—(c)所示, 模型基体(尤其是螺位错附近)产生大量错排原子, 600和823 K 下错排原子数目明显比300 K 多, 图右上角为螺位错及其附近原子结构放大图, 这些错排原子会促进螺位错滑移, 因此温度越高, 螺位错滑移应力越低(与图3(b)和图2相对应); 当e = 0.045时, 螺位错均已滑移出模型, 如图4(d)—(f)所示, 详细观察发现, 位错划过后在基体内会残留些许空位, 这些空位排布可用来近似表征位错的滑移痕迹. 与2 K 下模型对比发现, 300, 600和823 K 下位错滑移痕迹存在不同, 这主要归因于螺位错的交滑移行为. 已有研究表明, 螺位错典型滑移面为{112}和{110}[15], 在本文中, 当温度从2 K 升高到823 K 时, 由于温度热激活作用[13−15], 它会沿着( )和( )面发生交替滑移, 因此模型临界剪切应力逐渐降低, 与图2中e = 0—0.045时的切应力-应变曲线变化相对应;(d)(e)(f)Screw dislocationReading to slideKink -pairSliding along (211) plane-Remained vacanciesRemained vacanciesScrew dislocation图 3 随应变量增加的螺位错模型构型图 (a) e = 0; (b) e = 0.03; (c) e = 0.048; (d) e = 0.058; (e) e = 0.068; (f) e = 0.075Fig. 3. Configurations of screw dislocation model with increasing strain: (a) e = 0; (b) e = 0.03; (c) e = 0.048; (d) e = 0.058;(e) e = 0.068; (f) e = 0.075.当e > 0.045时, 300, 600和823 K 下位错均已滑移出模型, 由于交滑移的发生, 位错滑移出模型时距离底端边界的位置明显比2 K 时有所升高(823 K 较为明显), 而应变量有所降低, 这表明温度越高, 位错滑移速度越快, 交滑移越明显.11¯13.3 螺位错与½[ ]位错环相互作用11¯1¯211¯110¯110¯211当模型中存在位错环时, 不同温度下位错环对螺位错滑移行为产生不同影响. 图5(a)—(c)分别为2 K 下含½[ ]位错环的螺位错模型在不同应变量下z 截面构型图. 当e = 0.015时, 螺位错未发生滑移, 螺位错和位错环z 截面结构如图5(a)所示, y 截面结构和位错环结构放大图如右上角所示; 当e = 0.03时, 螺位错准备发生滑移, 位错环发生旋转[31](图5(b)); 当e = 0.06时, 螺位错未滑移通过位错环, 而是从位错环上面滑过并在模型右界面上划出, 位错环完整保留在基体内, 螺位错滑移轨迹上留下残余空位缺陷(图5(c)). 与不含位错环模型相比, 该模型中螺位错滑移轨迹存在不同,这主要是由于当螺位错滑移靠近位错环时, 两者之间的排斥作用[11]导致螺位错在( )面上滑移受阻, 它会沿着( )面滑移, 之后在( )和( )面之间发生交滑移, 因此螺位错滑移出模型时距离底端边界的位置明显升高, 模型切应力明显降低,与图2中切应力-应变曲线相对应.随着温度升高到300 K 和600 K 时, 螺位错¯21111¯1会滑移通过位错环并与位错环发生交互作用, 模型在不同应变量下z 截面构型如图6(a)—(c)和图6(d)—(f)所示. 当e = 0.015时, 由于温度升高,位错环容易翻转, 螺位错也在热激活作用下已经发生了滑移(图6(a)和图6(d)), 此时螺位错与位错环间距离明显比图5(a)近; 当e = 0.03时, 螺位错沿着( )面滑到位错环处, 与之相互作用形成螺旋(helix turn)[14](图6(b)和图6(e)), 该结构阻碍螺位错继续滑动, 因此切应力有所升高(图2); 之后, 螺旋只能沿着螺位错线柏氏矢量方向发生滑移, 当e = 0.045时, 新的½[ ]位错环从螺位错中分离出来(图6(c)和图6(f)), 该新位错环与螺位错柏氏矢量方向一致, 但沿着Z 轴向上滑移了一定距离, 这与文献[14]研究结论一致. 可见, 当温度为300和600 K 时, 螺位错在剪切运动过程中,会与位错环发生交互作用: 一方面, 位错环会阻碍螺位错运动, 起到硬化效果; 另一方面, 螺位错成为位错环快速滑移运动的通道, 促使位错环发生滑移.随着温度进一步升高到823 K, 模型在不同应变量下z 截面构型如图7(a)—(c)所示. 当e =0.015时, 由于温度很高, 位错环更容易发生翻转,螺位错也更容易发生交滑移(图7(a)); 当e = 0.03时, 螺位错继续交滑移, 位错环也因热激活继续翻转, 它们之间没有接触(图7(b)); 当e = 0.045时,位错环沿Z 轴向下滑移了一段距离, 同时螺位错也滑移接近模型右边界(图7(c)). 由此可知, 823 K(d)(e)(f)Remained vacancies Remained vacanciesRemained vacanciesDisorder atoms Moredisorder atomsScrew dislocationhas slidedScrew dislocationhas slidedSlip traceSlip traceSlip traceScrew dislocation has slided图 4 不同温度下螺位错模型在e = 0.03 (a), (b), (c)和0.045 (d), (e), (f)时的构型图 (a), (d) 300 K; (b), (e) 600 K; (c), (f) 823 K Fig. 4. Configurations of screw dislocation model when e = 0.03 (a), (b), (c) and 0.045 (d), (e), (f) under different temperatures:(a), (d) 300 K; (b), (e) 600 K; (c), (f) 823 K.下螺位错始终未滑移通过位错环, 这与2 K 下位错滑移过程相似, 但不同的是, 由于此时温度较高,位错滑移速度增加, 同时螺位错更易发生交滑移,因此螺位错滑移出模型的应变量和切应力与2 K 下模型要低.综上所述, 当模型中存在位错环时, 不同温度下螺位错与位错环相互作用机制可分为三方面: 低温2 K 时, 螺位错与位错环之间存在斥力作用, 当螺位错滑移靠近位错环时, 螺位错发生交滑移, 未通过位错环, 因此位错环没有产生阻碍作用, 剪切应力比纯螺位错模型要低; 中温300和600 K 时,位错环容易翻转, 螺位错与位错环间斥力对螺位错Remained vacanciesDislocation loopDislocation loop Dislocation loopScrew dislocation图 5 2 K 下随应变量增加含位错环的螺位错模型构型图 (a) e = 0.015; (b) e = 0.03; (c) e = 0.06Fig. 5. Configurations of screw dislocation model with dislocation loop with increasing strain at 2 K: (a) e = 0.015; (b) e = 0.03;(c) e = 0.06.(a)(b)(c)(d)(e)(f)Dislocation loopflippedDislocation loopflippedScrew dislocation has slided Screw dislocationhas slidedHelix turnHelix turnNew dislocation loopSliding alongaxisNew dislocation loopSliding alongaxis图 6 不同温度下含位错环的螺位错模型在 e = 0.015 (a), (d), 0.03 (b), (e)和0.045 (c), (f)时构型图 (a), (b), (c) 300 K;(d), (e), (f) 600 KFig. 6. Configurations of screw dislocation model with dislocation loop when e = 0.015 (a), (d), 0.03 (b), (e), and 0.045 (c), (f) un-der different temperatures: (a), (b), (c) 300 K; (d), (e), (f) 600 K.(a)(b)(c)Dislocation loop has flippedScrew dislocationhas slidedGo on flippingInitial dislocation loop Sliding along axis图 7 823 K 下随应变量增加含位错环的螺位错模型构型图 (a) e = 0.015; (b) e = 0.03; (c) e = 0.045Fig. 7. Configurations of screw dislocation model with dislocation loop with increasing strain at 823 K: (a) e = 0.015; (b) e = 0.03;(c) e = 0.045.滑移影响减弱, 两者靠近时螺位错滑移通过位错环, 螺位错继续滑移受位错环所阻碍, 因此剪切应力有所升高; 高温823 K 时, 斥力影响更弱, 位错环更容易翻转和滑移, 螺位错也更容易发生交滑移, 该温度下螺位错交滑移行为占主导, 在整个滑移过程中始终未通过位错环, 因此位错环没有产生阻碍作用, 剪切应力与纯螺位错模型差距不大.4 结 论在前人研究螺位错滑移行为的基础上, 本文进一步揭示了不同温度下螺位错的滑移行为, 详细探讨了位错环缺陷与螺位错的相互作用机制, 结论归结如下:¯211¯110¯2111) 当温度为2 K 时, 单根螺位错在剪切应力下沿着( )面滑移, 临界剪切应力为1.45 GPa;随温度逐渐升高到823 K, 切应力逐渐降低, 螺位错易在( )和( )面发生交滑移, 其中823 K 下交滑移行为较明显.2) 低温2 K 时, 螺位错易发生交滑移, 螺位错在整个滑移运动过程中未通过位错环, 切应力低于纯螺位错模型.3) 中温300 K 和600 K 时, 螺位错滑移通过位错环, 并与之相互作用形成螺旋结构, 阻碍螺位错继续滑移, 切应力高于纯螺位错模型.4) 高温823 K 时, 螺位错更易发生交滑移, 位错环也更容易翻转和滑移, 螺位错在整个滑移运动过程中始终未通过位错环, 切应力与纯螺位错模型差距不大.⟨111⟩⟨100⟩该研究对服役过程中核结构材料的塑性变形行为有一定理论指导意义, 但是由于结构材料基体内不仅存在 位错环, 还有 位错环, 而且不同类型位错环拥有不同柏氏矢量, 因此本文只是研究了位错环一隅, 为了完整全面地阐明位错环与螺位错相互作用机制, 还需进一步研究.参考文献U llmaier H 1984 Nucl. Fusion 24 1039[1]S okolov M A, Tanigawa H, Odette G R, Shiba K, Klueh R L2007 J. Nucl. Mater. 367 68[2]D ai Y, Long B, Tong Z F 2008 J. Nucl. Mater. 377 115[3]H ardie C D, Williams C A, Xu S, Roberts S G 2013 J. Nucl.Mater. 439 33[4]S uganuma K, Kayano H 1983 J. Nucl. Mater. 118 234[5]T erentyev D, Haghighat S M H, Schaublin R 2010 J. Appl.Phys. 107 55[6]J ia L X, He X F, Dou Y K, Wu S, Wang D J, Yang Wen2017 Nuclear Power Engineering 38 115 (in Chinese) [贾丽霞,贺新福, 豆艳坤, 吴石, 王东杰, 杨文 2017 核动力工程 38 115][7]W ang Y X, Xu Q, Yoshiie T, Pan Z Y 2008 J. Nucl. Mater.376 133[8]O setsky Y N, Stoller R E 2015 J. Nucl. Mater. 465 448[9]Y ang L, Zhu Z Q, Peng S M, Long X G, Zhou X S, Zu X T,Heinisch H L, Kurtz R J, Gao F 2013 J. Nucl. Mater. 441 6[10]T erentyev D, Bergner F, Osetsky Y 2013 Acta Mater. 61 1444[11]R ong Z, Osetsky Y N, Bacon D J 2005 Philos. Mag. 85 1473[12]J ia L X, He X F, Dou Y K, Wang D J, Wu S, Cao H, YangW 2019 Nucl. Instrum. Methods Phys. Res. 456 103[13]L iu X Y, Biner S B 2008 Scripta Mater. 59 51[14]H ale L M, Zimmerman J A, Weinberger C R 2014 Comput.Mater. Sci. 90 106[15]Y ang L, Gao F, Kurtz R J, Zu X T 2015 Acta Mater. 82 275[16]Z hang L, Fu C C, Hayward E, Lu G H 2015 J. Nucl. Mater.459 247[17]M artinez E, Schwen D, Caro A 2015 Acta Mater. 84 208[18]Z hurkin E E, Terentyev D, Hou M, Malerba L, Bonny G 2011J. Nucl. Mater. 417 1082[19]W akai E, Hishinuma A, Kato Y, Yano H, Takaki S, Abiko K1995 J. Phys. IV France 5 C7-277[20]X u H X, Stoller R E, Osetsky Y N, Terentyev D 2013 Phys.Rev. Lett. 110 265503[21]T erentyev D, Bacon D J, Osetsky Y N 2010 Philos. Mag. 901019[22]P ascale E T, Shehadeh M A 2018 Int. J. Plasticity 9 2[23]S ong G, Lee S W 2019 Comput. Mater. Sci. 168 172[24]X ia Z Y, Zhang Z J, Yan J X, Yang J B, Zhang Z F 2020Comput. Mater. Sci. 174 109503[25]L AMMPS Molecular Dynamics Simulator http://lammps./ [2020-10-7][26]C aro A, Hetherly J, Stukowski A, Caro M, Martinez E,Srivilliputhur S, Zepeda-Ruiz L, Nastasi M 2011 J. Nucl.Mater. 418 261[27]S tukowski A 2010 Modell. Simul. Mater. Sci. Eng. 18 015012[28]G ordon P A, Neeraj T, Li Y, Li J 2010 Modell. Simul. Mater.Sci. Eng. 18 085008[29]J aime M, Wei C, Vasily V B 2004 Nature Mater. 3 158[30]B acon D J, Osetsky Y N, Rong Z 2006 Philos. Mag. 86 3921[31]11¯1Screw dislocation slip and its interaction with ½[]dislocation loop in bcc-Fe at different temperatures *Wang Jin † He Xin -Fu Cao Han Jia Li -Xia Dou Yan -Kun Yang Wen(Reactor Engineering Technology Research Department , China Institute of Atomic Energy , Beijing 102413, China )( Received 8 October 2020; revised manuscript received 9 November 2020 )Abstract11¯1¯211¯110¯211Reduced activation ferritic/martensitic (RAFM) steel, as a typical body centered cubic (bcc) iron based structure material, has become a candidate material for future fusion reactor. Nano-scale prismatic interstitial dislocation loops formed in irradiated RAFM have been studied for many years because of their significant influences on the mechanical properties (e.g. irradiation embrittlement, hardening, creep, etc.). Compared with edge dislocation, screw dislocation has very important influence on plastic deformation behavior because of its low mobility. Thus, the mechanism of interaction between screw dislocation and interstitial dislocation loops has become an intense research topic of interest. In this study, the slip behavior of screw dislocation and the mechanisms of interaction between screw dislocation and ½[ ] dislocation loop in bcc-Fe at different temperatures are investigated by molecular dynamics simulation. The results show that the screw dislocation mainly slides along the ( ) plane at a low temperature of 2 K under the increase of shear stress. With the temperature increasing to 823 K, it is prone to cross slip, and then the cross slip occurs alternately in the ( ) plane and the ( ) plane. Therefore, with the increase of temperature, the critical shear stress decreases gradually. When the screw dislocation slips close to the dislocation loop, the mechanism of interaction between screw dislocation and dislocation loop is different at different temperature: at low temperature of 2 K, there is repulsive force between screw dislocation and dislocation loop, when screw dislocation slip approaches to the dislocation loop, the cross slip of screw dislocation can occur, and shear stress is lower than that from the model without dislocation loop; at medium temperatures of 300 K and 600 K, the influence of repulsive force on the cross slip of screw dislocation can be weakened, and screw dislocation will slip through the dislocation loop then form the new structure named helix turn, which further hinders screw dislocation slipping and results in the increase of shear stress; at a high temperature of 823 K, the screw dislocation is more likely to cross slip due to the thermal activation, and the slip of dislocation loop is also easier to occur, but the screw dislocation and the dislocation loop do not contact each other in the whole shearing process, therefore the shear stress is lowest.Keywords: bcc-Fe, screw dislocation, dislocation loop, molecular dynamicsPACS: 87.10.Tf, 87.15.A – DOI: 10.7498/aps.70.20201659* Project supported by the National Natural Science Foundation of China (Grant No. U1867217) and the National Key Research and Development Program of China (Grant No. 2018YFE0308104).† Corresponding author. E-mail: wangjin118114@。
高铬铸铁轧辊热处理工艺研究
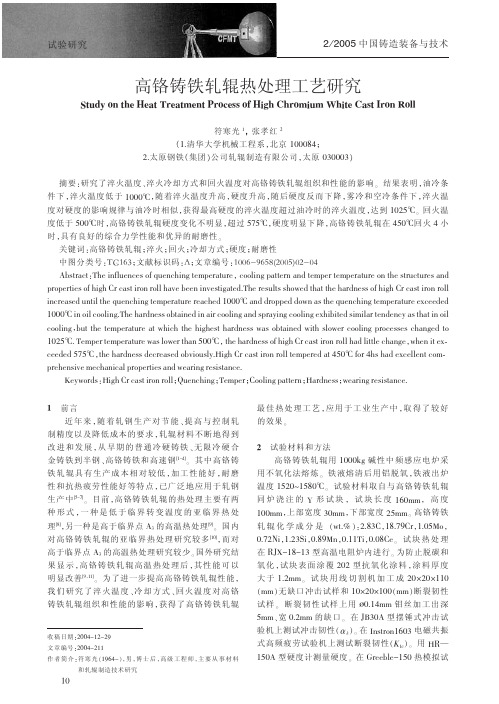
"
试验材料和方法 高铬铸铁轧辊用 "---./ 碱性中频感应电炉采
# 其中高铬铸
铁 轧 辊 具 有 生 产 成 本 相 对 较 低!加 工 性 能 好 !耐 磨 性和抗热疲劳性能好等特点 ! 已广泛地应用于轧钢 生产中
()% !&#’%
用不氧化法熔炼 $ 铁液熔清后用铝脱氧 ! 铁液出炉 温度 "&0-1"&)-2 $ 试验材料取自与高铬铸铁轧辊 同 炉 浇 注 的 3 形 试 块 ! 试 块 长 度 "4-55 ! 高 度
全 ! 碳化物尚 未长大 ! 细小 且均匀弥散 的分布铬铸铁轧辊 耐磨性的影响 ! 保温 #% "
氏体基体 上 ! 不 利 于 裂 纹 的 扩 展 ! 故 ! ) 值 和 !!* 值 较高 " 当温度高于 ,$"% 以后 ! 由于碳化物的聚集长 性 !又不易出现裂纹 !应用于实际生产中是可行的 " 大使其弥散度减小 -!,.! 导致 ! ) 值和 !!* 值有所降低 "
$ 为了进一步提高高铬铸铁轧辊性能 !
我 们 研 究 了 淬 火 温 度 %冷 却 方 式 "回 火 温 度 对 高 铬 铸铁轧辊组织和性能的影响 ! 获得了高铬铸铁轧辊
&55 % 宽 -8055 的缺口 # 在 EI+-* 型摆锤式冲击试
收稿日期 "0--$G"0G0, 文章编号 "0--$G0"" 作 者 简 介 " 符 寒 光 !",4$G "# 男 # 博 士 后 # 高 级 工 程 师 # 主 要 从 事 材 料 和轧辊制造技术研究
.@-
值较小 " 表明高铬铸铁轧辊具有较好的淬透性 %