AMESim热气动库资料
LMS_Imagine.Lab_AMESim_HVAC2nd 混合气体库建模
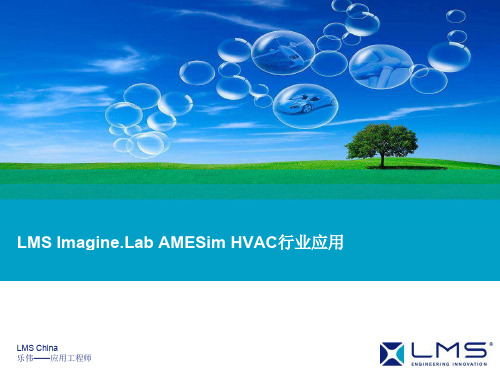
湿空气建模的能力
混合气体库+湿空气库 描述气体中的水 & 液相
特点
优点
用绝对/相对湿度/比湿度来定义湿度 冷凝/蒸发 可对多组分混合气体建模 :
(N2,O2,CO2,H2O,CH4…) 混合物组份可随时间和流程变化
可以对包含空气、水蒸气和液态水的系统
进行建模 可对动态时域的仿真过程中的湿度标定 进行评估 可对气/液回路进行建模
9 copyright LMS International - 2006
LMS b AMESim 热库
LMS b AMESim的电机及驱动库,动力
传动库中有很多部件都带有热端口,可以考虑温 度带来的非线性影响。 耦合LMS b热库,可以考虑部件与外 界热交换,造成的温度的变化。从而可以考察工 作状态下,温度对与系统或部件工作特性的影响。
14 copyright LMS International - 2006
AMESim平台及其热液压库提供了强大
LMS b AMESim 热液阻库
AMESim热液阻库通过一整套功能
热液阻库模型:
直管 节流 突然扩大管/突然缩小管 渐扩管/渐缩管 30°,45°,60°,90°弯管 通用弯管 90°,45°T型分叉管 交叉管:90°,180°, 3通 交叉管:90°, 2通, 轴交叉或不交叉 管接头:尖角进口 管接头:圆角进口 管接头:延伸进口 管接头:90°锥形进口 管接头:60°锥形进口 考虑离心作用的液压管道 圆柱环形液压管道 轴套带刻槽的圆柱环形液压管道 静压轴承和动压轴承 自定义轴承 静压传感器 离心泵和体积泵
LMS b Optimus
主要特点
通过实验规划和响应面建模技术快速探索 设计空间 找到最好的一组设计变量以满足所有的约 束条件并为给定的目标函数提供最优的值 找出由于加工误差、不同工况、磨损等原 因造成的参数偏差对性能的影响
AMESIM介绍资料讲解
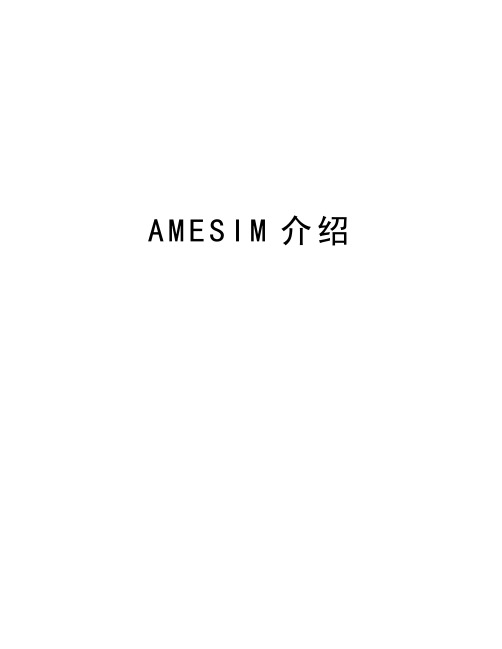
A M E S I M介绍第二章AMESim的应用方法2.1 AMESim简介AMESim表示系统工程高级建模和仿真平台(Advanced Modeling Environment for Simulations of engineering systems)。
它能够从元件设计出发,可以考虑摩擦、油液、和气体的本身特性、环境温度等非常难以建模的部分,直到组成部件和系统进行功能性能仿真和优化,并能够联合其他优秀软件进行联合仿真和优化,还可以考虑控制器在环构成闭环系统进行仿真,使设计出的产品完全满足实际应用环境的要求。
AMESim软件共由四个功能模块组成:AMESim、AMESet、AMECustom、AMERun,另外还有软件帮助模块AMEHelp。
其中,AMESim用于面向对象的系统建模、参数设置、仿真运行和结果分析,是该工具软件的主功能模块,主要工作模式为:按系统原理图建模一确定元件子模型一设定元件参数一仿真运行一结果观测和分析。
AMEest用于构建符合用户个人需求的元件子模型,主要通过两步进行:先设定子模型外部参数情况,系统自动生成元件代码框架,再通过用户的算法编程实现满足用户需要的元件,程序使用C或Fortnar77实现;AMECustom用于对软件提供的元件库中的元件进行改造,但不能深入到元件代码层次,只适用于元件的外部参数特性的改造;AMERun是提供给最终用户的只运行模块,最终用户可以修改模型的参数和仿真参数,执行稳态或动态仿真,输出结果图形和分析仿真结果,但不能够修改模型结构,不能够访问或修改元件代码等涉及技术敏感性的信息。
2.2AMESim的特点1.多学科的建模平台AMESim在统一的平台上实现了多学科领域的系统工程的建模和仿真,模型库丰富,涵盖了机械、液压、控制、液压管路、液压元件设计、液压阻力、气动、热流体、冷却、动力传动等领域,且采用易于识别的标准ISO图标和简单直观的多端口框图,方便用户建立复杂系统及用户所需的特定应用实例。
AMESim热气动库资料
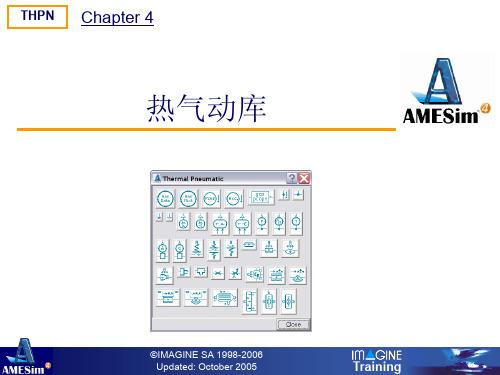
dt
dt
©IMAGINE SA 1998-2006
Training
16
阻性元件
17
计算质量流量(可压)
Pu
Pd
Tu
Td
m
m
dmh
dmh
Flow
m ACqCm Pu Tu
2
Cm r1
2
1
Pd Pu
Pd Pu
Pd
Pu
Pcr
(subsonic
)
1
1
r
211
r21 211
Pd Pu
Training
2
热气动库
3
库图标
热气动库元件:
©IMAGINE SA 1998-2006
Training
3
主要元件
气体热力学属性: 容性元件(绝热或传热): 阻性元件(压力损失): 容性 – 阻性元件(绝热或传热)
摩擦 + 可压 + 对流
©IMAGINE SA 1998-2006
4
©IMAGINE SA 1998-2006
Training
9
气体热力学属性- 混合气
10
通过‘Gas Mixt.’图标可以定义混合气
通过气体质量组分定义混合气组成: 例如空气
©IMAGINE SA 1998-2006
Training
10
气体热力学属性- 混合气
11
空气组成(% 体积比 = 摩尔组分):
©IMAGINE SA 1998-2006
Training
15
容性元件
16
压力为状态变量,通过理想气体状态方程计算:
dp 1 [
AMESimHCD库的使用4

图37图38默认值,流通面积为0时的开口量为0,然后随着正开口量线性增加。
第一个参数是开口量的下限,可能是由泄漏或是一些固定的小孔口导致的;第二个参数是开口量的上限,可能是由于环形孔口经过某个孔,或是如图39所示,阀芯的移动造成环形孔口的全部打开。
图39图40是这两个阀芯子模型的相关参数。
注意对应于最小面积的开口量没有出现在这里,是因为模型的泄漏已经通过缝隙和圆形边界明确的计算出来了。
将子模型BAO011换成BAO013,然后再运行仿真。
会发现再阀芯处于中间位置时,负载孔口存在一个小流量。
这里从P到T液一直有少量泄漏。
试着增大缝隙和圆形边界的半径,然后会发现增大这些参数会增大泄漏量。
图40这个例子的模型将所用问题都高度细化。
对阀门的设计者来说是非常适用的,但对于更多的使用者来说,更简单的方法要更合适一些。
然而许多情况下,阀的动态特性及其控制系统近似为二阶传递函数,通过软件中提供的数据来定义这些参数。
图41就是这样一个高度简化的系统。
动态特性由一个二阶滞后提供,包括自然频率和阻尼系数;位移限制由一个饱和元件来实现;位移的最终值是不同的,并产生一个相应得速度;其他需要用来计算的量是子模型BAO011和BAO012空间上的相关数据。
图412.5 带移动缸体的液压缸通常得液压缸体是固定不动的或可假定为静止。
然而,在其他情况下或是想得到更符合实际的结果就有必要将缸体得运动考虑在内。
HCD中的相对运动子模型可以实现这一要求。
可以用移动缸体来构建一个液压缸模型,并与固定缸进行比较。
系统如图42所示。
注意:在HCD中,不要把相对运动的子模型和绝对运动得子模型相混淆。
相对运动子模型在库中的标记处于下方,而绝对运动子模型的标记则在上方。
HCD中相对运动的子模型的终点挡板都是弹性的。
是因为在撞击中,两个质量块是有限定的,有必要得到之间的撞击力。
液压腔连有两端口液压节点,作为HCD液压流动端口与标准AMESim液压流动端口的分界。
amesim培训资料发动机01_ENG2_part3

0.99*Q0
t
P km
Q0 (1 e m
t0
P0
)
0.632*Q0
0
t0
2.3 * t0
注意: 本地时间从SOI开始
©IMAGINE SA 1998-2006 Updated: July 2006
20
ENGCYLH21
ENGCYLH22
ENGCYLH23
©IMAGINE SA 1998-2006 Updated: July 2006
Training
气门流动
1: Simple model简单模式
有效流动面积由阀门最大升程等效直径, 流动系数与最大升程计算得 到:
21
A eff lift
13
数据非常难以得到…
…但是默认参数在大部分情况下是可靠的 通过试验可以进行修正
气体温度Tgas 影响蒸发
©IMAGINE SA 1998-2006 Updated: July 2006
Training
非直喷
ENGCHW ET01
14
喷油器端口 (热液压端口)
©IMAGINE SA 1998-2006 Updated: July 2006
有效流通面积为阀门升程的函数,由试验或CFD计算得到. 结果保存 在ASCII文件中。或者采用近似的数学表达式描述. 同样可以区分正流 和逆流.
23
©IMAGINE SA 1998-2006 Updated: July 2006
Training
可变气门正时
V.V.T.
由ECU信号或map图确定可变正时
AMEsim学习文件
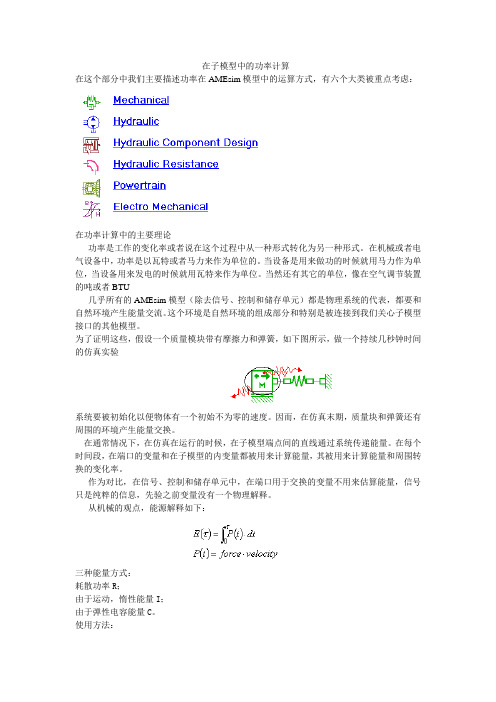
在子模型中的功率计算在这个部分中我们主要描述功率在AMEsim模型中的运算方式,有六个大类被重点考虑:在功率计算中的主要理论功率是工作的变化率或者说在这个过程中从一种形式转化为另一种形式。
在机械或者电气设备中,功率是以瓦特或者马力来作为单位的。
当设备是用来做功的时候就用马力作为单位,当设备用来发电的时候就用瓦特来作为单位。
当然还有其它的单位,像在空气调节装置的吨或者BTU几乎所有的AMEsim模型(除去信号、控制和储存单元)都是物理系统的代表,都要和自然环境产生能量交流。
这个环境是自然环境的组成部分和特别是被连接到我们关心子模型接口的其他模型。
为了证明这些,假设一个质量模块带有摩擦力和弹簧,如下图所示,做一个持续几秒钟时间的仿真实验系统要被初始化以便物体有一个初始不为零的速度。
因而,在仿真末期,质量块和弹簧还有周围的环境产生能量交换。
在通常情况下,在仿真在运行的时候,在子模型端点间的直线通过系统传递能量。
在每个时间段,在端口的变量和在子模型的内变量都被用来计算能量,其被用来计算能量和周围转换的变化率。
作为对比,在信号、控制和储存单元中,在端口用于交换的变量不用来估算能量,信号只是纯粹的信息,先验之前变量没有一个物理解释。
从机械的观点,能源解释如下:三种能量方式:耗散功率R;由于运动,惰性能量I;由于弹性电容能量C。
使用方法:计算方法只有在AMEsim中才能使用。
为了在仿真结果中有能量交换,有必要在'Run Parameters'选项框中检查the 'Activity index calculation' option in the 'Standard options。
在机械库中能量计算目的在AMEsim模型中,计算和周围能量的转换(相连的子模型和环境)变的可能,下面的文档就告诉我们能量是怎么在机械库模块中被计算的。
涉及到在AMEsim中能量计算理论,用于活性索引全局观点。
AMESim热气动库资料
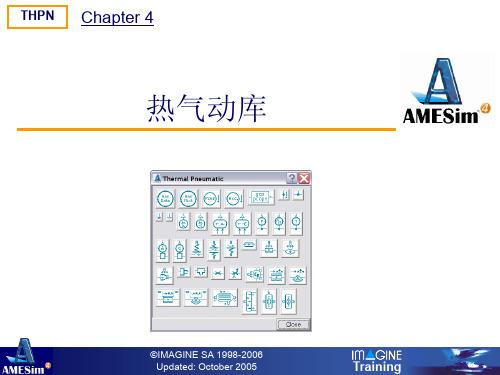
©IMAGINE SA 1998-2006
Training
19
©IMAGINE SA 1998-2006
Training
9
气体热力学属性- 混合气
10
通过‘Gas Mixt.’图标可以定义混合气
通过气体质量组分定义混合气组成: 例如空气
©IMAGINE SA 1998-2006
Training
10
气体热力学属性- 混合气
11
空气组成(% 体积比 = 摩尔组分):
6
其中: T=T-Tref
Training
6
气体热力学属性
7
AMESim内置22种 TPGD1子模型气体 属性
©IMAGINE SA 1998-2006
Training
7
气体热力学属性
8
气体属性按如下格式定义:
©IMAGINE SA 1998-2006
Training
8
气体热力学属性
9
AMESim提供了gasgen.exe用于计算多 项式系数 :
A
.01* 40 28.96
.014
©IMAGINE SA 1998-2006
Training
11
气体热力学属性- 混合气
12
定义空气为混合 气ቤተ መጻሕፍቲ ባይዱ分:
©IMAGINE SA 1998-2006
Training
12
气体热力学属性
13
热气动库包含图标:
©IMAGINE SA 1998-2006
Training
©IMAGINE SA 1998-2006
Training
15
容性元件
16
AMESim的热库
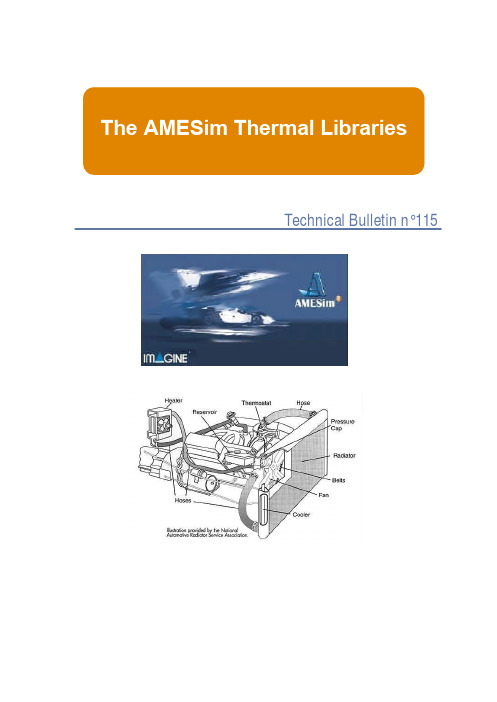
Technical Bulletin n°115How to contact IMAGINE:North America imagine-us@Europe imagine@Asia imagine-japan@Visit for further contact information and details on other countries.Copyright © IMAGINE S.A. 1995-2002AMESim® and AMESet® are the registered trademark of IMAGINE SA.All other product names are trademarks or registered trademarks of their respective companiesLatest update: Feb. 26th, 2002 –The AMESim Thermal Libraries 3/17 The AMESim Thermal Libraries Following the increasing demands for thermal-hydraulic system performance, IMAGINE has created, the new AMESim® Thermal, Thermal-hydraulic and Cooling System Libraries.1. The Thermal libraryIn this category all the components have thermal ports only and the variables passed at these ports are:Ä Temperature [degC]Ä Heat flow rate [W]Figure 1: The AMESim® Thermal Library –The AMESim Thermal Libraries 4/17 –The thermal components allow you to predict temperature transient phenomena in solid masses by using conduction, free and forced convection with the environment and radiation heat transfers.Heat transfer components can use: Ä analytical formulaeÄ empirical datato prescribe heat flow. For empirical data, this would be defined by signal input.Figure 2: convective exchangeFigure 3: radiative exchangeFor example, in an engine cylinder, the convective and the radiative heat transfer coefficient can be supplied by empirical data, which are function of the engine speed and the effective mean pressure.A combination of conductance and mass components enables you to model complex temperature and heat flow boundary conditions.Figure 4: temperature boundaryFigure 5: heat flow boundaryconditionconditionThe AMESim Thermal Libraries5/17 –The following table presents these thermal components:Three ports thermal nodeThermal temperature source Signal to temperature units Conversion between thermal variables and signal variables Thermal temperature transducer Thermal conduction Thermal convection Thermal radiation between two bodiesThermal radiation1D conductionTable 1: Models of the Thermal Library2. The Thermal-hydraulic LibraryThe AMESim ® Thermal-hydraulic library comprises a set of basic components from which, it is easy to model large thermal-hydraulic networks and develop the design of the systems studied.The AMESim Thermal Libraries 6/17 In this category, the components have thermal and thermal-hydraulic ports. The variables used on thermal-hydraulic ports are:Ä Absolute pressure [barA]Ä Temperature [degC]Ä Mass flow rate [kg/s]Ä Heat flow rate [W]Figure 6: AMESim® Thermal-hydraulic Library - Thermal-hydraulic categoryBy using the Thermal-hydraulic category it is possible to predict pressures, fluid flow rates, temperatures and enthalpy flow rates in a hydraulic system taking into account heat exchanges with environment.This category offers a set of components allowing you to model systems containing: Ä Thermal-hydraulic resistance components: these are orifices, expansions/ contractions, diffusers, annular pipes, bends, T-junctions… –The AMESim Thermal Libraries 7/17 –Ä Thermal-hydraulic pipe/hose components: these are adiabatic pipes/hoses and pipes/hoses with external heat exchanged. For hose models the pressure is computed taking into account the hose wall expansion. Also, in the thermal-hydraulic resistance and pipes/hoses models, the increase of fluid temperature due to the pressure drop is also taken into account. Ä Thermal-hydraulic accumulator components: these contain a constant mass of gas or a pneumatic port compatible with AMESim ® Pneumatic Library . Ä Thermal-hydraulic pump components: these are v olumetric pumps and centrifugal pumps. The temperature of the fluid flowing out of the pump is computed using an overall fractional efficiency parameter defined by the user. Ä Thermal-hydraulic transducers: these are pressure, temperature and fluid flow rate transducers. They are used to convert thermal-hydraulic variables into signals from which it is possible to control or pilot components, for example, it is possible to control the radiator's fan operating sequence as a function of the coolant temperature at radiator's outlet, or even by observing the volumetric flow rate between any thermal-hydraulic connection. Ä Multiple thermal-fluids: In AMESim ® Thermal-hydraulic Library , the fluid properties are evaluated at working pressure and temperature. A fluid i s defined in the AMESim ® Thermal-hydraulic Library by a special icon. By using several of these special icons on the sketch, the user can easily model systems containing different fluids. For example, it is possible to model on the same sketch an engine cooling circuit coupled with the lubricating circuit and study the heat exchanges between them.The following table presents the thermal components:The AMESim Thermal Libraries 8/17 –press-hydra ulicpipeBendThe AMESim Thermal Libraries 9/17 –--The AMESim Thermal Libraries 10/173. The Cooling System LibraryThe AMESim® Cooling System Library is a set of specific components fully compatible with AMESim® Thermal-hydraulic Library. It contains components from which complete engine cooling systems can be built.Figure 7: AMESim® Cooling System LibraryThese components combined with AMESim® Thermal and Thermal-hydraulic Library enable engineers to model dynamic behavior of engine system. For example you can study: –The AMESim Thermal Libraries 11/17 Ä The performance of a cooling system with a complex thermostat in which hysteresis and the thermal inertia of wax are taken into account.Ä Prediction of the temperature and the pressure at the pump inlet allowing you to investigate pump cavitation.Ä The time spent before an engine reaches its operating temperature after a cold starThe AMESim® Cooling System Library contains two types of components:1. Data components- with these components the user can specify all thecontrolling variables concerning the profiles adopted and the vehicle data.Ambient temperature and pressure –The AMESim Thermal Libraries 12/17 –ImmThe AMESim Thermal Libraries 13/17 Various radiator submodels exist to model the two different kinds of systems described before:Ä Vehicle simulation: the air velocity through the radiator is computed as a function of the vehicle velocity by using the radiator and condenser aerodynamics coefficients and the fan characteristic.Ä Test bench simulation: the air velocity through the radiator system is controlled by the user.4. ExampleThe system below shows a complete engine cooling system. It is built using both AMESim® Thermal-hydraulic Library and AMESim® Cooling System Library components:Figure 8: Complete engine cooling system sketchThe graphic below shows the duty profile for a test run used in the system: –The AMESim Thermal Libraries 14/17 –Figure 9: mission profileThe following figure is a zoom of this profile. It shows the engine speed variation as a function of the car velocity and the gearbox ratio:Figure 10: engine speed, car velocity and gearbox ratio as a function of timeThe AMESim Thermal Libraries 15/17 –With radiator and condenser aerodynamics coefficients and the fan characteristics, it is possible to compute the air mass flow rate as a function of the car velocity which is a control variable:Figure 11: air mass flow rate and fan operatingThe following figure shows temperature, pressure and coolant flow rate in specified parts of the system and the thermostat fractional area:Figure 12: temperature and pressuresThe AMESim Thermal Libraries 16/17 –Figure 13: coolant flow rate and thermostat fractional area5. Other applicationsBy using the standard AMESim ® Cooling System Library the user can predict the coolant temperature due to the heat transfer between engine and coolant as a function of the engine speed and the engine load. However, a higher level of detail can be adopted by using the convective and radiative transfer, thermal mass and conductance components of the thermal category. These components allow you to predict the transient engine mass temperature for a cold start, taking into account the heat transfer between combustion gas and cylinder, combustion gas and piston, the heat transfer through the cylinder and piston and the convective heat transfer between the engine mass and coolant.The thermal-hydraulic resistance components enable you to construct the engine oil system. Moreover, its interaction with the coolant system can be modeled by using both the oil-coolant heat exchanger from the AMESim ® Cooling System Library and the convective heat transfer oil/engine mass and coolant/engine mass.6. ExtensionsA new AMESim ® Pneumatic Library has been developed to enable you to model exhaust gas recirculation and its interaction with the engine temperature and fuel consumption. With this new library, the user will be able to take into account theThe AMESim Thermal Libraries 17/17 influence of the convective and radiative heat transfer between the exhaust gas and the engine head. In addition, some gas/liquid exchanger models is being developed to predict the influence on the exhaust gas and coolant temperatures. ConclusionUsing the libraries presented, AMESim® is able to offer a powerful tool including basic and specific components, which allow a complete transient engine cooling, oil and exhaust gas system simulation. Moreover, it makes it possible to take into account the interaction between them.The AMESim® multiport approach provides an easier understanding of the system designed, and the AMESim® graphical interface facilitates easy changes to the system sketch and parameters. These characteristics are extremely important when a complete engine system is being analyzed.By providing new libraries, AMESim® will allow you to simulate complete systems, to analyze them during transient and steady state simulation and will offer you a new design approach for your products. –。
- 1、下载文档前请自行甄别文档内容的完整性,平台不提供额外的编辑、内容补充、找答案等附加服务。
- 2、"仅部分预览"的文档,不可在线预览部分如存在完整性等问题,可反馈申请退款(可完整预览的文档不适用该条件!)。
- 3、如文档侵犯您的权益,请联系客服反馈,我们会尽快为您处理(人工客服工作时间:9:00-18:30)。
16
温度为状态变量,通过能力守恒方程计算:
dT 1 dQ dV i hi i m i ( Cv dT ) [i m p ] dt m Cv dt dt
©IMAGINE SA 1998-2006
Training
16
阻性元件
计算质量流量(可压)
Pu Tu Pd Td
采用2阶多项式定义:
比热Cp(T) [J/kg/K]
其中: T=T-Tref
Cp Cp 0 . a0 (a t )T (a t 2 )T 2
绝对粘度v(T) [Ns/m² ]
0 .10 b (b )T(b
0 t
t 2 ) T
2
热导率[W/m/K]
0 . C0 (C t )T (C t 2 )T 2
有多种经验关系式描述 与NTU 和Cr=Cmin/Cmax的函数关系.
同心换热管举例:
©IMAGINE SA 1998-2006
Training
19
9
AMESim提供了gasgen.exe用于计算多 项式系数 :
©IMAGINE SA 1998-2006
Training
9
气体热力学属性- 混合气
10
通过‘Gas Mixt.’图标可以定义混合气
通过气体质量组分定义混合气组成: 例如空气
©IMAGINE SA 1998-2006
Training
10
气体热力学属性- 混合气
空气组成(% 体积比 = 摩尔组分):
78% of Nitrogen 21% of Oxygen 1% of Argon
11
摩尔组分转换成质量组分
M air .78 * 28 .21* 32 .01* 40 28.96 g.mol 1
Mass fractions
©IMAGINE SA 1998-2006
Training
6
气体热力学属性
7
AMESim内置22种 TPGD1子模型气体 属性
©IMAGINE SA 1998-2006
Training
7
气体热力学属性
8
气体属性按如下格式定义:
©IMAGINE SA 1998-2006
Training
8
气体热力学属性
Training
4
气体热力学属性
全局图标进行定义:
5
1 icon = 1gas
气体属性由文件定义: Cp(T), λ(T), μ(T) 最多可以包含100种气体计算 (ex.: thermal exchangers)
©IMAGINE SA 1998-2006
Training
5
气体热力学属性
6
TPGD1 : 热气动属性
不同类型的元件
15
容性元件:
被视为气体容腔,腔内压力、温度为状态变量
阻性元件:
通过输入的温度和压力计算焓流量和质量流量
©IMAGINE SA 1998-2006
Training
15
容性元件
压力为状态变量,通过理想气体状态方程计算:
dp 1 dT dV i r T m r [i m p ] dt V dt dt
17
ACqCm Pu m Tu
1 2 2 Pd Pd Pd Pcr (subsonic) r 1 Pu Pu Pu Cm 1 1 1 1 2 Pd Pcr (sonic) 2 2 r1 1 Pu r 1
THPN
Chapter 4
热气动库
©IMAGINE SA 1998-2006 Updated: October 2005
Training
简介
2
模拟热气动系统管网 计算系统压力、温度、质量流量和焓流量 系统可以由多种气体组成 研究气体与壁面之间换热(热端口) 与热库兼容
©IMAGINE SA 1998-2006
m dmh
m dmh
Flow
计算焓流量
h m C p dT dmh m
©IMAGINE SA 1998-2006
Training
17
换热器模型
基于effectiveness – NTU 模型:
广泛应用于换热器计算
18
入口温度必须已知
3个元件:
Training
2
热气动库
3
库图标 热气动库元件:
©IMAGINE SA 1998-2006
Training
3
主要元件
气体热力学属性: 容性元件(绝热或传热): 阻性元件(压力损失): 容性 – 阻性元件(绝热或传热)
4
摩擦 + 可压 + 对流
©IMAGINE SA 1998-2006
冷、热流体:2个流体端口 通过换热计算元件连接冷、热边
©IMAGINE SA 1998-2006
Training
18
换热器模型
定义为:
q q max
19
q :实际热流量,qmax :理论最大热流量
传热单元数NTU 定义为:
NTU U*A C min
U :换热系数, A :总换热面积, Cmin :最小热容流量
Training
12
气体热力学属性
13
热气动库包含图标:
©IMAGINE SA 1998-2006
Training
13
多种气体仿真
14
换热器举例
Diameter = 25 mm
Length = 1m Exhaust Air
or
©IMAGINE SA 1998-2006
Training
14
.78 * 28 .21* 32 N2 .754 02 .232 28.96 28.96 .01* 40 A .014 28.96
©IMAGINE SA 1998-2006
Training
11
气体热力学属性- 混合气
定义空气为混合 气组分:
12
©IMAGINE SA 1998-2006