Composites of TiO2-aluminum pillared montmorillonite_ Synthesis, characterization
一种ti2alc增强钛基复合材料及其制备方法和应用与流程
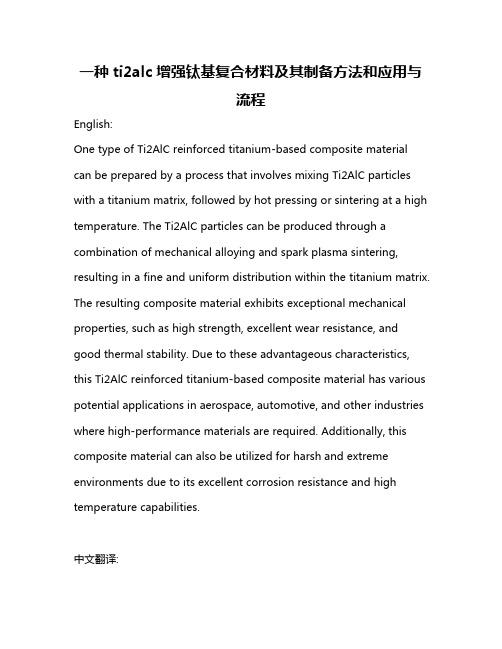
一种ti2alc增强钛基复合材料及其制备方法和应用与流程English:One type of Ti2AlC reinforced titanium-based composite material can be prepared by a process that involves mixing Ti2AlC particles with a titanium matrix, followed by hot pressing or sintering at a high temperature. The Ti2AlC particles can be produced through a combination of mechanical alloying and spark plasma sintering, resulting in a fine and uniform distribution within the titanium matrix. The resulting composite material exhibits exceptional mechanical properties, such as high strength, excellent wear resistance, and good thermal stability. Due to these advantageous characteristics, this Ti2AlC reinforced titanium-based composite material has various potential applications in aerospace, automotive, and other industries where high-performance materials are required. Additionally, this composite material can also be utilized for harsh and extreme environments due to its excellent corrosion resistance and high temperature capabilities.中文翻译:一种Ti2AlC增强钛基复合材料可以通过将Ti2AlC颗粒与钛基体混合,然后在高温下进行热压或烧结的工艺过程中制备。
非金属掺杂改性TiO2光催化剂的机理

收稿:2006年3月,收修改稿:2006年7月 3国家自然科学基金项目(N o.50478065)、安徽省科技厅重点科研项目(N o.05022126)、安徽省优秀青年科技基金项目(N o.04044077)和安徽省教育厅自然科学基金项目(N o.2006K J139B )资助33通讯联系人 e 2mail :tangyc @非金属掺杂改性TiO 2光催化剂的机理3唐玉朝1,233 黄显怀2 俞汉青1 胡 春3(1.中国科学技术大学化学与材料科学学院 合肥230026;2.安徽建筑工业学院环境工程学院 合肥230022;3.中国科学院生态环境研究中心 北京100085)摘 要 为了扩展T iO 2光催化剂对可见光的利用,以非金属元素对其进行掺杂和改性是近年来很活跃的研究内容,文献报道主要有氮、碳、硫、氟等非金属元素的改性结果。
各种不同的改性方法如高温气氛还原、脉冲激光沉积、离子溅射、机械化学、溶液湿法等都可以得到非金属元素改性。
本文重点探讨了氮改性T iO 2光催化剂的结果,氮改性T iO 2的方法和改性机理,讨论了氮改性T iO 2的结构及其对可见光的利用机理等,对碳、硫、氟等元素掺杂改性也作了简要介绍。
关键词 光催化 T iO 2 非金属元素 氮 碳 硫 氟中图分类号:O614.41+1;O643.36 文献标识码:A 文章编号:10052281X (2007)02Π320225209N onmetal E lement Doping Mechanisms of Titanium Oxide PhotocatalystTang Yuchao1,233 Huang Xianhuai 2 Yu Hanqing 1 Hu Chun3(1.School of Chemistry and Materials Science ,University of Science and T echnology of China ,Hefei 230026,China ;2.School of Environmental Engineering ,Anhui Institute of Architecture and Industry ,Hefei 230022,China ;3.Research Center for Eco 2Environmental Sciences ,Chinese Academy of Sciences ,Beijing 100085,China )Abstract In order to obtain visible light response ,doping or m odifying titania by nonmetal elements such as nitrogen has been investigated extensively in recent years.In this paper ,recent progress in mechanism of titania doping by nitrogen ,carbon ,sulphur ,fluorine is reviewed.Methods for preparing nitrogen doped titania are follows :i )reduce titania by amm onia gas under high tem perature ;ii )sputter titania target in nitrogen gas ;iii )pulsed laser deposition in nitrogen gas ;iv )mechanochemica1processing titania in amm onia ;v )direct oxidation titanic nitride ;vi )forming organic titanic nitride and then thermal treated ;vii )hydrolysis a titanic precurs or in amm onia s olution then thermal treated the precipitate.Structure and mechanism of visible light response of the nitrogen doped titania are discussed according to literature reports although there had many inconsistent conclusions.Narrowing the band gap of titania or forming a new is olated level in band gap may be the m ost probable reas on for visible response.T itania doping by carbon ,sulphur or fluorine can als o give rise to visible light response and high photo efficiency ,but there had many inconsistent conclusions too.K ey w ords photocatalysis ;titania ;nonmetal elements ;nitrogen ;carbon ;sulphur ;fluorine1 引言由于在太阳能转换和环境净化方面具有巨大的应用价值,光催化反应近年来受到了广泛的关注。
等离子体效应增强TiO2-SiO2-Ag复合薄膜的光吸收(英文)
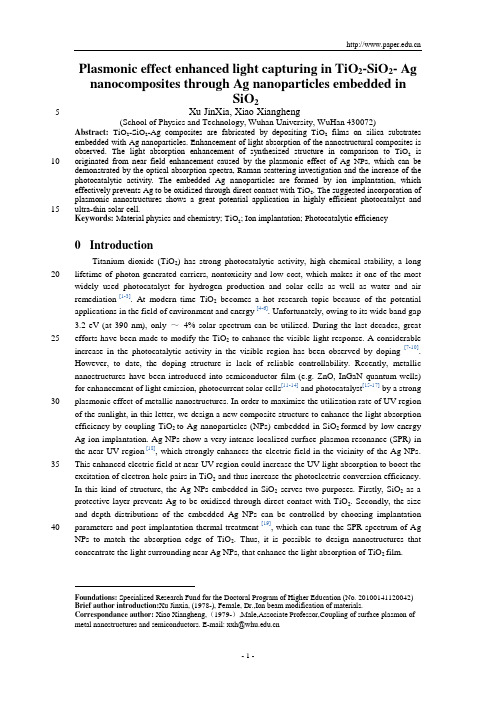
Plasmonic effect enhanced light capturing in TiO 2-SiO 2- Ag nanocomposites through Ag nanoparticles embedded inSiO 2Xu JinXia, Xiao Xiangheng5 (School of Physics and Technology, Wuhan University, WuHan 430072)Foundations: Specialized Research Fund for the Doctoral Program of Higher Education (No. 20100141120042) Brief author introduction:Xu Jinxia, (1978-), Female, Dr.,Ion beam modification of materials.Correspondance author: Xiao Xiangheng,(1979-),Male,Associate Professor,Coupling of surface plasmon of metal nanostructures and semiconductors. E-mail: xxh@Abstract: TiO 2-SiO 2-Ag composites are fabricated by depositing TiO 2 films on silica substrates embedded with Ag nanoparticles. Enhancement of light absorption of the nanostructural composites is observed. The light absorption enhancement of synthesized structure in comparison to TiO 2 is originated from near field enhancement caused by the plasmonic effect of Ag NPs, which can be 10demonstrated by the optical absorption spectra, Raman scattering investigation and the increase of the photocatalytic activity. The embedded Ag nanoparticles are formed by ion implantation, which effectively prevents Ag to be oxidized through direct contact with TiO 2. The suggested incorporation of plasmonic nanostructures shows a great potential application in highly efficient photocatalyst andultra-thin solar cell.15 Keywords: Material physics and chemistry; TiO 2; Ion implantation; Photocatalytic efficiency0 IntroductionTitanium dioxide (TiO 2) has strong photocatalytic activity, high chemical stability, a long lifetime of photon-generated carriers, nontoxicity and low cost, which makes it one of the most 20 widely used photocatalyst for hydrogen production and solar cells as well as water and air remediation [1-3]. At modern time TiO 2 becomes a hot research topic because of the potential applications in the field of environment and energy [4-6]. Unfortunately, owing to its wide band gap3.2 eV (at 390 nm), only ~ 4% solar spectrum can be utilized. During the last decades, great efforts have been made to modify the TiO 2 to enhance the visible light response. A considerable 25 increase in the photocatalytic activity in the visible region has been observed by doping [7-10]. However, to date, the doping structure is lack of reliable controllability. Recently, metallic nanostructures have been introduced into semiconductor film (e.g. ZnO, InGaN quantum wells) for enhancement of light emission, photocurrent solar cells [11-14] and photocatalyst [15-17] by a strong plasmonic effect of metallic nanostructures. In order to maximize the utilization rate of UV region 30 of the sunlight, in this letter, we design a new composite structure to enhance the light absorption efficiency by coupling TiO 2 to Ag nanoparticles (NPs) embedded in SiO 2 formed by low energy Ag ion implantation. Ag NPs show a very intense localized surface plasmon resonance (SPR) in the near-UV region [18], which strongly enhances the electric field in the vicinity of the Ag NPs. This enhanced electric field at near-UV region could increase the UV light absorption to boost the 35 excitation of electron-hole pairs in TiO 2 and thus increase the photoelectric conversion efficiency. In this kind of structure, the Ag NPs embedded in SiO 2 serves two purposes. Firstly, SiO 2 as a protective layer prevents Ag to be oxidized through direct contact with TiO 2. Secondly, the size and depth distributions of the embedded Ag NPs can be controlled by choosing implantation parameters and post-implantation thermal treatment [19], which can tune the SPR spectrum of Ag 40NPs to match the absorption edge of TiO 2. Thus, it is possible to design nanostructures that concentrate the light surrounding near Ag NPs, that enhance the light absorption of TiO 2 film.1 Experimental SectionHigh purity silica slides were implanted by Ag ions at 20, 40 and 60 kV to fluence of 5×1016 ions/cm 2 and at 40 kV to 1×1017 ions/cm 2 using a metal vapor vacuum arc ion source implanter, 45 respectively. The TiO 2-SiO 2-Ag nanostructural composites were obtained by depositing TiO 2 films (100 nm thick) on the surface of the as-implanted silica substrates using direct-current (DC) reactive magnetron sputtering system. For comparison, an un-implanted silica substrate was deposited with TiO 2 film under the same growth condition. Subsequently, all deposited samples were annealed at 500 °C in oxygen gas for 2 hours to obtain anatase phase TiO 2 film. The TiO 2 50covered silica substrates with embedded Ag NPs are named S1-S4 as shown in Table 1. The optical absorption spectra of all the samples were measured using a UV-vis-NIR dual-beam spectrometer (Shimadzu UV 2550) with wavelengths varied from 200 to 800 nm. Raman scattering spectra of all the samples were collected using a micro-Raman system (LabRAM HR800). An Ar laser (488.0 nm) was used as the excitation source, and the laser power was kept55 at 10 mW. The microstructure of the samples was investigated by using a JEOL JEM 2010 (HT) transmission electron microscopes (TEM) operated at 200 kV.Tab. 1 Ag ion implantation parameters for all samplessample Fluence of ion implantation(ions/cm2)Energy of ion implantation(kV)S1 5×1016 20S2 5×1016 40S3 1×1017 40 S4 5×1016 6060 2 Results and DiscussionThe photocatalytic efficiencies of TiO 2 and TiO 2-SiO 2-Ag nanostructural composites with an area of 4 cm 2 were evaluated by measuring the degradation rates of 5 mg/L methylene blue (MB) solution under UV-vis irradiation. A mercury lamp (OSRAM, 250 W with characteristic wavelength at 365 nm) was used as a light source. The TiO 2 and the TiO 2-SiO 2-Ag composites 65 films were placed into 40 mL MB solution with a concentration of 5 mg/L. Before irradiation, the samples were put into 40 mL MB solution for 30 minutes in the darkness to reach absorption equilibrium. The decolorization of MB solution was measured by an UV-vis spectrometer (Shimadzu UV 2550) at the wavelength of 664.0 nm. The absorption spectrum of the MB solution was measured at a time interval of 30 minutes and the total irradiation time was 4 hours. 70 Fig. 1 shows the optical absorption spectra of S1-S4 and the TiO 2 films. The absorption edge around 390 nm belongs to the intrinsic exciton absorption of TiO 2 [20]. The obvious absorption peaks at about 419-433 nm can be attributed to the SPR of Ag NPs formed by Ag ion implantation[21]. As seen the SPR of Ag NPs is close to the exciton edge (around 390 nm) of anatase TiO 2. Therefore, it is expected that an efficient energy transfer from the Ag NPs to TiO 2 can be occured. 75The position of Ag SPR absorption peak of the S2 is around 419 nm, which is blue shift comparing to that of the other three samples. The SPR peak of the S2 is the most close to the anatase TiO 2 exciton energy, therefore the strongest resonant coupling effect between Ag SPR and the excitons of the TiO 2 films maybe produce more effectively.80Fig. 1 The optical absorption spectra of S1-S4 and the pure TiO2 filmTo illustrate the strong near field induced by the SPR of Ag NPs, the Raman scattering spectra of S1-S4 and TiO2 are measured as presented in Fig. 2. The observed Raman bands at 144, 85199, 399, 516 and 640 cm-1 can be assigned to the Eg, Eg, B1g, A1g, or B1g and an Eg vibration modes of anatase phase, respectively, which are consistent with the characteristic patterns of pure anatase without any trace of rutile or brookite phase [22]. It is found that the Raman intensity for S1-S4 increase compared to that of TiO2, and the S2 shows the strongest Raman intensity. It is well known that Raman scattering intensity is proportional to the square of the electric field 90intensity [23], thus stronger Raman scattering attained from the TiO2-SiO2-Ag structure indicates that a stronger electric field is induced by Ag NPs embedded in SiO2 substrate. When the Ag NPs are irradiated by laser in spectral area of particle absorption band longer wavelength shoulder, a strong near field is produced due to the SPR, so Raman scattering is enhanced. As seen from Fig.2, the enhancement factors of Raman scattering of S1-S4 is different because of various coupling 95field efficiency. Thus, it is possible to conclude that the implantation energy and fluence have determined the Raman scattering enhancement factor.Fig. 2 The Raman scattering spectra of S1-S4 and the pure TiO2 filmTo understand the relationship between the size and depth distributions of Ag NPs in silica 100glass and the Raman scattering enhancement factor of the TiO2-SiO2-Ag nanocomposites, themicrostructural characterization of the S1-S4 were investigated by TEM as shown in Fig. 3. The TEM image of the S1 (Fig 3 (a)) shows that the size of Ag NPs appears to have a wide distribution.However, increasing the implantation energy to 40 kV as shown in Fig 3 (b), the Ag NPs in the S2 105are quite uniform in size (with an size of 20 nm) and distribute at nearly the same depth of 7 nm from the surface. Under high energy ion implantation, more heat will be induced in the sample in short time, which enhances the diffusion of Ag atoms. Therefore, the implanted Ag ions trend to aggregate to larger NPs around the projected range [24-26]. The near field induced by the SPR of Ag NPs is very strong due to the presence of the formed Ag NPs with bigger size and the near-field 110dipolar interactions between adjacent particles [27]. On the other hand, the dipolar interactions between adjacent particles with near the same size can result in a blue shift of SPR [28], thus the blue shift in the SPR peak of Ag NPs observed in the Fig. 1, which may produce a strongest resonant coupling effect between the SPR of Ag NPs and TiO2. It means the stronger near field can be induced. In this case, the S2 has the strongest Raman scattering enhancement factor. The 115size of Ag NPs in the S1 is smaller and the distribution is wider than that in the S2. It means that the near field induced by SPR of Ag NPs in the S1 is weaker than that in the S2. Further increasing the implantation energy to 60 kV as presented in Fig 3 (d), Ag NPs in the S4 reside deeper below the surface than that in the S2. Since the SP is an evanescent wave that exponentially decays with distance from the metal particles to the surface [29], the enhancement of 120Raman scattering decrease progressively with the increase of distance between Ag NPs with the TiO2 film, therefore Raman scattering intensity of the S4 has almost no enhancement. When the ion implantation fluence is increased to 1×1017 ions/cm2 with implantation energy of 40 kV (S3) as displayed in Figure 3 (c), large Ag NPs with size about 15 nm are formed near the surface and the small ones in deeper SiO2 matrix. The surface sputtering effect plays an important role for ion 125implantation at high fluence, the formed small Ag NPs near the surface are sputtered away by the subsequent implanted ions, as a result the large Ag NPs are populated near the surface of the S3[24].The Raman scattering enhancement factor is small with increasing the implantation fluence.Therefore, the Raman scattering enhancement demonstrates that the strong near field is actually induced by introducing Ag NPs, the increased field could locally concentrate the light surrounding the Ag NPs and thus enhance the absorption of light.130Fig. 3 Cross-sectional TEM images of the (a) S1, (b) S2, (c) S3, (d) S4.In order to study the enhancement of light absorption in TiO2-SiO2-Ag nanostructural 135composites, the photocatalysis activities of the S1-S4 are investigated by the UV degradation of MB solution at room temperature. For comparison, the TiO2 film is carried out under the same experimental conditions. As shown in Fig 4(a) inset one, the concentration of MB is decreased upon the irradiation time, and the TiO2 film can decompose 49% of MB after the UV irradiationfor 4 hours. However, the TiO 2-SiO 2-Ag nanostructural composite films obtained higherphotocatalytic efficiency than the pure TiO 2 film, and the S2 has the highest photocatalytic 140 efficiency than all other three samples, and degrade 72% MB. The enhancement ratio is as high as 47%. Meanwhile, the photodegradation of MB can be assumed to follow the classical Langmuir–Hinshelwood (L–H) kinetics [30], and its kinetics can be expressed as follows:0ln()A kt A = Where k is the apparent first-order reaction rate constant (min -1), 0A and A represent the145 absorbance before and after irradiation for time t , respectively. As displayed in Fig. 4(a), S2 shows the highest rate constant among all the samples, the k values of the S2 is about 2 times than that of the pure TiO 2. The kinetic rate constants follow the order S2>S3>S1>S4>TiO 2. This is consistent with the Raman scattering enhancement result.The near field enhancement in the TiO 2 layer due to the presence of the Ag NPs is also 150 simulated by Finite Difference Time Domain (FDTD) method as shown in Fig. 4(b). In our structure, we consider x as the light incident direction, the illuminating plane wave with a wavelength of 420 nm is y polarized, and an Ag NP with a diameter of 20 nm is embedded in SiO 2, and the distance to the surface of SiO 2 substrate is 7 nm. An amplitude enhancement to 3 can be observed. Theoretical and experimental results show that an enhancement of near field is induced 155by the SPR of Ag NPs. The SPR excitations cause a large increase in electromagnetic field in the vicinity of metal NPs, the localized amplification can increase the incident excitation field and boost the creation of hole-electron pairs, which results in the enhancement of the photocatalytic activity of TiO 2.160 Fig. 4 (a) The photodegradation of MB solution by S1-S4 and reference sample TiO 2 under UV light irradiation (inset one), and corresponding plots of 0ln()A Aversus the irradiation time, showing the linear fitting results; (b) amplitude enhancement of electric field inside a TiO 2 layer is simulated by FDTD method.3 Conclusion165 In conclusion, we have successfully demonstrated a plasmonic effect by simply incorporating Ag NPs with TiO 2 film. Optimum ion implantation conditions for Ag NPs synthesis in SiO 2 were experimentally estimated. The plasmonic effect occurring near interface of TiO 2 and silica glass has effectively enhanced the light trapping. Both the experimental data and the simulations show that the enhancement effect is attained from near field enhancement induced by the SPR of Ag 170 NPs. Our results have shown that the plasmonic effect has great potential in the application of increasing the UV light absorption in TiO 2 photocatalyst, and opening up opportunities for highly efficient ultra-thin film solar cells.References175[1] Wang D, Zou Y, Wen S and Fan D. A passivated codoping approach to tailor the band edges of TiO2 forefficient photocatalytic degradation of organic pollutants[J]. Appl. Phys. Lett. 2009, 95: 012106-1-3.[2] Han F, Kambala V S R, Srinivasan M, Rajarathnam D and Naidu R. Tailored titanium dioxide photocatalystsfor the degradation of organic dyes in wastewater treatment: A review[J]. Appl. Catal. A-Gen 2009, 359:25-40. 180[3] Yang J, You J, Chen C C, Hsu W C, Tan H R, Zhang X W, Hong Z and Yang Y. Plasmonic Polymer TandemSolar Cell[J]. ACS nano 2011, 5: 6210-6217.[4] Min B K, Heo J E, Youn N K, Joo O S, Lee H, Kim J H and Kim H S. Tuning of the photocatalytic1,4-dioxane degradation with surface Plasmon resonance of gold nanoparticles on titania[J]. Catal. Commun. 2009, 10:712 -715.185[5] Kumar M K, Krishnamoorthy S, Tan L K, Chiam S Y, Tripathy S and Gao H. Field Effects in PlasmonicPhotocatalyst by Precise SiO2 Thickness Control Using Atomic Layer Deposition[J]. ACS Catal. 2011, 1: 300-308.[6] Tong H, Quyang S, Bi Y, Umezawa N, Oshikiri M and Ye J. Nano- photocatalytic Materials: Possibilities andChallenges[J]. Adv. Mater. 2012, 24: 229-251.190[7] Anpo M. Preparation, Characterization, and Reactivities of Highly Functional Titanium Oxide-BasedPhotocatalysts Able to Operate under UV-Visible Light[J]. Bull. Chem. Soc. Jpn. 2004, 77:1427-1442.[8] Asahi R, Morikawa T, Ohwaki T, Aoki K and Taga Y. Visible-Light Photocatalysis in Nitrogen-DopedTitanium Oxides[J]. Science 2001, 293:269-271.[9] Ghicov A, Macak J M, Tsuchiya H, Kunze J, Haeublein V, Frey L and Schmuki P.Ion Implantation and 195Annealing for an Efficient N-Doping of TiO2 Nanotubes[J].Nano Lett.2006, 6 (5): 1080-1082.[10] Xu J H, Li J, Dai W L, Cao Y, Li H and Fan K. Simple fabrication of twist-like helix N,S-codoped titaniaphotocatalyst with visible-light response[J].Appl. Catal., B-Environ.2008, 79: 72-80.[11] Xiao X H, Ren F, Zhou X D, Peng T C, Wu W, Peng X N, Yu X F and Jiang C Z. Surface plasmon-enhancedlight emission using silver nanoparticles embedded in ZnO[J]. Appl. Phys. Lett.2010, 97:071909-1-3.200[12] Zhou X D, Xiao X H, Xu J X, Cai G X, Ren F, and Jiang C Z. Mechanism of the enhancement and quenchingof ZnO photoluminescence by ZnO-Ag coupling[J]. Europhys. Lett.2011, 93: 57009-p1-p6.[13] Zhang S G, Zhang X W, Yin Z G, Wang J X, Dong J J, Gao H L, Si F T, Sun S S and Tao Y. Localizedsurface plasmon-enhanced electroluminescence from ZnO-based heterojunction light-emitting diodes[J]. Appl.Phys. Lett.2011, 99: 181116-1-3.205[14] Okamoto K, Niki I, Shvartser A, Narukawa Y, Mukai T, and Scherer A. Surface-plasmon-enhanced lightemitters based on InGaN quantum wells[J]. Nature Mater.2004, 3: 601-605.[15] Awazu K, Fujimaki M, Rockstuhl C, Tominaga J, Murakami H, Ohki Y, Yoshida N, Watanabe T. APlasmonic Photocatalyst Consisting of Silver Nanoparticles Embedded in Titanium Dioxide[J]. J. Am. Chem.Soc.2008, 130: 1676 -1680.210[16] Oh J -H, Lee H, Kim D, Seong T Y. Effect of Ag nanoparticle size on the plasmonic photocatalytic propertiesof TiO2 thin films[J]. Surf. Coat. Technol. 2011, 206(1): 185-189.[17] Subrahmanyam A, Biju K P, Rajesh P, Jagadeesh Kumar K, Raveendra Kiran M. Surface modification ofsol gel TiO2 surface with sputtered metallic silver for Sun light photocatalytic activity: Initial studies[J]. Sol.Energy Mater. Sol. Cells 2012, 101: 241-248.215[18] Kerker M. The optics of colloidal silver: something old and something new[J]. J. Colloid Interface Sci.1985,105: 297-314.[19] Stepanov A L, Hole D E and Townsend P D. Modification of size distribution of ion implanted silvernanoparticles in sodium silicate glass using laser and thermal annealing[J]. Nucl. Instr. Meth. Phys. Res. B 1999, 149: 89-98.220[20] Linsebigler A L, Lu G Q and Yates Jr J T. Photocatalysis on TiO2 Surfaces: Principles, Mechanisms, andSelected Results[J]. Chem. Rev.1995, 95:735-758.[21] Ren F, Jiang C Z, Liu C, Fu D J and Shi Y. Interface influence on the surface plasmon resonance of Agnanocluster composite[J]. Solid State Commun. 2005, 135: 268-272.[22] Zhang W F, He Y L, Zhang M S, Yin Zand Chen Q. Raman scattering study on anatase TiO2 nanocrystals[J]. 225J. Phys. D: Appl. Phys. 2000, 33: 912-916.[23] Willets K A, Van Duyne R P. Localized Surface Plasmon Resonance Spectroscopy and Sensing[J]. Annu.Rev. Phys. Chem. 2007, 58: 267-297.[24] Ren F, Xiao X H, Cai G X, Wang J B, Jiang C Z. Engineering embedded metal nanoparticles with ion beamtechnology[J]. Appl. Phys. A.2009, 96: 317-325.230[25] Xiao X H, Ren F, Wang J B, Liu C, Jiang C Z. Formation of aligned silver nanoparticles by ionimplantation[J]. Mater. Lett.2007, 61: 4435-4437.[26] Ren F, Jiang C Z, Liu C, Wang J B and Oku T. Controlling the morphology of Ag nanoclusters by ionimplantation to different doses and subsequent annealing[J]. Phys. Rev. Lett. 2006, 97: 165501-1-4.[27] Biteen J S, Lewis N S, and Atwater H A. Spectral tuning of plasmon-enhanced silicon quantum dot 235luminescence[J]. Appl. Phys. Lett. 2006, 88:131109-1-3.[28] Maier S A and Atwater H A. Plasmonics Localization and guiding of electromagnetic energy inmetal/dielectric structures[J]. J. Appl. Phys. 2005, 98: 011101-1-10.[29] Chen C W, Wang C H, Wei C M, Chen Y F. Tunable emission based on the composite of Au nanoparticlesand CdSe quantum dots deposited on elastomeric film[J]. Appl. Phys. Lett. 2009, 94: 071906-1-3.240[30] Al-Ekabi H and Serpone N. kinetic studies in heterogeneous photocatalysis. 1. photocatalytic degradatlon ofchlorinated phenols in aerated aqueous solutions over TiO2 supported on a glass matrix[J]. J. Phys. Chem.1988, 92:5726-5731.等离子体效应增强TiO2-SiO2-Ag复合薄膜的光吸收徐进霞,肖湘衡245(武汉大学物理科学与技术学院,武汉 430072)摘要:利用离子注入方法将Ag离子注入到SiO2玻璃中,再利用反应磁控溅射沉积TiO2薄膜。
TiO2、MgO添加剂对Al2O3陶瓷材料烧结致密化影响的研究
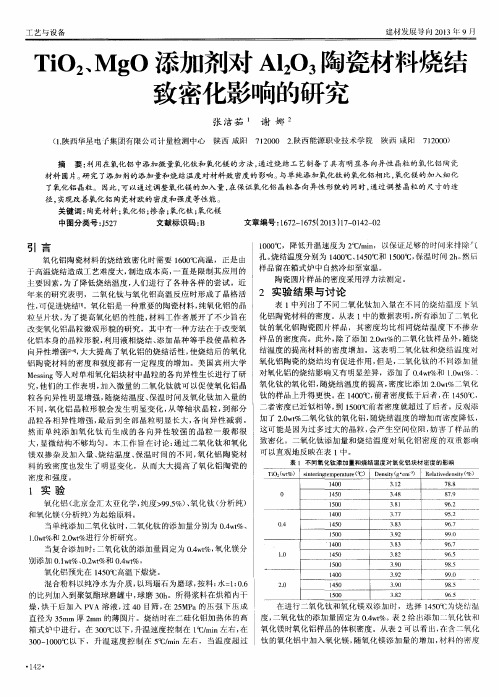
1 4 0 0 1 4 5 0 I 5 0 0
3 . 9 2 3 . 9 0 3 盘2
9 9( ) 9 8 5 9 6 . 5
在 进 行 二 氧 化 钛 和 氧 化 镁 双 添 加 时 ,选 择 1 4 5 0  ̄ C 为 烧 温 度 , 二 氧 化 钛 的 添加 量 固定 为 0 . 4 w t %。 表 2给 出添 加 氧 化 钛 和 直径为 3 5 a r m厚 2 m m 的薄 圆 片 。烧 结 时在 二 硅 化 钼加 热体 的高 箱式炉 中进行。在 3 0 0  ̄ C以下, 升温速度控制在 l  ̄ C / mi n左右 , 在 氧化镁时氧化铝样品的体积密度 。从表 2可以看 出, 存含 二氧化 3 0 0 ~ 1 0 0 0 ℃ 以下 ,升 温 速 度 控 制 在 5 ℃/ m i n左 右 , 当 温 度 超 过 钛 的氧 化 铝 中加 入氧 化镁 , 随 氧 化 镁 添 加 量 的增 加 , 材 料 的 窬 度
工艺 与 设 备
建 ‘ 发 展 导向 2 0 1 3年 9川
T i O2 、 Mg O添加剂对 A l 2 o 3 陶瓷材料烧结 致密化影响的研究
张 洁 茹
( 1 . 陕 西华 星 电f 集 团有 限公 司 计 量检 测 中心 陕西 成阳
谢 娜
7 1 2 0 0 0 2 . 陕西 能 源职 、 技术学院 陕西 成 阳 7 1 2 0 0 0 )
表 1 不同氧化钛添加 量和烧结温度对氧化铝块材 密度 的影响
T i O , ( wt %) s i n t e r i n g t e mp e r a t u r e ( ℃)
1 4 0 0
O 1 4 50 l 5 00 1 4 00 0. 4 1 4 50
进展与述评TiO2Al2O3复合氧化物载体研究进展

进展与述评TiO2Al2O3复合氧化物载体研究进展CHEMICAL INDUSTRY AND ENGINEERING PROGRESS 2011年第30卷第7期·1482·化工进展TiO2·Al2O3复合氧化物载体研究进展郭长友,沈智奇,张志民(中国石油化工股份有限公司抚顺石油化工研究院,辽宁抚顺113001)摘要:TiO2·Al2O3多孔复合氧化物是新型的催化剂载体材料,因其在加氢精制工艺上能够显著增加催化剂的脱硫、脱氮活性而逐渐受到重视。
本文综述了国内外关于TiO2·Al2O3多孔材料的制备方法,分析了制备方法对材料的比表面积、孔结构、表面酸性等载体性能影响的一般规律。
通过对国内外研究者的TiO2·Al2O3合成方法的综合评述,指出了有待发展提高的方向。
关键词:TiO2·Al2O3多孔复合氧化物;加氢精制;催化剂载体中图分类号:TQ 426.65 文献标志码:A 文章编号:1000–6613(2011)07–1482–07Res earch progress of TiO2·Al2O3 composite supportGUO Changyou,SHEN Zhiqi,ZHANG Zhimin(Fushun Research Institute of Petroleum and Petrochemicals,SINOPEC,Fushun 113001,Liaoning,China)Abstract:Porous composite oxide of TiO2·Al2O3 is a new type of catalyst support,which has attract- ed increasing attention due to its capability to improve catalytic activity for hydrodesulfurization (HDS)and hydrodenitrogenation(HDN)of hydrotreating catalyst. This paper reviews the studies on the synthesis of porous TiO2·Al2O3 an d analyzes influence factors of catalyst support properties,such as textural property andsurface acidity etc,in the synthesis process.Key words:porous composite oxide of TiO2·Al2O3;hydrotreating;catalyst support能源和环境问题一直是影响我国社会、经济可持续发展的核心问题。
钛铝金属间化合物多孔膜材料

钛铝金属间化合物多孔膜材料I am fascinated by the potential applications of porous membrane materials made from titanium-aluminum intermetallic compounds. These materials have shown great promise in various industries dueto their unique properties, such as high strength, corrosion resistance, and thermal stability.我对由钛铝金属间化合物制成的多孔膜材料的潜在应用着迷。
由于其独特的特性,如高强度、耐腐蚀性和热稳定性,这些材料在各个行业中显示出巨大的潜力。
From an environmental perspective, these porous membrane materials can play a crucial role in wastewater treatment processes. Their high porosity allows for efficient filtration of contaminants, leading to cleaner water sources and a healthier environment. Additionally, these materials can be easily regenerated and reused, reducing overall waste and promoting sustainability practices.从环境的角度看,这些多孔膜材料在废水处理过程中发挥着至关重要的作用。
它们的高多孔性能够有效过滤污染物,导致更清洁的水源和更健康的环境。
以胶原纤维为模板合成介孔TiO2-Al2O3二元复合氧化物纤维

摩 尔比为 4 8 : .8 1时,i2 TO 一
。
A 二元 复合 氧化 物纤 维的平 均孔 径 为 7 4 n 比表 面积 可达 110 c g 其 中 TO 1 O . m, 3 . m / , i2主要 以锐 钛矿 相存 在 , 关键 词 :胶 原纤维 ;模板 ;介孔 ;TO 一A2 3 i 2 1O 二元复合 氧化 物纤维 中图分 类号 :T 3 B3 文献标识码 :A 文章编号 :17 -1x 2 1 ) 135 - 6262 (0 1 140 50 5
21年 1 01 1月
3 券 0 第 1 1
绵 阳 师范 学 院学 报
o r a fMin a  ̄N r 1Un v r i u n lo a v n o m8 i es Βιβλιοθήκη NO .. V 201 1
V 1 3 No 1 o. 0 .1
以胶原 纤 维 为模 板 合 成 介 孑 i LT O2一 Alo3二 元 复 合 氧化 物 纤 维 2
陈 琛 吕 广 廖 学品 , , , 石 碧
( ̄JI I I I 大学 1生 物质 与化 学工程系 ;. . 2 制革清洁技术 国家工程实验室 , 四川成都 60 6 ) 10 5 摘 要 :以胶 原纤维为模板合成 了介孔 TO 一A 二元复合氧化物纤维 , i 1 O 并利 用扫描 电镜 ( E 、 S M) x射线衍
通讯作者 : 廖学品 (95 , 16 一)教授 , 博士生导师 , 主要研究方向 : 皮革化学与工程。E a : lo C.d.n m ix i @8Ueuc l pa
-
5 6・
绵 阳师范学 院学报 ( 自然科 学版 )
第3 0卷
12 材 料 制 备 .
12 1 胶 原 纤 维 负载 T 和 A¨ 配制 T/ 1 .. i l i 摩尔 比不 同的 T¨ 、 l A i A¨复合前 驱体 溶液 ; 1 胶 原纤 维 将 0g 加人 到 30m 去离 子水 中 , H s 和 H O H 的混合 液 ( 2O/ C O 0 l 用 0 CO H S H O H=l1V V) p 调 节 至 18 :, / 将 H . 20 室温下搅拌 2h加入 T¨、 l ., ; i A¨复合前驱体溶液( i + 1 = .6m 1, T A¨ 00 o)在室温下搅拌反应 4h 缓 ; 慢滴 加 N HC , 液 , 4h内将 p a O溶 在 H升高 至 38— . , 温至 4 . 40 升 0℃ 后再反 应 6h 。反 应结束 后 过滤 , 以去 离子 水洗 涤 , 6 在 0℃ 下真 空干燥 1 。 2h 122 介 孔 TO 一A 元复 合氧化 物 纤维的 制备 用 程序升 温法对 负载 了 r 和 A¨ 的胶 原 纤维 进 .. i: 1O 二 r i l 行热 处理 , 以去除模 板 。升温 程序如 下 : 空气 气 氛 , 升温 速率 5℃ / n 在 10℃ 下通空 气 2h 3 0℃ 下通 mi; 0 ,0 空 气 2h 5 0℃ 下通 空气 7h 自然冷去 至室 温后 即获得介 孑 i: 1 , ,5 ; L O 一A: 二元 复合 氧化 物纤维 。根 据前 驱 T O
TiO_(2)的添加对铝铬固溶体烧结动力学的影响
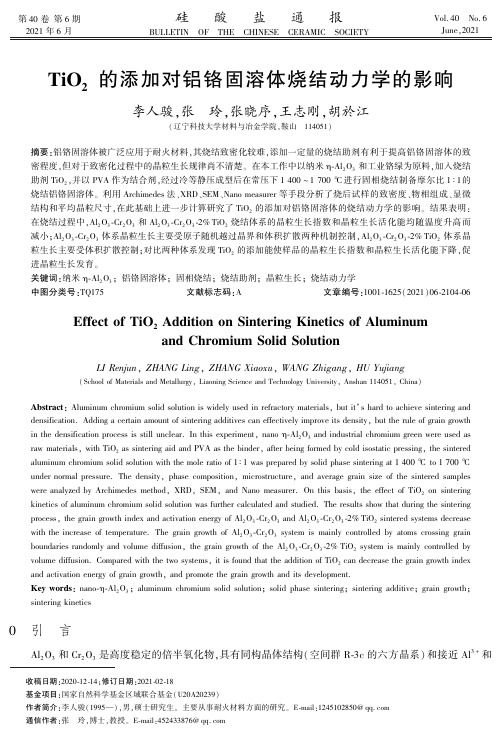
( School of Materials and Metallurgy, Liaoning Science and Technology University, Anshan 114051, China)
Abstract: Aluminum chromium solid solution is widely used in refractory materials, but it’ s hard to achieve sintering and densification. Adding a certain amount of sintering additives can effectively improve its density, but the rule of grain growth in the densification process is still unclear. In this experiment, nano η-Al2 O3 and industrial chromium green were used as raw materials, with TiO2 as sintering aid and PVA as the binder, after being formed by cold isostatic pressing, the sintered aluminum chromium solid solution with the mole ratio of 1 ∶ 1 was prepared by solid phase sintering at 1 400 ℃ to 1 700 ℃ under normal pressure. The density, phase composition, microstructure, and average grain size of the sintered samples were analyzed by Archimedes method, XRD, SEM, and Nano measurer. On this basis, the effect of TiO2 on sintering kinetics of aluminum chromium solid solution was further calculated and studied. The results show that during the sintering process, the grain growth index and activation energy of Al2 O3 -Cr2 O3 and Al2 O3 -Cr2 O3 -2% TiO2 sintered systems decrease with the increase of temperature. The grain growth of Al2 O3 -Cr2 O3 system is mainly controlled by atoms crossing grain boundaries randomly and volume diffusion, the grain growth of the Al2 O3 -Cr2 O3 -2% TiO2 system is mainly controlled by volume diffusion. Compared with the two systems, it is found that the addition of TiO2 can decrease the grain growth index and activation energy of grain growth, and promote the grain growth and its development. Key words: nano-η-Al2 O3 ; aluminum chromium solid solution; solid phase sintering; sintering additive; grain growth; sintering kinetics
- 1、下载文档前请自行甄别文档内容的完整性,平台不提供额外的编辑、内容补充、找答案等附加服务。
- 2、"仅部分预览"的文档,不可在线预览部分如存在完整性等问题,可反馈申请退款(可完整预览的文档不适用该条件!)。
- 3、如文档侵犯您的权益,请联系客服反馈,我们会尽快为您处理(人工客服工作时间:9:00-18:30)。
NoteComposites of TiO 2-aluminum pillared montmorillonite:Synthesis,characterization and photocatalytic degradation of methylene blueIs Fatimah a ,c ,⁎,Shaobin Wang b ,Narsito c ,Karna Wijaya ca Chemistry Department,Universitas Islam Indonesia,Yogyakarta,55581,Indonesiab Department of Chemical Engineering,Curtin University of Technology,GPO Box U1987,Perth,WA 6845,Australia cChemistry Department,Gadjah Mada University,Yogyakarta,Indonesiaa b s t r a c ta r t i c l e i n f o Article history:Received 20October 2009Received in revised form 7August 2010Accepted 21August 2010Available online 20October 2010Keywords:Pillared montmorillonite Photocatalyst Titania DyesAluminum pillared montmorillonite was prepared and used as a porous support for synthesis of TiO 2-based photocatalysts with varying TiO 2loading.The physicochemical properties of the TiO 2-pillared montmorillonite samples were characterized by X-ray diffraction,N 2adsorption isotherm,UV –visible diffuse re flectance spectroscopy,scanning electron microscopy and transmission electron microscopy.Photocatalytic activity of the materials was evaluated by methylene blue photodegradation in aqueous solution.The titania content in the composites signi ficantly in fluenced the physicochemical properties and catalytic activity.High TiO 2loading in the composite produced crystalline TiO 2and enhanced dye degradation.©2010Elsevier B.V.All rights reserved.1.IntroductionTitania (TiO 2)is a well known material in photocatalysis.As a semiconductor with wide band gap energy and nontoxicity,it is widely used for photocatalytic decomposition of various organic compounds in wastewater treatment (Rajeshwar et al.,2008;Fujishima et al.,2000,2007,2008).However,the use of titania in bulk form suffers from catalyst recovery and activity reduction,which results in ineffective application of the catalyst.In some cases,fine titania particles could also block the penetration of light into the solution.To overcome the limitations,several approaches were proposed e.g.attaching titania particles onto stable inorganic supports (Mahmoudi and Arami,2009;Reddy et al.,2003;Fukahora et al.,2003;Hisanaga and Tanaka,2002;Zhang et al.,1998;Li et al.,2008;Rao et al.,2003).In principle,inorganic materials with high speci fic surface area and chemical stability can be used as host matrix.Natural porous materials such as zeolites and clay minerals are also good and cheaper supports with eco-friendly properties.Clay minerals possess high porosity,exchangeable cations and swellable properties.Previously,several investigators reported the preparation of titania immobilization on clay minerals via pillarization processes.During the preparation,titania was incorporated in the interlayer space of the clay through an intercalation step of pillaring precursors via ionic species followed by calcination to convert the titanium hydroxide to oxide.However,highly acidic environment oftitanium pillaring precursor damages the structure of clay minerals (Yuan et al.,2006;Kooli et al.,1997;Bergaya et al.,2006).As a result,the low crystallinity of supported titania would have a reduced activity in heterogeneous catalysis.In addition,the preparation was not reproducible (Romero et al.,2006).Recently,some investigations reported the synthesis of supported TiO 2or TiO 2composites as catalysts (An et al.,2008;Belessi et al.,2007;Daniel et al.,2007,2008Menesi et al.,2008).Several metals i.e.Al,Zr and Fe were used as pillaring precursors for preparation of pillared clay minerals with higher thermal stability and speci fic surface area.Among them,poly(hydroxo)aluminum pillars were intensively studied due to easy Keggin ion formation.The enhanced speci fic surface area,porosity and thermal stability made these pillared clay minerals as good host matrices.Recently,several reports also showed that aluminum pillared clay minerals can host metal and metal oxide catalysts (Gil et al.,2000,2008;Vicente et al.,2004).In the present study,we report the preparation of an aluminum pillared Indonesian montmorillonite and its use as a support for titania immobilization.These photocatalysts were characterized by several techniques and tested for photodegradation of methylene blue.2.Materials and methods 2.1.Preparation of materialsAlCl 3⋅6H 2O,NaOH,and isopropanol were supplied by Merck and titanium isopropoxide (TTIP)was obtained from Sigma-Aldrich.TheApplied Clay Science 50(2010)588–593⁎Corresponding author.Chemistry Department,Universitas Islam Indonesia,Yogyakarta,55581,Indonesia.Tel.:+62818273001;fax:+62274895920.E-mail address:isfatimah@fmipa.uii.ac.id (I.Fatimah).0169-1317/$–see front matter ©2010Elsevier B.V.All rights reserved.doi:10.1016/j.clay.2010.08.016Contents lists available at ScienceDirectApplied Clay Sciencej o u r n a l h o me p a g e :w w w.e l sev i e r.c om /l o c a t e /c l aysynthesis of aluminum pillared montmorillonite consisted of (i)pre-paration of Keggin ion Al 13solution,(ii)Keggin ion intercalation and neutralization,and (iii)calcination at 500°C.The Keggin ion (Al 13)solution was prepared by slow titration of AlCl 3⋅6H 2O solution with a NaOH solution under vigorously stirring until the ratio of OH −/Al=2.2was reached.The intercalation process was then conducted by dropping this solution into a montmorillonite dispersion at a ratio of 5.0mmol Al/g of montmorillonite without any aging process and calcination at 500°C for 4h.The obtained aluminum pillared montmorillonite was denoted as PILM.Titania/PILM composites were prepared by an impregnation procedure using titanium tetraisopropoxide (TTIP).Firstly,TTIP was diluted with isopropanol to obtain a clear solution of varying Ti concentrations.This solution was slowly added to aluminum pillared montmorillonite dispersion with constant stirring of 6h at room temperature.The solvent was evaporated at 30°C under vacuum.The solid material was dried at 120°C for 6h,and then calcined at 450°C for 4h (TiO 2/PILM).TiO 2with various Ti loading of 0.2,0.6,1.0,3.0,10.0and 20mass%was prepared.The catalysts were referred as Ti-N/PILM where N indicates the Ti content.2.2.Characterization of support and catalystsA gas sorption analyzer (NOVA 1200e)was used to determine the nitrogen adsorption isotherms at −196°C.The surface area was calculated by the BET equation.A Shimadzu X 6000powder diffractometer with Cu-K αradiation was used to obtain X-ray powder diffraction (XRD)patterns.The titanium content was determined by X-ray fluorescence spectroscopy (XRF).The morphology of materials was observed by scanning electron microscopy (SEM JEOL 820)and transmission electron microscopy (TEM JEOL-JEM,120kV).UV –vis diffuse re flectance spectra were obtained at 25°C on a JASCO UNIDEC model V670spectrometer equipped with an integrating sphere,where a BaSO 4plate was used as a reference.The chemical contents of the prepared materials were measured by XRF and EDX.2.3.Photocatalytic activity testThe photocatalytic activity evaluation of TiO 2/PILM materials was carried out in a batch photoreactor.The reactor was made of a Pyrex glass beaker surrounded by a water jacket and four UV tube lamps (UVB lamp,10W).Methylene blue solution (MB)was prepared at the concentration of 5×10−5M and a volume of 500mL solution was used for reaction.In each run,catalyst content was 1g/L.The concentration of methylene blue during the reaction was determined by photometryTable 1Elemental analysis of material.SampleComponent (wt.%)SiO 2Al 2O 3Na 2O CaO FeO MgO TiO 2Montmorillonite 56.4021.628.250.58 3.58 3.39–PILM51.5237.33 2.520.310.89 1.84–Ti-0.2/PILM 54.2334.34 2.320.090.87 1.310.45Ti-0.6/PILM 51.2937.98 1.820.090.22 1.23 1.27Ti-1.0/PILM 51.6536.11 1.830.090.12 1.3 2.38Ti-3.0/PILM 50.0433.08 1.830.090.33 1.02 6.64Ti-10.0/PILM 45.3827.27 1.10.070.19 1.1218.24Ti-20.0/PILM35.3121.961.10.041.070.9933.46Fig.1.Corelation between Ti content in impregnation solution andcatalysts.Fig.2.XRD pattern of support and catalyst materials (m,Cr,q,A and R represent montmorillonite,crystoballite,quartz,anatase and rutile,respectively).589I.Fatimah et al./Applied Clay Science 50(2010)588–593at 630nm using a UV –visible spectrophotometer (HITACHI-U 2080)and further con firmed by HPLC (Shimadzu E-1003).3.Results and discussion 3.1.Catalyst characterizationTable 1presents the chemical composition determined by ele-mental analysis using EDX and XRF.XRF was used to determine Ti content in the samples and EDX was used to analyze other metalcontents.SiO 2and Al 2O 3were the main components of the mineral support and catalysts.Na content in the minerals was higher than Ca,which suggests that the mineral was sodium montmorillonite.For montmorillonite and PILM,no TiO 2was detected.The loading of TiO 2was 0.5–33.5mass%.Fig.1shows the rela-tionship of TiO 2concentration in preparation solution and actual loading of PILM with TiO 2.The good linear correlation suggests that impregnation of TiO 2on PILM was ef ficiently achieved and no sig-ni ficant TiO 2loss occurred during the preparation.The XRD patterns of montmorillonite showed the characteristic re flections at 2θ=6.3°(d 001=14.9Å)and 2θ=19.9°(d =4.5Å).Other re flections at 21.8°and 26.6°indicated crystoballite and quartz (Fig.2).The XRD pattern of PILM was similar to that of montmorillonite but with a different intensity of the re flections.The (001)re flection indicated a basal spacing of 19.5.Re flections of titania on TiO 2/PILM were not observed when the Ti content was 0.2to 0.6mass%.The position and the intensity of the (001)re flection were not affected.The titania phase was identi fied when its content was N 1.0mass%.At 2θ25.1°,37.7°,and 53.8°the (101),(004)and (105)re flections of tetragonal titania (anatase)were observed.With increasing titania content the (101)re flection of rutile at 27°was found.Titania crystallization at high loading was also observed in the preparation of nanoparticle titania membranes and titania incorpo-ration in gels reported in previous studies (Mogyor όsi et al.,2003),where both anatase and rutile forms were produced.In the pillared montmorillonite anatase was generally formed or sometimes no re flections of titania were found (Gil et al.,2000;Rezala et al.,2009;Ding et al.,2008;Binitha and Sugunan,2006;Bovey et al.,1996).Thus,titania crystallized only at higher TiO 2content while very small TiO 2aggregates were homogeneously dispersed at small particle size on clay mineral surface at low TiO 2concentration.The hydrolysis rate of titanium isopropoxide may affect the titania crystallization in the PILM.At high TTIP concentration,titanium would be fast deposited in gel form before it reached the PILM pores (Dhage et al.,2004).Due to the gel form with larger molecular size,titanium isopropoxide was dif ficult to penetrate into the pores of PILM and the condition would favor the formation of both anatase and rutile during the crystallization process.UV –visible diffuse re flectance spectra revealed the existence of smaller titania particles in the pillared montmorillonite matrix (Fig.3).The spectra showed the blue shift in all TiO 2/PILM samples compared to pure TiO 2spectrum.The edge wavelength of the TiO 2/PILM samples increased with increasing titanium content inPILMFig.3.UV –visible diffuse re flection spectra of TiO 2/PILM.Table 2Edge wavelengths of materials.Sample Edge wavelength (nm)Titania (Merck)380.9Ti-0.2/PILM 336.8Ti-0.6/PILM 338.7Ti-1.0/PILM 348.5Ti-3.0/PILM 348.6Ti-10.0/PILM 380.9Ti-20.0/PILM380.9Fig.4.Nitrogen adsorption –desorption isotherm on support and catalyst materials.590I.Fatimah et al./Applied Clay Science 50(2010)588–593(Table 2).At a high concentration of TiO 2,there was no difference in the edge wavelength between the bulk TiO 2and TiO 2/PILM.A similar observation was reported for TiO 2/ZSM5(Zhang et al.,1998).For the TiO 2/PILM samples with Ti loading between 0.2and 3.0mass%,there was no signi ficant change in the value of band gap energy.The values of the edge wavelengths indicated the formation of smaller titania particles.At higher Ti content i.e.10and 20mass%,the edge wavelength values were similar to TiO 2.The XRD pro files also clearly showed the presence of titania crystals on the surface.For TiO 2on ZSM5,the edge wavelength was also not signi ficantly different from bulk TiO 2(Reddy et al.,2003).At Ti content of 0.2–1.0mass%,the speci fic surface area decreased with increasing titanium content,at high Ti content,the speci fic surface area increased again (Fig.4,Table 3).The adsorption –desorption isotherm indicated that TiO 2/PILM was mesoporous at Ti loading of 0.2to 1.0mass%and micropores were formed at higher titanium contents.The BJH pore size distribution (Fig.5)showed a change of pore size at varying Ti loading.At higher Ti loading,micro-pores were developed.Without Ti impregnation,PILM has a peak of larger pore size between 4and 8nm.At Ti loading of 0.2mass%,no such pore peak occurred,due to packing of small TiO 2particles on the surface and pores,resulting in the reduction in speci fic surface area.When more Ti (N 3mass%)was loaded on PILM,the TiO 2aggregates could also form porous structure but with micropores.The developed micropores would enhance the speci fic surface area of TiO 2/PILM.The SEM images of TiO 2/PILM (Fig.6)showed the layered structure of PILM.The surface morphology of Ti-0.2/PILM was not signi ficantly different from the PILM,but abundant titania particles on the surface of Ti-3.0/PILM and Ti-20.0/PILM were observed.The increase of the speci fic surface area due to the formation of titania particles was also shown by TEM images (Fig.7).Large aggre-gates of titania particles were observed for Ti-3.0/PILM.Similar results were reported for titania-pillared montmorillonite (Yoda et al.,2004).Table 3Result of adsorption –desorption measurement.Sample Speci fic surface area (m 2/g)Micropore surface area (m 2/g)Pore volume ×10−1(cm 3/g)PILM 157.834.50.754Ti 0.292.928.80.651Ti0.675.453.60.847Ti 1.066.654.8 1.452Ti 3.0128.687.30.996Ti 10.0133.494.8 1.309Ti20.0187.8116.01.518Fig.5.Pore size distribution of PILM and TiO 2/PILMcomposites.Fig.6.SEM images of support and catalysts.(a)PILM,(b)Ti-0.2/PILM,(c)Ti-3.0/PILM and (d)Ti-20.0/PILM.591I.Fatimah et al./Applied Clay Science 50(2010)588–5933.2.Photodegradation of methylene blue (MB)The photocatalytic activity of various TiO 2/PILM catalysts in MB photodegradation is presented in Fig.8.From the variation of MBconcentration with time,the degradation kinetics was derived and the first order model (Eq.(1))was found to fit the curves.ln MB ½ =−kt +ln MB ½ 0ð1Þwhere,[MB]and [MB]0are MB concentration in sampling time (t )and initial concentration at t =0,respectively,and k is the kinetic rate constant of the first order reaction.Based on the equation,the degradation rate constants (k )were obtained and are presented in Table 4with equilibrium concentration of MB.PILM showed the lowest degradation rate of MB and its adsorption capacity was also low (b 10%).The TiO 2/PILM samples exhibited varying activity depending on TiO 2loading.The catalytic activity followed the order Ti-20.0/PILM N Ti-10.0/PILM N Ti-3.0/PILM NTi-0.6/Fig.7.TEM pro file of (a)PILM,(b)Ti-1.0/PILM and (c)Ti-3.0/PILM.Fig.8.Kinetic variation of MB photodegradation on various photocatalysts.Table 4Constant of MB degradation rate and concentration in equillibrium.Photocatalyst K (10−3/min)C e (10−5M)PILM 0.826 4.355Ti 0.2 3.327 3.085Ti0.6 3.313 3.215Ti 1.0 1.326 4.111Ti 3.0 6.696 1.145Ti 10.09.2280.345Ti20.016.7410.338592I.Fatimah et al./Applied Clay Science 50(2010)588–593PILM~Ti-0.2/PILM N Ti-1.0/PILM~PILM.Ti-0.6/PILM and Ti-0.2/PILM presented a similar activity and their activities were higher than Ti-1.0/PILM.This is probably due to the textural change and surface distribution of TiO2.Loading of TiO2reduced the specific surface area, which resulted in low adsorption of MB on catalyst surface for the MB to be contacted with TiO2for reaction.For all the catalysts,Ti-1.0/PILM had the lowest specific surface area.In addition,the aggregation of TiO2at1mass%might also reduce its dispersion on PILM,making less amount of active sites than those on Ti-0.6/PILM and Ti-0.2/PILM and thus a lower activity of Ti-1.0/PILM.The Pearson correlation was tested to relate the rate constant with other properties of the catalysts such as titania content,specific surface area,microporous surface area,band gap energy and pore volumes(Table5).The Pearson correlation is the parameter to identify the strength of the linear relationship between two variables. The formula of the Pearson correlation coefficient,r,is given by the following equation(Everitt,2006).r=∑n i=1x i−Àxy i−Ày−ffiffiffiffiffiffiffiffiffiffiffiffiffiffiffiffiffiffiffiffiffiffiffiffiffiffiffiffiffiffiffiffiffiffiffiffiffi∑ni=1x i−Àx2r∑ni=1y i−Ày2ð2Þwhere,x is the parameter of physicochemical character tested,and y is the photocatalytic activity.The specific surface area and pore volume dominantly affected the photodegradation rate,suggesting an adsorption driven mechanism. Furthermore,the titanium content also affected the rate at lower correlation value(0.754).However,the correlation of the band gap energy,though important to photocatalysis,to the rate constant was not high suggesting that the band gap energy was not the determinant parameter of the catalytic degradation of MB.4.ConclusionAluminum pillared montmorillonite is a promising support for titania particles.The small titania particles showed the quantum size effect,and the band gap energy was lower than that of bulk TiO2. However,the photocatalytic activity for methylene blue degradation was not affected by the band gap energy.The results suggested an adsorption driven mechanism.The specific surface area and pore volume strongly influenced the photodegradation rate. AcknowledgementsThe authors would like to express sincere gratitude to the Ministry of Education,Republic of Indonesia through Hibah Penelitian Berpotensi Publikasi Internasional,DP2M-DIKTI2009for thefinancial and technical support to this research.ReferencesAn,T.C.,Chen,J.X.,Li,G.Y.,Ding,X.J.,Sheng,G.Y.,Fu,J.M.,Mai,B.X.,O'Shea,K.E.,2008.Characterization and the photocatalytic activity of TiO2immobilized hydrophobic montmorillonite photocatalysts degradation of decabromodiphenyl ether(BDE 209).Catal.Today139,69–76.Belessi,V.,Lambropoulou,D.,Konstantinou,I.,Katsoulidis,A.,Pomonis,P.,Petridis,D., Albanis,T.,2007.Structure and photocatalytic performance of TiO2/clay nano-composites for the degradation of dimethachlor.Appl.Catal.B73,292–299. Bergaya,F.,Theng,B.K.G.,Lagaly,G.,2006.Handbook of Clay Science,Developments in Clay Science,Volume1.Elsevier Science,Amsterdam.Binitha,N.N.,Sugunan,S.,2006.Preparation,characterization and catalytic activity of titania pillared montmorillonite clays.Micromeso.93,82–89.Bovey,J.,Kooli,F.,Jones,W.,1996.Preparation and characterization of ti-pillared acid-activated clay catalysts.Clay Miner.31,501–506.Daniel,L.M.,Frost,R.L.,Zhu,H.Y.,2007.Synthesis and characterisation of clay-supported titania photocatalysts.J.Col.Inter.Sci.316,72–79.Daniel,L.M.,Frost,R.L.,Zhu,H.Y.,ponite-supported titania photocatalysts.J.Col.Int.Sci.322,190–195.Dhage,S.R.,Choube,V.B.,Samuel,V.,Rav,V.,2004.Synthesis of nanocrystalline TiO2at 100°C.Mat.Lett.58(17–18),2310–2313.Ding,X.,An,T.,Li,G.,Zhang,S.,Chen,J.,Yuan,J.,Zhao,H.,Chen,H.,Sheng,G.,Fu,J.,2008.Preparation and characterization of hydrophobic TiO2pillared clay:the effect of acid hydrolysis catalyst and doped Pt amount on photocatalytic activity.J.Col.Int.Sci320,501–507.Everitt,B.S.,2006.The Cambridge Dictionary of Statistics,Cambridge University Press, 3rd Edition:101.Fujishima,A.,Rao,T.N.,Tryk,D.A.,2000.Titanium dioxide photocatalysis1–21. Fujishima,A.,Zhang,X.,Tryk,D.A.,2007.Heterogeneous photocatalysis:from water photolysis to applications in environmental cleanup.Int.J.Hyd.Ene.32, 2664–2672.Fujishima, A.,Zhang,X.,Tryk,D.A.,2008.TiO2photocatalysis and related surface phenomena.Surf.Sci.Rep.63,515–582.Fukahori,S.,Ichiura,H.,Kitaoka,H.,Tanaka,H.,2003.Photocatalytic decomposition of bisphenol A in water using composite TiO2-zeolite sheets prepared by a papermaking technique.Environ.Sci.Technol.37(5),1048–1051.Gil,A.,Gandía,L.M.,Vicente,M.A.,2000.Recent advances in the synthesis and catalytic applications of pillared clays.Catal.Rev.42,145–212.Gil, A.,Korili,S.A.,Vicente,M.A.,2008.Recent advances in the control and characterization of the porous structure of pillared clay catalysts.Catal.Rev.50(2),153–221.Hisanaga,T.,Tanaka,K.,2002.Photocatalytic degradation of benzene on zeolite-incorporated TiO2film.J.Haz.Mat.93(3),331–337.Kooli,F.,Bovey,J.,Jones,W.,1997.Dependence of the properties of titanium-pillared clays on the host matrix:a comparison of montmorillonite,saponite and rectorite pillared materials.J.Mater.Chem.7(1),153–158.Li,F.,Sun,S.,Jiang,Y.,Xia,M.,Sun,M.,Xue,B.,2008.photodegradation of an azo dye using immobilized nanoparticles of TiO2supported by natural porous mineral.J.Haz.Mat.152(3),1037–1044.Mahmoudi,N.M.,Arami,M.,2009.Degradation and toxicity reduction of textile wastewater using immobilized titania nanophotocatalysis.J.Photochem.Photobiol.B.94(1),20–24.Menesi,J.,Korosi,L.,Bazso,E.,Zollmer,V.,Richardt,A.,Dekany,I.,2008.Photocatalytic oxidation of organic pollutants on titania-clay composites.Chemosphere70, 538–542.Mogyorόsi,K.,Dekány,,K.,Fendler,J.H.,2003.Preparation and characterization of clay mineral intercalated titanium dioxide ngmuir19,2938–2946. Rajeshwar,K.,Osugi,M.E.,Chanmanee,W.,Chenthamarakshan,C.R.,Zanoni,Kajitvi-chyanukul,P.,Krishnan-Ayera,R.,2008.Heterogeneous photocatalytic treatment of organic dyes in air and aqueous media.J.Photochem.Photobiol.C.9,15–36. Rao,V.S.,Rachel,A.,Subrahmanyam,M.,Boule,P.,2003.Immobilization of TiO2on pumice stone for the photocatalytic degradation of dyes and dye industry pollutants.Appl.Catal.B.46,77–85.Reddy,E.P.,Davydov,L.,Smirniotis,P.,2003.TiO2-loaded zeolites and mesoporous materials in the sonophotocatalytic decomposition of aqueous organic pollutants: the role of the support.Appl.Catal.B42(1),1–11.Rezala,H.,Khalaf,H.,Valverde,J.,Romero, A.,Molinari, A.,Maldotti, A.,2009.Photocatalysis with Ti-pillared clays for the oxofunctionalization of alkylaromatics by O2.Appl.Catal.A.352,234–242.Romero,A.,Dorado,F.,Asencio,I.,Garcia,P.B.,Valverde,L.,2006.Ti-pillared clays: synthesis and general characterization.Clays Clay Miner.54(6),737–747. Vicente,M.A.,Belver,C.,Trujillano,R.,Rives,V.,Álvarez,A.C.,Lambert,J.F.,Korili,A., Gandia,L.M.,Gil, A.,2004.Preparation and characterisation of Mn-and Co-supported catalysts derived from Al-pillared clays and Mn-and Co-complexes.Appl.Catal.A.267,47–58.Yoda,S.,Sakurai,Y.,Endo,A.,Miyata,T.,Yanagishita,H.,Otake,K.,Tsuchiya,T.,2004.Synthesis of titania-pillared montmorillonite via intercalation of titanium alkoxide dissolved in supercritical carbon dioxide.J.Mater.Chem.14,2763–2767.Yuan,Peng,Yin,Xiaolin He,Hongping,Yang,Dan,Wang,Zhu,Linjiang,Jianxi,2006.Investigation on the delaminated-pillared structure of TiO2-PILC synthesized by TiCl4hydrolysis method.Micro.Meso.Mat.93(1–3),240–247.Zhang,S.,Fujii,H.,Nosaka,Y.,1998.The dispersion effect of TiO2loaded over ZSM-5 zeolite.J.Mol.Catal.A.129,19–224.Table5Result of Pearson correlation analysis on the effect of physicochemical parameter to the MB photodegradation rate.Factor Pearson correlation(confidence interval=0.95)Titanium content0.754Band gap energy−0.685Specific surface area0.885Micropore surface area0.746Pore volume0.824593I.Fatimah et al./Applied Clay Science50(2010)588–593。