chapter 1 Introduction to Optical Analysis PPT课件
星敏感器光学系统设计

分类号V445 学号******** U D C 密级公开工程硕士学位论文星敏感器光学系统设计硕士生姓名赵超工程领域光学工程研究方向光电仪器与测控技术指导教师伏思华副教授协助指导教师周金鹏副教授国防科学技术大学研究生院二〇一六年十一月Optical System Design of Star SensorCandidate:Zhao ChaoAdvisor:Fu SihuaAssistant advisor:Zhou JinpengA thesisSubmitted in partial fulfillment of the requirementsfor the professional degree of Master of Engineeringin Optical EngineeringGraduate School of National University of Defense Technology Changsha,Hunan,P.R.China(November,2016)目录摘要 (i)ABSTRACT .................................................................................................................. i i 第一章绪论 (1)1.1选题背景和研究意义 (1)1.2 星敏感器简介 (2)1.3 星敏感器光学系统技术发展现状 (2)1.3.1 光学系统参数的确定方法 (3)1.3.2 光学镜头设计 (4)1.3.3 遮光罩设计 (7)1.4 本论文的研究内容 (9)第二章星敏感器光学系统设计需求分析 (11)2.1 星敏感器探测能力分析 (12)2.1.1 星光信号能量 (12)2.1.2 探测器星光能量 (13)2.1.3 星等探测信噪比计算 (13)2.1.4 星探测概率计算 (14)2.2 星敏感器光学系统参数确定 (15)2.2.1 视场、焦距和阈值星等的初步选择 (15)2.2.2 入瞳直径的确定 (19)2.2.3光谱范围和中心波长的确定 (22)2.2.4 其他参数确定 (22)2.3 定位精度对几何像差的要求的定性分析 (24)2.3.1 色差 (24)2.3.2 畸变和彗差 (25)2.3.3 球差、像散和场曲 (26)2.4 本章小结 (26)第三章星敏感器光学镜头设计 (28)3.1 光学系统选型 (28)3.2 光学镜头初始结构确定 (28)3.3 光学镜头优化 (29)3.4 光学镜头像质评价 (33)3.3.1 点列图 (33)3.3.2 光线像差 (35)3.3.3 垂轴色差曲线 (36)3.3.4 调制传递函数 (37)3.3.5 点扩散函数 (38)3.3.6 能量集中度 (39)3.3.7 光学镜头图像分析 (40)3.3.8 光学镜头透过率计算 (41)3.5 温度对光学镜头成像质量的影响 (41)3.4.1 温度变化对光斑大小的影响 (42)3.4.2 温度变化对焦距和后截距的影响 (43)3.4.3 温度变化对能量包围圆半径与能量中心偏移量的影响 (44)3.4.4 温度变化对畸变、垂轴色差和点扩散函数的影响 (46)3.6 公差分析 (46)3.7 光学镜头设计结果 (50)3.8 本章小结 (51)第四章星敏感器光学系统杂散光抑制 (52)4.1 杂散光分析与抑制理论 (52)4.2 星敏感器遮光罩设计 (56)4.2.1 遮光罩参数计算与设计 (56)4.2.2 挡光环设计 (59)4.3 星敏感器光学系统建模 (60)4.4 光学系统杂散光抑制结果 (62)4.5 杂散光抑制结果验证 (64)4.6 遮光罩优化设计 (65)4.7 本章小结 (69)第五章星敏感器光学系统模拟成像分析 (70)5.1 光学镜头模拟成像 (70)5.2 光学镜头视场内杂散光抑制分析 (74)5.3 光学系统视场外杂散光模拟成像分析 (75)5.4 本章小结 (77)第六章总结与展望 (78)6.1 本文完成的工作 (78)6.2 下一步研究计划 (79)致谢 (81)参考文献 (82)作者在学期间取得的学术成果 (87)表目录表2.1 SonyICX285AL探测器参数表 (11)表2.2 不同视场下不同阈值星等的探测概率 (15)表2.3 SKY2000星表中不弱于M V的全天星数的数目 (18)表2.4 不同视场下的焦距 (18)表2.5 不同视场不同入瞳直径下的设计难度系数C (20)表2.6 不同阈值星等不同入瞳直径下的信噪比SNR (20)表2.7 方案1不同孔径光学系统信噪比和设计难度 (21)表2.8 方案2不同孔径光学系统信噪比和设计难度 (21)表2.9 方案3不同孔径光学系统信噪比和设计难度 (21)表2.10 方案4不同孔径光学系统信噪比和设计难度 (21)表2.11 四种方案探测到恒星的概率和星表大小 (22)表2.12 弥散斑圆大小对内插星象中心的影响 (23)表2.13 光学系统设计参数表 (24)表3.1 光学系统各视场弥散斑直径 (34)表3.2 垂轴色差数据 (37)表3.3 包围85%和90%能量时半径大小 (40)表3.4 不同温度4个半视场下的光斑均方根半径值/μm (42)表3.5 不同温度下光学镜头焦距值 (44)表3.6 不同温度下镜头焦距值相对于20°C时焦距值的变化值 (44)表3.7 不同温度时光学系统后截距参数及其移动量 (44)表3.8 4.949°视场不同温度下包围85%和90%能量的包围圆半径 (45)表3.9 不同温度引起的不同半视场能量中心偏移 (46)表3.10 不同温度下垂轴色差最大值 (46)表3.11 光学镜头主要参数 (51)表4.1 挡光环高度和位置表 (61)表4.2 杂散光追迹值表 (63)表4.3 优化结构挡光环高度和位置表 (67)表4.4 优化结构杂散光追迹值表 (68)表5.1 竖直轴不同视场星象能量中心位置 (73)表5.2 竖直轴方向不同视场星象能量提取位置 (75)图目录图2.1 探测器量子效率曲线图 (11)图2.2 不同视场下不同阈值星等的平均星数目 (17)图2.3 阈值星等5.5时不同视场下探测到不同数目的星的概率 (17)图2.4 10°视场下不同阈值星等探测到不同星的数目的概率 (17)图2.5 不同视场下难度系数C与口径直径的关系曲线 (20)图2.6 不同阈值星等不同口径下的信噪比SNR曲线图 (21)图3.1 初步优化光学系统2D结构图 (30)图3.2 初步优化光学系统点列图 (30)图3.3 初步优化光学系统传递函数曲线 (31)图3.4 初步优化光学系统点扩散函数 (31)图3.5 初始光学系统能量分布图 (31)图3.6 优化后光学镜头数据 (32)图3.7 优化后光学系统2D结构图 (32)图3.8 优化后光学镜头数据 (33)图3.9 优化后光学系统点列图 (34)图3.10 光线像差曲线 (35)图3.11 光程差曲线 (35)图3.12 优化后光学系统场曲与畸变曲线 (36)图3.13 垂轴色差曲线 (36)图3.14 光学系统传递函数曲线 (38)图3.15 点扩散函数图 (39)图3.16 优化后光学系统包围能量曲线 (39)图3.17 原始星点图像 (40)图3.18 4.949 星象示意图 (41)图3.19 -30°C、-10°C、10°C、20°C、30°C和50°C的点列图 (43)图3.20 4.949°半视场不同温度下包围85%和90%能量的包围圆半径 (45)图3.21 光学镜头公差分析时公差设置 (47)图3.22 公差分析方法设置 (48)图3.23 灵敏度分析 (48)图3.24 蒙特卡罗分析结构 (49)图3.25 蒙特卡罗统计 (49)图3.26 光学镜头3D图 (50)图3. 27镜头各单个镜片参数 (51)图4.1 表面BRDF定义图 (53)图4.2 AB g模型示意图 (53)图4.3 基本能量方程传输图 (54)图4.4 星敏感器遮光罩设计流程图 (56)图4.5 星敏感器与杂光源夹角示意图 (57)图4.6 遮光罩结构示意图 (57)图4.7 延拓后的遮光罩结构 (59)图4.8 挡光环位置结构确定示意图 (59)图4.9 结构示意图 (61)图4.10 遮光罩与光学系统剖面图 (61)图4.11 辐照度图 (62)图4.12 PST曲线 (63)图4.13 30°杂散光光线追迹图 (64)图4.14 优化结构示意图 (65)图4.15 优化结构示意图 (67)图4.16 光线追迹示意图 (68)图4.17 PST曲线 (69)图5.1不同视场星场成像模拟 (70)图5.2 光学系统整体结构图 (70)图5.3 模拟成像光线追迹 (71)图5.4 探测器模拟星象图 (71)图5.5 探测器星象能量分布 (72)图5.6 探测器能量分布 (72)图5.7 光学镜头设置光阑后光线追迹 (74)图5.8 视场内杂散光抑制后探测器能量分布 (75)图5.9 光学系统光线追迹 (75)图5.10 探测器模拟星象图 (76)图5.11 探测器杂散光模拟成像 (76)图5.12 探测器平滑后模拟星象图 (77)摘要星敏感器是以恒星为探测对象的高精度空间姿态测量装置,已被广泛应用于航天、航空、航海、制导等领域。
光电子技术专业英语
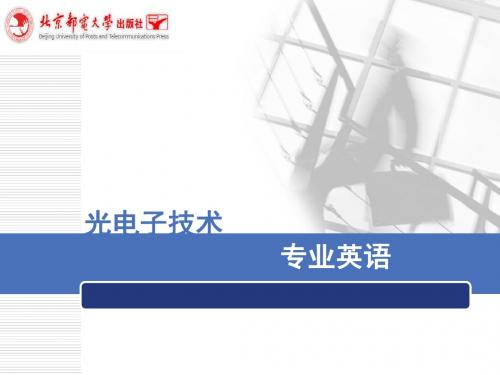
3. 增减词译法
由于两种语言表达方式不同,在把原文信息译成译文信息时,常常 需要删去或增添一些词。这样做并不损害原意,反而可以使译文更 为通顺,意思更为清楚。
如:You cannot build a ship, a bridge or a house if you don’t know how to make a design or how to read it.不会制图或看不懂图纸,就不可能造船、 架桥或盖房子。汉译中省略代词you和it、冠词a、连接词if和副词how。
5. 反译法
由于汉英两种语言表达习惯不同而且均可以从正面或反面来表达同一概念, 翻译时如果用正面表达译文有困难,欠通顺,则不妨用反面表达,或将反面 表达改用正面表达,这样可以使译文比较通顺而与原意并无出入。
如:I lay awake almost the whole night.我几乎一夜没睡着。例属正说反译。 I can’t agree with you more.我极其赞同你的意见。本例属反说正译。
1.2科技英语文体总貌
科技英语要求客观性、准确性和严密性,注重叙事逻辑上的 连贯(Coherence)及表达上的明晰(Clarity)与畅达(Fluency), 避免行文晦涩。 科技英语力求平易(Plainness)和精确(Preciseness),避免使 用旨在加强语言感染力和宣传效果的各种修辞格
光学显微镜PPT课件
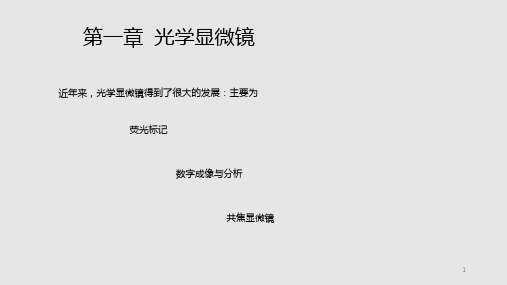
第一章 光学显微镜
第0节 预备知识
Thin Lenses
paraxial rays, in air
11 so si
if the lens is “thin,” then
(nlens
1)
1
R1
1 R2
Thin Lens Equation
11 1
so si
f
Gaussian Lens Formula
cancel some aberrations
21
Collection Efficiency
22
We Forgot Collection Efficiency
So, now we couple this system, and find out that we have too little light striking the tissue … what went wrong?
optic axis
15deg sin(.262 rad) 0.259 10 deg: sin(.175 rad) 0.174 5 deg: sin(.873 rad) 0.872 1 deg: sin(30.5 mrad) 30.5 E-3
30 deg: sin(.524 rad) .5 45 deg: sin(.785 rad) .707
object is REAL
when rays diverge from object:
so
so > 0
object is VIRTUAL when rays converge to object:
so < 0 so
usually only with lens combination1s7
高级激光雷达系统的大口径望远镜

Large aperture telescope for advanced lidar systemFrancesca SimonettiAlessandro Zuccaro MarchiLisa GambicortiVojko BratinaPiero MazzinghiNational Institute of Optics-National Research Council͑INO-CNR͒Largo Enrico Fermi6-50125Firenze,ItalyE-mail:francesca.simonetti@ino.it Abstract.Thefinal optical design for a space-borne light detection and ranging͑lidar͒mission is presented,in response to the European Space Agency“Advanced lidar concepts”proposal for use of a differential ab-sorption lidar system to measure water vapor distribution in atmosphere at935.5nm.The telescope adopts a double afocal concept͑i.e.,four reflections with two mirrors͒using a lightweight and large aperture pri-mary mirror.It is derived from a feasibility study that compares several different optical configurations,taking into account parameters such as cost,dimensions,complexity,and technological feasibility.Thefinal tele-scope optical design is described in detail,highlighting a trade-off with other solutions and its optical tolerances.©2010Society of Photo-Optical Instru-mentation Engineers.͓DOI:10.1117/1.3461976͔Subject terms:reflective optical system;large aperture telescope;space optics; lidar.Paper090508RR received Jul.9,2009;revised manuscript received Mar.30, 2010;accepted for publication May17,2010;published online Jul.14,2010.1IntroductionThe European Space Agency͑ESA͒call for“Advanced Li-dar Concepts”has been met with a proposal in the form of a feasibility study for the development of advanced tech-nologies of a light detection and ranging͑lidar͒system, devoted mainly to the implementation of telescopes with large aperture and active control,in order to retrieve a suf-ficient signal from the laser transmitter.The main advantages of using lidar systems from space are their high spatial,temporal,and spectral resolution. Successful space-based lidar missions have already gener-ated detailed profilometry of clouds and aerosols over earth and exquisite topographical maps.Large collection area receivers are needed for space-based lidars—with laser power transmission inversely pro-portional to the aperture size,a large aperture receiver tele-scope allows us to decrease the necessary laser power. Unfortunately,these systems are expensive to produce and must be suitable for launch into small fairings,typical of small satellites.Furthermore,the mirrors must guarantee a structural integrity during launch and operations,and there-fore,stiff and lightweight structure must be realized.The resulting concept for the primary mirror is a segmented geometry with a mass/area density goalϽ16kg/m2:a cen-tral portion,locked to the bus of the satellite,surrounded by a set of petals stored during launch and to be opened before the operational phase in space.In addition,the materials selected must also have good thermal characteristics in or-der to minimize the distortion in mirror shape.In this context,this paper presents a description of pre-liminary candidate designs of optical configuration for the lidar receiver subsystem.After an analysis of ESA requirements,a brief descrip-tion of the evolution of the design is given,analyzing the telescope concepts together with the auxiliary optics for filter and wavefront sensor stages.Some of the require-ments,such as those for thefilter,have been achieved even for the early stages of the layout.The overall constraints, such as dimensions,complexity,and technological feasibil-ity,have been considered and optimized step-by-step,up to thefinal configuration,which associates the optical perfor-mances with the satisfaction of all the requirements.A trade-off analysis shows how this design͑the“baseline”͒has been chosen,for which particular effort in optical tol-erances has been dedicated.As will be described,the baseline shows near diffraction-limited optical performances and an interesting combination of the other constraints.For this reason,the technology proposed in this paper,and generically for this ESA project,can be potentially extended even further,be-yond the specifications of the present project and toward a more generic usage—for instance,in large space telescopes for astronomical applications needing high-performance imaging.The complete characterization of the whole structure has been accomplished,leaving the description of the devel-oped technologies to other papers.12Requirements AnalysisThe definition of the optical design here presented has been driven by the scientific requirements for the analysis of the water vapor distribution in atmosphere from space-based lidar instrumentation͑Table1͒.All the requirements in terms of input aperture,field of view͑FOV͒,output angle, and image size can be achieved simultaneously for water vapor emission,because they are compliant with the optical invariant.Indeed,according to the Helmholtz2invariantD i·␣iഛD out·␣out,where␣i is the FOV͑corresponding to0.0033deg͒,D out and␣out are respectively the beam diam-eter and the acceptance angle at thefilter stage,by consid-ering the requirement on the effective aperture area,and D i0091-3286/2010/$25.00©2010SPIEOptical Engineering49͑7͒,073001͑July2010͒has been taken as 4000mm in order to include the effect of petals ͑i.e.,each single petal has 1m 2area,and the central hexagonal portion is 2m 2͒.During operation after launch and deployment,the /3wavefront error requirement is achieved by assigning dif-ferent values of wavefront errors for every optical compo-nent preceding the filter,depending on dimensions and types of optics.For instance,assigning the following sur-face error contribution:M 1=/8,M 2=/10,M 3=/20,the overall wavefront error w satisfies the system specifications W =/3.The maximum allowed error is achievable for the pri-mary mirror operation also due to the use of active control through suitable linear actuators 3͑1mm stroke and 5m accuracy ͒.This has been the topic of further studies about the overall space mission,including the deployment issue of the petals.3Selection of Optical SystemA preliminary study considered two different lidar optical architectures:a multipupil system ,i.e.,an array of tele-scopes realizing the required clear aperture as a sum of the clear pupil diameters of each single telescope,and a single-pupil system ,4–6formed by one suitable single telescope.All the cited optical configurations have been analyti-cally studied at the first order and successively optimized at the third order.The final optimizations and ray tracing simulations were performed with ZEMAX EE software ͑ZEMAX Development Corporation ͒.3.1Multipupil SystemThis system is formed by a set of seven subsystems,each having 1/7of the whole aperture.Therefore,all small tele-scopes behave equally,directing collimated light into the filters.The filter stage can be thought of as the combination of one filter for each small telescope or,alternatively,as asingle filter for the whole telescope array.The layouts are shown in Fig.1,where in the second configuration,the signal is brought by fibers.The optical configuration of the single telescope is an f/1afocal Galileian,formed by two confocal ͑one positive and one negative ͒parabolic mirrors.The telescope has the func-tion of beam reducer ,from 1000mm entrance beam diam-eter to the required exit diameter ͑Fig.2͒.Table 1Optical requirements for water vapor emission.Requirement Value Wavelength 935.5nm Field of view ͑FOV ͒115rad Beam diameter at filter stage Ͻ100mm Acceptance angle at filter stage±6mrad Collecting efficiency Ͼ95%Transmission up to filter stageϾ80%Obscuration Ͻ5%Effective aperture area Ͼ7m 2Wavefront error at filter stage Ͻ/3F/number at wavefront sensorϾ30Fig.1Multipupil system:one filter stage per telescope ͑left ͒;a single filter stage for the whole array ͑right ͒.(b)(a)Fig.2͑a ͒On-axis afocal multipupil system layout and ͑b ͒spot dia-gram on filter stage.On-axis and maximum field:one subsystem.In this preliminary design stage,the solutions are com-pliant to the technical requirements of the mission,being practically diffraction limited and free of any geometrical aberration within the requested FOV and wavelength range.Although the one-filter case is more convenient for weight,tolerance issues,and costs,the case of separate filters for each telescope seems preferable,since the single-filter so-lution would need a nontrivial optical recombination stage.3.2Single-Pupil SystemFor the preliminary study,some optical single-pupil con-figurations with effective collecting area corresponding to 7m 2were proposed to satisfy the optical requirements:off-axis afocal and on-axis focal/afocal designs.The off-axis afocal telescope is formed by three aspheric off-axis mirrors,meaning no obscuration but a higher real-ization risk and cost ͑Fig.3͒.Still,the filter specifications ͑Table 1͒are not completely fulfilled,even with a very small FOV ,although this configuration could have been attractive in terms of whole system of deployment,vehicle integration,and low sensitivity to mechanical tolerance.The on-axis focal telescope is made of two aspheric mir-rors,a beamsplitter,and a suitable set of lenses,located before the telescope focal planes:one beam crosses a posi-tive lens,which collimates the beam at the desired dimen-sions at the filter stage,and the other beam crosses a nega-tive aspheric lens,which focalizes it as requested.As for the multipupil system,the single pupil also can be thought of as on-axis afocal,with a total collecting area of 7m 2.In this case,the telescope is formed by two parabolic mirrors,which give an afocal image directly on the filter,reducing the collimated beam dimension from the primary mirror up to the filter size,as described earlier.This system satisfies the filter requirements.Being on-axis,these configurations are probably simpler to build and control with respect to the off-axis one,and optical,mechanical,and thermal tolerances can be identi-fied more easily.3.3Single-versus Multipupil SystemIt is quite clear that the multipupil configuration is complex from the point of view of holding and alignment of all the telescopes together with the filters,and the use of fibers in case of common relay optics up to the filter’s stage can degrade the single-vs.multipupil system of the overall system.Moreover,this solution has high costs,volume,and mass,and generally feasibility risks in space.Therefore,the development of the study shall be addressed to the single-pupil case,and the second part of this study is devoted to the analysis of particular single-pupil,on-axis afocal,and focal configurations.4Candidate Optical ConfigurationsDifferent candidate on-axis optical solutions have been in-dividuated and are here presented:focal telescope,afocal Galilean telescope,double afocal telescope ͑four mirrors ͒,and double afocal telescope ͑two mirrors ͒.The figures rela-tive to the proposed configurations show both the 2-D and the 3-D case,where division in petals is evident.In the frame of the ESA project,in order to fulfill all the requirements of Table 1,the telescope must be coupled with some auxiliary optics.In particular,the auxiliary lens was chosen for the designs to obtain f /#ജ30on a Pyramid 7wavefront sensor,since the smaller the angular size of the impinging light,the higher the measurement accuracy of the sensor.4.1Focal TelescopeThe first studied design is the on-axis focal telescope.A possible design considers an f/10telescope configuration followed by a negative lens that gives the f/30condition at the wavefront sensor with diffraction limit.After the beam-splitting,the collimating lens is conveniently located in or-der to achieve the suitable beam diameter at the filter stage ͑Fig.4͒.The telescope is formed by a 4m-diam primary and 0.3m secondary aspheric mirrors,with about 3m interdis-tance.The negative lens must be aspheric for aberrations issues;the other optical path is folded to reduce the dimen-sions of the auxiliary optics,so that the overall longitudinal length is about 3.7m.(a)(b)Fig.3Off-axis afocal telescope layout:͑a ͒general and ͑b ͒detail.4.2Afocal Galilean TelescopeThe telescope is formed by two parabolic mirrors ͑reflective-Galilean8type,also called Mersenne8,9͒,with 4m diam of the primary,0.25m of the secondary,and3m interdistance.It is followed by an aspheric lens that con-verges the beam toward a beamsplitter cube͑Fig.5͒,which in turn splits the light in two parts:one is focused to the wavefront sensor,and the other is sent to a collimating spherical lens͑through a folding mirror and an angularfield diaphragm͒andfinally to thefilter stage with the proper quality image͑Table1͒.Aspheric lens,beamsplitter cube, folding mirror,diaphragm,and spherical collimating lens form the auxiliary optics subsystem,the two lenses mod-eled in a refractive-Keplerian10design.Thefirst diopter is made aspheric for partially correcting spherical aberrations of the system up to the needed optical quality.Because of the design and the smallfield of view,astigmatism,field curvature,and distortion are almost zero,and coma contri-bution is not corrected but it does not influence the required performance.A disadvantage of this configuration is the overall size of the back optics:about2m long and6m wide.So de-signed,the telescope performance itself is not enough to have a collimated beam with the properϽ100mm diam-eter.This goal has been accomplished with the two lenses.(a)(b)Fig.4On-axis focal telescope with auxiliary optics layout.͑a͒2-D;͑b͒3-D.(a)(b)Fig.5Afocal telescope with auxiliary optics layout.͑a͒2-D;͑b͒3-D.However,the dimensions of the auxiliary optics system de-pend on the working conditions of thefirst lens—already, an f/15lens,which is out of the required working condi-tions of the wavefront sensor,makes the optical system cumbersome͑Fig.5͒.To maintain a compact instrumentation box,some other options may be possible—for instance,folding the beams or considering a smaller secondary mirror͑which could reach the f/#working condition͒—but this element would become more critical.4.3Double Afocal Telescope(Four Mirrors)To reduce the auxiliary optics dimensions,another option for the telescope was configured:a“double afocal”tele-scope͑four mirrors͒.11This layout gathers two afocal tele-scopes͑thefirst made of two parabolic mirrors,the second of two spherical ones͒and a beamsplitter that divides the beam in two parts,one going to thefilter stage͑the quality image satisfying the requirements͒,and the other converg-ing to the wavefront sensor stage by means of a spherical lens͑Fig.6͒.The longitudinal length of the telescope is 3m,the maximum aperture is4m,and the auxiliary optics is very compact due to three folding mirrors.The image quality is diffraction-limited͑within80m Airy disk diameter͒over all thefields of view͑Fig.7͒, while Strehl ratios are67%on-axis and75%at maximum FOV͑Fig.8͒.Also the quality image of thefilter stage satisfies the requirements of Table1.This solution collects the benefits of having many de-grees of freedom to deal with,in the form of many inde-pendent optical surfaces.Indeed,performances are achieved quite well.In addition,only one spherical lens is needed for focusing at the wavefront sensor stage.4.4Double Afocal Telescope(Two Mirrors)The idea is to reduce the number of mirrors͑and therefore costs and weight͒while keeping good performances at the filter stage—the telescope becomes a combination of only two parabolic mirrors with four reflections͑twice per mir-ror͒,another version of the double afocal telescope͑Fig.9͒, with a1.5%obscuration area͑see Table1͒.Complexity,costs,mass,and overall criticalities are consequently reduced.Like the previous configuration,af-ter the afocal system,a beamsplitter sends half of the beam to thefilter stage and the other half to the wavefront sensor stage,converging by means of a spherical lens.The auxiliary optics is very compact,as before.The in-novation lies in the simplified afocal system:the exit beamdiameter isϽ50mm͑from a4000mm entrance diameter, by using just two parabolic mirrors͒,and even at maximum field,the beam falls within the allowablefilter stage dimen-sions and acceptance angle͑Table2͒.In addition,the f/#=35at the wavefront sensor is achieved through a simple spherical lens.This configuration gives good image quality:diffraction-limited over all thefield of view͑80m Airy disks diam-eter͒on the wavefront sensor stage;image quality at the filter stage within the requirements͑Fig.10͒;and99.8% Strehl ratio on-axis and94%at maximum FOV͑Fig.11͒.5Trade-Off and Baseline SolutionA summary of the proposed designs is discussed here,high-lighting in particular the methods and the values of thedriving parameters used for the trade-off activity:filterspecifications,complexity͑i.e.,the number of optical sur-faces͒,size͑meaning length and volume͒,estimated costs ͑for optics and mechanics,considering surface dimensions and spherical/aspheric shape͒,wavefront sensor specifica-tions,and criticality͑estimating the risks of technologicalrealization͒.In order to select the baseline,a trade-off table(a)(b)Fig.6Two afocal telescopes with auxiliary optics layout.͑a͒2-D;͑b͒3-D.has been compiled͑Table3͒by attributing scores to the previously mentioned parameters͑Table2͒using the crite-ria here described.For thefilter and wavefront sensor specifications,theϮscores are associated to an optical configuration satisfying/ not satisfying them,respectively.For complexity,theϩscore is given when the telescope mirrors are two andϪwhen they are four.Likewise,for size,Ϫis given for the maximum volume andϩfor the minimum volume of the whole system.Similar criteria are used for cost and criti-cality.Notwithstanding the compliance to the requirements of thefilter stage for all the designs͑they are practically diffraction-limited within the requested FOV and wave-length͒,the afocal Galileian configuration hardly satisfies the wavefront sensor specifications with acceptable auxil-iary optics dimensions,while cost and manufacturability are also driven by the presence of an aspheric lens as large as the secondary mirror.The presented design with focal telescope,instead,sat-isfies all the requisites.However,the negative lens,needed to achieve the required f/#and the diffraction limit,is sen-sitive in terms of manufacturing and positioning12in the optical path with respect to the collimating lens of the other path.While the focal case may need aspheric͑not parabolic͒mirrors,which can be a critical issue,for instance,for the optical͑interferometric͒tests,for the two other systems with afocal telescopes,the requirements of thefilter stage and wavefront sensor are achieved by using only spherical and parabolic mirrors.In addition,from a4000mm en-trance beam diameter,a100mm exit beam diameter and an f/#Ͼ30on the wavefront sensor͑see Table1͒have been reached more easily with systems with an afocal telescope whose size is more compact than for the focal one.Further-more,the lens positioning in the collimated beam of the afocal cases is more relaxed and could be anyway compen-sated by detection system refocusing.About the two double afocal telescopes͑with four and two mirrors͒,a comparison of their tolerances͑see Sec.6͒shows that in both cases,the error budget is comparable. Tolerance issues being similar,an additional mechanical support structure of the fourth mirror would yield more obstruction͑i.e.,energy loss͒.On the other hand,having only two mirrors,the alignment maintenance is easier and the overall optomechanical complexity,in terms of cost and mass,is lower.Therefore,the best solution is a double afocal telescope with two mirrors and four reflections—for these reasons,it is the baseline.6Tolerance AnalysisOptical tolerance analysis is needed to evaluate the effects due to any error source on the real optical elements.It involves four fundamental processes:•Thefirst step is thefirst-order analysis,consisting of establishing the tolerance on the main parameters such as focal length of the single elements simulated as thin(a)(b)Fig.7Spot diagrams͑a͒onfilter stage and͑b͒on wavefront sen-sor.On-axis and maximumfield.Fig.8PSF on wavefront sensor on maximumfield.lenses,interdistance,and shifts/tilts with respect to the reference axis.•The second step is a sensitivity analysis of system per-formance,i.e.,evaluation of sensitivity for each pa-rameter.This analysis allows us to tackle the issue of each element’s weight on the overall performance͑im-age quality,alignment,etc.͒.•Third,an error budget13is needed for the determina-tion of the maximum error for all the parameters while keeping performance specifications within acceptablecost goals.In practice,the adopted criteria are neces-sary for an appropriate distribution of maximum ac-ceptable errors͑tolerance͒,whose values are smaller if the relative sensitivity is larger.This part influences many aspects of a project,from top-level performance specifications and cost targets to assembly and align-ment procedures.•The last procedure is a check of tolerance budget,us-ing a Monte Carlo analysis͑via ZEMAX software͒that randomly selects the maximum tolerance errors, thus obtaining a statistics of values within perfor-mance specifications.Optical tolerance analysis was performed for the base-line and for the four-mirrors configuration.It can be gener-ally stated that for both analyses,the secondary mirror is the most sensitive element,as the error budget analysis highlights,where the maximum acceptable errors for all the elements have been reported.In particular,the tolerance budget results for the two designs are described in Tables4 and5,with an indication of the tolerance that a parameter relative to an optical element must have to satisfy the most stringent requirement͑i.e.,the one on the wavefront sen-sor͒.In order to maintain a diffraction-limited image on the wavefront sensor,for the baseline and for the four-mirrors configuration,respectively,the maximum acceptable error on curvature radii is aboutϮ20m andϮ17m,while on distances between mirrors,it isϮ10m andϮ8m.Inboth cases,for decenters and tilts,the secondary mirror tolerances are tight,while for the lens,the maximum allow-able movements are more relaxed.The structural stability is not able to ensure alone the required level of precision given by the tolerance studies. While the active control of the primary mirror also man-ages its curvature tolerances,the support structure of the secondary mirror can be equipped with a suitable device(a)(b)Fig.9Afocal telescope͑two mirrors,four reflections͒with auxiliary optics layout.͑a͒2-D;͑b͒3-D.Table2Trade-off of candidate optical configurations.ConfigurationAfocalGalileantelescopeFocaltelescopeDoubleafocaltelescope͑fourmirrors͒Doubleafocaltelescope͑twomirrors͒Filter specificationsϩϩϩϩComplexityϩϩ—ϩLongitudinal size——ϩϩMass00—ϩCost——0ϩWavefront sensorspecifications—ϩϩϩCriticality—00ϩFinal valuation—ϩϩϩϩϩthat works on the micrometric mirrors’interdistance,tilts, and decenters,while a possible detection system movement may recover defocus.7ConclusionsThis research has been a good opportunity to develop,in the framework of the given optomechanical requirements for the“Advanced Lidar Concepts”ESA call,an innova-tive,lightweight,large-aperture space-borne system,com-pliant with the proposed mission in terms of optical quality in a relatively easy manner.The single-pupil system has been proposed in four configurations,differing from one another because of complexity,weight,manufacturability issues,tolerances of the elements,and overall volume.The comparison of the performances has been studied and is here listed,the trade-off showing that the double afocal telescope with two mirrors is currently the best solution.Table3Main parameters for candidate optical configurations.ConfigurationAfocalGalileantelescopeFocaltelescopeDoubleafocaltelescope͑fourmirrors͒Doubleafocaltelescope͑twomirrors͒Filter specifications70mm70mm45mm45mmComplexity2242 Longitudinal size5m 3.7m3m3mWavefront sensorspecificationsf/#=15f/#=30f/#=32f/#=35Table4Tolerance error budget for the four-mirror configuration,with third-order͑left͒andfirst-order͑right͒layouts.Surface no.Radius(mm)±TOLThickness(mm)±TOLDecenters(mm)Tilts xy(deg) 1:Primarymirror6400-0.015+0.02-3000-0.01+0.007--2:SecondaryMirror400-0.02+0.015305010±0.006±0.002 3:Tertiarymirror50001040.793-±1±0.08 4:Fourthmirror10001090010±1±0.4 7:Lens1026.510---0.0167(a)(b)Fig.10Spot diagrams͑a͒onfilter stage and͑b͒on wavefront sen-sor.On-axis and maximumfield.Fig.11PSF on wavefront sensor at maximumfield.It has been stated that small misalignments and/or varia-tions on nominal values for some parameters can be ac-cepted in terms of tolerances.The system here introduced is somehow “frozen.”However,it may happen that such a complex optics,especially the primary lightweight seg-mented mirror with movable petals,needs some adjust-ments that may exceed the calculated tolerances.A solution based on active movement of the mirror has been deeply investigated during the overall feasibility study for the lidar system.Since the overall results in the baseline show near diffraction-limited performances,the proposed optical con-figuration can be potentially extended even beyond the specifications of the present project—for instance,for new-generation,high-performance imaging space telescopes for astronomical applications—by using large-aperture,light-weight,deployable,and actively controlled primary mir-rors.AcknowledgmentsThe authors want to thank Dr.Joao Pereira do Carmo of ESA/ESTEC for his support and all the ALC collaboration for the important contributions at this design phase of the ESA-ALC project.References1. A.Zuccaro Marchi,L.Gambicorti,F.Simonetti,P.Salinari,F.Lisi,A.Bursi,M.Oliver,and D.Gallieni,“A technology demonstrator for development of ultra-lightweight,large aperture,deployable tele-scope for space applications,”in Proc.7th Int.Conf.Space Optics ,CNES,Toulouse,France ͑2008͒.2.M.Born and E.Wolf,“Elements of the theory of diffraction,”Chap-ter 8in Principles of Optics ,M.Born and E.Wolf,Eds.,pp.370–458,Cambridge University Press,New York ͑1980͒.3.P.Mazzinghi,V .Bratina,L.Gambicorti,F.Simonetti,A.ZuccaroMarchi,D.Ferruzzi,P.Salinari,F.Lisi,M.Oliver,A.Bursi,and J.Pereira do Carmo,“An ultra-lightweight,large aperture,deployable telescope for advanced lidar applications,”in Proc.6th Int.Conf.Space Optics ,ESTEC,Noordwjik,The Netherlands ͑2006͒.4.Y .Y .Gu,C.S.Gardner,P.A.Castleberg,G.C.Papen,and M.C.Kelley,“Validation of the Lidar In-Space Technology Experiment:stratospheric temperature and aerosol measurements,”Appl.Opt.36͑21͒,5148–5157͑1997͒.5. D.M.Winkler,R.H.Couch,and M.P.McCormick,“An overview of LITE:MASA’s Lidar In-space Technology Experiment,”84,164–180͑1996͒;available at /n_overview.html#inst_design.6. E.V .Browell,S.Ismail,and W.B.Grant,“Differential absorptionlidar ͑DIAL ͒measurements from air and space,”Appl.Phys.B 67,399–410͑1998͒.7.S.Esposito and A.Riccardi,“Pyramid wavefront sensor behavior in partial correction adaptive optic systems,”369,L9–L12͑2001͒.8. D.Korsch.Ed.,“Third-order correction of two-mirror systems,”Chapter 8in Reflective Optics ,pp.151–205,Academic Press,San Diego ͑1991͒.9.R.N.Wilson,“Historical introduction,”Chapter 1in Reflecting Tele-scope Optics I ,pp.1–15,Springer,New York ͑1996͒.10.W.B.Wetherell,“Afocal systems,”Chapter 2in Handbook of Optics ,2nd ed.,vol.2,M.Bass,Ed.,pp.2.1–2.23,McGraw-Hill,New York ͑1995͒.11.J.M.Sasian,“Flat field,anastigmatic,four-mirror optical system forlarge telescopes,”Opt.Eng.26͑12͒,1197–1199͑1987͒.12.W.B.Wetherell,“The calculation of image quality,”Chapter 6inApplied Optics and Optical Engineering ,vol.VIII ,R.R.Shannon and J.C.Wyant,Eds.,pp.171–315,Academic Press,London ͑1980͒.13.R.H.Ginsberg,“Outline of tolerancing ͑from performance specifi-cation to tolerance drawings ͒,”Opt.Eng.20͑2͒,175–180͑1981͒.Francesca Simonetti graduated in optics ͑specializing in space optics ͒from the Uni-versity of Florence ͑Italy ͒in 2004.She has performed commissioned work on optical design for the National Institute of Applied Optics ͑CNR-INOA,Florence ͒and Galileo Avionica Spa,sponsored by the Italian Space Agency ͑ASI ͒,within the CIA ͑“Hyper-spectral Advanced Camera”͒project ͑super-visor Dr.Andrea Romoli ͒.Since 2004,she has been working at INOA as a contractor,where she has been involved in optical designs for various systems devoted to space applications.In 2007,she also attended an 8-month stage at Galileo Avionica ͑supervisor Dr.Romoli ͒,for a fea-sibility study of a space telescope working in pushbroom mode for earth observation with a hyperspectral camera.Since 2008,she has been working at CNR-INOA for “Solar Thermal at High Rendering,”with Regione Toscana,developing a high-efficiency solar concentra-tor,with adaptive optical techniques,adopted in the most recent astronomicaltelescopes.Alessandro Zuccaro Marchi graduated in physics from the University of Padova in 1999and received a PhD in physics from the University of Trieste in 2003.Between 2000and 2004,he spent 24months at the University of Alabama in Huntsville and at NASA–Marshall Space Flight Center to work on the optical configuration of a space telescope for detection of ultra-high-energy cosmic rays.Since 2004,he has been working ͑as a postdoctorate and then as aresearcher ͒in the Aerospace Optics Group of the National Institute of Applied Optics ͑CNR-INOA,Florence,Italy ͒,involved in optical testing and design of space projects for fluid science ͑FSL Lab on the ISS/Columbus ͒,astrophysics ͑EUSO telescope for ultra-high-energy cosmic rays ͒,and development of new technologies,in col-laboration with various Italian and internationalinstitutions.Lisa Gambicorti graduated in physics ͑specialization in astrophysics ͒in 2003from the University of Firenze,with supervisors Dr.Mazzinghi ͑CNR-INOA,National Insti-tute of Applied Optics ͒and Dr.Pace ͑Firenze University ͒.In 2007,she received a PhD in astronomy working on wide-field optical systems and designing and testing of optical adapters prototypes for ultra-high-energy cosmic rays detection.She then took a postdoctorate position at CNR-INOAmainly to design the spectropolarimeter for the space telescope World Space Observatory,with the collaboration of Galileo Avionica,and she collaborates with the Department of Astronomy and Space Science of Firenze University and the INFN National Laboratories of Frascati.Since 2008,she won a postdoctorate position at CNR-INOA for project “Solar Thermal at High Rendering,”with Regione Toscana,developing a high-efficiency solar concentrator,based on adaptive optical techniques,adopted in the most recent astronomi-cal telescopes.Table 5Tolerance error budget for the baseline,with third-order ͑left ͒and first-order ͑right ͒layouts.Surfacenumber Radius (mm)±TOL Thickness (mm)±TOLDecenters(mm)Tilts_xy (deg)1:Primarymirror6400-0.018+0.024-2865-0.012+0.009--2:Secondarymirror 670-0.024+0.018310010±0.008±0.00146:Lens684.365±10--±5X →1Y →2。
ch01Introduction
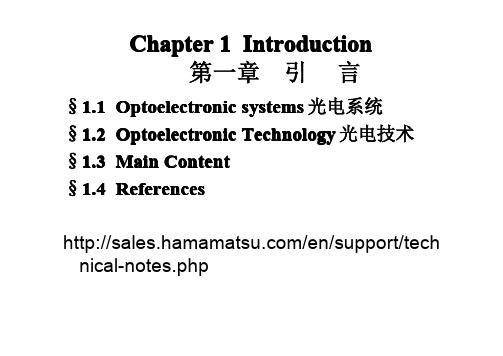
Chapter 1 Introduction第一章引言§1.1 Optoelectronic systems 光电系统§1.2 Optoelectronic Technology 光电技术§1.3 Main Content§1.4 References/en/support/tech nical-notes.php§1.1 Optoelectronic systems 光电系统Example 1 Pulsed laser ranging脉冲激光测距2/x cT L =2112T T T T x −+=•产品型号YARDAGE PRO ELITE1500•测距范围5-1500码(约5-1370米)•观察放大倍数7•倍物镜直径26毫米•视野范围1000米处340英尺(约104米)•尺寸43x129x94毫米•重量284克电池类型标准9伏碱性电池•反射性较好的目标1500码(约1370米)•树1000码(约913米)•鹿500码(约457米)•旗杆400码(约365米)•测距精度± 1码(米)•Example 3 LaserLASER =Light amplification by stimulated emission of radiation1. Gain medium 激光介质2. Laser pumping energy 激光泵浦2. Laser pumping energy3. High reflector 高反射镜3. High reflectorOutput coupler输出镜4. 4. 4. Output coupler5. Laser beam 激光束5. Laser beam•Lasercharacteristis: Laser characteristis •Homochromaticity Homochromaticity 单色性•Directionality 方向性•Coherence 相干性Laser-guided bomb•Optoelectronic systems•An optoelectronic system has following characteristics:The light or electricity is used as the carrier of information, both the methods of optics and electronics are used, both the electronics are used, both the opto opto opto-electronic -electronic conversion and electro-optic conversion are used during the collection, disposal, transfer, and recovery of the information.•光电系统•以光或电作为信息载体,综合利用光学和电学方法,综合利用光电及电光转换来采集、处理、传输、回复信息的系统。
第1章 绪论

发光与实时分析教育部重点实验室
化学化工学院 杨 睿
化学化工学院 杨 睿
2015 Spring Semester
仪器分析的创新成就
诺贝尔科学奖 近期重大突破和发明
原子吸收光谱分析的重大突破
多道毛细管电泳
化学化工学院 杨 睿
Related Nobel Prize
1991年诺贝Байду номын сангаас化学奖:
操作/数 手工操作、计算,繁琐 自动化,简便、快速;专业软件处理数 据处理 据;重现性好
仪器 应用
主要为玻璃仪器 定性、定量
较复杂、昂贵的特殊仪器 微量/痕量组分分析;状态、结构等分析 ;复杂混合物的分离、测定;生物分子 一级结构序列测定……
化学化工学院 杨 睿
仪器分析的局限性:仪器较为复杂、昂贵;某些
发明对生物大分子进行确认和结构
分析的质谱分析法
约翰·芬恩 田中耕一
发明对生物大分子的质谱分析法
化学化工学院 杨 睿
Related Nobel Prize
2003年诺贝尔医学奖: 保罗· 劳特布尔 (Paul Lauterbur)美国科学家 彼得· 曼斯菲尔德(Peter Mansfield )英国科学家
是以物质的化学反应为基础,通过已知物与待 测物的化学关系,而测出未知物的含量。
特点:
1、仪器简单 2、结果准确
3、应用范围广泛
4、局限性:不适于测定痕量或微量组分
化学化工学院 杨 睿
13
经典分析(化学分析)
化学分离: 沉淀、萃取、蒸馏等分离方法;
定性方法: 加入各种试剂,测量待测物的颜色、熔
沸点、气味、光学性质(折射、反射、衍射等)以
光学英语笔记大一下知识点

光学英语笔记大一下知识点AbstractThis article aims to summarize the key knowledge points of optical science for the second semester of year one. Topics covered include the fundamentals of optics, geometric optics, wave optics, and modern optics. Each section provides concise and comprehensive explanations to ensure a better understanding of the subject matter.1. Fundamentals of Optics1.1 Light and Electromagnetic SpectrumLight is a form of electromagnetic radiation that can be described as a wave and particle. The electromagnetic spectrum encompasses various forms of radiation, including radio waves, microwaves, infrared radiation, visible light, ultraviolet radiation, X-rays, and gamma rays. The visible light spectrum consists of different colors, ranging from red to violet.1.2 Reflection and RefractionReflection occurs when light bounces off a surface, following the law of reflection, which states that the incident angle is equal to the reflected angle. Refraction, on the other hand, happens when light transitions from one medium to another, causing a change in direction due to a change in speed. Snell's law describes the relationship between the angles of incidence and refraction, as well as the refractive indices of the two media involved.2. Geometric Optics2.1 Ray Optics and Basic TerminologiesGeometric optics deals with the behavior of light as rays. A ray is an imaginary line that represents the path of light. Key terms in geometric optics include the object, image, focal point, focal length, and magnification. These terms are crucial in understanding the formation of images through mirrors and lenses.2.2 Thin LensesA thin lens is a transparent device with two refracting surfaces that can converge or diverge light. It can be either convex (converging) or concave (diverging). Thin lenses follow the lensmaker's formula, which relates the focal length of a lens to its refractive indices and radii of curvature. The formula is especially useful in solving problems related to lens systems.3. Wave Optics3.1 Wave-Particle DualityWave optics studies the behavior of light as waves. The wave-particle duality principle states that light exhibits both wave-like and particle-like properties. This concept is crucial in understanding phenomena such as interference, diffraction, and polarization.3.2 InterferenceInterference occurs when two or more light waves overlap, leading to constructive or destructive interference. Constructive interference enhances the amplitude of the resultant wave, while destructiveinterference decreases it. This phenomenon is the basis for various applications, including anti-reflective coatings and thin film technology.3.3 DiffractionDiffraction refers to the bending of waves around obstacles or through narrow openings. It causes the spreading of light waves and affects the sharpness of images. Understanding diffraction is essential in areas such as optical microscopy and the design of optical instruments.4. Modern Optics4.1 PolarizationPolarization refers to the orientation of light waves in a specific direction. Linear polarization occurs when the waves vibrate in a single plane, while circular and elliptical polarization involve rotation of the wave's direction. Polarization plays a crucial role in applications such as optical filters, 3D glasses, and liquid crystal displays.4.2 Optical InstrumentsOptical instruments, such as microscopes and telescopes, utilize lenses and mirrors to enhance the visual perception of objects. Understanding the principles of image formation, magnification, and resolving power is fundamental in designing and using these instruments effectively.ConclusionThis article provides a concise overview of the key knowledge points in optical science for the second semester of year one. By grasping the fundamentals of optics, geometric optics, wave optics, and modern optics, students can develop a solid foundation in understanding the behavior of light and its applications in various fields.。
(完整版)超快光学第01章入门
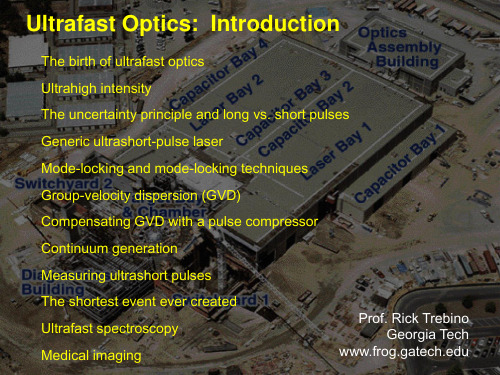
Mode-locking and mode-locking techniispersion (GVD)
Compensating GVD with a pulse compressor
Continuum generation
Measuring ultrashort pulses
Second-harmonic-generation of infrared light yields this beautiful
display of intense green light.
Continuum generation
Continuum Generation: focusing a femtosecond pulse into a clear medium turns the pulse white.
Laser power
A generic ultrashort-pulse laser
A generic ultrafast laser has a broadband gain medium, a pulseshortening device, and two or more mirrors:
Pulse-shortening devices include: Saturable absorbers Phase modulators Dispersion compensators Optical-Kerr media
Irradiance vs. time
Spectrum
Long pulse
time Short pulse
frequency
time
frequency
Ultrafast laser media