光解水综述
水的光解不需要酶
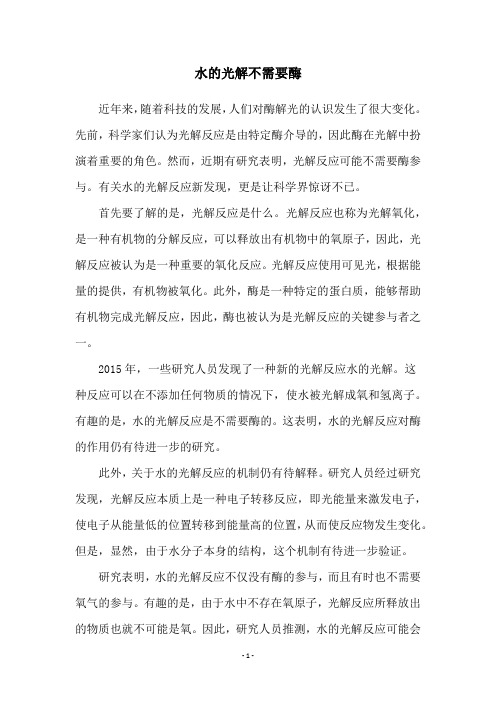
水的光解不需要酶近年来,随着科技的发展,人们对酶解光的认识发生了很大变化。
先前,科学家们认为光解反应是由特定酶介导的,因此酶在光解中扮演着重要的角色。
然而,近期有研究表明,光解反应可能不需要酶参与。
有关水的光解反应新发现,更是让科学界惊讶不已。
首先要了解的是,光解反应是什么。
光解反应也称为光解氧化,是一种有机物的分解反应,可以释放出有机物中的氧原子,因此,光解反应被认为是一种重要的氧化反应。
光解反应使用可见光,根据能量的提供,有机物被氧化。
此外,酶是一种特定的蛋白质,能够帮助有机物完成光解反应,因此,酶也被认为是光解反应的关键参与者之一。
2015年,一些研究人员发现了一种新的光解反应水的光解。
这种反应可以在不添加任何物质的情况下,使水被光解成氧和氢离子。
有趣的是,水的光解反应是不需要酶的。
这表明,水的光解反应对酶的作用仍有待进一步的研究。
此外,关于水的光解反应的机制仍有待解释。
研究人员经过研究发现,光解反应本质上是一种电子转移反应,即光能量来激发电子,使电子从能量低的位置转移到能量高的位置,从而使反应物发生变化。
但是,显然,由于水分子本身的结构,这个机制有待进一步验证。
研究表明,水的光解反应不仅没有酶的参与,而且有时也不需要氧气的参与。
有趣的是,由于水中不存在氧原子,光解反应所释放出的物质也就不可能是氧。
因此,研究人员推测,水的光解反应可能会释放出水中的氢离子。
这让科学家们更加疑惑,关于水的光解反应机制,看来还有待深入研究。
尽管关于水的光解反应还存在很多未解之谜,但是这一发现已经极大地改变了科学家们对光解反应的认识。
从某种程度上来说,水的光解反应可以被看作是一种自发反应,而不是由特定酶驱动的反应,这可能会改变酶在光解反应中所发挥的作用。
此外,水的光解反应对环境保护也具有重要意义。
因为光解反应可以分解有机物质,比如污染物,使它们变得清洁、无害,从而减少对环境的污染。
不过,目前的研究还很初步,这一技术可能在未来会得到进一步的发展,从而使我们能够更好地利用水的光解反应来帮助环境保护。
水的光解
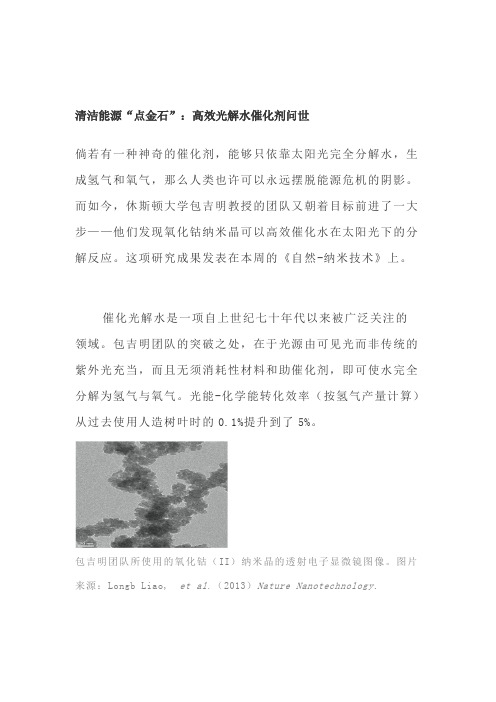
清洁能源“点金石”:高效光解水催化剂问世倘若有一种神奇的催化剂,能够只依靠太阳光完全分解水,生成氢气和氧气,那么人类也许可以永远摆脱能源危机的阴影。
而如今,休斯顿大学包吉明教授的团队又朝着目标前进了一大步——他们发现氧化钴纳米晶可以高效催化水在太阳光下的分解反应。
这项研究成果发表在本周的《自然-纳米技术》上。
催化光解水是一项自上世纪七十年代以来被广泛关注的领域。
包吉明团队的突破之处,在于光源由可见光而非传统的紫外光充当,而且无须消耗性材料和助催化剂,即可使水完全分解为氢气与氧气。
光能-化学能转化效率(按氢气产量计算)从过去使用人造树叶时的0.1%提升到了5%。
包吉明团队所使用的氧化钴(II)纳米晶的透射电子显微镜图像。
图片来源:Longb Liao,et al.(2013)Nature Nanotechnology.研究者通过球磨法及飞秒激光烧蚀法制备得到性质完全一致的氧化钴(II)纳米晶(CoO nanoparticles),将它们悬浮在呈中性的水里,装满烧瓶,再用一束由固态激光器发射的激光(波长为532nm)或由AM 1.5G(地表标准化太阳能)太阳模拟灯发出的光自烧瓶底部向上照射。
瓶中产生的混合气体被注射器采样,通过装有热导检测器(TCD)的气相色谱(GC)分离。
气相色谱分析结果表明,在光的照射和氧化钴纳米晶的催化下,水发生分解生成氢气和氧气。
图a:气相色谱的分析结果。
氢气(H2)和氧气(O2)的比值非常接近预期的2:1,预示水的完全分解。
此外,没有检测到明显的氮气信号,证明烧瓶气密性良好,氧气来源并非外界大气。
图b:水在约15mg氧化钴纳米晶的催化下光解产生的氢气和氧气的量。
氢气与氧气的生成源于光照,并与光照功率正相关。
图片来源:Longb Liao,et al.(2013)Nature Nanotechnology.为了证实生成的氧气中不含氧化钴纳米晶中的氧原子,实验团队又以18O标记的水为材料重复了实验,并通过质谱(MS)和残余气体分析仪(RGA)确认了氧原子来源仅为水。
光催化分解水综述..

缺点
光激发在同一个半导体微粒上产生的电子-空穴对极易复合 不但降低了光电转换效率,同时也影响光解水同时放氢放氧
MADE BY EAST6Biblioteka 2. 半导体光催化水解制氢
2.1 半导体光解水制氢的反应历程
半导体光催化剂吸收光子,形成电子-空穴对
电荷分离并转移到表面的反应活性点上
在表面进行化学反应,从而析出氢气和氧气
MADE BY EAST
7
半导体光解水制氢的原理
以TiO2(负载Pt和RuO2)为例。TiO2为n型半导体,其 价带(VB)和导带(CB)之间的禁带宽度为3.0eV左右。当它受 到其能量相当或高于该禁带宽度的光辐照时,半导体内的电 子受激发从价带跃迁到导带,从而在导带和价带分别产生自 由电子和空穴。水在这种电子-空穴对的作用下发生电离, 生成H2和O2。 表面所负载的Pt和RuO2分别能加速自由电子 向外部的迁移,促进氢气的产生和加速空穴的迁移有利于氧 气的生成
MADE BY EAST
27
Ni掺杂改性的作用
由于层间的K+具有较好的交换特性,所以层状铌酸盐比 较容易进行改性。例如Domen等将Ni 离子引入K4Nb6O17 的 层Ⅰ中,经还原- 氧化处理后,形成的新型催化剂具有较高的 催化活性
机理 在光的作用下,Ni-O 层中生成的自由电子(e - ) 移向
BaTa2O6(Ortho) *
4.1
126
59
*水中加入少量的Ba(OH)2
MADE BY EAST
22
3.1.3 过渡金属钽酸盐
可以看出,在没有共催化剂的条件下, Activity/μmol h-1 只有 NiTa O 可以分解纯水为氢和氧;在 Catalyst 2 7 Band gap/eV 负载NiO后,ZnTa2O7也具有了光催化分 H2 O2 解水活性。而其他过渡金属钽酸盐均不 CrTaO4 2.7 2 0 能产生氧气(CuTa2O7尽管可以分解水产 MnTa2O6 3.3 0.2 0 生氧气和氢气,但产生的量太少)
水光解产物
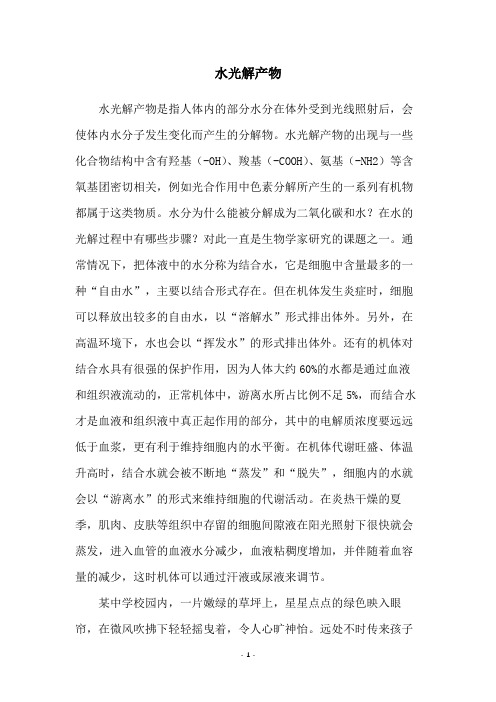
水光解产物水光解产物是指人体内的部分水分在体外受到光线照射后,会使体内水分子发生变化而产生的分解物。
水光解产物的出现与一些化合物结构中含有羟基(-OH)、羧基(-COOH)、氨基(-NH2)等含氧基团密切相关,例如光合作用中色素分解所产生的一系列有机物都属于这类物质。
水分为什么能被分解成为二氧化碳和水?在水的光解过程中有哪些步骤?对此一直是生物学家研究的课题之一。
通常情况下,把体液中的水分称为结合水,它是细胞中含量最多的一种“自由水”,主要以结合形式存在。
但在机体发生炎症时,细胞可以释放出较多的自由水,以“溶解水”形式排出体外。
另外,在高温环境下,水也会以“挥发水”的形式排出体外。
还有的机体对结合水具有很强的保护作用,因为人体大约60%的水都是通过血液和组织液流动的,正常机体中,游离水所占比例不足5%,而结合水才是血液和组织液中真正起作用的部分,其中的电解质浓度要远远低于血浆,更有利于维持细胞内的水平衡。
在机体代谢旺盛、体温升高时,结合水就会被不断地“蒸发”和“脱失”,细胞内的水就会以“游离水”的形式来维持细胞的代谢活动。
在炎热干燥的夏季,肌肉、皮肤等组织中存留的细胞间隙液在阳光照射下很快就会蒸发,进入血管的血液水分减少,血液粘稠度增加,并伴随着血容量的减少,这时机体可以通过汗液或尿液来调节。
某中学校园内,一片嫩绿的草坪上,星星点点的绿色映入眼帘,在微风吹拂下轻轻摇曳着,令人心旷神怡。
远处不时传来孩子们的嬉戏打闹声,笑声夹杂着哭喊声,充满了生机勃勃的气息。
在这春天的景色里,同学们在做什么呢?哦!原来他们正在这块草坪上开心的玩耍呢!忽然,几个孩子指着一个小女孩的后面叫了起来:“姐姐,你看!”小女孩一转身,脸刷的一下红了,她也不知道是怎么回事,连忙拉着妹妹朝着一位老师走去。
“您好!老师,我……我妹妹不小心摔倒了,是不是应该帮她擦一擦呢?”那位老师微笑着问道:“请问你们姐妹俩的父母在吗?”姐姐看了看那位老师,又看了看妹妹,小心翼翼地说道:“没关系,我们回家吧!”教室里回荡着同学们阵阵欢笑声,我在想:“她们为什么那么开心?”我不禁向窗外望去,一缕缕阳光透过树枝散落在草坪上,似乎又勾起了我的好奇心。
光解水制氢 无机半导体
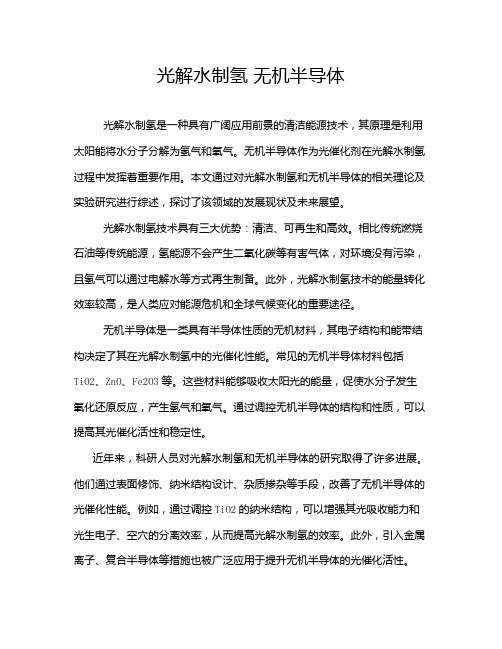
光解水制氢无机半导体光解水制氢是一种具有广阔应用前景的清洁能源技术,其原理是利用太阳能将水分子分解为氢气和氧气。
无机半导体作为光催化剂在光解水制氢过程中发挥着重要作用。
本文通过对光解水制氢和无机半导体的相关理论及实验研究进行综述,探讨了该领域的发展现状及未来展望。
光解水制氢技术具有三大优势:清洁、可再生和高效。
相比传统燃烧石油等传统能源,氢能源不会产生二氧化碳等有害气体,对环境没有污染,且氢气可以通过电解水等方式再生制备。
此外,光解水制氢技术的能量转化效率较高,是人类应对能源危机和全球气候变化的重要途径。
无机半导体是一类具有半导体性质的无机材料,其电子结构和能带结构决定了其在光解水制氢中的光催化性能。
常见的无机半导体材料包括TiO2、ZnO、Fe2O3等。
这些材料能够吸收太阳光的能量,促使水分子发生氧化还原反应,产生氢气和氧气。
通过调控无机半导体的结构和性质,可以提高其光催化活性和稳定性。
近年来,科研人员对光解水制氢和无机半导体的研究取得了许多进展。
他们通过表面修饰、纳米结构设计、杂质掺杂等手段,改善了无机半导体的光催化性能。
例如,通过调控TiO2的纳米结构,可以增强其光吸收能力和光生电子、空穴的分离效率,从而提高光解水制氢的效率。
此外,引入金属离子、复合半导体等措施也被广泛应用于提升无机半导体的光催化活性。
然而,目前光解水制氢技术仍然存在一些挑战。
例如,无机半导体材料的光电转化效率仍然较低,光照条件下制氢速率有限,光催化剂在长时间使用过程中稳定性不足等问题。
为了克服这些挑战,有必要进一步深入探讨无机半导体的电子结构和光物理性质,寻找新的光催化机制,提高光解水制氢技术的可持续性和经济性。
未来,随着材料科学和光电子学等领域的发展,相信光解水制氢技术和无机半导体材料会迎来更多的突破。
我们可以通过合成多孔结构材料、设计多功能合成复合体系、构建光催化界面等策略,进一步提高无机半导体的光催化性能,推动光解水制氢技术向商业化和工程化迈进。
光催化光解水制氢百科_解释说明
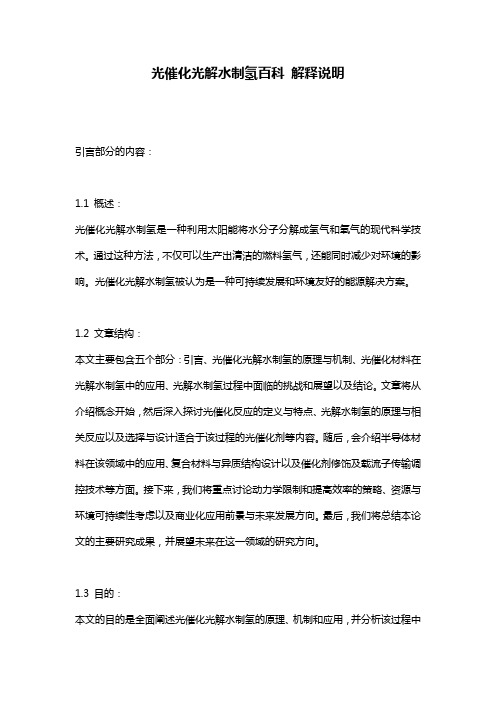
光催化光解水制氢百科解释说明引言部分的内容:1.1 概述:光催化光解水制氢是一种利用太阳能将水分子分解成氢气和氧气的现代科学技术。
通过这种方法,不仅可以生产出清洁的燃料氢气,还能同时减少对环境的影响。
光催化光解水制氢被认为是一种可持续发展和环境友好的能源解决方案。
1.2 文章结构:本文主要包含五个部分:引言、光催化光解水制氢的原理与机制、光催化材料在光解水制氢中的应用、光解水制氢过程中面临的挑战和展望以及结论。
文章将从介绍概念开始,然后深入探讨光催化反应的定义与特点、光解水制氢的原理与相关反应以及选择与设计适合于该过程的光催化剂等内容。
随后,会介绍半导体材料在该领域中的应用、复合材料与异质结构设计以及催化剂修饰及载流子传输调控技术等方面。
接下来,我们将重点讨论动力学限制和提高效率的策略、资源与环境可持续性考虑以及商业化应用前景与未来发展方向。
最后,我们将总结本论文的主要研究成果,并展望未来在这一领域的研究方向。
1.3 目的:本文的目的是全面阐述光催化光解水制氢的原理、机制和应用,并分析该过程中所面临的挑战和可能的解决办法。
通过对相关文献和研究成果进行综合整理和分析,希望为读者提供一个深入了解光催化光解水制氢以及其潜在应用价值和发展前景的全面指南。
此外,本文还将探讨存在于该领域中尚未解决问题,并提出未来进一步研究该技术时可能关注的重点方向。
根据以上内容撰写了文章"1. 引言"部分,请您查看并反馈满意度。
2. 光催化光解水制氢的原理与机制2.1 光催化反应的定义与特点光催化反应是指利用光能激发物质中的电子和空穴,在固体表面或溶液中进行化学反应的过程。
相比传统的热催化反应,光催化反应具有以下几个显著特点:首先,光能可以高效提供活性能量,使得部分惰性物质也能够发生反应;其次,光催化反应在温和条件下进行,减少了对环境的热污染;此外,光催化材料具有可再生性和可调控性等优点,在节约资源和环境可持续性方面具有潜力。
光催化分解水体系概述
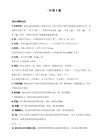
催化分解水的基本原理。
锐钛矿型的Ti02其价带到导带的禁带宽度约为3.2eV,当受到光子能量等于或高于禁带宽度的光辐照时,其价带上的电子(e一)就会受激跃迁至导带,在价带上产生相应的空穴(h+),形成了电子一空穴对。
产生的电子、空穴在内部电场作用下分离并迁移到粒子表面。
光生空穴有很强的得电子能力,具有强氧化性,可夺取半导体颗粒表面被吸附物质或溶剂中的电子,使原本不吸收光的物质被氧化,电子受体则通过接受表面的电子而被还原,完成光催化反应过程,如图1所示[¨:图1光催化分解水的基本过程模型①半导体光催化剂吸收能量足够大的光子,产生电子一空穴对;⑦电子一空穴对分离,向半导体光催化剂表面移动;③电子与水反应产生氢气I④空穴与水反应产生氧气I⑤部分电子与空穴复合,产生热或光。
光催化分解水反应式可写为(以Ti02为例)‘“l2Ti02+2hv—・2Ti02+2h++2e一(1)2e一+2H+—・H’+H‘一H2(Z)2h++2H20一2H。
o+一20H’+2H+(3)oH‘+oH‘—,H:o+1/202(4)但也并不是所有的半导体光催化剂都能作为光分解水的催化剂,必须满足一定的氧化还原化学反应条件,即首先其禁带宽度要大于水的分解电压(理论值1.23eV),且由于超电压的存在,半导体材料的禁带宽度要大于水的分解电压,其次,半导体光催化剂的价带位置应比O。
/H。
o的电位更正,而导带的位置应比H+/H。
更负,最合适的禁带宽度应为2.0eV左右‘…。
3光催化分解水体系自从Fujishima和Honda发现可以利用二氧・128・化钛(Ti02)光催化分解水制备氢气和氧气以来,各国学者一直致力于光催化分解水的研究,并在高效光催化剂的研究方面取得了重要进展,开发了为数众多的光催化剂o]。
目前,光催化分解水的评价体系主要是粉末直接光照的水溶早匝悬浮反应体系和光电化学体系。
其中,粉末悬浮作为光催化分解水制氢的反应体系可用来评价半导体光催化剂的许多性质,如导带与价带的位置、禁带宽度、材料本身在水溶液中的稳定性等。
光解水制氢技术的研究与应用前景
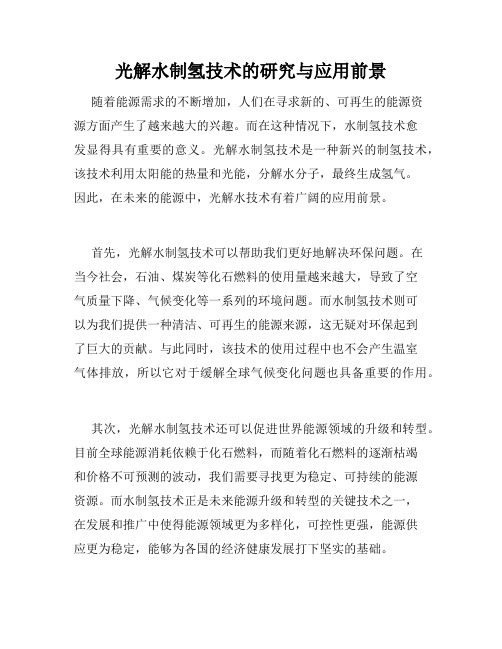
光解水制氢技术的研究与应用前景随着能源需求的不断增加,人们在寻求新的、可再生的能源资源方面产生了越来越大的兴趣。
而在这种情况下,水制氢技术愈发显得具有重要的意义。
光解水制氢技术是一种新兴的制氢技术,该技术利用太阳能的热量和光能,分解水分子,最终生成氢气。
因此,在未来的能源中,光解水技术有着广阔的应用前景。
首先,光解水制氢技术可以帮助我们更好地解决环保问题。
在当今社会,石油、煤炭等化石燃料的使用量越来越大,导致了空气质量下降、气候变化等一系列的环境问题。
而水制氢技术则可以为我们提供一种清洁、可再生的能源来源,这无疑对环保起到了巨大的贡献。
与此同时,该技术的使用过程中也不会产生温室气体排放,所以它对于缓解全球气候变化问题也具备重要的作用。
其次,光解水制氢技术还可以促进世界能源领域的升级和转型。
目前全球能源消耗依赖于化石燃料,而随着化石燃料的逐渐枯竭和价格不可预测的波动,我们需要寻找更为稳定、可持续的能源资源。
而水制氢技术正是未来能源升级和转型的关键技术之一,在发展和推广中使得能源领域更为多样化,可控性更强,能源供应更为稳定,能够为各国的经济健康发展打下坚实的基础。
除此之外,光解水制氢技术还有许多其他的应用前景。
例如,它可以用于家居、工业和农业等领域。
在家居领域中,氢气可以应用于厨房、热水器等场合中,也可以作为供电的替代品。
在工业领域中,氢气可以被用作化学原料,帮助生产更高质量以及绿色的化工产品。
在农业领域,氢气可以作为农业生产中的栽培介质,增加作物产量以及水分利用率。
虽然光解水制氢技术具有许多优势和应用前景,但该技术的开发和推广还需要面对一系列的挑战。
例如,目前商业化的效率和成本还不够优秀,仍需要更多的研究和创新技术来推动其成本下降和效率提升。
此外,其技术的推广也需要政策和市场的支持。
总的来说,光解水制氢技术是一个未来可再生能源的重要来源,具有着广泛的应用前景。
未来我们需要通过不断创新、技术突破和市场的拓展,让这项技术在实际生产和应用中得到广泛推广,以推动世界能源的升级和转型,为人类的健康、环境和经济发展做出应有的贡献。
- 1、下载文档前请自行甄别文档内容的完整性,平台不提供额外的编辑、内容补充、找答案等附加服务。
- 2、"仅部分预览"的文档,不可在线预览部分如存在完整性等问题,可反馈申请退款(可完整预览的文档不适用该条件!)。
- 3、如文档侵犯您的权益,请联系客服反馈,我们会尽快为您处理(人工客服工作时间:9:00-18:30)。
ReviewPhotochemical splitting of water for hydrogen productionby photocatalysis:A reviewAdel A.Ismail a,b,c,n,Detlef W.Bahnemann ca Advanced Materials Department,Central Metallurgical R&D Institute,CMRDI,P.O.Box87,Helwan11421,Cairo,Egyptb Advanced Materials and NanoResearch Center,Najran University,P.O.Box:1988,Najran11001,Saudi Arabiac Institute für Technische Chemie,Leibniz Universität Hannover,Callinstrasse3,D-30167Hannover,Germanya r t i c l e i n f oArticle history:Received26January2014Received in revised form21April2014Accepted27April2014Available online2June2014Keywords:Water splittingHydrogen productionPhotocatalystsUV and visible illuminationa b s t r a c tHydrogen production from water using a catalyst and solar energy is an ideal future fuel source.The searchfor suitable semiconductors as photocatalysts for water splitting into molecular hydrogen and oxygen hasbeen considered to be an urgent subject for our daily life.In this review,we aim to focus on the researchefforts that have been made so far for H2generation from water splitting by UV and visible light-drivenphotocatalysis.A number of synthetic modification methods for adapting the electronic structure to enhancethe charge separation in the photocatalyst materials are discussed.Sacrificial reagents and electronmediators for the overall water splitting are also reviewed.The quantum efficiency of photocatalystmaterials upon visible and UV illumination will be reviewed,summarized and discussed.&2014Elsevier B.V.All rights reserved. Contents1.Introduction (86)1.1.Basic principles of photocatalytic hydrogen generation (86)1.2.Main processes of photocatalytic hydrogen generation (87)1.3.Evaluation of photocatalytic water splitting systems (87)2.Photocatalysts for water splitting (87)2.1.UV photocatalysts for water splitting (87)2.1.1.TiO2and titanates as photocatalysts for H2production (87)2.1.2.Nb-and Ta-based oxides (88)2.1.3.W-,Mo-,and V-based oxides (89)2.1.4.d10Metal oxide photocatalysts (89)2.1.5.f0Metal oxide photocatalysts (89)2.1.6.Nonoxide photocatalysts (89)2.2.Visible light active photocatalysts for H2production (89)2.2.1.Efficient separation of photogenerated charge carriers (90)2.2.2.Cocatalyst loading (90)2.2.3.Z-scheme photocatalysts (90)2.2.4.Metal sulfides (92)2.2.5.GaN:ZnO solid solution photocatalysts (92)2.2.6.Overall water splitting employing dye-sensitized solar cells to harvest visible light (95)2.2.7.Electron mediators for overall water splitting (96)3.Main challenges and opportunities (97)4.Conclusions and outlook (97)Contents lists available at ScienceDirectjournal homepage:/locate/solmatSolar Energy Materials&Solar Cells/10.1016/j.solmat.2014.04.0370927-0248/&2014Elsevier B.V.All rightsreserved.n Corresponding author.E-mail address:adelali141@(A.A.Ismail).Solar Energy Materials&Solar Cells128(2014)85–101Acknowledgment (98)References (98)1.IntroductionPhotocatalytic water splitting into H2and O2using semiconduct-ing catalysts has received much attention due to the potential of this technology,as well as the great economic and environmental interest for the production of the clean fuel H2from water using solar energy.Fujishima and Honda demonstrated the potential of TiO2semiconductor materials to split water into H2and O2[1,2]. Their work triggered the development of semiconductor photo-catalysis for a wide range of environmental and energy applications [1,2].The photocatalytic activity of conventional metal-oxide photo-catalysts for the overall water splitting is known to be heavily dependent on the crystallinity and the particle size of the material, as determined by the preparation conditions[3–5].During the past 40years,various photocatalyst materials have been developed to split water into H2and O2under UV and visible light illumination. The direct splitting of water using a particulate photocatalyst would be a good way to produce clean and recyclable H2on a large scale[6–9].A number of photocatalysts have been proposed and achieved high quantum efficiencies under UV illumination.At present,there is a lack of suitable materials with sufficiently band gap positions for overall water splitting,and the stability necessary for practical applications.In general,efficient photocatalytic materi-als contain either transition-metal cations with a d0electronic configuration(e.g.,Ta5þ,Ti4þ,Zr4þ,Nb5þ,Ta5þ,W6þ,and Mo6þ)or typical metal cations with d10electronic configuration (e.g.,In3þand Sn4þ,Ga3þ,Ge4þ,Sb5þ)as principal cation components,the empty d or sp orbitals of which form the bottom of the respective conduction bands[6–9].The tops of the valence bands of metal oxide photocatalysts with d0-or d10-metal cations usually consist of O2p orbitals,which are located at aboutþ3eV or higher versus normal hydrogen electrode(NHE)and,as such, produce a band gap too wide to absorb visible light[9].Also, (oxy)nitrides containing d0transition-metal cations,such as Ta3N5, TaON,and LaTiO2N,are potential photocatalytic materials to achieve water splitting[10].The highest quantum efficiencies(QEs)have been reported for NiO-modified La/KTaO3(QE¼56%)using water under UV light[11],QE¼90%has been obtained for H2generation employing ZnS as photocatalysts in the presence of aqueous Na2S/ Na2SO3as sacrificial reductant under UV illumination[12]and for Cr/Rh-modified GaN/ZnO(QE¼2.5%),in pure water under visible light illumination[13–15].So far,no material capable of catalyzing reaction(Eq.(1))with visible light and a QE larger than10%has been found[15].Therefore,investigating the physical factors that govern the photocatalytic activity for H2production is an important and indispensable issue in the development of highly active photo-catalysts.While a number of visible-light-driven photocatalysts have been proposed as potential candidates for the overall water splitting,a satisfactory material has yet to be devised[16–19].At a power level of1000W/m2the solar energy incident on the earth's surface by far exceeds all human energy needs[20,21].Photovoltaic and electrochemical solar cells that convert solar energy into electricity can reach up to55–77%efficiency[22–25]but remain uneconomical because of high fabrication costs,insufficient light absorption[26],and inefficient charge transfer[22].In a process that mimics photosynthesis;solar energy can also be used to convert water into H2and O2,the fuels of a H2-based energy economy(Eq.(1))H2O-1=2O2ðΔÞþH2ðgÞ;ΔG¼þ237kJ=molð1:3eV=e;λmin¼1100nmÞð1ÞThe development of a photocatalytic system that functions efficiently under visible light,representing almost half of the available solar energy on the surface of the earth,is therefore essential for the practical utilization of solar energy.In this review, we aim to summarize the research efforts having been made so far, for H2generation from water splitting employing UV and visible light-driven photocatalysts in combination with the view on inspiring new ideas to tackle this important challenge.A number of synthetic and modification techniques for adjusting the band structure of the photocatalyst to harvest visible light and to improve the charge separation in photocatalysis are discussed. Sacrificial reagents and electron mediators for the overall water splitting are also reviewed.1.1.Basic principles of photocatalytic hydrogen generationFig.1a shows a schematic diagram of water splitting into H2and O2over photocatalysts.Photocatalysis on semiconductor particles involves three main steps:(i)absorption of photons with energies exceeding the semiconductor bandgap,leading to the generation of electron(eÀ)and hole(hþ)pairs in the semiconductor particles; (ii)charge separation followed by migration of these photogener-ated carriers in the semiconductor particles;(iii)surfacechemicalFig.1.Schematic illustration of water splitting over semiconductor photocatalysts.Source:Ref.[103].A.A.Ismail,D.W.Bahnemann/Solar Energy Materials&Solar Cells128(2014)85–10186reactions between these carriers with various compounds(e.g., H2O);electrons and holes may also recombine with each other without participating in any chemical reactions[3,7].When a photocatalyst is used for water splitting,the energetic position of the bottom of the conduction band must be more negative than the reduction potential of water to produce H2,and that of the top of the valence band must be more positive than the oxidation potential of water to produce O2,as shown in Fig.1b.Furthermore, the photocatalyst must be stable in aqueous solutions under photoirradiation.Scaife noted in1980that it is intrinsically difficult to develop an oxide semiconductor photocatalyst that has both, a sufficiently negative conduction band for H2production and a sufficiently narrow band gap(i.e.,o3.0eV)for visible light absorp-tion because of the highly positive position of the valence band (at ca.þ3.0V vs.NHE)formed by the O2p orbitals[27].To facilitate water oxidation,the potential of the valence band edge must exceed the oxidation potential of water ofþ1.23V vs NHE at pH¼0 (þ0.82V at pH¼7).Based on these parameters,a theoretical semiconductor band-gap energy of$1.23eV is required to drive the water-splitting reaction according to Eq.(1).The smallest band gap achieved so far in a functional catalyst is2.30eV in NiO/RuO2–Ni:InTaO4[28,29].Semiconductors with smaller band gaps or lowerflat-band potentials require a bias voltage or external redox reagents to drive the reaction.Alternatively,two or more small band-gap semicon-ductors can be combined to drive water oxidation/reduction pro-cesses separately via multiphoton processes.It is well known that flat-band potentials strongly depend on ion absorption(protonation of surface hydroxyl groups),crystallographic orientation of the exposed surface,surface defects,and surface oxidation processes [30,31].These factors are rarely considered in the preparation and testing of photochemical water-splitting catalysts[32–34].1.2.Main processes of photocatalytic hydrogen generationThe individual processes involved in the photocatalytic gen-eration of H2are illustrated in Fig.1.They include light absorption of the semiconductor photocatalyst,generation of excited charge carriers(electrons and holes),recombination of these charge carriers,separation of the excited charge carriers,migration of electrons and holes,charge carrier trapping,and transfer of the charge carriers to water or other molecules[3,7].All of these processes affect thefinal generation of H2from the semiconductor photocatalyst system.The total amount of H2generated is deter-mined by the amount of excited electrons at the water/photo-catalyst interface capable of reducing water.After the electron/ hole pairs are created,charge recombination and separation/ migration processes are two important competitive processes inside the semiconductor photocatalyst that largely affect the efficiency of the photocatalytic reaction for water splitting[35]. Charge recombination reduces the number of eÀ/hþpairs by emitting light or generating phonons.This includes both,surface and bulk recombination,and is classified as a deactivation process, and it is ineffective for water splitting.Efficient charge separation and fast charge carrier transport,avoiding any bulk/surface charge recombination,are thus fundamentally important for the photo-catalytic H2generation through water splitting.The reaction of photogenerated H2and O2to form H2O on the photocatalyst surface is normally called“surface backreaction(SBR)”.There are two main approaches to suppress the SBR:one involves the addition of sacrificial reagents into the photocatalytic reaction environment and the second creates a separation of the photo-active sites on the surface of the photocatalysts.In general, sacrificial reagents acting as electron donors or acceptors,respec-tively,drive the reaction into alternative pathways as they are reduced or oxidized,respectively.Taking into consideration the basic mechanism and the individual processes of photocatalytic water splitting,there are two keys for the development of a suitable high-efficiency semiconductor for the visible-light-driven photocatalytic splitting of water into H2and/or O2:(1)a photocatalyst should have a sufficiently narrow band gap (1.23eV o Eg o3.0eV)to both harvest visible light and possess the correct band structure;and(2)photoinduced charges in the photocatalyst should be separated efficiently in order to avoid bulk/surface electron/hole recombination.In addition,both charge carriers must migrate to the photocatalyst surface for H2and/or O2 evolution at the respective photocatalytic active sites[36].It is important that economical,highly efficient photocatalytic systems for light-to-H2energy conversion,in which aqueous solutions containing sacrificial reagents can be used to minimize the back-ward reaction of H2and O2to water on the surface of photo-catalysts,can be constructed.1.3.Evaluation of photocatalytic water splitting systemsThe rate of gas(O2and H2)evolution with units such asμmol hÀ1 is used to enable a measurable comparison between different photocatalysts under similar experimental conditions.The quantum yield,as an extension from the overall quantum yield defined in a homogeneous photochemical system,becomes important and accep-table to evaluate the photocatalytic activity for water splitting. The overall quantum yield is defined for H2and O2formation by Eqs.(2)and(3),respectively[37].The quantum yield is estimated to be smaller than the overall quantum yield because the number of absorbed photons is usually smaller than the number of photons available in the incident light.Activities of photochemical water-splitting catalysts are usually assessed by the rates of evolved gases [mol/h]per catalyst amount[g]under the specified irradiation conditions.From the measured evolution rate[H2],the apparent QE¼2[H2]/I of the catalyst can be calculated using the known photon fluxΙ[mol/s]incident on the reaction mixture.Overall quantum yieldð%Þ¼ð2ÂNumber of evolved H2moleculesÞNumber of incident photonsÂ100ðfor H2evolutionÞð2ÞOverall quantum yieldð%Þ¼ð4ÂNumber of evolvedO2moleculesÞNumber of incident photonsÂ100ðfor O2evolutionÞð3Þ2.Photocatalysts for water splitting2.1.UV photocatalysts for water splittingA wide range of semiconducting materials have been devel-oped as photocatalysts under UV irradiation.On the basis of their electronic configuration properties,these UV-active photocatalysts can be typically classified into four groups:(1)d0metal(Ti4þ, Zr4þ,Nb5þ,Ta5þ,W6þ,and Mo6þ)oxide photocatalysts,(2)d10 metal(In3þ,Ga3þ,Ge4þ,Sn4þ,and Sb5þ)oxide photocatalysts, (3)f0metal(Ce4þ)oxide photocatalysts,and(4)a small group of nonoxide photocatalysts.2.1.1.TiO2and titanates as photocatalysts for H2productionTiO2was thefirst material described as a photochemical water-splitting catalyst(Fig.2).It crystallizes in three structure types: Rutile,Anatase,and Brookite.All modifications contain TiO6 octahedra that are interconnected via two(Rutile),three(Broo-kite),or four(Anatase)common edges and via shared corners,andA.A.Ismail,D.W.Bahnemann/Solar Energy Materials&Solar Cells128(2014)85–10187as a result,the band gaps (3.0eV for Rutile and 3.15eV for Anatase)differ slightly [38].In their 1971/72papers,Fujishima and Honda described an electrochemical cell consisting of a n-type TiO 2(Rutile)anode and a Pt black cathode [1,2].When the cell was irradiated with UV light (o 415nm)from a 500W Xe lamp,O 2evolution was observed at the anode with a current flowing to the Pt counter electrode.Based on the current,a photoelectrochemical ef ficiency of approx.10%was estimated.Under UV irradiation,Pt –TiO 2was found to catalyze the water reduction with iodide as the sacri ficial electron donor,whereas TiO 2was observed to be the water oxidation catalyst itself in the presence of IO 3as the electron acceptor.After both catalysts were combined,H 2and O 2were formed stoichiometrically from a basic (pH ¼11)solution,with iodide serving as a redox shuttle.A similar system was realized with Pt –TiO 2and Pt –WO 3,giving QE ¼4%upon irradiation with UV light [39].Other recent efforts have sought to improve the optical response of TiO 2-based catalysts via doping with C or N,and S [40–43].Pt-modi fied catalysts evolve O 2from aqueous AgNO 3as the sacri ficial electron acceptor and traces of H 2from aqueous methanol as the sacri ficial electron donor under visible light [44].Newly synthesized tailored anatase/brookite mixtures as well as pure brookite TiO 2nanorods were also employed for H 2production.The results achieved so far revealed that anatase/brookite mixtures and brookite nanorods exhibit higher photocatalytic activity than anatase nanoparticles and even higher ef ficiencies than TiO 2P25for the photocatalytic H 2evolution from aqueous methanol solution [45,46].Further-more,mesoporous TiO 2nanostructures have been studied as a route to minimize Pt loading on TiO 2photocatalysts for H 2production.The results indicated that the amount of H 2evolved on 0.2wt%Pt/TiO 2calcined at 4501C is three times higher than that evolved on Pt/TiO 2-P25and twelve times higher than that evolved on Pt/TiO 2calcined at 3501C [47].Also,a series of TiO 2and graphene sheet(GSs)composites were synthesized [48].The photocatalytic activity of these samples was evaluated by H 2evolution from water photo-splitting under UV –vis illumination.On the other hand,when TiO 2is fused with metal oxides (SrO,PbO,etc.)metal titanates with intermediate band gaps can be obtained.Of these,SrTiO 3crystallizes in the Perovskite structure type and has a band gap of 3.2eV,slightly larger than that of TiO 2.It was first employed in 1976as a photocatalyst in a water-splitting electrochemical cell together with p-CdTe or p-GaP photocathodes [49].An optimized version of this system was reported in 1977by Ohashi and co-workers to be the first self-supported photoelec-trochemical cell [50]with a photon to-electron conversion ef fi-ciency of 0.044–0.67%.In 1980,it was shown that NiO-modi fiedSrTiO 3powder splits water vapor stoichiometrically under UV irradiation,while SrTiO 3alone did not show any activity [51].The conditions of the NiO deposition had a strong effect on the catalytic activity [52].Abe et ed Cr/Ta-doped Pt/SrTiO 3together with Pt –WO 3in a two-particle catalyst system for overall water splitting with visible light.Cr/Ta:SrTiO 3–Pt produced H 2and Pt –WO 3produced O 2,with an iodide/iodate redox couple serving as the redox mediator between the two catalysts [53].The effect of metal cocatalysts (Ru,Ir,Pd,Pt,Os,Re,Co)on the water-splitting activity of SrTiO 3was also studied [54,55].From pure water,H 2and O 2were evolved stoichiometrically,with the activity decreas-ing in the order Rh 4Ru 4Re 4Pt 4Ir 4Pd 4Os 4Co.SrTiO 3alone produced H 2but no O 2.A series of layered Perovskites as photochemical water-splitting catalysts was investigated [56,57].The NiO-modi fied catalyst is active for H 2/O 2evolution with a QE up to 12%[56–58].By doping with BaO and upon addition of NaOH to the catalyst suspension,this activity could be further increased to QE ¼50%[56],only slightly below that of the best catalyst,i.e.,La-doped NaTaO 3with QE ¼56%[59,60].2.1.2.Nb-and Ta-based oxidesNb 2O 5has a band gap of 3.4eV and it is not active for pure water splitting under UV irradiation.After modi fication with Pt as a cocatalyst,however,it can ef ficiently produce H 2from aqueous solutions containing methanol as an electron donor [61].Meso-porous Nb 2O 5,demonstrated a photocatalytic activity 20times higher for H 2evolution than a bulk Nb 2O 5without any porosity.Intercalation of In 2O 3into the mesoporous structure further increased the photoactivity of mesoporous Nb 2O 5by 2.7times [62].Besides Nb 2O 5,a large number of niobates can produce H 2and O 2via water splitting upon UV irradiation.K 4Nb 6O 17was developed as the first example of a niobate photocatalyst that showed high and stable activity for H 2evolution from aqueous methanol solution without any assistance from other materials such as the noble metals [63,64].There are various nanocompo-sites of SrNb 2O 6,Sr 2Nb 2O 7,and Sr 5Nb 4O 15that exhibited ef ficient photocatalytic activities for H 2and O 2production under UV irradiation [65–67].In particular,Sr 2Nb 2O 7with a highly donor-doped layered perovskite structure gave quantum yields as high as 23%.The activity of Sr 2Nb 2O 7was further enhanced achieving quantum yields up to 32%[65].Kudo and co-workers reported Ba 5Nb 4O 15with a layered perovskite structure for water splitting loaded with NiO cocatalysts and reported 17%quantum yield upon 270nm illumination [66,67].Partial substitution of Nb 5þwith Zn 2þto obtain BaZn 1/3Nb 2/3O 3with a distorted perovskite struc-ture showed favorable photocatalytic activities under UV irradia-tion [68,69].The photocatalytic activity of ZnNb 2O 6was negligible,whereas NiO-loaded ZnNb 2O 6revealed a high photoactivity [70].Chen and coworkers prepared ABi 2Nb 2O 9(A ¼Ca,Sr,Ba)perovs-kite photocatalysts for both H 2and O 2evolution from aqueous solutions containing sacri ficial reagents [71].The photocatalytic performance decreased in the order of SrBi 2Nb 2O 94BaBi 2N-b 2O 94CaBi 2Nb 2O 9.M 2BiNbO 7(M ¼In 3þ,Ga 3þ)with pyrochlore structures were sensitive to UV irradiation and had the ability to split water [72].Sabio et al.studied the photocatalytic water splitting activities of suspended KCa 2Nb 3O 10nanoscale and bulk particles and compared them using a kinetic model [73].Their model showed that the higher activity of the nanoniobate can be rationalized by shortened transport paths for electrons and holes to the reactive surface,which reduce the lattice recombination.This effect apparently outweighs the increase in surface recombi-nation that is to be expected for the nanomaterial,due to its increased speci fic surfacearea.Fig.2.Schematic representation of a photoelectrochemical cell (PEC).Source :Reprinted with permission from Ref.[1].A.A.Ismail,D.W.Bahnemann /Solar Energy Materials &Solar Cells 128(2014)85–101882.1.3.W-,Mo-,and V-based oxidesPbWO4incorporating a WO4tetrahedron showed high andstable photocatalytic activity for the overall splitting of water[74,75].H2and O2was produced under UV irradiation when RuO2was loaded onto the metal oxide.The photocatalytic performancewas attributed to large dispersions in both the valence andconduction bands.PbMoO4catalyzed the H2evolution fromaqueous methanol solution.It also exhibited photocatalytic O2evolution from aqueous AgNO3solution under UV irradiation withits O2evolution activity being comparable to that observed forTiO2[76].Na2W4O13and Bi2W2O9,with layered structures,wereactive for photocatalytic H2and O2evolution under UV irradiation[77,78].However,Bi2MoO6with a similar structure evolved onlyO2from aqueous AgNO3solution at a low rate[79].A new crystalstructure of VO2,with a body centered-cubic structure(bcc)and alarge optical band gap of2.7eV,surprisingly showed excellentphotocatalytic activity for H2production under UV irradiation[80].The bcc VO2phase exhibited a high quantum efficiency of38.7%when synthesized as nanorods.Also,Bi2GaVO7and Bi2YVO8withtetragonal structures were studied.These two compoundsinitiated both,H2and O2evolution,from water under UV irradia-tion.This is in spite of the fact that both of them showed strongoptical absorption in the visible region(λ4420nm)[81,82].2.1.4.d10Metal oxide photocatalystsVarious typical metal oxides(In3þ,Ga3þ,Ge4þ,Sn4þ,Sb5þ)with d10were shown to be effective photochemical water-splittingcatalysts under UV irradiation.Ni-loaded Ga2O3appears to be oneof the promising photocatalysts for overall water splitting[83].Itsphotocatalytic activity could be effectively improved by the additionof Ca,Cr,Zn,Sr,Ba,and Ta ions[84].Zn ion doping remarkablyimproved the photocatalytic activity,with an apparent quantumyield for Ni/Zn–Ga2O3of QE¼20%.By combining with Lu2O3,theresulting Zn-doped Lu2O3/Ga2O3proved to be a novel compositephotocatalyst for stoichiometric water splitting under UV irradia-tion.NiO was loaded as the cocatalyst,the quantum yield at320nmamounted to6.81%.Ga1.14In0.86O3showed the highest photocataly-tic activity for H2and O2evolution[85].In comparison,Y1.3In0.7O3,showed the highest photocatalytic activity for the overall watersplitting when combined with RuO2as a promoter[86].2.1.5.f0Metal oxide photocatalystsCeO2was reported to show a consistent activity towards O2production in aqueous solutions containing Fe3þand Ce4þaselectron acceptors[87].Sr2þ-doped CeO2was an active photo-catalyst for overall water splitting when RuO2was loaded as apromoter[88].Ce3þsupported zeolites showed higher photoca-talytic activity for water splitting[89].H2and O2evolution wasobserved.Photoirradiation of Ce3þspecies generated electrons(Ce3þþhν-Ce4þþeÀ)that were captured effectively by water molecules resulting the formation of molecular hydrogen.Yuanet al.[90]reported that BaCeO3produced H2and O2from aqueoussolutions containing CH3OH and AgNO3as sacrificial reagents,respectively.2.1.6.Nonoxide photocatalystsZnS in SO32Àsolutions were used for H2production under UVirradiation exhibiting90%quantum yield at313nm[91].CdSenanoribbons in Na2S/Na2SO3solution showed photocatalytic activ-ity for H2evolution under both,UV and visible light illumination.The quantum efficiency for this process has been reported to be0.09%[92].The photocatalytic water-splitting activity of GaN wasfound to be strongly dependent on the crystallinity of the materialand on the cocatalyst employed[93].Modification of well-crystallized GaN with Rh2Ày Cr y O3nanoparticles as a cocatalyst for H2evolution resulted in the stable stoichiometric decomposi-tion of H2O into H2and O2under UV irradiation.RuO2modifica-tion,on the other hand,did not bring about appreciable H2and O2evolution.However,Zn2þ,Mg2þ,and Be2þdoping of GaN con-verted it into a remarkably active and stable photocatalyst.Again,the presence of RuO2as a cocatalyst was required[94].β-Ge3N4 was another effective nitride photocatalyst to show efficientactivity for splitting water into H2and O2when combined withRuO2nanoparticles as reported by Domen's group[95,96].Thephotocatalytic activity of RuO2-loadedβ-Ge3N4was strongly dependent on the reaction conditions employed.The highest activity was obtained when the reaction was carried out in1M aqueous H2SO4solution.Moreover,treatment of as-prepared β-Ge3N4powder under high-pressure ammonia effectively increased the photocatalytic activity by up to4times.This was attributed to a decrease in the density of anion defects in the bulk and at the surface[97].A AgBr/SiO2catalyst showed a stable and high photocatalytic activity for H2generation from CH3OH/H2O solution under UV irradiation.The high activity of this AgBr/SiO2catalyst has been related to photogenerated Ag species acting as sites for H2 formation[98].Very recently,metal-free polymeric photocatalysts have attracted much attention.Wang et al.demonstrated that graphitic carbon nitrides(g-C3N4)can be photocatalytically active for H2evolution from water under visible light irradiation[99]. By introducing a mesoporous structure into polymeric C3N4,the efficiency of H2production from the photochemical reduction of water is increased by one order of magnitude and the quantum efficiency(QE)is1.4%[100,101].2.2.Visible light active photocatalysts for H2productionAlthough,more than100photocatalytic systems based on metal oxides have been reported to be active for the water splitting,most of them require ultraviolet(UV)light(λo400 nm)due to the large bandgap of these semiconductor materials [16–101].Since nearly half of the solar energy incident to the earth's surface lies in the visible region(400nm oλo800nm) (see Fig.3),it is essential to use visible light efficiently to realize H2 production on a large scale by photocatalytic water splitting.A maximum solar conversion efficiency for photocatalytic water splitting with a quantum efficiency of100%can be calculated using the standard solar spectrum.Even if all UV light up to400nm were utilized,the solar conversion efficiency would be only2%, which is similar to the maximum conversion efficiencies of photosynthesis in green plants under normalenvironmentalFig.3.Solar spectrum and maximum solar light conversion efficiencies for water splitting reaction with100%of quantum efficiency.Source:Ref.[103].A.A.Ismail,D.W.Bahnemann/Solar Energy Materials&Solar Cells128(2014)85–10189。