Transport of cimetidine by flounder and human renal organic anion transporter 1
葡萄糖转运蛋白SGLT1和GLUT2在槲皮素肠道吸收中的作用研究
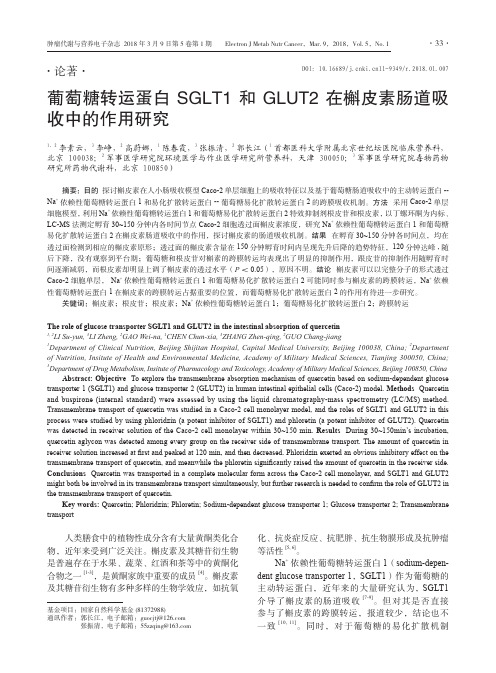
·论著·葡萄糖转运蛋白 SGLT1 和 GLUT2 在槲皮素肠道吸收中的作用研究1,2李素云,3李峥,2高蔚娜,1陈春霞,3张振清,2郭长江(1首都医科大学附属北京世纪坛医院临床营养科,北京 100038;2军事医学研究院环境医学与作业医学研究所营养科,天津 300050;3军事医学研究院毒物药物研究所药物代谢科,北京 100850)基金项目:国家自然科学基金(81372988)通讯作者:郭长江,电子邮箱:guocjtj@ 张振清,电子邮箱:55zzqing@摘要:目的 探讨槲皮素在人小肠吸收模型Caco-2单层细胞上的吸收特征以及基于葡萄糖肠道吸收中的主动转运蛋白--Na +依赖性葡萄糖转运蛋白1和易化扩散转运蛋白--葡萄糖易化扩散转运蛋白2的跨膜吸收机制。
方法 采用Caco-2单层细胞模型,利用Na +依赖性葡萄糖转运蛋白1和葡萄糖易化扩散转运蛋白2特效抑制剂根皮苷和根皮素,以丁螺环酮为内标、LC-MS 法测定孵育30~150分钟内各时间节点Caco-2细胞透过面槲皮素浓度,研究Na +依赖性葡萄糖转运蛋白1和葡萄糖易化扩散转运蛋白2在槲皮素肠道吸收中的作用,探讨槲皮素的肠道吸收机制。
结果 在孵育30~150分钟各时间点,均在透过面检测到相应的槲皮素原形;透过面的槲皮素含量在150分钟孵育时间内呈现先升后降的趋势特征,120分钟达峰,随后下降,没有观察到平台期;葡萄糖和根皮苷对槲素的跨膜转运均表现出了明显的抑制作用,跟皮苷的抑制作用随孵育时间逐渐减弱,而根皮素却明显上调了槲皮素的透过水平(P <0.05),原因不明。
结论 槲皮素可以以完整分子的形式透过Caco-2细胞单层, Na +依赖性葡萄糖转运蛋白1和葡萄糖易化扩散转运蛋白2可能同时参与槲皮素的跨膜转运,Na +依赖性葡萄糖转运蛋白1在槲皮素的跨膜转运占据重要的位置,而葡萄糖易化扩散转运蛋白2的作用有待进一步研究。
关键词:槲皮素;根皮苷;根皮素;Na +依赖性葡萄糖转运蛋白1;葡萄糖易化扩散转运蛋白2;跨膜转运The role of glucose transporter SGLT1 and GLUT2 in the intestinal absorption of quercetin 1, 2LI Su-yun, 3LI Zheng, 2GAO Wei-na, 1CHEN Chun-xia, 3ZHANG Zhen-qing, 2GUO Chang-jiang 1Department of Clinical Nutrition, Beijing Shijitan Hospital, Capital Medical University, Beijing 100038, China; 2Department of Nutrition, Insitute of Health and Environmental Medicine, Academy of Military Medical Sciences, Tianjing 300050, China; 3Department of Drug Metabolism, Insitute of Pharmacology and Toxicology, Academy of Military Medical Sciences, Beijing 100850, China Abstract: Objective To explore the transmembrane absorption mechanism of quercetin based on sodium-dependent glucose transporter 1 (SGLT1) and glucose transporter 2 (GLUT2) in human intestinal epithelial cells (Caco-2) model. Methods Quercetin and buspirone (internal standard) were assessed by using the liquid chromatography-mass spectrometry (LC/MS) method. Transmembrane transport of quercetin was studied in a Caco-2 cell monolayer model, and the roles of SGLT1 and GLUT2 in this process were studied by using phloridzin (a potent inhibitor of SGLT1) and phloretin (a potent inhibitor of GLUT2). Quercetin was detected in receiver solution of the Caco-2 cell monolayer within 30~150 min. Results During 30~150min’s incubation, quercetin aglycon was detected among every group on the receiver side of transmembrane transport. The amount of quercetin in receiver solution increased at first and peaked at 120 min, and then decreased. Phloridzin exerted an obvious inhibitory effect on the transmembrane transport of quercetin, and meanwhile the phloretin significantly raised the amount of quercetin in the receiver side. Conclusions Quercetin was transported in a complete molecular form across the Caco-2 cell monolayer, and SGLT1 and GLUT2 might both be involved in its transmembrane transport simultaneously, but further research is needed to confirm the role of GLUT2 in the transmembrane transport of quercetin.Key words: Quercetin; Phloridzin; Phloretin; Sodium-dependent glucose transporter 1; Glucose transporter 2; Transmembrane transportDOI:10.16689/11-9349/r.2018.01.007人类膳食中的植物性成分含有大量黄酮类化合物,近年来受到广泛关注。
药品生产技术《从天然活性物质中筛选和发现先导化合物》

如青蒿素是我国科学家从复合花序植物黄花蒿中提取得到的抗疟药,它被WHO评价为“继奎宁之后具有里程碑意 义的又一全新抗疟特效药”,后经结构修饰得到了青蒿素的衍生物蒿甲醚和青蒿琥酯。
第二页,共八页。
从天然活性物质中筛选和发现先导化合物
药品生产技术专业教学资源库
2从微生物及其代谢产物中筛选和发现先导化合物
如天然四环素类抗生素是从放线菌得到;第一个上市的羟甲戊二酰辅酶A还原酶抑制剂洛伐他汀为真菌代谢产物 。
第三页,共八页。
从天然活性物质中筛选和发现先导化合物
药品生产技术专业教学资源库
3从内源性活性物质结构研究中得到先导化合物
睾酮是由男性睾丸或女性卵巢分泌的内源性活性物质,但口服无效,对睾酮结构修饰得到甲睾酮,可供口服 。
第四页,共八页。
从天然活性物质中筛选和发现先导化合物
药品生产技术专业教学资源库
4从海洋生物发现先导化合物
海洋是个医药宝库,具备开发新药的巨大潜力,为新药的研发提供了大量的先导化合物。 在过去数十年间,在全球范围内已从海洋动物、海洋植物及海洋微生物中分离得到15000多个化合物。
如1945年,意大利科学家从撒丁岛海洋沉积淤泥中分离得到一株海洋真菌Ce Acremonium(顶头 孢霉菌),牛津大学Edward Abraham教授从中分离获得若干头孢霉素类化合物,其代表物是头孢 菌素C,进而对其结构修饰得到第一代头孢类抗生素头孢噻吩,成为从海洋微生物中发现并成功开发 的第一个“海洋抗生素”。
从天然活性物质中筛选和发现先导化合物
1从植物中提取分离的 有效成分发现先导化 合物。
药品生产技术专业教学资源库
2从微生物及其代谢产物 中筛选和发现先导化合物 。
3从内源性活性物质结 构研究中得到先导化
昆虫肠道菌群英语
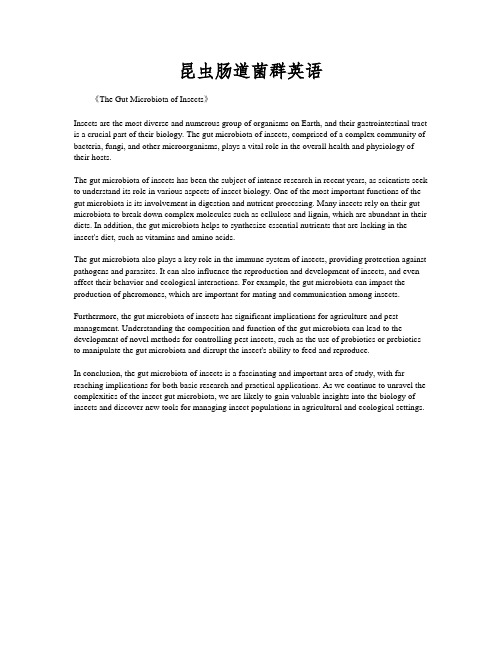
昆虫肠道菌群英语《The Gut Microbiota of Insects》Insects are the most diverse and numerous group of organisms on Earth, and their gastrointestinal tract is a crucial part of their biology. The gut microbiota of insects, comprised of a complex community of bacteria, fungi, and other microorganisms, plays a vital role in the overall health and physiology of their hosts.The gut microbiota of insects has been the subject of intense research in recent years, as scientists seek to understand its role in various aspects of insect biology. One of the most important functions of the gut microbiota is its involvement in digestion and nutrient processing. Many insects rely on their gut microbiota to break down complex molecules such as cellulose and lignin, which are abundant in their diets. In addition, the gut microbiota helps to synthesize essential nutrients that are lacking in the insect's diet, such as vitamins and amino acids.The gut microbiota also plays a key role in the immune system of insects, providing protection against pathogens and parasites. It can also influence the reproduction and development of insects, and even affect their behavior and ecological interactions. For example, the gut microbiota can impact the production of pheromones, which are important for mating and communication among insects.Furthermore, the gut microbiota of insects has significant implications for agriculture and pest management. Understanding the composition and function of the gut microbiota can lead to the development of novel methods for controlling pest insects, such as the use of probiotics or prebiotics to manipulate the gut microbiota and disrupt the insect's ability to feed and reproduce.In conclusion, the gut microbiota of insects is a fascinating and important area of study, with far-reaching implications for both basic research and practical applications. As we continue to unravel the complexities of the insect gut microbiota, we are likely to gain valuable insights into the biology of insects and discover new tools for managing insect populations in agricultural and ecological settings.。
中国科学家发现多糖口服吸收的秘密通道
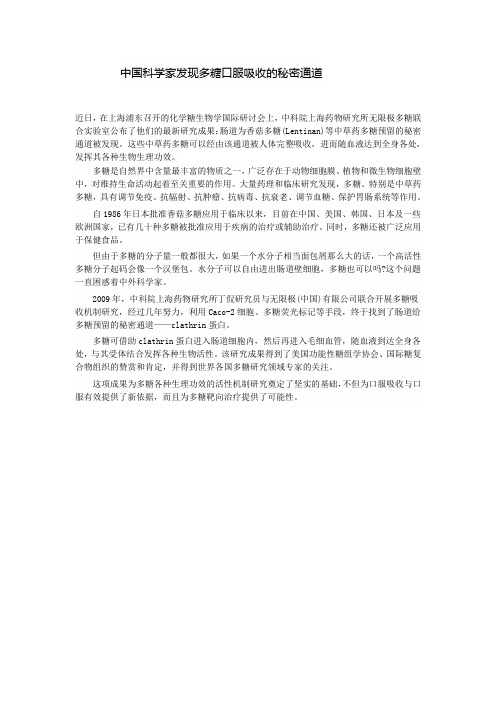
中国科学家发现多糖口服吸收的秘密通道近日,在上海浦东召开的化学糖生物学国际研讨会上,中科院上海药物研究所无限极多糖联合实验室公布了他们的最新研究成果:肠道为香菇多糖(Lentinan)等中草药多糖预留的秘密通道被发现。
这些中草药多糖可以经由该通道被人体完整吸收,进而随血液达到全身各处,发挥其各种生物生理功效。
多糖是自然界中含量最丰富的物质之一,广泛存在于动物细胞膜、植物和微生物细胞壁中,对维持生命活动起着至关重要的作用。
大量药理和临床研究发现,多糖、特别是中草药多糖,具有调节免疫、抗辐射、抗肿瘤、抗病毒、抗衰老、调节血糖、保护胃肠系统等作用。
自1986年日本批准香菇多糖应用于临床以来,目前在中国、美国、韩国、日本及一些欧洲国家,已有几十种多糖被批准应用于疾病的治疗或辅助治疗。
同时,多糖还被广泛应用于保健食品。
但由于多糖的分子量一般都很大,如果一个水分子相当面包屑那么大的话,一个高活性多糖分子起码会像一个汉堡包。
水分子可以自由进出肠道壁细胞,多糖也可以吗?这个问题一直困惑着中外科学家。
2009年,中科院上海药物研究所丁侃研究员与无限极(中国)有限公司联合开展多糖吸收机制研究,经过几年努力,利用Caco-2细胞、多糖荧光标记等手段,终于找到了肠道给多糖预留的秘密通道——clathrin蛋白。
多糖可借助clathrin蛋白进入肠道细胞内,然后再进入毛细血管,随血液到达全身各处,与其受体结合发挥各种生物活性。
该研究成果得到了美国功能性糖组学协会、国际糖复合物组织的赞赏和肯定,并得到世界各国多糖研究领域专家的关注。
这项成果为多糖各种生理功效的活性机制研究奠定了坚实的基础,不但为口服吸收与口服有效提供了新依据,而且为多糖靶向治疗提供了可能性。
【药品名】雌莫司汀【英文名】Estramustine

【药品名】雌莫司汀【英文名】Estramustine【别名】雌莫司汀磷酸钠;癌腺治;雌氮芥磷酸二钠;雌氮芥磷酸钠;雌二醇氮芥磷酸钠;雌莫司汀磷酸二钠;磷雌醇氮芥钠;Emecyt;Estracyt;EstramustinePhosphateSodium;EstramustineDisodiumPhosphate【剂型】1.胶囊:140mg;2.注射剂(粉):150mg,300mg,每盒有10瓶ETM及10注射稀释液。
【药理作用】磷酸雌二醇氮芥为烷化剂(正氮芥)与激素(雌二醇-17-β-磷酸盐)通过氨基甲酸酯连接组成。
该药进入体内后迅速脱磷酸,形成具有细胞毒活性的代谢物雌二醇氮芥(E2M),进而氧化成雌酮氮芥(E1M),发挥细胞毒性作用。
E2M和E1M与前列腺癌细胞中雌二醇结合蛋白(EMBP)有高度亲和力,能被前列腺癌组织大量摄取。
研究表明E2M与E1M细胞毒作用为抑制前列腺细胞有丝分裂,裂解已形成的微管并阻止其再形成。
E2M还促使前列腺癌细胞中谷胱甘肽的排空,增加其抗有丝分裂作用。
另外,该药还有微弱的雌激素作用和明显抗促性腺激素作用,减少睾酮的产生和分泌。
本药为细胞毒抗癌药,以雌二醇17磷酸酯为载体,具有烷化剂及雌激素的双重作用,可通过类固醇受体特异性地把药物导入前列腺组织,产生细胞毒效能和激素效果,达到破坏癌细胞的目的。
由于药物对肿瘤作用的专一性,从而提高了疗效,并减轻了烷化剂的全身反应。
药物的细胞毒效能包括:阻止前列腺癌细胞的有丝分裂;裂解已形成的微管并阻止微管的再形成;对前列腺癌的细胞核蛋白质起作用;促使前列腺癌细胞中谷胱甘肽的排空。
本药治疗剂量时对骨髓的抑制极少或无影响,对未经治疗的以及已经用过常规激素治疗失败的患者同样有效。
由于其严重不良反应的发生率低,可用于长期治疗。
【药动学】口服吸收良好,吸收率约为75%,药物浓集于前列腺组织中。
用药后,迅速脱磷氧基形成雌二醇氮芥,其大部分被氧化为雌酮氮芥。
昆虫谷氨酸门控氯离子通道研究进展
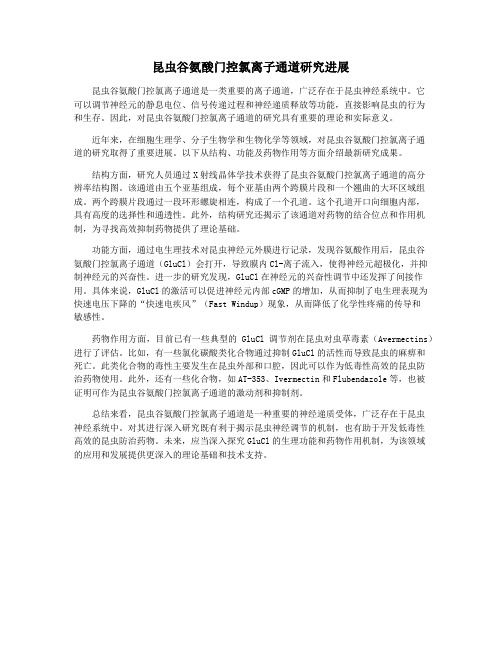
昆虫谷氨酸门控氯离子通道研究进展昆虫谷氨酸门控氯离子通道是一类重要的离子通道,广泛存在于昆虫神经系统中。
它可以调节神经元的静息电位、信号传递过程和神经递质释放等功能,直接影响昆虫的行为和生存。
因此,对昆虫谷氨酸门控氯离子通道的研究具有重要的理论和实际意义。
近年来,在细胞生理学、分子生物学和生物化学等领域,对昆虫谷氨酸门控氯离子通道的研究取得了重要进展。
以下从结构、功能及药物作用等方面介绍最新研究成果。
结构方面,研究人员通过X射线晶体学技术获得了昆虫谷氨酸门控氯离子通道的高分辨率结构图。
该通道由五个亚基组成,每个亚基由两个跨膜片段和一个翘曲的大环区域组成。
两个跨膜片段通过一段环形螺旋相连,构成了一个孔道。
这个孔道开口向细胞内部,具有高度的选择性和通透性。
此外,结构研究还揭示了该通道对药物的结合位点和作用机制,为寻找高效抑制药物提供了理论基础。
功能方面,通过电生理技术对昆虫神经元外膜进行记录,发现谷氨酸作用后,昆虫谷氨酸门控氯离子通道(GluCl)会打开,导致膜内Cl-离子流入,使得神经元超极化,并抑制神经元的兴奋性。
进一步的研究发现,GluCl在神经元的兴奋性调节中还发挥了间接作用。
具体来说,GluCl的激活可以促进神经元内部cGMP的增加,从而抑制了电生理表现为快速电压下降的“快速电疾风”(Fast Windup)现象,从而降低了化学性疼痛的传导和敏感性。
药物作用方面,目前已有一些典型的GluCl调节剂在昆虫对虫草毒素(Avermectins)进行了评估。
比如,有一些氯化碳酸类化合物通过抑制GluCl的活性而导致昆虫的麻痹和死亡。
此类化合物的毒性主要发生在昆虫外部和口腔,因此可以作为低毒性高效的昆虫防治药物使用。
此外,还有一些化合物,如AT-353、Ivermectin和Flubendazole等,也被证明可作为昆虫谷氨酸门控氯离子通道的激动剂和抑制剂。
总结来看,昆虫谷氨酸门控氯离子通道是一种重要的神经递质受体,广泛存在于昆虫神经系统中。
白念珠菌群体感应分子法尼醇对巨噬细胞Ana-1和RAW264.7增殖、凋亡和迁移能力的影响
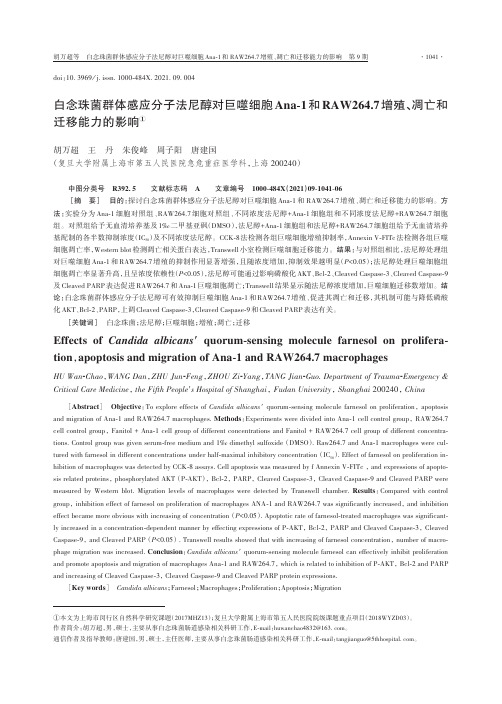
doi:10.3969/j.issn.1000-484X.2021.09.004白念珠菌群体感应分子法尼醇对巨噬细胞Ana-1和RAW264.7增殖、凋亡和迁移能力的影响①胡万超王丹朱俊峰周子阳唐建国(复旦大学附属上海市第五人民医院急危重症医学科,上海200240)中图分类号R392.5文献标志码A文章编号1000-484X(2021)09-1041-06[摘要]目的:探讨白念珠菌群体感应分子法尼醇对巨噬细胞Ana-1和RAW264.7增殖、凋亡和迁移能力的影响。
方法:实验分为Ana-1细胞对照组、RAW264.7细胞对照组、不同浓度法尼醇+Ana-1细胞组和不同浓度法尼醇+RAW264.7细胞组。
对照组给予无血清培养基及1‰二甲基亚砜(DMSO),法尼醇+Ana-1细胞组和法尼醇+RAW264.7细胞组给予无血清培养基配制的各半数抑制浓度(IC50)及不同浓度法尼醇。
CCK-8法检测各组巨噬细胞增殖抑制率,Annexin V-FITc法检测各组巨噬细胞凋亡率,Western blot检测凋亡相关蛋白表达,Transwell小室检测巨噬细胞迁移能力。
结果:与对照组相比,法尼醇处理组对巨噬细胞Ana-1和RAW264.7增殖的抑制作用显著增强,且随浓度增加,抑制效果越明显(P<0.05);法尼醇处理巨噬细胞组细胞凋亡率显著升高,且呈浓度依赖性(P<0.05),法尼醇可能通过影响磷酸化AKT、Bcl-2、Cleaved Caspase-3、Cleaved Caspase-9及Cleaved PARP表达促进RAW264.7和Ana-1巨噬细胞凋亡;Transwell结果显示随法尼醇浓度增加,巨噬细胞迁移数增加。
结论:白念珠菌群体感应分子法尼醇可有效抑制巨噬细胞Ana-1和RAW264.7增殖、促进其凋亡和迁移,其机制可能与降低磷酸化AKT、Bcl-2、PARP,上调Cleaved Caspase-3,Cleaved Caspase-9和Cleaved PARP表达有关。
2009-plant root growth, architecture and function - Hodge
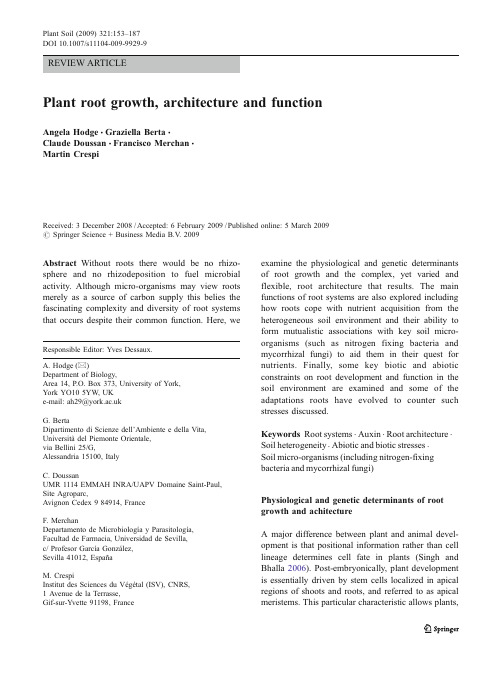
REVIEW ARTICLEPlant root growth,architecture and function Angela Hodge&Graziella Berta&Claude Doussan&Francisco Merchan&Martin CrespiReceived:3December2008/Accepted:6February2009/Published online:5March2009 #Springer Science+Business Media B.V.2009Abstract Without roots there would be no rhizo-sphere and no rhizodeposition to fuel microbial activity.Although micro-organisms may view roots merely as a source of carbon supply this belies the fascinating complexity and diversity of root systems that occurs despite their common function.Here,we examine the physiological and genetic determinants of root growth and the complex,yet varied and flexible,root architecture that results.The main functions of root systems are also explored including how roots cope with nutrient acquisition from the heterogeneous soil environment and their ability to form mutualistic associations with key soil micro-organisms(such as nitrogen fixing bacteria and mycorrhizal fungi)to aid them in their quest for nutrients.Finally,some key biotic and abiotic constraints on root development and function in the soil environment are examined and some of the adaptations roots have evolved to counter such stresses discussed.Keywords Root systems.Auxin.Root architecture. Soil heterogeneity.Abiotic and biotic stresses.Soil micro-organisms(including nitrogen-fixing bacteria and mycorrhizal fungi)Physiological and genetic determinants of root growth and achitectureA major difference between plant and animal devel-opment is that positional information rather than cell lineage determines cell fate in plants(Singh and Bhalla2006).Post-embryonically,plant development is essentially driven by stem cells localized in apical regions of shoots and roots,and referred to as apical meristems.This particular characteristic allows plants,Plant Soil(2009)321:153–187DOI10.1007/s11104-009-9929-9Responsible Editor:Yves Dessaux.A.Hodge(*)Department of Biology,Area14,P.O.Box373,University of York,York YO105YW,UKe-mail:ah29@G.BertaDipartimento di Scienze dell’Ambiente e della Vita, Universitàdel Piemonte Orientale,via Bellini25/G,Alessandria15100,ItalyC.DoussanUMR1114EMMAH INRA/UAPV Domaine Saint-Paul, Site Agroparc,Avignon Cedex984914,FranceF.MerchanDepartamento de Microbiología y Parasitología, Facultad de Farmacia,Universidad de Sevilla,c/Profesor García González,Sevilla41012,EspañaM.CrespiInstitut des Sciences du Végétal(ISV),CNRS,1Avenue de la Terrasse,Gif-sur-Yvette91198,Francewhich are sessile organisms,to adapt their morphol-ogy and organ development to the encountered environmental conditions.The spatial configuration of the root system(number and length of lateral organs),so-called root architecture,vary greatly depending on the plant species,soil composition, and particularly on water and mineral nutrients availability(Malamy2005).Plants can optimize their root architecture by initiating lateral root primordia and influencing growth of primary or lateral roots. The root system results from the coordinated control of both genetic endogenous programs(regulating growth and organogenesis)and the action of abiotic and biotic environmental stimuli(Malamy2005).The interactions between these extrinsic and intrinsic signals however complicate the dissection of specific transduction pathways.Such complex traits likely depending on multiple genes may be analyzed through quantitative genetics via the identification of quantitative trait loci(QTL)linked to root architecture (e.g.Mouchel et al.2004,Fitz Gerald et al.2006). Understanding the molecular mechanisms governing such developmental plasticity is therefore likely to be crucial for crop improvement in sustainable agricul-ture.In this section,we focus on hormonal and genetic determinants of root architecture.The embryonic root apical meristem(RAM) specification occurs very early in embryo develop-ment(Benfey and Scheres2000).The RAM con-stitutes the stem cell niche that eventually produces all below-ground organs,including lateral roots (Sabatini et al.2003).The cellular organization of Arabidopsis RAMs is very regular,with the initials of the various tissue types surrounding a quiescent center(QC).An asymmetric division of the hypoph-ysis generates a large basal daughter and a small apical daughter,called the lens-shaped cell,which is the progenitor of the QC.During root initiation,the QC appears to act like an organizing center that can direct surrounding cells to produce a set of initials (Aida et al.2004,Jenik et al.2007).Different mechanisms specify the initials above the QC that give rise to the ground tissue and the stele(proximal initials)as well as the initials below the QC that produce the root cap(distal initials).This root cap and the underlying root apical meristem form the root zone called the distal root apex,where cells divide actively.At certain distance from the RAM,the anticlinal and asymmetric divisions of a group of pericycle cells are the initial event of the formation of a lateral root.Several consequent divisions result in the formation of a dome-shape primordium that grows through outer cell layers and a meristem is estab-lished.Upon activation of this meristem,the lateral root emerges from the parental root and continue to grow in the soil(Malamy1995and references therein).Phytohormonal regulation of the root system:Auxin as a major playerThe different stages of root development are con-trolled and regulated by various phytohormones with auxin playing a major role(reviewed by Leyser 2006).In roots,auxin is involved in lateral root formation,maintenance of apical dominance and adventitious root formation.All these developmental events require correct auxin transport and signaling.During embryonic RAM formation many auxin-related mutants,such as those involved in its biosynthesis yuc1yuc4yuc10yuc11quadruple mutant (Cheng et al.2007),or mutants affected in transport or signaling(monopteros,bodenlos,auxin transport inhibitor resistant1(tir1)and related tir1/afb1–3 quadruple mutant(Dharmasiri et al.2005,Jenik et al. 2007)are unable to form the embryonic RAM.The auxin flux coming from the apical region of the embryo into the hypophysis leads to TIR1(and related redundant AFBs)pathway activation and induction of auxin-response genes such as PIN genes (coding for auxin efflux carriers),whose products will increase auxin transport and accumulation to further differentiate the hypothesis(Benkova et al.2003).Auxin also plays a major role in lateral root initiation and teral root development can be divided in different steps:primordium initia-tion and development,emergence,and meristem activation.Auxin local accumulation in Arabidopsis root pericycle cells adjacent to xylem vessels,triggers lateral root initiation by re-specifying these cells into lateral root founder cells(Dubrovsky et al.2008). Furthermore,it is also involved in the growth and organization of lateral root primordia and emergence from the parent root(Laskowski et al.2006).Indeed, mutants or transgenic lines with elevated auxin biosynthesis and endogenous levels of IAA display significant increased root branching(Seo et al.1998, King et al.1995).In addition,overexpression of theDFL1/GH3-6or the IAMT1genes,which encode enzymes modulating free IAA levels,results in a reduction in lateral root formation(Staswick et al. 2005;Qin et al.2005).Hence,normal auxin biosyn-thesis and homeostasis are necessary for lateral root initiation in Arabidopsis.However,overall auxin content is not always positively correlated with lateral root number(Ivanchenko et al.2006).Auxin transport into the regions where lateral root initiate also seems crucial for the regulation of root branching(Casimiro et al.2001).Functional analyses of mutants impaired in auxin transport,such as the aux1,axr4,and pin multiple mutants(Benkova et al. 2003;Marchant et al.2002),have demonstrated the crucial role of auxin transport in lateral root forma-tion.In the root,auxin is transported in both an acropetal(base-to-apex;Ljung et al.2005)and basipetal(apex-to-base)direction within inner and outer tissues of the root apex(Swarup and Bennett 2003).Studies using auxin transport inhibitors have shown that the polarity of auxin movement provides an important developmental signal during lateral root initiation(Casimiro et al.2001)and root gravitropism (Parry et al.2001).The strong bias in the direction of auxin transport within a tissue results from the asymmetrical cellular localization of an influx system involving the AUX1protein(Swarup and Bennett 2003)and an efflux apparatus with PIN-type efflux facilitators(reviewed by Paponov et al.2005).The auxin efflux system regulated by PIN protein family members is also crucial for lateral root development(Benkova et al.2003).Although not all pin mutations affect lateral root formation,multiple pin mutants have dramatic defects in root patterning, including lateral root development(Benkova et al. 2003;Blilou et al.2005).Cellular localisation of PIN proteins is also important as a gnom mutant allele is defective in PIN-dependent developmental processes, including lateral root primordium development,pre-sumably due to disorganized PIN1localization (Geldner et al.2004).When auxin reaches the target tissue it induces a transcriptional response.Several auxin-responsive genes and gene families involved in auxin signalling have been identified,of which the Aux/IAAs and ARFs are the best studied.Auxin induces expression of Aux/IAA proteins,which in many cases reduces the sensitivity of cells toward auxin.This induction is mediated by ARF proteins that bind to the auxin-responsive elements(AuxREs)in the promoters of auxin-responsive genes,and activate or repress transcription through interaction with specific Aux/ IAA proteins(Liscum and Reed2002).These loops represent extensive feedback as well as feed-forward regulation(for further information on Aux/IAA pro-teins,their degradation and their interaction with ARF transcription factors see the review by Benjamins and Scheres2008).Alterations in these control systems affect several auxin-dependent plant pathways includ-ing root development.For example,normal differen-tiation of a root cap from the distal initials depends on a pair of redundantly acting auxin response factors, ARF10and ARF16(Wang et al.2005).In double mutants,the root cap initials overproliferate,thereby generating a mass of undifferentiated progeny. Another example are the gain-of-function solitary root(slr)mutants which carries a stabilizing mutation in the IAA14negative regulator of auxin signaling and cannot form lateral root primordia.Detailed analyses of the slr mutant demonstrated that the slr mutation blocks early cell divisions during initiation (Fukaki et al.2002)Other hormones involved in the control of root developmentAlthough auxin plays a fundamental role in root growth and development,several other phytorho-mones modulate auxin action and consequently affect root development and architecture.In contrast to auxin,exogenous cytokinin application suppresses lateral root formation,and transgenic Arabidopsis plants with decreased cytokinin levels display in-creased root branching and enhanced primary root growth(Werner et al.2003).The notion that cytokinin negatively regulates root growth has also been verified by studies of cytokinin perception and signalling.For instance,double mutants for the redundant Arabidopsis cytokinin receptors AHK2 and AHK3display a faster growing primary root and greatly increased root branching(Riefler et al. 2006).To identify the stage of lateral root develop-ment sensitive to cytokinin,Laplaze et al.(2007) performed transactivation experiments that revealed that xylem-pole pericycle cells,but not lateral root primordial,are sensitive to cytokinin.Cytokinins perturb the expression of PIN genes in lateral root founder cells and prevent the formation of the auxingradient required to pattern the primordia.Recently cytokinin have also been shown to induce differenti-ation of cells situated between the root meristem and the elongation zone(Dello Ioio et al.2007),providing new insights on the molecular mechanisms involved in root meristem maintenance.The actively growing primary root of dicotyledon plants may exhibit apical dominance forcing their lateral roots to develop further away from the root tip (Lloret and Casero2002).Root apical dominance may be triggered by the root-cap-synthesized cytoki-nin and the balance between auxin and cytokinin regulates root gravitropism,a major determinant of root architecture(Aloni et al.2006).The control of lateral root initiation depends on the primary root vascular system which conveys spatial information as well as photosynthetic carbon to the newly formed primordial(Parizot et al.2008).Three types of vascular differentiation can be distinguished:(1) primary differentiation,which occurs in cells origi-nating from the primary vascular meristem,the procambium;(2)secondary differentiation,where the derivates originate from the vascular cambium; and(3)regenerative differentiation,in which the vascular elements re-differentiate from parenchyma cells at lateral root junctions.The vascular tissues are induced by polar auxin movement along the plant body,from the hydathodes of young leaves downward to the root tips.Cytokinin by itself does not induce vascular tissues,but in the presence of auxin,it promotes vascular differentiation and regeneration,a critical process for the establishment of a root system (Aloni et al.2006).Another hormone involved in lateral root for-mation is ethylene.Treatments that induce C2H4 production(i.e.flooding)promote adventitious and lateral root formation(Mergemann and Sauter2000). Ethylene stimulates auxin biosynthesis and basipetal auxin transport toward the elongation zone,where it activates a local auxin response leading to inhibition of cell elongation.Stepanova et al.(2005)showed that ethylene-triggered inhibition of root growth is mediated by the action of the weak ethylene insensitive2/anthranilate synthaseα1(WEI2/ASA1) and wei7/anthranilate synthaseß1(ASB1)genes that encode rate-limiting tryptophan biosynthesis enzymes In addition,ethylene modulates the tran-scription of several components of the auxin trans-port machinery and induces a local activation of the auxin signaling pathway to regulate root growth (Ruzicka et al.2007).Abscisic acid(ABA),a universal stress hor-mone,plays a significant role in stimulating elongation of the main root and emergence of lateral roots in response to drought(De Smet et al. 2006b).As with other hormones,the complexity of the plant responses induced by this hormone makes it difficult to distinguish primary from secondary effects(e.g.stomata closure affects water movement in the plant).In general,ABA has an antagonistic effect on lateral root primordial formation and emergence to auxin,which initiates lateral roots (Malamy2005).This role of ABA in the control of the activity of the lateral root meristems has a significant impact on the final size and architecture of the root system(De Smet et al.2006b).Indeed, responsiveness to certain abiotic factors is lost in certain ABA signaling mutants(Signora et al.2001; He et al.2005;Fujii et al.2007)such as the systemic inhibition of lateral root formation in high N conditions in the ABA-insensitive mutant abi4 (Signora et al.2001).Genetic and genomic approaches to analyze root growth and architectureForward and reverse genetic approaches have been undertaken to elucidate the genetic control of lateral root formation.As expected,most of the lateral root mutants(approximately40%)identified in A.thaliana are affected in a specific component of the auxin pathways.Their phenotypes can usually be rescued or mimicked through auxin application.Several mutants having lateral root phenotypes(De Smet et al.2006a) have been linked to auxin signaling(axr mutants,tir1, msg2-1,shy2),auxin transport(aux1and pin mutants),and auxin homeostasis(alf1,ydk1,dfl1). Analysis of these mutants has led to the identification of several genes that regulate lateral root formation and/or coordinate this process in response to environ-mental cues(reviewed in Osmont et al.2007).Few Arabidopsis mutants such as the previously men-tioned slr-1/AUXIAA14(Fukaki et al.2002)and alf4-1(Celenza et al.1995),are not capable of initiating any lateral roots.The encoded ALF4protein is conserved among plants and has no similarities to proteins from other kingdoms.The Arabidopsis gene is involved in lateral root initiation but not in primaryroot formation as the alf4-1mutant forms a primary root.DiDonato et al.(2004)have shown that ALF4 functions are required to maintain the pericycle in a mitosis-competent state needed for lateral root forma-tion.Other mutants are affected in vascular tissues and consequently cannot produce lateral roots(e.g. Ohashi-Ito and Bergmann2007).In contrast,ectopic expression of PLT genes, coding for AP2-type transcription factors,trigger de novo formation of roots from shoot structures.PLT1 was isolated by reverse genetics technologies based on the identification of a QC–and stem-cell–specific enhancer trap line.Indeed,PLT genes are pivotal determinants of QC and stem cell identity(Aida et al.2004).Although plt1mutants only display mild root growth defects,the establishment and mainte-nance of the QC and stem cells in the root meristem, are severely impaired in the plt1plt2double mutant. NAC1is another transcription factor implicated in lateral root development since its overexpression enhances lateral root initiation(Xie et al.2002).A genetic framework for the control of cell division and differentiation in root meristematic cells have been recently proposed(Dello Ioio et al.2008).A second strategy used to identify non-essential modulators of root development,is exploiting natural genetic variation by analysis of quantitative trait loci(QTL).QTL mapping has the advantage of identifying genomic regions containing genes with subtle effects masked in a particular genetic background.For instance,in A.thaliana and maize QTLs linked to root architecture have been identified (Mouchel et al.2004;Tuberosa et al.2002;Loudet et al.2005).Exploring the polymorphisms underlying natural variation can identify the DNA sequence changes that lead to a modified root system architec-ture in nature.In recent years,several new genomics resources and tools(e.g.genome sequences,tens of thousands of molecular markers,microarrays,and knock-out collections)have become available to assist in QTL mapping and cloning,even if these genes have subtle effects on phenotype(see Paran and Zamir2003).For example,quantitative morpholog-ical and physiological variation analysis of a cross between isogenized Arabidopsis accessions revealed that the BREVIS RADIX(BRX)transcription factor controls the extent of cell proliferation and elonga-tion in the growth zone of the root tip(Mouchel et al. 2004).Genomics have provided new tools to explore downstream and upstream genes of broad regulatory signals such as hormone-responsive factors.Tran-scriptional changes during root branching in Arabi-dopsis have been monitored to identify key regulators of root architecture(Himanen et al.2004),including several core cell cycle genes.In addition,Vanneste et al.(2005)compared the early transcriptome of lateral root formation using synchronised induction of these organs in wild type and slr mutants.This revealed negative and positive feedback mechanisms that regulate auxin homeostasis and signalling in the pericyle initial cells.The advent of genomic resources have allowed the combination of global profiling with novel biological approaches such as enhancer trap lines driving green fluorescent protein(GFP)expression in specific root cell types(Brady et al.2007).These authors used transgenic lines that expressed GFP in specific root tissues to isolate corresponding fluores-cent protoplast populations derived from them and characterise their expression patterns using micro-arrays.Thus were able to determine which genes were expressed and at what level in a particular root cell type.Recently,these transgenic plants were used to characterise the transcriptional response to high salinity of different cell layers and developmental stages of the Arabidopsis root(Dinneny et al.2008). Transcriptional responses are highly constrained by developmental parameters further revealing interac-tions between developmental fates and environmental stresses.Genomic approaches mainly rely on the expression patterns of protein-coding genes.However,the dis-covery of small RNAs(microRNAs,miRNA;and small interfering RNAs,siRNA)in the last decade altered the paradigm that protein coding genes are the only significant components of gene regulatory net-works(Chapman and Carrington2007).Small RNAs are involved in a variety of phenomena that are essential for genome stability,establishment or main-tenance of organ identity,adaptive responses to biotic and abiotic stresses.In plants,MIRNAs are genes generally encoded in intergenic regions whose matu-ration requires a particular type III RNase named DICER-LIKE1(DCL1)(Chapman and Carrington 2007).The small mature miRNA is then incorporated in a protein complex,the so-called RISC(RNA-Induced Silencing Complex)that can recognizemRNAs partially complementary to the miRNA nucleotide sequence.This recognition event mediated by the RISC-loaded miRNA leads to cleavage or translational inhibition of the target mRNA.Mutants affected in miRNA metabolism(biosynthesis,action and transport as dcl1,ago1,hen1,hyl1,hst1,se)show pleiotropic phenotypes confirming the role of miR-NAs in diverse developmental processes(Chapman and Carrington2007).Several MIRNAs have been involved in root development.For example,Arabidopsis mir164a and mir164b mutant plants produced more lateral roots than wild-type plants(Guo et al.2005).These phenotypes are similar to those obtained by NAC1 overexpression,a transcription factor targetted by miR164(Xie et al.2002).Overexpression of miRNA-resistant NAC1mRNA results in slight increases in lateral root numbers.In addition,the auxin-related transcription factors ARF10and ARF16 are also targets of three related microRNA(miRNA) genes,miR160a,miR160b and miR160c.These miR-NAs limit the expression domain of ARF10and ARF16 to the columella,and have a complementary pattern. The over-expression of a miRNA-resistant ARF16 mRNA under its own promoter results in pleiotropic developmental defects.MIR160overexpressing plants, in which the expression of ARF10and ARF16is repressed,and the arf10-2arf16-2double mutants display the same root tip defect,with uncontrolled cell division and blocked cell differentiation in the root distal region showing a tumor-like root apex and loss of gravity-sensing(Wang et al.2005).Apart from these examples,due to the large diversity of these novel regulatory RNAs,it is likely that many other miRNAs or other small RNAs participate in the regulation of root development and architecture.Root meristematic cells integrate signals from the environment to regulate specific developmental responses and cope with external constraints.This post-embryonic growth and development requires the activation of hormone homeostasis and signalling pathways,transcriptional regulation by specific tran-scription factors and post-transcriptional regulation of developmental regulators by non-coding RNAs. These regulatory mechanisms may be particularly relevant to adjust differentiation processes to the environmental conditions encountered during growth, notably on primary and lateral root developmental programs.Root system achitectureRoot systems and architecture definitionsSoil is a complex medium with high spatial and temporal environmental variability at a wide range of scales,including those relevant to plant roots.The root system,with its extensive but structured devel-opment,can be considered as an evolutionary response to such spatio-temporal variability in re-source supply and associated constraints upon growth (Harper et al.1991).As a consequence,the extension in space and time of the root system is governed by genetically driven developmental rules which are modulated by environmental conditions.As demon-strated by QTL mapping which recently revealed that root morphology is in most cases regulated by a suite of small-effect loci that interact with the environment (de Dorlodot et al.2007).The architecture of a root system is the result of developmental processes and is a dynamic notion. Root architecture addresses two important concepts: the shape of the root system and its structure.The shape defines the location of roots in space and the way the root system occupies the soil.Its quantifica-tion is generally achieved by measuring variables such as root depth,lateral root expansion and root length densities.In contrast,root structure describes the variety of the components constituting the root system(roots and root segments)and their relation-ship(e.g.topology:connexion between roots;root gradients).While,root differentiation has important impacts upon structure—function relations(Clarkson 1996).The rhizosphere(i.e.the volume of soil around living plant roots that is influenced by root activity; Hinsinger et al.2005),is often simply thought of as a cylindrical shape around the root.However,this oversimplification does not account for integration at the root system level or for the inherent complexity of root systems which arise from geometry,temporal dynamics and the heterogeneous aspects of roots. These complexities are incorporated into the concept of root architecture.Root geometry is complex because of the specific motion in space of each root, the relative locations between roots and the possible overlapping of their zones of influence.The temporal dynamic comes both from the growth of the different root axes and from physiological processes associatedwith root segments (i.e.tissue differentiation)result-ing in temporal and spatial variability of function along the root axes.The diversity among roots within the root system and soil heterogeneity further increase this variability.Root classification and elaboration of the root system It has long been recognized that the in situ morphol-ogy of a root system can be complex and may vary greatly,even within a species (Weaver 1926;Cannon 1949;Kutschera 1960),reflecting the interplay be-tween developmental processes and environmental constraints (Fig.1).Consequently,the complexity of root systems has led to a number of different classification systems.These classifications can be based on:branch structure (topology)(Fitter 1987;2002),root activity (Wahid 2000)or development (Cannon 1949).The latter is the more classical approach and is useful in understanding growth as well as obtaining a more global view of root architecture,for example,in relation to plant habitat.Thus,developmental classification has been widely used in modelling approaches to simulate architecture (cf section below).From a developmental stand point roots are classified according to their ontogenesis into three main categories:primary,nodal and lateral roots(Cannon 1949;Harper et al.1991;Klepper 1992),the formation of which has been shown to be genetically separable (Hochholdinger et al.2004).This classification also reflects differences between monocot –and dicotyledon species:dicots root system is derived from primary roots and lateral branching (primary root system),with roots that may exhibit radial growth.Depending on the extension of laterals relative to the primary axis,the morphology of the root system will vary between taprooted and diffuse or fasciculate (Fig.1).In monocots,root systems derive not only from branching of primary roots but also from emmited nodal roots (adventitious root system).Monocot roots do not undergo secondary radial growth.The primary root differentiates from the seed ’s radicle already present in the seed embryo.This generally gives rise to a single-axis root system,or taproot system,with dominant vertical growth (gravitropism).Adventitious (or nodal)roots differ-entiate from organs other than roots (e.g.rhizomes,stems etc)and are initiated at precise locations (near stem nodes for example)with a defined temporal pattern,in coordination with the development of the shoot.They are often abundant and give rise to a fasciculated root system.Adventitious roots are much less sensitive to gravitropism than primary roots (Klepper 1992).Lateral roots originate from the branching of a parent axis,often at near rightangles,Fig.1Diversity of root systems.On the top row,root systems are little branched,while more profusely branched on the bottom row.Dominance of a single main axis increases from left to right (from Kutschera 1960)。
- 1、下载文档前请自行甄别文档内容的完整性,平台不提供额外的编辑、内容补充、找答案等附加服务。
- 2、"仅部分预览"的文档,不可在线预览部分如存在完整性等问题,可反馈申请退款(可完整预览的文档不适用该条件!)。
- 3、如文档侵犯您的权益,请联系客服反馈,我们会尽快为您处理(人工客服工作时间:9:00-18:30)。
Transport of cimetidine byflounder and human renal organic anion transporter1BIRGITTA C.BURCKHARDT,STEFAN BRAI,SO¨NKE WALLIS,WOLFGANG KRICK,NATASCHA A.WOLFF,AND GERHARD BURCKHARDTZentrum Physiologie und Pathophysiologie,Abteilung VegetativePhysiologie und Pathophysiologie,37073Go¨ttingen,GermanySubmitted12August2002;accepted infinal form15November2002Burckhardt,Birgitta C.,Stefan Brai,So¨nke Wallis, Wolfgang Krick,Natascha A.Wolff,and Gerhard Burckhardt.Transport of cimetidine byflounder and hu-man renal organic anion transporter1.Am J Physiol Renal Physiol284:F503–F509,2003.First published November12, 2002;10.1152/ajprenal.00290.2002.—The H2-receptor antag-onist cimetidine is efficiently excreted by the kidneys.In vivo studies indicated an interaction of cimetidine not only with transporters for basolateral uptake of organic cations but also with those involved in excretion of organic anions.We therefore tested cimetidine as a possible substrate of the organic anion transporters cloned from winterflounder (fROAT)and from human kidney(hOAT1).Uptake of[3H]ci-metidine into fROAT-expressing Xenopus laevis oocytes ex-ceeded uptake into control oocytes.AtϪ60-mV clamp poten-tial,1mM cimetidine induced an inward current,which was smaller than that elicited by0.1mM PAH.Cimetidine con-centrations exceeding0.1mM decreased PAH-induced in-ward currents,indicating interaction with the same trans-porter.At pH6.6,no current was seen with0.1mM cimetidine, whereas at pH8.6a current was readily detectable,suggesting preferential translocation of uncharged cimetidine by fROAT. Oocytes expressing hOAT1also showed[3H]cimetidine uptake. These data reveal cimetidine as a substrate for fROAT/hOAT1 and suggest that organic anion transporters contribute to cime-tidine excretion in proximal tubules.winterflounder renal organic anion transporter;organic an-ion transport;organic cation transport;kidneyAS A SPECIFIC ANTAGONIST OF histamine H2receptors, cimetidine acts on gastric parietal cells to inhibit HCl secretion stimulated by histamine,pentagastrin,and acetylcholine.Between50and80%of the dose admin-istered intravenously was recovered in urine as un-changed cimetidine,and elimination half-life wasϳ2h in healthy volunteers with normal kidney and hepatic function(24).The mean steady-state plasma concen-tration on a standard1,000-mg daily dose was1g/ml (range0.64–1.64g/ml).A renal clearance of600ml/ min(24),exceeding the glomerularfiltration rate by approximatelyfivefold,indicated an efficient tubular secretion of cimetidine.Therefore,cimetidine must in-teract with renal transporters,most probably with those located in the proximal tubules.Because at physiological pH part of the cimetidine is positively charged,the organic cation transport sys-tems of the proximal tubule cell are expected to be involved in cimetidine secretion.The two-step model of transcellular organic cation secretion consists of elec-trogenic uptake at the basolateral side,moving organic cations down their electrochemical gradient from blood into proximal tubular cells,and organic cation/proton exchange mediating uphill exit into the lumen.The cloned organic cation transporters,OCT1,OCT2,and OCT3,are electrogenic and likely contribute to organic cation uptake across the basolateral membrane(for reviews,see Refs.6and18).In accordance with their function,rat OCT1and OCT2were localized to the basolateral membrane of proximal tubule cells(16,25, 29).However,the precise location of OCT3is not yet known.Tetraethylammonium uptake by rat(29)and human(34)OCT1was inhibited by unlabeled cimeti-dine in the medium with apparent K i values of5.7and 166M,respectively.Similarly,transport of1-methyl-4-phenylpyridinium by rabbit OCT1was also inhibited by cimetidine(26).Translocation of radiolabeled cime-tidine,however,was slow or negligible in human and rat OCT1(10,34).Moreover,OCT1Ϫ/Ϫmice did not show reduced renal transport of cimetidine compared with their wild-type littermates(14),suggesting that OCT1may not play a significant role in renal cimeti-dine secretion.In contrast,rat OCT2showed signifi-cant,saturable transport of labeled cimetidine(10). The K m was21M,a value close to the K i of9.4M determined earlier for the inhibition of rat OCT2-me-diated tetraethylammonium uptake by cimetidine(29). In a chronic renal failure model,5⁄6nephrectomy,OCT2 expression and renal clearance of cimetidine were re-duced,whereas the expression of OCT1was unchanged in the remnant kidney(13).These data collectively suggested that OCT2plays a dominant role in cimeti-dine secretion in the rat.Tetraethylammonium trans-port by human OCT2was inhibited by cimetidine,with a K i of10.9M(at pH7.0)(1).The same K i(11M)Address for reprint requests and other correspondence: B. C.Burckhardt,Zentrum Physiologie und Pathophysiologie,Abteilung Vegetative Physiologie und Pathophysiologie,Georg-August Univer-sita¨t Go¨ttingen,Humboldtallee23,37073Go¨ttingen,Germany(E-mail:bcburckhardt@veg-physiol.med.uni-goettingen.de).The costs of publication of this article were defrayed in part by the payment of page charges.The article must therefore be hereby marked‘‘advertisement’’in accordance with18U.S.C.Section1734 solely to indicate this fact.Am J Physiol Renal Physiol284:F503–F509,2003. First published November12,2002;10.1152/ajprenal.00290.2002.was found for the inhibition of organic cation transport by isolated human proximal tubules (22).Because the expression of OCT1in the human kidney is low (9,33),OCT2may well dominate OCT1with respect to proxi-mal tubular cimetidine transport.An interaction of cimetidine with mouse (31)and rat (17,32)OCT3has also been demonstrated,and hence OCT3may add to overall cimetidine secretion,if it is indeed located in the basolateral membrane of proximal tubule cells.In the intact rat kidney,cimetidine inhibited the uptake not only of N 1-methylnicotinamide,a prototyp-ical substrate of organic cation transporters,but also of PAH,the classic substrate of the renal organic anion transporter (27,28).Similarly,the uptake of radiola-beled cimetidine from capillaries into proximal tubule cells was inhibited by tetraethylammonium and probe-necid,compounds known to interact with organic cat-ion and organic anion transporters,respectively (27,28).Analogous to organic cations,secretion of organic anions is a two-step process.For organic anions,up-take across the basolateral membrane occurs against an electrochemical potential difference,followed by an energetically downhill exit across the brush-border membrane (3).Of the cloned organic anion transport-ers,rat and human OAT1and OAT3have been local-ized to the basolateral membrane of proximal tubule cells,with OAT1being expressed mainly in the S2segment and OAT3in all three segments (4,12,15).Human (4)and rat (7,20)OAT3transported radiola-beled cimetidine with K m values of 40(rat OAT3)and 57M (human OAT3).Unlabeled cimetidine inhibited the uptake of labeled organic anions by human (4,15)and rat OAT3(5,7,20),and a K i of 46.8M has been determined for rat OAT3(21).Thus there is no doubt that cimetidine is a substrate of human and rat OAT3.With regard to OAT1,con flicting results have been re-ported.Human OAT1was inhibited by cimetidine (15),whereas rat OAT1was not signi ficantly affected (21).So far,a translocation of labeled cimetidine by any OAT1has not been shown,leaving open whether OAT1contrib-utes to cimetidine secretion in proximal tubules.PAH and cimetidine are small,polar compounds,as indicated by their structure and their octanol-water partition coef ficients (Fig.1).At physiological pH,the COOH group of PAH is negatively charged,whereas cimetidine is present as an uncharged as well as a positively charged molecule.Given a p K a of 6.9(1),76%of cimetidine is uncharged,and only 24%is positively charged at pH 7.4.Human OCT2has recently been shown to interact preferentially with positively charged cimetidine (1).Secretion of the uncharged cimetidineshould then be mediated by another transporter,pos-sibly OAT1and OAT3.Here,we report that flounder renal organic anion transporter,fROAT,and human OAT1translocate cimetidine.These data indicate that both organic cation and anion transporters are in-volved in proximal tubular cimetidine secretion and allow for an ef ficient renal excretion of this drug.MATERIALS AND METHODSIn vitro cRNA transcription.fROAT (30)and human OAT1(23)cDNAs were used as templates for cRNA synthesis.Plasmids were linearized with Not I,and in vitro cRNA tran-scription was performed using a T7mMessage mMachine Kit (Ambion,Huntingdon,UK).The resulting cRNAs were resus-pended in puri fied,RNAse-free water to a final concentration of 1–1.5g/l.Oocyte preparation and storage.Stage V and VI oocytes from Xenopus laevis (Nasco,Fort Atkinson,WI)were treated with collagenase (type CLS II,Biochrom,Berlin,Germany)and maintained at 16–18°C in control solution (in mM):90NaCl,3KCl,2CaCl 2,1MgCl 2,and 5HEPES/Tris,pH 7.6.One day after removal,oocytes were injected either with 30nl of cRNA (1g/l)coding for fROAT or with 23nl of cRNA (1.5g/l)encoding for human OAT1and maintained at 16–18°C in control solution supplemented with 100kU/IU penicillin,0.1mg/l streptomycin,and 2.5mM sodium pyru-vate.After 4days of incubation with daily medium changes,oocytes were used for electrophysiological as well as for tracer uptake studies.Oocytes injected with water served as controls.Uptake and electrophysiological studies.Expression of fROAT and human OAT1in oocytes was con firmed by com-paring the uptake of radiolabeled PAH between cRNA-in-jected oocytes and water-injected oocytes.Uptake of [3H]PAH (5Ci/ml;p -[glycyl-2-3H]aminohippuric acid)and [3H]cime-tidine (10Ci/ml;[N -methyl-3H]cimetidine)in oocytes was assayed for 30(fROAT)or 60min (human OAT1)at room temperature in control solution additionally containing ei-ther 0.099mM unlabeled and 0.001mM labeled PAH or 0.99mM unlabeled and 0.01mM labeled cimetidine.The incuba-tion was stopped by aspiration of the incubation medium and several washes with ice-cold control solution,and the oocytes were assayed for radioactivity as described by Wolff et al.(30).Electrophysiological studies were performed by the con-ventional two-microelectrode,voltage-clamp method (2).Oo-cytes were superfused with control solution followed by the same medium additionally containing PAH or cimetidine.The membrane potential of the oocytes was clamped at Ϫ60mV,and the current induced by 0.1mM PAH was measured to demonstrate functional expression of the fROAT protein.Voltage pulses between Ϫ90and ϩ10mV,in 10-mV incre-ments,were applied for 5s each,and steady-state currents were recorded to obtain current-voltage (I -V )relationships.In general,the I -V protocol was applied first undercontrolFig. 1.Chemical structures,p K a ,and octanol-water partition coef ficients (log P)of PAH and cimetidine as obtained from Refs.1,8,and 24.F504CIMETIDINE TRANSPORT BY F ROAT AND H OAT1conditions and then 30s after the superfusion solution was changed to the test solution.The difference between the steady-state currents measured in the presence and absence of substrates was considered as substrate-induced current.Chemicals.All chemicals,including PAH and cimetidine were of analytic grade and purchased from Merck (Darm-stadt,Germany)or Sigma (Deisenhofen,Germany).[3H]PAH and [3H]cimetidine were from New England Nuclear (Co-logne,Germany)and from Amersham Pharmacia Biotech (Buckinghamshire,UK),respectively.The concentrations of uncharged and positively charged cimetidine were calculated using the Henderson-Hasselbalch equation and the p K a listed in Fig.1.RESULTSPAH-and cimetidine uptake into fROAT-expressing oocytes.To test whether the fROAT translocates cime-tidine,evis oocytes were injected with cRNA cod-ing for fROAT.Four days later,these oocytes mediated uptake of 132.1Ϯ15.7pmol [3H]PAH ⅐30min Ϫ1⅐oocyte Ϫ1and 14.4Ϯ 4.23pmol [3H]cimetidine ⅐30min Ϫ1⅐oocyte Ϫ1.Water-injected control oocytes showed uptakes of 5.5Ϯ0.8pmol [3H]PAH ⅐30min Ϫ1⅐oocyte Ϫ1and 2.7Ϯ0.1pmol [3H]cimetidine ⅐30min Ϫ1⅐oocyte Ϫ1(Fig.2;representative experiment of a total of 3inde-pendent experiments,each with an average of 6–12oocytes/experimental condition).From these data,the fROAT-mediated uptake rates for [3H]PAH and [3H]ci-metidine are 126.6and 11.7pmol ⅐30min Ϫ1⅐oocyte Ϫ1,respectively.Despite a 10times lower substrate con-centration,PAH uptake exceeded that of cimetidine by a factor of 10.8.Nevertheless,cimetidine was translo-cated in fROAT-expressing oocytes signi ficantly more quickly than in water-injected control cells,indicating that this compound is a substrate for the organic anion transporter.Because the uptake of PAH through fROAT gener-ated an inward current (2),we tested next whethercimetidine is also able to induce such a current.The two-electrode,voltage-clamp technique allows a direct comparison of PAH-and cimetidine-induced currents in the same oocyte.As measured at a holding potential of Ϫ60mV,in paired experiments on 20oocytes from 14donors,application of 0.1mM PAH and of 1mM cimetidine resulted in inward currents of Ϫ22Ϯ2(data from Fig.3C )and Ϫ4Ϯ1nA (data from Fig.3D ),respectively.Despite a 10times lower concentration,the average PAH-induced current exceeded that of cimetidine by a factor of 5.5.No such currents were observed in water-injected control oocytes (data not shown).The small inward currents observed on application of cimetidine could be due either to a low af finity of cimetidine for fROAT or to low rates of translocation.To distinguish between these two possibilities,the con-centration whereby half-maximal saturation of the ci-metidine-induced current was observed (K 0.5)was de-termined.At Ϫ60mV and pH 7.6,cimetidine-induced currents tended to saturate at concentrations Ͼ250M.In three experiments,K 0.5was 45Ϯ9M,and the maximal cimetidine-induced current (⌬I max )ap-proached 2.92Ϯ0.03nA.Steady-state currents in fROAT-expressing oocytes were measured in the absence and presence of PAH and are plotted as a function of membrane potential in Fig.3A .Respective currents in the absence and pres-ence of cimetidine are shown in Fig.3B .PAH and,to a much lesser extent,cimetidine increased the slope con-ductance,indicating electrogenic uptake.When the substrate-induced currents for PAH (Fig.3C )and ci-metidine (Fig.3D )were plotted as a function of mem-brane potential between Ϫ90and ϩ10mV,a linear I -V relationship was obtained for PAH as well as for cime-tidine.Again,at all potentials,the cimetidine-induced currents were smaller than the PAH-induced currents.At pH 7.4,the reversal potential (E rev )for the PAH-induced current was Ϫ11.2Ϯ2.4mV (Fig.3C )and that for the cimetidine-induced current was Ϫ27.8Ϯ4.6mV (Fig.3D ).Superposition of PAH-and cimetidine-induced cur-rents.To test whether PAH and cimetidine utilize the same transporter,fROAT,we added increasing cimeti-dine concentrations in the presence of 0.1mM PAH to fROAT-expressing oocytes.PAH plus cimetidine-in-duced currents (I )were normalized to the PAH-induced current in the absence of cimetidine (I 0)as shown in Fig.4.The smallest cimetidine concentration applied (0.1mM)increased the total current,whereas a further increase in cimetidine concentration decreased it.At 5mM cimetidine,Ͻ40%of the current elicited by PAH alone was found.Thus PAH-and cimetidine-induced currents are additive only at low,and nonadditive at higher,cimetidine concentrations.The nonadditive be-havior indicates that PAH and cimetidine utilize the same transporter rather than two separate,electro-genic transporters in oocytes.Cimetidine-induced currents at pH 6.6and 8.6.To examine the in fluence of uncharged cimetidine (CIM 0)on fROAT,cimetidine-induced currents at pH 6.6andFig.2.[3H]PAH-and [3H]cimetidine-uptake in winter flounder or-ganic anion transporter (fROAT)-expressing oocytes.Oocytes were injected with cRNA coding for fROAT,and 4days later transport of 0.1mM PAH (filled bars)or of 1mM cimetidine (open bars)within an uptake period of 30min was assayed.Water-injected oocytes were treated similarly.A representative experiment of a total number of 3independent experiments,each with an average of 6–12oocytes/experimental condition,is shown.*Signi ficant difference between fROAT-expressing oocytes and water-injected controls,P Ͻ0.01.F505CIMETIDINE TRANSPORT BY F ROAT AND H OAT18.6were compared.At pH 6.6(Fig.5A ,F ),0.1mM total cimetidine did not induce a detectable current over the tested voltage range,indicating that 0.033mM CIM 0was insuf ficient to induce measurable currents.At 1mM,total cimetidine (0.333mM CIM 0)evoked inward currents at membrane potentials more negative than Ϫ20mV and outward currents at more depolarized potentials.At pH 8.6(Fig.5B ),comparable currents were induced by 0.1(0.098mM CIM 0)as well as by 1mM total cimetidine (0.98mM CIM 0).[3H]PAH and [3H]cimetidine uptake by human OAT1-expressing oocytes.To explore whether cimeti-dine is also a substrate of human OAT1,oocytes were injected with cRNA coding for human OAT1and as-sayed 4days later.In two-electrode voltage-clamp studies,neither PAH nor cimetidine induced an inward current.However,oocytes expressing human OAT1exhibited [3H]PAH and [3H]cimetidine uptake.Within 60min,the [3H]PAH-and the [3H]cimetidine-asso-ciated uptakes were 43.6Ϯ4.3and 20.1Ϯ4.3pmol/oocyte,respectively,whereas water-injected oocytes showed uptakes of 9.7Ϯ1.1and 6.8Ϯ0.3pmol/oocyte (Fig.6;representative experiment of a total of 4,each with an average of 6–15oocytes/experimental condi-tion).Thus human OAT1-mediated uptake rates were 33.9and 13.3pmol ⅐60min Ϫ1⅐oocyte Ϫ1for [3H]PAH and [3H]cimetidine,respectively.The ratio of human OAT1-mediated PAH over cimetidine uptake was 2.5in these experiments.DISCUSSIONIn the rat kidney in situ,cimetidine uptake from the peritubular capillaries into proximal tubule cells in-volved the organic cation and an organic anion trans-port system.Uptake of the organic cation N 1-methylni-cotinamide was inhibited by cimetidine with a K i of 0.16mM and uptake of the organic anion PAH with a K i of 1.7mM (28).Thus the af finity of the organic cation transporter for cimetidine was 10times higher than that of the organic anion transporter.TheuptakeFig. 3.PAH-and cimetidine-induced currents in oocytes expressing fROAT at pH 7.6.Steady-state currents were recorded in the absence (s )and pres-ence (ᮀ)of 0.1mM PAH (A )and in the absence (Œ)and presence of 1mM ci-metidine (‚;B ).The holding potential (V c )was Ϫ60mV,and the hyper-and depolarizing test pulses were applied for 5s and ranged from Ϫ90to ϩ10mV in 10-mV increments.C :PAH-depen-dent currents (F ),i.e.,currents ob-tained in the presence of 0.1mM PAH minus the currents obtained in the ab-sence of 0.1mM PAH.D :cimetidine-dependent currents (E ).The data were obtained in 20oocytes from 14donors in pairedexperiments.Fig.4.In fluence of increasing cimetidine concentrations on PAH-induced inward current.The effect of cimetidine concentrations from 0.1to 5mM was tested on the current evoked by 0.1mM PAH at a clamp potential of Ϫ60mV.Currents were normalized to currents induced by 0.1mM PAH in the absence of cimetidine.Data were obtained in 6oocytes from 3donors.F506CIMETIDINE TRANSPORT BY F ROAT AND H OAT1of radiolabeled cimetidine from the capillaries was inhibited by tetraethylammonium and probenecid,proving translocation by organic cation and anion transporters.As expected from uptake through paral-lel systems,the inhibitions by tetraethylammonium and probenecid were additive.Interestingly,probene-cid inhibited a larger portion of cimetidine uptake in situ than did tetraethylammonium (28).Also in the intact kidney,it was noted that organic cation and organic anion transport systems “do not see the degree of substrate ionization,”because interaction with cimetidine with both systems was rather unaffected,when capillary pH was changed between 6.0and 8.0(27).Moreover,the uptake of labeled cimetidine was the same at pH 6.0,7.4,and 8.0,suggesting that the transporters possibly interacted with both positively charged and un-charged cimetidine.These and related findings with dis-sociable primary,secondary,and tertiary amines led Ull-rich and colleagues (28)to postulate that substrates qualify for transport by the presence of a hydrophobic core and by their ability to form hydrogen bonds.Meanwhile,it is known that several transporters for organic ions exist in the kidneys.Of the cloned organic cation transporters,OCT1and OCT2have been local-ized to the basolateral membrane of rat kidney proxi-mal tubule cells (16).Translocation of labeled cimeti-dine was slow or absent for human (34)and rat (10)OCT1but clearly demonstrable for expressed rat OCT2(10).Thus OCT2may contribute signi ficantly to cime-tidine secretion,particularly in humans,where renal expression of OCT1is low (6).Human OCT2expressed in Chinese hamster ovary K1cells preferred the posi-tively charged cimetidine over uncharged cimetidine:at higher pH,i.e.,at a lesser abundance of charged cimetidine (CIM ϩ),human OCT2showed a decreased af finity for total (CIM 0plus CIM ϩ)cimetidine (1).Of the cloned organic anion transporters,OAT1and OAT3have been localized to the basolateral membrane of proximal tubule cells in rat (19)and human (4,12)kidneys.Because both transport PAH,they may have contributed to the PAH transport as determined in the intact rat kidney.There is no doubt that rat and human OAT3transport cimetidine (4,7,20).The in-teraction with the organic cation cimetidine has been taken as a characteristic of OAT3.Mutational analysis of rat OAT3revealed that the conserved cationic amino acids lysine 370and arginine 454are involved in the binding of PAH but not of cimetidine (7).Hydrophobic amino acid residues in the seventh transmembrane domain (tryptophan 334,phenylalanine 335,tyrosine 341,and other nearby residues)were found to be im-portant for both PAH and cimetidine transport by allowing the formation of hydrogen bonds and hydro-phobic interactions (8).Because these hydrophobic amino acids are fully conserved in OAT1,this trans-porter could accept cimetidine as well.evis oocytes expressing fROAT translocated la-beled PAH ϳ12times faster than did water-injected control oocytes.Transport of labeled cimetidine was also faster in fROAT-expressing oocytes,but the trans-port ratio of fROAT-expressing to water-injected oo-cytes was only four.In absolute terms,fROAT-mediatedFig.6.[3H]PAH-and [3H]cimetidine-uptake in human organic anion transporter 1(hOAT1)-expressing oocytes.Oocytes were injected with cRNA coding for hOAT1,and 4days later the uptake of 0.1mM PAH (filled bars)or of 1mM cimetidine (open bars)within 60min was assayed.Water-injected oocytes were treated similarily.A rep-resentative experiment from 4independent experiments with an average of 7–15oocytes/experimental condition is shown.*Signi fi-cant difference between uptake of [3H]PAH and [3H]cimetidine com-pared with control,P Ͻ0.01.Fig.5.Cimetidine-induced currents at pH 6.6and 8.6.Currents evoked by 0.1(F )or 1mM cimetidine (E )were determined at pH 6.6(top )and 8.6(bottom )as a function of clamp potential.Data were obtained in 6oocytes from 4different donors.F507CIMETIDINE TRANSPORT BY F ROAT AND H OAT1PAH uptake,i.e.,uptake in expressing minus uptake in water-injected oocytes wasϳ10times higher than uptake of cimetidine,although PAH concentration(0.1mM)was one-tenth of the cimetidine concentration(1mM).PAH and cimetidine induced inward currents.The current evoked by0.1mM PAH was approximatelyfive times higher than that induced by1mM cimetidine.If PAH and cimetidine were transported at equal rates,CIM0/␣-ketoglutarate2Ϫexchange would produce an inward cur-rent twice as large as PAHϪ/␣-ketoglutarate2Ϫexchange. However,because cimetidine uptake was only one-tenth of PAH uptake,the cimetidine-induced current should be one-fifth of the PAH-induced current.This was actually observed.Taken together,these data show that fROAT-expressing oocytes translocate both PAH and cimetidine, albeit at considerably different rates.To exclude that cimetidine uptake occurred through an endogenous transporter upregulated by the expres-sion of fROAT,we added PAH and cimetidine simulta-neously to oocytes and measured the resulting inward current.If cimetidine was taken up by a separate endogenous,electrogenic transporter,PAH-and cime-tidine-induced currents should be additive at all cime-tidine concentrations.If,however,PAH and cimetidine use the same carrier,increasing cimetidine concentra-tions should replace more and more PAH from fROAT. Because the maximum current induced by saturating cimetidine concentrations is lower than that induced by PAH,replacement of PAH by cimetidine should reduce the total current.This was actually observed at cimetidine concentrations exceeding0.5mM.Only at 0.1mM cimetidine was a small increase in total cur-rent observed,most likely due to translocation of cime-tidine by transporter units not saturated with PAH. Taken together,our experiments provide direct evi-dence for transport of cimetidine by fROAT.The next question was whether CIMϩ,CIM0,or both forms of cimetidine interact with fROAT.To discrimi-nate between these possibilities,we changed bath pH from7.6to either6.6or8.6.Given a p K a of6.9,the relative abundance of CIM0is33.4,83.4,and98.0%at pH6.6,7.6,and8.6,respectively.Conversely,CIMϩmakes up66.6,16.6,and2.0%of total cimetidine at these pH values.If fROAT would prefer CIM0over CIMϩ,currents should increase at a constant cimeti-dine concentration when pH is raised.Unfortunately, fROAT itself is pH dependent,as seen with the anionic PAH as a substrate:PAH-induced currents strongly decreased with increasing pH(data not shown),a be-havior found earlier for PAH and adefovir uptake by human OAT1(11).Thus it is not possible to clearly discern the effects of pH on fROAT itself and on the availability of the suited substrate(CIM0vs.CIMϩ). Taking a different approach,we added0.1or1mM cimetidine at pH6.6and8.6to fROAT-expressing oocytes and determined the current.At pH6.6,only1mM cime-tidine evoked a current,whereas at pH8.6both concen-trations induced similar currents.This result is best explained by assuming that CIM0is preferred over CIMϩ.At pH6.6and0.1mM total cimetidine,CIM0 concentration is33M,which was obviously too low to elicit a measurable current.At1mM total cimetidine,333M CIM0amply sufficed to show inward currents.AtpH8.6,98M CIM0at0.1mM total cimetidine concen-tration produced measurable currents as did980MCIM0at1mM cimetidine.We emphasize that our data donot exclude that CIMϩmay bind to,and be translocatedby,fROAT with low affinity.However,the majority ofcimetidine appears to be transported as uncharged CIM0.Thereby,fROAT does see the degree of ionization.How-ever,in the intact rat kidney,inhibition of PAH uptakeby cimetidine was pH independent(27).A possible expla-nation is that PAH uptake in situ occurred throughOAT1and OAT3in parallel.If OAT1and OAT3showopposing preferences for CIM0and CIMϩ,the overall pHdependence of cimetidine interaction with PAH transportshould vanish.The I-V relationships of PAH-and cimetidine-inducedcurrents reverse at inside negative membrane potentials.Although a negative E rev has also been found in ourearlier study(2),it remains to be clarified which ionscontribute to PAH-and cimetidine-induced currents.Ourfirst assumption was that the inward currents are due tothe electrogenic exchange of one PAHϪagainst one␣-ke-toglutarate2Ϫmolecule,causing the net efflux of onenegative charge.However,assuming that the PAH con-centration outside the oocyte is higher than inside,and ␣-ketoglutarate concentration outside lower than inside, E rev should be far on the positive side.Replacement ofPAHϪby CIM0or CIMϩwould theoretically shift E rev tothe left,i.e.,to less positive values.Such a shift wasindeed observed:E rev was aroundϪ10mV for PAH-induced current and approximatelyϪ27mV for cimeti-dine current(cf.Fig.3).However,the problem remainsthat E rev was negative under both conditions.It is possi-ble that fROAT interacts,in addition to PAH(or cimeti-dine)and␣-ketoglutarate,with chloride or hydroxyl ions.Chloride removal inhibited PAH transport and abolishedPAH-induced currents(data not shown),suggesting thatchloride ions may modify or even be translocated byfROAT.The large shift in E rev observed when bath pHwas changed from6.6to8.6suggests that hydroxyl ionsmay be translocated by fROAT.However,more experi-ments are needed to elucidate the ionic basis for thesubstrate-induced currents generated in fROAT-express-ing oocytes.In the phylogenetic tree,fROAT is positioned be-tween OAT1and OAT3and may,therefore,combinetransport properties of OAT1,i.e.,PAH/␣-ketogluta-rate exchange,and OAT3,i.e.,interaction with bothorganic anions and cimetidine.Therefore,it was notclear a priori whether ourfindings can be extrapolatedto mammals,particularly to human OAT1.We ex-pressed human OAT1in oocytes and found that ittransports both radiolabeled PAH and cimetidine.Rel-ative to PAH,cimetidine transport was even higher inhuman OAT1than in fROAT.Thus translocation ofcimetidine is no longer a specific feature of OAT3.Wetherefore propose that human OCT2,OAT1,and OAT3contribute to cimetidine excretion.Most likely,OCT2isresponsible for the cationic form,whereas OAT1takescare of the uncharged form of cimetidine,which actu-F508CIMETIDINE TRANSPORT BY F ROAT AND H OAT1。