Dust diffusion in protoplanetary discs by magnetorotational turbulence
Shen 1992 10 Be in Chinese loess
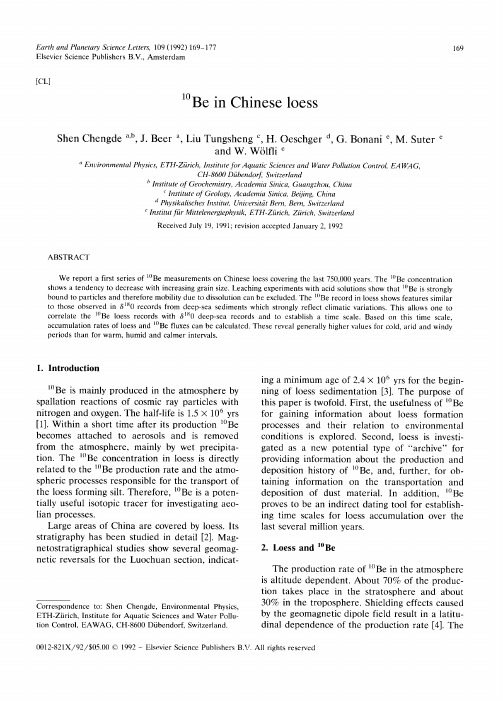
0012-821X/92/$05.00 © 1992 - Elsevier Science Publishers B.V. All rights reserved
170
S. C H E N G D E
E T AL.
global average production rate of J°Be determined from calculations based on large numbers of measurements is in the range 1.5-5.0 × 10 -2 a t o m s / c m 2 , s [5] [6]. After a mean residence time of 1-2 years ~°Be is removed from the atmosphere onto the surface of the earth, mainly by precipitation. Due to the transport processes the original latitudinal distribution is completely changed. The fallout pattern is strongly influenced by the discontinuity in the tropopause at mid-latitudes where, during the spring season, large stratospheric air masses are injected into the troposphere. ~°Be concentrations in rainwater therefore show seasonal effects [7]. About two-thirds of the ~°Be removed from the atmosphere is deposited in the ocean, from where it is scavenged in association with particulate matter and stored in the sediments. About one-third of the atmospheric ~°Be is deposited, on continents where it is strongly bound to particles. The subsequent history seems to be that of the particles: some particles are transported by wind and water, and they are finally deposited on land (e.g., loess) or in the sediments of the oceans, rivers and lakes, as well as in the ice of glaciated areas (polar regions and high mountains). Loess is an accumulation of wind-borne dust and silt which settles from the air both by rain and by dry deposition. The deposition of dust is controlled by many different parameters, such as atmospheric circulation on various scales and site-specific weather conditions. After deposition, loess formations develop in different ways, depending on the environmental conditions. During cold, arid periods loess is not much affected by weathering processes and biological activity. During warm, humid periods, however, high biological activity and strong weathering processes change the composition of loess and convert it into soils. This is clearly visible in the stratigraphy of loess, which is characterized by an alternation of loess layers with different types of palaeosol layers. The clear boundaries between loess and palaeosol indicate that the switching between the two conditions of the climate system was fast. This interpretation is confirmed by investigations into the grain-size distribution, carbonate content, pollen profiles and variations in the population of snails [8]. Since the formation of loess and
英语材基试卷
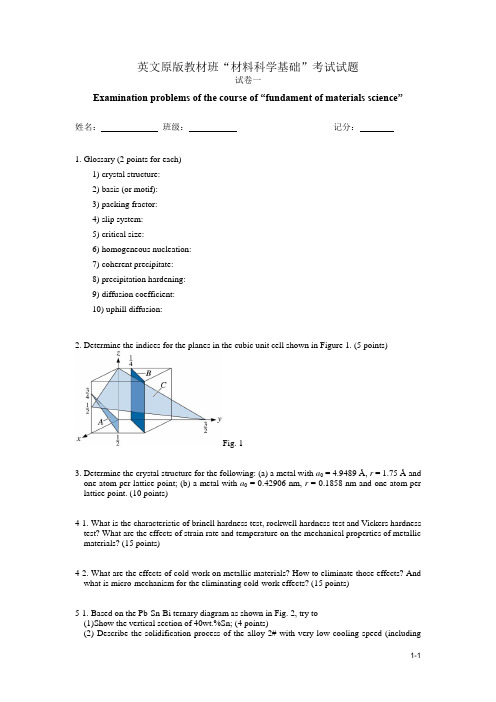
英文原版教材班“材料科学基础”考试试题试卷一Examination problems of the course of “fundament of materials science”姓名:班级:记分:1. Glossary (2 points for each)1) crystal structure:2) basis (or motif):3) packing fractor:4) slip system:5) critical size:6) homogeneous nucleation:7) coherent precipitate:8) precipitation hardening:9) diffusion coefficient:10) uphill diffusion:2. Determine the indices for the planes in the cubic unit cell shown in Figure 1. (5 points)Fig. 13. Determine the crystal structure for the following: (a) a metal with a0 =4.9489 Å, r = 1.75 Å and one atom per lattice point; (b) a metal with a0 = 0.42906 nm, r = 0.1858 nm and one atom per lattice point. (10 points)4-1. What is the characteristic of brinell hardness test, rockwell hardness test and Vickers hardness test? What are the effects of strain rate and temperature on the mechanical properties of metallic materials? (15 points)4-2. What are the effects of cold-work on metallic materials? How to eliminate those effects? And what is micro-mechanism for the eliminating cold-work effects? (15 points)5-1. Based on the Pb-Sn-Bi ternary diagram as shown in Fig. 2, try to(1)Show the vertical section of 40wt.%Sn; (4 points)(2) Describe the solidification process of the alloy 2# with very low cooling speed (includingphase and microstructure changes); (4 points)(3)Plot the isothermal section at 150o C. (7 points)Fig. 25-2. A 1mm sheet of FCC iron is used to contain N2in a heated exchanger at 1200o C. The concentration of N at one surface is 0.04 atomic percent and the concentration at the second surface is 0.005 atomic percent. At 1000 o C, if same N concentration is demanded at the second surface and the flux of N becomes to half of that at 1200o C, then what is the thickness of sheet?(15 points)6-1. Supposed that a certain liquid metal is undercooled until homogeneous nucleation occurs. (15 points)(1)How to calculate the critical radius of the nucleus required? Please give the deductionprocess.(2)For the Metal Ni, the Freezing Temperature is 1453︒C, the Latent Heat of Fusion is 2756J/cm3, and the Solid-liquid Interfacial Energy is 255⨯10-7 J/cm2. Please calculate the critical radius at 1353︒C. (Assume that the liquid Ni is not solidified.)6-2. Fig.3 is a portion of the Mg-Al phase diagram. (15 points)(1)If the solidification is too rapid, please describe the solidification process of Mg-10wt%Alalloy.(2)Please describe the equilibrium solidification process of Mg-20wt%Al alloy, and calculate theamount of each phase at 300︒C.Fig. 37-1. Figure 4 shows us the Al-Cu binary diagram and some microstructures found in a cooling process for an Al-4%Cu alloy. Please answer following questions according to this figure. (20 points)Fig. 4(1)What are precipitate, matrix and microconstituent? Please point them out in the in the figure and explain.(2)Why is need-like precipitate not good for dispersion strengthening? The typical microstructure shown in the figure is good or not? why?(3)Please tell us how to obtain the ideal microstructure shown in this figure.(4)Can dispersion strengthened materials be used at high temperature? Please give the reasons (comparing with cold working strengthening)7-2. Please answer following questions according to the time-temperature-transformation (TTT) diagram as shown in Fig. 5. (20 points)(1)What steel is this TTT diagram for? And what means P, B, and M in the figure? (2)Why dose the TTT diagram exhibi ts a ‘C’ shape?(3)Point out what microconstituent will be obtained after austenite is cooled according to the curves I, II, III and IV .(4)What is microstructural difference between the curve I and the curve II? (5)How to obtain the steel with the structure of(a) P+B(b) P+M+A (residual) (c) P+B+M+A (residual)(d) Full tempered martensiteIf you can, please draw the relative cooling curve or the flow chart of heat treatment.Fig. 5III III IV英文原版教材班“材料科学基础”考试试题答案Solution s of the course of “fundament of materials science”1. Glossary (2 points for each)1) The arrangement of the atoms in a material into a repeatable lattice.2) A group of atoms associated with a lattice.3) The fraction of space in a unit cell occupied by atoms.4) The combination of the slip plane and the slip direction.5) The minimum size that must be formed by atoms clustering together in the liquid before thesolid particle is stable and begins to grow.6) Formation of a critically sized solid from the liquid by the clustering together of a largenumber of atoms at a high undercooling (without an external interface).7) A precipitate whose crystal structure and atomic arrangement have a continuousrelationship with matrix from which precipitate is formed.8) A strengthening mechanism that relies on a sequence of solid state phase transformationsin a dispersion of ultrafine precipitates of a 2nd phase. This is same as age hardening. It is a form of dispersion strengthening.9) A temperature-dependent coefficient related to the rate at which atom, ion, or otherspecies diffusion. The DC depends on temperature, the composition and microstructure of the host material and also concentration of the diffusion species.10) A diffusion process in which species move from regions of lower concentration to that ofhigher concentration.2. Solution: A(-364), B(-340), C(346).3. Solution: (a)fcc; (b) bcc.4-1. What is the characteristic of brinell hardness test, rockwell hardness test and Vickers hardness test? What are the effects of strain rate and temperature on the mechanical properties of metallic materials? (15 points)4-2. What are the effects of cold-work on metallic materials? How to eliminate those effects? And what is micro-mechanism for the eliminating cold-work effects? (15 points)5-1. Based on the Pb-Sn-Bi ternary diagram as shown in Fig. 2, try to(1)Show the vertical section of 40wt.%Sn; (5 points)(2) Describe the solidification process of the alloy 2# with very low cooling speed (includingphase and microstructure changes); (5 points)(3)Plot the isothermal section at 150o C. (5 points)Fig. 25-2. A 1mm sheet of FCC iron is used to contain N2in a heated exchanger at 1200o C. The concentration of N at one surface is 0.04 atomic percent and the concentration at the second surface is 0.005 atomic percent. At 1000 o C, if same N concentration is demanded at the second surface and the flux of N becomes to half of that at 1200o C, then what is the thickness of sheet?(15 points)6-1. Supposed that a certain liquid metal is undercooled until homogeneous nucleation occurs. (15 points)(3)How to calculate the critical radius of the nucleus required? Please give the deductionprocess.(4)For the Metal Ni, the Freezing Temperature is 1453︒C, the Latent Heat of Fusion is 2756J/cm3, and the Solid-liquid Interfacial Energy is 255⨯10-7 J/cm2. Please calculate the critical radius at 1353︒C. (Assume that the liquid Ni is not solidified.)6-2. Fig.3 is a portion of the Mg-Al phase diagram. (15 points)(3)If the solidification is too rapid, please describe the solidification process of Mg-10wt%Alalloy.(4)Please describe the equilibrium solidification process of Mg-20wt%Al alloy, and calculate theamount of each phase at 300︒C.Fig. 37-1. Figure 4 shows us the Al-Cu binary diagram and some microstructures found in a cooling process for an Al-4%Cu alloy. Please answer following questions according to this figure. (20 points)Fig. 4(1)What are precipitate, matrix and microconstituent? Please point them out in the in the figure and explain.(2)Why is need-like precipitate not good for dispersion strengthening? The typical microstructure shown in the figure is good or not? why?(3)Please tell us how to obtain the ideal microstructure shown in this figure.(4)Can dispersion strengthened materials be used at high temperature? Please give the reasons (comparing with cold working strengthening)7-2. Please answer following questions according to the time-temperature-transformation (TTT) diagram as shown in Fig. 5. (20 points)(1)What steel is this TTT diagram for? And what means P, B, and M in the figure? (2)Why dose the TTT diagram exhibits a ‘C’ shape?(3)Point out what microconstituent will be obtained after austenite is cooled according to the curves I, II, III and IV .(4)What is microstructural difference between the curve I and the curve II? (5)How to obtain the steel with the structure of(a) P+B(b) P+M+A (residual) (c) P+B+M+A (residual)(d) Full tempered martensiteIf you can, please draw the relative cooling curve or the flow chart of heat treatment.Fig. 5III III IV英文原版教材班“材料科学基础”考试试题试卷二Examination problems of the course of “fundament of materials science”姓名:班级:记分:1. You would like to be able to physically separate different materials in a scrap recycling plant. Describe some possible methods that might be used to separate materials such as polymers, aluminum alloys, and steels from one another. (5 points)2. Plot the melting temperature of the elements in the 1A column of the periodic table versus atomic number (i.e., plot melting temperatures of Li through Cs). Discuss this relationship, based on atomic bonding and binding energy. (10 points)3.Above 882℃, titanium has a BCC crystal structure, with a = 0.332 nm. Below this temperature, titanium has a HCP structure, with a = 0.2978 nm and c = 0.4735 nm. Determine the percent volume change when BCC titanium transforms to HCP titanium. Is this a contraction or expansion? (10 points)4. The density of BCC iron is 7.882 g/cm3and the lattice parameter is 0.2866 nm whenhydrogen atoms are introduced at interstitial positions. Calculate (a) the atomic fraction of hydrogen atoms and (b) the number of unit cells required on average to contain one hydrogen atom. (15 points)5. A carburizing process is carried out on a 0.10% C steel by introducing 1.0% C at the surface at 980℃, where the iron is FCC. Calculate the carbon content at 0.01 cm, 0.05 cm, and 0.10 cm beneath the surface after 1 h. (15 points)6. The following data were collected from a standard 0.505-in.-diameter test specimen of acopper alloy (initial length (t o) = 2.0 in.):Load Gage Length Stress Strain(lb) (in.) (psi) (in/in.)0 2.00000 0 0.03,000 2.00167 15,000 0.0008356,000 2.00333 30,000 0.0016657,500 2.00417 37,500 0.0020859,000 2.0090 45,000 0.004510,500 2.040 52,500 0.0212,000 2.26 60,000 0.1312,400 2.50 (max load) 62,000 0.2511,400 3.02 (fracture) 57,000 0.51After fracture, the gage length is 3.014 in. and the diameter is 0.374 in. Plot the data and calculate (a) the 0.2% offset yield strength, (b) the tensile strength, (c) the modulus of elasticity, (d) the %Elongation, (e) the %Reduction in area, (f) the engineering stress at fracture, (g) the true stress at fracture, and (h) the modulus of resilience. (15 points)7. A 1.5-em-diameter metal bar with a 3-cm gage length is subjected to a tensile test. Thefollowing measurements are made.Change in Force (N) Gage length (cm) Diameter (cm)16,240 0.6642 1.202819,066 1.4754 1.088419,273 2.4663 0.9848Determine the strain hardening coefficient for the metal. Is the metal most likely to be FCC, BCC, or HCP? Explain.(15 points)8. Based on Hume-Rothery’s conditions, which of the following systems would be expected todisplay unlimited solid solubility? Explain. (15 points)(a) Au-Ag (b) Al-Cu (c) Al-Au (d)U-W(e) Mo-Ta (f) Nb-W (g) Mg-Zn (h) Mg-Cd英文原版教材班“材料科学基础”考试试题答案Solutions of the course of “fundament of materials science”1.Steels can be magnetically separated from the other materials; steel (or carbon-containing iron alloys) are ferromagnetic and will be attracted by magnets. Density differences could be used—polymers have a density near that of water; the specific gravity of aluminum alloys is around2.7;that of steels is between 7.5 and 8. Electrical conductivity measurements could be used—polymers are insulators, aluminum has a particularly high electrical conductivity.(5 points)2.T (o C)L i–180.7N a– 97.8K – 63.2R b– 38.9As the atomic number increases, the melting temperature decreases, (10 points)3. We can find the volume of each unit cell. Two atoms are present in both BCC and HCP titanium unit cells, so the volumes of the unit cells can be directly compared.V BCC = (0.332 nm)3 = 0.03659 nm3V HCP= (0.2978 nm)2(0.4735 nm)cos30 = 0.03637 nm3△V=x 100 =×100= -0.6%Therefore titanium contracts 0.6% during cooling. (10 points)4. (a) 7.882 g/cm3 =x = 0.0081 H atoms/cellThe total atoms per cell include 2 Fe atoms and 0.0081 H atoms. Thus:(10 points)(b) Since there is 0.0081 H/cell, then the number of cells containing H atoms is:cells = 1/0.0081 = 123.5 or 1 H in 123.5 cells (5 points)5. D = 0.23 exp[-32,900/(1.987)(1253)] = 42 × 10-8 cm2/sC x= 0.87% CC x= 0.43% CC x= 0.18% C(15 points)6. σ=FI (π/4)(0.505)2 = F/0.2ε = (l-2)/2(a) 0.2% offset yield strength = 45,000 psi(b)tensile strength = 62,000 psi(c) E = (30,000 - 0) / (0.001665 - 0) = 18 x 106 psi(d)%Elongation =(e) %Reduction in area =(f) engineering stress at fracture = 57,000 psi(g)true stress at fracture = 11,400 lb / (TC/4)(0.374)2= 103,770 psi (h) From the graph, yielding begins at about 37,500 psi. Thus:(15 points)7.Force(lb) Gage length(in.) Diameter(in.) True stress(psi) True strain(psi)16,240 3.6642 12.028 143 0.20019,066 4.4754 10.884 205 0.40019,273 5.4663 9.848 249 0.600σt=Kεt2or ln143=ln K + n ln0.2ln 249 = ln K + nln 0.6n=0.51A strain hardening coefficient of 0.51 is typical of FCC metals.(15 points)8.The Au–Ag, Mo–Ta, and Mg–Cd systems have the required radius ratio, the same crystal structures, and the same valences. Each of these might be expected to display complete solid solubility. [The Au –Ag and Mo –T a d o have isomorphous phase diagrams. In addition, the Mg–Cd alloys all solidify like isomorphous alloys; however a number of solid state phase transformations complicate the diagram.] (15 points)英文原版教材班“材料科学基础”考试试题试卷三Examination problems of the course of “fundament of materials science”姓名:班级:记分:1. You would like to be able to identify different materials without resorting to chemical analysis or lengthy testing procedures. Describe some possible testing and sorting techniques you might be able to use based on the physical properties of materials. (5 points)2. Plot the melting temperatures of elements in the 4A to 8-10 columns of the periodic table versus atomic number (i.e., plot melting temperatures of Ti through Ni, Zr through Pd, and Hf through Pt). Discuss these relationships, based on atomic bonding and binding energy, (a) as the atomic number increases in each row of the periodic table and (b) as the atomic number increases in each column of the periodic table. (10 points)3. Beryllium has a hexagonal crystal structure, with a o= 0.22858 nm and c o= 0.35842 nm. The atomic radius is 0.1143 nm, the density is 1.848 g/cm3, and the atomic weight is 9.01 g/mol. Determine (a) the number of atoms in each unit cell and (b) the packing factor in the unit cell.(10 points)4. Suppose we introduce one carbon atom for every 100 iron atoms in an interstitial position in BCC iron, giving a lattice parameter of 0.2867 nm. For the Fe-C alloy, find (a) the density and (b) the packing factor. (15 points)5. Iron containing 0.05% C is heated to 912oC in an atmosphere that produces 1.20% C at the surface and is held for 24 h. Calculate the carbon content at 0.05 cm beneath the surface if (a) the iron is BCC and (b) the iron is FCC. Explain the difference. (15 points)6. The following data were collected from a 0.4-in. diameter test specimen of poly vinyl chloride(l0 = 2.0 in):Load(lb) Gage Length(in.) Stress(psi) Strain(in/in.)0 2.00000 0 0.0300 2.00746 2,387 0.00373600 2.01496 4,773 0.00748900 2.02374 7,160 0.011871200 2.032 9,547 0.0161500 2.046 11,933 0.0231660 2.070 (max load) 13,206 0.0351600 2.094 12,729 0.0471420 2.12 (fracture) 11,297 0.06After fracture, the gage length is 2.09 in. and the diameter is 0.393 in. Plot the data and calculate (a) the 0.2% offset yield strength, (b) the tensile strength, (c) the modulus of elasticity, (d) the %Elongation, (e) the %Reduction in area, (f) the engineering stress at fracture, (g) the true stress at fracture, and (h) the modulus of resilience. (15 points)7. A titanium alloy contains a very fine dispersion of tiny Er203 particles. What will be the effectof these particles on the grain growth temperature and the size of the grains at any particular annealing temperature? Explain. (15 points)8. Suppose 1 at% of the following elements is added to copper (forming a separate alloy witheach element) without exceeding the solubility limit. Which one would be expected to give the higher strength alloy? Is any of the alloying elements expected to have unlimited solid solubility in copper?(a) Au (b) Mn (c) Sr (d) Si (e) Co (15 points)英文原版教材班“材料科学基础”考试试题答案Solutions of the course of “fundament of materials science”1.Steels can be magnetically separated from the other materials; steel (or carbon-containing iron alloys) are ferromagnetic and will be attracted by magnets. Density differences could be used—polymers have a density near that of water; the specific gravity of aluminum alloys is around2.7;that of steels is between 7.5 and 8. Electrical conductivity measurements could be used—polymers are insulators, aluminum has a particularly high electrical conductivity.(5 points)2. Ti –1668 Zr – 1852 Hf – 2227V –1900 Nb –2468 Ta – 2996Cr –1875 Mo–2610 W–3410Mn–1244 Tc –2200 Re–3180Fe –1538 Ru –2310 Os–2700Co –1495 Rh –1963 Ir –2447Ni –1453 Pd –1552 Pt –1769For each row, the melting temperature is highest when the outer “d” energy level is partly full. In Cr, there are 5 electrons in the 3d shell; in Mo, there are 5 electrons in the 4d shell; in W there are 4 electrons in the 5d shell. In each column, the melting temperature increases as the atomic number increases—the atom cores contain a larger number of tightly held electrons, making the metals more stable. (10 points)3.V= (0.22858 nm)2(0.35842 nm)cos 30 = 0.01622 nm3 = 16.22 × 10-24 cm3(a)From the density equation:1.848 g/cm3 =x = 2 atoms/cell(b)The packing factor (PF) is:PF == 0.77 (10 points)4. There is one carbon atom per 100 iron atoms, or 1 C/50 unit cells, or 1/50 C per unit cell:(a)(b)(15 points)5. t= (24 h)(3600 s/h) = 86,400 sD BCC = 0.011 exp[-20,900/(1.9871185)] = 1.54 × 10-6 cm2/sD FCC = 0.23 exp[-32,900/(1.987)(1185)] = 1.97×10-7 cm2/sBCC: = erf[0.0685] = 0.077C x= 1.11% CFCC: = erf[0.192] = 0.2139C x = 0.95% CFaster diffusion occurs in the looser packed BCC structure, leading to the higher carbon content at point “x”. (15 points)6. σ=F /(π/4)(0.4)2 = F/0.1257ε = (l-2)/2(a)0.2% offset yield strength = 11,600 psi(b) tensile strength = 12,729 psi(c) E= (7160 - 0) / (0.01187 - 0) = 603,000 psi(d)%Elongation =(e) %Reduction in area =(f) engineering stress at fracture = 11,297 psi(g)true stress at fracture = 1420 lb / (TC/4)(0.393)2= 11,706 psi (h) From the figure, yielding begins near 9550 psi. Thus:(15 points)7. These particles, by helping pin die grain boundaries, will increase the grain growth temperature and decrease the grain size. (15 points)8.The Cu-Sr alloy would be expected to be strongest (largest size difference). The Cu-Au alloy satisfies Hume-Rothery ’s conditions and might be expected to display complete solid solubility—in fact it freezes like an isomorphous series of alloys, but a number of solid state transformations occur at lower temperatures.(15 points)英文原版教材班“材料科学基础”考试试题试卷四Examination problems of the course of “fundament of materials science”姓名:班级:记分:1.Aluminum has a density of2.7 g/cm3. Suppose you would like to produce a compositematerial based on aluminum having a density of 1.5 g/cm3. Design a material that would have this density. Would introducing beads of polyethylene, with a density of 0.95 g/cm3, into the aluminum be a likely possibility? Explain. (5 points)2. (a) Aluminum foil used for storing food weighs about 0.3 g per square inch. How many atomsof aluminum are contained in this sample of foil?(b) Using the densities and atomic weights given in Appendix A, calculate and compare thenumber of atoms per cubic centimeter in (i) lead and (ii) lithium. (10 points)3. The density of potassium, which has the BCC structure and one atom per lattice point, is0.855 g/cm3. The atomic weight of potassium is 39.09 g/mol. Calculate (a) the latticeparameter; and (b) the atomic radius of potassium. (10 points)4. The density of a sample of HCP beryllium is 1.844 g/cm3 and the lattice parameters are a0=0.22858 nm and c0= 0.35842 nm. Calculate (a) the fraction of the lattice points that containvacancies and (b) the total number of vacancies in a cubic centimeter. (15 points)5. A ceramic part made of MgO is sintered successfully at 1700℃in 90 minutes. To minimizethermal stresses during the process, we plan to reduce the temperature to 1500℃. Which will limit the rate at which sintering can be done: diffusion of magnesium ions or diffusion of oxygen ions? What time will be required at the lower temperature? (15 points)6. (a) A thermosetting polymer containing glass beads is required to deflect 0.5 mm when aforce of 500 N is applied. The polymer part is 2 cm wide, 0.5 cm thick, and 10 cm long. If the flexural modulus is 6.9 GPa, determine the minimum distance between the supports. Will the polymer fracture if its flexural strength is 85 MPa? Assume that no plastic deformation occurs.(b) The flexural modulus of alumina is 45 x 106 psi and its flexural strength is 46,000 psi. Abar of alumina 0.3 in. thick, 1.0 in. wide, and 10 in. long is placed on supports 7 in. apart.Determine the amount of deflection at the moment the bar breaks, assuming that no plastic deformation occurs. (15 points)7. Based on the following observations, construct a phase diagram. Element A melts at 850°Cand element B melts at 1200°C. Element B has a maximum solubility of 5% in element A, and element A has a maximum solubility of 15% in element B. The number of degrees of freedom from the phase rule is zero when the temperature is 725°C and there is 35% B present. At room temperature 1% B is soluble in A and 7% A is soluble in B. (15 points)8.Suppose that age hardening is possible in the Al-Mg system (see Figure 10-11). (a)Recommend an artificial age-hardening heat treatment for each of the following alloys, and(b) compare the amount of the precipitate that forms from your treatment of each alloy. (i)Al-4% Mg (ii) Al-6% Mg (iii) Al-12% Mg (c) Testing of the alloys after the heat treatment reveals that little strengthening occurs as a result of the heat treatment. Which of the requirements for age hardening is likely not satisfied? (15 points)英文原版教材班“材料科学基础”考试试题答案Solutions of the course of “fundament of materials science”1. In order to produce an aluminum-matrix composite material with a density of 1.5 g/cm 3, we wouldneed to select a material having a density considerably less than 1.5 g/cm 3. While polyethylene’s density would make it a possibility, the polyethylene has a very low melting point compared to aluminum; this would make it very difficult to introduce the polyethylene into a solid aluminum matrix —processes such as casting or powder metallurgy would destroy the polyethylene .Therefore polyethylene would NOT be a likely possibility.One approach, however, might be to introduce hollow glass beads .Although ceramic glasses have densities comparable to that of aluminum, a hollow bead will have a very low density. The glass also has a high melting temperature and could be introduced into liquid aluminum for processing as a casting. (5 points)2. (a) In a one square inch sample:number ==6.69 × 1021 atoms(b) (i) In lead:= 3.3 × 1022 atoms/cm 3(ii) In lithium:= 4.63 × 1022 atoms/cm 3 (10 points)3. (a) Using Equation 3-5:0.855 g/cm 3 =a o 3 = 1.5189 × 10-22 cm 3 or a o = 5.3355 × 10-8 cm(b) From the relationship between atomic radius and lattice parameter:r == 2.3103 × 10-8cm (10 points)4. V u.c.= (0.22858 nm)2(0.35842 nm)cos30 = 0.01622 nm 3= 1.622 x 10~23 cm 3 (a) From the density equation:x = 1.9984fraction =29984.12 = 0.0008(b) number == 0.986 x 1020 vacancies/cm 3 (15 points)5. Diffusion of oxygen is the slower of the two, due to the larger ionic radius of the oxygen.D 1700= 0.000043 exp[-82,100/(1.987)(1973)] = 3.455 × 10-14 cm 2/sD1500= 0.000043 exp[-82,100/(1.987)(1773)] = 3.255 × 10-15 cm2/st1500 = D1700 t1700/D1500== 955 min = 15.9 h (15 points)6. (a) Solution:The minimum distance L between the supports can be calculated from the flexural modulus.L3 = 4w/z3δ(flexural modulus)/3FL3 = (4)(20 mm)(5 mm)3(0.5 mm)(6.9 GPA)(1000 MPa/GPa) / 500 NL3 = 69,000 mm3 or L = 41 mmThe stress acting on the bar when a deflection of 0.5 mm is obtained isσ= WL/2wh2 = (3)(500 N)(41 mm) / (2)(20 mm)(5 mm)2 = 61.5 MPaThe applied stress is less than the flexural strength of 85 MPa; the polymer is not expected to fracture.(b) Solution:The force required to break the bar isF = 2w/z2(flexural strength)/3LF= (2)(1 in)(0.3 in)2(46,000 psi / (3)(7 in.) = 394 lbThe deflection just prior to fracture is8 = FZ3/4wh3(flexural modulus)8 = (394 lb)(7 in)3/(4)(l in)(0.3 in)3(45 x 106 psi) = 0.0278 in. (15 points)7.(15 points)8. (a) The heat treatments for each alloy might be:Al-4% Mg Al-6% Mg Al-12% MgT Eutectic451°C 451°C 451°CT Solvs210°C 280°C 390°CSolutionTreat at: 210-451°C 280-451°C 390-451°CQuench Quench QuenchAge at: <210°C <280°C <390°C(b) Answers will vary depending on aging temperature selected. If all threeare aged at 200°C, as an example, the tie line goes from about 3.8 to 35% Mg:A1-4% Mg: %β = (4− 3.82)/(35 − 3.82) X 100 = 0.6%Al-6% Mg: %β = (6 − 3.82)/(35 − 3.82) X 100 = 7.1%Al-12% Mg: %β = (12 −3.82)/(35− 3.82) X 100 = 26.8%(c) Most likely, a coherent precipitate is not formed; simple dispersionstrengthening, rather than age hardening, occurs. (15 points)英文原版教材班“材料科学基础”考试试题试卷五Examination problems of the course of “fundament of materials science”姓名:班级:记分:1. You would like to design an aircraft that can be flown by human power nonstop for adistance of 30 km. What types of material properties would you recommend? What materials might be appropriate? (5 points)2. Boron has a much lower coefficient of thermal expansion than aluminum, even though bothare in the 3B column of the periodic table. Explain, based on binding energy, atomic size, and the energy well, why this difference is expected. (10 points)3. Determine the ASTM grain size number if 20 grains/square inch are observed at amagnification of 400. (10 points)4. We currently can successfully perform a carburizing heat treatment at 1200o C in 1 h. In aneffort to reduce the cost of the brick lining in our furnace, we propose to reduce the carburizing temperature to 950℃. What time will be required to give us a similar carburizing treatment? (15 points)5.The data below were obtained from a series of Charpy impact tests performed on foursteels, each having a different manganese content. Plot the data and determine (a) the transition temperature (defined by the mean of the absorbed energies in theductile and brittle regions) and (b) the transition temperature (defined as the temperature that provides 50 J absorbed energy). Plot the transition temperature versus manganese content and discuss the effect of manganese on the toughness of steel. What would be the minimum manganese allowed in the steel if a part is to be used at 0°C?Test temperature°C Impact snergy (J)0.30% Mn 0.39% Mn 1.01% Mn 1.55% Mn-100 2 5 5 15-75 2 5 7 25-50 2 12 20 45-25 10 25 40 700 30 55 75 11025 60 100 110 13550 105 125 130 14075 130 135 135 140100 130 135 135 140(15 points)。
物理学名词
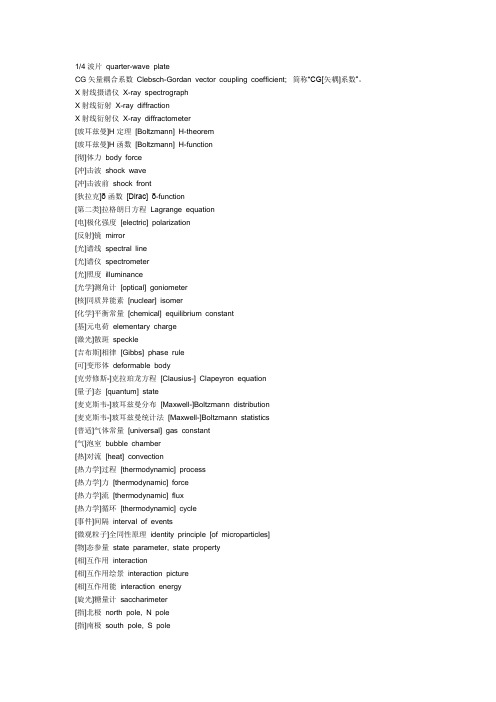
1/4波片quarter-wave plateCG矢量耦合系数Clebsch-Gordan vector coupling coefficient; 简称“CG[矢耦]系数”。
X射线摄谱仪X-ray spectrographX射线衍射X-ray diffractionX射线衍射仪X-ray diffractometer[玻耳兹曼]H定理[Boltzmann] H-theorem[玻耳兹曼]H函数[Boltzmann] H-function[彻]体力body force[冲]击波shock wave[冲]击波前shock front[狄拉克]δ函数[Dirac] δ-function[第二类]拉格朗日方程Lagrange equation[电]极化强度[electric] polarization[反射]镜mirror[光]谱线spectral line[光]谱仪spectrometer[光]照度illuminance[光学]测角计[optical] goniometer[核]同质异能素[nuclear] isomer[化学]平衡常量[chemical] equilibrium constant[基]元电荷elementary charge[激光]散斑speckle[吉布斯]相律[Gibbs] phase rule[可]变形体deformable body[克劳修斯-]克拉珀龙方程[Clausius-] Clapeyron equation[量子]态[quantum] state[麦克斯韦-]玻耳兹曼分布[Maxwell-]Boltzmann distribution[麦克斯韦-]玻耳兹曼统计法[Maxwell-]Boltzmann statistics[普适]气体常量[universal] gas constant[气]泡室bubble chamber[热]对流[heat] convection[热力学]过程[thermodynamic] process[热力学]力[thermodynamic] force[热力学]流[thermodynamic] flux[热力学]循环[thermodynamic] cycle[事件]间隔interval of events[微观粒子]全同性原理identity principle [of microparticles][物]态参量state parameter, state property[相]互作用interaction[相]互作用绘景interaction picture[相]互作用能interaction energy[旋光]糖量计saccharimeter[指]北极north pole, N pole[指]南极south pole, S pole[主]光轴[principal] optical axis[转动]瞬心instantaneous centre [of rotation][转动]瞬轴instantaneous axis [of rotation]t 分布student's t distributiont 检验student's t testK俘获K-captureS矩阵S-matrixWKB近似WKB approximationX射线X-rayΓ空间Γ-spaceα粒子α-particleα射线α-rayα衰变α-decayβ射线β-rayβ衰变β-decayγ矩阵γ-matrixγ射线γ-rayγ衰变γ-decayλ相变λ-transitionμ空间μ-spaceχ 分布chi square distributionχ 检验chi square test阿贝不变量Abbe invariant阿贝成象原理Abbe principle of image formation阿贝折射计Abbe refractometer阿贝正弦条件Abbe sine condition阿伏伽德罗常量Avogadro constant阿伏伽德罗定律Avogadro law阿基米德原理Archimedes principle阿特伍德机Atwood machine艾里斑Airy disk爱因斯坦-斯莫卢霍夫斯基理论Einstein-Smoluchowski theory 爱因斯坦场方程Einstein field equation爱因斯坦等效原理Einstein equivalence principle爱因斯坦关系Einstein relation爱因斯坦求和约定Einstein summation convention爱因斯坦同步Einstein synchronization爱因斯坦系数Einstein coefficient安[培]匝数ampere-turns安培[分子电流]假说Ampere hypothesis安培定律Ampere law安培环路定理Ampere circuital theorem安培计ammeter安培力Ampere force安培天平Ampere balance昂萨格倒易关系Onsager reciprocal relation凹面光栅concave grating凹面镜concave mirror凹透镜concave lens奥温电桥Owen bridge巴比涅补偿器Babinet compensator巴耳末系Balmer series白光white light摆pendulum板极plate伴线satellite line半波片halfwave plate半波损失half-wave loss半波天线half-wave antenna半导体semiconductor半导体激光器semiconductor laser半衰期half life period半透[明]膜semi-transparent film半影penumbra半周期带half-period zone傍轴近似paraxial approximation傍轴区paraxial region傍轴条件paraxial condition薄膜干涉film interference薄膜光学film optics薄透镜thin lens保守力conservative force保守系conservative system饱和saturation饱和磁化强度saturation magnetization本底background本体瞬心迹polhode本影umbra本征函数eigenfunction本征频率eigenfrequency本征矢[量] eigenvector本征振荡eigen oscillation本征振动eigenvibration本征值eigenvalue本征值方程eigenvalue equation比长仪comparator比荷specific charge; 又称“荷质比(charge-mass ratio)”。
Mach_数和壁面温度对HyTRV_边界层转捩的影响
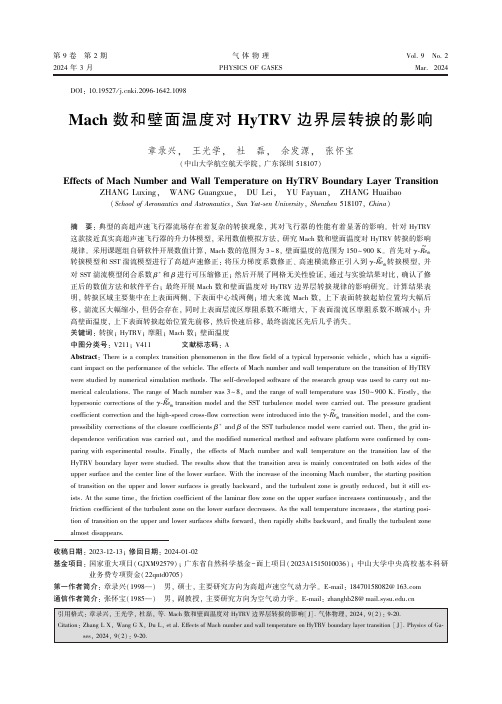
第9卷㊀第2期2024年3月气体物理PHYSICSOFGASESVol.9㊀No.2Mar.2024㊀㊀DOI:10.19527/j.cnki.2096 ̄1642.1098Mach数和壁面温度对HyTRV边界层转捩的影响章录兴ꎬ㊀王光学ꎬ㊀杜㊀磊ꎬ㊀余发源ꎬ㊀张怀宝(中山大学航空航天学院ꎬ广东深圳518107)EffectsofMachNumberandWallTemperatureonHyTRVBoundaryLayerTransitionZHANGLuxingꎬ㊀WANGGuangxueꎬ㊀DULeiꎬ㊀YUFayuanꎬ㊀ZHANGHuaibao(SchoolofAeronauticsandAstronauticsꎬSunYat ̄senUniversityꎬShenzhen518107ꎬChina)摘㊀要:典型的高超声速飞行器流场存在着复杂的转捩现象ꎬ其对飞行器的性能有着显著的影响ꎮ针对HyTRV这款接近真实高超声速飞行器的升力体模型ꎬ采用数值模拟方法ꎬ研究Mach数和壁面温度对HyTRV转捩的影响规律ꎮ采用课题组自研软件开展数值计算ꎬMach数的范围为3~8ꎬ壁面温度的范围为150~900Kꎮ首先对γ ̄Re~θt转捩模型和SST湍流模型进行了高超声速修正:将压力梯度系数修正㊁高速横流修正引入到γ ̄Re~θt转捩模型ꎬ并对SST湍流模型闭合系数β∗和β进行可压缩修正ꎻ然后开展了网格无关性验证ꎬ通过与实验结果对比ꎬ确认了修正后的数值方法和软件平台ꎻ最终开展Mach数和壁面温度对HyTRV边界层转捩规律的影响研究ꎮ计算结果表明ꎬ转捩区域主要集中在上表面两侧㊁下表面中心线两侧ꎻ增大来流Mach数ꎬ上下表面转捩起始位置均大幅后移ꎬ湍流区大幅缩小ꎬ但仍会存在ꎬ同时上表面层流区摩阻系数不断增大ꎬ下表面湍流区摩阻系数不断减小ꎻ升高壁面温度ꎬ上下表面转捩起始位置先前移ꎬ然后快速后移ꎬ最终湍流区先后几乎消失ꎮ关键词:转捩ꎻHyTRVꎻ摩阻ꎻMach数ꎻ壁面温度㊀㊀㊀收稿日期:2023 ̄12 ̄13ꎻ修回日期:2024 ̄01 ̄02基金项目:国家重大项目(GJXM92579)ꎻ广东省自然科学基金-面上项目(2023A1515010036)ꎻ中山大学中央高校基本科研业务费专项资金(22qntd0705)第一作者简介:章录兴(1998 )㊀男ꎬ硕士ꎬ主要研究方向为高超声速空气动力学ꎮE ̄mail:184****8082@163.com通信作者简介:张怀宝(1985 )㊀男ꎬ副教授ꎬ主要研究方向为空气动力学ꎮE ̄mail:zhanghb28@mail.sysu.edu.cn中图分类号:V211ꎻV411㊀㊀文献标志码:AAbstract:Thereisacomplextransitionphenomenonintheflowfieldofatypicalhypersonicvehicleꎬwhichhasasignifi ̄cantimpactontheperformanceofthevehicle.TheeffectsofMachnumberandwalltemperatureonthetransitionofHyTRVwerestudiedbynumericalsimulationmethods.Theself ̄developedsoftwareoftheresearchgroupwasusedtocarryoutnu ̄mericalcalculations.TherangeofMachnumberwas3~8ꎬandtherangeofwalltemperaturewas150~900K.Firstlyꎬthehypersoniccorrectionsoftheγ ̄Re~θttransitionmodelandtheSSTturbulencemodelwerecarriedout.Thepressuregradientcoefficientcorrectionandthehigh ̄speedcross ̄flowcorrectionwereintroducedintotheγ ̄Re~θttransitionmodelꎬandthecom ̄pressibilitycorrectionsoftheclosurecoefficientsβ∗andβoftheSSTturbulencemodelwerecarriedout.Thenꎬthegridin ̄dependenceverificationwascarriedoutꎬandthemodifiednumericalmethodandsoftwareplatformwereconfirmedbycom ̄paringwithexperimentalresults.FinallyꎬtheeffectsofMachnumberandwalltemperatureonthetransitionlawoftheHyTRVboundarylayerwerestudied.Theresultsshowthatthetransitionareaismainlyconcentratedonbothsidesoftheuppersurfaceandthecenterlineofthelowersurface.WiththeincreaseoftheincomingMachnumberꎬthestartingpositionoftransitionontheupperandlowersurfacesisgreatlybackwardꎬandtheturbulentzoneisgreatlyreducedꎬbutitstillex ̄ists.Atthesametimeꎬthefrictioncoefficientofthelaminarflowzoneontheuppersurfaceincreasescontinuouslyꎬandthefrictioncoefficientoftheturbulentzoneonthelowersurfacedecreases.Asthewalltemperatureincreasesꎬthestartingposi ̄tionoftransitionontheupperandlowersurfacesshiftsforwardꎬthenrapidlyshiftsbackwardꎬandfinallytheturbulentzonealmostdisappears.气体物理2024年㊀第9卷Keywords:transitionꎻHyTRVꎻfrictionꎻMachnumberꎻwalltemperature引㊀言高超声速飞行器具有突防能力强㊁打击范围广㊁响应迅速等显著优势ꎬ正逐渐成为各国空天竞争的热点[1]ꎮ高超声速飞行器边界层转捩是该类飞行器气动设计中的重要问题[2]ꎮ在边界层转捩过程中ꎬ流态由层流转变为湍流ꎬ飞行器的表面摩阻急剧增大到层流时的3~5倍ꎬ严重影响飞行器的气动性能与热防护系统ꎬ转捩还会导致飞行器壁面烧蚀㊁颤振加剧㊁飞行姿态控制难度大等一系列问题ꎬ对飞行器的飞行安全构成严重的威胁[3 ̄5]ꎬ开展高超声速飞行器边界层转捩研究具有十分重要的意义ꎮ影响边界层转捩的因素很多ꎬ例如ꎬMach数㊁Reynolds数㊁湍流强度㊁表面传导热等ꎮ在高超声速流动条件下ꎬ强激波㊁强逆压梯度㊁熵层等高超声速现象及其相互作用ꎬ会使得转捩流动的预测和研究难度进一步增大[6]ꎮ目前高超声速飞行器转捩数值模拟方法主要有直接数值模拟(DNS)㊁大涡模拟(LES)和基于Reynolds平均Navier ̄Stokes(RANS)的转捩模型方法ꎬ由于前两种计算量巨大ꎬ难以推广到工程应用ꎬ基于Reynolds平均Navier ̄Stokes的转捩模型在工程实践中应用最为广泛ꎬ其中γ ̄Re~θt转捩模型基于局部变量ꎬ与现代CFD方法良好兼容ꎬ目前已经有多项研究尝试从一般性的流动问题拓展到高超声速流动转捩模拟[6 ̄9]ꎮ目前高超声速流动转捩的研究对象主要是结构相对简单的构型ꎮMcDaniel等[10]研究了扩口直锥在高超声速流动条件下的转捩现象ꎮPapp等[11]研究了圆锥在高超声速流动条件下的转捩特性ꎮ美国和澳大利亚组织联合实施的HIFiRE计划[12]ꎬ研究了圆锥形状的HIFiRE1和椭圆锥形的HIFiRE5的转捩问题ꎮ杨云军等[13]采用数值模拟方法ꎬ分析了椭圆锥的转捩影响机制ꎬ并研究了Reynolds数对转捩特性的影响规律ꎮ另外ꎬ袁先旭等[14]于2015年成功实施了圆锥体MF ̄1航天模型飞行试验ꎮ以上对高超声速流动的转捩研究ꎬ都取得了比较理想的结果ꎬ然而所采用的模型都是圆锥㊁椭圆锥等简单几何外形ꎬ这与真实高超声速飞行器有较大差异ꎬ较难反映真实的转捩特性ꎮ为了有效促进对真实高超声速飞行器的转捩问题研究ꎬ中国空气动力研究与发展中心提出并设计了一款接近真实飞行器的升力体模型ꎬ即高超声速转捩研究飞行器(hypersonictransitionresearchvehicleꎬHyTRV)[15]ꎬ模型详细的参数见参考文献[16]ꎮHyTRV外形如图1所示ꎬ其整体外形较为复杂ꎬ不同区域发生转捩的情况也不尽相同ꎮ对HyTRV的转捩问题研究能够显著提高对真实高超声速飞行器转捩特性的认识水平ꎮLiu等[17]采用理论分析㊁数值模拟和风洞实验3种方法对HyTRV的转捩特性进行了研究ꎻ陈坚强等[15]分析了HyTRV的边界层失稳特征ꎻChen等[18]对HyTRV进行了多维线性稳定性分析ꎻQi等[19]在来流Mach数6㊁攻角0ʎ的条件下对HyTRV进行了直接数值模拟ꎻ万兵兵等[20]结合风洞实验与飞行试验ꎬ利用eN方法预测了HyTRV升力体横流区的转捩阵面形状ꎮ目前ꎬ相关研究主要集中在HyTRV的稳定性特征及转捩预测两个方面ꎬ而对若干关键参数ꎬ特别是Mach数和壁面温度对转捩的影响研究还比较少ꎮ(a)Frontview(b)Sideview㊀㊀㊀图1㊀HyTRV外形Fig.1㊀ShapeofHyTRV基于此ꎬ本文采用数值模拟方法ꎬ应用课题组自研软件开展Mach数和壁面温度对HyTRV转捩流动的影响规律研究ꎮ1㊀数值方法1.1㊀控制方程和数值方法控制方程为三维可压缩RANS方程ꎬ采用结构网格技术和有限体积方法ꎬ变量插值方法采用2阶MUSCL格式ꎬ通量计算采用低耗散的通量向量差分Roe格式ꎬ黏性项离散采用中心格式ꎬ时间推进方法采用LU ̄SGS格式ꎮ壁面采用等温㊁无滑移壁面条件ꎬ入口采用Riemann远场边界条件ꎬ出口采用零梯度外推边界条件ꎮ1.2㊀γ ̄Re~θt转捩模型γ ̄Re~θt转捩模型是Menter等[21ꎬ22]于2004年提01第2期章录兴ꎬ等:Mach数和壁面温度对HyTRV边界层转捩的影响出的一种基于拟合公式的间歇因子转捩模型ꎬ在2009年公布了完整的拟合公式及相关参数[23]ꎮ许多学者也开发了相应的程序ꎬ并进行了大量的算例验证[24 ̄28]ꎬ证明了该模型具有较好的转捩预测能力ꎬ预测精度较高ꎻ通过合适的标定ꎬγ ̄Re~θt转捩模型可以适用于多种情况下的转捩模拟ꎮ该模型构建了关于间歇因子γ的输运方程和关于转捩动量厚度Reynolds数Re~θt的输运方程ꎮ具体来说ꎬγ表示该位置是湍流流动的概率ꎬ取值范围为0<γ<1ꎮ关于γ的控制方程为Ə(ργ)Ət+Ə(ρujγ)Əxj=Pγ-Eγ+ƏƏxjμ+μtσfæèçöø÷ƏγƏxjéëêêùûúú其中ꎬPγ为生成项ꎬEγ为破坏项ꎮ关于Re~θt的输运方程为Ə(ρRe~θt)Ət+Ə(ρujRe~θt)Əxj=Pθt+ƏƏxjσθt(μ+μt)ƏRe~θtƏxjéëêêùûúú其中ꎬPθt为源项ꎬ其作用是使边界层外部的Re~θt等于Reθtꎬ定义式为Pθt=cθtρt(Reθt-Re~θt)(1.0-Fθt)Reθt采用以下经验公式Reθt=1173.51-589 428Tu+0.2196Tu2æèçöø÷F(λθ)ꎬTuɤ0.3Reθt=331.50(Tu-0.5658)-0.671F(λθ)ꎬTu>0.3ìîíïïïïF(λθ)=1+(12.986λθ+123.66λ2θ+405.689λ3θ)e-(Tu1.5)1.5ꎬ㊀λθɤ0F(λθ)=1+0.275(1-e-35.0λθ)e-(Tu0.5)ꎬλθ>0ìîíïïïï在实际计算中ꎬ通过γ ̄Re~θt转捩模型获得间歇因子ꎬ再通过间歇因子来控制SSTk ̄ω湍流模型中湍动能的生成ꎮγ ̄Re~θt转捩模型与SSTk ̄ω湍流模型耦合为Ə(ρk)Ət+Ə(ρujk)Əxj=γeffτijƏuiƏxj-min(max(γeffꎬ0.1)ꎬ1.0)ρβ∗kω+ƏƏxjμ+μtσkæèçöø÷ƏkƏxjéëêêùûúúƏ(ρω)Ət+Ə(ρujω)Əxj=γvtτijƏuiƏxj-βρω2+ƏƏxj(μ+σωμt)ƏωƏxjéëêêùûúú+2ρ(1-F1)σω21ωƏkƏxjƏωƏxj模型中具体参数定义见文献[23]ꎮ1.3㊀高超声速修正原始SST湍流模型及γ ̄Re~θt转捩模型都是基于不可压缩流动发展的ꎬ为了更好地预测高超声速流动转捩ꎬ本节引入了3种重要的高超声速修正方法ꎮ1.3.1㊀压力梯度修正压力梯度对边界层转捩的影响较大ꎬ在高Mach数情况下ꎬ边界层厚度较大ꎬ进而影响压力梯度的大小ꎬ因此在模拟高超声速流动时应该考虑Mach数对压力梯度的影响ꎮ本文采用张毅峰等[29]提出的压力梯度修正方法ꎬ具体修正形式如下λᶄθ=λθ1+γᶄ-12Maeæèçöø÷其中ꎬMae为边界层外缘Mach数ꎬγᶄ为比热比ꎮ1.3.2㊀高速横流修正在原始γ ̄Re~θt转捩模型中ꎬ没有考虑横流不稳定性对转捩的影响ꎬ对于横流模态主导的转捩ꎬ原始转捩模型计算的结果并不理想ꎮLangtry等[30]在2015年对γ ̄Re~θt转捩模型进行了低速横流修正ꎬ向星皓等[9]在Langtry低速横流修正的基础上ꎬ对高超声速椭圆锥转捩DNS数据进行了拓展ꎬ提出了高速横流转捩判据ꎬ本文直接采用向星皓提出的高速横流转捩方法ꎮLangtry将横流强度引入转捩发生动量厚度Reynolds数输运方程中Ə(ρRe~θt)Ət+Ə(ρujRe~θt)Əxj=Pθt+DSCF+ƏƏxjσθt(μ+μt)ƏRe~θtƏxjéëêêùûúú式中ꎬDSCF为横流源项ꎬLangtry低速横流修正为DSCF=cθtρtccrossflowmin(ReSCF-Re~θtꎬ0.0)Fθt2其中ꎬReSCF为低速横流判据ReSCF=θtρUlocal0.82æèçöø÷μ=-35.088lnhθtæèçöø÷+319.51+f(+ΔHcrossflow)-f(-ΔHcrossflow)其中ꎬh为壁面粗糙度高度ꎬθt为动量厚度ꎬ11气体物理2024年㊀第9卷ΔHcrossflow是横流强度抬升项ꎮ向星皓提出的高速横流转捩判据ꎬ其中高速横流源项DSCF ̄H为DSCF ̄H=cCFρmin(ReSCF ̄H-Re~θtꎬ0)FθtReSCF ̄H=CCF ̄1lnhlμ+CCF ̄2+(Hcrossflow)其中ꎬCCF ̄1=-9.618ꎬCCF ̄2=128.33ꎻlμ为粗糙度参考高度ꎬlμ=1μmꎻf(Hcrossflow)为抬升函数f(Hcrossflow)=60000.1066-ΔHcrossflow+50000(0.1066-ΔHcrossflow)2其中ꎬΔHcrossflow与Langtry低速横流修正中保持一致ꎮ1.3.3㊀SST可压缩修正高超声速流动具有强可压缩性ꎬ所以在进行高超声速计算时ꎬ应该对湍流模型进行可压缩修正ꎮSarkar[31]提出了膨胀耗散修正ꎬ对SST湍流模型中的闭合系数β∗ꎬβ进行了可压缩修正ꎬWilcox[32]在Sarkar修正的基础上考虑了可压缩生成项产生时的延迟效应ꎬ使得可压缩修正在湍流Mach数较小的近壁面关闭ꎬ在湍流Mach数较大的自由剪切层打开ꎬ本文采用Wilcox提出的可压缩性修正β∗=β∗0[1+ξ∗F(Mat)]β=β0-β∗0ξ∗F(Mat)其中ꎬβ0ꎬβ∗均为原始模型中的系数ꎬξ∗=1.5ꎮF(Mat)=[Mat-Mat0]H(Mat-Mat0)Mat0=1/4ꎬH(x)=0ꎬxɤ01ꎬx>0{其中ꎬMat=2k/a为湍流Mach数ꎬa为当地声速ꎮ2㊀网格无关性验证及数值方法确认2.1㊀网格无关性验证计算采用3套网格ꎬ考虑到HyTRV的几何对称性ꎬ生成3套半模网格ꎬ第1层网格高度为1ˑ10-6mꎬ确保y+<1ꎬ流向ˑ法向ˑ周向的网格数分别为:网格1是301ˑ201ˑ201ꎬ网格2是301ˑ301ˑ201ꎬ网格3是401ˑ381ˑ281ꎮ全模下表面如图2所示ꎬ选取y/L=0中心线和x/L=0.5处ꎬ对比3套网格的表面摩阻系数ꎬ计算结果如图3所示ꎮ采用网格1时ꎬ表面摩阻系数分布与另外两个结果存在明显差异ꎻ而采用网格2和网格3时ꎬ表面摩阻系数曲线基本重合ꎬ表明在流向㊁法向和周向均满足网格无关性ꎬ后续数值计算采用网格2ꎮ图2㊀截取位置示意图Fig.2㊀Schematicdiagramoftheinterceptionlocation(a)Surfacefrictionaty/L=0(b)Surfacefrictionatx/L=0.5图3㊀采用3套网格计算得到的摩阻对比Fig.3㊀Comparisonofthefrictiondragcalculatedusingthreesetsofgrids2.2㊀数值方法和自研软件的确认采用修正后的转捩模型对HyTRV开展计算ꎬ计算工况为Ma=6ꎬ来流温度Tɕ=97Kꎬ单位21第2期章录兴ꎬ等:Mach数和壁面温度对HyTRV边界层转捩的影响Reynolds数为Re=1.1ˑ107/mꎬ攻角α=0ʎꎬ来流湍流度FSTI=0.8%ꎬ壁面温度T=300Kꎮ为方便对比分析ꎬ计算结果与参考结果均采用上下对称形式布置ꎬ例如ꎬ图4是模型下表面计算结果与实验结果对比:对于下表面两侧转捩的起始位置ꎬ高超声速修正前的转捩位置在x=0.68m附近ꎬ高超声速修正后的计算结果与实验结果吻合良好ꎬ均在x=0.60m附近ꎬ并且湍流边界层区域形状基本一致ꎬ说明修正后的转捩模型能够较好地预测HyTRV转捩的位置ꎮ(a)Calculationofthefrictiondistribution(beforehypersoniccorrection)(b)Calculationofthefrictiondistribution(afterhypersoniccorrection)(c)Experimentalresultsoftheheatfluxdistribution[17]图4㊀下表面计算结果和实验结果对比Fig.4㊀Comparisonofthecalculatedandexperimentalresultsonthelowersurface3㊀HyTRV转捩的基本流动特性计算工况采用Ma=6ꎬ攻角α=0ʎꎬ来流湍流度FSTI=0.6%ꎬ分析HyTRV转捩的基本流动特性ꎮ从图5可以看出ꎬ模型两侧和顶端均出现高压区ꎬ高压区之间为低压区ꎬ横截面上存在周向压力梯度ꎬ流动从高压区向低压区汇集ꎬ从而在下表面中心线附近和上表面两侧腰部区域均形成流向涡结构(见图6)ꎬ沿流动方向ꎬ高压区域逐渐扩大ꎬ流向涡结构的影响范围也越大ꎮ在流向涡结构的边缘位置ꎬ壁面附近的低速流体被抬升到外壁面区域ꎬ外壁面区域的高速流体又被带入到近壁面区域ꎬ进而导致流向涡结构边缘处壁面的摩阻显著增加ꎬ最终诱发转捩ꎬ这些流动特征与文献[15]的结果一致ꎮ图7显示了上下表面摩阻的分布情况ꎬ其中上表面两侧区域在x/L=0.80附近ꎬ摩阻显著增加ꎬ出现明显的转捩现象ꎬ转捩区域分布在两侧边缘位置ꎻ而下表面两侧区域在x/L=0.75附近ꎬ也出现明显的转捩ꎬ转捩区域相对集中在中心线两侧ꎮ图5㊀不同截面位置处的压力云图Fig.5㊀Pressurecontoursatdifferentcross ̄sectionlocations图6㊀不同截面位置处的流向速度云图Fig.6㊀Streamwisevelocitycontoursatdifferentcross ̄sectionlocations31气体物理2024年㊀第9卷(a)Uppersurface㊀㊀㊀㊀㊀(b)Lowersurface图7㊀上下表面摩阻分布云图Fig.7㊀Frictioncoefficientcontoursontheupperandlowersurfaces4㊀不同Mach数对HyTRV转捩的影响保持来流湍流度FSTI=0.6%不变ꎬMach数变化范围为3~8ꎮ图8是不同Mach数条件下HyTRV上下表面的摩阻分布云图ꎬ从图中可知ꎬ随着Mach数的增加ꎬ上下表面的湍流区域均逐渐减少ꎬ其中上表面两侧转捩起始位置由x/L=0.56附近后移至x/L=0.92附近ꎬ下表面两侧转捩起始位置由x/L=0.48附近后移至x/L=0.99附近ꎬ上下表面两侧转捩起始位置均大幅后移ꎬ说明Mach数对HyTRV转捩的影响很大ꎮuppersurface㊀㊀㊀㊀㊀㊀㊀㊀㊀㊀㊀㊀㊀㊀㊀㊀lowersurface(a)Ma=3uppersurface㊀㊀㊀㊀㊀㊀㊀㊀㊀㊀㊀㊀㊀㊀㊀㊀lowersurface(b)Ma=4uppersurface㊀㊀㊀㊀㊀㊀㊀㊀㊀㊀㊀㊀㊀㊀㊀㊀lowersurface(c)Ma=541第2期章录兴ꎬ等:Mach数和壁面温度对HyTRV边界层转捩的影响uppersurface㊀㊀㊀㊀㊀㊀㊀㊀㊀㊀㊀㊀㊀㊀㊀㊀lowersurface(d)Ma=6uppersurface㊀㊀㊀㊀㊀㊀㊀㊀㊀㊀㊀㊀㊀㊀㊀㊀lowersurface(e)Ma=7uppersurface㊀㊀㊀㊀㊀㊀㊀㊀㊀㊀㊀㊀㊀㊀㊀㊀lowersurface(f)Ma=8图8㊀不同Mach数条件下摩阻系数分布云图Fig.8㊀FrictioncoefficientcontoursatdifferentMachnumbers上表面选取图7中z/L=0.12的位置ꎬ下表面选取z/L=0.10的位置进行分析ꎮ从图9中可以分析出ꎬ随着Mach数的增加ꎬ上表面转捩起始位置不断后移ꎬ当Mach数增加到7时ꎬ由于湍流区的缩小ꎬ此处位置不再发生转捩ꎬ此外ꎬMach数越高层流区摩阻系数越大ꎻ下表面转捩起始位置也不断后移ꎬ当Mach数增加到8时ꎬ此处位置不再发生转捩ꎬ此外ꎬMach数越高ꎬ湍流区的摩阻系数越小ꎬ这些结论与关于来流Mach数对转捩位置影响的普遍研究结论一致ꎮ(a)Uppersurface㊀㊀㊀㊀㊀(b)Lowersurface图9㊀不同位置摩阻系数随Mach数的变化Fig.9㊀VariationoffrictioncoefficientwithMachnumberatdifferentlocations51气体物理2024年㊀第9卷5㊀不同壁面温度对HyTRV转捩的影响保持来流湍流度FSTI=0.6%及Ma=6不变ꎬ壁面温度的变化范围为150~900Kꎮ图10是不同壁面温度条件下HyTRV上下表面的摩阻分布云图ꎬ可以看出随着壁面温度的增加ꎬ上表面两侧湍流区域先是缓慢扩大ꎬ在壁面温度为500K时湍流区域快速缩小ꎬ增加到900K时ꎬ已无明显湍流区域ꎻ下表面两侧湍流区域先是无明显变化ꎬ同样当壁面温度升高到500K时ꎬ湍流区域快速缩小ꎬ当壁面温度升高到700K时ꎬ两侧已经无明显的湍流区域ꎬ相比上表面两侧湍流区域ꎬ下表面湍流区域消失得更早ꎮ由此可以得出壁面温度对转捩的产生有较大的影响ꎬ壁面温度增加到一定程度将导致HyTRV没有明显的转捩现象ꎮuppersurface㊀㊀㊀㊀㊀㊀㊀㊀㊀㊀㊀㊀㊀㊀㊀㊀lowersurface(a)T=150Kuppersurface㊀㊀㊀㊀㊀㊀㊀㊀㊀㊀㊀㊀㊀㊀㊀㊀lowersurface(b)T=200Kuppersurface㊀㊀㊀㊀㊀㊀㊀㊀㊀㊀㊀㊀㊀㊀㊀㊀lowersurface(c)T=300Kuppersurface㊀㊀㊀㊀㊀㊀㊀㊀㊀㊀㊀㊀㊀㊀㊀㊀lowersurface(d)T=500K61第2期章录兴ꎬ等:Mach数和壁面温度对HyTRV边界层转捩的影响uppersurface㊀㊀㊀㊀㊀㊀㊀㊀㊀㊀㊀㊀㊀㊀㊀㊀lowersurface(e)T=700Kuppersurface㊀㊀㊀㊀㊀㊀㊀㊀㊀㊀㊀㊀㊀㊀㊀㊀lowersurface(f)T=900K图10㊀不同壁面温度条件下摩阻系数分布云图Fig.10㊀Frictioncoefficientcontoursatdifferentwalltemperatureconditions上表面选取z/L=0.125的位置ꎬ下表面选取z/L=0.100的位置进行分析ꎮ从图11中可以分析出ꎬ随着壁面温度的增加ꎬ上表面转捩起始位置先前移ꎬ当壁面温度增加到500K时ꎬ转捩起始位置后移ꎬ转捩区长度逐渐增加ꎬ层流区域的摩阻系数逐渐增加ꎬ当壁面温度增加到700K时ꎬ该位置已不再出现转捩ꎻ下表面转捩起始位置先小幅后移ꎬ当壁面温度增加到300K时ꎬ转捩起始位置开始后移ꎬ当壁面温度增加到700K时ꎬ由于湍流区域的减小ꎬ该位置不再发生转捩ꎮ(a)Uppersurface㊀㊀㊀㊀㊀(b)Lowersurface图11㊀不同位置摩阻系数随壁面温度的变化Fig.11㊀Variationoffrictioncoefficientwithwalltemperatureatdifferentlocations为进一步分析壁面温度的影响ꎬ本文分别在上下表面湍流区选取一点(0.9ꎬ0.029ꎬ0.14)ꎬ(0.97ꎬ-0.34ꎬ0.12)ꎬ分析边界层湍动能剖面ꎬ结果如图12所示ꎮ从图中可以看到ꎬ随着壁面温度升高ꎬ边界层厚度先略微变厚ꎬ再变薄ꎬ当壁面温度升高到700K时ꎬ边界层厚度迅速降低ꎮ这些结果与转捩位置先前移再后移的结论相符合ꎬ因为边界层厚度会影响不稳定波的时间和空间尺度ꎬ边界层厚度低时ꎬ不稳定波增长速度变慢ꎬ延迟转捩发生ꎮ需要指出的是ꎬ仅采用当前使用的方法ꎬ无法从更深层71气体物理2024年㊀第9卷次揭示转捩反转的流动机理ꎬ而须另外借助稳定性分析方法ꎬ例如ꎬ使用eN方法开展基于模态的稳定性研究ꎮ文献[33]采用该手段研究了大掠角平板钝三角翼随壁温比变化出现转捩反转的内在机理:壁温比升高促进横流模态和第1模态扰动增长ꎬ抑制第2模态发展ꎬ在第1㊁2模态联合作用影响下ꎬ出现转捩反转现象ꎮ我们将在后续开展进一步研究ꎮ(a)Uppersurface(b)Lowersurface图12㊀不同位置湍动能剖面随壁面温度的变化Fig.12㊀Variationofturbulentkineticenergywithwalltemperatureatdifferentlocations6㊀结论针对HyTRV转捩问题ꎬ在Mach数Ma=3~8ꎬ壁面温度T=150~900K的条件下ꎬ基于课题组自研软件ꎬ对γ ̄Re~θt转捩模型和SST湍流模型进行了高超声速修正ꎬ研究了Mach数和壁面温度对HyTRV转捩的影响ꎬ得出以下结论:1)经过高超声速修正后的γ ̄Re~θt转捩模型和SST湍流模型能够较为准确地预测HyTRV转捩位置ꎬ并且湍流边界层区域形状与实验结果基本一致ꎻHyTRV存在多个不同的转捩区域ꎬ上表面两侧转捩区域分布在两侧边缘位置ꎬ下表面两侧转捩区域分布在中心线两侧ꎮ2)Mach数的增加会导致上下表面转捩起始位置均大幅后移ꎬ湍流区大幅缩小ꎬ但当Mach数增加到8时ꎬ湍流区仍然存在ꎬ并没有消失ꎻ上表面层流区摩阻不断增加ꎬ下表面湍流区摩阻不断减小ꎮ3)壁面温度的增加会导致上下表面转捩起始位置先前移ꎬ再后移ꎬ这与边界层厚度变化规律一致ꎬ当壁面温度增加到700K时ꎬ下表面湍流区已经基本消失ꎬ当壁面温度增加到900K时ꎬ上表面湍流区也基本消失ꎻ上表面在层流区域的摩阻系数逐渐增大ꎬ在湍流区的摩阻系数逐渐减小ꎮ致谢㊀感谢中国空气动力研究与发展中心和空天飞行空气动力科学与技术全国重点实验室提供的HyTRV模型数据和实验数据ꎮ参考文献(References)[1]㊀OberingIIIHꎬHeinrichsRL.Missiledefenseforgreatpowerconflict:outmaneuveringtheChinathreat[J].Stra ̄tegicStudiesQuarterlyꎬ2019ꎬ3(4):37 ̄56. [2]ChengCꎬWuJHꎬZhangYLꎬetal.Aerodynamicsanddynamicstabilityofmicro ̄air ̄vehiclewithfourflappingwingsinhoveringflight[J].AdvancesinAerodynamicsꎬ2020ꎬ2(3):5.[3]BertinJJꎬCummingsRM.Fiftyyearsofhypersonics:whereweᶄvebeenꎬwhereweᶄregoing[J].ProgressinAerospaceSciencesꎬ2003ꎬ39(6/7):511 ̄536. [4]陈坚强ꎬ涂国华ꎬ张毅锋ꎬ等.高超声速边界层转捩研究现状与发展趋势[J].空气动力学学报ꎬ2017ꎬ35(3):311 ̄337.ChenJQꎬTuGHꎬZhangYFꎬetal.Hypersnonicboundarylayertransition:whatweknowꎬwhereshallwego[J].ActaAerodynamicaSinicaꎬ2017ꎬ35(3):311 ̄337(inChinese).[5]段毅ꎬ姚世勇ꎬ李思怡ꎬ等.高超声速边界层转捩的若干问题及工程应用研究进展综述[J].空气动力学学报ꎬ2020ꎬ38(2):391 ̄403.DuanYꎬYaoSYꎬLiSYꎬetal.Reviewofprogressinsomeissuesandengineeringapplicationofhypersonicboundarylayertransition[J].ActaAerodynamicaSinicaꎬ2020ꎬ38(2):391 ̄403(inChinese).[6]ZhangYFꎬZhangYRꎬChenJQꎬetal.Numericalsi ̄81第2期章录兴ꎬ等:Mach数和壁面温度对HyTRV边界层转捩的影响mulationsofhypersonicboundarylayertransitionbasedontheflowsolverchant2.0[R].AIAA2017 ̄2409ꎬ2017. [7]KrauseMꎬBehrMꎬBallmannJ.Modelingoftransitioneffectsinhypersonicintakeflowsusingacorrelation ̄basedintermittencymodel[R].AIAA2008 ̄2598ꎬ2008. [8]YiMRꎬZhaoHYꎬLeJL.Hypersonicnaturalandforcedtransitionsimulationbycorrelation ̄basedintermit ̄tency[R].AIAA2017 ̄2337ꎬ2017.[9]向星皓ꎬ张毅锋ꎬ袁先旭ꎬ等.C ̄γ ̄Reθ高超声速三维边界层转捩预测模型[J].航空学报ꎬ2021ꎬ42(9):625711.XiangXHꎬZhangYFꎬYuanXXꎬetal.C ̄γ ̄Reθmodelforhypersonicthree ̄dimensionalboundarylayertransitionprediction[J].ActaAeronauticaetAstronauticaSinicaꎬ2021ꎬ42(9):625711(inChinese).[10]McDanielRDꎬNanceRPꎬHassanHA.Transitiononsetpredictionforhigh ̄speedflow[J].JournalofSpacecraftandRocketsꎬ2000ꎬ37(3):304 ̄309.[11]PappJLꎬDashSM.Rapidengineeringapproachtomodelinghypersoniclaminar ̄to ̄turbulenttransitionalflows[J].JournalofSpacecraftandRocketsꎬ2005ꎬ42(3):467 ̄475.[12]JulianoTJꎬSchneiderSP.InstabilityandtransitionontheHIFiRE ̄5inaMach ̄6quiettunnel[R].AIAA2010 ̄5004ꎬ2010.[13]杨云军ꎬ马汉东ꎬ周伟江.高超声速流动转捩的数值研究[J].宇航学报ꎬ2006ꎬ27(1):85 ̄88.YangYJꎬMaHDꎬZhouWJ.Numericalresearchonsupersonicflowtransition[J].JournalofAstronauticsꎬ2006ꎬ27(1):85 ̄88(inChinese).[14]袁先旭ꎬ何琨ꎬ陈坚强ꎬ等.MF ̄1模型飞行试验转捩结果初步分析[J].空气动力学学报ꎬ2018ꎬ36(2):286 ̄293.YuanXXꎬHeKꎬChenJQꎬetal.PreliminarytransitionresearchanalysisofMF ̄1[J].ActaAerodynamicaSinicaꎬ2018ꎬ36(2):286 ̄293(inChinese).[15]陈坚强ꎬ涂国华ꎬ万兵兵ꎬ等.HyTRV流场特征与边界层稳定性特征分析[J].航空学报ꎬ2021ꎬ42(6):124317.ChenJQꎬTuGHꎬWanBBꎬetal.Characteristicsofflowfieldandboundary ̄layerstabilityofHyTRV[J].ActaAeronauticaetAstronauticaSinicaꎬ2021ꎬ42(6):124317(inChinese).[16]陈坚强ꎬ刘深深ꎬ刘智勇ꎬ等.用于高超声速边界层转捩研究的标模气动布局及设计方法.中国:109969374B[P].2021 ̄05 ̄18.ChenJQꎬLiuSSꎬLiuZYꎬetal.Standardmodelaero ̄dynamiclayoutanddesignmethodforhypersonicboundarylayertransitionresearch.CNꎬ109969374B[P].2021 ̄05 ̄18(inChinese).[17]LiuSSꎬYuanXXꎬLiuZYꎬetal.Designandtransitioncharacteristicsofastandardmodelforhypersonicboundarylayertransitionresearch[J].ActaMechanicaSinicaꎬ2021ꎬ37(11):1637 ̄1647.[18]ChenXꎬDongSWꎬTuGHꎬetal.Boundarylayertran ̄sitionandlinearmodalinstabilitiesofhypersonicflowoveraliftingbody[J].JournalofFluidMechanicsꎬ2022ꎬ938(408):A8.[19]QiHꎬLiXLꎬYuCPꎬetal.Directnumericalsimulationofhypersonicboundarylayertransitionoveralifting ̄bodymodelHyTRV[J].AdvancesinAerodynamicsꎬ2021ꎬ3(1):31.[20]万兵兵ꎬ陈曦ꎬ陈坚强ꎬ等.三维边界层转捩预测HyTEN软件在高超声速典型标模中的应用[J].空天技术ꎬ2023(1):150 ̄158.WanBBꎬChenXꎬChenJQꎬetal.ApplicationsofHyTENsoftwareforpredictingthree ̄dimensionalboundary ̄layertransitionintypicalhypersonicmodels[J].AerospaceTechnologyꎬ2023(1):150 ̄158(inChinese). [21]MenterFRꎬLangtryRBꎬLikkiSRꎬetal.Acorrelation ̄basedtransitionmodelusinglocalvariables PartⅠ:modelformulation[J].JournalofTurbomachineryꎬ2006ꎬ128(3):413 ̄422.[22]LangtryRBꎬMenterFRꎬLikkiSRꎬetal.Acorrelation ̄basedtransitionmodelusinglocalvariables PartⅡ:testcasesandindustrialapplications[J].JournalofTur ̄bomachineryꎬ2006ꎬ128(3):423 ̄434.[23]LangtryRBꎬMenterFR.Correlation ̄basedtransitionmodelingforunstructuredparallelizedcomputationalfluiddynamicscodes[J].AIAAJournalꎬ2009ꎬ47(12):2894 ̄2906.[24]孟德虹ꎬ张玉伦ꎬ王光学ꎬ等.γ ̄Reθ转捩模型在二维低速问题中的应用[J].航空学报ꎬ2011ꎬ32(5):792 ̄801.MengDHꎬZhangYLꎬWangGXꎬetal.Applicationofγ ̄Reθtransitionmodeltotwo ̄dimensionallowspeedflows[J].ActaAeronauticaetAstronauticaSinicaꎬ2011ꎬ32(5):792 ̄801(inChinese).[25]牟斌ꎬ江雄ꎬ肖中云ꎬ等.γ ̄Reθ转捩模型的标定与应用[J].空气动力学学报ꎬ2013ꎬ31(1):103 ̄109.MouBꎬJiangXꎬXiaoZYꎬetal.Implementationandcaliberationofγ ̄Reθtransitionmodel[J].ActaAerody ̄namicaSinicaꎬ2013ꎬ31(1):103 ̄109(inChinese). [26]郭隽ꎬ刘丽平ꎬ徐晶磊ꎬ等.γ ̄Re~θt转捩模型在跨声速涡轮叶栅中的应用[J].推进技术ꎬ2018ꎬ39(9):1994 ̄2001.91气体物理2024年㊀第9卷GuoJꎬLiuLPꎬXuJLꎬetal.Applicationofγ ̄Re~θttran ̄sitionmodelintransonicturbinecascades[J].JournalofPropulsionTechnologyꎬ2018ꎬ39(9):1994 ̄2001(inChinese).[27]郑赟ꎬ李虹杨ꎬ刘大响.γ ̄Reθ转捩模型在高超声速下的应用及分析[J].推进技术ꎬ2014ꎬ35(3):296 ̄304.ZhengYꎬLiHYꎬLiuDX.Applicationandanalysisofγ ̄Reθtransitionmodelinhypersonicflow[J].JournalofPropulsionTechnologyꎬ2014ꎬ35(3):296 ̄304(inChi ̄nese).[28]孔维萱ꎬ阎超ꎬ赵瑞.γ ̄Reθ模式应用于高速边界层转捩的研究[J].空气动力学学报ꎬ2013ꎬ31(1):120 ̄126.KongWXꎬYanCꎬZhaoR.γ ̄Reθmodelresearchforhigh ̄speedboundarylayertransition[J].ActaAerody ̄namicaSinicaꎬ2013ꎬ31(1):120 ̄126(inChinese). [29]张毅锋ꎬ何琨ꎬ张益荣ꎬ等.Menter转捩模型在高超声速流动模拟中的改进及验证[J].宇航学报ꎬ2016ꎬ37(4):397 ̄402.ZhangYFꎬHeKꎬZhangYRꎬetal.ImprovementandvalidationofMenterᶄstransitionmodelforhypersonicflowsimulation[J].JournalofAstronauticsꎬ2016ꎬ37(4):397 ̄402(inChinese).[30]LangtryRBꎬSenguptaKꎬYehDTꎬetal.Extendingtheγ ̄Reθtcorrelationbasedtransitionmodelforcrossfloweffects(Invited)[R].AIAA2015 ̄2474ꎬ2015. [31]SarkarS.Thepressure ̄dilatationcorrelationincompressi ̄bleflows[J].PhysicsofFluidsAꎬ1992ꎬ4(12):2674 ̄2682.[32]WilcoxDC.Dilatation ̄dissipationcorrectionsforadvancedturbulencemodels[J].AIAAJournalꎬ1992ꎬ30(11):2639 ̄2646.[33]马祎蕾ꎬ余平ꎬ姚世勇.壁温对钝三角翼边界层稳定性及转捩影响[J].空气动力学学报ꎬ2020ꎬ38(6):1017 ̄1026.MaYLꎬYuPꎬYaoSY.Effectofwalltemperatureonstabilityandtransitionofhypersonicboundarylayeronabluntdeltawing[J].ActaAerodynamicaSinicaꎬ2020ꎬ38(6):1017 ̄1026(inChinese).02。
一种小天体旋转参数估计方法
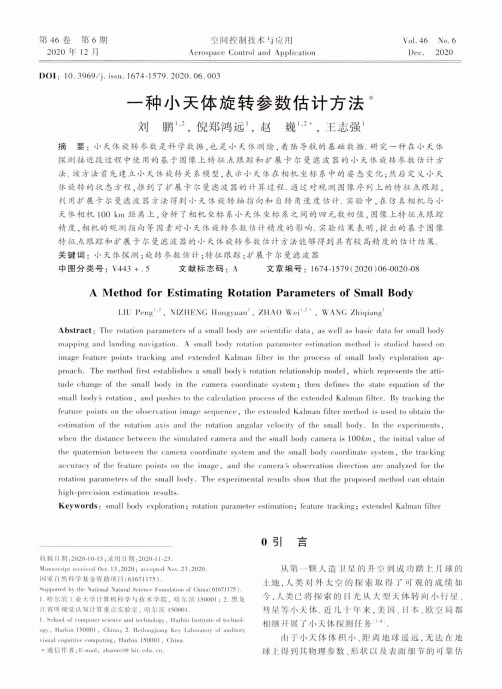
第46卷第6期 2020年12月空间控制技术与应用Aerospace Control and ApplicationVol.46 No.6Dec.2020D O I:10. 3969/j.issn. 1674-1579.2020.06.003一种小天体旋转参数估计方法+刘鹏K2,倪郑鸿远1,赵巍王志强1摘要:小天体旋转参数是科学教据,也是小天体测绘,着陆导航的基础数据.研究一种在小天体探测接近段过程中使用的基于图像上特征点跟踪和扩展卡尔曼滤波器的小天体旋转参数估计方法.该方法首先建立小天体旋转关系模型,表示小天体在相机坐标系中的姿态变化;然后定义小天体旋转的状态方程,推到了扩展卡尔曼滤波器的计算过程.通过对观测图像序列上的特征点跟踪,利用扩展卡尔曼滤波器方法得到小天体旋转轴指向和自转角速度估计.实验中,在仿真相机与小天体相机100 k m距离上,分析了相机坐标系小天体坐标系之间的四元数初值,图像上特征点跟踪精度,相机的观测指向等因素对小天体旋转参数估计精度的影响.实验结果表明,提出的基于图像特征点跟踪和扩展卡尔曼滤波器的小天体旋转参数估计方法能够得到具有较高精度的估计结果.关键词:小天体探测;旋转参教估计;特征跟踪;扩展卡尔曼滤波器中图分类号:V443 +.5文献标志码:A文章编号:1674-1579(2020)06-0020-08A Method for Estimating Rotation Parameters of Small BodyUU Peng12,NIZHENG Hongyuan1 ,ZHAO Wei1'2* ,WANG Zhi(|iang' Abstract:The rotation parameters of a small body are scientific data,as well as basic data for small body mapping and landing navigation.A small body rotation parameter estimation method is studied based on image feature points tracking and extended Kalman filter in the process of small body exploration ap-proach.The method first establishes a small body^ rotation relationship model,whidh represents the attitude change of the small body in the camera coordinate system;then defines the state equation of the small body s rotation,and pushrs to the calculation process of the extended Kalman filter.By tracking the feature points on the observation image sequence,the extended Kalman filter method is used to obtain the estimation of the rotation axis and the rotation angular velocity of the small body.In the experiments, when the distance between the simulated camera and the small body camera is\00km,the initial value of the(juaternion between the camera coordinate system and the small body coordinate system,the tracking accuracy of the feature points on the image,and the cameras observation direction are analyzed for the rotation parameters of the small body.The experimental results show that the proposed method can obtain high-precision estimation results.Keywords:small l)〇d y exploration;rotation parameter estimation;feature tracking;extended Kalman filter收稿日期:2020-丨0-13 ;录用日期:2020-11 -23.\1amis<Tipl received Oct. 13,2020;atcepled Nov. 23 ,2020.间家A然科学基金资助项目(61671175 ).SupporttMl l)\ Nationiil Natural Sci«*ncr Foundation of 61671175 ).I.哈尔滨丨:业大学计算机科学与技术学院,哈尔滨150001; 2.黑龙江拧听视觉认知计算重点实验室,哈尔滨150001.1. School of computer science and technology, Harbin Institute of technology, Harbin 150001, China;2. Heilongjiang Key Laboratory of auditory visual cognhivt* computing, Harbin 150001, China.* 通 fij•作片:K,mail: zliaowt*i@ hit. rn.0引言从第一颗人造卫星的升空到成功踏上月球的土地,人类对外太空的探索取得了可观的成绩如今,人类已将探索的目光从大型天体转向小行星、彗星等小天体.近几十年来,美国、日本、欧空局都相继开展了小天体探测任务14 .由于小天体体积小、距离地球遥远,无法在地球上得到其物理参数、形状以及表面细节的可靠估第6期刘鹏等:一种小天体旋转参数估计方法‘计,并且由于小天体形状不规则,观测难度大,探测 器很难获取其大量的高分辨率的图像,所以利用图像中小天体的轮廓信息来恢复三维模型的方法具有重要的研究意义.对小天体旋转参数的估计包括旋转轴方向估计和自转角速度估计,典型方法包括光变曲线法和视觉运动参数估计法.用光变曲线估计小天体的旋转参数假设小天体为椭球体,通过光变曲线的变化估计小天体的旋转轴指向和自转速度.物体运动参数估计也是计算机视觉中的一项重要研究内容,在探测器导航着陆、目标跟踪等方面都会涉及到这一问题.本文研究一种基于小天体探测接近过程中使用图像上特征点跟踪和扩展卡尔曼滤波器的小天体旋转参数估计方法.该方法首先建立小天体旋转关系模型,表示小天体在相机坐标系中的姿态变化;然后定义小天体旋转的状态方程,通过对观测图像序列上的特征点跟踪,利用扩展卡尔曼滤波器方法得到小天体旋转轴指向和自转角速度估计.在实验中,分析了相机坐标系小天体坐标系之间的四元数初值,图像上特征点跟踪精度,相机的观测指向等因素对小天体旋转参数估计精度的影响.实验结果表明,本文提出的基于图像特征点跟踪和扩展卡尔曼滤波器的小天体旋转参数估计方法能够得到具有较高精度的估计结果.1相关工作1.1基于光变曲线的小天体旋转参数估计当小天体在视野中成像为小面积扩点目标状态时,探测器相机无法观察到小天体的轮廓形状和具体细节,此时光变曲线的信息可以为旋转轴的估计提供参考.假设小天体的形状用椭球体表示,记 椭球体的三个半轴长度分别为6為C,假设c为旋转轴可以使椭球体的动能最小,即处于稳定状态.在人射光为平行光且能量相同的情况下,小天体的反射亮度会与其视截面的面积大小成正比,而其视截面的面积大小会随着小天体的旋转呈周期性变化.同时,当相机的视线方向与小天体旋转轴的夹角发生变化时,视截面的面积也会发生相应的变化,进而导致光变曲线的结果不同.通过光变曲线的振幅反演可以得到小天体的旋转轴指向和旋转周期[5].1.2基于视觉的旋转参数估计方法运动参数估计是计算机视觉领域的一项重要的研究内容,在相机标定、探测器导航着陆、目标跟踪等方面都会涉及到这一问题.按照估计的原理可以分为直接法、光流法和基于特征的方法三类161.直接法是最简单的运动参数估计方法,它既不需要提前进行特征提取和匹配也不需要计算光流,直接利用图像中每个像素点的亮度来估计物体的运动.文献[7]提出了一个直接利用亮度梯度来估计物体运动信息的方法,该方法对两帧图像选择一个任意的固定点,这个点包含一个面片(patch)和对 应的速度.通过解算两个线性方程组,可以得到像素平移参数,将这些平移参数应用于第二帧图像中的每一个像素点就得到了固定的第二帧图像.最后求解3 x3矩阵的特征值和特征向量,利用最小特征值对应的特征向量,第一帧图像以及固定的第二帧图像可以求出平移方向、旋转速度和相对深度.Heel 提出了基于卡尔曼滤波器的直接估计场景结构和相机相对于场景的位姿的方法.随着时间的推移,卡尔曼滤波器可以逐渐地提升预测的效果.在更新阶段,卡尔曼滤波器会结合当前的深度估计和测量值对估计做出校正.Gibson提出了光流的概念:物体在空间中运动,由相机成像后,图像上像素运动的瞬时速度称为光流.对场景中的运动物体进行连续拍摄得到图像序列,相邻图像中物体运动具有一定的相关性,光流法利用这种相关性,通过分析像素随时间产生的变化,可以计算出物体的运动信息.根据引人的约束条件的不同,分析方法也不同,例如基于梯度的Lu-cas-Kanade算法W、区域匹配法等.由于光流法假设物体亮度不变,且计算量较大无法进行实时预测.所以较难应用于小天体的运动参数估计中.基于特征的方法是目前位姿参数估计领域的研究热门,也是成功应用于小天体运动参数估计的方法,首先要从图像中提取的特征,然后进行特征匹配,根据跟踪的特征点信息来估计物体的运动参数.根据使用的特征的不同,方法的适用场景也不相同.常用的特征提取算法可以分为尺度不变特征点算法和角点特征点算法.S I F T特征〜是尺度不变特征检测算法的代表,这一类特征的优点是对图像的尺度、旋转以及亮度变化不敏感,但是执行效率较低;而以H arris角点算法计算简单,但是由于不具有尺度不变性.基于特征的方法可以分为两类:批 处理算法和递归算法.批处理算法不满足实时性,而递归算法只需要上一次的状态估计和当前的测量值,就能得到当前的状态估计,占用的存储资源少,执行效率高.BR O ID A提出了基于循环扩展卡尔曼滤波器(1E K F)的递归算法n° .I U等人提出的方.22•空间控制技术与应用第46卷法将旋转平移表示为任意阶多项式的形式,将问题转化为多项式系数的估计问题11 .2小天体旋转参数估计2.1姿态表示和观测方程小天体旋转参数估计主要涉及三个坐标系:世界坐标系- ATFZ、小行星坐标系相机坐标系- x p,如图1所示.用四元数表示相机坐标系与小天体坐标系之间的姿态关系.对于一个四元数^其数学代数形式为:g= </,+ ;+</2+也+ A:</4,去满足正交化约束+ fd=i(i)如果一个物体绕其x y z三个轴以角速度w (〇>:,))旋转,那么对应的旋转矩阵为:R(h ~ Ql ~ <ll+ (ll 2 (<•/,(/,+ q3qA ) 2(q tq, -q2qt)2(9i<?2-9394) +q2-q\ +q]2(n+ n)-2(<7i93 + H)2(H H)- q'\- 9:+ <7i+ <?4-设(时刻小天体坐标系原点0、在相机坐标系中的坐标定义为s n(0- [*〇(0 r〇(020(〇 ]1(2)在小天体坐标系0、.- .r y z中的小天体表面一点声,/(•)为状态转移函数.状态变量的微分近似为: s⑴=[丨 S,'M+12]= + 士 .、⑴(7)表1状态变量的描述S,。
第五批天文学新名词-lamost

发布试用新词第五批天文学新名词天文学名词审定委员会在全国科学技术名词审定委员会第二届委员会的任务中,有一项是审定新词。
《自然科学术语研究》(内部刊)1991年第1期刊登了天文学第一批新词362个,1994年第1期刊登了第二批298个,1997年第1期刊登了第三批425个。
《科技术语研究》1999年第3期刊登了第四批103个。
下列是第五批天文学新词125个。
希望读者提出意见和建议,以期更好地完成最终审定。
下列天文学名词仍按汉语拼音顺序排列。
A暗能量 dark energyB白昼流星 daylight meteor百武彗星 comet Hyakutake伴星云 satellite nebula闭合型叉式装置 closed fork mounting表面亮度起伏方法 surface2brightness2fluctuation technique并址观测 colocational observation薄环 ethereal ringC超大行星 superplanet超密天体 ultradense object超密中子星 ultradense neutron starCCD成像 CCD imaging磁层吸积 magnetospheric accretion 磁耀斑 magnetic flareD大孔径全天巡天望远镜 Large2aperture Synoptic Sur2 vey Telescope,L SST待估参数 solve2for parameter单口径远红外天文台 Single2Aperture Far2Infra2Red Observatory,SAFIR丹尼斯[近红外深空巡天] Deep Near2Infrared Sur2 vey,DEN IS低吸收空间 absorption2poor space地球化 terraforming地球时 Terrestrial Time,TT地心常数 geocentric constant地心坐标时 G eocentric Coordinate Time,TCG 多光纤分光 multi2fiber spectroscopy多光纤分光仪 multi2fiber spectrometerF[飞镖]球载望远镜 Balloon Observations of Millimet2 ric Extragalactic Radiation and G eophysics telescope, Boomerang telescopeG改进型马蹄式装置 modified horseshoe mounting 高能星系 high2energy galaxy 古在共振 K ozai resonance 光学钟 optical clock6科技术语研究 第4卷 第2期 2002年滚筒仪星云 Spirograph Nebula,IC418国际地球参考系 International Terrestrial Reference System,ITRS国际天球参考系 International Celestial Reference System,ICRS国家虚拟天文台 National Virtual Observatory,NVO H哈勃后续计划 Hubble Heritage Project 海尔-波普彗星 comet Hale2Bopp海外天体 trans2Neptunian object,TNO 恒星质量黑洞 stellar2mass black hole彗星区 cometary zone火星尘暴 Martian dust storm 火星大冲 Martian opposition 火星年 Martian yearJ激光干涉引力波观测台 Laser Interferometer G ravita2 tional2wave Observatory,L IG O极早期状态 earliest state角尺度干涉仪 Degree Angular Scale Interferometer, DASI角要素 angular element近地小行星跟踪 Near2Earth Asteroid Tracking, N EA T巨超新星 hypernova巨型拼合镜面望远镜 G iant Segmented Mirror Tele2 scope,GSM TK柯伊伯-埃奇沃思带 Kuiper2Edgeworth belt柯伊伯带天体 Kuiper Belt Object,K BOL类织女天体 Vega2like object类重力 paragravity冷原子钟 cold atom clock猎户FU型天体 FU Orionis object林肯近地空间研究 Lincoln Near2Earth Research, L IN EAR洛韦尔天文台近地小天体搜索 Lowell Observatory Near2Earth Object Search,LON EOSM拇指印星云 Thumbprint NebulaP盘吸积 disk accretion喷泉钟 fountain clock碰撞后并合天体 post2collision merger偏振测光仪 photopolarimeter 贫星区 star2poor regionQ奇异星 exotic star钱德拉塞卡北深空区 Chandra Deep Field North, CDFN钱德拉塞卡南深空区 Chandra Deep Field S outh, CDFS钱德拉塞卡深空区 Chandra Deep Field,CDF钱德拉塞卡天文台 Chandra Observatory钱德拉塞卡X射线天文台 Chandra X2ray Observato2 ry,CXO潜在威胁小行星 potentially hazardous asteroid,PHA 强磁星 magnetar2μm全天巡视 Two2Micron All Sky Survey,2MASS R日地关系天文台 S olar2Terrestrial Relation Observa2 tory,STEREOS少体问题 few2body problem射电褐矮星 radio brown dwarf 射电望远镜阵 Allen Telescope Array,A TA射电余辉 radio afterglow7发布试用新词X射线余辉 X2ray afterglow甚小质量恒星 very low2mass star舒梅克-列维彗星 comet Shoemaker2Levy疏散旋涡星系 open spiral 双行星 double2planetT太初黑洞 primordial black hole太阳系外类太阳风 exo2solar wind 特快自转 super2rotation天体演化学家 cosmogoner天卫十八 Prospero天卫十九 Setebos天卫二十 Stephano天文爱好者 stargazer天文政策 astropolitics W外星行星 exoplanet外星行星凌星 exoplanet transit 外星行星系 exoplanet system 外星人 alien creation望远镜观测时间 telescope time 微型类冥行星 plutino微型卫星 mini2moon,moonlet位力参数 virial parameter未名类太阳风 X wind未名物质 X matter无吸收空间 absorption2free space X下一代空间望远镜 Next G eneration S pace T elescope, NG ST星尘风 dust driven wind星像切割技术 imaging2chip technologyⅠ型类星体 TypeⅠquasarⅡ型类星体 TypeⅡquasar虚拟天文台 virtual observatory 虚影物质 shadow matterY亚恒星系 substellar system一平方公里天线阵 Square K ilometer Array,SK A 引力透镜深空巡天 Deep Lens Survey,DL S有生命行星 life2bearing planet宇宙物理学 cosmophysics宇宙物质 celestial matter宇宙灾变 cosmic catastrophe原恒星坍缩 protostellar collapse Z再电离时期 reionization era滞涨 expansion lag质量因子 mass factor中等质量黑洞 intermediate2mass black hole 中介天体 in2between object祝融型小行星 Vulcan2like asteroid,vulcanoid增容甚大天线阵 Expanded Very Large Array,EVLA 最内行星 innermost planet◇简讯 第三届地理学名词审定分委员会于2002年4月3~4日在京召开第二次审定工作会议。
英文期刊写作与投稿 JMST编辑部主任 罗东
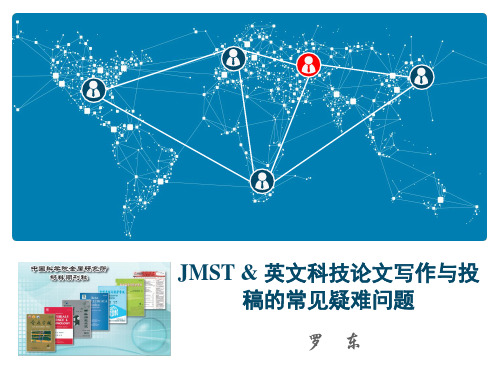
论文发表 ≠ 结束
为什么要关注论文的被引用情况?
1. 论文被广泛引用是该论文学术水平和影响力的完美体现。 2. 高被引论文将为下一篇(将来)论文的投稿与发表提供 助力。 3. 零引用(低引用)是编辑和出版商所不愿意看到的。
高被引论文是怎么炼成的?高被引论文不是梦
3. 一周当中哪天投稿?周三和周四是投稿的理想时间,其 次是周二;尽量不在周五~周一投稿。
4. 千万不要在临近毕业才投稿(具体需提前多长时间投稿, 要根据各大学不同的要求以及目标期刊的发表效率)。
如何正确回应评审意见?
理性看待评审意见: 评审意见未必正确; 退稿未必是质量差。
按照编辑和审稿人提 出的意见修改,并逐 条回复说明这些修改。
Prof. Zhongguang Wang Institute of Metal Research, CAS
期刊简介:历史大事记
1985年 创刊
1993年 变更刊名
EI收录
1995年被SCI收录 2010,同Elsevier合作
Байду номын сангаас 期刊简介:总体发展规划
“三步走”打造国际一流大材料学术期刊
I. 2011-2015
4. 准备评估自身论文的质量与水平。 5. 根据自己的需求选择合适的期刊完成投稿。
选择合适的投稿时间?(审稿效率&尺度)
1.选择合适的投稿时间只能对一般论文有帮助;高水平稿 件什么时间投稿或者投稿到哪里几乎没有任何影响。 2. 避开编辑所在地区的重大节假日,如中国的春节;欧美 的圣诞节;元旦(日本、韩国);大学编辑的暑假等假期。
首先:要看论文的性质,排除抄袭和剽窃等不端行为; 其次:要看投稿的时间以及工作的重要性。
国际权威物理学期刊影响因子排行

物理学期刊影响因子 SCI影响因子 2011年 - 大类:物理 SCI影响因子
ISSN 刊名简称 刊名全称 小类名称(中文) 小类名称(英文) 小类分区 大类名称 大类分区 06-08均影响因子 2011年影响因子 1951-6355 EUR PHYS J-SPEC TOP European Physical Journal-Special Topics 物理:综合 PHYSICS, MULTIDISCIPLINARY 4 物理 4 0.69 0.84
1895-1082 CENT EUR J PHYS CENTRAL EUROPEAN JOURNAL OF PHYSICS 物理:综合 PHYSICS, MULTIDISCIPLINARY 4 物理 4 0.60 0.69
1882-0778 APPL PHYS EXPRESS Applied Physics Express 物理:应用 PHYSICS, APPLIED 4 物理 4 0.00 2.75 1863-8880 LASER PHOTONICS REV Laser & Photonics Reviews 光学 OPTICS 1 物理 2 4.36 9.30 1863-8880 LASER PHOTONICS REV Laser & Photonics Reviews 物理:应用 PHYSICS, APPLIED 2 物理 2 4.36 9.30 1863-8880 LASER PHOTONICS REV Laser & Photonics Reviews 物理:凝聚态物理 PHYSICS, CONDENSED MATTER 2 物理 2 4.36 9.30 1862-6254 PHYS STATUS SOLIDI-R Physica Status Solidi-Rapid Research Letters 材料科学:综合 MATERIALS SCIENCE, MULTIDISCIPLINARY 3 物理 3 2.15 2.66 1862-6254 PHYS STATUS SOLIDI-R Physica Status Solidi-Rapid Research Letters 物理:凝聚态物理 PHYSICS, CONDENSED MATTER 3 物理 3 2.15 2.66 1862-6254 PHYS STATUS SOLIDI-R Physica Status Solidi-Rapid Research Letters 物理:应用 PHYSICS, APPLIED 3 物理 3 2.15 2.66 1815-2406 COMMUN COMPUT PHYS Communications in Computational Physics 物理:数学物理 PHYSICS, MATHEMATICAL 2 物理 3 1.98 1.84 1751-8113 J PHYS A-MATH THEOR Journal of Physics A-Mathematical and Theoretical 物理:综合 PHYSICS, MULTIDISCIPLINARY 3 物理 3 1.61 1.64 1751-8113 J PHYS A-MATH THEOR Journal of Physics A-Mathematical and Theoretical 物理:数学物理 PHYSICS, MATHEMATICAL 3 物理 3 1.61 1.64 1749-4885 NAT PHOTONICS Nature Photonics 光学 OPTICS 1 物理 1 24.98 26.44 1749-4885 NAT PHOTONICS Nature Photonics 物理:应用 PHYSICS, APPLIED 1 物理 1 24.98 26.44 1745-5030 WAVE RANDOM COMPLEX Waves in Random and Complex Media 物理:综合 PHYSICS, MULTIDISCIPLINARY 4 物理 4 1.15 0.68 1745-2473 NAT PHYS Nature Physics 物理:综合 PHYSICS, MULTIDISCIPLINARY 1 物理 1 14.51 18.42 1742-8297 INT J EXERGY International Journal of Exergy 能源与燃料 ENERGY & FUELS 4 物理 4 0.65 1.09 1742-8297 INT J EXERGY International Journal of Exergy 热力学 THERMODYNAMICS 4 物理 4 0.65 1.09 1742-5468 J STAT MECH-THEORY E JOURNAL OF STATISTICAL MECHANICS-THEORY AND EXPERIMENT 物理:数学物理 PHYSICS, MATHEMATICAL 2 物理 2 2.45 1.82
- 1、下载文档前请自行甄别文档内容的完整性,平台不提供额外的编辑、内容补充、找答案等附加服务。
- 2、"仅部分预览"的文档,不可在线预览部分如存在完整性等问题,可反馈申请退款(可完整预览的文档不适用该条件!)。
- 3、如文档侵犯您的权益,请联系客服反馈,我们会尽快为您处理(人工客服工作时间:9:00-18:30)。
Anders Johansen1 and Hubert Klahr Max-Planck-Institut f¨ ur Astronomie, K¨ onigstuhl 17, 69117 Heidelberg, Germany
arXiv:astro-ph/0501641v3 17 Aug 2005
johansen@mpia.de ABSTRACT We measure the turbulent diffusion coefficient of dust grains embedded in magnetorotational turbulence in a protoplanetary disc directly from numerical simulations and compare it to the turbulent viscosity of the flow. The simulations are done in a local coordinate frame comoving with the gas in Keplerian rotation. Periodic boundary conditions are used in all directions, and vertical gravity is not applied to the gas. Using a two-fluid approach, small dust grains of various sizes (with friction times up to Ω0 τf = 0.02) are allowed to move under the influence of friction with the turbulent gas. We measure the turbulent diffusion coefficient of the dust grains by applying an external sinusoidal force field acting in the vertical direction on the dust component only. This concentrates the dust around the mid-plane of the disc, and an equilibrium distribution of the dust density is achieved when the vertical settling is counteracted by the turbulent diffusion away from the mid-plane. Comparing with analytical expressions for the equilibrium concentration we deduce the vertical turbulent diffusion coefficient. The vertical diffusion coefficient is found to be lower than the turbulent viscosity and to have an associated vertical diffusion Prandtl number of about 1.5. A similar radial force field also allows us to measure the radial turbulent diffusion coefficient. We find a radial diffusion Prandtl number of about 0.85 and also find that the radial turbulent diffusion coefficient is around 70% higher than the vertical. As most angular momentum transport happens through magnetic Maxwell stresses, both the vertical and the radial diffusion coefficients are found to be significantly higher than suggested by the angular momentum transport by Reynolds stresses alone. We also find evidence for trapping of dust grains of intermediate friction time in turbulent eddies.
–3– and outer nebula. Bockel´ ee-Morvan et al. (2002) consider disc models where crystallization of silicates happens in the inner, hot parts of the disc. They calculate that in a few times 104 years the crystalline silicate fraction reaches a uniform value outside the crystallization region due to radial turbulent diffusion, and that the value can approach unity for realistic disc parameters. From high resolution observations of three protoplanetary discs, van Boekel et al. (2004) find that the inner 1-2 AU of these discs contain a higher crystalline silicate fraction than the outer 2-20 AU. This supports the theory that crystalline dust grains form in the hot inner disc and are subsequently transported to the outer disc by turbulent gas motion. The existence of chondrules (millimeter-sized solid inclusions found in primitive meteorites, see e.g. Norton 2002) is believed to be the result of collisions and coagulation of small dust grains (Blum & Wurm 2000). The size distribution of chondrules may be explained by selective sorting in the turbulent solar nebula (Cuzzi et al. 2001). The first step in planet formation is the build-up of kilometer-sized rocky and icy planetesimals (in the planetesimal hypothesis of Safronov 1969), either from sticking or due to a gravitational instability in the vertically settled dust layer. In the latter case, the equilibrium scale height of the dust layer is determined by the turbulent diffusion coefficient of the dust grains in the vertical direction (Cuzzi et al. 1993). An alternative planet formation hypothesis, the gravitational instability hypothesis (see Boss 2003, and references therein), states that planets form as a direct gravitational instability in the gas of a protoplanetary disc. The ability of a disc to undergo gravitational instability depends on its density and temperature structure, which is again dependent on the distribution and thus the turbulent transport of tiny dust grains. It is a modern paradigm of protoplanetary discs that shear instabilities in the gas flow lead to turbulence, which is again responsible for such diverse effects as heating, angular momentum transport and diffusion. The actual turbulence is often parametrized in a single parameter, the turbulent viscosity (which can be non-dimensionalized into the α-value of Shakura & Sunyaev 1973). This single parameter determines both heating, angular momentum transport and diffusion. Candidates for protoplanetary disc turbulence are many. Most pronounced linear instabilities are vertical convective instability (Lin & Papaloizou 1980) and the magnetorotational instability (Balbus & Hawley 1991), although the former has proved to lead to inward rather than outward transport of angular momentum (Ryu & Goodman 1992). Other instabilities have been proposed, such as the baroclinic instability of Klahr & Bodenheimer (2003) which must be non-linear according to Klahr (2004), a linear Rossby wave instability (Li et al. 2000) and a linear stratorotational instability (Dubrulle et al. 2005; Shalybkov & R¨ udiger 2005). Today’s most accepted source of turbulence is magnetorotational turbulence (MRI).