{0COPSTMBasedAutomobileInstrumentCluster}0{基于
无人驾驶汽车英语PPT课件

Urban transportation services
1 2 3
Robotaxi
Autonomous taxi service, providing efficient and safe point-to-point travel.
Autonomous Public Transport
It enables scheduled and scheduled public transportation services by driving buses or subways automatically.
• Millimeter wave radar: Utilizing electromagnetic waves in the millimeter wave band for detection, it can operate under harsh weather conditions and measure the speed and distance of objects.
01
solves the problem of "last mile" delivery in cities
through autonomous driving.
Autonomous Trucking
02
Automated long-distance freight transportation to
• Transfer learning: Transfer the knowledge learned in one task to other related tasks, accelerating model training and improving performance.
自动驾驶汽车路径跟踪控制
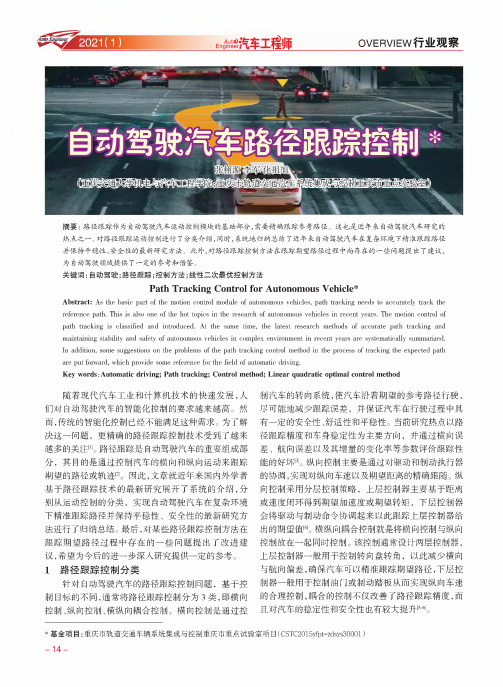
Auto.En 鑰汽车工程师OVERVIEW 行业观察«重庆交通大学机电与汽车工程学院重庆市轨道交通汽车系统集成与控制重庆市重点^室摘要:路径跟踪作为自动驾驶汽车运动控制模块的基础部分,需要精确跟踪参考路径。
这也是近年来自动驾驶汽车研究的热点之一。
对路径跟踪运动控制进行了分类介绍,同时,系统地归纳总结了近年来自动驾驶汽车在复杂环境下精准跟踪路径并保持平稳性、安全性的最新研究方法。
此外,对路径跟踪控制方法在跟踪期望路径过程中尚存在的一些问题提出了建议,为自动驾驶领域提供了一定的参考和借鉴。
关键词:自动驾驶;路径跟踪;控制方法;线性二次最优控制方法Path Tracking Control for Autonomous Vehicle **基金项目:重庆市轨道交通车辆系统集成与控制重庆市重点试验室项目(CSTC215yfpt-zdsys30001)Abstract : As the basic part of the motion control module of autonomous vehicles, path tracking needs to accurately track thereference path. This is also one of the hot topics in the research of autonomous vehicles in recent years. The motion control ofpath tracking is classified and introduced. At the same time, the latest research methods of accurate path tracking and maintaining stability and safety of autonomous vehicles in complex environment in recent years are systematically summarized. In addition, some suggestions on the problems of the path tracking control method in the process of tracking the expected pathare put forward, which provide some reference for the field of automatic driving.Key words : Automatic driving; Path tracking; Control method; Linear quadratic optimal control method随着现代汽车工业和计算机技术的快速发展,人 们对自动驾驶汽车的智能化控制的要求越来越高。
基于边缘检测的抗遮挡相关滤波跟踪算法

基于边缘检测的抗遮挡相关滤波跟踪算法唐艺北方工业大学 北京 100144摘要:无人机跟踪目标因其便利性得到越来越多的关注。
基于相关滤波算法利用边缘检测优化样本质量,并在边缘检测打分环节加入平滑约束项,增加了候选框包含目标的准确度,达到降低计算复杂度、提高跟踪鲁棒性的效果。
利用自适应多特征融合增强特征表达能力,提高目标跟踪精准度。
引入遮挡判断机制和自适应更新学习率,减少遮挡对滤波模板的影响,提高目标跟踪成功率。
通过在OTB-2015和UAV123数据集上的实验进行定性定量的评估,论证了所研究算法相较于其他跟踪算法具有一定的优越性。
关键词:无人机 目标追踪 相关滤波 多特征融合 边缘检测中图分类号:TN713;TP391.41;TG441.7文献标识码:A 文章编号:1672-3791(2024)05-0057-04 The Anti-Occlusion Correlation Filtering Tracking AlgorithmBased on Edge DetectionTANG YiNorth China University of Technology, Beijing, 100144 ChinaAbstract: For its convenience, tracking targets with unmanned aerial vehicles is getting more and more attention. Based on the correlation filtering algorithm, the quality of samples is optimized by edge detection, and smoothing constraints are added to the edge detection scoring link, which increases the accuracy of targets included in candi⁃date boxes, and achieves the effects of reducing computational complexity and improving tracking robustness. Adap⁃tive multi-feature fusion is used to enhance the feature expression capability, which improves the accuracy of target tracking. The occlusion detection mechanism and the adaptive updating learning rate are introduced to reduce the impact of occlusion on filtering templates, which improves the success rate of target tracking. Qualitative evaluation and quantitative evaluation are conducted through experiments on OTB-2015 and UAV123 datasets, which dem⁃onstrates the superiority of the studied algorithm over other tracking algorithms.Key Words: Unmanned aerial vehicle; Target tracking; Correlation filtering; Multi-feature fusion; Edge detection近年来,无人机成为热点话题,具有不同用途的无人机频繁出现在大众视野。
汽车发动机外文文献翻译中英文参考
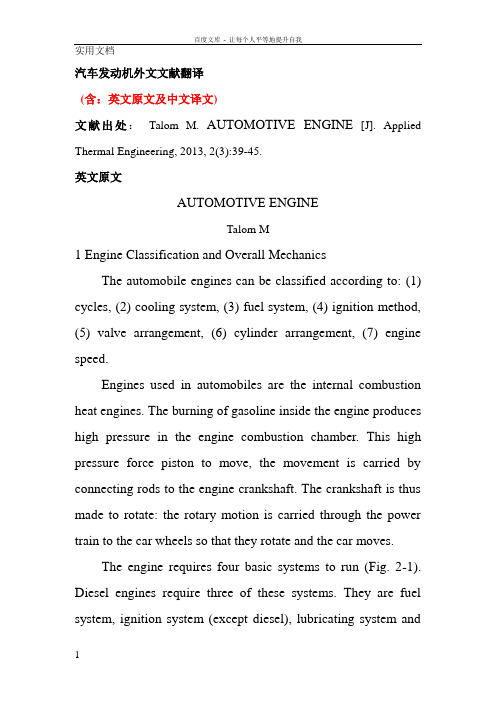
汽车发动机外文文献翻译(含:英文原文及中文译文)文献出处:Talom M. AUTOMOTIVE ENGINE[J]. Applied Thermal Engineering, 2013, 2(3):39-45.英文原文AUTOMOTIVE ENGINETalom M1 Engine Classification and Overall MechanicsThe automobile engines can be classified according to: (1) cycles, (2) cooling system, (3) fuel system, (4) ignition method, (5) valve arrangement, (6) cylinder arrangement, (7) engine speed.Engines used in automobiles are the internal combustion heat engines. The burning of gasoline inside the engine produces high pressure in the engine combustion chamber. This high pressure force piston to move, the movement is carried by connecting rods to the engine crankshaft. The crankshaft is thus made to rotate: the rotary motion is carried through the power train to the car wheels so that they rotate and the car moves.The engine requires four basic systems to run (Fig. 2-1). Diesel engines require three of these systems. They are fuel system, ignition system (except diesel), lubricating system andcooling system. However, three other related systems are also necessary. These are the exhaust system, the emission-control system, and the starting system. Each performs a basic job in making the engine run.2 Engine Operating PrinciplesThe term “stroke” is used to desc ribe the movement of the piston within the cylinder. The movement of the piston from its uppermost position (TDC, top dead center) to its lowest position (BDC, bottom dead center) is called a stroke. The operating cycle may require either two or four strokes to complete. Most automobile engines operate on the four stroke cycle.In four-stroke engine, four strokes of the piston in the cylinder are required to complete one full operating cycle. Each stroke is named after the action. It performs intake, compression, power, and exhaust in that order.The intake strokeThe intake stroke begins with the piston near the top of its travel. As the piston begins its descent, the exhaust valve closes fully, the intake valve opens and the volume of the combustion chamber begins to increase, creating a vacuum. As the piston descends, an air/fuel mixture is drawn from the carburetor into the cylinder through the intake manifold. The intake stroke endswith the intake valve close just after the piston has begun its upstroke.Compression strokeAs the piston is moved up by the crankshaft from BDC, the intake valve closes. The air/fuel mixture is trapped in the cylinder above the piston. Future piston travel compresses the air/fuel mixture to approximately one-eighth of its original volume (approximately 8:1 compression ratio) when the piston has reached TDC. This completes the compression stroke. Power strokeAs the piston reaches TDC on the compression stroke, an electric spark is produced at the spark plug. The ignition system delivers a high-voltage surge of electricity to the spark plug to produce the spark. The spark ignites, or sets fire to, the air/fuel mixture. It now begins to burn very rapidly, and the cylinder pressure increases to as much as 3-5MPa or even more. This terrific push against the piston forces it downward, and a powerful impulse is transmitted through the connecting rod to the crankpin on the crankshaft. The crankshaft is rotated as the piston is pushed down by the pressure above it.Exhaust strokeAt the end of the power stroke the camshaft opens theexhaust valve, and the exhaust stroke begins. Remaining pressure in the cylinder, and upward movement of the piston, force the exhaust gases out of the cylinder. At the end of the exhaust stroke, the exhaust valve closes and the intake valve opens, repeating the entire cycle of events over and over again.3 Engine Block and Cylinder HeadEngine BlockThe engine block is the basic frame of the engine. All other engine parts either fit inside it or fasten to it. It holds the cylinders, water jackets and oil galleries (Fig. 2-4). The engine block also holds the crankshaft, which fastens to the bottom of the block. The camshaft also fits in the block, except on overhead-cam engines. In most cars, this block is made of gray iron, or an alloy (mixture) of gray iron and other metals, such as nickel or chromium. Engine blocks are castings.Some engine blocks, especially those in smaller cars, are made of cast aluminum. This metal is much lighter than iron. However, iron wears better than aluminum. Therefore, the cylinders in most aluminum engines are lined with iron or steel sleeves. These sleeves are called cylinder sleeves. Some engine blocks are made entirely of aluminum.Cylinder SleevesCylinder sleeves are used in engine blocks to provide a hard wearing material for pistons and piston rings. The block can be made of one kind of iron that is light and easy to cast while the sleeves uses another that is better able to stand up wear and tear.There are two main types of sleeves: dry and wet (Fig. 2-5).Dry sleeve Wet sleeveCylinder HeadThe cylinder head fastens to the top of the block, just as a roof fits over a house. The underside forms the combustion chamber with the top of the piston. In-line engine of light vehicles have just one cylinder head for all cylinders; larger in-line engines can have two or more. Just as with engine blocks, cylinder heads can be made of cast iron or aluminum alloy. The cylinder head carries the valves, valve springs and the rockers on the rocker shaft, this part of valve gear being worked by the pushrods. Sometimes the camshaft is fitted directly into the cylinder head and operates on the valves without rockers. This is called an overhead camshaft arrangement.GasketThe cylinder head is attached to the block with high-tensile steel studs. The joint between the block and the head must begas-tight so that none of the burning mixture can escape. This is achieved by using cylinder head gasket. Gaskets are also used to seal joins between the other parts, such as between the oil pan, manifolds, or water pump and the blocks.Oil PanThe oil pan is usually formed of pressed steel. The oil pan and the lower part of cylinder block together are called the crankcase; they enclose, or encase, the crankshaft. The oil pump in the lubricating system draws oil from the oil pan and sends it to all working parts in the engine. The oil drains off and run down into the pan. Thus, there is a constant circulation of oil between the pan and the working parts of the engine.4 Piston Assembly, piston rings, The piston pin ,Connecting Rods, Crankshafts And FlywheelPistonPiston rings and the piston pin are together called the piston assembly.The piston is an important part of a four-stroke cycle engine. Most pistons are made from cast aluminum. The piston, through the connecting rod, transfers to the crankshaft the force created by the burning fuel mixture. This force turns the crankshaft.To withstand the heat of the combustion chamber, the piston must be strong. It also must be light, since it travels at high speeds as it moves up and down inside the cylinder. The piston is hollow. It is thick at the top where it takes the brunt of the heat and the expansion force. It is thin at the bottom, where there is less heat. The top part of the piston is the head, or crown. The thin part is the skirt. Most pistons have three ring grooves at the top. The sections between the ring grooves are called ring lands.piston ringsPiston rings fit into ring grooves near the top of the piston. In simplest terms, piston rings are thin, circular pieces of metal that fit into grooves in the tops of the pistons. In modern engines, each piston has three rings. (Piston in older engines sometimes had four rings, or even five.) The inside surface of the ring fits in the groove on the piston. The ring's outside surface presses against the cylinder walls. Rings provide the needed seal between the piston and the cylinder walls. That is, only the rings contact the cylinder walls. The top two rings are to keep the gases in the cylinder and are called compression rings. The lower one prevents the oil splashed onto the cylinder bore fro m entering the combustion chamber, and is called an oil ring.The piston pinThe piston pin holds together the piston and the connecting rod. This pin fits into th e piston pin holes and into a hole in the top end of the connecting rod. The top end of t he rod is much smaller than the end that fits on the crankshaft. This small end fits inside the bottom of the piston. The piston pin fits through one side of the piston, through the small end of the rod, and then through the other side of the piston. It holds the rod firmly in place in the center of the piston. Pins are made of high-strength steel and have a hollow center. Many pins are chrome-plated to help them wear better. A piston pin fits into a round hole in the piston. The piston pin joins the piston to the connecting rod. The thick part of the piston that holds the piston pin is the pin boss. Connecting RodsThe connecting rod little end is connected to the piston pin.A bush made from a soft metal, such as bronze, is used for this joint. The lower end of the connecting rod f its the crankshaft journal. This is called the big end. For this big-end bearing, steel-backed lead or tin shell bearings are used. These are the same as those used for the main bearings. The split of the big end is sometimes at an angle, so that it is small enough t o be withdrawn through the cylinder bore. The connecting rod ismade from forged alloy steel.CrankshaftsThe crankshaft is regarded as the “backbone” of the engine (Fig. 2-7). The crankshaft, in conjunction with the connecting rod, converts the reciprocating motion of the piston to the rotary motion needed to drive the vehicle. It is usually made from car-bon steel which is alloyed with a small proportion of nickel. The main bearing journals fit into the cylinder block and the big end journals align with the connecting rods. At the rear end of the crankshaft is attached the flywheel, and at the front end are the driving wheels for the timing gears, fan, cooling water and alternator. The throw of the crankshaft, . the distance between the main journal and the big end centers, controls the length of the stroke. The stroke is double the throw, and the stroke length is the distance that the piston travels from TDC to BDC and vice versa.中文译文汽车发动机Talom M1发动机分类和一般力学(1)循环,(2)冷却系统,(3)燃料系统,(4)点火方法,(5)阀门布置,(6)气缸布置,(7)发动机速度。
Robust Control

Robust ControlRobust control is a crucial aspect of engineering and technology that focuses on designing systems that can perform effectively in the presence of uncertainties and variations. It is essential for ensuring stability and performance in a wide range of applications, from aerospace and automotive systems to industrial processes and robotics. Robust control techniques aim to account for uncertainties in the system model, disturbances, and variations in operating conditions, to ensure that the system can still meet its performance requirements. One of thekey challenges in robust control is dealing with uncertainties in the system model. In many real-world applications, it is difficult to obtain an accurate model ofthe system dynamics, and there may be uncertainties in parameters or disturbances that affect the system's behavior. Robust control techniques address thischallenge by designing controllers that can guarantee stability and performance even in the presence of these uncertainties. This is typically done by formulating the control problem as an optimization problem, where the goal is to find a controller that minimizes the impact of uncertainties on the system's performance. Another important aspect of robust control is ensuring stability of the system. Stability is a fundamental requirement for any control system, as an unstable system can lead to catastrophic failures and safety hazards. Robust control techniques often involve analyzing the system's stability properties and designing controllers that can ensure stability under a wide range of operating conditions. This may involve using robust stability analysis tools, such as the small gain theorem or the circle criterion, to assess the system's stability margins and ensure that the controller can stabilize the system in the presence of uncertainties. In addition to stability, robust control also aims to achieve good performance in terms of tracking accuracy, disturbance rejection, and robustnessto variations in operating conditions. Performance requirements can vary depending on the specific application, but common objectives include minimizing tracking errors, reducing the impact of disturbances, and ensuring that the system can maintain its performance even in the presence of variations in parameters or operating conditions. Robust control techniques often involve designingcontrollers that can achieve these performance objectives while also ensuringstability and robustness. Robust control techniques have been widely used in a variety of applications, ranging from aerospace and automotive systems toindustrial processes and robotics. In aerospace applications, for example, robust control is essential for ensuring the stability and performance of aircraft and spacecraft in the presence of uncertainties in aerodynamic forces, engine dynamics, and environmental conditions. In automotive systems, robust control is used to design controllers for vehicle stability control, adaptive cruise control, andanti-lock braking systems, to ensure safe and reliable operation under varyingroad conditions. Overall, robust control plays a critical role in ensuring the stability, performance, and safety of complex engineering systems. By accountingfor uncertainties and variations in system dynamics, robust control techniques enable engineers to design controllers that can effectively regulate the system's behavior and meet its performance requirements. As technology continues to advance and systems become more complex, robust control will remain a key area of research and development, helping to drive innovation and progress in a wide range of industries.。
基于无模型自适应控制的无人驾驶汽车横向控制方法
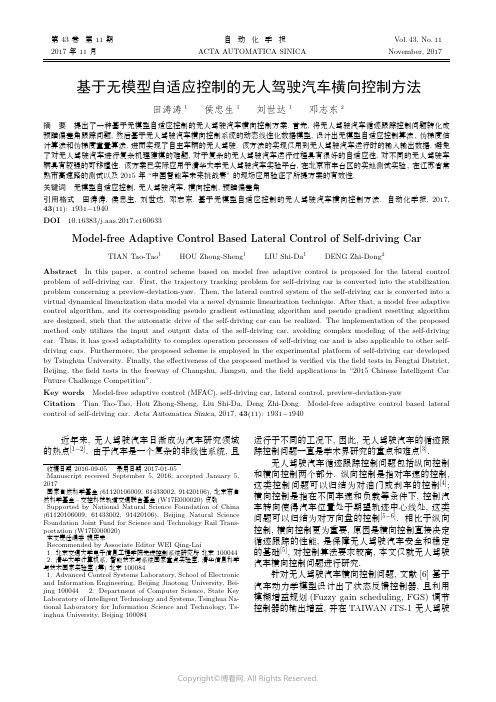
第43卷第11期自动化学报Vol.43,No.11 2017年11月ACTA AUTOMATICA SINICA November,2017基于无模型自适应控制的无人驾驶汽车横向控制方法田涛涛1侯忠生1刘世达1邓志东2摘要提出了一种基于无模型自适应控制的无人驾驶汽车横向控制方案.首先,将无人驾驶汽车循迹跟踪控制问题转化成预瞄偏差角跟踪问题,然后基于无人驾驶汽车横向控制系统的动态线性化数据模型,设计出无模型自适应控制算法、伪梯度估计算法和伪梯度重置算法,进而实现了自主车辆的无人驾驶.该方法的实现仅用到无人驾驶汽车运行时的输入输出数据,避免了对无人驾驶汽车进行复杂机理建模的难题,对于复杂的无人驾驶汽车运行过程具有很好的自适应性,对不同的无人驾驶车辆具有较强的可移植性.该方案已实际应用于清华大学无人驾驶汽车实验平台,在北京市丰台区的实地测试实验、在江苏省常熟市高速路的测试以及2015年“中国智能车未来挑战赛”的现场应用验证了所提方案的有效性.关键词无模型自适应控制,无人驾驶汽车,横向控制,预瞄偏差角引用格式田涛涛,侯忠生,刘世达,邓志东.基于无模型自适应控制的无人驾驶汽车横向控制方法.自动化学报,2017, 43(11):1931−1940DOI10.16383/j.aas.2017.c160633Model-free Adaptive Control Based Lateral Control of Self-driving Car TIAN Tao-Tao1HOU Zhong-Sheng1LIU Shi-Da1DENG Zhi-Dong2Abstract In this paper,a control scheme based on model free adaptive control is proposed for the lateral control problem of self-driving car.First,the trajectory tracking problem for self-driving car is converted into the stabilization problem concerning a preview-deviation-yaw.Then,the lateral control system of the self-driving car is converted into a virtual dynamical linearization data model via a novel dynamic linearization technique.After that,a model free adaptive control algorithm,and its corresponding pseudo gradient estimating algorithm and pseudo gradient resetting algorithm are designed,such that the automatic drive of the self-driving car can be realized.The implementation of the proposed method only utilizes the input and output data of the self-driving car,avoiding complex modeling of the self-driving car.Thus,it has good adaptability to complex operation processes of self-driving car and is also applicable to other self-driving cars.Furthermore,the proposed scheme is employed in the experimental platform of self-driving car developed by Tsinghua University.Finally,the effectiveness of the proposed method is verified via thefield tests in Fengtai District, Beijing,thefield tests in the freeway of Changshu,Jiangsu,and thefield applications in“2015Chinese Intelligent Car Future Challenge Competition”.Key words Model-free adaptive control(MFAC),self-driving car,lateral control,preview-deviation-yawCitation Tian Tao-Tao,Hou Zhong-Sheng,Liu Shi-Da,Deng Zhi-Dong.Model-free adaptive control based lateral control of self-driving car.Acta Automatica Sinica,2017,43(11):1931−1940近年来,无人驾驶汽车日渐成为汽车研究领域的热点[1−2].由于汽车是一个复杂的非线性系统,且收稿日期2016-09-05录用日期2017-01-05Manuscript received September5,2016;accepted January5, 2017国家自然科学基金(61120106009,61433002,91420106),北京市自然科学基金–交控科技轨道交通联合基金(W17E000020)资助Supported by National Natural Science Foundation of China (61120106009,61433002,91420106),Beijing Natural Science Foundation Joint Fund for Science and Technology Rail Trans-portation(W17E000020)本文责任编委魏庆来Recommended by Associate Editor WEI Qing-Lai1.北京交通大学电子信息工程学院先进控制系统研究所北京1000442.清华大学计算机系,智能技术与系统国家重点实验室,清华信息科学与技术国家实验室(筹)北京1000841.Advanced Control Systems Laboratory,School of Electronic and Information Engineering,Beijing Jiaotong University,Bei-jing1000442.Department of Computer Science,State Key Laboratory of Intelligent Technology and Systems,Tsinghua Na-tional Laboratory for Information Science and Technology,Ts-inghua University,Beijing100084运行于不同的工况下,因此,无人驾驶汽车的循迹跟踪控制问题一直是学术界研究的重点和难点[3].无人驾驶汽车循迹跟踪控制问题包括纵向控制和横向控制两个部分.纵向控制是指对车速的控制,这类控制问题可以归结为对油门或刹车的控制[4];横向控制是指在不同车速和负载等条件下,控制汽车转向使得汽车位置处于期望轨迹中心线处,这类问题可以归结为对方向盘的控制[5−6].相比于纵向控制,横向控制更为重要,原因是横向控制直接决定循迹跟踪的性能,是保障无人驾驶汽车安全和稳定的基础[5],对控制算法要求较高,本文仅就无人驾驶汽车横向控制问题进行研究.针对无人驾驶汽车横向控制问题,文献[6]基于汽车动力学模型设计出了状态反馈控制器,且利用模糊增益规划(Fuzzy gain scheduling,FGS)调节控制器的输出增益,并在TAIWAN i TS-1无人驾驶1932自动化学报43卷汽车平台上进行了验证;文献[7]利用模型预测控制(Model predictive control,MPC)分别就汽车非线性模型和汽车时变线性模型设计了两类控制器,并给出了仿真分析和真车实验结果;文献[8]基于汽车运动学模型设计了参数随车速变化的比例积分微分(Proportional-integral-derivative,PID)控制器,并将该算法应用于Babieca原型车中;文献[9]利用PID控制器作内环较低精度控制器,外环利用模糊控制器做高精度控制,在实际的道路环境中进行了尝试.然而,上述基于模型的方法需要满足相应的假设条件.例如,侧向加速度小于0.4g、车辆的纵向速度为常数、前轮转角直接作为输入等[6],这些假设在实际的无人驾驶汽车运行过程中很难被满足.另外,从理论上分析,除了车辆本身的动力学非线性外,车辆动力学行为与运行速度、道路以及其他天气等因素密切相关,欲想全部精确建立汽车以及环境的动力学模型,然后进行控制系统设计,显然不是可行的方法[5].而采用模糊控制方法,其隶属度函数的确定和专家经验都很难遍历所有工况下的情况,难度大,可应用性差[9].在汽车运行的过程中,会产生大量的输入输出(Input/output,I/O)数据,这些I/O数据包含了车辆的动力学信息,利用数据驱动的方式对无人驾驶汽车进行控制,是目前一个新颖的方法.在数据驱动控制方面,近些年一些典型的数据驱动控制方法相继提出并得到广泛的研究与应用,包括虚拟参考反馈整定[10−11]、自适应动态规划[12−16]、无模型自适应控制[16−17]等.无模型自适应控制(Model-free adaptive con-trol,MFAC)是一种典型的在线数据驱动控制方法[16,18],其原理是在闭环系统的每一个动态工作点处建立一个等价的动态线性化数据模型,然后基于此等价的虚拟数据模型设计控制器,进而实现非线性系统的自适应控制[18].截至目前,MFAC已经得到了广泛的应用,包括广域电力系统[19]、网络延时与丢包问题[20]、多自由度机器人外骨骼控制[21]、交直流微电网[22]以及自动泊车系统[23]等.理论和实践表明,MFAC结构简单、鲁棒性强、便于工程实现,而且MFAC不使用模型的信息,上述由于模型假设带来的不匹配问题可以有效地被避免.本文提出了基于MFAC的无人驾驶汽车横向控制方案,首先将横向控制问题转化为预瞄偏差角(汽车当前位置点和预瞄点的连线与汽车运行方向延长线形成的夹角)跟踪问题,然后利用动态线性化技术将预瞄偏差角跟踪系统转化为等价的偏格式数据模型,进而设计出无模型自适应控制算法、伪梯度估计算法和伪梯度重置算法.该方案仅利用无人驾驶汽车运行过程中的I/O数据,不包含车辆的模型信息,对于不同的车辆具有很强的可移植性.本文方案已经应用于清华大学“睿龙”号无人驾驶汽车平台,北京市丰台区的实地测试以及江苏省常熟市高速环线的实验结果表明,MFAC对于无人驾驶汽车横向控制问题具有很明显的优势.“睿龙”号无人驾驶汽车应用MFAC预瞄偏差角跟踪方案参加了2015年第七届中国智能车未来挑战赛,其控制算法在比赛全程中没有出现任何问题.本文结构安排如下:第1节对无人驾驶汽车控制问题进行描述,第2节提出基于预瞄偏差角跟踪系统的无人驾驶汽车横向控制方案,第3节针对预瞄偏差角设计MFAC控制器,第4节给出在实际的无人驾驶汽车平台和实际道路上的实验结果,第5节得出结论.1无人驾驶控制问题描述1.1无人驾驶汽车平台简介清华大学“睿龙”号无人驾驶汽车平台以及汽车各个设备的分布情况如图1所示.该无人驾驶汽车平台由长安CS35自动挡汽车改装而成,保留原车中车载汽车状态传感器,并外接无人驾驶必需的定位定姿和环境感知传感器,包括GPS天线及接收机、摄像头、64线激光雷达、4线激光雷达以及毫米波雷达等,这些传感器相当于无人驾驶汽车的“眼睛”;汽车后备箱处放置主控计算机以实现数据采集、分析以及对汽车进行闭环控制等工作,相当于无人驾驶汽车的“大脑”;汽车的执行机构(油门、刹车和转向)通过CAN总线与主控计算机通信,执行机构相当于无人驾驶汽车的“四肢”,CAN总线和千兆以太网通讯相当于无人驾驶汽车的“神经连接”.其中,无人驾驶汽车的定位定姿与环境感知采用多传感器信息融合技术,例如利用差分GPS与惯性测量单元、里程计等多传感信息融合实现汽车位姿信息的获取[24];利用64线激光雷达(HDL)实现路缘的检测[25];利用黑白摄像头进行路面车道线图像信息的提取[6,26],利用彩色摄像头进行红绿灯以及交通标志的识别[27]等.定位定姿和环境感知设备的作用如表1所示.图1车载传感器分布图Fig.1Vehicle sensors distribution11期田涛涛等:基于无模型自适应控制的无人驾驶汽车横向控制方法1933表1定位定姿和环境感知设备的作用Table1Navigation and environmental perceptionequipments常用设备作用64线激光雷达感知周边环境信息4线激光雷达探测障碍物GPS天线定位以获取当前汽车位置信息黑白摄像头检测车道线彩色摄像头获取路口红绿灯、交通标志信息毫米波雷达检测汽车前方障碍物信息其他车载传感器检测汽车速度、汽车加速度等1.2无人驾驶汽车的控制结构无人驾驶控制问题的横向控制和纵向控制的控制结构如图2所示.图2控制系统结构图Fig.2Control system structure在无人驾驶汽车运行过程中,车载传感器不断将车辆状态信息数据传输给主控计算机,主控计算机计算出车辆的期望速度和期望位姿,传感器同时提供车辆当前速度以及当前位姿的信息.根据车辆的期望速度和当前速度可以计算出油门和刹车的控制量,即纵向控制;根据当前车辆的位姿和期望的位姿可以计算出方向盘转角的控制量,即横向控制.算出的控制量通过CAN总线驱动相应的执行机构,以便达到循迹跟踪的目的.纵向控制关注速度,而横向控制关注方向,在变化的车速、变化的负载以及变化的路况下控制汽车方向盘使得汽车跟踪上期望轨迹,横向控制直接决定了无人驾驶汽车循迹跟踪的性能.2基于预瞄偏差角跟踪的无人驾驶汽车横向控制方案2.1基本概念当汽车的运行速度较快时(例如在高速路上行驶时),司机会盯着前方较远的一个点;当汽车的速度较慢时(例如即将转弯时),则会盯着前面较近的一个点.无人驾驶参考这样的过程,引入了预瞄点和预瞄距离的概念[8],如图3所示.图3预瞄点与预瞄距离示意图Fig.3Preview point and preview distance profile预瞄点指的是在期望轨迹上、汽车前方某一距离的点,这段距离称为预瞄距离.需要注意的是预瞄距离是期望轨迹上距离汽车当前点最近的点与预瞄点的弧长距离,并不是预瞄点和汽车当前点连线的长度.预瞄距离与汽车运行速度之间的关系可以用经验公式(1)表示[8]l(v)=l min,v≤v minav+l min,v min<v≤v maxl max,v>v max(1)其中,l是预瞄距离(m),v是当前汽车的速度(m/s),lmin是最小预瞄距离(m),lmax是最大的预瞄距离(m),a是常数.这里存在最大的预瞄距离,是汽车设备感知能力具有限制的缘故,而存在最小的预瞄距离,则是为了在汽车速度为零时保证预瞄点在汽车前方位置处(汽车自身具有一定长度,而汽车当前点的选取在汽车中心处).在实际应用中,基于预瞄的控制策略多被采用.然而因为预瞄点和预瞄距离的参与,对无人驾驶汽车循迹跟踪问题进行数学建模的难度会增大,这是由于车辆模型和预瞄控制策略需要同时被考虑进建模过程中.无人驾驶汽车在驾驶过程中一些常用的概念如图4(a)所示,相关的符号含义见表2.图4横向控制问题示意图Fig.4Schematic diagram of lateral control problem1934自动化学报43卷表2常用概念符号意义Table2The meaning of common concepts and symbols图示符号意义O,E,N坐标原点、正东方、正北方α汽车航向角(汽车运行方向与正北方向夹角)β预瞄点航向角(预瞄点运动方向与正北方向夹角)l预瞄距离len当前点和预瞄点之间的距离LD汽车预瞄点与汽车运行方向延长线的距离AD预瞄点航向角与汽车航向角的差X汽车当前点与预瞄点运行方向延长线的距离θ当前点与预瞄点连线和汽车运行方向形成的角度连接预瞄点与当前位置点,假设汽车的运动方向与这条连线的夹角为θ,定义该夹角为预瞄偏差角(Preview-deviation-yaw)[28],规定当预瞄点在汽车运行方向右前侧时的预瞄偏差角为正.2.2预瞄偏差角跟踪方案由图4(a)可知,当LD→0且AD→0时,无人驾驶汽车便能跟踪上期望轨迹.由图4(a)中几何关系可知θ=arctan Xl−AD(2)当θ→0时,由式(2)可知汽车的运行情况如图4(b)所示,由于汽车朝向预瞄点的方向运动,所以有X(k+1)<X(k),所以序列{X(k)|X(k)≥0, k∈N+}的极限为0,即X(k)→0,又由于预瞄距离l(k)=0,故有arctan(X/l)→0,则由式(2)可知AD→0.另一方面,由图4(a)可知sinθ=LDlen(3)当θ→0时,由于两点距离len=0,所以有LD→0.通过分析可知,控制θ使之趋于0为无人驾驶汽车循迹跟踪问题提供了解决思路.为了便于工程实现,对θ跟踪系统的描述应采用离散化的表达形式.令θ(k)表示当前时刻k 的预瞄偏差角,u(k)表示当前时刻k的方向盘转角.由于无人驾驶汽车横向控制系统的非线性[7],所以θ(k)和u(k)之间的关系也是非线性的.对于离散时间非线性系统的一般描述,可以采用带外部输入的非线性自回归滑动平均(Nonlinear auto-regressive moving average with exogenous input, NARMAX)模型[29−31]来表示,其表达为θ(k+1)=f(θ(k),···,θ(k−kθ),u(k),···,u(k−k u))(4)其中,f(·):R kθ+k u+2→R是一个未知的非线性函数,kθ和k u分别是两个未知的的参数.下文称式(4)表示的系统为预瞄偏差角跟踪系统.对于无人驾驶横向控制问题,就是求取合适的u(k),使得θ(k)能够尽快、无超调地跟踪到0.预瞄偏差角的跟踪情况与汽车运行情况的对应关系如图5所示.图5预瞄偏差角跟踪情况与汽车运行情况图Fig.5Preview-deviation-yaw tracking condition and self-driving car operation condition由图5可以看出,对于预瞄偏差角跟踪系统的控制,控制算法的设计至关重要.3预瞄偏差角跟踪系统控制器设计3.1问题描述对于预瞄偏差角跟踪系统,利用数学模型设计横向控制器的思路会遇到很大的阻碍,这是因为汽车系统的数学模型难以建立,循迹跟踪问题也很难用数学表达式精确描述,即式(4)中的f(·)很难有11期田涛涛等:基于无模型自适应控制的无人驾驶汽车横向控制方法1935精确数学表达式.预瞄偏差角跟踪系统的阶数也是不确定的,即式(4)中的kθ和k u是不确定的.由此可知,预瞄偏差角跟踪系统是一个难建模、复杂的非线性系统,其固有的关于控制输入的非线性的特点使得这类系统的控制器设计变得非常困难,利用线性化方法将此类系统转化到线性系统的框架中进行研究是处理此类一般非线性系统的常用做法[18].本文采用无模型自适应控制算法(MFAC)作为无人驾驶汽车横向控制算法,参考文献[18],可以将预瞄偏差角系统(4)等价转化为基于I/O增量式的动态线性化数据模型,并基于此数据模型设计控制器.3.2MF AC动态线性化对于预瞄偏差角跟踪系统(4),定义U L(k)∈R L为一个滑动时间窗口[k−L+1,k]内所有控制输入信号组成的向量,如式(5).U L(k)=[u(k),···,u(k−L+1)]T(5)且满足当k≤0时,有U L(k)=0L,其中L为控制输入线性化长度参数;0L是维度为L的零向量.对于预瞄偏差角跟踪系统,很容易满足下面的假设:假设1.系统(4)输入输出可测可控.即对于一致有界的期望输出信号θ∗(k),存在一致有界可行的输入信号u(k),使得在该输入信号驱动下,系统的输出θ(k)趋于期望信号θ∗(k).假设2.f(·)关于第(kθ+2)个变量到第(kθ+L+1)个变量分别存在连续偏导数.假设3.系统(4)是广义Lipschitz的,即满足对任意的k1=k2,k1,k2≥0以及U L(k1)= U L(k2),有|θ(k1+1)−θ(k2+1)|≤b U L(k1)−U L(k2) (6)其中,θ(k i+1)=f(θ(k i),···,θ(k i−kθ),u(k i),···, u(k i−k u)),i=1,2,b>0是一个常数.记∆U L(k)=U L(k)−U L(k−1),可以给出如下引理.引理1[18].若非线性系统(4)满足假设1∼3,给定L,那么当 ∆U L(k) =0时,一定存在一个称为伪梯度(Pseudo gradient,PG)的时变参数向量φp,L(k)∈R L,使得系统(4)可以转化为如下数据模型∆θ(k+1)=φTp,L(k)∆U L(k)(7)且对于任意时刻k,φp,L(k)=[φ1(k),···,φL(k)]T 是有界的.这种动态线性化称为基于偏格式的动态线性化(Partial form dynamic linearization, PFDL).其中,φp,L(k)下标中的p即PFDL首字母,L表示向量的长度.根据引理1,无人驾驶汽车预瞄偏差角跟踪系统动态线性化模型可以表示为θ(k+1)=θ(k)+φTp,L(k)∆U L(k)(8) 3.3控制器设计考虑如下的控制输入准则函数J(u(k))=|θ∗(k+1)−θ(k+1)|2+λ|u(k)−u(k−1)|2(9)其中,第1项的引入是为了使得系统的输出与期望输出一致;第2项是为了使方向盘不产生突变,柔化执行器的处理过程,λ>0是一个权重因子.将式(8)代入准则函数(9)中,对u(k)求导,并令其等于零,得u(k)=u(k−1)+ρ1φ1(k)(θ∗(k+1)−θ(k))λ+|φ1(k)|2−φ1(k)Li=2ρiφi(k)∆u(k−i+1)λ+|φ1(k)|2(10)其中,φi(k)是时变参数向量φp,L(k)的第i个元素,步长因子ρi∈(0,1],i=1,2,···,L的引入是为了使控制算法设计具有更大的灵活性.由式(10)可知,为实现控制算法,需要知道PG 的值,然而由于预瞄偏差角跟踪系统的模型未知,且PG是时变参数,其精确的真实值很难获取,需要利用系统输入输出数据对其进行估计.考虑如下的PG估计准则函数J(φp,L(k))=|θ(k)−θ(k−1)−φTp,L(k)∆U L(k−1)|2+µ φp,L(k)−ˆφp,L(k−1) 2(11)其中,µ>0是权重因子.对式(11)关于φp,L(k)求极值,得到PG的估计算法为ˆφp,L(k)=ˆφp,L(k−1)+η∆U L(k−1)(θ(k)−θ(k−1))µ+ ∆U L(k−1)||2−η∆U L(k−1)(ˆφTp,L(k−1)∆U L(k−1))µ+ ∆U L(k−1)||2(12)其中,步长因子η∈(0,2]是为了使控制算法设计具有更大的灵活性;ˆφp,L(k)为未知PGφp,L(k)的估计值.1936自动化学报43卷为了使PG估计算法具有更强的跟踪时变参数的能力,并更好地适应无人驾驶实际情况,需要引入重置算法如下ˆφp,L(k)=ˆφp,L(1)(13)如果 ˆφp,L(k) ≤ε,或 ∆U L(k−1) ≤ε,或sign(ˆφ1(k))=sign(ˆφ1(1)),其中ˆφp,L(1)是ˆφp,L(k)的初始值,ε是一个小的正数.式(10)、式(12)和式(13)即为所设计的控制算法,文献[18]中有该控制算法稳定性的严格数学证明.4实验分析本文分别进行无人驾驶汽车的低速实验和高速实验.对于低速实验,文献[9]设定的纵向速度为8∼24km/h,为了进行对比分析,本文设定纵向速度为15km/h,实验场地以及轨迹如图6所示,本文先利用PID控制方法进行跟踪实验,然后利用第3.3节中设计的MFAC控制算法进行跟踪实验,并比较两种算法对预瞄偏差角跟踪系统的跟踪效果以及它们针对无人驾驶汽车循迹跟踪控制问题的控制效果,并与文献[8−9]的结果进行对比.图6低速实验场地图Fig.6Low speed experimental site对于高速实验,文献[6]设定的纵向速度为60∼80km/h,出于安全考虑,本文设定纵向速度为60km/h,在图7所示的常熟三环高架桥进行实验.本文对低速实验中的MFAC控制算法的参数不做任何修改,直接进行高速实验,分析控制效果,并与文献[6−7]的结果进行比较.4.1设备连接关系及调试软件框架无人驾驶汽车各个设备的连接关系以及调试软件框架如图8所示.外加的定位和感知传感器数据通过以太网传输给主控计算机,车速和踏板状态等原车自带传感器数据通过CAN总线传入主控计算机,本文从交换机接入外部调试计算机,编写控制算法调试软件,通过TCP/IP协议直接对无人驾驶汽车进行控制.图7高速公路实验场地图Fig.7Highway experimental site图8设备连接关系及调试软件框架图Fig.8Equipment connection anddebugging software framework整个系统的控制周期为100ms,调试计算机在一个控制周期内需要完成计算控制量、绘制图形以及保存数据等工作.外部调试计算机可以获取的数据如表3所示.调试计算机向主控计算机发送的内容如下:1)计算出的方向盘的转角;2)计算出的油门和刹车的开度(两者互斥,用同一变量表示,该变量为正表示油门输入,为负则表11期田涛涛等:基于无模型自适应控制的无人驾驶汽车横向控制方法1937示刹车输入).表3汽车状态数据符号及意义Table 3Symbols and meanings ofself-driving car s state data符号意义x ,y ,α汽车当前点的横坐标、纵坐标、航向角x ∗,y∗,β预瞄点的横坐标、纵坐标、航向角v 汽车当前的纵向速度a 汽车当前的纵向加速度w汽车当前点航向角的角速度当主控计算机(客户端)和调试计算机(服务器端)握手成功后即开始循环控制,客户端在每个控制周期内向服务器端传输汽车的状态信息,服务器端则根据相应的算法计算出控制量并传回给客户端,客户端通过CAN 总线将计算出的控制量作用于执行机构(油门、刹车和方向盘),进而实现闭环控制.4.2横向控制实验分析实验平台采用式(1)的预瞄距离表达式,参数分别是l min =4,l max =30,v min =0,v max =26,a =1.在低速实验中,对于无人驾驶汽车横向控制问题的分别利用增量式PID 方法和MFAC 方案进行对比实验,其中PID 控制器如式(14)所示:du (k )=K p (e (k )−e (k −1))+K i e (k )+K d (e (k )−2e (k −1)+e (k ))u (k )=u (k −1)+du (k )(14)用Z-N 法辅助以及大量的实验分析得到PID 的最佳参数为K p =500,K i =15,K d =30.对于MFAC 方案,式(10)、式(12)和式(13)中的控制输入线性化长度参数、步长因子、权重因子和PG 估计值的初值分别设定为L =3;ρ1=ρ2=ρ3=1;η=1;λ=22;µ=1;ˆφp,L(1)=[0.50.50.5]T ;ε设为10−5;系统延迟时间为3.7s.为了比较两种控制算法对于预瞄偏差角的跟踪效果,参考文献[9],定义均方根误差指标为RMSE(·)=1N N n =1|l n |2(15)其中,l n 是第n 个采样时刻的当前点与参考轨迹的距离,N 是总采样点数.两种算法的循迹跟踪效果、预瞄偏差角的跟踪效果和轨迹跟踪误差绝对值分别如图9∼11所示.两种控制算法的性能对比如表4所示.图9两种控制方法的跟踪效果对比图Fig.9Tracking effect comparison betweentwo control methods图10预瞄偏差角跟踪效果对比图Fig.10Preview-deviation-yaw trackingeffect comparison图11跟踪误差绝对值对比图Fig.11Absolute value of tracking error comparison1938自动化学报43卷表4两种控制算法性能对比列表Table4Theperformance oftwo control algorithms项目PID MFAC预瞄偏差角最大绝对值(rad)0.59450.4828预瞄偏差角均方根0.22590.1498跟踪误差的均方根0.52570.3320建立时间(s)85从图9∼11可以看出,PID方法在直线和小曲率转弯时的跟踪效果较好,但是PID方法的自适应性不强,很难同时适应直线和转弯的路况,对于曲率比较大的转弯(例如图中的直角转弯),不能很好地跟踪期望轨迹,最大的转弯误差为1.5m左右.另一方面,由于MFAC算法具有自适应性,在直线、小曲率转弯和大曲率转弯都能很好地适应系统的变化,故跟踪效果较好,最大的转弯误差为0.4m左右.另外,文献[8]中提出的控制算法在纵向速度为10km/h和20km/h时的建立时间分别是20s和22s;文献[9]中提出的控制算法在纵向速度为12km/h和16km/h时的跟踪误差均方根分别为0.774和0.716.本文提出的MFAC算法在低速实验中的建立时间为5s,在纵向速度为15km/h时的跟踪误差均方根为0.3320.对于高速实验,低速实验中MFAC控制算法参数不做任何修改,设定纵向速度为60km/h.跟踪效果如图12所示.图12高速跟踪轨迹图Fig.12Highway trajectory tracking参考文献[6],截取其中耗时100s的路段,本文截取路段为图7中AB段.该路段的跟踪误差绝对值以及预瞄偏差角的跟踪情况如图13所示.在AB段的跟踪误差的均方根为0.0738,最大跟踪误差绝对值为0.1824m;预瞄偏差角的均方根为0.0025,最大绝对值为0.0068rad.文献[7]所设计的控制器在纵向车速为19m/s(68.4km/h)时的跟踪误差均方根为0.0751.文献[6]中实验路段上车速由80km/h变为60km/h,又变回80km/h,整个路段的最大误差约为0.5m.图13高速公路轨迹跟踪性能Fig.13Performance of highway trajectory tracking通过图9∼13以及表4的实验数据分析,以及通过与相关文献的对比可以看出,不论低速实验还是高速公路的实验,针对无人驾驶汽车横向控制问题,本文提出的基于MFAC的预瞄偏差角跟踪方案都具有明显的优势.本文提出的控制算法利用C++语言编程实现,矩阵和向量全部转化成简单的四则运算进行处理,没有借助于任何专门的矩阵库或最优化库(文献[7]的算法实现需要借助用NPSOL软件包),对于不同无人驾驶汽车平台具有很强的可移植性.清华大学“睿龙”号无人驾驶汽车使用本文提出的预瞄偏差角跟踪方案,并利用MFAC算法做核心控制算法,参加第七届中国智能车未来挑战赛,如图14所示.该赛程全程约13.5公里,途经典型城郊、城区、快速道路、居民小区以及越野路面等真实交通环境.在道路直行、直角转弯、U形转弯(180◦转弯)等复杂路况的考验下,本文设计的算法都令人满意地完成了指定任务,没有出现任何故障.5结论本文针对无人驾驶汽车横向控制问题,将无人驾驶汽车循迹跟踪控制问题转化成预瞄偏差角跟踪问题,然后基于无人驾驶汽车横向控制系统的动态线性化数据模型,设计出无模型自适应控制算法、伪梯度估计算法和伪梯度重置算法,相比于传统的控制方案,该方案优点如下:。
基于红外与单目视觉的机器人自动回充系统

传感器与微系统(Transducer and Microsystem Technologies )2019年第38卷第7期檸檸檸檸檸檸檸檸檸檸檸檸殠殠殠殠设计与制造DOI :10.13873/J.1000—9787(2019)07—0064—03基于红外与单目视觉的机器人自动回充系统魏银,李垚(中国科学技术大学信息科学技术学院,安徽合肥230026)摘要:自动充电作为家庭扫地机器人不可或缺的重要一环,保证了扫地机器人可以在电量不足时,自行寻找到充电座对接充电。
使用红外传感器与摄像头完成扫地机器人与充电座的对接过程中,摄像头弥补了红外精确度不高的问题,红外弥补了摄像头视野范围小的问题。
测试表明机器人与座对准精度优于ʃ5mm 。
关键词:红外信号;单目视觉;自动充电;对接中图分类号:TP 242文献标识码:A文章编号:1000—9787(2019)07—0064—03Robot auto-charge system based on infrared and monocular visionWEI Yin ,LI Yao(School of Information Science and Technology ,University of Science and Technology of China ,Hefei 230026,China )Abstract :As an essential part of family cleaning-robot ,automatic charging is an important function ,it ensures the robot to find the charge-dock and charge when the battery is low.Infrared sensors and cameras are used to complete process of cleaning-robot docking to the charge-dock ,camera covers the shortage of low precision of infrared sensor ,infrared sensor covers the shortage of small vision of camera.Tests show that the precision of docking is prior to ʃ5mm.Keywords :infrared-signal ;monocular vision ;auto-charge ;docking引言自动充电是家用移动机器人如扫地机必不可少的一个功能,这个过程中机器人与充电座的对接离不开各种传感器的使用[1],以往的扫地机器人在自动充电任务中基本单独采用红外传感器技术进行对接[2],通过能接收到的信号红外传感器的位置与数量来判断机器人与充电座之间的相对位置从而进行自动充电,这种方式需要使用较多的红外传感器;或者是采用较少的红外传感器,利用检测出信号的强度来判断机器人的方向[3,4],因此需要红外信号强度检测精度非常高的接收电路;也可以通过红外信号反射的原理,机器人在不同位置时发出红外信号通过充电座反射回来接收信号,判断出机器人与充电座的相对位置进行对接[5];第四种是使用激光器和摄像头的方案[6,7],激光方向性好,一旦检测到信号对接精度高,缺点无论激光还是摄像头探测范围小,一开始不能确定目标大致方位,则需要漫游,或者只能通过固定的角度进入充电座检测范围内;还有一种充电座外形具有凹凸不平区域,两种区域分别覆盖有光线吸收率高的材料和光线反射率高的材料,这样采用激光雷达传感器进行检测,为了使充电座容易被检测到,充电收稿日期:2018—05—27座体积较大,而且激光雷达的成本也相对较高。
06-Automotive Catalyst

22
3.2 The Honeycomb Catalyst
In the mid-1960s, Engelhard began investigating the use of monolithic structures for reducing emissions from forklift trucks, mining vehicles, stationary engines, and so on. Advantages: Low pressure drop (high open frontal area (~70%))
18
3.1 The Beaded Catalyst
The most traditional way: Spherical particulate γ-Al2O3 particles, anywhere from 1/8 to 1/4 in. in diameter, into which the stabilizers and active catalytic components (i.e., precious metals) would be incorporated.
5
US Clean Air Act 1975/76 federal requirements: HC: 1.5 g/mile CO: 15 g/mile NOX: 3.1 g/mile
6
USEPA established the Federal Test Procedure (FTP) simulating the average driving conditions: (1) cold start, after the engine was idle for 8h (2) hot start (3) a combination of urban and highway driving conditions called FTP cycle.
- 1、下载文档前请自行甄别文档内容的完整性,平台不提供额外的编辑、内容补充、找答案等附加服务。
- 2、"仅部分预览"的文档,不可在线预览部分如存在完整性等问题,可反馈申请退款(可完整预览的文档不适用该条件!)。
- 3、如文档侵犯您的权益,请联系客服反馈,我们会尽快为您处理(人工客服工作时间:9:00-18:30)。
基于COPSTM的汽车仪表摘要Dedicated microprocessor systems find increasing applications in automobile instrumentation. 人们已经发现专用微处理器系统越来越地应用在汽车仪表上。
Fuel injection systems, digital radio tuners and similar applications employing the microcontroller have become common place. 燃油喷射系统、数字广播调谐器和类似的应用程序已共同采用微控制器。
This paper describes a cost effective microcontroller implementation of an automobile instrument cluster by the COPS group of National Semiconductor, Santa Clara. 本文介绍一种美国国家半导体COPS集团制造的,具有成本效益的汽车仪表微控制器的应用,该微控制器名为圣克拉拉(Santa Clara)。
The instrument cluster provides a vacuum fluorescent display of the vehicle speed, engine RPM, odometers, battery voltage, engine oil pressure and the fuel level. A modular design involving a single microcontroller in conjunction with peripherals to aid in data acquisition from the transducers allows the quantities to be computed with high accuracies and displayed on a real time basis. 该仪表对车速、发动机转速、里程计、电池电压、机油压力和燃料水平提供了真空荧光显示。
The single microcontroller environment places severe restrictions on the availability of RAM and ROM. 单片机的环境严重限制了RAM和ROM的可用性。
Coupled with the requirement of real time operation the application poses a non trivial challenge. 再加上实施操作的要求,应用受到了不小的挑战。
A nonvolatile RAM accumulates the mileage covered. 非易失性的内存计算了所行驶的里程。
Hamming code techniques ensure the integrity of the data contained in the nonvolatile memory. 汉明码技术确保了非易失性存储器中数据的完整性。
Inclusion of diagnostics allows a rapid and thorough check against improper operation of the microcontroller, peripherals and the nonvolatile memory. 列入诊断可以迅速彻底地检查微控制器、外围设备和非易失性内存的操作不当。
This paper describes the implementation with a COP444L containing 128 nybbles of RAM and 2K bytes of ROM. 本文介绍了对含128四位元组COP444L和2K字节ROM的实施。
A display updation rate of 16 Hz can be comfortably realized. 16赫兹的升级显示率可以轻松地得到实现。
Over the microcomputer usage has diversified dramatically in its scope and breadth. 微机使用的范围和宽度已经大幅度地多样化了。
Dedicated microprocessor systems find increasing application in automobile instrumentation and control. 专用微处理器系统已经越来越多地应用在汽车仪表和控制方面。
From its inception the automobile has acquired considerable sophistication. 从一开始,汽车已经得到了相当的成熟。
Increasing demands have been made of the car. 越来越多的需求成就了汽车。
Fuel efficiency, higher acceleration rates, simplicity of control and improved ride quality rank high in the demands made of the car. 燃油效率、更高的加速率、控制的简单化和改善了的乘坐质量在汽车制造要求中极为重要。
In response the automobile engine has evolved into a complex machine. 相对地,汽车发动机已经演变成了复杂的机器。
Crude methods to control or monitor its performance no longer suffice. 原油价格已经不再足以控制或监督其执行情况了。
Microprocessor based fuel injection techniques and ignition control are becoming quite ubiquitous. 基于微处理器的燃油喷射技术和点火控制已经相当普及。
The automobile instrument cluster monitors the engine and regularly updates a statusdisplay for the operator's benefit. 汽车仪表监控引擎并定期更新状态显示了运营商的利益。
Pertinent information includes the vehicle speed, the engine crankshaft rotational speed, oil pressure in the engine cylinders, condition of the battery and the mileage accumulated. 有关资料包括车速、发动机曲轴转速、机油压力、发动机气缸、条件电池和里程累计。
The instrument cluster provides a visual feedback link to the operator allowing corrective action to be initiated as the need arises. 仪表对运作者提供了视觉反馈,允许其视需要纠正行为。
THE AUTOMOBILE INSTRUMENT CLUSTER 汽车仪表。
The heart of the Automobile Instrument Cluster (AIC) lies in obtaining raw data from various transducers and manipulating it to a form suitable for feedback to the human operator. 汽车仪表(Automobile Instrument Cluster,简称AIC)的核心在于从各种传感器获得原始数据并操纵它适当反馈给人类运作者。
The feedback, normally visual, conveys the vehicle speed, the engine rpm, the engine temperature, oil pressure, the battery voltage and the odometer values. 反馈、正常视觉传达了车速、发动机转速、发动机温度、油压、电池电压和里程表价值。
The AIC can be viewed as a collection of either inherently independent or weakly linked subtasks. 汽车工业可以被看成是收集一切内在联系的独立或弱子。
Each subtask can be further partitioned into three blocks viz. of raw data collection, processing and displaying it. 每个子可以进一步被划分为三个区,即:原始数据采集,处理,及显示。
The component subtasks, in spite of their high degree of independence, can be grouped on the basis of signal available from the transducers. 尽管组成的各子有高度的独立性,但可以根据传感器提供的信号进行归纳。
Grouping the subtasks modularizes the design. Partitioning the design in this manner highlights two groups, the first requires a frequency to be measured and the second a voltage level.分组子使设计模块化了。
分区设计以该方式突出两个群体,首先要求衡量频率,其次要求衡量电压水平。
The two major groupings are briefly examined. 这两个主要集体得到了简要检查。