技术简介-ABAQUS显式的流固耦合仿真
abaqus与fluent流固耦合
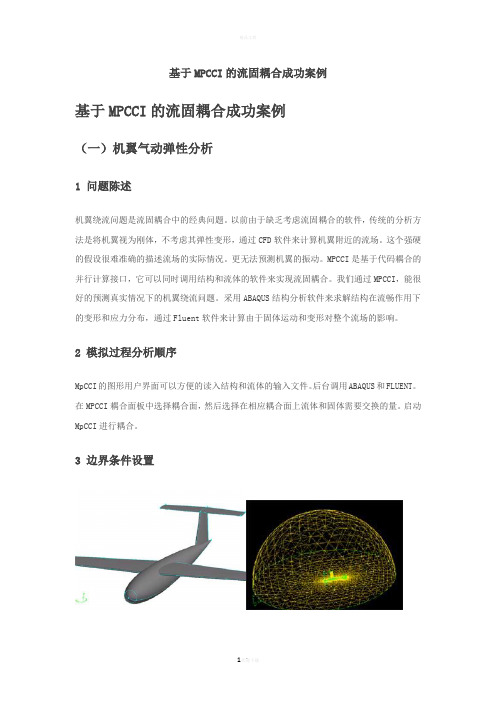
基于MPCCI的流固耦合成功案例基于MPCCI的流固耦合成功案例(一)机翼气动弹性分析1 问题陈述机翼绕流问题是流固耦合中的经典问题。
以前由于缺乏考虑流固耦合的软件,传统的分析方法是将机翼视为刚体,不考虑其弹性变形,通过CFD软件来计算机翼附近的流场。
这个强硬的假设很难准确的描述流场的实际情况。
更无法预测机翼的振动。
MPCCI是基于代码耦合的并行计算接口,它可以同时调用结构和流体的软件来实现流固耦合。
我们通过MPCCI,能很好的预测真实情况下的机翼绕流问题。
采用ABAQUS结构分析软件来求解结构在流畅作用下的变形和应力分布,通过Fluent软件来计算由于固体运动和变形对整个流场的影响。
2 模拟过程分析顺序MpCCI的图形用户界面可以方便的读入结构和流体的输入文件。
后台调用ABAQUS和FLUENT。
在MPCCI耦合面板中选择耦合面,然后选择在相应耦合面上流体和固体需要交换的量。
启动MpCCI进行耦合。
3 边界条件设置图1 无人机模型和流体计算模型结构部分单个机翼跨度在1.5m左右,厚度为0.1m左右。
边界条件为机翼端部的固定,三个方向的位移完全固定,另一端完全自由。
在固体中除了固定端的面外,其他三个面为耦合面。
流体部分采用四面体网格,采用理想气体作为密度模型。
流体的入口和出口以及对称性边界条件如下图所示。
图2 固体有限元模型4 计算方法的选择通过结合ABAQUS和FLUENT,使用MPCCI计算流固耦合。
在本例中,固体在流场作用下产生很大的变形和运动。
在耦合区域,固体结构部分计算耦合面上的节点位移,通过MPCCI传输给FLUENT的耦合界面,FLUENT 计算出耦合区域上的节点力载荷,然后通过MPCCI传给结构软件ABAQUS。
在MPCCI的耦合面板中选择的耦合面如图所示,交换量为:节点位移、相对受力。
采用ABAQUS中的STANDARD算法,时间增量步长为0.1毫秒。
5 计算结论通过MPCCI结合ABAQUS和FLUENT,成功地计算在几何非线性条件下的气动弹性问题,得到了整个流体区域的流场分布以及结构的动态响应历程。
仿真笔记——流固耦合问题研究概述及几类典型应用

仿真笔记——流固耦合问题研究概述及几类典型应用一、流固耦合概述历史上,人们对流固耦合现象的早期认识源于飞机工程中的气动弹性问题。
Wright兄弟和其它航空先驱者都曾遇到过气动弹性问题。
直到1939年二战前夕,由于飞机工业的迅猛发展,大量出现的飞机气动弹性问题的需要,有一大批科学家和工程师投入这一问题的研究。
从而,气动弹性力学开始发展成为一门独立的力学分支。
如果将与飞机颤振密切相关的气动弹性研究作为流固耦合的第一次高潮的话,则与风激振动及化工容器密切相关的研究可作为流固耦合研究的第二次高潮。
事实上,从美国ASME应用力学部召开的历次流固耦合研讨会上可以看出,流固耦合问题涉及到很多方面。
比如:空中爆炸及响应,噪声相互作用问题,气动弹性,水弹性问题,充液结构内的爆炸分析,管道中的水锤效应,充液容器的晃动及毛细流中血细胞的变形,沉浸结构的瞬态运动,流固相互冲击,板的颤振及流体引起的振动,圆柱由于热交换引起支持附件松动的非线性流固耦合系统,声音与结构的相互作用,涡流与结构的相互作用,机械工程中的机械气动弹性问题等等。
1. 流固耦合力学定义和特点流固耦合力学是流体力学与固体力学交叉而生成的力学分支。
顾名思义,它是研究变形固体在流场作用下的各种行为以及固体位形对流场影响这二者交互作用的一门科学。
流固耦合力学的重要特征是两相介质之间的交互作用(fluid-solid interaction)。
变形固体在流体载荷作用下会产生变形或运动,而变形或运动又反过来影响流场,从而改变流体载荷的分布和大小。
正是这种相互作用将在不同条件下产生形形色色的流固耦合现象。
流固耦合问题可由其耦合方程来定义,这组方程的定义域同时有流体域与固体域,而未知变量含有描述流体现象的变量及描述固体现象的变量,一般而言,具有以下两点特征:•流体域或固体域均不可能单独地求解;•无法显式地消去描述流体运动的独立变量或描述固体运动的独立变量。
2. 流固耦合力学涉及领域及分类流固耦合问题涉及到很多方面。
一种基于abaqus-starccm+的流固耦合计算方法
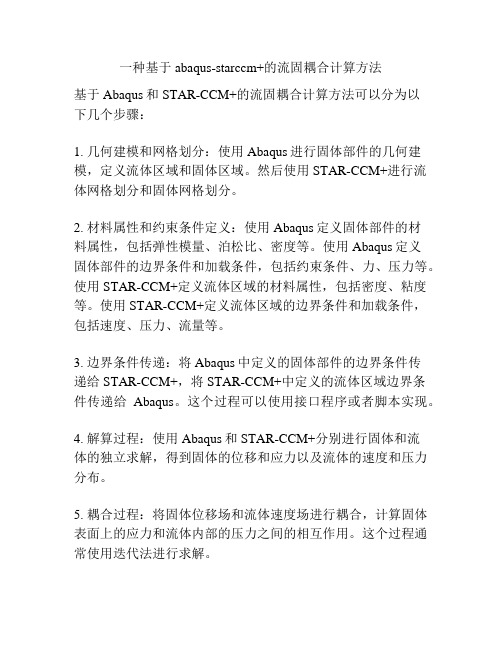
一种基于abaqus-starccm+的流固耦合计算方法基于Abaqus和STAR-CCM+的流固耦合计算方法可以分为以下几个步骤:1. 几何建模和网格划分:使用Abaqus进行固体部件的几何建模,定义流体区域和固体区域。
然后使用STAR-CCM+进行流体网格划分和固体网格划分。
2. 材料属性和约束条件定义:使用Abaqus定义固体部件的材料属性,包括弹性模量、泊松比、密度等。
使用Abaqus定义固体部件的边界条件和加载条件,包括约束条件、力、压力等。
使用STAR-CCM+定义流体区域的材料属性,包括密度、粘度等。
使用STAR-CCM+定义流体区域的边界条件和加载条件,包括速度、压力、流量等。
3. 边界条件传递:将Abaqus中定义的固体部件的边界条件传递给STAR-CCM+,将STAR-CCM+中定义的流体区域边界条件传递给Abaqus。
这个过程可以使用接口程序或者脚本实现。
4. 解算过程:使用Abaqus和STAR-CCM+分别进行固体和流体的独立求解,得到固体的位移和应力以及流体的速度和压力分布。
5. 耦合过程:将固体位移场和流体速度场进行耦合,计算固体表面上的应力和流体内部的压力之间的相互作用。
这个过程通常使用迭代法进行求解。
6. 后处理:根据需要,进行结果的后处理和分析,包括应力分布、位移分布、流速分布、压力分布等等。
总结起来,基于Abaqus和STAR-CCM+的流固耦合计算方法主要包括几何建模和网格划分、材料属性和约束条件定义、边界条件传递、解算过程、耦合过程以及后处理等步骤。
这样的方法可以用来研究流固耦合问题,如液体在固体表面的流动、液体对固体的冲击、固体表面对液体的阻力等等。
Abaqus流固耦合仿真方法大全,总有你的菜,哪怕是佛系
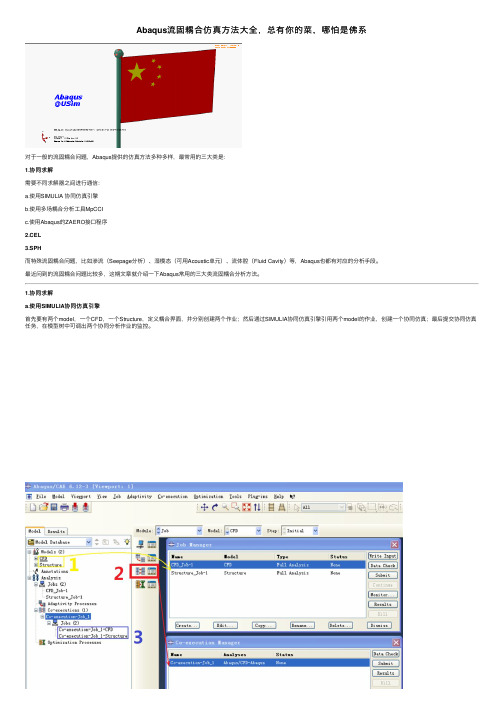
Abaqus流固耦合仿真⽅法⼤全,总有你的菜,哪怕是佛系对于⼀般的流固耦合问题,Abaqus提供的仿真⽅法多种多样,最常⽤的三⼤类是:1.协同求解需要不同求解器之间进⾏通信:a.使⽤SIMULIA 协同仿真引擎b.使⽤多场耦合分析⼯具MpCCIc.使⽤Abaqus的ZAERO接⼝程序2.CEL3.SPH⽽特殊流固耦合问题,⽐如渗流(Seepage分析)、湿模态(可⽤Acoustic单元)、流体腔(Fluid Cavity)等,Abaqus也都有对应的分析⼿段。
最近问到的流固耦合问题⽐较多,这期⽂章就介绍⼀下Abaqus常⽤的三⼤类流固耦合分析⽅法。
1.协同求解a.使⽤SIMULIA协同仿真引擎⾸先要有两个model,⼀个CFD,⼀个Structure,定义耦合界⾯,并分别创建两个作业;然后通过SIMULIA协同仿真引擎引⽤两个model的作业,创建⼀个协同仿真;最后提交协同仿真任务,在模型树中可调出两个协同分析作业的监控。
Abaqus/CFD特点:能够进⾏不可压缩流体(通常认为是液体或者密度变化相对较⼩的⽓体,0≤Ma≤0.1~0.3)动⼒学分析,可以是层流或湍流(4种湍流模型)、稳态或瞬态(能够使⽤ALE变形⽹格)。
流体参数:密度、粘度、初始速度、等压⽐热容、热膨胀系数。
⼯程应⽤领域:⼤⽓扩散、汽车⽓动设计、⽣物医药、⾷品加⼯、电器冷却、模具填充等。
6.10版引⼊CFD求解器,2017版取消,因此该⽅法只能在Abaqus有限版本内使⽤:SIMULIA Co-simulation Engine简介:达索SIMULIA的多场耦合求解平台,内置于Abaqus Job模块,功能强⼤,可以⽤于耦合Abaqus不同求解器或第三⽅求解器,⽐如单独在Abaqus内可以做到:①流固耦合将⼀个Abaqus/Standard或Abaqus/Explicit分析过程与⼀个Abaqus/CFD分析过程进⾏协同;②共轭热传导将⼀个Abaqus/Standard分析过程与⼀个Abaqus/CFD分析过程进⾏协同;③电磁-热或电磁-⼒学耦合将两个Abaqus/Standard分析过程进⾏协同;④隐式瞬态分析和显式动态分析之间耦合将⼀个Abaqus/Standard分析过程与⼀个Abaqus/Explicit分析过程进⾏协同。
一种基于abaqus-starccm+的流固耦合计算方法

一种基于abaqus-starccm+的流固耦合计算方法1. 引言1.1 概述本篇文章介绍了一种基于ABAQUS和STAR-CCM+的流固耦合计算方法。
流固耦合问题是指涉及流体和固体之间相互作用的问题,如在液态金属凝固过程中的热传导和流动问题、风力发电机叶轮的气动力学行为等。
该方法结合ABAQUS 和STAR-CCM+两个强大的计算软件,通过将它们的优势互补起来,可以更准确地模拟和分析流固耦合问题。
1.2 文章结构本文共分为五个部分。
首先,在引言部分,我们会对本文进行概述,并介绍文章的结构。
其次,在第二部分中,我们将详细介绍ABAQUS和STAR-CCM+这两个软件及其功能和特点。
第三部分将给出对流固耦合问题的概述,包括定义以及应用领域。
接下来,在第四部分中,我们将详细介绍基于ABAQUS和STAR-CCM+的流固耦合计算方法,包括在这两个软件中采用的具体算法及其原理。
最后,在结论与展望部分,我们将总结文章得出的结果,并提出存在问题与改进方向。
1.3 目的本文的目的是介绍一种基于ABAQUS和STAR-CCM+的流固耦合计算方法。
通过本文的阐述,读者将了解到这两个软件在流固耦合问题中的应用及其计算方法,以及如何运用它们进行模拟和分析。
希望通过这篇文章的撰写和分享,能够推动流固耦合问题研究领域的发展,提供更准确可靠的计算方法,并为相关领域工程师和研究人员提供参考与借鉴。
2. ABAQUS和STAR-CCM+简介2.1 ABAQUS简介ABAQUS是由Dassault Systèmes公司开发的一种强大的有限元分析软件,广泛应用于工程结构分析领域。
它能够模拟和分析复杂的结构破坏、变形、疲劳寿命等行为,提供准确的数值解。
ABAQUS具有多种计算功能,包括线性和非线性分析、静态和动态分析、热力学和热传导分析等。
它支持各种材料类型的建模,如金属、塑料、复合材料等,并且可以考虑不同加载条件下的材料本构关系。
Abaqus热流固耦合——围绕圆柱形热源进行固结
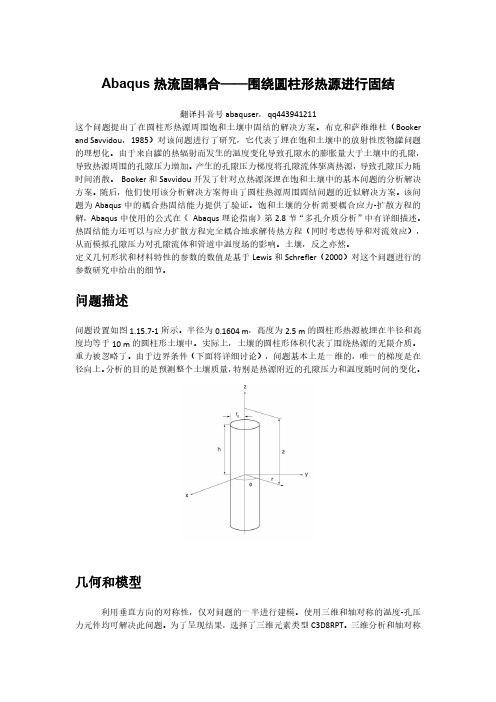
Abaqus热流固耦合——围绕圆柱形热源进行固结翻译抖音号abaquser,qq443941211这个问题提出了在圆柱形热源周围饱和土壤中固结的解决方案。
布克和萨维维杜(Booker and Savvidou,1985)对该问题进行了研究,它代表了埋在饱和土壤中的放射性废物罐问题的理想化。
由于来自罐的热辐射而发生的温度变化导致孔隙水的膨胀量大于土壤中的孔隙,导致热源周围的孔隙压力增加。
产生的孔隙压力梯度将孔隙流体驱离热源,导致孔隙压力随时间消散。
Booker和Savvidou开发了针对点热源深埋在饱和土壤中的基本问题的分析解决方案。
随后,他们使用该分析解决方案得出了圆柱热源周围固结问题的近似解决方案。
该问题为Abaqus中的耦合热固结能力提供了验证。
饱和土壤的分析需要耦合应力-扩散方程的解,Abaqus中使用的公式在《Abaqus理论指南》第2.8节“多孔介质分析”中有详细描述。
热固结能力还可以与应力扩散方程完全耦合地求解传热方程(同时考虑传导和对流效应),从而模拟孔隙压力对孔隙流体和管道中温度场的影响。
土壤,反之亦然。
定义几何形状和材料特性的参数的数值是基于Lewis和Schrefler(2000)对这个问题进行的参数研究中给出的细节。
问题描述问题设置如图1.15.7-1所示。
半径为0.1604m,高度为2.5m的圆柱形热源被埋在半径和高度均等于10m的圆柱形土壤中。
实际上,土壤的圆柱形体积代表了围绕热源的无限介质。
重力被忽略了。
由于边界条件(下面将详细讨论),问题基本上是一维的,唯一的梯度是在径向上。
分析的目的是预测整个土壤质量,特别是热源附近的孔隙压力和温度随时间的变化。
几何和模型利用垂直方向的对称性,仅对问题的一半进行建模。
使用三维和轴对称的温度-孔压力元件均可解决此问题。
为了呈现结果,选择了三维元素类型C3D8RPT。
三维分析和轴对称分析均使用基本三维8节点或轴对称4节点元素以及修饰的四面体元素的不同变体(例如,积分和混合)进行。
abaqus和Fluent的流固耦合模拟
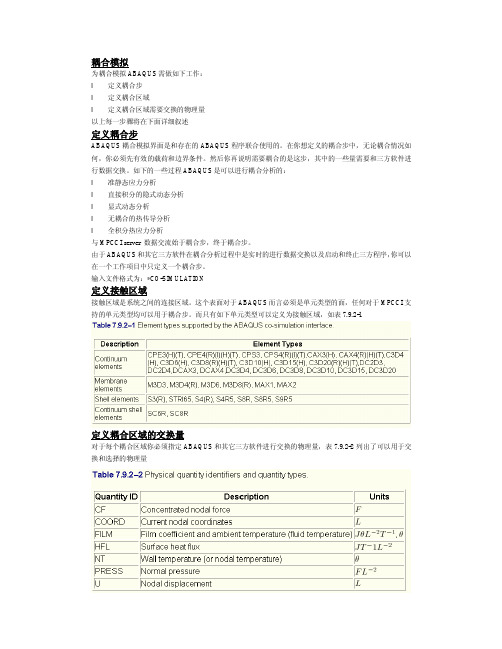
耦合模拟为耦合模拟ABAQUS需做如下工作:l定义耦合步l定义耦合区域l定义耦合区域需要交换的物理量以上每一步骤将在下面详细叙述定义耦合步ABAQUS耦合模拟界面是和存在的ABAQUS程序联合使用的。
在你想定义的耦合步中,无论耦合情况如何,你必须先有效的载荷和边界条件。
然后你再说明需要耦合的是这步,其中的一些量需要和三方软件进行数据交换。
如下的一些过程ABAQUS是可以进行耦合分析的:l准静态应力分析l直接积分的隐式动态分析l显式动态分析l无耦合的热传导分析l全积分热应力分析与MPCCI server 数据交流始于耦合步,终于耦合步。
由于ABAQUS和其它三方软件在耦合分析过程中是实时的进行数据交换以及启动和终止三方程序,你可以在一个工作项目中只定义一个耦合步。
输入文件格式为:*CO-SIMULATION定义接触区域接触区域是系统之间的连接区域。
这个表面对于ABAQUS而言必须是单元类型的面,任何对于MPCCI支持的单元类型均可以用于耦合步。
而只有如下单元类型可以定义为接触区域,如表7.9.2-1定义耦合区域的交换量对于每个耦合区域你必须指定ABAQUS和其它三方软件进行交换的物理量,表7.9.2-2列出了可以用于交换和选择的物理量输入输出的物理量的选择取决于分析的类型,如表7.9.2-3所示输入文件的格式为:*CO-SIMULA TION,IMPORTsurface_A,quantity_I1,quantity_I2,…surface_B,quatity_I3*CO-SIMULA TION,EXPORTsurface_A,quantity_E1surface_B,quantity_E2当前节点坐标和位移因为在CFD代码中流体形状可以变化,不保持初始几何构型,所以在流固耦合(FSI)中选择当前节点坐标(COORD),而不是选择节点位移(U)。
不管是做小变形还是大变形,COORD的定义是当前节点坐标。
abaqus FSI流固耦合教程(模板参考)
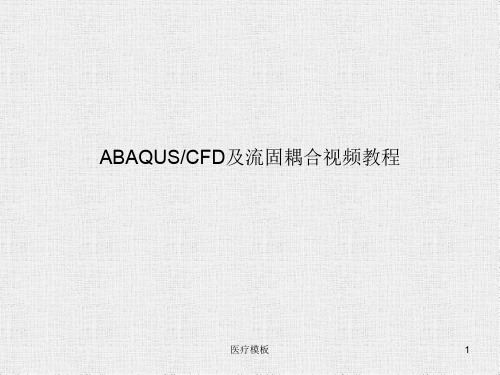
医疗模板
45
3、流固耦合操作与实例
后处理: 1、管道的压力云图 2、管道转弯处的位移随时间变化 3、流体的速度剖面图 4、显示流线
医疗模板
46
4、流热耦合操作与实例
医疗模板
47
4、流热耦合操作与实例
实例题目:单芯片的电路板流热耦合分析[1] 分析对象:芯片与周围介质 分析平台:ABAQUS 6.12 分析类型:双向流热耦合 分析目标:了解芯片传导换热的状况
医疗模板
28
2 abaqus流固耦合简介
医疗模板
29
2 abaqus流固耦合简介
(4)定义边界和载荷
医疗模板
30
2 abaqus流固耦合简介
医疗模板
31
2 abaqus流固耦合简介
医疗模板
32
2 abaqus流固耦合简介
医疗模板
33
2 abaqus流固耦合简介
医疗模板
34
2 abaqus流固耦合简介
48
4、热流耦合操作与实例
1、建立几何模型 PCB板尺寸 7.8X11.6X0.16 cm 芯片尺寸 3X3X0.7 cm 发热块尺寸 1.8X1.8X0.3cm 核心尺寸 0.75X0.75X0.2cm 空气尺寸 27.8X20X12.56 cm
医疗模板
49
4、热流耦合操作与实例
• 单元类型DC3D8 • 初始温度293K • 体热通量50mW/s/mm3 • 瞬态热传递分析步,初始增量0.01s;CFD分析;总仿真
医疗模板
13
1 abaqus/CFD模块简介
医疗模板
14
1 abaqus/CFD模块简介
1.3 入门实例
- 1、下载文档前请自行甄别文档内容的完整性,平台不提供额外的编辑、内容补充、找答案等附加服务。
- 2、"仅部分预览"的文档,不可在线预览部分如存在完整性等问题,可反馈申请退款(可完整预览的文档不适用该条件!)。
- 3、如文档侵犯您的权益,请联系客服反馈,我们会尽快为您处理(人工客服工作时间:9:00-18:30)。
ABAQUS Technology BriefTB-04-FSIS-1 Revised: June 2004Fluid-Structure Interaction Simulations with ABAQUS/ExplicitCopyright © 2004 ABAQUS, Inc.SummaryStructures that contain fluid must be analyzed under a variety of load types to determine design effectiveness. In the design of containers and consumer products, typical loading scenarios considered include drop testing, temperature change, pressurization, and stacking. For dynamic loading situations, ABAQUS/Explicit includes a number of advanced features that allow certain types of fluid-structure interaction, including sloshing and inertial loading effects, to be modeled accurately. ABAQUS has been used extensively in the consumer products and packaging industries for these types of analyses.Key ABAQUS Features and Benefits• Explicit dynamic solution method for efficient analysis of transient, highly nonlinear problems. • Equation of state models for fluid constitutive behavior. • Automatic adaptive meshing to maintain fluid mesh quality. • Robust contact algorithm. • Material and element failure for simulation of container rupture.BackgroundFluid that partially fills a structure may undergo sloshing whenever the containment structure experiences motion. In a sufficiently dynamic event the inertial loading of the fluid on the structure becomes a critical component of the analysis. As a result of the coupling between the fluid and the structure, large deformations may be experienced by both, possibly causing rupture of a closed container or fluid loss from an open container.While not offering full computational fluid dynamics (CFD) capabilities, ABAQUS/Explicit is well suited for analyzing this type of fluid-structure interaction.Two examples of fluid-structure interaction analyses are presented in this technology brief.The first example is a fluid containment simulation. A container consisting of a box with a lid is partially filled with fluid, and the box is given a velocity history. The purpose of the analysis is to determine if the fluid sloshing will cause the lid to lift from the box.The second example is a 3-ft. drop test of a partially filled consumer bottle. The purpose of theanalysis is to assess whether the bottle will rupture.In both problems the effect of the inertial fluidstructure coupling on the structural response is of primary interest.Finite Element Analysis ApproachThe fluid and structure in both problems are meshed as separate bodies, with contact definitions defined at the interfacing surfaces. The general contact algorithm, which allows for very simple definitions of the contact interactions, is employed.Fluid containment analysisThe purpose of this analysis is to assess whether the sloshing of fluid in a partially filled box would lift the lid when a velocity history is applied to the box. Shell elements are used to represent both the box and the lid, and solid hexahedral elements are used for the fluid. The lid and the box are significantly stiffer than the fluid, so these components are modeled as rigid bodies. Figure 1, Figure 2, and Figure 3 show the meshes used for each portion of the model.Figure 1: Rigid element mesh for the lid. Figure 2: Solid element mesh for the fluid.The box is constrained to remain on the horizontal plane throughout the analysis; that is, it is not allowed to rotate in space or to lift from the ground. Gravity loads are applied to the lid and to the fluid; and a sinusoidal, time-varying velocity is applied to the box. The velocity history is such that the box moves only in the horizontal plane; no vertical motion has been prescribed.The lid simply sits on the box; therefore, an airtight seal is not assumed at this interface. Consequently, there is no gas pressure in the space above the fluid. If the effect of a gas were to be included in the analysis, the following modeling approaches could be taken:• The gas could be modeled with solid elements (using an equation of state material model) and a contact interface between the fluid and the gas.• A surface-based hydrostatic fluid cavity could be defined for the gas.• A simple surface pressure load could be applied to the free surface of the fluid.Bottle drop analysisThe purpose of this analysis is to determine the integrity of a fluid-filled bottle when dropped from a height of 3 ft. Shell elements are used to represent the bottle, and solid hexahedral elements are used for the fluid. A single rigid element is used to model the floor. The undeformed model is shown in Figure 4.Figure 3: Rigid element mesh for the box.Contact is defined between the lid and the box and between the fluid and the entire container. No attachment has been defined between the lid and the box.ABAQUS has a number of contact algorithms; the general contact capability is the easiest to use and the most comprehensive. An advantage of the general contact algorithm is its ability to include the “edge-to-edge” contact at the top of the box in the contact definition. With edge-toedge contact, geometric feature edges, perimeter edges of shell and solid elements, and segments of beam and truss elements can be included in the contact domain. This feature allows contact interactions that cannot be detected as penetrations of nodes into faces to be enforced.Figure 4: Undeformed bottle, fluid, and rigid floor.Contact is defined between the bottle and the fluid and between the bottle and the floor. Gravity loads are applied to both the fluid and the container. The initial conditions of the bottle are consistent with a drop of 3 ft. The bottle is positioned slightly above the point of contact, and the fluid and the container are given an initial velocity of 168 in/s. The rigid floor is fully constrained.2The elastic-plastic constitutive properties of the bottle are those of high-density polyethylene (HDPE). A failure model is included for the HDPE, based on the tensile hydrostatic pressure stress in the elements. This failure model allows elements to be deleted from the mesh once failure has been detected. The general contact algorithm will automatically eliminate failed elements from the contact domain and update contact surfaces so that the resulting surface lies on elements that have not failed; surface erosion is a key capability in modeling the contact as the fluid sloshes out of a broken container. The base of the bottle is thicker than the walls, making the material in this region slightly more resistant to failure. This region is shown in red in Figure 5.Figure 5: Regions of different thicknesses in the bottle.The effect of any gas pressure in the bottle has been ignored. In this case the size of the enclosed gas cavity is small compared to the fluid, so the effect of the gas has been assumed negligible. The additional features common to both models are discussed below.Equation of state material model In both examples the fluid is considered as incompressible and inviscid. An equation of state material model is typically used for such applications and is chosen here. The equation of state determines the volumetric strength of a hydrodynamic material and specifies the pressure in the material as a function of density and internal energy. With this approach the deviatoric strength of the material is considered separately and can be included if viscous behavior is needed.Section properties of the fluid elements ABAQUS/Explicit offers alternative kinematic formulations for solid hexahedral elements: when appropriate for the analysis, choosing a nondefault formulation can significantly reduce computational expense. For the elementsrepresenting the fluid in the present simulations, an orthogonal formulation is chosen. This formulation provides a good balance between computational speed and accuracy. If the objective of the analyses was to determine the shape of the fluid free surface with the highest possible accuracy, the default kinematic formulation would be appropriate. However, because the inertial coupling of the fluid and structure is of primary importance, a less computationally expensive formulation can be used.Automatic adaptive meshing for the fluid Automatic adaptive meshing in ABAQUS/Explicit allows it to maintain high-quality element shapes as the fluid undergoes large deformation during sloshing. While a regular Lagrangian approach could be used to model the fluid, the elements would become very distorted after a short period of time. Adaptive meshing maintains well-shaped elements, allowing for a longer simulation time by periodically adjusting the element shapes in the fluid domain. Initially regular, relatively coarse meshes of hexahedral elements are used for the fluid. A single adaptive mesh domain that incorporates the entire fluid region is defined. In the bottle drop example a graded smoothing objective is used so that the initial mesh gradation of the water is preserved approximately while continuous adaptive meshing is performed. In addition, the default curvature refinement weighting is increased, causing the adaptive meshing algorithm to retain more elements in areas of high concave curvature.Analysis Results and Discussion Some representative results from the analyses are presented.Fluid containment analysis Figure 6–Figure 9 display the deformed shape of the fluid at several points of the analysis.Figure 6: Deformed shape after 0.12 seconds.3No restraint mechanism is applied to the lid; it is simply placed on the box. Since the lid is modeled as rigid, the history of the vertical displacement of the center of the lid (Figure 10) clearly shows that the sloshing induced in the fluid will cause the lid to separate from the box.Figure 7: Deformed shape after 0.375 seconds.Figure 10: History of vertical displacement of the lid center.Bottle drop analysisThe deformation and damage sustained by the bottle are shown in Figure 11–Figure 15.Figure 8: Deformed shape after 0.6 seconds.Figure 11: Deformed shape after 6.3 milliseconds.Figure 9: Deformed shape after 0.877 seconds.The deformed shape plots show the large deformations achieved by the fluid as the box moves. The automatic adaptive meshing capability in ABAQUS/Explicit maintains well shaped elements in the fluid, allowing the fluid to achieve high levels of deformation.Figure 12: Deformed shape after 10.8 milliseconds.4Figure 13: Deformed shape after 12.6 milliseconds.Figure 15: Deformed shape after 18 milliseconds.The deformed shape plots clearly show the buckling response of the bottle on impact and the instant of rupture (the failed elements have been removed from the plots). The tensile failure material model produces an output variable that indicates whether failure has occurred for each element, and the Visualization module in ABAQUS/CAE can remove the failed elements from the display. As the failure propagates, it can be seen that the tear travels down the corner of the bottle and turns along the interface between the thicker base section and the thinner bottle wall.Figure 14: Deformed shape after 14.4 milliseconds.ConclusionsAs demonstrated in the above analyses, ABAQUS/Explicit can be used to incorporate the effects of sloshing-type fluid-structure interaction into dynamic analyses. While it is generally not possible in ABAQUS/Explicit to model complex fluid flow behaviors or phenomena such as freesurface interactions and splashing, inclusion of the inertial loading caused by the fluid deformation allows for a more complete simulation capability.ABAQUS ReferencesFor additional information on the ABAQUS capabilities referred to in this brief, see the following ABAQUS Version 6.4 documentation references:• Analysis User’s Manual- “Explicit dynamic analysis,” Section 6.3.3 - “Adaptive meshing,” Section 7.16 - “Equation of state,” Section 10.9.1• Example Problems Manual- “Cask drop with foam impact limiter,” Section 2.1.12 - “Water sloshing in a baffled tank,” Section 2.1.14• Benchmarks Manual- “Water sloshing in a pitching tank,” Section 1.11.75。