风能介绍外文翻译
风能电能英文作文
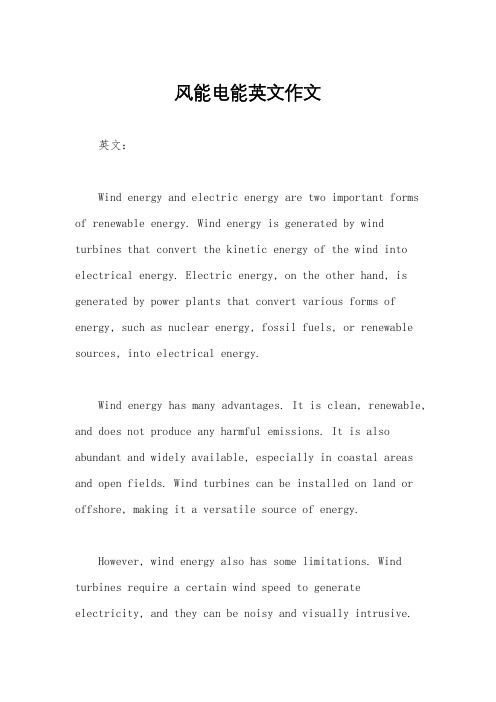
风能电能英文作文英文:Wind energy and electric energy are two important forms of renewable energy. Wind energy is generated by wind turbines that convert the kinetic energy of the wind into electrical energy. Electric energy, on the other hand, is generated by power plants that convert various forms of energy, such as nuclear energy, fossil fuels, or renewable sources, into electrical energy.Wind energy has many advantages. It is clean, renewable, and does not produce any harmful emissions. It is also abundant and widely available, especially in coastal areas and open fields. Wind turbines can be installed on land or offshore, making it a versatile source of energy.However, wind energy also has some limitations. Wind turbines require a certain wind speed to generate electricity, and they can be noisy and visually intrusive.They also have a limited capacity and cannot generate electricity continuously.Electric energy, on the other hand, has a higher capacity and can generate electricity continuously. It can also be produced from a variety of sources, including renewable sources such as wind, solar, and hydroelectric power. Electric energy is also more efficient than other forms of energy, such as fossil fuels, and produces fewer emissions.However, electric energy also has some drawbacks. It requires a lot of infrastructure, including power plants, transmission lines, and distribution networks. It can also be expensive to produce and distribute, especially if it is generated from non-renewable sources.In conclusion, both wind energy and electric energy have their advantages and disadvantages. While wind energy is clean and renewable, it has limitations in terms of capacity and reliability. Electric energy, on the other hand, has a higher capacity and can be produced from avariety of sources, but it requires a lot of infrastructure and can be expensive.中文:风能和电能是两种重要的可再生能源形式。
风能英汉互译作文
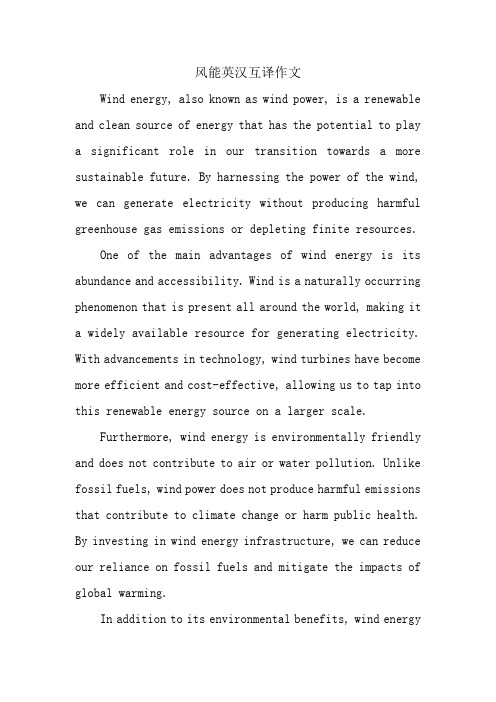
风能英汉互译作文Wind energy, also known as wind power, is a renewable and clean source of energy that has the potential to play a significant role in our transition towards a more sustainable future. By harnessing the power of the wind, we can generate electricity without producing harmful greenhouse gas emissions or depleting finite resources.One of the main advantages of wind energy is its abundance and accessibility. Wind is a naturally occurring phenomenon that is present all around the world, making it a widely available resource for generating electricity. With advancements in technology, wind turbines have become more efficient and cost-effective, allowing us to tap into this renewable energy source on a larger scale.Furthermore, wind energy is environmentally friendly and does not contribute to air or water pollution. Unlike fossil fuels, wind power does not produce harmful emissions that contribute to climate change or harm public health. By investing in wind energy infrastructure, we can reduce our reliance on fossil fuels and mitigate the impacts of global warming.In addition to its environmental benefits, wind energyalso has the potential to create jobs and stimulate economic growth. The development and maintenance of wind farms require skilled workers, engineers, and technicians, providing employment opportunities in the renewable energy sector. Moreover, investing in wind energy can help diversify our energy sources and reduce our dependence on imported fuels.In conclusion, harnessing wind energy is a sustainable and environmentally responsible way to meet our growing energy needs. By investing in wind power infrastructure and promoting the use of renewable energy sources, we can create a cleaner and healthier planet for future generations.中文翻译:风能,也被称为风力能源,是一种可再生且清洁的能源来源,有潜力在我们向更可持续未来转型中发挥重要作用。
风能英文简介
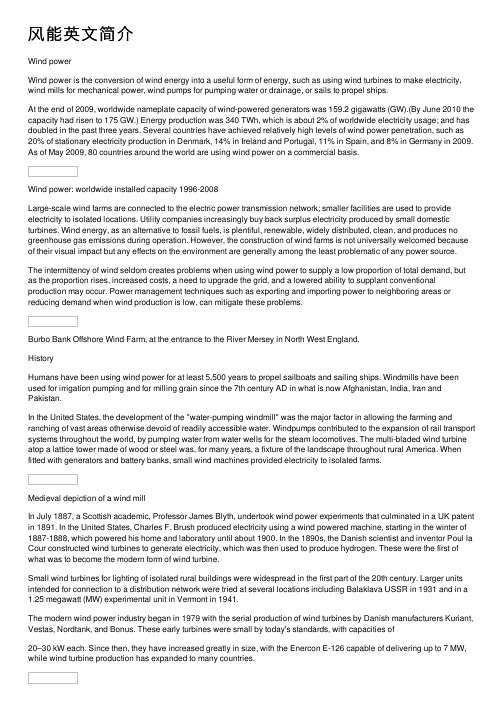
风能英⽂简介Wind powerWind power is the conversion of wind energy into a useful form of energy, such as using wind turbines to make electricity, wind mills for mechanical power, wind pumps for pumping water or drainage, or sails to propel ships.At the end of 2009, worldwide nameplate capacity of wind-powered generators was 159.2 gigawatts (GW).(By June 2010 the capacity had risen to 175 GW.) Energy production was 340 TWh, which is about 2% of worldwide electricity usage; and has doubled in the past three years. Several countries have achieved relatively high levels of wind power penetration, such as 20% of stationary electricity production in Denmark, 14% in Ireland and Portugal, 11% in Spain, and 8% in Germany in 2009. As of May 2009, 80 countries around the world are using wind power on a commercial basis.Wind power: worldwide installed capacity 1996-2008Large-scale wind farms are connected to the electric power transmission network; smaller facilities are used to provide electricity to isolated locations. Utility companies increasingly buy back surplus electricity produced by small domestic turbines. Wind energy, as an alternative to fossil fuels, is plentiful, renewable, widely distributed, clean, and produces no greenhouse gas emissions during operation. However, the construction of wind farms is not universally welcomed because of their visual impact but any effects on the environment are generally among the least problematic of any power source. The intermittency of wind seldom creates problems when using wind power to supply a low proportion of total demand, but as the proportion rises, increased costs, a need to upgrade the grid, and a lowered ability to supplant conventional production may occur. Power management techniques such as exporting and importing power to neighboring areas or reducing demand when wind production is low, can mitigate these problems.Burbo Bank Offshore Wind Farm, at the entrance to the River Mersey in North West England.HistoryHumans have been using wind power for at least 5,500 years to propel sailboats and sailing ships. Windmills have been used for irrigation pumping and for milling grain since the 7th century AD in what is now Afghanistan, India, Iran and Pakistan.In the United States, the development of the "water-pumping windmill" was the major factor in allowing the farming and ranching of vast areas otherwise devoid of readily accessible water. Windpumps contributed to the expansion of rail transport systems throughout the world, by pumping water from water wells for the steam locomotives. The multi-bladed wind turbine atop a lattice tower made of wood or steel was, for many years, a fixture of the landscape throughout rural America. When fitted with generators and battery banks, small wind machines provided electricity to isolated farms.Medieval depiction of a wind millIn July 1887, a Scottish academic, Professor James Blyth, undertook wind power experiments that culminated in a UK patent in 1891. In the United States, Charles F. Brush produced electricity using a wind powered machine, starting in the winter of 1887-1888, which powered his home and laboratory until about 1900. In the 1890s, the Danish scientist and inventor Poul la Cour constructed wind turbines to generate electricity, which was then used to produce hydrogen. These were the first of what was to become the modern form of wind turbine.Small wind turbines for lighting of isolated rural buildings were widespread in the first part of the 20th century. Larger units intended for connection to a distribution network were tried at several locations including Balaklava USSR in 1931 and in a 1.25 megawatt (MW) experimental unit in Vermont in 1941.The modern wind power industry began in 1979 with the serial production of wind turbines by Danish manufacturers Kuriant, Vestas, Nordtank, and Bonus. These early turbines were small by today's standards, with capacities of20–30 kW each. Since then, they have increased greatly in size, with the Enercon E-126 capable of delivering up to 7 MW, while wind turbine production has expanded to many countries.Windmills are typically installed in favourable windy locations. In the image, wind power generators in Spain near an Osborne bullWind energyThe Earth is unevenly heated by the sun, such that the poles receive less energy from the sun than the equator; along with this, dry land heats up (and cools down) more quickly than the seas do. The differential heating drives a global atmospheric convection system reaching from the Earth's surface to the stratosphere which acts as a virtual ceiling. Most of the energy stored in these wind movements can be found at high altitudes where continuous wind speeds of over 160 km/h (99 mph) occur. Eventually, the wind energy is converted through friction into diffuse heat throughout the Earth's surface and the atmosphere.The total amount of economically extractable power available from the wind is considerably more than present human power use from all sources. The most comprehensive study as of 2005 found the potential of wind power on land and near-shore to be 72 TW, equivalent to 54,000 MToE (million tons of oil equivalent) per year, or over five times the world's current energy use in all forms. The potential takes into account only locations with mean annual wind speeds ≥ 6.9 m/s at 80 m. The study assumes six 1.5 megawatt, 77 m diameter turbines per square kilometer on roughly 13% of the total global land area (though that land would also be available for other compatible uses such as farming). The authors acknowledge that many practical barriers would need to be overcome to reach this theoretical capacity.Map of available wind power for the United States. Color codes indicate wind power density classThe practical limit to exploitation of wind power will be set by economic and environmental factors, since the resource available is far larger than any practical means to develop it.Distribution of wind speedThe strength of wind varies, and an average value for a given location does not alone indicate the amount of energy a wind turbine could produce there. To assess the frequency of wind speeds at a particular location, a probability distribution function is often fit to the observed data. Different locations will have different wind speed distributions. The Weibull model closely mirrors the actual distribution of hourly wind speeds at many locations. The Weibull factor is often close to 2 and therefore a Rayleigh distribution can be used as a less accurate, but simpler model.Distribution of wind speed (red) and energy (blue) for all of 2002 at the Lee Ranch facility in Colorado. The histogram shows measured data, while the curve is the Rayleigh model distribution for the same average wind speedBecause so much power is generated by higher wind speed, much of the energy comes in short bursts. The 2002 Lee Ranch sample is telling; half of the energy available arrived in just 15% of the operating time. The consequence is that wind energy from a particular turbine or wind farm does not have as consistent an output as fuel-fired power plants.Electricity generationIn a wind farm, individual turbines are interconnected with a medium voltage (often 34.5 kV), power collection system and communications network. At a substation, this medium-voltage electric current is increased in voltage with a transformer for connection to the high voltage electric power transmission system.Typical components of a wind turbine (gearbox, rotor shaft and brake assembly) being lifted into positionThe surplus power produced by domestic microgenerators can, in some jurisdictions, be fed into the network and sold to the utility company, producing a retail credit for the microgenerators' owners to offset their energy costs.Grid managementInduction generators, often used for wind power, require reactive power for excitation so substations used in wind-power collection systems include substantial capacitor banks for power factor correction. Different types of wind turbine generators behave differently during transmission grid disturbances, so extensive modelling of the dynamic electromechanical characteristics of a new wind farm is required by transmission system operators to ensure predictable stable behaviour during system faults (see: Low voltage ride through). In particular, induction generators cannot support the system voltage during faults, unlike steam or hydro turbine-driven synchronous generators. Doubly-fed machines generally have more desirable properties for grid interconnection. Transmission systems operators will supply a wind farm developer with a gridcode to specify the requirements for interconnection to the transmission grid. This will include power factor, constancy of frequency and dynamic behavior of the wind farm turbines during a system fault.Capacity factorSince wind speed is not constant, a wind farm's annual energy production is never as much as the sum of the generator nameplate ratings multiplied by the total hours in a year. The ratio of actual productivity in a year to this theoretical maximum is called the capacity factor. Typical capacity factors are 20–40%, with values at the upper end of the range in particularly favourable sites. For example, a 1 MW turbine with a capacity factor of 35% will not produce8,760 MW·h in a year (1 × 24 × 365), but only 1 × 0.35 × 24 ×365 = 3,066 MW·h, averaging to 0.35 MW. Online data is available for some locations and the capacity factor can be calculated from the yearly output.Unlike fueled generating plants, the capacity factor is limited by the inherent properties of wind. Capacity factors of other types of power plant are based mostly on fuel cost, with a small amount of downtime for maintenance. Nuclear plants have low incremental fuel cost, and so are run at full output and achievea 90% capacity factor. Plants with higher fuel cost are throttled back to follow load. Gas turbine plants using natural gas as fuel may be very expensive to operate and may be run only to meet peak power demand. A gas turbine plant may have an annual capacity factor of 5–25% due to relatively high energy production cost.In a 2008 study released by the U.S. Department of Energy's Office of Energy Efficiency and Renewable Energy, the capacity factor achieved by the wind turbine fleet is shown to be increasing as the technology improves. The capacity factor achieved by new wind turbines in 2004 and 2005 reached 36%.PenetrationWind energy "penetration" refers to the fraction of energy produced by wind compared with the total available generation capacity. There is no generally accepted "maximum" level of wind penetration. The limit for a particular grid will depend on the existing generating plants, pricing mechanisms, capacity for storage or demand management, and other factors. An interconnected electricity grid will already include reserve generating and transmission capacity to allow for equipment failures; this reserve capacity can also serve to regulate for the varying power generation by wind plants. Studies have indicated that 20% of the total electrical energy consumption may be incorporated with minimal difficulty. These studies have been for locations with geographically dispersed wind farms, some degree of dispatchable energy, or hydropower with storage capacity, demand management, and interconnection to a large grid area export of electricity when needed. Beyond this level, there are few technical limits, but the economic implications become more significant. Electrical utilities continue to study the effects of large (20% or more) scale penetration of wind generation on system stability and economics.At present, a few grid systems have penetration of wind energy above 5%: Denmark (values over 19%), Spain and Portugal (values over 11%), Germany and the Republic of Ireland (values over 6%). But even with a modest level of penetration, there can be times where wind power provides a substantial percentage of the power on a grid. For example, in the morning hours of 8 November 2009, wind energy produced covered more than half the electricity demand in Spain, setting a new record. This was an instance where demand was very low but wind power generation was very high.Variability and intermittencyElectricity generated from wind power can be highly variable at several different timescales: from hour to hour, daily, and seasonally. Annual variation also exists, but is not as significant. Related to variability is the short-term (hourly or daily) predictability of wind plant output. Like other electricity sources, wind energy must be "scheduled". Wind power forecasting methods are used, but predictability of wind plant output remains low for short-term operation.Because instantaneous electrical generation and consumption must remain in balance to maintain grid stability, this variability can present substantial challenges to incorporating large amounts of wind power into a grid system. Intermittency and the non-dispatchable nature of wind energy production can raise costs for regulation, incremental operating reserve, and (at highpenetration levels) could require an increase in the already existing energy demand management, load shedding, or storage solutions or system interconnection with HVDC cables. At low levels of wind penetration, fluctuations in load and allowance for failure of large generating units requires reserve capacity that can also regulate for variability of wind generation. Wind power can be replaced by other power stations during low wind periods. Transmission networks must already cope with outages of generation plant and daily changes in electrical demand. Systems with large wind capacity components may need more spinning reserve (plants operating at less than full load).Wildorado Wind Ranch in Oldham County in the Texas Panhandle, as photographed from U.S. Route 385Pumped-storage hydroelectricity or other forms of grid energy storage can store energy developed by high-wind periods and release it when needed. Stored energy increases the economic value of wind energy since it can be shifted to displace higher cost generation during peak demand periods. The potential revenue from this arbitrage can offset the cost and losses of storage; the cost of storage may add 25% to the cost of any wind energy stored, but it is not envisaged that this would apply to a large proportion of wind energy generated. The 2 GW Dinorwig pumped storage plant in Wales evens out electrical demand peaks, and allows base-load suppliers to run their plant more efficiently. Although pumped storage power systems are only about 75% efficient, and have high installation costs, their low running costs and ability to reduce the required electrical base-load can save both fuel and total electrical generation costs.In particular geographic regions, peak wind speeds may not coincide with peak demand for electrical power. In the US states of California and Texas, for example, hot days in summer may have low wind speed and high electrical demand due to air conditioning. Some utilities subsidize the purchase of geothermal heat pumps by their customers, to reduce electricity demand during the summer months by making air conditioning up to 70% more efficient; widespread adoption of this technology would better match electricity demand to wind availability in areas with hot summers and low summer winds. Another option is to interconnect widely dispersed geographic areas with an HVDC"Super grid". In the USA it is estimated that to upgrade the transmission system to take in planned or potential renewables would cost at least $60 billion.In the UK, demand for electricity is higher in winter than in summer, and so are wind speeds. Solar power tends to be complementary to wind. On daily to weekly timescales, high pressure areas tend to bring clear skies and low surface winds, whereas low pressure areas tend to be windier and cloudier. On seasonal timescales, solar energy typically peaks in summer, whereas in many areas wind energy is lower in summer and higher in winter. Thus the intermittencies of wind and solar power tend to cancel each other somewhat. The Institute for Solar Energy Supply Technology of the University of Kassel pilot-tested a combined power plant linking solar, wind, biogas and hydrostorage to provide load-following power around the clock, entirely from renewable sources.A report on Denmark's wind power noted that their wind power network provided less than 1% of average demand 54 days during the year 2002. Wind power advocates argue that these periods of low wind can be dealt with by simply restarting existing power stations that have been held in readiness or interlinking with HVDC. Electrical grids with slow-responding thermal power plants and without ties to networks with hydroelectric generation may have to limit the use of wind power.[42]Three reports on the wind variability in the UK issued in 2009, generally agree that variability of wind needs to be taken into account, but it does not make the grid unmanageable; and the additional costs, which are modest, can be quantified. A 2006 International Energy Agency forum presented costs for managing intermittency as a function of wind-energy's share of total capacity for several countries, as shown: Increase in system operation costs, Euros per MW·h, for 10% and 20% wind share10% 20%Germany 2.5 3.2Denmark 0.4 0.8Finland 0.3 1.5Norway 0.1 0.3Sweden 0.3 0.7。
风能基础知识PPT(英文版)
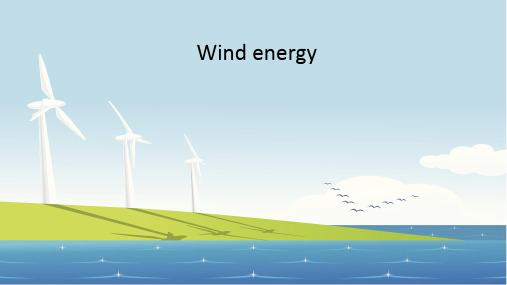
Contents
Wind Power: Talk 1
• Why wind? • How wind turbines
work • The power in the
wind
Key factors in wind power
• Wind consistency (how much of the time does the wind blow, at a useful speed?)
• Wind speed (how high is the average wind speed?) • Spacing of turbines in the array (are they far enough apart to
require yaw device
• Vertical axis
– Don’t need to point into the wind
– Easy to construct
– Less efficient – Mounted lower, less
wind – More turbulent, stress
access undisturbed wind?) • Size of turbines (how large is the area of e circle covered by
the blades?) • How large an area of land (or sea) can be dedicated to the
Why wind?
• Wind is widely available • Largely independent from imports,
风电专业术语英文对照及解释

风电专业术语英文对照及解释风电专业术语中英对照及解释经电气网小编整理,下面是有关风电的一些专业术语的英汉对照及解释,希望对各位有用哦。
1.风能 /wind energy 空气流动所具有的能量。
2.风能资源 /wind energy resources 大气沿地球表面流动而产生的动能资源。
3.空气的标准状态 /standard atmospheric state 空气的标准状态是指空气压力为101 325Pa,温度为15℃(或绝对288.15K),空气密度1.225kg/m 3 时的空气状态。
4.风速/wind speed 空间特定点的风速为该点空气在单位时间内所流过的距离。
5.平均风速 /average wind speed 给定时间内瞬时风速的平均值。
6.年平均风速 /annual average wind speed 时间间隔为一整年的瞬时风速的平均值。
7.最大风速 /maximum wind speed 10分钟平均风速的最大值。
8.极大风速 /extreme wind speed 瞬时风速的最大值。
9.阵风 /gust 超过平均风速的突然和短暂的风速变化。
10.年际变化/inter-annual variation 以30年为基数发生的变化。
风速年际变化是从第1年到第30年的年平均风速变化。
11.[风速或风功率密度]年变化 /annual variation 以年为基数发生的变化。
风速(或风功率变化)年变化是从1月到12月的月平均风速(或风功率密度)变化。
12.[风速或风功率密度]日变化 /diurnal variation 以日为基数发生的变化。
月或年的风速(或风功率密度)日变化是求出一个月或一年内,每日同一钟点风速(或风功率密度)的月平均值或年平均值,得到0点到23点的风速(或风功率密度)变化。
风切变 /wind shear 风速在垂直于风向平面内的变化。
13.风切变指数 /wind shear exponent 用于描述风速剖面线形状的幂定律指数。
风能的优缺点英文作文
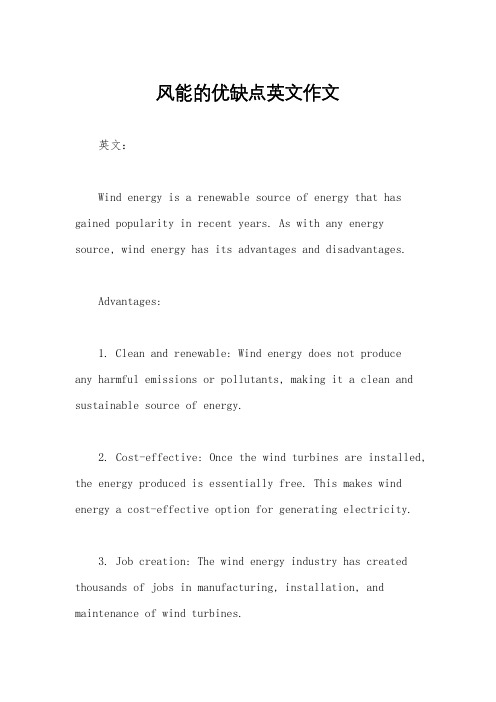
风能的优缺点英文作文英文:Wind energy is a renewable source of energy that has gained popularity in recent years. As with any energy source, wind energy has its advantages and disadvantages.Advantages:1. Clean and renewable: Wind energy does not produceany harmful emissions or pollutants, making it a clean and sustainable source of energy.2. Cost-effective: Once the wind turbines are installed, the energy produced is essentially free. This makes wind energy a cost-effective option for generating electricity.3. Job creation: The wind energy industry has created thousands of jobs in manufacturing, installation, and maintenance of wind turbines.Disadvantages:1. Intermittent: Wind energy is dependent on wind speed, which can vary greatly throughout the day and seasonally. This means that wind energy cannot be relied upon as a constant source of energy.2. Wildlife impact: Wind turbines can pose a threat to birds and bats, which can collide with the blades.3. Visual impact: Some people find wind turbines to be unsightly and a blight on the landscape.Overall, wind energy has many advantages, but it also has some drawbacks. It is important to carefully consider these factors when deciding whether or not to invest inwind energy.中文:风能是一种可再生能源,近年来越来越受到关注。
风力发电外文文献翻译中英文
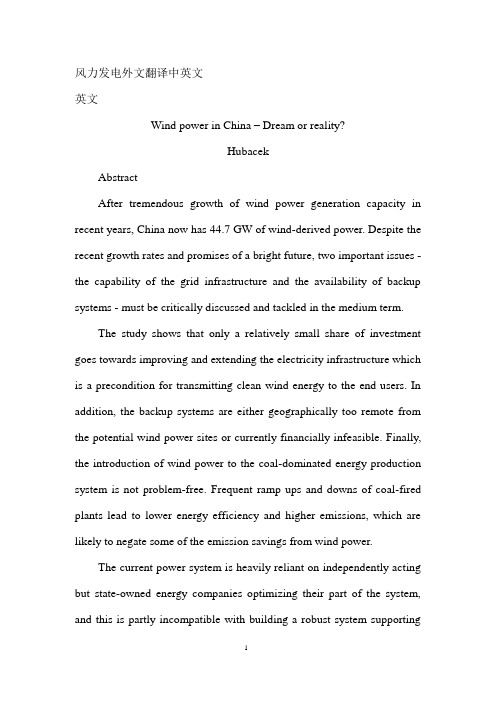
风力发电外文翻译中英文英文Wind power in China – Dream or reality?HubacekAbstractAfter tremendous growth of wind power generation capacity in recent years, China now has 44.7 GW of wind-derived power. Despite the recent growth rates and promises of a bright future, two important issues - the capability of the grid infrastructure and the availability of backup systems - must be critically discussed and tackled in the medium term.The study shows that only a relatively small share of investment goes towards improving and extending the electricity infrastructure which is a precondition for transmitting clean wind energy to the end users. In addition, the backup systems are either geographically too remote from the potential wind power sites or currently financially infeasible. Finally, the introduction of wind power to the coal-dominated energy production system is not problem-free. Frequent ramp ups and downs of coal-fired plants lead to lower energy efficiency and higher emissions, which are likely to negate some of the emission savings from wind power.The current power system is heavily reliant on independently acting but state-owned energy companies optimizing their part of the system, and this is partly incompatible with building a robust system supportingrenewable energy technologies. Hence, strategic, top-down co-ordination and incentives to improve the overall electricity infrastructure is recommended.Keywords: Wind power, China, Power grids, Back-up systems1. IntroductionChina’s wind energy industry has exper ienced a rapid growth over the last decade. Since the promulgation of the first Renewable Energy Law in 2006, the cumulative installed capacity of wind energy amounted to 44.7 GW by the end of 2010 [1]. The newly installed capacity in 2010 reached 18.9 GW which accounted for about 49.5% of new windmills globally. The wind energy potential in China is considerable, though with differing estimates from different sources. According to He et al. [2], the exploitable wind energy potential is 600–1000 GW onshore and 100–200 GW offshore. Without considering the limitations of wind energy such as variable power outputs and seasonal variations, McElroy et al. [3] concluded that if the Chinese government commits to an aggressive low carbon energy future, wind energy is capable of generating 6.96 million GWh of electricity by 2030, which is sufficient to satisfy China’s electricity demand in 2030.The existing literature of wind energy development in China focuses on several discussion themes. The majority of the studies emphasize the importance of government policy on the promotion of wind energyindustry in China [4], [5], [6], [7]. For instance, Lema and Ruby [8] compared the growth of wind generation capacity between 1986 and 2006, and addressed the importance of a coordinated government policy and corresponding incentives. Several studies assessed other issues such as the current status of wind energy development in China [9]; the potential of wind power [10]; the significance of wind turbine manufacturing [11]; wind resource assessment [5]; the application of small-scale wind power in rural areas [12]; clean development mechanism in the promotion of wind energy in China [4], social, economic and technical performance of wind turbines [13] etc.There are few studies which assess the challenge of grid infrastructure in the integration of wind power. For instance, Wang [14] studied grid investment, grid security, long-distance transmission and the difficulties of wind power integration at present. Liao et al. [15] criticised the inadequacy of transmission lines in the wind energy development. However, we believe that there is a need to further investigate these issues since they are critical to the development of wind power in China. Furthermore, wind power is not a stand-alone energy source; it needs to be complemented by other energy sources when wind does not blow. Although the viability and feasibility of the combination of wind power with other power generation technologies have been discussed widely in other countries, none of the papers reviewed thesituation in the Chinese context. In this paper, we discuss and clarify two major issues in light of the Chinese wind energy distribution process: 1) the capability of the grid infrastructure to absorb and transmit large amounts of wind powered electricity, especially when these wind farms are built in remote areas; 2) the choices and viability of the backup systems to cope with the fluctuations of wind electricity output.2. Is the existing power grid infrastructure sufficient?Wind power has to be generated at specific locations with sufficient wind speed and other favourable conditions. In China, most of the wind energy potential is located in remote areas with sparse populations and less developed economies. It means that less wind powered electricity would be consumed close to the source. A large amount of electricity has to be transmitted between supply and demand centres leading to several problems associated with the integration with the national power grid system, including grid investment, grid safety and grid interconnection.2.1. Power grid investmentAlthough the two state grid companies-(SGCC) State Grid Corporation of China and (CSG) China Southern Grid - have invested heavily in grid construction, China’s powe r grid is still insufficient to cope with increasing demand. For example, some coal-fired plants in Jiangsu, which is one of the largest electricity consumers in China, had to drop the load ratio to 60 percent against the international standard of 80percent due to the limited transmission capacity [16]. This situation is a result of an imbalanced investment between power grid construction and power generation capacity. For example, during the Eighth Five-Year Plan, Ninth Five-Year Plan and Tenth Five-Year Plan,1 power grid investments accounted for 13.7%, 37.3% and 30% of total investment in the electricity sector, respectively. The ratio further increased from 31.1% in 2005 to 45.94% in 2008, the cumulative investment in the power grid is still significantly lower than the investments in power generation [17]. Fig. 1 gives a comparison of the ratios of accumulative investments in power grid and power generation in China, the US, Japan, the UK and France since 1978. In most of these countries, more than half of the electric power investment has been made on grid construction. By contrast, the ratio is less than 40% in China.According to the Articles 14 and 21 of the Chinese Renewable Energy Law, the power grid operators are responsible for the grid connection of renewable energy projects. Subsidies are given subject to the length of the grid extension with standard rates. However, Mo [18] found that the subsidies were only sufficient to compensate for capital investment and corresponding interest but excluding operational and maintenance costs.Again, similar to grid connection, grid reinforcement requires significant amounts of capital investment. The Three Gorges power planthas provided an example of large-scale and long-distance electricity transmission in China. Similar to wind power, hydropower is usually situated in less developed areas. As a result, electricity transmission lines are necessary to deliver the electricity to the demand centres where the majority are located; these are the eastern coastal areas and the southern part of China. According to SGCC [19], the grid reinforcement investment of the Three Gorges power plants amounted to 34.4 billion yuan (about 5 billion US dollars). This could be a lot higher in the case of wind power due to a number of reasons. First, the total generating capacity of Three Gorges project is approximately 18.2 GW at this moment and will reach 22.4 GW when fully operating [20], whilst the total generating capacity of the massive wind farms amount to over 100 GW. Hence, more transmission capacities are absolutely necessary. Second, the Three Gorges hydropower plant is located in central China. A number of transmission paths are available, such as the 500 kV DC transmission lines to Shanghai (with a length of 1100 km), Guangzhou (located in Guangdong province, with a length of 1000 km) and Changzhou (located in Jiangsu province, with a length of 1000 km) with a transmission capacity of 3 GW each and the 500 kV AC transmission lines to central China with transmission capacity of 12 GW. By contrast, the majority of wind farm bases, which are located in the northern part of China, are far away from the load centres. For example, Jiuquan locatedin Gansu has a planned generation capacity of 20 GW. The distances from Jiuquan to the demand centres of the Central China grid and the Eastern China grid are 1500 km and 2500 km, respectively. For Xinjiang, the distances are even longer at 2500 km and 4000 km, respectively. As a result, longer transmission lines are required. Fig. 2 depicts the demand centres and wind farms in detail.2.2. Grid safetyThe second problem is related to grid safety. The large-scale penetration of wind electricity leads to voltage instability, flickers and voltage asymmetry which are likely to cause severe damage to the stability of the power grid [21]. For example, voltage stability is a key issue in the grid impact studies of wind power integration. During the continuous operation of wind turbines, a large amount of reactive power is absorbed, which lead to voltage stability deterioration [22]. Furthermore, the significant changes in power supply from wind might damage the power quality [23]. Hence, additional regulation capacity would be needed. However, in a power system with the majority of its power from base load provider, the requirements cannot be met easily [24]. In addition, the possible expansion of existing transmission lines would be necessary since integration of large-scale wind would cause congestion and other grid safety problems in the existing transmission system. For example, Holttinen [23] summarized the majorimpacts of wind power integration on the power grid at the temporal level (the impacts of power outputs at second, minute to year level on the power grid operation) and the spatial level (the impact on local, regional and national power grid). Besides the impacts mentioned above, the authors highlight other impacts such as distribution efficiency, voltage management and adequacy of power on the integration of wind power [23].One of the grid safety problems caused by wind power is reported by the (SERC) State Electricity Regulatory Commission [25]. In February and April of 2011, three large-scale wind power drop-off accidents in Gansu (twice) and Hebei caused power losses of 840.43 MW, 1006.223 MW and 854 MW, respectively, which accounted for 54.4%, 54.17% and 48.5% of the total wind powered outputs. The massive shutdown of wind turbines resulted in serious operational difficulties as frequency dropped to 49.854 Hz, 49.815 Hz and 49.95 Hz in the corresponding regional power grids.The Chinese Renewable Energy Law requires the power grid operators to coordinate the integration of windmills and accept all of the wind powered electricity. However, the power grid companies have been reluctant to do so due to the above mentioned problems as well as technical and economic reasons. For instance, more than one third of the wind turbines in China, amounting to 4 GW capacity, were not connectedto the power grid by the end of 2008 [17]. Given that the national grid in China is exclusively controlled by the power companies –SGCC and CSG - the willingness of these companies to integrate wind energy into the electricity generation systems is critical.2.3. The interconnection of provincial and regional power gridsThe interconnection of trans-regional power grids started at the end of 1980s. A (HVDC) high voltage direct current transmission line was established to link the Gezhouba2 dam with Shanghai which signifies the beginning of regional power grids interconnection. In 2001, two regional power grids, the North China Power Grid and Northeast China Power Grid were interconnected. This was followed by the interconnection of the Central China Power Grid and the North China Power Grid in 2003. In 2005, two other interconnection agreements were made between the South China Power Grid with North, Northeast and Central China Power Grid, and the Northwest China Power Grid and the Central China Power Grid. Finally, in 2009, the interconnection of Central China Power Grid and the East China Power Grid was made. In today’s China, the Chinese power transmission systems are composed of 330 kV and 500 kV transmission lines as the backbone and six interconnected regional power grids and one Tibet power grid [26].It seems that the interconnectivity of regional power grids would help the delivery of wind powered outputs from wind-rich regions todemand centres. However, administrative and technical barriers still exist. First, the interconnectivity among regions is always considered as a backup to contingencies, and could not support the large-scale, long-distance electricity transmission [27]. In addition, the construction of transmission systems is far behind the expansion of wind power. The delivery of large amounts of wind power would be difficult due to limited transmission capacity. Furthermore, the quantity of inter-regional electricity transmission is fixed [27]. Additional wind power in the inter-regional transmission might have to go through complex administrative procedures and may result in profit reductions of conventional power plants.3. Are the backup systems geographically available and technically feasible?Power system operators maintain the security of power supply by holding power reserve capacities in operation. Although terminologies used in the classification of power reserves vary among countries [28], power reserves are always used to keep the production and generation in balance under a range of circumstances, including power plant outages, uncertain variations in load and fluctuations in power generations (such as wind) [29]. As wind speed varies on all time scales (e.g. from seconds to minutes and from months to years), the integration of fluctuating wind power generation induces additional system balancing requirements onthe operational timescale [29].A number of studies have examined the approaches to stabilize the electricity output from wind power plants. For example, Belanger and Gagnon [30] conducted a study on the compensation of wind power fluctuations by using hydropower in Canada. Nema et al. [31] discussed the application of wind combined solar PV power generation systems and concluded that the hybrid energy system was a viable alternative to current power supply systems in remote areas. In China, He et al. [2]investigated the choices of combined power generation systems. The combinations of wind-hydro, wind-diesel, wind-solar and wind-gas power were evaluated respectively. They found that, for instance, the wind-diesel hybrid systems were used at remote areas and isolated islands. This is because the wind-diesel hybrid systems have lower generation efficiency and higher generation costs compared to other generation systems. Currently, the wind-solar hybrid systems are not economically viable for large-scale application; thus, these systems have either been used at remote areas with limited electricity demand (e.g. Gansu Subei and Qinghai Tiansuo) or for lighting in some coastal cities [2]. Liu et al. [32] adopted the EnergyPLAN model to investigate the maximum wind power penetration level in the Chinese power system. The authors derived a conclusion that approximately 26% of national power demand could be supplied by wind power by the end of 2007. However, theauthors fail to explain the provision of power reserves at different time scales due to wind power integration.Because of the smoothing effects of dispersing wind turbines at different locations (as exemplified by Drake and Hubacek [33] for the U.K., Roques [34] for the E.U. and Kempton et al. [35] for the U.S.), the integration of wind power has a very small impact on the primary reserves which are available from seconds to minutes [36]. However, the increased reserve requirements are considerable on secondary reserves (available within 10–15 min) which mainly consist of hydropower plants and gas turbine power plants [29]. Besides, the long-term reserves, which are used to restore secondary reserves after a major power deficit, will be in operation to keep power production and consumption in balance for a longer timescale (from several minutes to several hours). In the following subsection, we examine the availability of power plants providing secondary and long-term reserves and investigate the viability of energy storage system in China.中文中国的风力发电–梦想还是现实?胡巴切克摘要经过近几年风力发电能力的巨大增长,中国现在拥有44.7吉瓦的风力发电。
风能介绍外文翻译
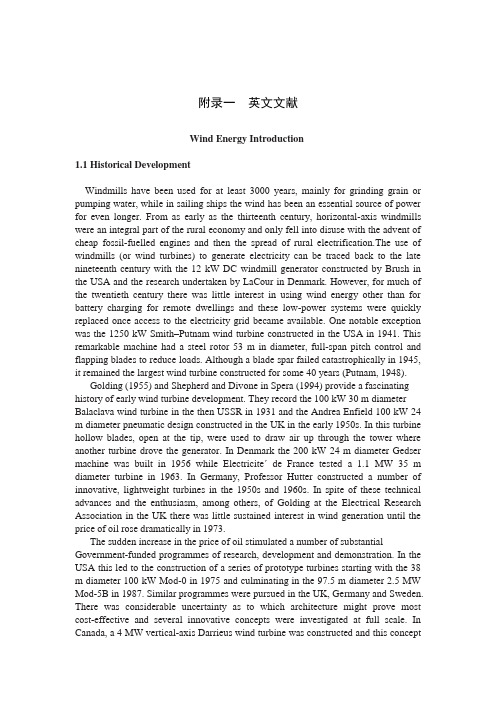
historyof early wind t00 kW30 mdiameter
附录
Wind Energy Introduction
1.1 Historical Development
Windmills have been used for at least 3000 years, mainly for grinding grain or pumping water, while in sailing ships the wind has been an essential source of power for even longer. From as early as the thirteenth century, horizontal-axis windmills were an integral part of the rural economy and only fell into disuse with the advent of cheap fossil-fuelled engines and then the spread of rural electrification.The use of windmills (or wind turbines) to generate electricity can be traced back to the late nineteenth century with the 12 kW DC windmill generator constructed by Brush in the USA and the research undertaken by LaCour in Denmark. However, for much of the twentieth century there was little interest in using wind energy other than for battery charging for remote dwellings and these low-power systems were quickly replaced once access to the electricity grid became available. One notable exception was the 1250 kW Smith–Putnam wind turbine constructed in theUSAin 1941. This remarkable machine had a steel rotor53 min diameter, full-span pitch control and flapping blades to reduce loads. Although ablade spar failed catastrophically in 1945, it remained the largest wind turbine constructed for some 40 years (Putnam, 1948).
- 1、下载文档前请自行甄别文档内容的完整性,平台不提供额外的编辑、内容补充、找答案等附加服务。
- 2、"仅部分预览"的文档,不可在线预览部分如存在完整性等问题,可反馈申请退款(可完整预览的文档不适用该条件!)。
- 3、如文档侵犯您的权益,请联系客服反馈,我们会尽快为您处理(人工客服工作时间:9:00-18:30)。
Wind Energy Introduction 1.1 Historical Development Windmills have been used for at least 3000 years, mainly for grinding grain or pumping water, while in sailing ships the wind has been an essential source of power for even longer. From as early as the thirteenth century, horizontal-axis windmills were an integral part of the rural economy and only fell into disuse with the advent of cheap fossil-fuelled engines and then the spread of rural electrification.The use of windmills (or wind turbines) to generate electricity can be traced back to the late nineteenth century with the 12 kW DC windmill generator constructed by Brush in the USA and the research undertaken by LaCour in Denmark. However, for much of the twentieth century there was little interest in using wind energy other than for battery charging for remote dwellings and these low-power systems were quickly replaced once access to the electricity grid became available. One notable exception was the 1250 kW Smith–Putnam wind turbine constructed in the USA in 1941. This remarkable machine had a steel rotor 53 m in diameter, full-span pitch control and flapping blades to reduce loads. Although ablade spar failed catastrophically in 1945, it remained the largest wind turbine constructed for some 40 years (Putnam, 1948). Golding (1955) and Shepherd and Divone in Spera (1994) provide a fascinating history of early wind turbine development. They record the 100 kW 30 mdiameter Balaclava wind turbine in the then USSR in 1931 and the Andrea Enfield 100 kW 24 m diameter pneumatic design constructed in the UK in the early 1950s. In this turbine hollow blades, open at the tip, were used to draw air up through the tower where another turbine drove the generator. In Denmark the 200 kW 24 m diameter Gedser machine was built in 1956 while Electricite´ de France tested a 1.1 MW 35 m diameter turbine in 1963. In Germany, Professor Hutter constructed a number of innovative, lightweight turbines in the 1950s and 1960s. In spite of these technical advances and the enthusiasm, among others, of Golding at the Electrical Research Association in the UK there was little sustained interest in wind generation until the price of oil rose dramatically in 1973. The sudden increase in the price of oil stimulated a number of substantial Government-funded programmes of research, development and demonstration. In the USA this led to the construction of a series of prototype turbines starting with the 38 m diameter 100 kW Mod-0 in 1975 and culminating in the 97.5 m diameter 2.5 MW Mod-5B in 1987. Similar programmes were pursued in the UK, Germany and Sweden. There was considerable uncertainty as to which architecture might prove most cost-effective and several innovative concepts were investigated at full scale. In Canada, a 4 MW vertical-axis Darrieus wind turbine was constructed and this concept was also investigated in the 34 m diameter Sandia Vertical Axis Test Facility in the