光催化分解水综述..共61页文档
光催化水分解产氢技术研究
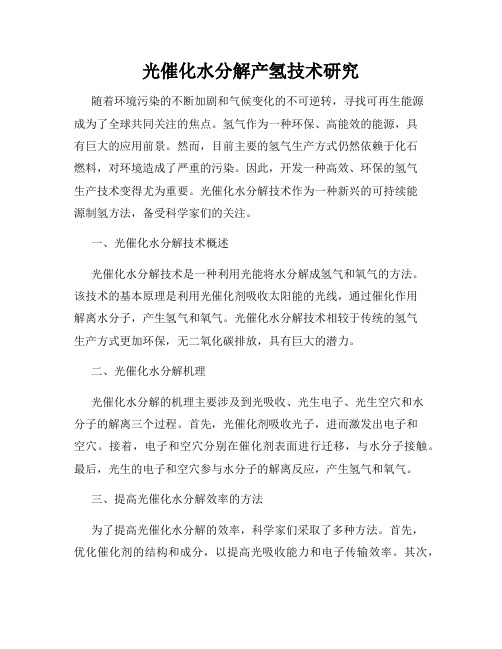
光催化水分解产氢技术研究随着环境污染的不断加剧和气候变化的不可逆转,寻找可再生能源成为了全球共同关注的焦点。
氢气作为一种环保、高能效的能源,具有巨大的应用前景。
然而,目前主要的氢气生产方式仍然依赖于化石燃料,对环境造成了严重的污染。
因此,开发一种高效、环保的氢气生产技术变得尤为重要。
光催化水分解技术作为一种新兴的可持续能源制氢方法,备受科学家们的关注。
一、光催化水分解技术概述光催化水分解技术是一种利用光能将水分解成氢气和氧气的方法。
该技术的基本原理是利用光催化剂吸收太阳能的光线,通过催化作用解离水分子,产生氢气和氧气。
光催化水分解技术相较于传统的氢气生产方式更加环保,无二氧化碳排放,具有巨大的潜力。
二、光催化水分解机理光催化水分解的机理主要涉及到光吸收、光生电子、光生空穴和水分子的解离三个过程。
首先,光催化剂吸收光子,进而激发出电子和空穴。
接着,电子和空穴分别在催化剂表面进行迁移,与水分子接触。
最后,光生的电子和空穴参与水分子的解离反应,产生氢气和氧气。
三、提高光催化水分解效率的方法为了提高光催化水分解的效率,科学家们采取了多种方法。
首先,优化催化剂的结构和成分,以提高光吸收能力和电子传输效率。
其次,改善催化剂的表面特性,增加活性位点,提高催化活性。
此外,还可以通过控制反应条件,如温度、压力和pH值等,来优化反应过程。
四、光催化水分解技术的应用前景光催化水分解技术具有广阔的应用前景。
首先,该技术可以应用于氢气生产领域,为解决能源危机和环境问题提供可持续的能源解决方案。
其次,光催化水分解技术还可以应用于光催化反应和有机合成等领域,提高反应效率,减少环境污染。
综上所述,光催化水分解技术作为一种新兴的可持续能源制氢方法,具有巨大的潜力和应用前景。
在未来的研究中,科学家们将继续努力改进催化剂设计和反应条件控制,以提高光催化水分解效率,促进其商业化应用。
相信在不久的将来,光催化水分解技术将在能源领域发挥重要作用,为构建可持续发展的社会做出贡献。
光电催化水分解的研究与应用
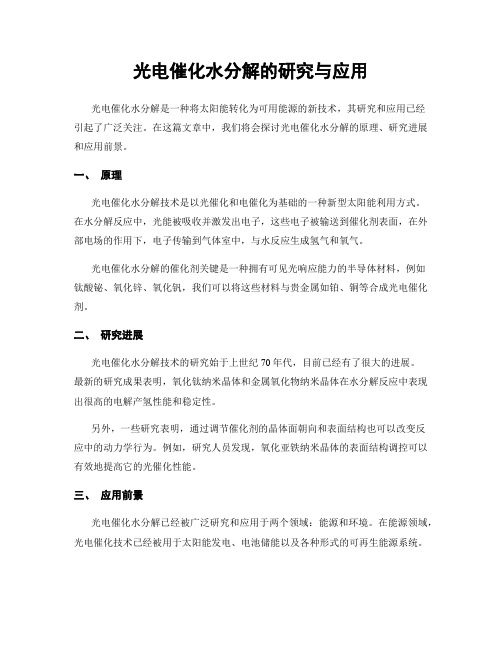
光电催化水分解的研究与应用
光电催化水分解是一种将太阳能转化为可用能源的新技术,其研究和应用已经
引起了广泛关注。
在这篇文章中,我们将会探讨光电催化水分解的原理、研究进展和应用前景。
一、原理
光电催化水分解技术是以光催化和电催化为基础的一种新型太阳能利用方式。
在水分解反应中,光能被吸收并激发出电子,这些电子被输送到催化剂表面,在外部电场的作用下,电子传输到气体室中,与水反应生成氢气和氧气。
光电催化水分解的催化剂关键是一种拥有可见光响应能力的半导体材料,例如
钛酸铋、氧化锌、氧化钒,我们可以将这些材料与贵金属如铂、铜等合成光电催化剂。
二、研究进展
光电催化水分解技术的研究始于上世纪70年代,目前已经有了很大的进展。
最新的研究成果表明,氧化钛纳米晶体和金属氧化物纳米晶体在水分解反应中表现出很高的电解产氢性能和稳定性。
另外,一些研究表明,通过调节催化剂的晶体面朝向和表面结构也可以改变反
应中的动力学行为。
例如,研究人员发现,氧化亚铁纳米晶体的表面结构调控可以有效地提高它的光催化性能。
三、应用前景
光电催化水分解已经被广泛研究和应用于两个领域:能源和环境。
在能源领域,光电催化技术已经被用于太阳能发电、电池储能以及各种形式的可再生能源系统。
在环境领域,光电催化水分解技术可以通过分解污染物来清除水污染。
同时还可以生产纳米材料、制备电极和刻写电子器件等各种应用。
总之,光电催化水分解技术是一种相对较新的技术,在科研和产业界都有广泛应用前景。
随着人们对低碳、绿色能源的需求增加,相信光电催化水分解技术将会在未来发挥越来越大的作用。
光催化分解水综述..

缺点
光激发在同一个半导体微粒上产生的电子-空穴对极易复合 不但降低了光电转换效率,同时也影响光解水同时放氢放氧
MADE BY EAST6Biblioteka 2. 半导体光催化水解制氢
2.1 半导体光解水制氢的反应历程
半导体光催化剂吸收光子,形成电子-空穴对
电荷分离并转移到表面的反应活性点上
在表面进行化学反应,从而析出氢气和氧气
MADE BY EAST
7
半导体光解水制氢的原理
以TiO2(负载Pt和RuO2)为例。TiO2为n型半导体,其 价带(VB)和导带(CB)之间的禁带宽度为3.0eV左右。当它受 到其能量相当或高于该禁带宽度的光辐照时,半导体内的电 子受激发从价带跃迁到导带,从而在导带和价带分别产生自 由电子和空穴。水在这种电子-空穴对的作用下发生电离, 生成H2和O2。 表面所负载的Pt和RuO2分别能加速自由电子 向外部的迁移,促进氢气的产生和加速空穴的迁移有利于氧 气的生成
MADE BY EAST
27
Ni掺杂改性的作用
由于层间的K+具有较好的交换特性,所以层状铌酸盐比 较容易进行改性。例如Domen等将Ni 离子引入K4Nb6O17 的 层Ⅰ中,经还原- 氧化处理后,形成的新型催化剂具有较高的 催化活性
机理 在光的作用下,Ni-O 层中生成的自由电子(e - ) 移向
BaTa2O6(Ortho) *
4.1
126
59
*水中加入少量的Ba(OH)2
MADE BY EAST
22
3.1.3 过渡金属钽酸盐
可以看出,在没有共催化剂的条件下, Activity/μmol h-1 只有 NiTa O 可以分解纯水为氢和氧;在 Catalyst 2 7 Band gap/eV 负载NiO后,ZnTa2O7也具有了光催化分 H2 O2 解水活性。而其他过渡金属钽酸盐均不 CrTaO4 2.7 2 0 能产生氧气(CuTa2O7尽管可以分解水产 MnTa2O6 3.3 0.2 0 生氧气和氢气,但产生的量太少)
光催化分解水的研究
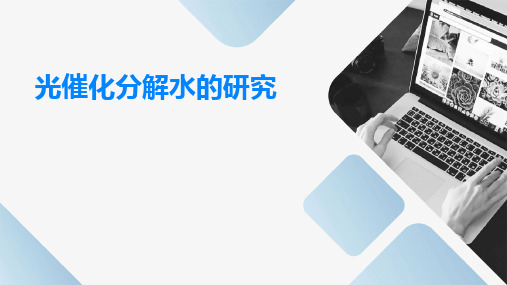
对实验数据进行统计分析,可以得出光催化分解水的反应速率、转化率和选择性等参数。这些参数可以用于评估催化剂的性能和优化光催化分解水的过程。
VS
实验结果表明,光催化分解水是一种有效的水分解方法。催化剂在光催化反应中起到关键作用,其性能直接影响水分解的效果。因此,开发高效、稳定的催化剂是光催化分解水研究的重点方向。此外,光源的选择和优化也是提高光催化分解水效率的重要因素。
催化剂的分类与选择
光催化分解水的研究现状
02
近年来,我国在光催化分解水领域取得了一系列重要进展,研究团队不断壮大,研究成果逐渐增多。国内研究者们针对光催化材料的合成、改性以及反应机理等方面进行了深入研究,取得了一系列创新性成果。
国内研究进展
在国际上,光催化分解水的研究起步较早,欧美和日本等发达国家在该领域具有较高的研究水平和丰富的经验。国外研究者们致力于开发高效、稳定的光催化材料,并探索其在工业生产中的应用前景。
光催化分解水的研究
CATALOGUE
目录
光催化分解水的基本原理 光催化分解水的研究现状 光催化分解水的应用前景 光催化分解水的未来研究方向 光催化分解水的实际案例分析
光催化分解水的基本原理
01
光催化分解水是指利用光催化剂在光照条件下将水分子分解为氧气和氢气的过程。
光催化分解水是一种可持续、环保的能源生产方式,具有高效、低成本、无污染等优点。
01
光催化与电化学的结合
利用电化学方法提高光催化分解水的效率,如光电化学池的设计与应用。
02
光催化与热力学的结合
利用热力学方法优化光催化分解水的反应过程,如热力学循环系统的建立。
光催化分解水与其他技术的结合
光催化分解水的实际案例分析
光催化分解水的经典综述:Semiconductor-based Photocatalytic Hydrogen Generation

Semiconductor-based Photocatalytic Hydrogen GenerationXiaobo Chen,*,†Shaohua Shen,†,‡Liejin Guo,‡and Samuel S.Mao†Lawrence Berkeley National Laboratory,Berkeley,California94720,United States,and State Key Laboratory of Multiphase Flow in PowerEngineering,Xi’an Jiaotong University,Xi’an,Shaanxi710049,ChinaReceived May29,2010Contents1.Introduction65032.Basic Principles of Photocatalytic HydrogenGeneration65052.1.Fundamental Mechanism of PhotocatalyticHydrogen Generation65052.2.Main Processes of Photocatalytic HydrogenGeneration65052.3.Evaluation of Photocatalytic Water Splitting65072.3.1.Photocatalytic Activity65072.3.2.Photocatalytic Stability65073.UV-Active Photocatalysts for Water Splitting65073.1.d0Metal Oxide Photocatalyts65073.1.1.Ti-,Zr-Based Oxides65073.1.2.Nb-,Ta-Based Oxides65143.1.3.W-,Mo-Based Oxides65173.1.4.Other d0Metal Oxides65183.2.d10Metal Oxide Photocatalyts65183.3.f0Metal Oxide Photocatalysts65183.4.Nonoxide Photocatalysts65184.Approaches to Modifying the Electronic BandStructure for Visible-Light Harvesting65194.1.Metal and Nonmetal Doping65194.1.1.Metal Ion Doping65194.1.2.Nonmetal-Ion Doping65294.1.3.Metal/Nonmetal-Ion Codoping65314.2.Controlling Band Structure through SolidSolutions65324.2.1.(Oxy)sulfide Solid Solutions65324.2.2.Oxide Solid Solutions65334.2.3.Oxynitride Solid Solutions65344.3.Dye Sensitization to Harvest Visible Light65354.3.1.Sensitization Using Ruthenium ComplexDyes65354.3.2.Sensitization Using Other Transition-MetalComplex Dyes65364.3.3.Sensitization Using Metal-Free Dyes65364.4.Developing Novel Single-PhaseVisible-Light-Responsive Photocatalysts65374.4.1.d-block Metal Oxides65384.4.2.p-block Metal Oxides65394.4.3.f-block Metal Oxides65404.4.4.Miscellaneous Photocatalysts65415.Approaches for Efficient Photogenerated ChargeSeparation65415.1.Cocatalyst Loading65415.1.1.Noble Metal Cocatalysts65425.1.2.Transition-Metal Oxide Cocatalysts65425.1.3.Nonmetal-Oxide Cocatalysts65435.2.Semiconductor Combinations65445.3.Modification of Crystal Structure andMorphology65475.3.1.Modification of Crystal Structure65475.3.2.Modification of Size and Morphology65486.Photocatalytic Hydrogen Generation Systems65526.1.Hydrogen Generation Systems ContainingSacrificial Reagents65526.1.1.Inorganic Sacrificial Reagent Systems6552anic Sacrificial Reagent System65556.2.Overall Water-Splitting Systems65566.2.1.Pure Water-Splitting System65566.2.2.Biomimetic Z-Scheme Water-SplittingSystem65567.Summary and Prospects65578.Acknowledgments65589.References6558 1.IntroductionBecause of its high energy capacity and environmental friendliness,hydrogen has been identified as a potential energy carrier in many low greenhouse gas(GHG)energy scenarios.1,2In a proposed hydrogen energy system,3hydrogen-containing compounds such as fossil fuels,biomass,or even water are potential sources of hydrogen.4-11When hydrogen is derived from hydrocarbons such as fossil fuels or biomass, CO2capture and sequestration are requirements in a low GHG scenario.12-14On the other hand,hydrogen produced from water does not present the challenge of unwanted emissions at the point of conversion,but it does require that energy be supplied from an external resource.15If this energy can be obtained from a renewable energy source such as solar energy,hydrogen can then be considered a green energy alternative capable of powering everything from laptops to submarines.Figure1shows a diagram of photocatalytic hydrogen generation in the hydrogen energy system.Such an approach to energy production is one that exhibits due concern for environmental issues and that is becoming increasingly relevant in our world.16,17However,the technol-ogy to produce hydrogen in a cost-effective,low-GHG manner has not yet been developed.Since the discovery of hydrogen evolution through the photoelectrochemical splitting of water on n-type TiO2*Corresponding author:Xiaobo Chen.E-mail:chenxiaobolbl@(Xiaobo Chen);shshen_xjtu@(Shaohua Shen);lj-guo@(Liejin Guo);SSMao@(Samuel S.Mao).†Lawrence Berkeley National Laboratory.‡Xi’an Jiaotong University.Chem.Rev.2010,110,6503–6570650310.1021/cr1001645 2010American Chemical SocietyPublished on Web11/10/2010electrodes,18the technology of semiconductor-based photo-catalytic water splitting to produce hydrogen using solar energy has been considered as one of the most important approaches to solving the world energy crisis.19Hence,the development of the necessary semiconductor photocatalysts has undergone considerable research.Over the past40years, many photocatalysts reportedly exhibited high photocatalytic activities for splitting water into a stoichiometric mixture of H2and O2(2:1by molar ratio)in the ultraviolet(UV)light region.These include La doped NaTaO3,20Sr2M2O7(M) Nb,Ta),21La2Ti2O7,22K2La2Ti3O10,23and -Ge3N4,24among others.Of particular note is the NiO/NaTaO3:La photocata-lyst,which shows the highest activity with a quantum yield amounting to56%at270nm.20However,these oxide photocatalysts are only active under UV irradiation.With respect to the solar spectrum,only a small fraction(ca.4%) of the incoming solar energy lies in the ultraviolet region, whereas the visible light in the solar spectrum is far more abundant(ca.46%).It is essential,therefore,as an alternative to UV-active photocatalysts to develop visible-light-driven photocatalysts that are stable and highly efficient for the practical,large-scale production of hydrogen using solar energy.Over the recent years,continuing breakthroughs have been made in the development of novel visible-light-driven photocatalysts,leading to the enhancement of photocatalytic activity for water splitting and inspiring great enthusiasm.A large number of semiconductor materials have been developed as photocatalysts for water splitting to hydrogen under visible-light irradiation.A significant process has been achieved on semiconductor-based photocatalytic hydrogen generation through waterDr.Xiaobo Chen is at Lawrence Berkeley National Laboratory.He obtained his Ph.D.Degree in Chemistry from Case Western Reserve University. His research interests include materials and devices development, renewable energy science and technology,environmental pollution,and health.Dr.Shaohua Shen is an assistant professor at Xi’an Jiaotong University, China.He obtained his Ph.D.Degree in Thermal Engineering from Xi’an Jiaotong University in2010.During2008-2009,he worked as a guest researcher at Lawrence Berkeley National Laboratory,U.S.A.His research interests include photocatalysis,photoelectrochemistry,and the related materials and devices development.Dr.Liejin Guo is a professor and the director of the State Key Laboratory of Multiphase Flow in Power Engineering in Xi’an Jiaotong University, China.He obtained his Ph.D.Degree in Engineering Thermophysics from Xi’an Jiaotong University in1989.His research interest includes multiphase flow,heat transfer,and renewable energy technologies.Dr.Samuel S.Mao is a career staff scientist at Lawrence Berkeley National Laboratory and an adjunct faculty at The University of California at Berkeley.He obtained his Ph.D.degree in Engineering from The University of California at Berkeley in2000.He is leading a multidisciplinary research team developing solar-active materials and devices and investigating fundamental energy conversion processes.Figure1.Schematic diagram of photocatalytic hydrogen genera-tion in the hydrogen energy system.6504Chemical Reviews,2010,Vol.110,No.11Chen etal.splitting over the past several decades,25-31and many excellent reviews have been published.32-62In this review, we aim to put together the research effort having been made so far,with a view of providing a good reference and inspiring new ideas for tackling this important challenge. Starting with a brief introduction to semiconductor-based photocatalysts for hydrogen generation from water splitting, we overview the development of high-efficiency,visible-light-driven photocatalysts.A number of synthetic and modification techniques for adjusting the band structure to harvest visible light and improve the charge separation in photocatalysis are discussed.Photocatalytic systems for water splitting are also reviewed and classified into two main kinds: sacrificial reagent-containing water-splitting systems and overall water-splitting systems.2.Basic Principles of Photocatalytic Hydrogen Generation2.1.Fundamental Mechanism of Photocatalytic Hydrogen GenerationIn Fujishima and Honda’s pioneering work,the electro-chemical cell they constructed for the decomposition of water into hydrogen and oxygen is shown in Figure2.18When the surface of the TiO2electrode was irradiated by UV light,as a result of a water oxidation reaction,oxygen evolution occurred at the TiO2electrode.Concomitant reduction led to hydrogen evolution at the platinum black electrode.This concept,which emerged from the use of photoelectrochemi-cal cells with semiconductor electrodes,was later applied by Bard to the design of a photocatalytic system using semiconductor particles or powders as photocatalysts.63-65 A photocatalyst absorbs UV and/or visible(Vis)light irradiation from sunlight or an illuminated light source.The electrons in the valence band of the photocatalyst are excited to the conduction band,while the holes are left in the valence band.This,therefore,creates the negative-electron(e-)and positive-hole(h+)pairs.This stage is referred to the semiconductor’s“photo-excited”state,and the energy dif-ference between the valence band and the conduction band is known as the“band gap”.This must correspond to the wavelength of the light for it to be effectively absorbed by the photocatalyst.After photoexcitation,the excited electrons and holes separate and migrate to the surface of photocatalyst. Here,in the photocatalytic water-splitting reaction,they act as reducing agent and oxidizing agent to produce H2and O2,respectively.A schematic representation of the principle of the photocatalytic system for water is depicted in Figure 3.Water splitting into H2and O2is an uphill reaction.It needs the standard Gibbs free energy change∆G0of237kJ/mol or1.23eV,as shown in eq1.Therefore,the band gap energy(E g)of the photocatalyst should be>1.23eV(<1000nm)to achieve water splitting. However,to use visible light,it should be<3.0eV(>400 nm).To facilitate both the reduction and oxidation of H2O by photoexcited electrons and holes,the match of the band gap and the potentials of the conduction and valence bands are important.Both the reduction and oxidation potentials of water should lie within the band gap of the photocatalyst. The bottom level of the conduction band has to be more negative than the reduction potential of H+/H2(0V vs normal hydrogen electrode(NHE)),whereas the top level of the valence band has to be more positive than the oxidation potential of O2/H2O(1.23V).Parts A and B of Figure4 show the conduction band edge and valence band edge of some oxide-and sulfide-based semiconductor materials.66We can see that there are many semiconductor systems whose electronic structures match well with the redox potential of water into hydrogen and oxygen molecules.The band structure requirement is a thermodynamic requirement for water splitting.Other factors,such as overpotentials,charge separation,mobility,and lifetime of photogenerated electrons and holes,affect the photocatalytic generation of hydrogen from water splitting as well.For example,the band edges of the semiconductor photocatalyst usually vary with the change of pH,as shown in Figure4C.66The phase stability of the semiconductor photocatalyst changes in different pH environments as well.2.2.Main Processes of Photocatalytic Hydrogen GenerationThe processes in the photocatalytic generation of hydrogen are illustrated in Figure5.They include light absorption of the semiconductor photocatalyst,generation of excited charges(electrons and holes),recombination of the ex-cited charges,separation of excited charges,migration of the charges,trap of excited charges,and transfer of excitedFigure2.Schematic representation of a photoelectrochemical cell (PEC).Reprinted with permission from ref18.Copyright1972 Nature Publishing Group.Figure3.Fundamental principle of semiconductor-based photo-catalytic water splitting for hydrogen generation.H2O f12O2+H2;∆G)+237kJ/mol(1)Photocatalytic Hydrogen Generation Chemical Reviews,2010,Vol.110,No.116505charges to water or other molecules.All of these proces-ses affect the final generation of hydrogen from the semi-conductor photocatalyst system.The total amount of hydrogen generated is mainly determined by the amount of excited electrons in the water/photocatalyst interface in reducing water.Apparently,any other processes that consume excited electrons should be avoided in order to maximize the efficiency of the hydrogen generation of the photocatalyst system.Any process that generates excited electrons should be considered to act in a possible way to improve the efficiency.Thus,if we look atthe charge-generation process,the semiconductor photocata-lyst should first have a low band gap to absorb as much light as possible,and reflection or scattering of light by the photocatalyst should be minimized.Second,using the absorbed photons,the semiconductor photocatalyst should have a high efficieny in generating excited charges,instead of generating phonons or heat.After excited charges are created,charge recombination and separation/migration processes are two important com-petitive processes inside the semiconductor photocatalyst that largely affect the efficiency of the photocatalytic reaction for water splitting.67Charge recombination reduces the excited charges by emitting light or generating phonons.It includes both surface and bulk recombination and is classified as a deactivation process,and it is ineffective for water splitting.The separation of excited electrons and holes sometimes may need to overcome an energy barrier,which is the sometimes binding energy of the excited electron -hole pairs,excitons.Charge separation and migration,on the other hand,is an activation process.This is as a result of the charges being on the surface of the photocatalyst ready for the desired chemical reaction.It is beneficial for hydrogen generation through water splitting.Efficient charge separation and fast charge transport,avoiding any bulk/surface charge recombination avoided,are fundamentally important for photocatalytic hydrogen generation through water splitting.Any approach beneficial to the charge separation and transport should be taken into account such as design of internal-built electric field and use of high photoconductive semiconductor materials.The reaction of photogenerated H 2and O 2to form H 2O on the photocatalyst surface is normally called “surface back-reaction (SBR)”.It will inevitably have a negative effect on any enhancement of the photocatalytic activity,because it reduces the amount of H 2emitted from the photocatalyst.There are two main approaches to suppress SBR:one involves the addition of sacrificial reagents into the photo-catalytic reaction environment and the second creates a separation of the photoactive sites on the surface of the photocatalysts.In general,the electron donor and acceptor sacrificial reagents that are added work as an external driving force for the surface chemical reaction and depress the H 2O formation from H 2and O 2.The separation of the photoactive sites necessary for hydrogen and oxygen evolution,andFigure4.Calculated energy positions of conduction band edges and valence band edges at pH 0for selected metal oxide (A)and metal sulfide (B)semiconductors.The bottom of open squares represent conduction band edges,and the top of solid squares represent valence band edges.The solid lines indicate water stability limits.(C)pH dependence of the conduction band edge and valence band edge in an aqueous electrolyte solution.Reprinted with permission from ref 66.Copyright 2000The Mineralogical Society of America.Figure 5.Processes in photocatalytic water splitting.Reprinted with permission from ref 67.Copyright 1995American Chemical Society.6506Chemical Reviews,2010,Vol.110,No.11Chen et al.which is always accompanied by the surface separation ofthe photogenerated electrons and holes,has been shown tobe greatly affected by the surface properties of the photo-catalysts.As well as the surface reaction sites themselves,the surface states and morphology also play an importantrole.Taking into consideration the basic mechanism andprocesses of photocatalytic water splitting,there are two keysto developing a suitable high-efficiency semiconductor forthe visible-light-driven photocatalytic splitting of water intoH2and/or O2:(1)A photocatalyst should have a sufficientlynarrow band gap(1.23eV<E g<3.0eV)to both harvest visible light and possess the correct band structure.(2)Photoinduced charges in the photocatalyst should be sepa-rated efficiently in order to avoid bulk/surface electron/holerecombination.In addition,they must migrate to the pho-tocatalyst surface for hydrogen and/or oxygen evolution at the respective photocatalytic active sites.58In summary,it is generally accepted that the correct band structure for efficient visible-light harvesting and effective separation between the photoexcited electrons and the holes is essential to improve the photocatalytic properties of the semiconduc-tor.In addition,with the development of efficient visible-light-driven photocatalysts,it is important that economical, highly efficient photocatalytic systems for light-to-hydrogen energy conversion,in which aqueous solutions containing sacrificial reagents can be used to depress the backward reaction of hydrogen and oxygen to water on the surface of photocatalysts,can be constructed.2.3.Evaluation of Photocatalytic Water Splitting There are two apparent indicators that should be paid attention in evaluating the hydrogen generation through photocatalytic water splitting.One is photocatalytic activity, and the other one is photocatalytic stability.2.3.1.Photocatalytic ActivityThe efficiency of photocatalytic hydrogen generation from water splitting can be measured directly on the amount of hydrogen gas evoluted or indirectly on the electrons trans-ferred from semiconductor to water within a certain time period under light irradiation.Different photocatalytic setup configurations,such as inner irradiation type and top irradia-tion type,and light sources,such as Xe lamp and Hg lamp, are commonly used by different research groups and scientists,which may give different rates of gas evolution when exactly the same photocatalyst is used.This makes it difficult to make direct comparison across the results from different research groups and photocatalytic hydrogen gen-eration systems.Nevertherless,it seems helpful to get approximate correlations between various results if we normalize the rates of gas evolution to the amount of photocatalyst employed within a unit of time.Here,we use the rate of gas(O2and H2)evolution with units such as µmol·h-1andµmol·h-1·g-1catalyst to make the mensurable comparison between different photocatalysts under similar experimental conditions.The(apparent)quantum yield,as an extension from the overall quantum yield in a homogeneous photochemical system,becomes important and acceptable to evaluate the photocatalytic activity for water splitting.The overall quantum yield and apparent quantum yield are defined by eqs2and3,respectively.68The apparent quantum yield is estimated to be smaller than the total quantum yield because the number of absorbed photons is usually smaller than that of incident light.In addition to the quantum yield,the solar energy conversion efficiency that is usually used for evaluation of solar cells is also sometimes reported in the literature.It is defined as 2.3.2.Photocatalytic StabilityAs a good photocatalyst,it should have a good stability for H2and/or O2production,besides a high photocatalytic activity or quantum yield.To test the photocatalytic stability, a long-time experiment or a repeated experiment is always necessary.Photocorrosion is considered to be the main reason causing the poor stability of photocatalysts,especially the metal sulfide photocatalysts.CdS has frequently been reported to be unstable for photocatalytic H2evolution.S2-in CdS rather than water is self-oxidized by photoinduced holes in the valence band of CdS.The photocorrosion reaction occurs as in eq5,553.UV-Active Photocatalysts for Water SplittingA wide range of semiconducting materials have been developed as photocatalysts for use under UV irradiation. These are shown in Table1.On the basis of their electronic configuration properties,these UV-active photocatalysts can be typically classified into four groups:(1)d0metal(Ti4+, Zr4+,Nb5+,Ta5+,W6+,and Mo6+)oxide photocatalysts,(2) d10metal(In3+,Ga3+,Ge4+,Sn4+,and Sb5+)oxide photo-catalysts,(3)f0metal(Ce4+)oxide photocatalysts,and(4)a small group of nonoxide photocatalysts.3.1.d0Metal Oxide Photocatalyts3.1.1.Ti-,Zr-Based OxidesTiO2is thefirst reported photocatalyst for water splitting under UV irradiation.69TiO2can produce hydrogen and/or oxygen from water vapor,pure water,and aqueous solutions containing electron donor.70-77It was found that,under UV irradiation,colloidal TiO2combined with ultrafine Pt and RuO2particles generated H2with a high quantum yield of 30(10%and O2in stoichiometric proportions from water.70 The reaction solution had a pH of1.5,which was adjusted Overall quantum yield(%))Number of reacted electronsNumber of absorbed photons×100%(2)(Apparent)Quantum yield(QY,%))Number of reacted electronsNumber of incident photons×100%)2×Number of evolved H2moleculesNumber of incident photons×100%(for H2evolution))4×Number of evolved O2moleculesNumber of incident photons×100%(for O2evolution)(3)Solar energy conversion(%))Output energy of hydrogen evolvedEnergy of incident solar light×100(4)CdS+2h+f Cd2++S(5)Photocatalytic Hydrogen Generation Chemical Reviews,2010,Vol.110,No.116507T a b l e 1.U V -L i g h t -A c t i v e P h o t o c a t a l y s t s f o r W a t e r S p l i t t i n g t o H y d r o g e n a n d /o r O x y g e n a c t i v i t y (µm o l ·h -1·g -1)p h o t o c a t a l y s t s y n t h e t i c m e t h o dm a s s (g )l i g h t s o u r c e i n c i d e n t l i g h t a q u e o u s r e a c t i o n s o l u t i o n c o -c a t a l ./H 2c o -c a t a l ./O 2Q Y (%)r e f e r e n c eT i O 2(a n a t a s e )M C B T i O 20.3500-W H g b250-400n m w a t e r v a p o r R h /149729(340n m )74T i O 2(a n a t a s e )M C B T i O 21450-W H g q u a r t z fil t e r N a O H N i O x /32N i O x /1475T i O 2(a n a t a s e ,78%)P 25T i O 20.3400-W H g q u a r t z fil t e r N a 2C O 3P t /1893P t /95771T i O 2(a n a t a s e ,78%)P 25T i O 20.3250-W H g P y r e x fil t e r p u r e w a t e r P t /353P t /1771.4(300-400n m )76T i O 2h y d r o l y s i s ,c a l c i n a t i o n 0.2300-W X e cP y r e x fil t e r C H 3O H P t /∼330072r u t i l e /a n a t a s e T i O 2i m p r e g n a t i o n ,c a l c i n a t i o n 0.2300-W X e P y r e x fil t e r C H 3O H P t /∼670073c o l l o i d T i O 2h y d r o l y s i s r e a c t i o n 0.025450-W H g P y r e x fil t e r H C l P t -R u O 2/400030(310n m )70m e s o p o r o u s T i O 2s o l g e l m e t h o d 0.2300-W H g P y r e x fil t e r C H 3O H P t /6925889,934,941T i O 2n a n o w i r e s e l e c t r o s p i n n i n g a n d s o l -g e l 1450-W H g P y r e x fil t e r C H 3O H 54964,975T i O 2n a n o t u b e s h y d r o t h e r m a l m e t h o d 1450-W H g P y r e x fil t e r C H 3O H 285966T i O 2n a n o s h e e t s h y d r o t h e r m a l m e t h o d 1450-W H g P y r e x fil t e rC H 3O H 117.61003N i -i n t e r c a l a t e d N a 2T i 2O 5n a n o t u b e s h y d r o t h e r m a l m e t h o d 0.1450-W H g C H 3O H P t /∼85099T i O 2:G a s o l v o t h e r m a l m e t h o d 1.5U V -l a m p s P y r e x fil t e r P u r e w a t e r 20.8679T i O 2:N i h y d r o t h e r m a l m e t h o d 0.3300-W H g P y r e x fil t e r C H 3O H P t /∼566.780T i O 2:S c h y d r o l y s i s ,c a l c i n a t i o n 0.1300-W H g P y r e x fil t e r C H 3O H P t /∼750082T i O 2:Y h y d r o l y s i s ,c a l c i n a t i o n 0.1300-W H g P y r e x fil t e r C H 3O H P t /∼780082T i O 2:L a h y d r o l y s i s ,c a l c i n a t i o n 0.1300-W H g P y r e x fil t e r C H 3O H P t /∼768082T i O 2:C e h y d r o l y s i s ,c a l c i n a t i o n 0.1300-W H g P y r e x fil t e r C H 3O H P t /∼480082T i O 2:P r h y d r o l y s i s ,c a l c i n a t i o n 0.1300-W H g P y r e x fil t e r C H 3O H P t /∼660082T i O 2:N d h y d r o l y s i s ,c a l c i n a t i o n 0.1300-W H g P y r e x fil t e r C H 3O H P t /∼930082T i O 2:S m h y d r o l y s i s ,c a l c i n a t i o n 0.1300-W H g P y r e x fil t e r C H 3O H P t /∼1020082T i O 2:G d h y d r o l y s i s ,c a l c i n a t i o n 0.1300-W H g P y r e x fil t e r C H 3O H P t /∼1080082T i O 2:E u h y d r o l y s i s ,c a l c i n a t i o n 0.1300-W H g P y r e x fil t e r C H 3O H P t /∼1320082T i O 2:T b h y d r o l y s i s ,c a l c i n a t i o n 0.1300-W H g P y r e x fil t e r C H 3O H P t /∼480082T i O 2:D y h y d r o l y s i s ,c a l c i n a t i o n 0.1300-W H g P y r e x fil t e r C H 3O H P t /∼840082T i O 2:H o h y d r o l y s i s ,c a l c i n a t i o n 0.1300-W H g P y r e x fil t e r C H 3O H P t /∼1020082T i O 2:E r h y d r o l y s i s ,c a l c i n a t i o n 0.1300-W H g P y r e x fil t e r C H 3O H P t /∼840082T i O 2:T m h y d r o l y s i s ,c a l c i n a t i o n 0.1300-W H g P y r e x fil t e r C H 3O H P t /∼870082T i O 2:Y b h y d r o l y s i s ,c a l c i n a t i o n 0.1300-W H g P y r e x fil t e r C H 3O H P t /∼840082T i O 2:L u h y d r o l y s i s ,c a l c i n a t i o n 0.1300-W H g P y r e x fil t e r C H 3O H P t /∼720082T i O 2:S n /E u p o l y o l m e t h o d 0.2288-WF dq u a r t z fil t e r C H 3O H P d /∼9240.481T i O 2/C u x O i m p r e g n a t i o n m e t h o d 1400-W H g q u a r t z fil t e r C H 3O H 1850085-87T i O 2/S n O 2p o l y o l m e t h o d 0.05288-W F q u a r t z fil t e r C H 3O H P d /∼8383A g x O /T i O 2i m p r e g n a t i o n m e t h o d 0.05s o l a r l i g h t q u a r t z fil t e rC H 3O H 6700088-90S r T i O 3/T i O 2s o l i d -s t a t e r e a c t i o n 0.1150-W H g H C O O H 56091B /T i o x i d e s o l -g e l m e t h o d 0.3400-W H g q u a r t z fil t e r p u r e w a t e r P t /73P t /36.792,93m e s o -T i O 2/Z r O 2e v a p o r a t i o n i n d u c e d s e lf -a s s e m b l y p r o c e s s 0.06500-W Hg P y r e x fil t e r C H 3O H P t /∼2484C a T i O 3S a k a i C h e m i c a l I n d u s t r y 0.5500-W H g s i l i c a g l a s s N a O H P t /76P t /18120S r T i O 3A l f a -V e n t r o n 0.5400-W H g P y r e x fil t e r N a O H N i O x /∼70N i O x /∼32109-114S r T i O 3:L a s o l i d -s t a t e r e a c t i o n 0.1400-W H g q u a r t z fil t e r N a 2C O 3C o O x /∼2800C o O x /∼1300116S r T i O 3:T a s o l i d -s t a t e r e a c t i o n 0.3450-W H g P y r e x fil t e r p u r e w a t e r R h x C r 2-x O 3/∼14160R h x C r 2-x O 3/∼7000117S r T i O 3:N a s o l i d -s t a t e r e a c t i o n 0.3450-W H g P y r e x fil t e r p u r e w a t e r R h x C r 2-x O 3/∼22220R h x C r 2-x O 3/∼11110117S r 3T i 2O 7p o l y m e r i z e d c o m p l e x m e t h o d 1400-W H g q u a r t z fil t e r p u r e w a t e r N i O x /144N i O x /72118S r 4T i 3O 10p o l y m e r i z e d c o m p l e x m e t h o d 1400-W H g q u a r t z fil t e r p u r e w a t e r N i O x /1704.5(360n m )119K 2L a 2T i 3O 10p o l y m e r i z e d c o m p l e x m e t h o d 1450-W H g q u a r t z fil t e r K O H N i O x /2186N i O x /113123,135,139R b 2L a 2T i 3O 10s o l i d -s t a t e r e a c t i o n 1450-W H g q u a r t z fil t e r R b O H N i O x /869N i O x /4305(330n m )135R b 1.5L a 2T i 2.5N b 0.5O 10s o l i d -s t a t e r e a c t i o n 1450-W H g q u a r t z fil t e r R b O H N i O x /725N i O x /358135R b L a 2T i 2N b O 10s o l i d -s t a t e r e a c t i o n 1450-W H g q u a r t z fil t e r R b O H N i O x /79N i O x /30135C s 2L a 2T i 3O 10s o l i d -s t a t e r e a c t i o n 1450-W H g q u a r t z fil t e r p u r e w a t e r N i O x /700N i O x /340135C s 1.5L a 2T i 2.5N b 0.5O 10s o l i d -s t a t e r e a c t i o n 1450-W H g q u a r t z fil t e r p u r e w a t e r N i O x /540N i O x /265135C s L a 2T i 2N b O 10s o l i d -s t a t e r e a c t i o n 1450-W H g q u a r t z fil t e r p u r e w a t e r N i O x /115N i O x /50135L a T i O 3s o l i d -s t a t e r e a c t i o n 1450-W H g q u a r t z fil t e r p u r e w a t e r N i O x /137125L a 2T i O 5s o l i d -s t a t e r e a c t i o n 1450-W H g q u a r t z fil t e r p u r e w a t e r N i O x /442125L a 2T i 3O 9s o l i d -s t a t e r e a c t i o n 1450-W H g q u a r t z fil t e r p u r e w a t e r N i O x /386125L a 4T i 3O 12p o l y m e r i z e d c o m p l e x m e t h o d 0.5400-W H g q u a r t z fil t e r p u r e w a t e r N i O /714N i O /358133L a 2T i 2O 7p o l y m e r i z e d c o m p l e x m e t h o d 1450-W H g q u a r t z fil t e r p u r e w a t e r N i O x /960N i O x /4782722,122-127,131,134L a 2T i 2O 7:S r s o l i d -s t a t e r e a c t i o n 1450-W H g q u a r t z fil t e rp u r e w a t e r N i O x /15101256508Chemical Reviews,2010,Vol.110,No.11Chen et al.。
光催化分解水体系概述
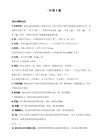
催化分解水的基本原理。
锐钛矿型的Ti02其价带到导带的禁带宽度约为3.2eV,当受到光子能量等于或高于禁带宽度的光辐照时,其价带上的电子(e一)就会受激跃迁至导带,在价带上产生相应的空穴(h+),形成了电子一空穴对。
产生的电子、空穴在内部电场作用下分离并迁移到粒子表面。
光生空穴有很强的得电子能力,具有强氧化性,可夺取半导体颗粒表面被吸附物质或溶剂中的电子,使原本不吸收光的物质被氧化,电子受体则通过接受表面的电子而被还原,完成光催化反应过程,如图1所示[¨:图1光催化分解水的基本过程模型①半导体光催化剂吸收能量足够大的光子,产生电子一空穴对;⑦电子一空穴对分离,向半导体光催化剂表面移动;③电子与水反应产生氢气I④空穴与水反应产生氧气I⑤部分电子与空穴复合,产生热或光。
光催化分解水反应式可写为(以Ti02为例)‘“l2Ti02+2hv—・2Ti02+2h++2e一(1)2e一+2H+—・H’+H‘一H2(Z)2h++2H20一2H。
o+一20H’+2H+(3)oH‘+oH‘—,H:o+1/202(4)但也并不是所有的半导体光催化剂都能作为光分解水的催化剂,必须满足一定的氧化还原化学反应条件,即首先其禁带宽度要大于水的分解电压(理论值1.23eV),且由于超电压的存在,半导体材料的禁带宽度要大于水的分解电压,其次,半导体光催化剂的价带位置应比O。
/H。
o的电位更正,而导带的位置应比H+/H。
更负,最合适的禁带宽度应为2.0eV左右‘…。
3光催化分解水体系自从Fujishima和Honda发现可以利用二氧・128・化钛(Ti02)光催化分解水制备氢气和氧气以来,各国学者一直致力于光催化分解水的研究,并在高效光催化剂的研究方面取得了重要进展,开发了为数众多的光催化剂o]。
目前,光催化分解水的评价体系主要是粉末直接光照的水溶早匝悬浮反应体系和光电化学体系。
其中,粉末悬浮作为光催化分解水制氢的反应体系可用来评价半导体光催化剂的许多性质,如导带与价带的位置、禁带宽度、材料本身在水溶液中的稳定性等。
cof光催化全分解水

cof光催化全分解水
COF光催化全分解水是一种利用共轭有机框架材料(COF)
作为光催化剂,在光照条件下将水分解为氧气和氢气的过程。
光催化全分解水的过程是通过吸收光能,激发电子从COF中
跃迁到导带带底部,形成电子空穴对。
电子和空穴对的形成促使水分子发生光解反应,将水分解为氧气和氢气。
COF作为光催化剂具有很多优势。
首先,COF具有高特异表
面积和可控的孔结构,有利于提供更多活性位点,并增加光吸收量。
其次,COF材料通常具有良好的光稳定性和光学性能,能够有效地利用太阳光进行光催化反应。
此外,COF材料具
有可调节的化学组成和结构,可以通过合成方法的调控来改变催化性能。
虽然COF光催化全分解水技术在实验研究中取得了一定的进展,但目前仍面临一些挑战。
首先,COF材料的合成和制备
方法需要进一步优化,以提高光催化性能和稳定性。
其次,COF材料的光吸收范围还需要拓宽,使其能够吸收更多的可
见光谱范围。
此外,还需要解决光催化过程中产生的副产物问题,以提高水分解的效率和选择性。
总体来说,COF光催化全分解水是一种具有潜力的技术,可
以为可持续能源的应用提供新的途径。
然而,还需要进一步的研究和开发,以克服现有的挑战并实现实际应用。
光催化研究发展综述性报告

光催化研究发展综述性报告本人申请攻读动力工程与工程热物理专业博士学位,由于对后续能源与新能源技术专业太阳能分解水制氢方向有浓厚的兴趣,通过对相关文献的阅读和参加相关报告,对太阳能光催化分解水制氢有了详细的了解,对其发展简述如下:/ 、八1.前言当今人类社会面临能源和环境两大问题[1-2]。
能源的短缺和环境的污染严重制约着人类社会的发展。
一方面,社会的高速发展使得人类对于能源的需求越来越大,而我们目前所用的能源还是以传统的化石燃料为主,但是因为化石燃料的不可再生性,或者说是形成的时间周期太长,使得其必有枯竭的一天。
据估计,按照目前的开采水平和消耗量,石油还能够维持四十年左右,煤炭最多也就是两百年,而天然气还可以维持大概六十多年。
另一方面,化石燃料的燃烧,引起严重的环境污染和对环境的危害,如温室效应、酸雨、光化学烟雾等等,对人类的生存产生了严重的威胁。
研究自然的、社会的、生态的、经济的以及利用自然资源过程中的基本关系,以确保全球的可持续发展已经成为各国都十分关注的一个话题。
就像美国,在2009 年提出的7870 亿美元的巨额经济刺激计划中,把发展新能源定位于抢占未来发展制高点的重要战略产业,并提出在未来的三年的时间里,国内可再生能源产量要增加一倍。
而我国人口众多,常规能源储备远低于世界平均水平,而且近几十年来,环境污染也是日益严峻。
这使得寻找一种清洁可持续的替代能源变得更加迫切。
而我国幅员辽阔,拥有极为丰富的太阳能资源,开发潜力巨大,从长远发展来看完全可以满足国家可持续发展的需求。
但太阳能能量密度低、分散性强、不稳定、不连续的缺点使得我们至今仍缺乏对其高效低成本大规模利用的有效手段。
但是考虑到占地表约3/4 的水域和植物的光合作用,我们是不是可以利用太阳能分解水,制取氢气,而氢气又是是一种无色无臭无味无毒的清洁燃料,具有储能密度高、无污染、易于与电能相互转换等优点,被视为一种最为理想的替代能源。
1972年,日本学者Fujishima和Honda[3]对光照TiO?电极导致水分解产生氢气的发现,使得太阳能转化为氢能成为了现实,也为利用太阳能过程中各种困难的解决提供了一个理想的途径。