席夫碱金属SOD模型配合物
席夫碱金属配合物

Binucleating Ligands:Synthesis of Acyclic Achiral and Chiral Schiff Base-Pyridine and Schiff Base-Phosphine LigandsFung Lam,Jia Xi Xu,and Kin Shing Chan*Department of Chemistry,The Chinese University of Hong Kong,Shatin,Hong KongReceived May31,1996X5-tert-Butyl-3-(2′-pyridyl)salicyaldehyde and5-tert-butyl-3-(diphenylphosphino)salicyaldehyde were synthesized from4-tert-butylphenol in good overall yields.Condensation of the salicyaldehydes with2,3-diamino-2,3-dimethylbutane afforded the desired dinucleating Schiff base-pyridine and Schiff base-phosphine ligands,respectively.5-tert-Butyl-3-(2′-pyridyl)salicyaldehyde reacted with optically active1,2-diaminocyclohexanes to give chiral dinucleating Schiff base-pyridine ligands.IntroductionThe synthesis of dinucleating ligands capable of bind-ing metal ions in close proximity has continued to arouseinterest among chemists.Since1970,1,2the preparationof dinucleating ligands plays a central role in bimetallicchemistry which is currently the area of extensiveinvestigation due to its importance in the fields ofbioinorganic chemistry,homogeneous catalysis,and mag-netic exchange processes.3The design of dinucleatingsystem determines the nature of metal ions to be incor-porated,coordination environment,and metal-metal separation of the bimetallic complexes.Several kinds of dinucleating ligands have been reported1,2to afford binuclear complexes.Previous efforts have been focused on the synthesis and complexation of a vast array of symmetrical dinucleating macrocycles due to the relative ease of their synthesis.4However,this kind of ligand is usually more useful in the preparation of homobimetallic complexes while the synthesis of heterobimetallic com-plexes is better accomplished by using the heteroditopic ligands.5An important category of the heteroditopic ligands is the Schiff base-crown ether macrocyclic com-pounds.6The Schiff base-crown ether ligands have been demonstrated to complex soft and hard metal ions in their soft(Schiff base)and hard(crown ether)cavities.Another type is the acyclic Schiff base-carboxylic acids which were reported to complex soft and hard metal ions selectively.7While the aforementioned heteroditopic ligands bear hard binding sites coupled to the Schiff base moieties,here we report the synthesis of achiral and chiral acyclic side-off Schiff base-pyridine and Schiff base-phosphine ligands containing the relatively softer pyridyl and phosphino groups which may allow complex-ation of low valent transition metal ions.8The general structure of the dinucleating ligands is shown in Figure 1.As shown in Figure1,in these phenolic bridged dinucleating ligands,the Schiff base moieties provide the internal salen type N2O2cavity while the functional groups X serve as the extra binding ends to constitute the external O2X2donor set.Schiff base ligands are well known to afford stable transition metal complexes,9and their monometallic chemistry has been extensively stud-ied.10The dinucleating properties of our Schiff base system are attributed to the donor groups X(X) 2-pyridyl or diphenylphosphino)at3′-positions giving rise to the external O2X2binding sites.While the O2N2sites of the pyridyl derivative are expected to bind first row transition metal ions,the O2P2system of the Schiff base-phosphine ligand can complex the softer second and third row transition metal ions as well.11In order to enhance the solubility of the ligands and their bimetallic com-plexes in organic solvents,the lipophilic tert-butyl groups and the tetramethylethylene bridge are introduced in ourX Abstract published in Advance ACS Abstracts,November1,1996.(1)(a)Casellato,U.;Vigato,P.A.;Vidali,M.Coord.Chem.Rev. 1977,23,31.(b)Casellato,U.;Vigato,P.A.;Fenton,D.E.;Vidali,M. Chem.Soc.Rev.1979,8,199.(c)Lehn,J.M.Pure Appl.Chem.1980, 52,2441.(2)(a)Zanello,P.;Tamburini,S.;Vigato,P.A.;Mazzocchin,G.A. Coord.Chem.Rev.1987,77,165.(b)Vigato,P.A.;Tamburini,S.; Fenton,D.E.Coord.Chem.Rev.1990,106,25.(c)Fraser,C.;Johnston, L.;Rheingold,A.L.;Haggerty,B.S.;Williams,G.K.;Whelan,J.; Bosnich,B.Inorg.Chem.1992,31,1835.(d)van Veggel,F.C.J.M.; Verboom,W.;Reinhoudt,D.N.Chem.Rev.1994,94,279.(3)(a)Fenton,D.E.,in A.G.Sykes(Ed.)Advances in Inorganic and Bioinorganic Mechanisms;Sykes,A.G.,Ed.;Academic Press: London,1983;Vol.2.(b)Karlin,K.D.;Zubieta,J.Biological and Inorganic Copper Chemistry;Adenine Press:Guilderland,NY,1986; Vols.1,2.McKenzie,C.J.;Robson,R.J.Chem.Soc.,mun. 1988,112.(c)Willett,R.D.;Gatteschi,D.;Kahn,O.Magneto-structural Correlations in Exchange Coupled Systems;Nato ASI Series,D.ReidelPublishing Co.:Dordrecht,1983.(4)(a)Himmelsbath,M.;Lintvedt,R.L.;Zehetmair,J.K.;Nanny, M.;Heeg,M.G.J.Am.Chem.Soc.1987,109,8003.(b)Nelson,S.M. Pure Appl.Chem.1980,52,2461.(c)Atkins,J.A.;Black,D.;Blake, A.J.;Marin-Becerra,A.;Parsons,S.;Ruiz-Ramirez,L.;Schro¨der,M. J.Chem.Soc.,mun.1996,457.(5)Lehn,J.M.Pure Appl.Chem.1980,52,2441.(6)(a)van Veggel,F.C.J.M.;Harkema,S.;Bos,M.;Verboom,W.; van Staveren,C.J.;Gerritsma,G.J.;Reinhoudt,D.N.Inorg.Chem. 1989,28,1133.(b)van Staveren,C.J.;van Eerden,J.;van Veggel,F.C.J.M.;Harkema,S.;Reinhoudt,D.N.J.Am.Chem.Soc.1988,110, 4994.(c)van Veggel,F.C.J.M.;Bos,M.;Harkema,S.;Verboom,W.; Reinhoudt,D.N.Angew.Chem.,Int.Ed.Engl.1989,28,746.(7)(a)Torihara,N.;Okawa,H.;Kida,S.Inorg.Chim.Acta.1976, 26,97.(b)Okawa,H.;Tanaka,M.;Kida,S.Chem.Lett.1974,987.(c) Vidali,M.;Vigato,P.A.;Casellato,U.Inorg.Chim.Acta.1976,17, L5.(d)Okawa,H.;Nishida,Y.;Tanaka,M.;Kida,S.Bull.Chem.Soc. Jpn.1977,50,127.(8)Lockemeyer,J.R.;Rheingold,A.L.;Bulkowski,J,ano-metallics1993,12,256.(9)Dabrowska,D.;Rzoska,W.Acta Pol.Pharm.1978,35,69.(10)Hobday,M.D.;Smith,T.D.Coord.Chem.Rev.1972-73,9, 311.(11)(a)Dunbar,K.R.;Quilleve´re´,anometallics1993,12,618.(b)Soulivong,D.;Matt,D.;Ziessel,R.Tetrahedron Lett.1993,34,1151. Figure1..Chem.1996,61,8414-8418S0022-3263(96)01020-1CCC:$12.00©1996American Chemical Societyligand design.1,2-Diaminocyclohexanes,readliy avail-able chiral diamines,are employed for the synthesis of chiral ligands.The class of chiral dinucleating ligands is especiallyinteresting since the asymmetric induction coupled with bimetallic cooperativity in a multiple redox reaction for these heterobimetallic complexes would be promising biomimetics as well as potential asymmetric catalysts such as aerobic oxidation catalysts.12We now disclose our full results in the synthesis of acyclic achiral and chiral Schiff base-pyridine and achiral Schiff base-phosphine ligands.13Results and DiscussionThe dinucleating ligands7and13were prepared by the condensation of2,3-diamino-2,3-dimethylbutane and optically active1,2-diaminocyclohexanes with5-tert-butyl-3-(2′-pyridyl)salicyaldehyde and5-tert-butyl-3-(diphenylphosphino)salicyaldehyde which were synthe-sized according to Schemes1and2.As shown in Scheme1,the ligand7was synthesized from4-tert-butylphenol in four steps in50%overall yield. Formylation of4-tert-butylphenol with formaldehyde14in the presence of SnCl4afforded the salicyaldehyde2which was then brominated with Br2/AcOH15to give3-bromo-5-tert-butylsalicyaldehyde(3)in95%yield.Stille type palladium-catalyzed cross coupling16of3with2-(tri-n-butylstannyl)pyridine17in THF produced5-tert-butyl-3-(2′-pyridyl)salicyaldehyde(5)in93%yield.Condensation of5with2,3-diamino-2,3-dimethylbutane18afforded the desired dinucleating ligand7in76%yield.Similarly,ligand13was prepared by the condensation reaction with5-tert-butyl-3-(diphenylphosphino)salicy-aldehyde(11)in six steps starting from4-tert-butylphenol as shown in Scheme2.Bromination of4-tert-butylphenol (1)gave a96%yield of the dibromophenol8which was then methylated with Me2SO4to afford the dibromo-anisole9in94%yield.Lithiation of7with n-BuLi in Et2O at-78°C followed by quenching with PPh2Cl produced(3-bromo-5-tert-butyl-2-anisyl)diphenylphos-phine(10)in75%yield.9was lithiated with n-BuLi/ TMEDA in Et2O at-78°C and was subsequently quenched with anhydrous DMF to afford the o-methoxy-benzaldehyde11in70%yield.Without the addition of TMEDA,no aldehyde11was formed even though the lithiation was succcessful as evidenced by the formation of deuteriated arene upon trapping reaction with D2O. Demethylation of11with BBr3yielded the salicyaldehyde 11in80%yield which was then condensed with2,3-diamino-2,3-dimethylbutane to afford ligand13in70% yield.The enantiomeric pair of chiral acyclic pyridine ligands was prepared via the condensation of5-tert-butyl-3-(2′-pyridyl)salicyaldehyde(5)with either(R,R)-and(S,S)-1,2-diaminocyclohexanes in the form of mono(+)-tar-trates19in85and86%yield,respectively(Scheme3).16 and17showed equal but opposite sign of optical rotation confirming their enantiomeric relationship.The characteristic functionalities of Schiff base ligands include the hydrogen bonded hydroxyl groups and the azomethine groups(-C d N-).These functional groups were identified in7,13,16,and17by comparing the spectroscopic data with literature values of similar systems.1a,6Broad peaks were observed in the range 3600-2400cm-1in their IR spectra which strongly suggested the presence of hydrogen bonded OH groups. Low field broad singlets appeared atδ14.76,14.37,14.14, and14.14in the1H NMR spectra of7,13,16,and17 respectively,and supported hydrogen bonded phenolic protons.In the IR spectra of these compounds,strong(12)For leading references:Larrow,J.F.;Jacobsen,E.N.J.Am. Chem.Soc.1994,116,12919.(13)Lam,F.;Chan,K.S.Tetrahedron Lett.1996,35,2439.(14)Casiraghi,G.;Casnati,G.;Puglia,G.;Sartori,G.;Terenghi,G. J.Chem.Soc.Perkin Trans.11980,1862.(15)Wriede,U.;Fernandez,M.;West,K.F.;Harcourt,D.;Moore,.Chem.1987,52,4485.(16)Stille,J.K.Angew.Chem.,Int.Ed.Engl.1986,25,508.(17)Jutzi,P.;Gilge,anomet.Chem.1983,246,163.(18)Sayre,R.J.Am.Chem.Soc.1955,77,6899.(19)Larrow,J.F.;Jacobsen,E.N.Gao,Y.;Hong,Y.;Nie,X.;Zepp,.Chem.1994,59,1939.Scheme1Scheme2Binucleating Ligands .Chem.,Vol.61,No.24,19968415signals at1620-1630cm-1were observed and likely arose from the C d N stretch of the imine linkage.The singlets atδ8.3-8.4in their NMR spectra,indicating the presence of azomethine protons,20substantiated the IR assignment.Compounds7and13also exhibited characteristic UV-vis spectra of Schiff base ligands.Schiff base ligands exhibit two absorption peaks at a lower energy region (>300nm).Bosnich21has made general assignments of the electronic transitions.The stronger,higher energy peak is attributed to theπfπ*transition of the azomethine chromophore while the weaker and less energetic peak is assigned to the n fπ*transition involving the promotion of the lone pair electron of azomethine nitrogen atom to the antibondingπorbital associated with the azomethine group.Both7and13 exhibited a pair of absorption bands in their electronic spectra.The strong peak at340-350nm and the less intense peak at430-440nm corresponded well to the expectedπfπ*and n fπ*transitions of Schiff base derivatives.Preliminary studies showed that both ligands16and 17formed mononuclear metal complexes with copper and nickel ions in high yields(Scheme4).Both homo and hetero binuclear complexes were prepared,and the full characterization by X ray crystallography as well as their catalytic activity are in progress.ConclusionIn conclusion,we have synthesized acyclic chiral and achiral dinucleating ligands of Schiff bases of pyridine and phosphine groups.Preliminary complexation studies show that these ligands formed heterobimetallic com-plexes with Ni and Cu.22Further studies of the bi-metallic chemistry of these ligands are in progress.Experimental SectionUV-vis spectra were recorded using CH2Cl2as the solvent. IR spectra were recorded on a FT-IR spectrophotometer as neat film on KBr plates.1H NMR spectra were recorded either at250MHz.Chemical shifts(δ)were reported in ppm downfield from internal standard tetramethylsilane,and coupling constants(J)were reported in hertz.13C Spectra were obtained at62.9or125MHz.Mass spectra(EI)were obtained at70eV,and FABMS was recorded using m-nitrobenzyl alcohol(NBA)as the matrix at National Tsing Hua University,Taiwan.Elemental analyses were performed by the Medac Ltd,Department of Chemistry,Brunel University, United Kingdom.Specific rotation were determined on a polarimeter.Unless otherwise noted,all materials were obtained from commercial suppliers and used without further purification. 2-(Tributylstannyl)pyridine(4),172,3-diamino-2,3-dimethyl-butane(6),182,6-dibromo-4-tert-butylphenol(8),232,6-dibromo-4-tert-butylanisole(9),24(R,R)-1,2-diammoniocyclohexane mono-(+)-tartrate,19and(S,S)-1,2-diammoniocyclohexane mono-(-)-tartrate salt(5)19were prepared according to the literature methods.Tetrahydrofuran(THF)and diethyl ether were distilled from sodium benzophenone ketyl immediately prior to use.Hexane was distilled over calcium chloride,and toluene was distilled from sodium.Silica gel(70-230mesh)was used for column chromatography.Thin layer chromatography was performed on Merck precoated silica gel60F254plates.All melting points were uncorrected.3-Bromo-5-tert-butyl-2-hydroxybenzaldehyde(3).To a solution of219(0.52g,2.93mmol)and sodium acetate(0.44 g,5.37mmol)in glacial acetic acid(13mL),was added bromine(0.47g,2.93mmol)in acetic acid(5mL)dropwise within0.5h.The mixture was heated at50°C for12h.The solvent was removed in vacuo,and the residue was poured into water and then extracted with dichloromethane.The organic layer was washed with Na2S2O5and NaHCO3solution and dried (MgSO4).The solvent was removed,and the crude product was purified by column chromatography using hexane/ethyl acetate(5:1)as the eluent(R f)0.70)to give pale yellow solids of15(0.71g,95%):mp81-83°C(CH2Cl2/hexane);1H NMR (CDCl3,250MHz)δ1.30(s,9H),7.48(d,1H,J)2.2Hz), 7.79(d,1H,J)2.2Hz),9.82(s,1H),11.39(s,1H);mass spectrum m/e(%relative intensity)258(M++2,35),256(M+, 35),243(100),241(100);IR(film)3600-3200,2958,1656, 1456,736,724cm-1.Anal.Calcd for C11H13BrO2:C,51.38; H,5.10.Found:C,51.20;H,5.08.3-(2′-Pyridyl)-5-tert-butyl-2-hydroxybenzaldehyde(5). Salicyaldehyde3(0.040g,0.156mmol),2-(tri-n-butylstannyl)-pyridine(0.17g,0.468mmol),and Pd(PPh3)4(0.018g,0.0156 mmol)were dissolved in anhydrous THF(5mL).The mixture was degassed by the freeze-thaw-pump method(three cycles)(20)Alyea,E.C.;Malek,A.Can.J.Chem.1975,53,939.(21)Bosnich,B.J.Am.Chem.Soc.1968,90,627.(22)Lam,F.;Wang,R.-J.;Mak,T.C.W.;Chan,K.S.J.Chem.Soc., mun.1994,2439.(23)Kajigaeshi,S.;Kakinimi,T.;Tokiyama,H.;Hirakawa,T.; Okamoto,T.Chem.Lett.1987,627.(24)Koike,K.;Murata,K.;Kosugi,K.Chem.Abstr.1991,114, 101336s.Scheme3Scheme4.Chem.,Vol.61,No.24,1996Lam et al.and then heated at100°C under nitrogen for72h.The solvent was removed at reduced pressure,and the residue was purified by column chromatography using hexane/ethyl acetate (10:1)as the eluent to give yellow solids(R f)0.20)as the product(0.037g,93%):mp89-91°C(CH2Cl2/hexane);1H NMR(CDCl3,250MHz)δ1.37(s,9H),7.30(m,1H),7.89 (td,1H,J)1.8,8.3Hz),7.90(d,1H,J)2.5Hz),7.99(d,1 H,J)8.3Hz),8.09(d,1H,J)2.5Hz),8.56(d,1H,J)4.3 Hz),10.60(s,1H)15.09(bs,1H);13C NMR(CDCl3,62.9MHz)δ31.30,34.24,119.57,120.10,122.01,124.25,127.13,129.75, 137.93,140.05,145.89,157.00,161.20,190.80;mass spectrum m/e(%relative intensity)255(M+,8),240(22),227(100),212 (56);IR(film)3600-2400,1680,1597,1480,1255cm-1.Anal. Calcd for C16H17NO2:C,75.27;H,6.71;N,5.49.Found:C, 74.89;H,6.60;N,5.42.Bis[5′-tert-butyl-3′-(2′′-pyridyl)salicylidene]-1,1,2,2-tet-ramethylethylenediamine(7).To a solution of5(0.042g, 0.165mmol)in refluxing absolute ethanol(5mL)was added slowly in20min2,3-diamino-2,3-dimethylbutane(6)(0.0096 g,0.083mmol)in absolute ethanol(5mL)to yield a yellow solution.The solution was refluxed for1h.After cooling, yellow crystals were collected by filtration.The rest of product was obtained by cooling the filtrate at0°C for several hours followed by filtration to afford totally0.037g of bright yellow solids as the product(76%):mp218-220°C(ethanol);1H NMR(CDCl3,250MHz)δ1.35(s,18H),1.41(s,12H),7.15-7.20(m,2H),7.33(d,2H,J)2.5Hz),7.69(td,2H,J)1.9,7.5Hz),7.96(d,2H,J)2.5Hz),8.05(d,2H,J)8.3Hz),8.44(s,2H),8.68(d,2H,J)4.3Hz),14.76(bs,2H);13C NMR(CDCl3,62.9MHz)δ23.63,32.14,34.77,65.82,119.67, 122.15,125.31,127.66,129.86,131.51,136.45,141.57,149.81, 156.65,158.57,162.48;mass spectrum m/e(%relative inten-sity)590(M+,11);IR(film)3600-2400,2962,1628,1598, 1469,1257cm-1;UV-vis[λmax,nm( ,L mol-1cm-1)]342(21.5×103),434(1.65×103).Anal.Calcd for C38H46N4O2‚1/2H2O: C,76.09;H,7.90;N,9.34.Found:C,76.13;H,7.85;N,9.35.(3-Bromo-5-tert-butyl-2-methoxyphenyl)diphenylphos-phine(10).To a solution of924(5.01g,15.6mmol)in anhydrous ether(20mL)under nitrogen-78°C was added n-BuLi(1.6M,10mL,16.0mmol)dropwise.The mixture was stirred for0.5h at-78°C to give a yellow solution to which PPh2Cl(3.44g,15.6mol)in anhydrous ether(10mL)was added dropwise at-78°C under nitrogen.The solution was allowed to warm up to room temperature and stirred for2h. Dilute HCl(10mL)was added to the solution at5°C.The mixture was extracted with ether,and the organic layer was collected and dried over MgSO4.After removal of solvent,a pale yellow oil was obtained.White crystals were collected by crystallization from ethanol,and the crude product from the mother liquor was purified by column chromatography using hexane/ethyl acetate(10:1)as the eluent(R f)0.70)to give totally5.0g of10(75%):mp101-103°C(CH2C2/hexane); 1H NMR(CDCl3,250MHz)δ1.08(s,9H),3,77(s,3H),6.64 (dd,1H,J)2.5,4.2Hz),7.20-7.36(m,10H),7.50(d,1H, J)2.5Hz);mass spectrum m/e(%relative intensity)428 (M++2,98),426(M+,100).IR(film)3069,2962,1474,1267 cm-1.Anal.Calcd for C23H24BrOP:C,64.65;H, 5.66. Found:C,64.95;H,5.69.5-tert-Butyl-3-(diphenylphosphino)-2-methoxybenzal-dehyde(11).To a well-stirred solution of10(2.94g,6.9 mmol)and TMEDA(1.04g,9.0mmol)in anhydrous ether(10 mL)at-78°C was added n-BuLi(1.6M,4.4mL,7.0mmol) dropwise under nitrogen.The orange solution was stirred at -78°C for1h.Distilled DMF(2.52g,34.5mmol)was added to the solution via a syringe at-78°C under nitrogen.The mixture was allowed to warm up to room temperature and stirred for2h.Dilute HCl was slowly added to the reaction mixture until pH)2.After stirring for1-2h,the mixture was extracted with dichloromethane,and the organic layer was dried(Na2SO4)to afford yellow oil after removal of solvent. The crude product was purified by column chromatography (hexane/ethyl acetate)10:1)to give white solids of22(1.82 g,70%):R f)0.55;mp125-126°C(CH2Cl2/hexane);1H NMR (CDCl3,250MHz)δ1.10(s,9H),3.86(s,3H),7.01(m,1H), 7.24-7.35(m,10H),7.82(d,1H,J)2.3Hz),10.35(s,1H); MS m/e(relative intensity)376(M+,88),348(97),185(14);IR(film)2963,1687,1586,1247cm-1.Anal.Calcd for C24H25O2P:C,76.58;H,6.69.Found:C,76.61;H,6.73.5-tert-Butyl-3-(diphenylphosphino)-2-hydroxybenzal-dehyde(12).To a solution of11(0.83g, 2.2mmol)in anhydrous CH2Cl2(20mL)at-78°C under nitrogen,was added slowly BBr3(2.8g,11mmol)in anhydrous CH2Cl2(20 mL)via a syringe.The yellow solution was stirred at-78°C under nitrogen for2h and allowed to stir for further10h at room temperature.The mixture was added to ice-water and extracted with CH2Cl2.The aqueous layer was neutralized by saturated NaHCO3and extracted with CH2Cl2.The dichlo-romethane extract was dried over Na2SO4to give a yellow oil which was purified by column chromatography(hexane-ethyl acetate10:1)to afford a pale yellow solid(0.63g,80%):R f) 0.60;mp110-112°C(CH2Cl2/hexane);1H NMR(CDCl3,250 MHz)δ1.28(s,9H),7.06(dd,1H,J)2.5,4.8Hz),7.37(m, 10H),7.54(d,1H,J)2.5Hz),9.90(s,1H),11.43(d,1H,J )2.8Hz);13C NMR(CDCl3,62.9MHz)δ30.87,33.99,119.34, 122.47,125.62(d,J CP)25.2Hz),127.53,128.48(d,J CP)6.9 Hz),128.72(d,J CP)22.0Hz),130.72(d,J CP)14.5Hz),133.81 (d,J CP)20.8Hz),135.79(d,J CP)11.3Hz),138.94,172.77, 161.13(d,J CP)17.0Hz),199.80;mass spectrum m/e(relative intensity)362(M+,36),334(100);calcd for C23H23O2P:362.1436; found:362.1410;IR(film)3600-3200,2963,1651cm-1.Bis[5′-tert-butyl-3′-(diphenylphosphino)salicylidene]-1,1,2,2-tetramethylethylenediamine(13).To a solution of 12(0.20g,0.55mmol)in refluxing absolute ethanol(10mL), was added slowly in20min2,3-diamino-2,3-dimethylbutane (6)(0.033g,0.28mmol)in absolute ethanol(10mL)to yield a yellow solution.The ethanolic solution was refluxed for4h. After cooling,yellow crystals were collected after filtration to afford13(0.155g,70%):mp186-189°C(ethanol);1H NMR (CDCl3,250MHz)δ1.07(s,18H),1.34(s,12H),6.76(dd,2 H,J)2.5,4.5Hz),7.19(d,2H,J)2.0Hz),7.32(m,20H), 8.31(s,2H),14.37(bs,2H);13C NMR(CDCl3,62.9MHz)δ23.19,31.17,33.98,65.21,117.16,124.20(d,J CP)13.2Hz), 128.32(m),129.25,133.83(d,J CP)19.5Hz),134.60,136.99 (d,J CP)11.3Hz),140.81,162.11(m);FABMS m/e(%relative intensity)805(M+1+,85);IR(film)3600-2400,2962,1625, 1598,1435cm-1;UV-vis[λmax,nm( ,L mol-1cm-1)]340(7.77×103),436(1.15×103).Anal.Calcd for C52H58N2O2P2.3/ 2H2O:C,75.07;H,7.39;N,3.37.Found:C,75.06;H,7.31; N,3.25.Bis[5′-tert-butyl-3′-(2′′-pyridyl)salicylidene]-(R,R)-1,2-cyclohexanediamine(16).(R,R)-1,2-Diammoniocyclohexane mono-(+)-tartrate19(0.132g,0.5mmol),K2CO3(0.138g,1.0 mmol),and distilled water(0.7mL)were stirred together to form a solution,and then ethanol(2.7mL)was added.The resulting mixture was heated to reflux,and a solution of aldehyde5(0.132g,0.5mmol)in ethanol(1.3mL)was added dropwise.The funnel was rinsed with ethanol(0.2mL),and the yellow solution was stirred at refluxed for4h.Water(0.7 mL)was added,and the stirred mixture was cooled to rt.The mixture was extracted with CH2Cl2.The extracted mixture was washed with water(2×1.5mL)and brine(1.5mL).After drying over Na2SO4,the solvent was removed under vaccum, and the residue was recrystallized from hexane to give yellow solids(0.25g,85%yield):mp170-1°C,(phase change118-119°C).R f)0.32(hexane/ethyl acetate)5:1);1H NMR (CDCl3,250MHz)δ1.29(s,18H),1.40-2.10(m,8H),3.33 (m,2H),7.22(dd,2H,J)5.2,7.0Hz),7.26(d,2H,J)2.5 Hz),7.73(ddd,2H,J)1.8,7.0,and7.8Hz),7.85(d,2H,J )2.5Hz),7.96(d,2H,J)7.8Hz),8.38(s,2H),8.71(d,2H, J)5.2Hz),14.14(bs,2H);13C NMR(125MHz,CDCl3),24.19, 31.38,33.13,34.05,72.59,118.91,121.56,124.44,126.60, 128.97,130.59,135.82,141.13,149.21,156.10,156.93,164.87; mass spectrum,m/e(%relative intensity),588(M+,32),334 (70),294(5),254(100),212(9),IR(neat film),733,796,1256, 1451,1565,1596,1629,1633,2862,2936,2960,2400-3600 cm-1.[R]20D)-518°(c)1.0,CH2Cl2);UV-vis[λmax,nm(L mol-1cm-1)]342(16.6×103).Anal.Calcd for C38H44N4O2: C,77.52;H,7.53;N,9.52.Found:C,77.38,H,7.56;N,9.46.Bis[5′-tert-butyl-3′-(2′′-pyridyl)salicylidene]-(S,S)-1,2-cyclohexanediamine(17).(S,S)-1,2-Diammoniocyclohexane mono-(-)-tartrate19(0.132g,0.5mmol),K2CO3(0.138g,1.0 mmol),and distilled water(0.7mL)were stirred until dissolu-Binucleating Ligands .Chem.,Vol.61,No.24,19968417tion was achieved,and then ethanol(2.7mL)was added.The resulting mixture was heated to reflux,and a solution of aldehyde5(0.132g,0.5mmol)in ethanol(1.1mL)was added dropwise.The funnel was rinsed with ethanol(0.2mL),and the yellow solution was stirred at reflux for4h before heating was discontinued.Water(0.7mL)was added,and the stirred mixture was cooled to rt.The mixture was extracted with CH2Cl2.Then the extracted mixture was washed with water (2×1.5mL)and brine(1.5mL)and dried over Na2SO4.The solvent was removed under vaccum,and the residue was recrystallized in hexane to give the yellow solid(0.253g,86% yield):mp169-70°C(phase change118-119°C);R f)0.32 (hexane/ethyl acetate:5:1);1H NMR(CDCl3,250MHz): 1.29 (s,18H),1.40-2.10(m,8H),3.33(m,2H),7.22(d,2H,J) 5.2,7.0Hz),7.26(d,2H,J)2.5Hz),7.73(ddd,2H,J)1.8, 7.0,and7.8Hz),7.85(d,2H,J)2.5Hz),7.96(d,2H,J) 7.8Hz),8.38(s,2H),8.71(d,2H,J)5.2Hz),14.14(bs,2H); 13C NMR(125MHz,CDCl3),24.20,31.38,33.14,34.05,72.59, 118.91,121.57,124.44,126.60,128.97,130.60,135.83,141.14, 149.21,156.10,156.93,164.88;mass spectrum,m/e(%relative intensity),588(M+,22),334(73),294(9),254(100),212(11); IR(neat film)734,796,1256,1451,1565,1596,1630,1634, 2935,2960,2400-3600cm-1;[R]20D)+517°(c)1.0,CH2Cl2); UV-vis[λmax,nm(L mol-1cm-1)]342(16.1×103).Anal.Calcd for C38H44N4O2:C,77.52;H,7.53;N,9.52.Found:C,76.04, H,7.64;N,9.22.Copper(II)Bis[5′-tert-butyl-3′-(2′′-pyridyl)salicylidene]-(R,R)-1,2-cyclohexanediamine(18).To a refluxing solution of(R,R)Schiff base16(50mg,0.085mmol)in ethanol(10mL) was added dropwise Cu(OAc)2‚H2O(17mg,0.085mmol), dissolved in hot ethanol(10mL),within20min.The resulting brown suspension was refluxed for1h.After the mixture was cooled,the precipitate was filtered and recrystallized in CHCl3/ EtOH to give90mg of brown solid(yield81%):mp352-353°C;IR(neat film)2949,2927,1622,1536,1444,1429,1249, 1226,797cm-1;[R]20D)-1.1×103°(c)0.02,CH2Cl2),UV-vis[λmax,nm( ,L mol-1cm-1)]387(21.0×103).Anal.Calcd for C38H42N4O2Cu:C,70.23;H,6.52;N,8.63.Found:C,69.96, H,6.66;N,8.50.HRMS calcd650.2682,found650.2745.Copper(II)Bis[5′-tert-butyl-3′-(2′′-pyridyl)salicylidene]-(S,S)-1,2-cyclohexanediamine(19).To a refluxing solution of(S,S)Schiff base17(50mg,0.085mmol)in ethanol(10mL) was added dropwise Cu(OAc)2‚H2O(17mg,0.085mmol), dissolved in hot ethanol(10mL),within20min.The resulting brown suspension was refluxed for1h.After the mixture was cooled,the precipitate was filtered and recrystallized in CHCl3/ EtOH to give90mg of brown solid(yield81%):mp352-353°C;IR(neat film)2949,2927,1622,1536,1444,1429,1249, 1226,798cm-1.[R]20D)1.1×103o(c)0.02,CH2Cl2),UV-vis [λmax,nm( ,L mol-1cm-1)]388(20.4×103).Anal.Calcd for C38H42N4O2Cu:C,70.23;H,6.52;N,8.63.Found:C,70.29, H,6.52;N,8.63.HRMS calcd650.2682,found650.2705.Nickel(II)Bis[5′-tert-butyl-3′-(2′′-pyridyl)salicylidene]-(S,S)-1,2-cyclohexanediamine(20).To a refluxing solution of(S,S)Schiff base17(50mg,0.085mmol)in ethanol(10mL) was added dropwise Ni(OAc)2‚4H2O(21mg,0.085mmol), dissolved in hot ethanol(10mL),within20min.The yellow precipitate appeared,and the mixture was refluxed for1h. After cooling,the precipitate was filtered and recrystallized in CHCl3/EtOH to give53.4mg of a muddy yellow solid(yield 97%):mp380-381°C;1H NMR(CDCl3,250MHz)1.32(s,18H),1.62(br,2H),1.76(br,4H),2.36(br,2H),3.24(br,2H),6.94(dd,2H,J)7.6,7.8Hz),7.01(dd,2H,J)6.9,7.6Hz),7.09(s,2H),7.46(s,2H),8.17(d,2H,J)2.2Hz),8.21(d, 2H,J)7.8Hz),8.57(d,2H,J)2.2Hz);IR(neat film)2949, 2927,1622,1537,1445,1251,797cm-1;[R]20D)1.1×103o(c )0.05,CH2Cl2);UV-vis[λmax,nm( ,L mol-1cm-1)]425(13.6×103),329(16.3×103).Calcd for C38H42N4O2Ni:HRMS calcd 645.2739,found645.2743.Acknowledgment.We thank the Croucher Foun-dation for the award of a studentship to F.L.and the Dow Chemical Co.for financial support.JO961020F.Chem.,Vol.61,No.24,1996Lam et al.。
《2024年席夫碱构筑的金属—有机配位化合物的合成、结构及性质》范文

《席夫碱构筑的金属—有机配位化合物的合成、结构及性质》篇一席夫碱构筑的金属-有机配位化合物的合成、结构及性质一、引言近年来,金属-有机配位化合物因其独特的结构特性和潜在的应用价值,已成为化学领域的研究热点。
其中,席夫碱构筑的金属-有机配位化合物因其结构多样性和良好的配位能力,在材料科学、生物医学和催化等领域具有广泛的应用前景。
本文旨在探讨席夫碱构筑的金属-有机配位化合物的合成方法、结构特征及性质研究。
二、席夫碱构筑的金属-有机配位化合物的合成1. 合成原料与试剂本实验所使用的原料主要包括席夫碱类化合物、金属盐以及溶剂等。
其中,席夫碱类化合物通过醛类与胺类化合物缩合反应制备;金属盐如铜盐、锌盐等为常见的配位金属源。
2. 合成方法本实验采用溶液法进行席夫碱构筑的金属-有机配位化合物的合成。
首先,将席夫碱类化合物与金属盐分别溶解在适当的溶剂中,然后混合并搅拌一定时间,使金属离子与席夫碱配位形成配合物。
最后,通过离心、洗涤、干燥等步骤得到目标产物。
三、席夫碱构筑的金属-有机配位化合物的结构特征1. 结构类型席夫碱构筑的金属-有机配位化合物具有多种结构类型,如一维链状、二维网状和三维框架结构等。
这些结构类型与金属离子、席夫碱配体的种类及配位方式密切相关。
2. 晶体结构分析通过X射线单晶衍射技术,可以详细分析席夫碱构筑的金属-有机配位化合物的晶体结构。
从晶体结构中可以观察到金属离子与席夫碱配体之间的配位键、氢键等相互作用,以及化合物的空间排列方式。
四、席夫碱构筑的金属-有机配位化合物的性质研究1. 光学性质席夫碱构筑的金属-有机配位化合物往往具有优异的光学性质,如发光、荧光等。
这些光学性质与化合物的晶体结构、能级分布等密切相关,可以应用于光电材料、荧光探针等领域。
2. 磁学性质某些席夫碱构筑的金属-有机配位化合物具有磁学性质,如铁磁性、反铁磁性等。
这些磁学性质与金属离子的电子排布、配体的电子云密度等有关,可以应用于磁性材料、催化剂等领域。
《2024年基于多齿席夫碱构筑的3d、3d-4f金属配合物的合成、结构及性质》范文
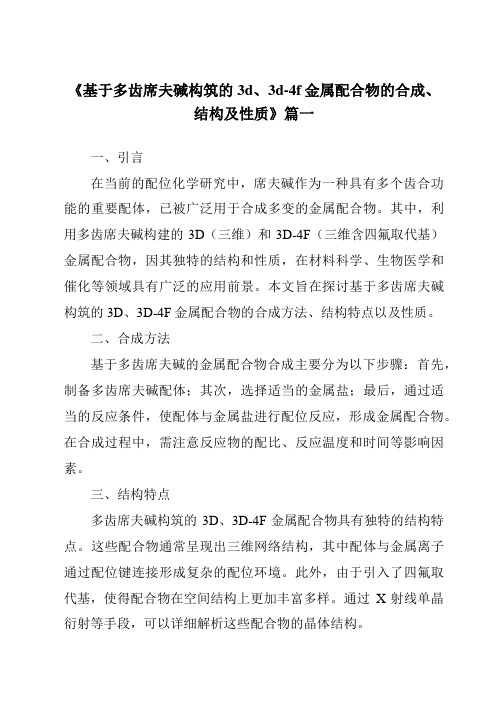
《基于多齿席夫碱构筑的3d、3d-4f金属配合物的合成、结构及性质》篇一一、引言在当前的配位化学研究中,席夫碱作为一种具有多个齿合功能的重要配体,已被广泛用于合成多变的金属配合物。
其中,利用多齿席夫碱构建的3D(三维)和3D-4F(三维含四氟取代基)金属配合物,因其独特的结构和性质,在材料科学、生物医学和催化等领域具有广泛的应用前景。
本文旨在探讨基于多齿席夫碱构筑的3D、3D-4F金属配合物的合成方法、结构特点以及性质。
二、合成方法基于多齿席夫碱的金属配合物合成主要分为以下步骤:首先,制备多齿席夫碱配体;其次,选择适当的金属盐;最后,通过适当的反应条件,使配体与金属盐进行配位反应,形成金属配合物。
在合成过程中,需注意反应物的配比、反应温度和时间等影响因素。
三、结构特点多齿席夫碱构筑的3D、3D-4F金属配合物具有独特的结构特点。
这些配合物通常呈现出三维网络结构,其中配体与金属离子通过配位键连接形成复杂的配位环境。
此外,由于引入了四氟取代基,使得配合物在空间结构上更加丰富多样。
通过X射线单晶衍射等手段,可以详细解析这些配合物的晶体结构。
四、性质研究1. 光学性质:多齿席夫碱构筑的金属配合物往往具有优异的光学性质,如较强的荧光性能。
这些性质使得它们在荧光探针、生物成像等领域具有潜在应用价值。
2. 磁学性质:部分金属配合物具有明显的磁学性质,如顺磁性或抗磁性。
这些性质使得它们在磁性材料和磁学研究中具有应用价值。
3. 催化性质:某些金属配合物具有优异的催化性能,可用于催化有机反应、光催化反应等。
此外,由于引入了四氟取代基,使得这些配合物在含氟有机化合物的合成中具有特殊的应用价值。
4. 热稳定性:通过热重分析等手段,可以研究金属配合物的热稳定性。
这些数据对于评估材料的实际应用性能具有重要意义。
五、结论基于多齿席夫碱构筑的3D、3D-4F金属配合物具有独特的结构和性质,为材料科学、生物医学和催化等领域提供了新的研究方向。
《2024年席夫碱构筑的金属—有机配位化合物的合成、结构及性质》范文
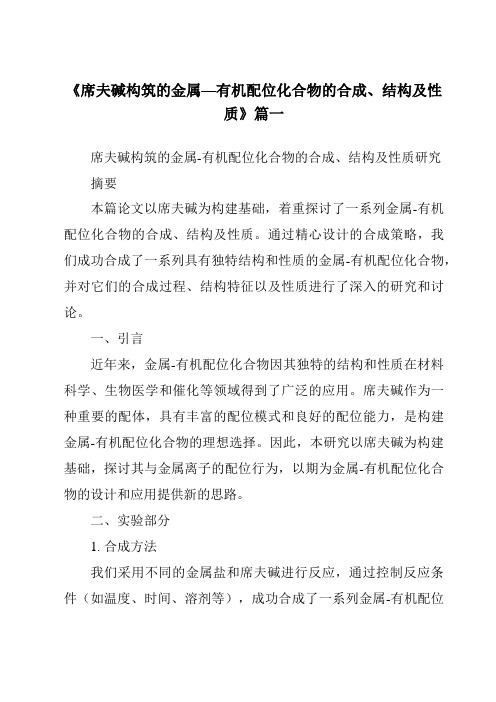
《席夫碱构筑的金属—有机配位化合物的合成、结构及性质》篇一席夫碱构筑的金属-有机配位化合物的合成、结构及性质研究摘要本篇论文以席夫碱为构建基础,着重探讨了一系列金属-有机配位化合物的合成、结构及性质。
通过精心设计的合成策略,我们成功合成了一系列具有独特结构和性质的金属-有机配位化合物,并对它们的合成过程、结构特征以及性质进行了深入的研究和讨论。
一、引言近年来,金属-有机配位化合物因其独特的结构和性质在材料科学、生物医学和催化等领域得到了广泛的应用。
席夫碱作为一种重要的配体,具有丰富的配位模式和良好的配位能力,是构建金属-有机配位化合物的理想选择。
因此,本研究以席夫碱为构建基础,探讨其与金属离子的配位行为,以期为金属-有机配位化合物的设计和应用提供新的思路。
二、实验部分1. 合成方法我们采用不同的金属盐和席夫碱进行反应,通过控制反应条件(如温度、时间、溶剂等),成功合成了一系列金属-有机配位化合物。
具体合成步骤如下:首先,制备席夫碱配体;然后,将金属盐与席夫碱在适当的溶剂中进行反应,得到目标产物。
2. 结构表征利用X射线单晶衍射、X射线粉末衍射、红外光谱等手段对合成的金属-有机配位化合物进行结构表征。
结果表明,这些化合物具有独特的结构和良好的结晶度。
三、结果与讨论1. 结构分析通过X射线单晶衍射分析,我们得到了金属-有机配位化合物的详细结构信息。
结果表明,这些化合物具有多样的配位模式和空间构型,其中金属离子与席夫碱配体之间形成了稳定的配位键。
此外,化合物中还存在氢键、π-π相互作用等非共价相互作用,进一步稳定了化合物的结构。
2. 性质研究我们对合成的金属-有机配位化合物进行了性质研究。
首先,通过热重分析研究了化合物的热稳定性。
结果表明,这些化合物具有较高的热稳定性,能够在较高的温度下保持结构稳定。
其次,我们研究了化合物在催化、荧光、磁性等方面的性质。
结果表明,这些化合物在催化领域具有潜在的应用价值,同时部分化合物还具有荧光和磁性等特殊性质。
席夫碱金属配合物的合成及其在催化领域的应用

席夫碱金属配合物的合成及其在催化领域的应用《席夫碱金属配合物的合成及其在催化领域的应用》摘要:席夫碱金属配合物是一类重要的配体,它们的合成及其在催化领域的应用受到越来越多的关注。
本文介绍了席夫碱金属配合物的结构和合成方法,以及它们在催化领域的应用,包括呋喃类化合物的氧化、氮化合成、碱金属催化剂的构筑等。
关键词:席夫碱金属配合物;合成;催化1 引言席夫碱金属配合物是一类重要的过渡金属配合物,它们包括一个或多个具有同极性的碱性部分,如硝酸根、氯酸根、磷酸根和硫酸根,以及一个或多个具有异极性的亲电态部分,如氮、硫、氟等。
近年来,由于它们的强催化性能、宽范围的反应活性和安全、稳定的构型特性,席夫碱金属配合物的合成及其在催化领域的应用受到了越来越多的关注。
2 结构及合成席夫碱金属配合物一般由金属核心、配体部分和叉形部分组成。
其中,金属核心可以是稳定的金属原子或金属离子,配体部分是由一个或多个同极性的碱性配体组成,叉形部分是由一个或多个异极性的亲电态分子组成,这些分子来自于常用的氮、硫、氟等元素。
席夫碱金属配合物的合成方法也多种多样,一般可分为以下几类: (1) 直接合成法:在固定的条件下,将金属原子、配体及叉形部分加在一起,通常产物的结构稳定,也可能由多种结构组成的混合物。
(2) 结构优化法:在固定的条件下,将金属原子、配体及叉形部分加在一起,然后通过结构优化得到最稳定的结构形态,如碱基配合物的侧面氨基化反应。
(3) 离子交换法:将金属原子及配体部分添加到某一离子交换层上,然后将叉形部分结合到金属原子上,形成席夫碱金属配合物。
3 在催化领域的应用席夫碱金属配合物在催化领域有着广泛的应用,其中包括呋喃类化合物的氧化、氮化合成、碱金属催化剂的构筑等。
(1) 呋喃类化合物的氧化席夫碱金属配合物可以用于活性碳氧化及其它重要的氧化反应,如呋喃类化合物的氧化催化剂的合成和应用。
最近有一类新型的席夫碱金属配合物已被用于呋喃的氧化反应,结果表明,这类新型的催化剂具有广泛的反应活性,能够有效地解决一些有机合成中的困难反应。
《2024年基于多齿席夫碱构筑的3d、3d-4f金属配合物的合成、结构及性质》范文

《基于多齿席夫碱构筑的3d、3d-4f金属配合物的合成、结构及性质》篇一一、引言多齿席夫碱在配合物合成中因其结构灵活和易于构建三维结构等优点被广泛应用。
本研究通过席夫碱合成一系列3D和3D-4F金属配合物,探究其结构及性质。
这一类金属配合物具有广泛的化学应用前景,可广泛应用于光催化、电化学和生物医药等各个领域。
本文首先阐述金属配合物的重要性以及其可能的应用场景,随后简要介绍基于多齿席夫碱的配合物研究现状和本次研究的意义。
二、合成方法1. 实验材料与试剂本实验主要采用多齿席夫碱作为主要原料,配合适当的金属盐和其他化学试剂进行合成。
所有试剂均为分析纯,且在实验前都经过适当处理。
2. 合成步骤在适当的条件下,将多齿席夫碱与金属盐溶液混合,调节pH 值,加入辅助配体(若需要),加热反应,冷却结晶,最终得到目标金属配合物。
三、结构分析通过X射线单晶衍射、元素分析等手段对合成的金属配合物进行结构分析。
X射线单晶衍射结果表明,这些金属配合物具有三维或三维四氟化物(3D-4F)结构。
元素分析则进一步验证了金属配合物的组成。
四、性质研究1. 光学性质通过紫外-可见光谱和荧光光谱等手段研究金属配合物的光学性质。
结果表明,这些金属配合物具有良好的光吸收性能和荧光性能,可应用于光催化、光电材料等领域。
2. 电化学性质采用循环伏安法等电化学方法研究金属配合物的电化学性质。
结果表明,这些金属配合物具有良好的电化学性能,可应用于电化学传感器、电池材料等领域。
3. 生物活性通过生物活性测试,发现这些金属配合物具有良好的抗菌、抗肿瘤等生物活性,为生物医药领域的应用提供了可能性。
五、结论本文通过多齿席夫碱合成了一系列3D和3D-4F金属配合物,并通过X射线单晶衍射、元素分析等方法对其结构进行了详细研究。
同时,对其光学性质、电化学性质和生物活性进行了探究。
这些研究结果表明,基于多齿席夫碱的金属配合物具有广泛的应用前景,特别是在光催化、电化学和生物医药等领域。
《2024年席夫碱构筑的金属—有机配位化合物的合成、结构及性质》范文
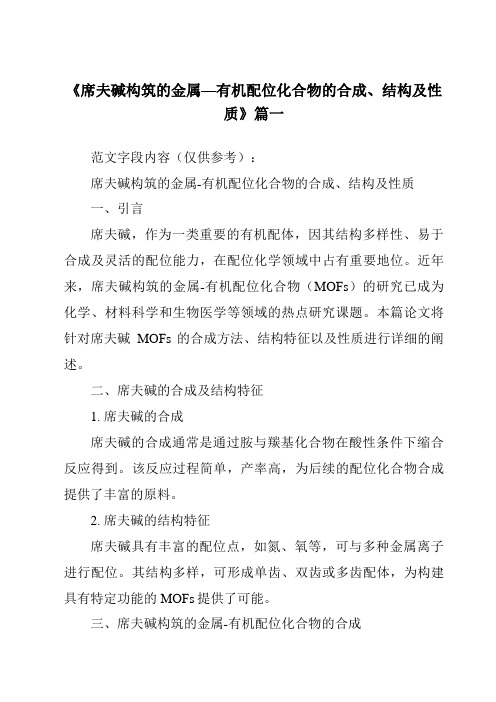
《席夫碱构筑的金属—有机配位化合物的合成、结构及性质》篇一范文字段内容(仅供参考):席夫碱构筑的金属-有机配位化合物的合成、结构及性质一、引言席夫碱,作为一类重要的有机配体,因其结构多样性、易于合成及灵活的配位能力,在配位化学领域中占有重要地位。
近年来,席夫碱构筑的金属-有机配位化合物(MOFs)的研究已成为化学、材料科学和生物医学等领域的热点研究课题。
本篇论文将针对席夫碱MOFs的合成方法、结构特征以及性质进行详细的阐述。
二、席夫碱的合成及结构特征1. 席夫碱的合成席夫碱的合成通常是通过胺与羰基化合物在酸性条件下缩合反应得到。
该反应过程简单,产率高,为后续的配位化合物合成提供了丰富的原料。
2. 席夫碱的结构特征席夫碱具有丰富的配位点,如氮、氧等,可与多种金属离子进行配位。
其结构多样,可形成单齿、双齿或多齿配体,为构建具有特定功能的MOFs提供了可能。
三、席夫碱构筑的金属-有机配位化合物的合成1. 合成方法席夫碱MOFs的合成方法主要包括溶液法、溶剂热法、微波辅助法等。
其中,溶液法是最常用的方法,通过将金属盐和席夫碱在适当溶剂中混合,控制反应条件,得到目标MOFs。
2. 合成实例以某一种席夫碱和铜离子为例,详细介绍MOFs的合成过程。
首先,将铜盐和席夫碱溶解在适当的溶剂中,加热搅拌,待溶液变为澄清后,继续反应一段时间,得到晶体。
通过X射线单晶衍射等技术对晶体进行表征,确认其结构和纯度。
四、席夫碱构筑的金属-有机配位化合物的结构特征1. 拓扑结构席夫碱MOFs具有丰富的拓扑结构,包括一维链状、二维网状、三维框架等。
这些结构使得MOFs具有独特的物理化学性质。
2. 空间构型通过X射线单晶衍射等技术,可以确定MOFs的空间构型。
这些构型对于理解MOFs的性质及其应用具有重要意义。
五、席夫碱构筑的金属-有机配位化合物的性质1. 光学性质席夫碱MOFs具有优异的光学性质,如荧光、磷光等。
这些性质使得MOFs在光电器件、生物探针等领域具有潜在的应用价值。
《2024年席夫碱构筑的金属—有机配位化合物的合成、结构及性质》范文
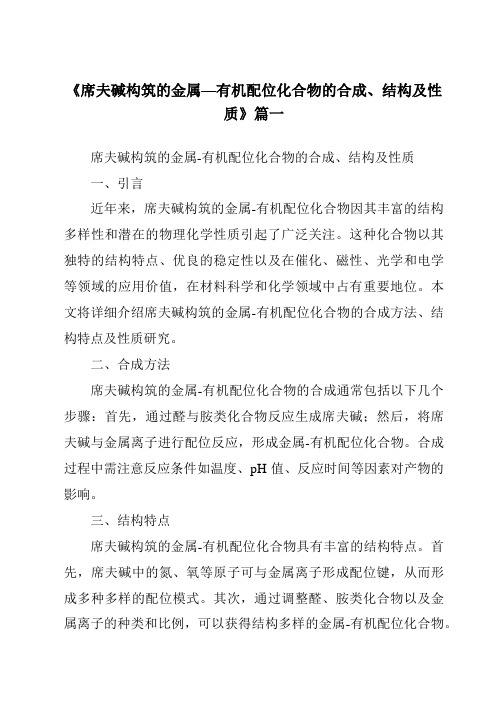
《席夫碱构筑的金属—有机配位化合物的合成、结构及性质》篇一席夫碱构筑的金属-有机配位化合物的合成、结构及性质一、引言近年来,席夫碱构筑的金属-有机配位化合物因其丰富的结构多样性和潜在的物理化学性质引起了广泛关注。
这种化合物以其独特的结构特点、优良的稳定性以及在催化、磁性、光学和电学等领域的应用价值,在材料科学和化学领域中占有重要地位。
本文将详细介绍席夫碱构筑的金属-有机配位化合物的合成方法、结构特点及性质研究。
二、合成方法席夫碱构筑的金属-有机配位化合物的合成通常包括以下几个步骤:首先,通过醛与胺类化合物反应生成席夫碱;然后,将席夫碱与金属离子进行配位反应,形成金属-有机配位化合物。
合成过程中需注意反应条件如温度、pH值、反应时间等因素对产物的影响。
三、结构特点席夫碱构筑的金属-有机配位化合物具有丰富的结构特点。
首先,席夫碱中的氮、氧等原子可与金属离子形成配位键,从而形成多种多样的配位模式。
其次,通过调整醛、胺类化合物以及金属离子的种类和比例,可以获得结构多样的金属-有机配位化合物。
此外,这些化合物通常具有较高的稳定性,可在不同环境下保持其结构不变。
四、性质研究1. 催化性质:席夫碱构筑的金属-有机配位化合物在催化领域具有广泛应用。
例如,某些化合物可催化有机反应,如烷基化、酰基化等。
此外,它们还可作为光催化剂或电催化剂,用于光催化反应或电化学反应。
2. 磁学性质:部分金属-有机配位化合物具有磁学性质,可用于制备磁性材料。
通过调整金属离子和配体的种类及比例,可以调控化合物的磁学性能。
3. 光学性质:某些席夫碱构筑的金属-有机配位化合物具有优异的光学性质,如荧光性质。
这些化合物在生物成像、光电器件等领域具有潜在应用价值。
4. 电学性质:这些化合物在电学领域也有广泛应用,如制备导电材料、超级电容器等。
五、结论席夫碱构筑的金属-有机配位化合物因其丰富的结构多样性和潜在的物理化学性质在材料科学和化学领域中占有重要地位。
- 1、下载文档前请自行甄别文档内容的完整性,平台不提供额外的编辑、内容补充、找答案等附加服务。
- 2、"仅部分预览"的文档,不可在线预览部分如存在完整性等问题,可反馈申请退款(可完整预览的文档不适用该条件!)。
- 3、如文档侵犯您的权益,请联系客服反馈,我们会尽快为您处理(人工客服工作时间:9:00-18:30)。
席夫碱金属SOD模型配合物李金芳屠淑洁周荫庄*(首都师范大学化学系北京 100037)O的清除表现出一定的活性且明摘要席夫碱为配体的Cu、Zn、Mn等单核、双核配合物对•2显高于非席夫碱单核及双核配合物。
近年来该领域新化合物的合成、结构表征及构效关系的研究甚为活跃,对CuZn-SOD、Mn-SOD、Fe-SOD的席夫碱SOD模型配合物的研究已取得进展。
本文介绍了席夫碱金属SOD模型配合物的合成、表征及相关活性的研究进展。
关键词超氧化物歧化酶席夫碱模型配合物生物活性The Superoxide Dismutase Mimics of Metal Complexes of Schiff-basesLi Jinfang, Tu Shujie, Zhou Yinzhuang*(Department of Chemistry, Capital Normal University, Beijing 100037)Abstract The design and application of the synthesis of low molecular weight metal complexes containing Cu, Zn, Mn etc. as SOD mimics have received considerable attention during the last decades. Large number of the complexes of Cu, Zn, Mn etc with various donor ligands have been characterized as •O promising models of the enzyme, including Schiff-base ligands.The results showed that the 2Schiff-base complexes exhibited that the abilities of eliminating •O are much higher than that of other2kinds of complexes. The research progress in the studies of the SOD model complexes with Schiff-base ligands and its biological activities have been introduced in this paper.Key words Superoxide dismutase, Schiff-bases, Mimic complexes, Biological activity 超氧化物歧化酶(SOD)广泛存在于生物体内,是机体清除超氧阴离子自由基的一种重要酶,在维持机体的氧化——抗氧化平衡中起着重要作用。
研究表明SOD活力的高低与机体衰老、肿瘤、炎症等疾病有关。
由于天然SOD在稳定性、膜穿透性、生物利用度和免疫原性等方面存在一些限制。
因此,对具有SOD活性且能在生理条件下保持稳定的小分子模型物的研究成为热点。
当前报道的SOD模型物多为苯并咪唑类、氨羧类、氨基酸及席夫碱等金属配合物。
相对于天然SOD,这些小分子模型物具有分子量小、脂溶性好、膜渗透性强、性质稳定等优点,最有望用于药物筛选。
SOD模型化合物的活性优劣,取决于中心金属原子的配位结构与天然SOD活性部位微环境的相似程度,配位结构越相似,活性越强,而与核的多寡无关。
含有N、O、S给体的席夫碱配体被广泛应用于生物模型配合物的研究中[1,2]。
比较以席夫碱为配体和以非席夫碱为配体的单、双核模型配合物对•O清除表现出的活性,则前者明显高于后者[3]。
为此,对以席夫碱为2李金芳女,24岁,硕士生,现从事功能配合物的研究。
*联系人,E-mail: zhouyz7813@2004-03-18收稿,2005-01-27接受配体的金属SOD 模型配合物的合成、表征及构效关系的研究等工作倍受关注并已取得显著成果。
本文对天然SOD 的席夫碱金属模型物的研究及相关工作加以介绍。
1酶促机制氧自由基或称活性氧(Reactive Oxygen Species ,ROS),主要包括超氧阴离子自由基(•2O )、羟自由基以及有机过氧化物自由基等。
生命体在正常代谢中产生ROS ,一些外界环境因素能增加细胞中的ROS 浓度,若不能及时清除以恢复平衡,会引起生物膜的过氧化损伤,甚至造成细胞器的损害和DNA 与蛋白质的降解与失活。
生命体在进化过程中形成并发展了清除活性氧的系统,例如抗氧化酶系统和非酶性小分子抗氧化系统。
超氧化物歧化酶(SOD)于1968年被发现,它广泛存在于生物体内,在生命体的自我保护系统中起着极为重要的作用,在免疫系统中也有重要的功能。
目前,在人体中仅发现3种SOD ,即CuZn-SOD 、Mn-SOD 和Ni-SOD 。
植物中还有一种 Fe-SOD 。
Mn-SOD 主要分布在线粒体中而CuZn-SOD 主要分布在细胞浆中。
SOD 是催化•2O 歧化反应、专一清除生物体内的•2O ,而平衡机体氧自由基的金属酶,其催化作用是通过所含金属的氧化和还原过程实现的。
在生理条件下,SOD 可使2O 歧化产生O 2的反应速度提高104倍[3]。
n n M O MO 22+⎯→⎯++• 1222M O H M OH +•+⎯→⎯+n n 在CuZn-SOD 的活性中心部位,Cu 与四个His 残基的咪唑基N 原子配位形成畸变的平面四方形,Zn 与三个His 残基N 原子和一个门冬氨酸残基的O 原子配位形成四方锥构型。
Ni-SOD 的活性中心结构尚未知,基于SOD 进化保守性,推测Ni 仍与3个组氨酸残基、1个Asp 残基及1个H 2O 配位[4]。
Mn-SOD 活性部位有一个是底物或其它内源性配体接近Mn II 离子的通道,该通道由疏水残基构成的疏水穴和2个亚基链共同形成,终止于金属离子附近的Tyr36和His32残基。
2席夫碱金属SOD 模型配合物2.1席夫碱CuZn-SOD 与Ni-SOD 模型配合物席夫碱SOD 模型配合物的合成、表征与生物活性研究已成为SOD 模型物研究的一个重要领域。
最近研究表明[5],超氧化物歧化酶Cu 伴护蛋白质(CCS )对SOD1的功能完善至关重要。
SOD1的结构表明,除金属中心的特性外,Cys55和Cys144间的二硫键对于其发挥功能亦很重要。
SOD1的活化极可能由二分子的杂二聚体引起。
Ni-SOD 具有与CuZn-SOD 相类似的抑制类型和与其相当的生物活性。
呈四方锥构型且轴向配位被水或碱(羧酸根)的氧占据的铜配合物和天然SOD 结构非常接近因而具有极其相似的活性。
但报道铜配合物多近似平面结构。
席夫碱铜配合物{[Cu(bppn)](ClO 4)2}2·H 2O 的EPR 谱测定g ||/A ||值恰在135cm -1,具有可逆的构象转变,配位方式从Cu(Ⅱ)平面四方构型转化为Cu(Ⅰ)四面体构型,满足了还原-氧化循环过程,具有较高SOD 活性[6]。
而类似四方锥构型如[Cu(apy)L](ClO 4)2、CuPuPhePy(ClO 4)2和{[Cu(bppn)](ClO 4)2}2由于轴向配位原子非氢氧化物分子而阻碍了构象转变,导致活性较低[7]。
为进一步获得Cu 2Zn 2SOD 的Cu(Ⅱ)和Cu(Ⅰ)结构的相关信息,研究了6-甲基吡啶-2-甲醛双缩1,4-丁二胺配合物[Cu 2Ⅰ-(Pu-6-MePy)2](ClO 4)2·MeOH 及[Cu Ⅱ-(Pu-6-MePy)(H 2O)](ClO 4)2晶体结构和SOD 活性[8](见表1)。
Cu()Ⅱ为平面四方构型而二聚的Cu()Ⅰ为三角双锥构型。
热力学稳定的二聚Cu(Ⅰ)SOD 模型物可应用于类胞液需氧环境,为获得更加稳定、有效的Cu-di-席夫Cu()Ⅰ模型物提供了可能[8]。
近年来相继发现大量以席夫碱为配体的单核Cu Ⅱ、Zn Ⅱ、Co Ⅱ、Ni Ⅱ的新型结构配合物具有一定的SOD 样活性[10~21]。
表1 黄嘌呤-黄嘌呤氧化酶介质NBT 还原测定的铜配合物的抑制率[8]Tab.1 Inhibitory effect of copper complexes on the xanthine-XOD mediated reduction of NBT [8]目前,SOD 活性测试主要采取黄嘌呤-黄嘌呤氧化酶-Luminol 化学发光体系法、邻苯三酚自氧化法、核黄素-蛋氨酸光照法、荧光和吸收光谱法等。
半数抑制率IC 50可进行定性比较,其值越小活性越强,但因一些配合物未达到IC 50或溶解性方面的原因,这一指标的使用受到限制。
乔艳红等[9]测得一系列邻香兰素氨基酸席夫碱及其配合物IC 50,邻香兰素-半胱氨酸席夫碱IC 50=29.04µg/mL ,其锌配合物IC 50=6.28µg/mL ,钴配合物IC 50=53.3µg/mL ,镍配合物IC 50=18.41µg/mL ;铜配合物及其它邻香兰素氨基酸席夫碱金属配合物的最大抑制率未达到50%而未获其IC 50值。
席夫碱及其金属配合物的SOD 活性测试为进一步研究提供了重要信息。
在天然酶中,Cu(Ⅱ)和Zn(Ⅱ)配位环境不同、空间结构各异,而模型配合物中Cu(Ⅱ)和Zn(Ⅱ)分别与相同的配体键合,其结构等同,但由于Zn(Ⅱ)的配位环境和构型与天然酶中的Zn(Ⅱ)不尽相似,Cu(Ⅱ)配合物通常表现出更强的SOD 活性。
综合目前已见诸报道的结果,在一定程度上反映出席夫碱配体和相关配合物的浓度,取代基的种类、大小和位置,及中心金属原子的电子组态等对模型配合物SOD 活性的影响关系,并表现出一定的相关性。
苯甲醛类氨基酸席夫碱金属配合物不仅具有与SOD 相似的清除•2O 作用,而且可与DNA 作用为进一步研究其它相关生物活性提供了依据。
2-氯代苯甲醛丙氨酸锌配合物与DNA 侧链碱基发生作用、钴配合物可发生对DNA 的插入作用,但对•2O 抑制能力较弱;Cu()Ⅱ、Ni()Ⅱ配合物有较强的清除作用,但与SOD 相比相差较远,其余化合物几乎没有作用[20,21]。
新型席夫酰腙邻羟基苯乙酮缩2-氨-4-肼-6-甲基嘧啶[25]和对称的双-(7-甲醛基-8-羟基喹啉-5-磺酸)缩二胺类席夫碱[26]金属配合物的研究表明,配体中极性基团磺酸基的引入利于配合物在靶部位的分布,增大了其与微生物的作用机会。
吸电子基取代的席夫碱也会对配体的生物活性产生一定的影响。