各种尺寸纳米金的合成
纳米金的制备方法
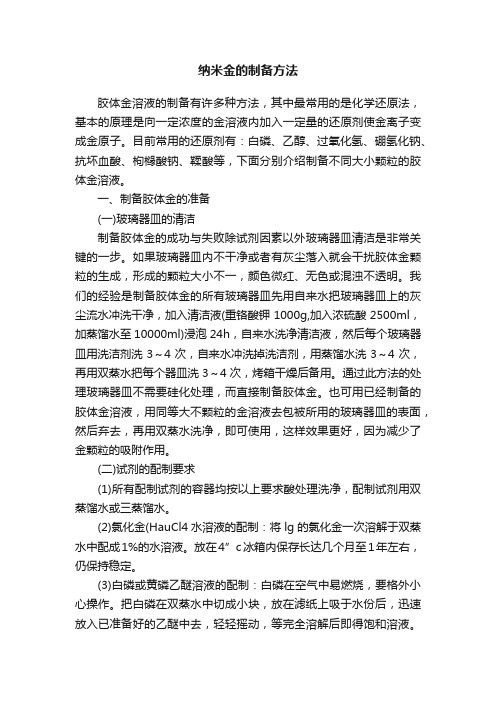
纳米金的制备方法胶体金溶液的制备有许多种方法,其中最常用的是化学还原法,基本的原理是向一定浓度的金溶液内加入一定量的还原剂使金离子变成金原子。
目前常用的还原剂有:白磷、乙醇、过氧化氢、硼氢化钠、抗坏血酸、枸橼酸钠、鞣酸等,下面分别介绍制备不同大小颗粒的胶体金溶液。
一、制备胶体金的准备(一)玻璃器皿的清洁制备胶体金的成功与失败除试剂因素以外玻璃器皿清洁是非常关键的一步。
如果玻璃器皿内不干净或者有灰尘落入就会干扰胶体金颗粒的生成,形成的颗粒大小不一,颜色微红、无色或混浊不透明。
我们的经验是制备胶体金的所有玻璃器皿先用自来水把玻璃器皿上的灰尘流水冲洗干净,加入清洁液(重铬酸钾1000g,加入浓硫酸2500ml,加蒸馏水至10000ml)浸泡24h,自来水洗净清洁液,然后每个玻璃器皿用洗洁剂洗3~4次,自来水冲洗掉洗洁剂,用蒸馏水洗3~4次,再用双蒸水把每个器皿洗3~4次,烤箱干燥后备用。
通过此方法的处理玻璃器皿不需要硅化处理,而直接制备胶体金。
也可用已经制备的胶体金溶液,用同等大不颗粒的金溶液去包被所用的玻璃器皿的表面,然后弃去,再用双蒸水洗净,即可使用,这样效果更好,因为减少了金颗粒的吸附作用。
(二)试剂的配制要求(1)所有配制试剂的容器均按以上要求酸处理洗净,配制试剂用双蒸馏水或三蒸馏水。
(2)氯化金(HauCl4水溶液的配制:将lg的氯化金一次溶解于双蒸水中配成1%的水溶液。
放在4”c冰箱内保存长达几个月至1年左右,仍保持稳定。
(3)白磷或黄磷乙醚溶液的配制:白磷在空气中易燃烧,要格外小心操作。
把白磷在双蒸水中切成小块,放在滤纸上吸于水份后,迅速放入已准备好的乙醚中去,轻轻摇动,等完全溶解后即得饱和溶液。
储藏于棕色密闭瓶内,放在阴凉处保存。
二、制备胶体金的方法和步骤(一)白磷还原法1.白磷还原法(z Sigmondy 1905年)(1)取1%的HAuCl4水溶液1ml,加双蒸水99ml配成0.01%的HAuCl4水溶液。
金纳米粒子
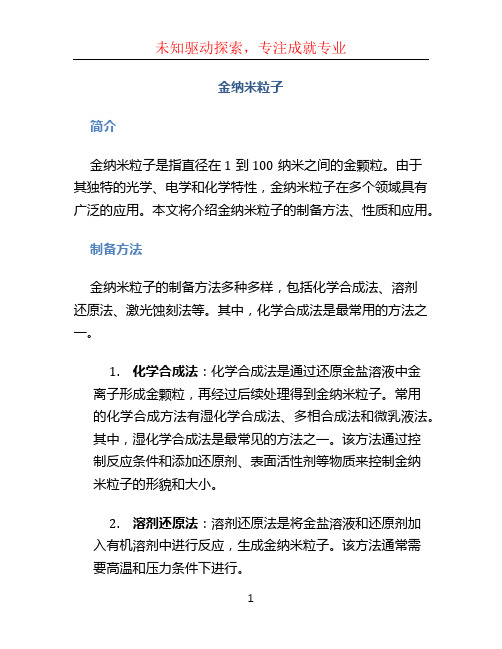
金纳米粒子简介金纳米粒子是指直径在1到100纳米之间的金颗粒。
由于其独特的光学、电学和化学特性,金纳米粒子在多个领域具有广泛的应用。
本文将介绍金纳米粒子的制备方法、性质和应用。
制备方法金纳米粒子的制备方法多种多样,包括化学合成法、溶剂还原法、激光蚀刻法等。
其中,化学合成法是最常用的方法之一。
1.化学合成法:化学合成法是通过还原金盐溶液中金离子形成金颗粒,再经过后续处理得到金纳米粒子。
常用的化学合成方法有湿化学合成法、多相合成法和微乳液法。
其中,湿化学合成法是最常见的方法之一。
该方法通过控制反应条件和添加还原剂、表面活性剂等物质来控制金纳米粒子的形貌和大小。
2.溶剂还原法:溶剂还原法是将金盐溶液和还原剂加入有机溶剂中进行反应,生成金纳米粒子。
该方法通常需要高温和压力条件下进行。
3.激光蚀刻法:激光蚀刻法是利用激光在金膜表面进行局部蚀刻,形成金纳米粒子。
该方法具有高精度和高控制性。
性质金纳米粒子的性质主要包括形状、大小和表面等。
这些性质对金纳米粒子的光学、电学和化学特性有重要影响。
1.形状:金纳米粒子的形状多样,包括球形、棒状、多面体等。
不同形状的金纳米粒子有不同的表面能和电荷分布,从而影响其物理化学性质。
2.大小:金纳米粒子的大小直接影响其表面积和光学性质。
通常情况下,金纳米粒子的光学性质会随着尺寸的减小而发生变化。
3.表面:金纳米粒子的表面往往具有较大的比表面积,在催化、传感等领域具有重要作用。
此外,金纳米粒子的表面还可以进行功能化修饰,以增加其稳定性和特定的化学反应。
应用金纳米粒子因其独特的性质在多个领域具有广泛的应用。
1.生物传感:金纳米粒子可以通过表面修饰与生物分子特异性结合,用于生物传感和检测领域。
例如,利用金纳米粒子可以制备出高灵敏度的生物传感器,用于检测蛋白质、DNA等生物分子。
2.催化剂:金纳米粒子在催化领域具有重要应用。
由于其高比表面积和活性位点,金纳米粒子可以作为有效的催化剂,用于半导体制备、化学反应等。
金纳米粒子的制备方法

紫外照射是能够证明金纳米粒子质量的另一种参数。近红外光照射能够证明用硫醇稳定的金纳米粒子的粒径生长。在少量2-丙醇中,超声波(200kHz)能控制AuCl4-水溶液中的还原速率,同时,通过调结溶液温度和反应器的配置等参数,可以调控纳米粒子的大小。声化学可以利用娃孔进行金纳米粒子的合成,也可以进行Au/Pd双金属粒子的合成。福射已经用于控制金纳米粒子的大小,或是在特殊自由基存在下合成。根据t福射可以检测金纳米粒子的形成机制。在气/液界面处,通过[AuCl(PPh3)]分解,可以得到金纳米粒子。在180℃,N2存在情况下,[C14H29-Me3N][Au(SC12H25)2]热解5小时,制备26nm的焼基组纯化的金纳米粒子。
图1 Au化合物在硫醇溶液中被还原,其Au纳米粒子表面被有机外壳所覆盖
其反应机理如下:
3其它含硫配体
其它含硫配体已经用于稳定金纳米粒子,如黄酸盐和二硫化物等。二硫化物不如硫醇的稳定,但是在催化方面有明显的效果。同样,硫醚不能很好的约束金纳米粒子,但是Rheinhout团队利用聚硫醚就能很好的解决这个问题。另外,利用碘氧化以硫醇为包覆剂的金纳米粒子,使其分解为金的碘化物和二硫化物。Crook等人利用这一现象制备了以金纳米粒子为模版的环胡精的空心球。
2 Brust-Schiffrin法:两相合成并通过硫醇稳定
人们于1994年提出了合成金纳米粒子的Brust-Schiffrin方法。由于热稳定合成方法简单易行,在不到十年的时间内,此方法在所有领域都有重要的影响。金纳米粒子在有机溶剂中能分散和再溶解,并且没有ay的两相合成体系给予合成技术一定的启发,由于Au和S的软性质,这种方法便利用硫醇配体强烈绑住金。四正辛基溴化按作为相转移试剂将AuCV转移到甲苯溶液中,并用NaBH4在正十二硫醇中还原AuCLT。在NaBH4还原过程中,橙色相在几秒内向深棕色转变(图1):
纳米金的制备与表征
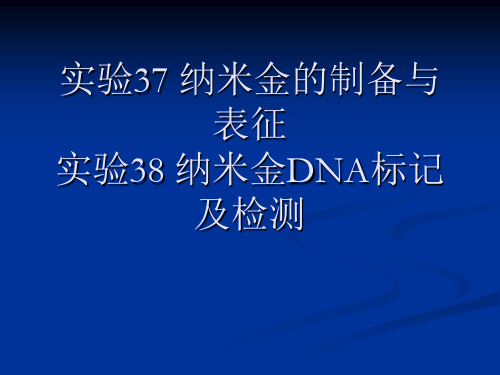
Taton等将这种检测模式用于单核苷酸多态性分析,具体过 Taton等将这种检测模式用于单核苷酸多态性分析,具体过 程如下: 程如下: 针对目的碱基,分别设计四条具有四种不同碱基的 捕获探针; 捕获探针; 按上述流程在支持物上形成由捕获探针、靶基因、 纳米金探针三种成分组成的夹心结构。随后,逐渐升高反应 体系的温度,与目的碱基错配的捕获探针先与靶基因发生变 性,经过冲洗后,靶基因与纳米探针均被洗掉; 性,经过冲洗后,靶基因与纳米探针均被洗掉; 而与目的碱 基配对的捕获探针仍和靶基因及纳米金探针保持夹心结构, 固定在固相支持物上。 因此,当加入银增强液时,存在错配 碱基的固相支持物上无银壳出现,而配对碱基处可见明显的 银壳。
纳米金粒径与等离子吸收峰的关系
纳米金探针的不同检测模式及其在 基因检测中的应用
基因检测主要包括基因序列识别和点突变分析 两大内容。 基因序列识别在基因诊断中具有重要意 义。 点突变检测在诊断遗传性疾病、确定癌基因激 活、抑癌基因失活以及与药物抗性相关的突变中发 挥着重要作用。将纳米金探针应用在基因检测 中,不但可以简化实验步骤,还可大大降低检测成 本。
纳米粒子的光学性质部分依赖于它们在聚合网络中的距离, 当此距离远大于粒子的平均直径时显红色,大致相等时显蓝 色。 杂交能使粒子间距缩短,形成纳米粒子的聚合物,从而 导致体系产生相应的颜色变化。 因此,随着杂交的进行,体 系的颜色将逐渐由红色变成蓝色,根据颜色的变化即可判断 体系中是否含有靶基因。 在该检测模式中,纳米探针比传统 探针具有更好的选择特异性,其检测灵敏度能够达到fmol级。 探针具有更好的选择特异性,其检测灵敏度能够达到fmol级。 Reynolds等采用粒径较大(50nm或100nm)的纳米金探针进 Reynolds等采用粒径较大(50nm或100nm)的纳米金探针进 行基因检测,也取得了较为理想的结果。
纳米金制备
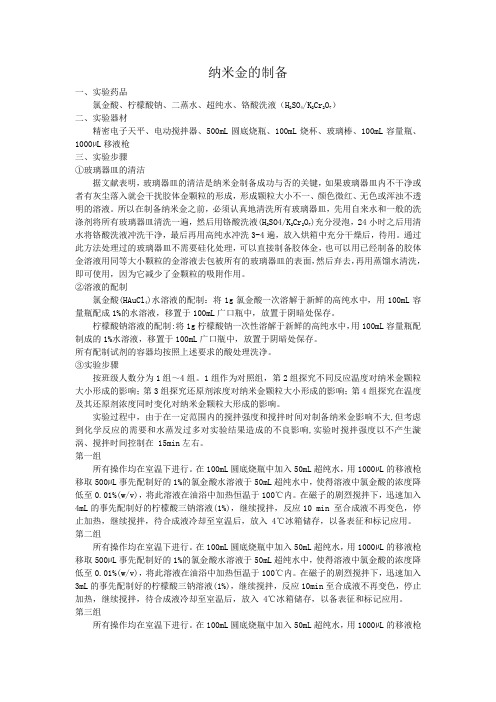
纳米金的制备一、实验药品氯金酸、柠檬酸钠、二蒸水、超纯水、铬酸洗液(H2SO4/K2Cr2O7)二、实验器材精密电子天平、电动搅拌器、500mL圆底烧瓶、100mL烧杯、玻璃棒、100mL容量瓶、1000µL移液枪三、实验步骤①玻璃器皿的清洁据文献表明,玻璃器皿的清洁是纳米金制备成功与否的关键,如果玻璃器皿内不干净或者有灰尘落入就会干扰胶体金颗粒的形成,形成颗粒大小不一、颜色微红、无色或浑浊不透明的溶液。
所以在制备纳米金之前,必须认真地清洗所有玻璃器皿,先用自来水和一般的洗涤剂将所有玻璃器皿清洗一遍,然后用铬酸洗液(H2SO4/K2Cr2O7)充分浸泡,24小时之后用清水将铬酸洗液冲洗干净,最后再用高纯水冲洗3-4遍,放入烘箱中充分干燥后,待用。
通过此方法处理过的玻璃器皿不需要硅化处理,可以直接制备胶体金,也可以用已经制备的胶体金溶液用同等大小颗粒的金溶液去包被所有的玻璃器皿的表面,然后弃去,再用蒸馏水清洗,即可使用,因为它减少了金颗粒的吸附作用。
②溶液的配制氯金酸(HAuCl4)水溶液的配制:将1g氯金酸一次溶解于新鲜的高纯水中,用100mL容量瓶配成1%的水溶液,移置于100mL广口瓶中,放置于阴暗处保存。
柠檬酸钠溶液的配制:将1g柠檬酸钠一次性溶解于新鲜的高纯水中,用100mL容量瓶配制成的1%水溶液,移置于100mL广口瓶中,放置于阴暗处保存。
所有配制试剂的容器均按照上述要求的酸处理洗净。
③实验步骤按班级人数分为1组~4组。
1组作为对照组,第2组探究不同反应温度对纳米金颗粒大小形成的影响;第3组探究还原剂浓度对纳米金颗粒大小形成的影响;第4组探究在温度及其还原剂浓度同时变化对纳米金颗粒大形成的影响。
实验过程中,由于在一定范围内的搅拌强度和搅拌时间对制备纳米金影响不大,但考虑到化学反应的需要和水蒸发过多对实验结果造成的不良影响,实验时搅拌强度以不产生漩涡、搅拌时间控制在 15min左右。
金纳米粒子的合成及应用
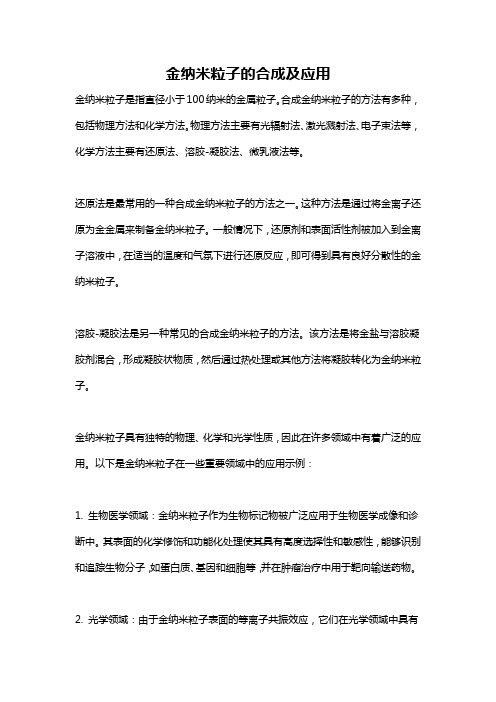
金纳米粒子的合成及应用金纳米粒子是指直径小于100纳米的金属粒子。
合成金纳米粒子的方法有多种,包括物理方法和化学方法。
物理方法主要有光辐射法、激光溅射法、电子束法等,化学方法主要有还原法、溶胶-凝胶法、微乳液法等。
还原法是最常用的一种合成金纳米粒子的方法之一。
这种方法是通过将金离子还原为金金属来制备金纳米粒子。
一般情况下,还原剂和表面活性剂被加入到金离子溶液中,在适当的温度和气氛下进行还原反应,即可得到具有良好分散性的金纳米粒子。
溶胶-凝胶法是另一种常见的合成金纳米粒子的方法。
该方法是将金盐与溶胶凝胶剂混合,形成凝胶状物质,然后通过热处理或其他方法将凝胶转化为金纳米粒子。
金纳米粒子具有独特的物理、化学和光学性质,因此在许多领域中有着广泛的应用。
以下是金纳米粒子在一些重要领域中的应用示例:1. 生物医学领域:金纳米粒子作为生物标记物被广泛应用于生物医学成像和诊断中。
其表面的化学修饰和功能化处理使其具有高度选择性和敏感性,能够识别和追踪生物分子,如蛋白质、基因和细胞等,并在肿瘤治疗中用于靶向输送药物。
2. 光学领域:由于金纳米粒子表面的等离子共振效应,它们在光学领域中具有广泛的应用。
金纳米粒子可用作传感器、光学增强剂和表面改性剂等,可用于改善太阳能电池的效率、调控光信号和增强拉曼散射等。
3. 催化剂领域:金纳米粒子由于其特殊的晶格结构和表面活性,可用作高效催化剂。
金纳米粒子能够催化多种反应,如还原、氧化、氢化和重整等。
例如,金纳米粒子催化的氧化反应广泛应用于生物质能源转化和有机合成等领域。
4. 电子器件领域:金纳米粒子在电子器件中的应用也越来越广泛。
它们可用作柔性电子器件中的导电电极和场发射材料,也可用作表面增强拉曼光谱(SERS)传感器中的基底材料,提高传感器的灵敏度和稳定性。
总之,金纳米粒子作为具有独特性质的纳米材料,其合成方法和应用领域都十分丰富。
随着技术和研究的不断发展,金纳米粒子的合成和应用将进一步拓展,并在更多领域发挥重要作用。
金纳米材料的合成概述

金纳米材料的合成概述纳米材料又称纳米级结构,其广义上指的是在三维空间中,至少有一维处于纳米尺寸范围,因此又称为超精细颗粒材料。
粒子尺寸一般在1~100 nm之间,是处于原子簇和宏观物体交界的过渡区域,从宏观和微观角度来说,它既非处于宏观又非处于微观系统,而是一种典型的介观系统,从而具有小尺寸效应,宏观量子隧道效应和表面效应。
1. 金纳米的合成方法(1)微乳液法Brust-Schiffrin通过反复实验,于1994年通过以微乳液为介质,制备出既能够溶于有机溶剂,又拥有较好稳定性的纳米金粒子。
(2)晶生长法通常情况下在晶生长法中,金纳米棒的模板采用的是表面活性剂,利用种子生长法来进行制备。
(3)模板法起初,模板法是利用电化学中的镀层方法在聚碳酸酯膜和氧化铝板膜上沉积金,后来,随着技术的发展,该方法不仅应用于纳米复合材料的制备,还能够对用过模板法合成的金纳米棒起到再分散的作用。
由于金纳米棒和氧化铝复合材料在可见光范围内都是透明的,所以想要得到不同程度的颜色复合膜可以通过改变沉积的金纳米棒的长径比来实现。
该方法大致步骤如下:一,将少量的银或者铜电镀到铝板模上作为电化学沉积的传导层;二,使金通过氧化铝纳米孔道进行电化学沉积;三,选择性地溶解氧化铝分子膜和银或者铜的薄膜(反应过程中的稳定剂选择PVP);四,通过超声波或者搅拌,使金纳米棒分散在水或者有机溶剂中。
由于金粒子的直径与氧化铝相同,因此可以通过控制膜孔的直径以达到控制金纳米棒直径的目的。
金纳米管、纳米结构复合材料均可通过该技术来实现。
(4)电化学法该方法的实验装置是由金的金属板做为阳极,相同面积的铂金属板作为阴极组成的电化学电池的构成,生成金纳米棒过程中利用CTAB作为诱导表面活性剂,将电极浸在含有C16TAB和少量C12TAB的电解质溶液中,置于室温下超声,电解前在电解质溶液中加入适量丙酮和环己烷,电解30 min,电流控制在3 mA。
反应过程中金先在阳极形成AuBr4-,然后迅速与阳离子表面活性剂结合并转至阴极被还原。
纳米金的制备与性能课件
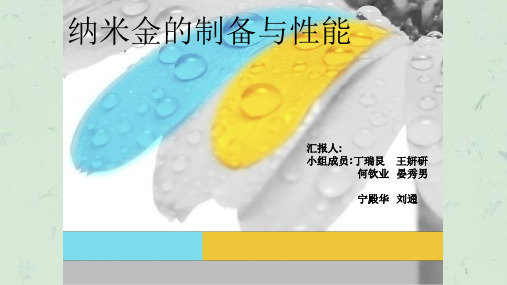
Challenge纳米金探针的放大信号。
需要提高实际样品检 测分析过程中的稳定 性
挑战 Hope
希望降低金纳米粒 子尺寸,改善形貌, 制备尺寸均一、形 状可控的金纳米粒 子
纳米金的制备与性能
19
Thank you
纳米金的制备与性能
20
此课件下载可自行编辑修改,供参考! 感谢您的支持,我们努力做得更好!
content increase from sample no. 1 to 5.(b) The aspect ratios of NRs
obtained from size measurements show an纳i米n金cr的ea制s备e 与性能
9
CHEM.MATER.2013,25,4537-4544
纳米金的制备与性能研究历史19世纪20世纪4世纪变色的罗马酒杯lycurguscup法拉第首次报道纳米金溶胶有关纳米金溶胶的制备和性质研究明显增21世纪各向异性的纳米金颗粒制备及在生物医学等方面的应用纳米金的制备与性能纳米金特性势垒宽度电子能量和多种有机或生物配体相互作用量子尺寸效应大比表面积纳米金特性单电子跃迁库仑阻塞制备简单颗粒稳定纳米金的制备与性能表征手段结构表征xrdedirnmrraman性质表征光电磁热力uvvisplvms形貌表征temsemstmafm成份分析aasaesmseaxpsxrf纳米金的制备与性能goldnanoparticle晶种生长法合成不同长径比的金棒光化学法合成不同长径比的金棒电化学法合成金纳米棒超声化学法合成金纳米带模板法合成sio2au核壳颗粒纳米金的制备与性能seedmediatedgrowthmethod制备种子溶液ctab5ml02m加入haucl5ml0005m06ml001m搅拌2min25水浴黄褐色
- 1、下载文档前请自行甄别文档内容的完整性,平台不提供额外的编辑、内容补充、找答案等附加服务。
- 2、"仅部分预览"的文档,不可在线预览部分如存在完整性等问题,可反馈申请退款(可完整预览的文档不适用该条件!)。
- 3、如文档侵犯您的权益,请联系客服反馈,我们会尽快为您处理(人工客服工作时间:9:00-18:30)。
Seeding Growth for Size Control of5-40nm DiameterGold NanoparticlesNikhil R.Jana,*Latha Gearheart,and Catherine J.Murphy* Department of Chemistry and Biochemistry,University of South Carolina,631Sumter Street,Columbia,South Carolina29208Received March22,2001.In Final Form:August7,2001Following a seeding growth approach,gold nanoparticles of diameters5-40nm were prepared with 10-15%standard deviation in diameter from3.5(0.7nm gold particle seeds.Particle size can be controlled by varying the ratio of seed to metal salt,and thus any size in the range5-40nm can be prepared.The method can also be scaled up to produce10-100mg of gold nanoparticles.IntroductionMetal particles in the nanometer size regime show characteristic size-dependent properties different from bulk metals with the most significant size effects occurring for1-10nm diameters.1-7Consequently,extensive in-vestigations involving metallic,especially gold,nanopar-ticles as building blocks for nanoscale materials and devices are currently underway.8-19To expedite such studies,bulk quantities of particles of uniform size are necessary.There are many synthetic methods to make gold nanoparticles;20-39however,only a few methods produce particles of uniform size.The most common approach involves citrate reduction of a gold salt to produce 12-20nm size gold particles with a relatively narrow size distribution(standard deviation,∼10-16%).20,21The most popular method for producing smaller gold particles was developed by Brust et al.This method utilizes borohydride reduction of gold salt in the presence of an alkanethiol capping agent to produce1-3nm particles.22 By varying the thiol concentration,sizes can be controlled between2and5nm.23Phosphine-stabilized gold clusters (1.4(0.4nm)have also been prepared and further converted to thiol-capped clusters by ligand exchange in order to improve their stability,24-30and recently phos-phine-stabilized monodispersed gold particles(1.5(0.4 nm)were prepared using a similar protocol to the Brust method.30Most of the other methods to make small gold clusters (1-5nm)take advantage of the strong capping action of thiols.31,34,36Capping agents include disulfides,31polymers with mercapto and cyano functional groups,34and den-drimers.32,33Varying the capping agent concentration allows for size control between1and4nm with good monodispersity,but any attempts to make larger size particles(>5nm)with these procedures lead to a wider particle size distribution(standard deviation∼25-100%),23,39and further narrowing of size distribution is required.40-43Occasionally,aging,annealing,or ligand*To whom correspondence should be addressed.E-mail murphy@ and jana@.(1)Creighton,J.A.;Eadon,D.G.J.Chem.Soc.,Faraday Trans. 1991,87,3881.(2)Henglein,A.J.Phys.Chem.1993,97,5457.(3)Belloni,J.Curr.Opin.Colloid Interface.Sci.1996,1,184.(4)Valden,M.;Lai,X.;Goodman,D.W.Science1998,281,1647.(5)Link,S.;Burda,C.;Wang,Z.L.;El-Sayed,M.A.J.Chem.Phys. 1999,111,1255.(6)Link,S.;El-Sayed,M.A.J.Phys.Chem.B1999,103,8410.(7)Hodak,J.H.;Henglein,A.;Hartland,G.V.J.Phys.Chem.B 2000,104,9954.(8)Schmid,G.;Hornyak,G.L.Curr.Opin.Solid State Mater.Sci. 1997,2,204.(9)Whetten,R.L.;Shafigullin,M.N.;Khoury,J.T.;Schaaff,T.G.; Vezmar,I.;Alvarez,M.M.;Wilkinson,A.Acc.Chem.Res.1999,32,397.(10)Dirix,Y.;Bastiaansen,C.;Caseri,W.;Smith,P.J.Mater.Sci. 1999,34,3859.(11)Dirix,Y.;Bastiaansen,C.;Caseri,W.;Smith,P.Adv.Mater. 1999,11,223.(12)Caseri,W.Macromol.Rapid Commun.2000,21,705.(13)Kell,A.J.;Stringle,D.L.B.;Workentin,.Lett.2000, 2,3381.(14)Labande,A.;Astruc,mun.2000,1007.(15)Wu,M.L.;O’Neill,S.A.;Brousseau,L.C.;McConnell,W.P.; Shultz,D.A.;Linderman,R.J.;Feldheim,mun.2000, 775.(16)Hainfeld,J.F.;Powell,R.D.J.Histochem.Cytochem.2000,48, 471.(17)Templeton,A.C.;Wuelfing,M.P.;Murray,R.W.Acc.Chem. Res.2000,33,27.(18)Martin,J.E.;Wilcoxon,J.P.;Odinek,J.;Provencio,P.J.Phys. Chem.B2000,104,9475.(19)Pileni,M.P.;Tanori,J.;Filankembo,A.Colloid Surf.A1997, 123,561.(20)Turkevich,J.;Garton,G.;Stevenson,P.C.J.Colloid Sci.1954, 9,26.(21)Frens,G.Nature1973,241,20.(22)Brust,M.;Walker,M.;Bethell,D.;Schiffrin,D.J.;Whyman,R. mun.1994,801.(23)Hostetler,M.J.;Wingate,J.E.;Zhong,C.J.;Harris,J.E.;Vachet, R.W.;Clark,M.R.;Londono,J.D.;Green,S.J.;Stokes,J.J.;Wignall, G.D.;Glish,G.L.;Porter,M.D.;Evans,N.D.;Murray,ngmuir 1998,14,17.(24)Schmid,G.;Pfeil,R.;Boese,R.;Bandrmann,F.;Meyer,S.;Calis,G.H.M.;van der Velden,J.W.A.Chem.Ber.1981,114,3634.(25)Rapoport,D.H.;Vogel,W.;Colfen,H.;Schlogl,R.J.Phys.Chem. B1997,101,4175.(26)Schmid,G.;Meyer-Zaika,W.;Pugin,R.;Sawitowski,T.;Majoral, J.P.;Caminade,A.M.;Turrin,C.O.Chem.Eur.J.A2000,6,1693.(27)Brown,L.O.;Hutchison,J.E.J.Am.Chem.Soc.1997,119, 12384.(28)Brown,L.O.;Hutchison,J.E.J.Am.Chem.Soc.1999,121,882.(29)Warner,M.G.;Reed,S.M.;Hutchison,J.E.Chem.Mater.2000, 12,3316.(30)Weare,W.W.;Reed,S.M.;Warner,M.G.;Hutchison,J.E.J. Am.Chem.Soc.2000,122,12890.(31)Yonezawa,T.;Yasui,K.;Kimizuka,ngmuir2001,17,271.(32)Zhao,M.Q.;Sun,L.;Crooks,R.M.J.Am.Chem.Soc.1998,120, 4877.(33)Esumi,K.;Hosoya,T.;Suzuki,A.;Torigoe,K.J.Colloid Interface Sci.2000,229,303.(34)Teranishi,T.;Kiyokawa,I.;Miyake,M.Adv.Mater.1998,10, 596.(35)Yonezawa,T.;Sutoh,M.;Kunitake,T.Chem.Lett.1997,619.(36)Chen,S.H.;Kimura,ngmuir1999,15,1075.(37)Green,M.;O’Brien,mun.2000,183.(38)Brust,M.;Bethell,D.;Kiely,C.J.;Schiffrin,ngmuir 1998,14,5425.(39)Leff,D.V.;Ohara,P.C.;Heath,J.R.;Gelbart,W.M.J.Phys. Chem.1995,99,7036.6782Langmuir2001,17,6782-678610.1021/la0104323CCC:$20.00©2001American Chemical SocietyPublished on Web10/02/2001exchange improves size dispersion,but at the expense of small particle size.18,28Difficulty in controlling the nucleation and growth steps occurring at intermediate stages of particle formation results in a broad particle size distribution.3For the citrate reduction method,citrate acts both as a reducing agent and as a capping agent,and due to its weak reducing and capping character,the average size is relatively large.In comparison,the Brust method uses a strong reducing agent(NaBH4),causing more nucleation,and a strong capping agent(alkanethiol)which drastically inhibits growth.Even with a strong capping agent,however, Murray et al.have shown that thiol-capped particles slowly grow within the following60h of preparation.43 Another common approach for controlling the size of nanoparticles is seeding growth.44-55In seeding growth methods,small metal particles are prepared first and later used as seeds(nucleation centers)for the preparation of larger size particles.Recently,seeding growth methods were developed for size control of Au,Ag,Ir,Pd,and Pt particles.48-55Providing a controlled number of preformed seeds(as nucleation centers)and a growth condition that inhibits any secondary nucleation,the particle size can be controlled simply by varying the ratio of seed to metal salt.However,difficulty in finding a suitable growth condition that inhibits additional nucleation during the growth stage limits the application of such methods.46,47 In general,these conditions include using a reducing agent too weak to reduce the metal salt(in the growth stage) without the presence of seeds.We have observed in other work that the presence of seeds often induced further nucleation rather than growth when the ratio of seed to metal salt was relatively small,resulting in a broad size distribution.56To avoid additional nucleation,a step by step particle enlargement method was more useful, allowing a large seed to metal salt ratio to be maintained throughout successive growth steps.56Another limitation of seeding growth is the lack of availability of smaller seeds with a narrow size distribution,and thus small particles1-10nm are difficult to synthesize.In this paper,we used3.5nm(standard deviation∼20%) gold particles as seeds to prepare gold nanoparticles in the size range5-40nm that are relatively uniform in size (standard deviation∼10-15%).The gold seeds were prepared by borohydride reduction of gold salt in the presence of citrate,a capping agent.52Secondary nucle-ation during the growth stage was inhibited by carefully controlling the growth conditions using a weak reducing agent,aqueous surfactant,and performing step by step seeding.In addition,the presence of surfactant stabilizes the particles for over1month.Experimental SectionMaterials.Trisodium citrate(Fisher),HAuCl4‚3H2O(Sigma), ascorbic acid(Aldrich),NaBH4(Mallinckrodt),and cetyltrim-ethylamonium bromide(CTAB)(Aldrich)were used as received. Ultrapure deionized water(Continental Water Systems)was used for all solution preparations.All glassware was cleaned with aqua regia.Preparation of3.5(0.7nm Gold Seed.A20mL aqueous solution containing2.5×10-4M HAuCl4and2.5×10-4M trisodium citrate was prepared in a conical flask.Next,0.6mL of ice-cold,freshly prepared0.1M NaBH4solution was added to the solution while stirring.The solution turned pink immediately after adding NaBH4,indicating particle formation.The particles in this solution were used as seeds within2-5h after preparation. Here,citrate serves only as a capping agent since it cannot reduce the gold salt at room temperature(25°C).Preparation of Growth Solution.A200mL aqueous solution of2.5×10-4M HAuCl4was prepared in a conical flask. Next,6g of solid cetyltrimethylammonium bromide(0.08M final concentration)was added to the solution,and the mixture was heated until the solution turned a clear orange color.The solution was cooled to room temperature and used as a stock growth solution.Seeding Growth.Four sets of50mL conical flasks were labeled A,B,C,and D.In set A,7.5mL of growth solution was mixed with0.05mL of freshly prepared0.1M ascorbic acid solution.Next,2.5mL of seed solution was added while stirring. Stirring continued for10min after the solution turned wine red. Particles prepared this way were spherical with a diameter of 5.5(0.6nm.Similarly,9mL of growth solution and0.05mL of0.1M ascorbic acid solution were mixed as set B,and1.0mL of seed solution was added while vigorously stirring.Stirring continued for10min.The solution’s final color was deep red.Particles prepared this way were spherical with a diameter of8.0(0.8 nm.The particles prepared here were used as seeds in set C30 min after preparation.In set C,9mL of growth solution was mixed with0.05mL of 0.1M ascorbic acid solution,and1.0mL from set B was added while stirring vigorously.Stirring was continued for10min.The final color of the solution was reddish brown.Particles prepared in this way were roughly spherical with a diameter of17(2.5 nm.This solution was used as seed in set D30min after preparation.In set D,9mL of growth solution was mixed with0.05mL of 0.1M ascorbic acid solution,and1.0mL from set C was added while stirring vigorously.Stirring continued for the next10min, and the final color of the solution was brown.Particles prepared this way consisted of a mixture of spheres(37(5nm diameter) and rods(with an average major axis of200nm and minor axis of17nm).The solutions A,B,C,and D were stable for more than a month due the presence of CTAB as a particle stabilizer.Each solution,A-D,contained2.5×10-4M gold(atoms).Thiol Capping and Subsequent Solvent Extraction.First, 10µL of dodecanethiol was added to10mL of particle solution (to make17-fold excess of dodecanethiol compared to gold)and stirred for1h.Next,20mg of KI and0.5mL of toluene were added to the particle solution and shaken for1-2min.The upper toluene portion was red due to particle extraction,and the water phase was colorless.The KI was added to enhance phase transfer. Often salts or acids are added to induce nanoparticle phase transfer by screening the interparticle electrostatic repulsion.57-59 We tested HCl,NaCl,and NaBr;however,the phase separation using these acid and salts was slower(separation after24h)(40)Whetten,R.L.;Khoury,J.T.;Alvarez,M.M.;Murthy,S.;Vezmar,I.;Wang,Z.L.;Stephens,P.W.;Cleveland,C.L.;Luedtke,W.D.; Landman,U.Adv.Mater.1996,8,428.(41)Schaaff,T.G.;Knight,G.;Shafigullin,M.N.;Borkman,R.F.; Whetten,R.L.J.Phys.Chem.B1998,102,10643.(42)Schaaff,T.G.;Whetten,R.L.J.Phys.Chem.B2000,104,2630.(43)Chen,S.W.;Templeton,A.C.;Murray,ngmuir2000, 16,3543.(44)Zsigmondy,R.;Thiessen,P. A.Das kolloide Gold;Akad. Verlagsges:Leipzig,1925.(45)Turkevich,J.;Hillier,J.Anal.Chem.1949,21,475.(46)Overbeek,J.Th.G.Adv.Colloid Interface Sci.1982,15,251.(47)Wiesner,J.;Wokaun,A.Chem.Phys.Lett.1989,157,569.(48)Schneider,S.;Halbig,P.;Grau,H.;Nickel,U.Photochem. Photobiol.1994,60,605.(49)Watzky,M.A.;Finke,R.G.Chem.Mater.1997,9,3083.(50)Brown,K.R.;Natan,ngmuir1998,14,726.(51)Brown,K.R.;Walter,D.G.;Natan,M.J.Chem.Mater.2000, 12,306.(52)Henglein,A.;Giersig,M.J.Phys.Chem.B1999,103,9533.(53)Henglein,ngmuir1999,15,6738.(54)Teranishi,T.;Miyake,M.Chem.Mater.1998,10,594.(55)Teranishi,T.;Hosoe,M.;Tanaka,T.;Miyake,M.J.Phys.Chem. B1999,103,3818.(56)Jana,N.R.;Gearheart,L.;Murphy,C.J.Chem.Mater.2001, 13,2313.(57)Hirai,H.;Aizawa,H.;Shiozaki,H.Chem.Lett.1992,1527.(58)Sarathy,K.V.;Kulkarni,G.U.;Rao,mun. 1997,537.(59)Pal,T.;Sau,T.K.;Jana,N.R.J.Colloid Interface Sci.1998, 202,30.Gold Nanoparticles Langmuir,Vol.17,No.22,20016783compared to using KI,which occurred in5-6h.Similar results were observed for the phase transfer of Ag nanoparticles.59 Procedure for Scaling Up.Seeding growth was performed similar to the procedure outline above for sets A,B,C,and D, but with higher concentrations of seed and gold salt.The procedure for the3.5nm seed preparation was the same.(Seed solutions prepared from concentrated gold salt were unstable.) The growth solution contained the same CTAB concentration but10times more HAuCl4(2.5×10-3M).The volume of growth solution used in each set,labeled A′,B′,C′,and D′,was the same as above.For sets A′and B′,10times more volume of3.5nm seed was used(25and10mL,respectively).1.0mL of B′was used for preparing C′,and1.0mL of C′was used for preparing D′.Ten times more volume of ascorbic acid(0.1M)was used for all sets. The samples were centrifuged or extracted in toluene,depending on the particle size,to concentrate the particles and remove excess surfactant.This procedure was also scaled up by increasing the volume of growth solution to100mL and accordingly increased the volume of the seed solutions and ascorbic acid solution.Thus, we can prepare10-100mg of solid particles(including CTAB) that can be redispersed.Instrumentation.Absorption spectra of the prepared solu-tions were measured using a CARY500Scan UV-vis-NIR spectrophotometer.Transmission electron microscopy(TEM) images were acquired with a JEOL JEM-100CXII electron microscope.TEM grids were prepared by placing1µL of the particle solution on a carbon-coated copper grid and drying at room temperature.TEM images were made from different parts of the grid and with different magnifications.Approximately200 particles were counted and measured for size distribution using AMT Kodak digital camera software.Results and DiscussionThe orange color of the gold salt in the CTAB solution disappeared when ascorbic acid was added.We have attributed this color change to the reduction of Au3+to Au+according to the reduction potential of Au3+/Au+(+0.8 V vs NHE in the presence of Br-)and ascorbic acid(+0.13 V vs NHE).However,the reduction of Au+to Au0does not occur,and we do not observe the gold plasmon band indicative of Au0nanoparticles even after24h.This indicates that ascorbic acid is too weak to reduce Au+under our experimental conditions.However,the gold salt reduction to elemental gold nanoparticles occurs in the presence of3.5nm seed particles.This is consistent with our earlier work in which we observed that the rate of gold salt reduction by ascorbic acid was enhanced in the presence of12nm size gold particle seeds.56Seeding growth methods,involving the growth of small particles(seeds)into larger particles,were used to prepare different sized gold nanoparticles.We followed either one step or step by step seeding growth depending on the target particle size.Step by step seeding employs subsequently prepared larger particles as seeds for later stage particle enlargement.Three sets of spherical particles corresponding to sets A,B,and C having diameters of5.5(0.6,8.0(0.8and 17(2.5nm,respectively,were prepared from the3.5( 0.7nm seed by this seeding growth method(Table1).The 5.5and8.0nm particles were prepared by one-step seeding but by varying the ratio of seed to metal salt.The17nm size particles were prepared by step by step seeding where 3.5and8nm particles were used as seeds in the first and second steps of the growth processes,respectively.When 17nm particles were used as seed for further size enlargement,a fraction of particles were nonspherical (rodlike,oblate,and platelike)in shape.For example,in set D we prepared37(5nm spheres with a significant amount(∼10%)of rods(∼200nm major axis and17nm minor axis).By centrifugation,the rods were removed from the solutions of spheres.When one-step seeding was used to make particles20-50nm in size using3.5nm seeds,short rods(with∼60nm major axis and16nm short axis)and plates were formed.60The UV-vis spectra,TEM images,and histograms for different size particles are shown in Figures1,2,and3, respectively.The particle plasmon absorption band at524 nm becomes sharp and intense with increasing particle size from3.5to37nm.The plasmon band red shifts from 520to530nm for particle sizes between3.5and37nm. The absorbance increase for37nm size particles was not prominent(∼2%)because some of the gold salt reduced to form rods(∼10%in number)which were subsequently separated.The calculated molar extinction coefficients for the different size gold particles are given in Table1. These values increase linearly with the particle volume and are on the order of or greater than the absorptivity of organic dyes.The extinction coefficient values of our particles are consistent with other results.6The relative standard deviation of the average particle size decreased (compared to seed)for5.5and8.0nm size particles (standard deviation11%and10%,respectively)but became wider for17nm diameter particles(standard deviation15%)due to the formation of nonspherical shapes.For37nm particles polydispersity was very high due to the formation of rods and plates.When spheres(60)Jana,N.R.;Gearheart,L.;Murphy,C.J.J.Phys.Chem.B2001, 105,4065.Table1.Data for Gold Nanoparticles sample,measdparticle diam(nm),%SDcalcdparticle size(nm)aplasmonband max(nm),M-1cm-1(molesparticles)b seed,3.5(0.7,20%5200.4×107 A,5.5(0.6,11% 5.6524 1.8×107 B,8.0(0.8,10%7.55247.0×107 C,17(2.5,15%1652478×107 D,37(5,14%35530540×107a See text for formula.b We estimated the concentration of nanoparticles produced from knowing the total amount of Au atoms in solution for seed plus gold from the added salt and then determining how many atoms would fit in each particle.The volume of a sphere corresponding to the seed or the resultant particle was calculated,and the total number of atoms fitting into each volume was determined using the crystal structure of gold(cubic unit cell )4.0786Åon edge,4gold atoms/unit cell),giving us the average number of atoms/particle.The number of particles in each sample was calculated by dividing the total number of gold atoms by the atoms/particle.This was converted to number of moles of particles/liter.Figure1.UV-vis spectra of3.5(0.7nm gold seed and the larger gold particles prepared from seed.The spectra for the 37(5nm sample was taken after separation of rods.The gold concentration(in terms of gold salt)was10-4M for all sizes.6784Langmuir,Vol.17,No.22,2001Jana et al.were separated from rods and plates,the standard deviation of the 37nm size particles was 14%.The utility of our synthetic method is that the desired size particles are prepared by varying the seed to metal salt ratio.The expected size can be calculated from a simple theoretical equation:r )r seed {([M added ]+[M seed ])/[M seed ]}1/3where r s and r indicate particle radius for seed and larger particle,respectively,and [M seed ]and [M added ]indicate metal concentrations in seed and added ion,respec-tively.45,46,48Table 1compares the calculated and experi-mental results.The measured diameters closely match the calculated diameters (the calculation assumes only growth occurs to give spherical particles)and provides support for the notion that only growth occurs in our experiments.Surface derivatization of nanoparticles is often neces-sary to control their self-assembly and physical proper-ties.17,61Successful surface derivatization has been achieved by ligand exchange reactions of alkanethiol-capped gold particles with other functionalized thiol derivatives.17Such derivatization processes often lead to particle aggrega-tion.29Our surfactant stabilized gold nanoparticles have two advantages in this respect.First,the particle stabi-lization does not involve the formation of a strong metal -ligand bond (as in gold particle -thiol composite),and the surfactant can be easily removed.Second,the presence of a surfactant can solubilize the organic functional com-pounds that are otherwise insoluble in water.We have surface derivatized our nanoparticles with dodecanethiol,a widely used surface derivatizing agent,17,61to make the particles hydrophobic and extractable in organic solvent.Particles of 3.5-8nm in size were extracted into organic solvent after thiol capping.Dodecanethiol capped 8(0.8nm particles showed a tendency to form a superlatice viaself-assembly (Figure 4).Particles >8nm were not extracted into the organic solvent even after thiol capping and formed particle films at the aqueous -organic inter-face.Sastry et al.observed a similar film formation during the solvent extraction of carboxylic acid-derivatized 13nm gold particles in the presence of octadecylamine.62They also observed a size dependence for the particle partition-ing process.63,64Pileni et al.observed the influence of particle size and alkyl chain length on the extraction of alkanethiol-capped Ag 2S into organic phase.65Particles 2-5nm in size can be extracted into organic solvent but 7.6nm particles cannot,even after varying the alkyl chain length.65This size-dependent solvent extraction behavior has been used for separation of different sized particles(61)Collier,C.P.;Vossmeyer,T.;Heath,J.R.Annu.Rev.Phys.Chem.1998,49,371.(62)Mayya,K.S.;Sastry,ngmuir 1999,15,1902.(63)Mayya,K.S.;Sastry,M.J.Phys.Chem.B 1997,101,9790.(64)Gole,A.M.;Sathivel,chke,A.;Sastry,M.J.Chromatogr.A 1999,848,485.(65)Motte,L.;Pileni,M.P.J.Phys.Chem.B 1998,102,4104.Figure 2.TEM image of larger gold particles prepared from seed:(a)5.5(0.6,(b)8.0(0.8,(c)17(2.5,and (d)37(5nm after separation of rods.The 5.5(0.6nm particles were extracted into toluene after thiol capping for TEM in order to remove excess surfactant.The other size particles were separated from excess surfactant bycentrifugation.Figure 3.Histograms of gold particles grown from 3.5nm seed:(a)5.5(0.6,(b)8.0(0.8,(c)17(2.5,and (d)37(5nm after separation of rods.Gold Nanoparticles Langmuir,Vol.17,No.22,20016785and to narrow the particle size distribution.19,61The exact mechanism of the phase transfer is not well established;however,it is related to the formation of a stable hydrophobic monolayer of thiols on the particle surface,where the stability depends on particle size.65Many studies involving nanoparticles need appreciable amounts of particles in the solid phase.The Brust method of making gold nanoparticles allows for this,but few other methods are conducive to scaling up.22Accordingly,we prepared more concentrated solutions of nanoparticles.The method works well for a 10-fold increase in reagent concentrations but with a compromise in standard devia-tion (∼20%)for gold nanoparticles 5.5nm in size.However,for our 8,17,and 37nm size particles,the standard deviations were 10-15%.For 37nm size particles,formation of rods are suppressed,possibly due to less availability of rodlike micelles at high reagent concentra-tions.66Seeding growth methods have been employed to prepare gold particles of varied sizes,from 20to 50nm.50-53Unfortunately,the preparation of particles less than 10nm by seeding growth has been problematic because of the difficulty in preparing small,monodisperse seeds and because of the tendency for both particles and seeds toaggregate.51,67In this work we used citrate-capped,borohydride-reduced 3.5nm (standard deviation 20%)size gold particles as the seed.We have used an aqueous surfactant (CTAB)media for a growth environment which inhibited particle aggregation during growth and stabi-lized the particles after they formed.Earlier,Natan et ed citrate-capped,borohydride-reduced 2.6nm and citrate-reduced 12nm gold particles for making particles >20nm and observed a decrease,or focusing,of the percent relative standard deviation of the average particle size as the particles grew.51Henglein et ed ∼2nm particles as seed (prepared by γirradiation in the presence of polymer)to make 5-50nm particles with particle size distribution focused for 53nm (standard deviation ∼13%)compared to 5nm (standard deviation ∼44%)particles.53Our results indicate a similar focusing of the size distribution with increasing particle size from 3.5to 8nm.However,for particles larger than 8nm,the appear-ance of nonspherical faceted shapes,rods,and plates contribute to the size polydispersity.For diffusion-controlled growth,the particle size distribution should be focused with increasing size since small particles will grow faster than larger particles.68-70The particle mediated growth of metal nanoclusters has been extensively stud-ied.7,71The presence of particles strongly catalyzes the reduction and growth processes,and hence a diffusional growth mechanism may be contributing to growth.ConclusionWe have developed a seeding growth approach to prepare gold nanoparticles 5-40nm in diameter with a very narrow size distribution (SD ∼10-15%).The particle sizes can be easily manipulated by varying the ratio of seed to metal salt.To avoid any secondary nucleation,a step by step particle enlargement is more effective than a one-step seeding method.In addition,this method can be used for larger scale synthesis of gold nanoparticles.Acknowledgment.We thank the National Science Foundation for funding this research.LA0104323(66)Tornblom,M.;Henriksson,U.J.Phys.Chem.B 1997,101,6028.(67)Mallick,K.;Wang,Z.L.;Pal,T.J.Photochem.Photobiol.A 2001,140,75.(68)Reiss,H.J.Chem.Phys.1951,19,482.(69)Peng,X.G.;Wickham,J.;Alivisatos,A.P.J.Am.Chem.Soc.1998,120,5343.(70)Liu,H.;Penner,R.M.J.Phys.Chem.B 2000,104,9131.(71)Gachard,E.;Remita,H.;Khatouri,J.;Keita,B.;Nadjo,L.;Belloni,J.New J.Chem.1998,22,1257.Figure 4.TEM image of the self-assembly of dodecanethiol-capped 8.0(0.8nm gold particles.6786Langmuir,Vol.17,No.22,2001Jana et al.。