对比三种纳米金的催化活性
纳米金属催化剂的制备方法及其比较_宁慧森
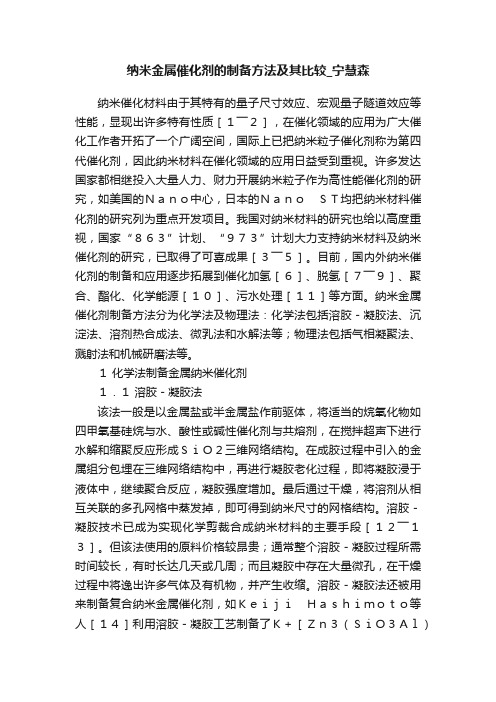
纳米金属催化剂的制备方法及其比较_宁慧森纳米催化材料由于其特有的量子尺寸效应、宏观量子隧道效应等性能,显现出许多特有性质[1 ̄2],在催化领域的应用为广大催化工作者开拓了一个广阔空间,国际上已把纳米粒子催化剂称为第四代催化剂,因此纳米材料在催化领域的应用日益受到重视。
许多发达国家都相继投入大量人力、财力开展纳米粒子作为高性能催化剂的研究,如美国的Nano中心,日本的NanoST均把纳米材料催化剂的研究列为重点开发项目。
我国对纳米材料的研究也给以高度重视,国家“863”计划、“973”计划大力支持纳米材料及纳米催化剂的研究,已取得了可喜成果[3 ̄5]。
目前,国内外纳米催化剂的制备和应用逐步拓展到催化加氢[6]、脱氢[7 ̄9]、聚合、酯化、化学能源[10]、污水处理[11]等方面。
纳米金属催化剂制备方法分为化学法及物理法:化学法包括溶胶-凝胶法、沉淀法、溶剂热合成法、微乳法和水解法等;物理法包括气相凝聚法、溅射法和机械研磨法等。
1化学法制备金属纳米催化剂1.1溶胶-凝胶法该法一般是以金属盐或半金属盐作前驱体,将适当的烷氧化物如四甲氧基硅烷与水、酸性或碱性催化剂与共熔剂,在搅拌超声下进行水解和缩聚反应形成SiO2三维网络结构。
在成胶过程中引入的金属组分包埋在三维网络结构中,再进行凝胶老化过程,即将凝胶浸于液体中,继续聚合反应,凝胶强度增加。
最后通过干燥,将溶剂从相互关联的多孔网格中蒸发掉,即可得到纳米尺寸的网格结构。
溶胶-凝胶技术已成为实现化学剪裁合成纳米材料的主要手段[12 ̄13]。
但该法使用的原料价格较昂贵;通常整个溶胶-凝胶过程所需时间较长,有时长达几天或几周;而且凝胶中存在大量微孔,在干燥过程中将逸出许多气体及有机物,并产生收缩。
溶胶-凝胶法还被用来制备复合纳米金属催化剂,如KeijiHashimoto等人[14]利用溶胶-凝胶工艺制备了K+[Zn3(SiO3Al)10(OH)2]-纳米粒子用于醇脱氢反应。
CTAB包被的不同形态纳米金的制备及其抗菌性能研究
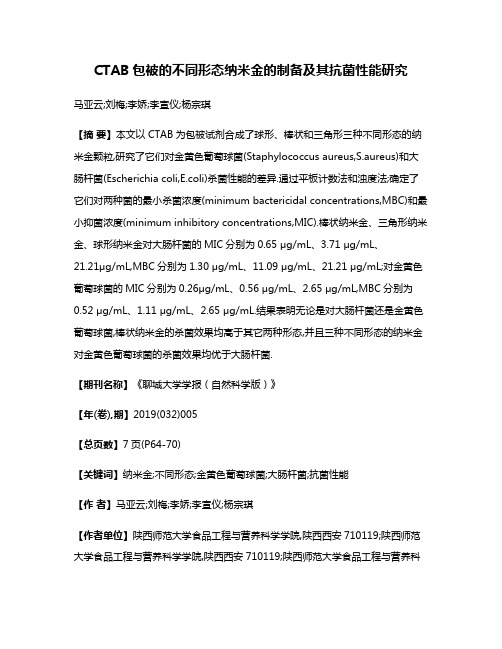
CTAB包被的不同形态纳米金的制备及其抗菌性能研究马亚云;刘梅;李娇;李宣仪;杨宗琪【摘要】本文以CTAB为包被试剂合成了球形、棒状和三角形三种不同形态的纳米金颗粒,研究了它们对金黄色葡萄球菌(Staphylococcus aureus,S.aureus)和大肠杆菌(Escherichia coli,E.coli)杀菌性能的差异.通过平板计数法和浊度法;确定了它们对两种菌的最小杀菌浓度(minimum bactericidal concentrations,MBC)和最小抑菌浓度(minimum inhibitory concentrations,MIC).棒状纳米金、三角形纳米金、球形纳米金对大肠杆菌的MIC分别为0.65 μg/mL、3.71 μg/mL、21.21μg/mL,MBC分别为1.30 μg/mL、11.09 μg/mL、21.21 μg/mL;对金黄色葡萄球菌的MIC分别为0.26μg/mL、0.56 μg/mL、2.65 μg/mL,MBC分别为0.52 μg/mL、1.11 μg/mL、2.65 μg/mL.结果表明无论是对大肠杆菌还是金黄色葡萄球菌,棒状纳米金的杀菌效果均高于其它两种形态,并且三种不同形态的纳米金对金黄色葡萄球菌的杀菌效果均优于大肠杆菌.【期刊名称】《聊城大学学报(自然科学版)》【年(卷),期】2019(032)005【总页数】7页(P64-70)【关键词】纳米金;不同形态;金黄色葡萄球菌;大肠杆菌;抗菌性能【作者】马亚云;刘梅;李娇;李宣仪;杨宗琪【作者单位】陕西师范大学食品工程与营养科学学院,陕西西安710119;陕西师范大学食品工程与营养科学学院,陕西西安710119;陕西师范大学食品工程与营养科学学院,陕西西安710119;陕西师范大学食品工程与营养科学学院,陕西西安710119;陕西师范大学食品工程与营养科学学院,陕西西安710119【正文语种】中文【中图分类】TS207.30 引言食品安全问题已成为当今世界极为关注的公共卫生热点问题,而食源性致病菌是引起食源性疾病的主要原因之一,抗生素的出现在控制这类疾病上发挥了很大作用.然而抗生素的滥用,使得细菌逐渐对传统抗生素产生了耐药性,抗生素耐药性病原体的出现严重危及了人类健康.因此,研究新型、安全、高效的抗菌材料迫在眉睫[1].近年来随着纳米技术的发展,纳米材料的抗菌性研究成为当前研究的一大热点.已报道过的抗菌纳米材料[2]包括纳米化的传统抗菌材料(如纳米化抗生素、纳米化抗菌肽等)、无机金属和金属氧化物纳米颗粒(如金、银、铜、氧化锌等)以及新型表面改性的纳米颗粒.纳米颗粒作为一种新型抗菌药物,被认为具有不同于常规药物的作用机理,与传统抗生素相比,很难诱导细菌产生耐药性,因此成为新型药物研发方向之一,引起了研究人员极大的兴趣[3,4].作为纳米颗粒中极具代表性的金纳米粒子(gold nanoparticles,AuNPs)其应用极其广泛.纳米金以其良好的稳定性、尺寸效应、表面效应、光学效应以及独特的生物亲和性,在催化[5]、生物医学[6]等领域具有广泛的应用.在生物医学领域,“AuNPs”已经成为了一种备受青睐的纳米材料,例如将其广泛应用于生物传感方向[7-9],药物输送载体[10,11],不同抗菌药物对纳米金表面进行修饰还可以将其作为一种新型的抗菌药物[3].但是,与得到广泛关注的银纳米粒子的抗菌性能研究相比,对纳米金自身抗菌性能的研究相对较少.2015年Z.Vivian Feng课题组[12]通过在纳米金表面包被不同试剂以获得带不同电荷的纳米金,从而进行抗菌效果的比较,发现负电荷的纳米金基本不具备杀菌效果,而正电荷的纳米金具备杀菌效果,且随着电荷密度的增加,杀菌效果不断增强.2017年,Jelle Penders课题组[13]分别研究了带负电的金纳米微球,金纳米花,金纳米星对金黄色葡萄球菌生长的滞后时间和指数增长率的影响,观察到一种明显的浓度和形状依赖效应.由于形状的变化引起了抗菌效果的显著差异,推测这是由于表面面积较高,表面突起较多,这可能会使金纳米花更容易附着在细菌上,然后使膜破裂,从而导致细胞死亡.十六烷基三甲基溴化铵(Hexadecyl trimethyl ammonium Bromide,CTAB)是合成表面带正电荷的纳米金最为常用的一种包被试剂,同时基于CTAB包被的方法已经被发现可以合成诸如棒、三角形、球形、立方体等众多形状的纳米金[14],其中棒状、球形、三角形纳米金是稳定性较好、合成方法简单、并且应用较为广泛的典型三种形态的纳米金.基于此我们选择以CTAB为包被剂,表面带正电荷的球形、棒状和三角形三种不同形貌的金纳米颗粒的抗菌活性作为研究对象.本文以CTAB为包被试剂合成了球形、棒状和三角形三种不同形貌的表面带正电荷的纳米金颗粒,并以革兰氏阳性菌中的金黄色葡萄球菌及革兰氏阴性菌中的大肠杆菌为测试菌株,通过平板计数法和浊度法,确定纳米金颗粒对两种菌的最小杀菌浓度和最小抑菌浓度,研究了三种不同形态的正电荷纳米金对测试菌株的抗菌效果,并对其抗菌作用机理进行了探讨.1 实验部分1.1 试剂与仪器氯金酸(HAuCl4)、十六烷基三甲基溴化铵(CTAB)、硝酸银(AgNO3)、硼氢化钠(NaBH4)、抗坏血酸(AA)、柠檬酸三钠(C6H9Na3O9 )、异丙醇(C3H8O)、无水乙醇(C2H5OH)、氯化钠(NaCl)、碘化钾(KI)、磷酸盐缓冲溶液(PBS)、琼脂粉、脑心浸液培养基(BHI)、肉汤培养基(LB)以上试剂均为市售分析纯,实验用水为超纯水.供试菌株金黄色葡萄球菌(CICC 10384)、大肠杆菌(K12)均购于中国工业微生物菌种保藏管理中心.LDZX-30 KBS立式压力蒸汽灭菌器,上海申安医疗器械厂;YT-CJ-2 D型超净工作台,北京亚泰科隆仪器技术有限公司;DH 4000 II电热恒温培养箱,天津市泰勒斯仪器有限公司;PB-10 Satorius Basic pH Meter,北京赛多利斯科学仪器有限公司;ZD-85 A双功能恒温气浴摇床,江苏省金坛市友联仪器研究所;HC-3018 R高速冷冻离心机,科大创新股份有限公司中海分公司;DF-101 S集热式恒温加热磁力搅拌器,巩义市予华仪器有限责任公司;UV-1800型紫外-可见光谱仪,日本日立公司;JEM-2100型透射电子显微镜,日本 Jeol 公司;Cannon 500 D数码相机,佳能有限责任公司.1.2 纳米金的制备1.2.1 球形纳米金的制备.根据文献[15],在20 mL HAuCl4(2.5×10-4 M)溶液中加入0.0015 g柠檬酸三钠搅拌使柠檬酸三钠浓度为2.5×10-4 M,再加入0.6 mL 冰水配置的NaBH4溶液(0.1 M),溶液立刻变为粉色,再继续搅拌2-5 h,作为种子液.在200 mL HAuCl4 (2.5×10-4 M)溶液中加入6 g CTAB使CTAB浓度为0.08 M,在45 ℃条件下加热直至溶液变为橘色,冷却至室温,作为生长液待用(注意:若形成结晶则慢慢地用温和的温度溶解).在9 mL生长液中加入0.05 mL现配的Vc(0.1 M),在剧烈搅拌下加入1 mL种子液,继续搅拌10 min,使其变为深红色,然后在9 ml生长液中加入0.05 mL Vc(0.1 M),在剧烈搅拌下加入1 mL 深红色溶液,继续搅拌10 min,使其变为棕红色,即可得到粒径为17±2.5 nm 的球形纳米金.后处理:在10000 r/min,30 ℃ 条件下离心15 min,除去上清液,补水至10mL,重复上述离心过程2次,最后将所得的沉积物用水稀释至原体积的一半,并在4 ℃条件下避光保存.1.2.2 棒状纳米金的制备.根据文献方法[16],在5 mL CTAB(0.2 M)溶液中,加入5 mL HAuCl4(5.0 mM)溶液,搅拌,溶液由亮黄色变为橙色,继续搅拌并加入0.6 mL NaBH4(0.01 M)溶液(现用现配),搅拌2 min,溶液变为黄棕色,最后将金纳米粒子溶液在30 ℃水浴锅中恒温加热2 h,作为种子液待用.在5 mLCTAB(0.2 M)溶液中,在搅拌的过程中加入0.1 mL AgNO3 (0.004 M)溶液,再加入5 ml HAuCl4(1 mM)溶液,继续加入70 mL AA(0.0788 M)溶液.待溶液变为无色后,加入12 μL晶种.继续搅拌15 min,溶液变为紫色.最后将金纳米粒子溶液在30 ℃水浴锅中恒温加热2 h,溶液变为深蓝色,即可得到棒状纳米金.后处理过程同球形纳米金一样.1.2.3 三角形纳米金的制备.根据文献[17],在37.6 mL水中加入0.4 mLHAuCl4(2.5×10-4 M)和1 mL柠檬酸钠(10 mM),再加入1 mL冰水配置的NaBH4 溶液(0.1 M),大力搅拌2 min溶液变为橙红色,再放置2 h,确保未反应的NaBH4 完全水解,制成种子液.100 mL的生长液中包含2.5×10-4 M HAuCl4 溶液和0.05 M CTAB,再往溶液里加入55 μL KI(0.1 M)、0.55 mL Vc(0.1 M)、0.55 mL NaOH(0.1 M)轻轻地搅拌直至溶液变为橘色,冷却至室温,作为生长液待用.在生长液中加入0.1 mL种子液,添加后,生长溶液的颜色由透明变为淡红色,然后在30 min内转为深红色(反应溶液保持在30 ℃),即得到三角形纳米金.后处理过程同球形纳米金一样.1.3 样品的表征用UV-1800 紫外-可见光谱仪(UV-Vis,日本公司,日本)记录样品的紫外光谱,迅速区分球形纳米金、棒状纳米金与三角形纳米金.用JEM-2100型透射电子显微镜(TEM,日本 Jeol 公司,日本 )观测三种不同形态纳米金的形态和粒径.1.4 抗菌性能的测试以 E.coli和S.aureus 为测试菌种,采用平板计数法及96孔板法来检测纳米金对测试菌种的最小杀菌浓度(MBC)和最小抑菌浓度(MIC).1.4.1 培养基的制备.称取37 g脑心浸出液培养基于1 L蒸馏水中,煮沸使其充分溶解后,调节pH至7.2-7.3,加入15 g琼脂粉(若配制液体培养基则不需要加入琼脂粉),煮沸溶解后,于121 ℃高压灭菌20 min.灭菌后,于无菌操作台上倾注平板备用.用同样制备方法制备肉汤培养基.1.4.2 菌悬液的制备.从80 ℃冰箱中取出菌冻存管,待菌液溶解后,采用二倍稀释法[18,19]将菌液分别稀释至10-2、10-4、10-6 倍,各取100 μL涂于平板上,在37 ℃下培养18 h.在超净台中,取出20 mL灭菌后的液体培养基转移到100 mL锥形瓶中,用10 μL移液枪吸取一个完整的菌落,将枪头打入锥形瓶中,放入摇床(37 ℃,260 r/min)孵育14 h,之后取5 mL细菌悬浮液于10 mL离心管中,离心 (6000 r/min,2 min),除去上清液后,加入5 mL生理盐水,混匀后离心(6000 r/min,2 min),重复两次,再将洗去培养基的菌体中加入5 mL生理盐水,混合均匀后待用.1.4.3 最小杀菌浓度.将稀释到不同浓度的纳米金加到PBS缓冲溶液中,再加入100 μL稀释倍数为2×104的菌液混匀,使反应体系为1000 μL.放入摇床孵育4h后,取出100 μL涂于培养平板上,于37 ℃下倒置培养18 h,观察菌落生长情况.以只有PBS缓冲溶液和菌液的混合液作为实验空白对照.平行实验3次.观察菌落生长情况,以观察到少于5个菌落的样品对应的浓度作为最低杀菌浓度MBC值.1.4.4 最小抑菌浓度.将稀释到不同浓度的纳米金加到液体培养基中,再加入100μL稀释倍数为2×104的菌液混匀,使反应体系为1000 μL.取200 μL加入到96孔板对应区域作为实验组;把实验组的纳米金溶液换成等量的生理盐水,然后取200 μL加入到96孔板对应区域作为阳性对照;把实验组的菌液和液体培养基换成等量的生理盐水,然后取200 μL加入到96孔板对应区域作为阴性对照.将96孔板放入恒温培养箱中,在37 ℃条件下培养24 h,能阻止样品形成明显浊度的纳米金浓度即为最小抑菌浓度MIC.2 结果与讨论2.1 纳米金的表征2.1.1 紫外-可见光吸收光谱分析.图1为纳米金合成之后进紫外-可吸收光谱分析所得,紫外光谱分析显示:球形纳米金吸收峰在520 nm处,棒状纳米金吸收峰在525 nm和604 nm处,三角形纳米金吸收峰在728 nm和928 nm处,三者都与参考文献中的吸收峰基本一致,证实成功合成了球形纳米金、棒状纳米金和三角形纳米金.图1 CTAB包被的球形纳米金(a)、棒状纳米金(b)、三角形纳米金(c)的紫外-可见吸收光谱图2.1.2 透射电镜分析(TEM) .通过透射电镜可以直观的观察到所合成的纳米金的三种不同形态和粒径,如图2所示.从图中可以看出,三种不同形态的纳米金制备成功,并且均相对均匀,粒子之间分散较好.所合成的球形纳米金粒径大约为17 ± 2.5 nm,所合成的棒状纳米金的长度约为52.31 ± 0.86 nm,宽约为22.49 ± 0.56 nm,长径比约为2.3,所合成的三角形纳米金为正三角形,边长为100 ± 25 nm.图2 CTAB包被的球形纳米金(a)、棒状纳米金(b)、三角形纳米金(c)的TEM图2.2 不同形态纳米金的杀菌作用研究2.2.1 球形纳米金最小杀菌浓度.从图3可知,球形纳米金对大肠杆菌的MBC,当纳米金浓度大于21.21 μg/mL时,观察到平板上的菌落数均少于5个菌落,因此MBC为21.21 μg/mL.球形纳米金对金黄色葡萄球菌的MBC,当纳米金浓度大于5.30 μg/mL时,观察到平板上的菌落数均少于5个菌落,因此球形纳米金的MBC为5.30 μg/mL.图3 不同浓度的球形纳米金下大肠杆菌(a)、金黄色葡萄球菌(b)的平板图(纳米金与菌液反应4 h,平板在37℃下培育18 h)2.2.2 棒状纳米金最小杀菌浓度.从图4可知,棒状纳米金对大肠杆菌的MBC,当纳米金浓度大于1.30 μg/mL时,观察到平板上的菌落数均少于5个菌落,因此MBC为1.30 μg/mL.棒状纳米金对金黄色葡萄球菌的MBC,当纳米金浓度大于0.52 μg/mL时,观察到平板上的菌落数均少于5个菌落,因此棒状纳米金的MBC为0.52 μg/mL.图4 不同浓度的棒状纳米金下大肠杆菌(a)、金黄色葡萄球菌(b)的平板图(纳米金与菌液反应4 h,平板在37 ℃下培育18 h)2.2.3 三角形纳米金最小杀菌浓度.从图5可知,三角形纳米金对大肠杆菌的MBC,当纳米金浓度大于11.09 μg/mL时,观察到平板上的菌落数均少于5个菌落,因此MBC为11.09 μg/mL.三角形纳米金对金黄色葡萄球菌的MBC,当纳米金浓度大于1.11 μg/mL时,观察到平板上的菌落数均少于5个菌落,因此三角形纳米金的MBC为1.11 μg/mL.图5 不同浓度的三角形纳米金下大肠杆菌(a)、金黄色葡萄球菌(b)的平板图(纳米金与菌液反应4 h,平板在37℃下培育18 h)2.3 不同形态纳米金的抑菌作用研究2.3.1 金黄色葡萄球菌.以金黄色葡萄球菌作为研究对象,将合成的不同形态纳米金进行稀释,球形纳米金稀释倍数分别为10、20、40、80、160倍,棒状纳米金稀释倍数为1000、2000、4000、8000、10000倍,三角形纳米金稀释倍数为50、100、200、400、800倍,使用稀释倍数对应的纳米金浓度分别进行最小抑菌浓度的测定,其96孔板结果如图6所示.结果显示对于金黄色葡萄球菌,球形纳米金的最小抑菌浓度为2.65 μg/mL,棒状纳米金的最小抑菌浓度为0.26 μg/mL,三角形纳米金的MIC为0.56 μg/mL.图6 CTAB包被的球形纳米金(a)、棒状纳米金(b)、三角形纳米金(c)对金黄色葡萄球菌37 ℃下培育24 h的得到的最小抑菌浓度图(图中数字为纳米金的浓度,单位为μg/mL)2.3.2 大肠杆菌.以大肠杆菌作为研究对象,将合成的不同形态纳米金进行稀释,球形纳米金稀释倍数分别为5、10、20、40、80倍,棒状纳米金稀释倍数为400、800、1000、2000、4000倍,三角形纳米金稀释倍数为30、60、120、240、800、480倍,不同形态的纳米金在不同稀释倍数对应的浓度下进行最小抑菌浓度的测定,其96孔板结果如图7所示.结果显示对于大肠杆菌,球形纳米金的最小抑菌浓度为21.21 μg/mL,棒状纳米金的最小抑菌浓度为0.65 μg/mL,三角形纳米金的MIC为3.70 μg/mL.图7 CTAB包被的球形纳米金(a)、棒状纳米金(b)、三角形纳米金(c)对大肠杆菌37℃培育24 h的得到的最小抑菌浓度图(图中数字为纳米金的浓度,单位为μg/mL)综上所述,通过平板计数法和96孔板法,确定了不同形貌纳米金颗粒对金黄色葡萄球菌及大肠杆菌的最小杀菌浓度(MBC)和最小抑菌浓度(MIC)如下表1所示,可以知道无论是对金黄色葡萄球菌还是对大肠杆菌,三种形态纳米金中棒状纳米金的杀菌效果是最好的,杀菌效果明显大于其它两种形态纳米金的杀菌效果.并且无论是何种形态的纳米金,其对金黄色葡萄球菌的杀菌效果都明显优于对大肠杆菌. 表1 不同形貌纳米金MIC和MBCMBC/μg·mL-1MIC/μg·mL-1E.coliS.aureusE.coliS.aureus球形21.215.3021.212.65棒状1.300.520.650.26三角形11.091.113.700.562.4 抗菌机理的探讨2014年,蒋兴宇课题组[20]就通过转录和蛋白质组学的方法研究了金纳米颗粒对革兰氏阴性菌大肠杆菌的杀菌机制,发现其作用方式有两种,一种是通过破坏细菌细胞膜的膜电位,来抑制ATP酶的活性,降低ATP水平,另一种是抑制核糖体亚基结合转运RNA.并且发现虽然生成ROS是大多数抗生素和抗菌纳米材料杀菌的主要原因,但金纳米颗粒的抗菌活性不会诱发任何与之相关的过程.张鹏等人[21]利用聚乙烯亚胺和牛血清蛋白修饰的金纳米球和金纳米棒来作为基因载体,发现棒状金纳米颗粒的尖端具有较大曲率,导致其电荷密度比球状金纳米颗粒大;而且棒状颗粒大多会通过尖端与细胞膜接触,导致以棒状颗粒作为基因载体时,可有较高的基因转染效率,棒状纳米金由于自身特殊的形态使其在与细菌接触方面有优势.我们的实验结果中,棒状纳米金的杀菌效果较其它两种形态纳米金更好,推测有可能是因为这种特殊的接触方式.纳米颗粒的表面电荷在其抗菌能力上起着明显的作用[22].比如,Angélique团队[23]研究不同的粒子直径和Zeta电位对二氧化钛纳米颗粒的杀菌活性的影响时发现,直径约为相同大小的二氧化钛纳米颗粒,当Zeta电位较高时表现出更强的抗菌效.这表明表面电荷的增强也是一种增强杀菌效果的途径,并且纳米粒子的表面带负电荷,会对表面带负电的细菌有一定的排斥作用[24,25].我们做了Zeta电位来验证本实验中不同形态的纳米金的杀菌效果与电荷密度的关系,棒状纳米金Zeta电位为56.8 mV,球形纳米金Zeta电位为42.1 mV,三角形纳米金Zeta电位为33.2 mV.如图8所示,三种形态的纳米金确实都带正电,且棒状纳米金较其它两种形态纳米金所带电荷密度较高,同时我们实验结果表明相较于其它两种形态,棒状纳米金的杀菌效果更好,这也进一步说明了表面带有正电荷的纳米金会随着电荷密度的增加,杀菌效果不断增强.图8 CTAB包被的球形纳米金、棒状纳米金、三角形纳米金的Zeta电位3 结论采用CTAB包被制备出不同形态(球形、棒状、三角形)的纳米金,将金黄色葡萄球菌和大肠杆菌作为受试菌株进行抗菌研究,从抗菌特性的研究结果中可以很明显的看出,无论是对金黄色葡萄球菌还是大肠杆菌,棒状纳米金的杀菌效果都要高于其它两种形态的纳米金.细菌表面带负电荷,带正电的纳米粒子被表面带负电的细菌所吸引,与细菌的细胞膜接触,破坏细菌的细胞膜从而进入细菌内部杀死细菌.而棒状纳米金从空间效应上更易于与细菌接触,这也是它之所以具有较高杀菌特性的原因.三种形态的纳米金均对金黄色葡萄球菌表现出优于大肠杆菌的杀菌效果,这可能是因为两种细菌的细胞壁不一样所导致的,这些结果为我们进一步开展工作奠定了基础.参考文献【相关文献】[1] Dizaj S M,Lotfipour F,Barzegarjalali M,et al.Antimicrobial activity of the metals and metal oxide nanoparticles [J].Materials Science & Engineering C,2014,44(44):278-284.[2] 马万顺,崔燕,赵玉云,等.纳米颗粒抗菌机理的研究进展[J].生物物理学报,2010,26(8):638-648.[3] Zhao Y,Tian Y,Cui Y,et al.Small molecule-capped gold nanoparticles as potent antibacterial agents that target Gram-negative bacteria[J].Journal of the American Chemical Society,2010,132(35):12349-12356.[4] 李艳华,陈曦萌.石墨烯基银抗菌材料的制备方法及抗菌机理研究[J].聊城大学学报(自然科学版),2014,27(3):71-74.[5] Avelino C,Hermenegildo G.Supported gold nanoparticles as catalysts for organic reactions[J].Chemical Society Reviews,2008,37:2096-2126.[6] Prabaharan M,Grailer J J,Pilla S,et al.Gold nanoparticles with a monolayer of doxorubicin-conjugated amphiphilic block copolymer for tumor-targeted drugdelivery[J].Biomaterials,2009,30(30):6065-6075.[7] Yanez-Sedeno P,Pingarron J M.Gold nanoparticle-based electrochemical biosensors[J].Electrochimica Acta,2005,382(4):884-886.[8] Lin Y W,Huang C C,Chang H T.Gold nanoparticle probes for the detection of mercury,lead and copper ions[J].Analyst,2011,136(5):863-871.[9] Guo Y,Wang Z,Qu W,et al.Colorimetric detection of mercury,lead and copper ions simultaneously using protein-functionalized gold nanoparticles[J].Biosensors & Bioelectronics,2011,26(10):4064-4069.[10] Gu H W,Ho P L,Tong E,et al.Presenting vancomycin on nanoparticles to enhance antimicrobial activities[J].Nano Letters,2003,3(9):1261-1263.[11] Tom R T,Suryanarayanan V,Reddy P G,et al.Ciprofloxacin-protected gold nanoparticles[J].Langmuir,2004,20(5):1909-1914.[12] Pal S,Tak Y K,Song J M.Does the antibacterial activity of silver nanoparticles depend on the shape of the nanoparticle? A study of the Gram-negative bacterium Escherichia coli[J].Applied & Environmental Microbiology,2007,73(6):1712-1720. [13] Penders J,Stolzoff M,Hickey D J,et al.Shape-dependent antibacterial effects of non-cytotoxic gold nanoparticles[J].International Journal of Nanomedicine,2017,12:2457-2468.[14] Yang X,Yang M,Pang B,et al.Gold nanomaterials at work inbiomedicine[J].Chemical Reviews,2015,115(19):10410-10488.[15] Jana N R,Gearheart L,Murphy C J.Seeding growth for size control of 5-40 nm diameter gold nanoparticles[J].Langmuir,2001,17(22):6782-6786.[16] Wang Y,Zhou X,Xu C,et al.Gold nanorods as visual sensing platform for chiral recognition with naked eyes[J].Scientific Reports,2018,8(1):5296-5304.[17] Guo Z,Fan X,Liu L,et al.Achieving high-purity colloidal gold nanoprisms and their application as biosensing platforms[J].Journal of Colloid & Interface Science,2010,348(1):29-36.[18] Fang M,Chen J H,Xu X L,et al.Antibacterial activities of inorganic agents on six bacteria associated with oral infections by two susceptibility tests[J].International Journal of Antimicrobial Agents,2006,27(6):513-517.[19] Kim J,Marshall M R,Wei C I.Antibacterial activity of some essential oil components against five foodborne pathogens[J].Journal of Agricultural & Food Chemistry,1995,43(11):1027-1037.[20] Cui Y,Zhao Y,Tian Y,et al.The molecular mechanism of action of bactericidal gold nanoparticles on Escherichia coli[J].Biomaterials,2012,33(7):2327-2333.[21] Zhang P,Li B,Du J,et al.Gold nanoparticles coated by polyethylenimine-g-bovine serum albumin with different morphologies for effective gene delivery[J].Journal of Controlled Release,2017,259:102-103.[22] Seil J T,Webster T J.Antimicrobial applications of nanotechnology:methods and literature[J].International Journal of Nanomedicine,2012,7(1):2767-2781.[23] Angelique S,Sylvain L,Martine M ,et al.Size-,composition- and shape-dependent toxicological impact of metal oxide nanoparticles and carbon nanotubestoward bacteria[J].Environmental Science & Technology,2009,43(21):8423-8429. [24] Silhavy T J,Kahne D,Walker S.The bacterial cell envelope[J].Cold Spring Harbor Perspectives in Biology,2010,2(5):a000414.[25] Dickson J S,Koohmaraie M.Cell surface charge characteristics and their relationshipto bacterial attachment to meat surfaces[J].Applied & Environmental Microbiology,1989,55(4):832-836.。
纳米金催化 -回复

纳米金催化-回复纳米金催化:开启纳米世界的催化新纪元在当代科技领域中,纳米技术已经成为一个热门的话题。
纳米技术的应用涵盖了诸多领域,其中之一就是纳米金催化。
纳米金催化作为一种新型催化技术,具有独特的优势和巨大的应用潜力。
本文将详细介绍纳米金催化的概念、原理、制备方法以及其在各个领域中的应用。
第一部分:纳米金催化概述首先,我们来了解一下纳米金催化的基本概念。
纳米金催化是指利用纳米金颗粒作为催化剂,在化学反应中起到加速反应速率、降低活化能和改善反应选择性的作用。
纳米金催化凭借其高比表面积、尺寸效应和特殊的电子结构等特点,被广泛地应用于有机合成、能源转换、环境保护等领域。
第二部分:纳米金催化原理纳米金催化所依据的原理是催化剂介质与反应物之间的相互作用。
在纳米金催化中,纳米金颗粒作为催化剂,与反应物发生物理吸附或化学吸附,从而降低反应物的活化能。
此外,纳米金颗粒具有较高的催化活性,并能提供良好的催化环境,使得反应可在相对低的温度和压力下进行。
第三部分:纳米金催化制备方法纳米金催化的制备方法多种多样。
常见的方法包括溶液法、沉积法、共沉淀法、化学还原法和物理气相法等。
其中,化学还原法是最常用的一种方法。
该方法利用还原剂将金盐溶液中的金离子还原成金原子或金纳米颗粒,并在合适的温度和pH条件下进行反应。
第四部分:纳米金催化在有机合成领域中的应用纳米金催化在有机合成领域中有着广泛的应用。
其主要应用于氧化、氢化、异构和多组分反应等。
例如,纳米金催化剂在氧化反应中可用于醛和醇的氧化、炔烃的氧化、醇的脱氧等。
此外,纳米金催化也可用于卤代烃的氢化反应、酮的氢化反应等。
纳米金催化在有机合成领域的应用,大大提高了反应效率和产物选择性。
第五部分:纳米金催化在能源转换领域中的应用能源转换是纳米金催化领域的另一个重要应用方向。
纳米金催化材料在能源转换中具有重要作用,例如在燃料电池中的氧还原反应中可作为催化剂,显著提高电池的效率和稳定性。
不同方法制备的纳米金催化剂Au_Co3O4对CO的催化氧化_
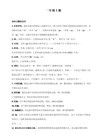
表4 空速对催化活性的影响 空速/ml/g·h 反00 11250 0 81.1 12857 0 74.2 15000 0 67.8 18000 0 58.6 *催化剂:1wt%Au/Co3O4;焙烧温度:300?C 2.5 制备方法对催化剂活性的影响
1.2 反应装置流程
采用小型固定床连续流动反应装置,反应管为硬质玻璃管,内径为?3.5mm,放置于加热炉或冷阱内以便控制反应温度。由反应管出来的气体经流量计计量后放空,在反应管后有取样点。
1.3 催化剂活性的评价方法
称取制备好的催化剂0.2g(粒径为0.45~0.90mm)装入反应管中,调节原料气(1%CO,10%O2,89%N2)流速以维持一定的气体流量(25~30mL/min),所进行的反应为CO氧化反应,反应温度由高逐渐降低,一直降低到可使CO完全转化,又能稳定15min,此时的反应温度称为最低全转化温度,以T1/1表示,此温度越低,表明催化剂的活性越好。使用GC-1102型气相色谱仪热导池检测器分析反应混合气中CO的含量,CO最少可检测体积浓度为5.0×10-5。
2.3 活性组分前体对催化剂活性的影响
采用金属有机络合物固载法,分别以HAuCl4及有机Au的络合物Au(PPh3)Cl、Au(PPh3)CH3、Au(PPh3)C2H5、Au(PPh3)Ph(Ph:苯基)代替Au(PPh3)NO3作为前体(其余条件均不变)制备Au催化剂,其活性评价结果见表3。可见,活性组分前体对催化活性有很大影响,影响顺序为:Au(PPh3)NO3>Au(PPh3)CH3≈Au(PPh3)C2H5≈Au(PPh3)Ph>HAuCl4≈Au(PPh3)Cl。这可能是因为(1)Au(PPh3)CH3、Au(PPh3)C2H5、Au(PPh3)Ph均为极性较低的有机物,难以在丙酮中溶解并高度分散在Co(OH)2表面上,因而制备出的Au催化剂活性不高。(2)HAuCl4、Au(PPh3)Cl含Cl离子,可使催化剂中毒而无活性。可见,在上述所试验的化合物中,Au(PPh3)NO3是最适合于固载法制备Au催化剂的活性组分前体。
纳米金催化 -回复
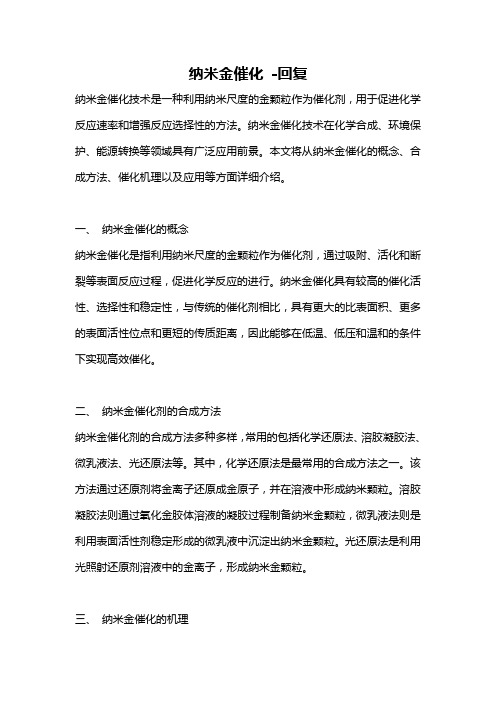
纳米金催化-回复纳米金催化技术是一种利用纳米尺度的金颗粒作为催化剂,用于促进化学反应速率和增强反应选择性的方法。
纳米金催化技术在化学合成、环境保护、能源转换等领域具有广泛应用前景。
本文将从纳米金催化的概念、合成方法、催化机理以及应用等方面详细介绍。
一、纳米金催化的概念纳米金催化是指利用纳米尺度的金颗粒作为催化剂,通过吸附、活化和断裂等表面反应过程,促进化学反应的进行。
纳米金催化具有较高的催化活性、选择性和稳定性,与传统的催化剂相比,具有更大的比表面积、更多的表面活性位点和更短的传质距离,因此能够在低温、低压和温和的条件下实现高效催化。
二、纳米金催化剂的合成方法纳米金催化剂的合成方法多种多样,常用的包括化学还原法、溶胶凝胶法、微乳液法、光还原法等。
其中,化学还原法是最常用的合成方法之一。
该方法通过还原剂将金离子还原成金原子,并在溶液中形成纳米颗粒。
溶胶凝胶法则通过氧化金胶体溶液的凝胶过程制备纳米金颗粒,微乳液法则是利用表面活性剂稳定形成的微乳液中沉淀出纳米金颗粒。
光还原法是利用光照射还原剂溶液中的金离子,形成纳米金颗粒。
三、纳米金催化的机理纳米金催化的机理主要包括吸附、活化和断裂三个过程。
首先,在纳米金颗粒表面,反应物分子通过物理吸附或化学吸附与金颗粒发生相互作用。
吸附过程可以通过吸附能力、吸附位点密度和吸附活性等因素来影响催化反应的进行。
然后,吸附的反应物分子在金颗粒表面发生活化,通过吸附位点上催化剂与反应物分子之间的化学键形成和断裂,促进反应物的转化。
最后,活化后的反应物分子脱附离开金颗粒表面,形成生成物。
四、纳米金催化的应用纳米金催化技术在化学合成、环境保护、能源转换等领域具有广泛的应用前景。
在化学合成方面,纳米金催化已被用于各类有机反应,如有机合成、偶联反应、氧化反应等。
纳米金催化对于复杂有机分子的合成具有较高的选择性和效率。
在环境保护方面,纳米金催化技术可应用于有机污染物降解和废水处理等领域,通过催化氧化反应,将有毒有害物质转化为无害的物质。
纳米金催化剂及其应用
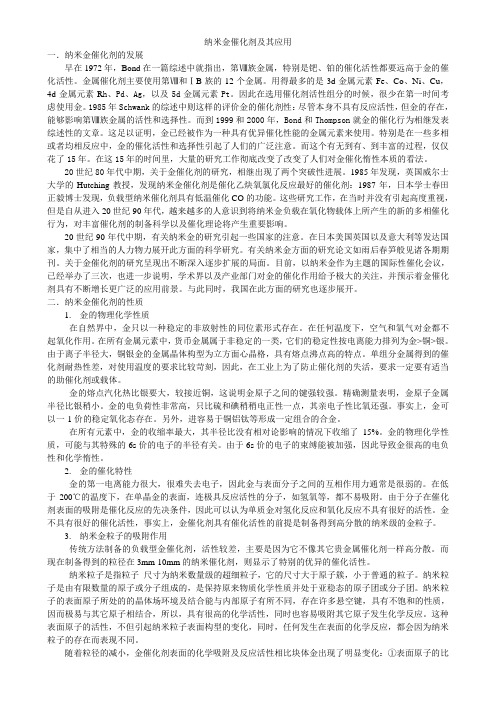
纳米金催化剂及其应用一.纳米金催化剂的发展早在1972年,Bond在一篇综述中就指出,第Ⅷ族金属,特别是钯、铂的催化活性都要远高于金的催化活性。
金属催化剂主要使用第Ⅷ和ⅠB族的12个金属。
用得最多的是3d金属元素Fe、Co、Ni、Cu,4d金属元素R h、Pd、Ag,以及5d金属元素Pt。
因此在选用催化剂活性组分的时候,很少在第一时间考虑使用金。
1985年Schwank的综述中则这样的评价金的催化剂性:尽管本身不具有反应活性,但金的存在,能够影响第Ⅷ族金属的活性和选择性。
而到1999和2000年,Bond和Thompson就金的催化行为相继发表综述性的文章。
这足以证明,金已经被作为一种具有优异催化性能的金属元素来使用。
特别是在一些多相或者均相反应中,金的催化活性和选择性引起了人们的广泛注意。
而这个有无到有、到丰富的过程,仅仅花了15年。
在这15年的时间里,大量的研究工作彻底改变了改变了人们对金催化惰性本质的看法。
20世纪80年代中期,关于金催化剂的研究,相继出现了两个突破性进展。
1985年发现,英国威尔士大学的Hutching教授,发现纳米金催化剂是催化乙炔氧氯化反应最好的催化剂:1987年,日本学士春田正毅博士发现,负载型纳米催化剂具有低温催化CO的功能。
这些研究工作,在当时并没有引起高度重视,但是自从进入20世纪90年代,越来越多的人意识到将纳米金负载在氧化物载体上所产生的新的多相催化行为,对丰富催化剂的制备科学以及催化理论将产生重要影响。
20世纪90年代中期,有关纳米金的研究引起一些国家的注意。
在日本美国英国以及意大利等发达国家,集中了相当的人力物力展开此方面的科学研究。
有关纳米金方面的研究论文如雨后春笋般见诸各期期刊。
关于金催化剂的研究呈现出不断深入逐步扩展的局面。
目前,以纳米金作为主题的国际性催化会议,已经举办了三次,也进一步说明,学术界以及产业部门对金的催化作用给予极大的关注,并预示着金催化剂具有不断增长更广泛的应用前景。
铂钯双金属纳米催化剂的催化活性
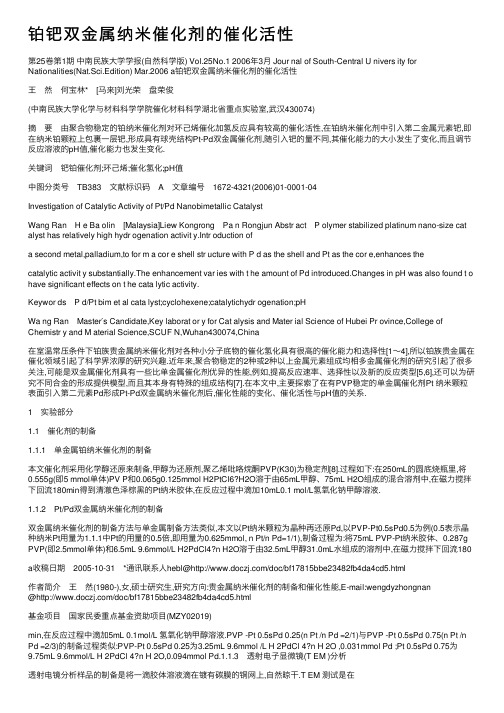
铂钯双⾦属纳⽶催化剂的催化活性第25卷第1期中南民族⼤学学报(⾃然科学版) Vol.25No.1 2006年3⽉ Jour nal of South-Central U nivers ity for Nationalities(Nat.Sci.Edition) Mar.2006 a铂钯双⾦属纳⽶催化剂的催化活性王 然 何宝林* [马来]刘光荣 盘荣俊(中南民族⼤学化学与材料科学学院催化材料科学湖北省重点实验室,武汉430074)摘 要 由聚合物稳定的铂纳⽶催化剂对环⼰烯催化加氢反应具有较⾼的催化活性,在铂纳⽶催化剂中引⼊第⼆⾦属元素钯,即在纳⽶铂颗粒上包裹⼀层钯,形成具有球壳结构Pt-Pd双⾦属催化剂,随引⼊钯的量不同,其催化能⼒的⼤⼩发⽣了变化,⽽且调节反应溶液的pH值,催化能⼒也发⽣变化.关键词 钯铂催化剂;环⼰烯;催化氢化;pH值中图分类号 TB383 ⽂献标识码 A ⽂章编号 1672-4321(2006)01-0001-04Investigation of Catalytic Activity of Pt/Pd Nanobimetallic CatalystWang Ran H e Ba olin [Malaysia]Liew Kongrong Pa n Rongjun Abstr act P olymer stabilized platinum nano-size cat alyst has relatively high hydr ogenation activit y.Intr oduction ofa second metal,palladium,to for m a cor e shell str ucture with P d as the shell and Pt as the cor e,enhances thecatalytic activit y substantially.The enhancement var ies with t he amount of Pd introduced.Changes in pH was also found t o have significant effects on t he cata lytic activity.Keywor ds P d/Pt bim et al cata lyst;cyclohexene;catalytichydr ogenation;pHWa ng Ran Master′s Candidate,Key laborat or y for Cat alysis and Mater ial Science of Hubei Pr ovince,College of Chemistr y and M aterial Science,SCUF N,Wuhan430074,China在室温常压条件下铂族贵⾦属纳⽶催化剂对各种⼩分⼦底物的催化氢化具有很⾼的催化能⼒和选择性[1~4],所以铂族贵⾦属在催化领域引起了科学界浓厚的研究兴趣.近年来,聚合物稳定的2种或2种以上⾦属元素组成均相多⾦属催化剂的研究引起了很多关注,可能是双⾦属催化剂具有⼀些⽐单⾦属催化剂优异的性能,例如,提⾼反应速率、选择性以及新的反应类型[5,6],还可以为研究不同合⾦的形成提供模型,⽽且其本⾝有特殊的组成结构[7].在本⽂中,主要探索了在有PVP稳定的单⾦属催化剂Pt 纳⽶颗粒表⾯引⼊第⼆元素Pd形成Pt-Pd双⾦属纳⽶催化剂后,催化性能的变化、催化活性与pH值的关系.1 实验部分1.1 催化剂的制备1.1.1 单⾦属铂纳⽶催化剂的制备本⽂催化剂采⽤化学醇还原来制备,甲醇为还原剂,聚⼄烯吡咯烷酮PVP(K30)为稳定剂[8].过程如下:在250mL的圆底烧瓶⾥,将0.555g(即5 mmol单体)PV P和0.065g0.125mmol H2PtCl6?H2O溶于由65mL甲醇、75mL H2O组成的混合溶剂中,在磁⼒搅拌下回流180min得到清澈⾊泽棕⿊的Pt纳⽶胶体,在反应过程中滴加10mL0.1 mol/L氢氧化钠甲醇溶液.1.1.2 Pt/Pd双⾦属纳⽶催化剂的制备双⾦属纳⽶催化剂的制备⽅法与单⾦属制备⽅法类似,本⽂以Pt纳⽶颗粒为晶种再还原Pd,以PVP-Pt0.5sPd0.5为例(0.5表⽰晶种纳⽶Pt⽤量为1.1.1中Pt的⽤量的0.5倍,即⽤量为0.625mmol, n Pt/n Pd=1/1),制备过程为:将75mL PVP-Pt纳⽶胶体、0.287g PVP(即2.5mmol单体)和6.5mL 9.6mmol/L H2PdCl4?n H2O溶于由32.5mL甲醇31.0mL⽔组成的溶剂中,在磁⼒搅拌下回流180 a收稿⽇期 2005-10-31 *通讯联系⼈hebl@/doc/bf17815bbe23482fb4da4cd5.html作者简介 王 然(1980-),⼥,硕⼠研究⽣,研究⽅向:贵⾦属纳⽶催化剂的制备和催化性能,E-mail:wengdyzhongnan@/doc/bf17815bbe23482fb4da4cd5.html基⾦项⽬ 国家民委重点基⾦资助项⽬(MZY02019)min,在反应过程中滴加5mL 0.1mol/L 氢氧化钠甲醇溶液.PVP -Pt 0.5sPd 0.25(n Pt /n Pd =2/1)与PVP -Pt 0.5sPd 0.75(n Pt /n Pd =2/3)的制备过程类似:PVP-Pt 0.5sPd 0.25为3.25mL 9.6mmol /L H 2PdCl 4?n H 2O ,0.031mmol Pd ;Pt 0.5sPd 0.75为9.75mL 9.6mmol/L H 2PdCl 4?n H 2O,0.094mmol Pd.1.1.3 透射电⼦显微镜(T EM )分析透射电镜分析样品的制备是将⼀滴胶体溶液滴在镀有碳膜的铜⽹上,⾃然晾⼲.T EM 测试是在FEI Tacnac G 220S -Twin 透射电镜仪上进⾏,操作电压为200kV .统计测量300个⾦属颗粒的粒径⼤⼩后计算粒⼦的平均直径,绘出粒径分布图.1.2 环⼰烯的提纯因环⼰烯为化学纯,使⽤前必需提纯.⽅法如下:先配制0.5mol/L 的NaH SO 3溶液,将NaHSO 3溶液少量多次的加⼊环⼰烯于分液漏⽃中进⾏洗涤,分液漏⽃中的混合液即分为2层,上层乳⽩⾊浑浊,为环⼰烯;下层透明,为NaHSO 3洗涤液,去掉下层,分别洗涤3次,然后⽤蒸馏⽔洗,去掉⽔相,将所得乳⽩⾊溶液置于烧杯中,⽤⽆⽔CaCl 2进⾏⼲燥,然后在N 2的保护下进⾏蒸馏,得⾼纯度的环⼰烯.1.3 环⼰烯催化氢化在配有恒温⽔浴槽和磁⼒搅拌器的30mL 双⼝瓶内,⽤移液管准确移取4.0mL 的纳⽶催化剂溶液,再加⼊8.0mL 的甲醇,⽤纯N 2多次置换装置中的空⽓,再⽤纯H 2多次置换N 2,设定恒温⽔浴槽的温度为30℃,H 2压⼒为0.1MPa 温度为30℃,打开磁⼒搅拌器开始搅拌1h 激活催化剂,然后向反应器内加⼊2.0mL 0.5mol/L 的环已烯甲醇溶液,反应开始并记录时间和耗氢量,由量⽓管读取吸氢的体积,反应结束后取样做GC 分析(HP -5⾊谱柱:12m ×0.22mm ).使⽤稀HCl 调节溶液的pH 值,做不同pH 值条件下的催化氢化.2 结果与讨论2.1 催化剂的T EM 表征结果利⽤醇还原法制的PVP-Pt 单⾦属纳⽶催化剂及双⾦属纳⽶催化剂的TEM 表征列于图1.(a)PVP-Pt ;(b)PVP-Pt 0.5sPd 0.25;(c)PVP-Pt 0.5s Pd 0.5;(d)PVP-Pt 0.5sPd 0.75图1 催化剂透射电镜图⽚(上)和粒径分布图(下)Fig 1 T EM micrographs (left )and par ticle s ize dis trib ution h istograms (righ t )2中南民族⼤学学报(⾃然科学版)第25卷从图1中可以看到以PVP-Pt 为晶种制备的双⾦属纳⽶催化剂,随钯含量的增加,粒径在增⼤,粒径分布也稍有扩⼤.粒径的增⼤,⽽其粒径仍为单分布,显⽰Pd 原⼦已聚累在Pt 核上,形成较⼤的颗粒.虽然颗粒增⼤的幅度不符合积累Pd 的量,很可能是因为测量颗粒⼤⼩时误差所致.2.2 环⼰烯催化氢化结果⾦属纳⽶铂与钯均为⾼效加氢催化剂.在30℃、0.1MPa 下,使⽤PVP 稳定的纳⽶铂单⾦属、纳⽶铂钯双⾦属催化氢化环⼰烯,其过程可以其吸氢量衡量.图2显⽰以不同的纳⽶⾦属为催化剂的吸氢量与时间的关系.可见在反应前期吸氢量与反应时间成直线关系,是零级反应,反应常数可求⾃直线的斜率.实验结果列于表1中.由表1中看到单⾦属纳⽶催化剂PVP-Pt 对环⼰烯的催化氢化具有较低活性,在未调节pH 值时,即pH =6.5,反应速率低只有3mL H 2(min -1m -2),反应时间长达77min,使⽤稀HCl 调节反应体系pH 值,pH =5.5、4.5、3.5,则催化速率提⾼了4倍,分别为11、10、12,反应时间也缩短,分别为20、28、15min,转化率均为100%,在pH=3.5时催化速率最⾼.使⽤双⾦属纳⽶为催化剂在调节溶液酸度pH 值低时,催化活性有不同程度的提⾼,如使⽤PVP -Pt 0.5sPd 0.25,反应速率⽐在pH=6.5时提⾼了2~3倍.纵向⽐较,在pH=6.5,即未调节反应体系酸碱性,双⾦属纳⽶催化剂的催化活性要⽐单⾦属纳⽶催化剂PVP-Pt ⾼,催化速率快,其中PVP-Pt 0.5sPd 0.5反应速率最快为14mL H 2min -1m -2,其次为PVP-Pt 0.5sPd 0.75、PVP-Pt 0.5sPd 0.25.Pd 的催化活性原⽐Pt ⾼[4],因此加⼊Pd 后活性提⾼在预想之中.可是在PVP-Pt 0.5sPd 0.75体系中,如果我们的制备催化剂确如所期望的核壳结构,Pt 0.5sPd 0.75的催化活性应⽐其他的⾼,可见Pt 虽然被Pd 包裹,它仍然影响Pd 的活性.(a )Pt ;(b )Pt 0.5s Pd 0.25;(c )Pt 0.5sPd 0.5;(d )Pt 0.5s Pd 0.75图2 环⼰烯催化加氢曲线环⼰烯1.0×10-3m ol ,催化剂3.3×10-3m mol ,压⼒0.1M Pa .温度303K Fig 2 Hydrogenation of cyclohex ene with different catalysts with cyclohex ene1.0×10-3mol and catalys t,3.3×10-3mm ol at 0.1MPa and 303K3第1期王 然,等:铂钯双⾦属纳⽶催化剂的催化活性表1 单⾦属催化剂、双⾦属催化剂催化氢化环⼰烯1) T ab1 Hyd rog enation of cyclohexene by PVP-Pt andPVP-Pt0.5sPd y催化剂pH 时间2)t/minH2平均速度/(m L?min-1?m-2)转化率3)/%PVP-Pt 6.5773100 5.52011100 4.52810100 3.51512100PVP-Pt0.5sPd0.256.548994 5.5112592 4.51225100 3.5931100PVP-Pt0.5sPd0.56.5181491 5.582397 4.51121100 3.51220100PVP-Pt0.5sPd0.756.53010100 5.592199 4.51817100 3.51516100*1)Pd与Pt含量的相对⽐值,y分别为0.25、0.5、0.75;2)反应结束时的耗氢量;3)由⽓相⾊谱所得数据分析得到的反应的转化率⼤⼩.综上所述,在单⾦属纳⽶催化剂PVP-Pt中引⼊Pd元素,形成双⾦属催化剂可有效的提⾼催化剂的催化活性,⽽且催化剂的催化活性与溶液的pH 值有关,在不同的pH条件下催化剂表现出不同的催化活性.参 考 ⽂ 献[1] Yu W,Wang Y,Liu H,et al.P repar ation and charac-ter ization of polymer-pr ot ect ed P t/Co bimetallic col-loids and t heir cat alytic proper ties in the selective hy-drogenation of cinnamaldehyde[J].Mol Cata l A:Chem,1996,112:105.[2] Liu M,Yu W,Liu H,et a l.Pr epar ation and char ac-teriza tion of polymer-stabilized ruthenium-platinum and ruthenium-palladium Bimetallic colloids and theircatalytic proper ties for hydrogenation of o-chloroni-trobenzene[J].Colloid Interfa ce Sci,1999,214:231-237.[3] Choo H P,Liew K Y,Liu H F,et al.Activity and se-lect ivity of noble metal colloids for the hydrogenation of polyunsat ur ated soybean oil[J].Mol Cata A:Chem,2003,191:113-121.[4] Choo H P,Liew K Y,Liu H F,et al.Hydr ogena-tion of palm olein catalyzed by polymer stabilized P t colloids[J].M ol Catal A:Chem,2001,165:127-134.[5] M isumi Y,Ishii Y,Hidai M.Homogeneous multi-metallic catalysts:part10for mylation of ar yland alkenyl iodides by palladium-r uthenium bimet alliccatalysts[J].M ol Catal,1993,78:1.[6] Tosima N,Har ada M,Yonezawa T,et a l.St ructuralanalysis of polymer-protected palladium/platinum bimetallic cluster s as dispersed catalysts by using ex-tended x-ra y absor pt ion fine st ructure spectroscopy [J].Phys Chem,1991,95:7448.[7] Br onstein L M,Cher nyshov D M,Volkov I O,etal.Structur e and pr oper ties of bimetallic colloidsfor med in polyst yr ene-block-Poly-4-vinylpyridine mi-celles:ca talytic behavior in selective hydr ogenation ofdehydr olinalool[J].Cata,2000,196:302-314.[8] Liu M.I nstitute of chemistr y(PhD Thesis)[D].Bei-jing:Chinese Academy of Science,1999.4 中南民族⼤学学报(⾃然科学版)第25卷。
纳米金催化剂研究进展
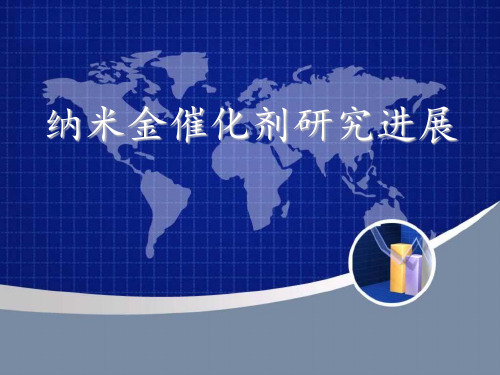
研究现状
纳米多孔金催化剂:(甲醇氧化偶联反应制甲酸甲酯)
合成:
熔化
Ag 70 at% Au 30 at% 合金母体
140h 875°C
Байду номын сангаас
4 h 800 °C
铸锭 退火,氩 退火
结晶 自然腐蚀 HNO3 65wt%
48 h后,Ag原子含量低于1%
合金样品
电位腐蚀 HNO3 5mol/L 电压:60mV
直径:5.0mm 厚度:0.2mm
水浴控制反应温度
研究现状
结果分析:
CH3OH
研究现状
结果分析:(转化率、选择性与温度关系)
一、温度影响 由于完全氧化成 CO2需要的能量高, 升温有利于此反应 进行。 二、O2量影响 氧气含量升高,选 择性降低
低于1 at% Ag nano-Au
Ag含量的影响 猜想:1、Ag的加 入减弱Au的贵金 属性质;2、O2从 Ag溢流到Au。 (10%Ag 80°C 时全部转化为CO2)
32 h后,Ag原子含量10% 48 h后,Ag原子含量2.5%
A. Wittstock, V. Zielasek, J. Biener, C. M. Friend, and M. Bäumer Science 15 January 2010: 319-322.
研究现状
电位腐蚀:
60mV
研究现状
2.5 at% Ag nano-Au
研究现状
Au/CuO/SBA-15:(高H2气氛下氧化CO)
合成:
1.3g P123 10mL DIH2O
40°C 15min 加入 6.7ml 37%HCl 31ml H2O 8h 60 °C 17h 40°C
- 1、下载文档前请自行甄别文档内容的完整性,平台不提供额外的编辑、内容补充、找答案等附加服务。
- 2、"仅部分预览"的文档,不可在线预览部分如存在完整性等问题,可反馈申请退款(可完整预览的文档不适用该条件!)。
- 3、如文档侵犯您的权益,请联系客服反馈,我们会尽快为您处理(人工客服工作时间:9:00-18:30)。
DOI:10.1002/cphc.201100906Comparison of the Peroxidase-Like Activity of Unmodified, Amino-Modified,and Citrate-Capped Gold Nanoparticles Sheng Wang,Wei Chen,*Ai-Lin Liu,Lei Hong,Hao-Hua Deng,and Xin-Hua Lin*[a]1.IntroductionRecently,increasing attention has been paid to nanoscale per-oxidase mimetics and their potential applications,since the discovery that iron oxide magnetic nanoparticles(NPs)have in-trinsic enzyme-mimetic activity similar to that of natural peroxi-dases.[1]FeS nanosheets,[2]graphene oxide,[3]bimetallic alloy nanoparticles,[4]V2O5nanowires,[5]single-walled carbon nano-tubes,[6]cubic Pt nanocrystals,[7]Au@Pt nanostructures,[8]and cupric oxide nanoparticles[9]have been shown to catalyze the oxidation of peroxidase substrates by hydrogen peroxide.This attractive property has made these enzyme nanomimetics can-didates for applications in bioanalysis[10–18]and environmental chemistry.[19–23]Gold,which is one of the most ancient subjects of investiga-tion in science,is now attracting renewed attention as an in-dispensable part of nanoscience and nanotechnology because of its appealing features.The unique electronic and optical properties of gold nanoparticles have made them model sys-tems for exploring a wide range of phenomena such as self-as-sembly,[24]near-field plasmon coupling,[25]crystal growth,[26] and electron-transfer theories.[27]In addition,gold nanoparti-cles offer good biocompatibility,facile synthesis,and conjuga-tion to a variety of biomolecular ligands,antibodies,and other targeting moieties,making them suitable for use in biosens-ing,[28,29]biological imaging,[30]medical diagnostics,[31,32]and therapeutic applications.[33]Although Au0is traditionally considered to be catalytically inert,catalytic activity in the form of nanoparticles on metal oxide supports has been clearly established recently.[34]Many attempts have been made to utilize gold nanoparticles sup-ported on various matrices as catalysts in reactions such as low-temperature CO oxidation,[35]selective oxidation of ole-fins,[36,37]and oxidative dehydrogenation of alcohols.[38]Howev-er,there are few reports on the catalytic activity and related applications of unsupported gold nanoparticles.The most suc-cessful application of unsupported gold nanoparticles as cata-lysts in the liquid phase may be a series of chemiluminescence reactions.[39–41]Recently,Jv et al.made the surprising discovery that cysteamine-modified positively charged gold nanoparti-cles and citrate-capped negatively charged gold nanoparticles have different peroxidase-like activities.[42]They considered the surface charge properties of gold nanoparticles to be the main factor which affects their catalytic activity.It is well known that, in heterogeneous catalysis,chemical bonds of the reacting molecules adsorbed on the catalytically active solid surface are broken and formed,and eventually the products are released back into the liquid or gas phase.Therefore,occupation of the gold surface by cysteamine or citrate should influence the cat-alytic activity of gold nanoparticles.To elucidate the origin of the peroxidase-like activity,unmodified gold nanoparticles were fabricated and investigated in this pared to cysteamine-or citrate-modified gold nanoparticles,unmodified gold nanoparticles show significantly high catalytic activity toward peroxidase substrates.Furthermore,some influencing factors,such as fabrication process,redox property of the modifier,and charge property of the substrate,were studied.2.Results and DiscussionAs shown in Figure1,HAuCl4can directly oxidize3,3’,5,5’-tetra-methylbenzidine(TMB)to form a blue product,which is the same as that produced in the catalytic oxidation of TMB byhy-[a]S.Wang,Prof.Dr.W.Chen,Prof.Dr.A.-L.Liu,L.Hong,H.-H.Deng,Prof.X.-H.LinDepartment of Pharmaceutical AnalysisFujian Medical UniversityFuzhou350004(China)E-mail:chenandhu@xhl1963@Supporting information for this article is available on the WWW under /10.1002/cphc.201100906.drogen peroxide in the presence of peroxidase or gold nano-particles.Therefore,the fabrication processes of gold nanopar-ticles should be checked carefully to avoid the influence of re-maining HAuCl 4in investigations on peroxidase-like activity.The unmodified and citrate-capped gold nanoparticles were formed rapidly in the presence of excess reductant,either sodium borohydride or trisodium citrate.[43]No direct oxidation effect of HAuCl 4can be observed after 1h in these two reac-tion systems,that is,depletion of HAuCl 4in the reaction solu-tions is rapid.Gold nanoparticles formed by the reduction of HAuCl 4by sodium borohydride and trisodium citrate exhibited a red color with absorption at 516and 518nm,respectively (Figure 2).This feature is commonly known as the surface plas-mon resonance (SPR)peak and is dependent on particle size.In the fabrication of amino-modified gold nanoparticles,a small amount of sodium borohydride was used to initiate the reaction.[44]The formation of gold nanoparticles can be di-vided into two stages.In the first stage,the solution was opaque and brownish soon after the addition of NaBH 4.Ac-cording to the reaction stoichiometry,a large amount of HAuCl 4should remain after depletion of sodium borohydride (over 10min).In the second stage,further consumption of HAuCl 4could be observed in the presence of 2-aminoethane-thiol at a relatively slow rate.The brownish solution slowly changed to clear wine red in this period.As shown in Figure 3,an absorption peak was observed at 530nm within the first hour.This peak shifted slightly to a shorter wavelength of 523nm and intensified with time until a maximum was ach-ieved after 6h.At the same time,the concentration of HAuCl 4in the reaction system decreased.As shown in Figure 4,the direct oxidation effect of HAuCl 4can be neglected after 6h,which matches well with the result mentioned above.This fabrication process of amino-modified gold nanoparti-cles in the presence of 2-aminoethanethiol was proposed by Niidome et al.,and then applied for DNA transfection and col-orimetric determination of glucose.[42,44]However,a detailed mechanism was not reported.According to the phenomena observed in the fabrication process,2-aminoethanethiol should be not only a modifier but also a reductant in the formation of gold nanoparticles.However,gold nanoparticles can not form without initiation by NaBH 4.No SPR peak of gold nanoparticles can be observed even after 7h for a mixture of 2-aminoetha-nethiol and HAuCl 4.A reasonable explanation is that the ad-sorption of 2-aminoethanethiol on gold nanoparticles can result in formation of Au ÀS bonds and produce free hydrogen atoms,which should be reductive.[45]To verify this explanation,a small amount of unmodified gold nanoparticles was then added to the reaction solution,and formation of gold nano-particles could be initiated in this case.As shown in Figure 5,a clear SPR peak of gold nanoparticles then can be observed.The average sizes of unmodified,amino-modified,andcitrate-Figure 1.Typical absorption curves of TMB a)before and b)after oxidation by HAuCl 4.The concentrations of TMB and HAuCl 4were 1.0m m and21.2m m ,respectively.Inset:TMB solution before (left)and after (right)oxida-tion by HAuCl 4.Figure 2.UV/Vis absorption spectra of a)unmodified and b)citrate-capped goldnanoparticles.Figure 3.UV/Vis absorption spectra of amino-modified gold nanoparticles in the fabrication process:a)20min,b)1h,c)2h,d)3h,and e)6h.Figure 4.Time evolution of the direct oxidizing effect of HAuCl 4during the synthesis of amino-modified gold nanoparticles.W.Chen,X.-H.Lin et al.capped gold nanoparticles were determined by TEM imaging to be 10,30,and 13nm,respectively (Figure 6).The peroxidase-like behavior of the synthesized gold nano-particles was examined by using 3,3’,5,5’-tetramethylbenzidine (TMB)and 2,2’-azino-bis(3-ethylbenzothiazoline-6-sulfonic acid)diammonium salt (ABTS)as chromogenic substrates.ABTS and TMB were chosen because they have opposite charge charac-teristics.As a commonly used peroxidase substrate,TMB con-tains two amino groups,which likely result in stronger affinity to a negatively charged nanoparticle surface.[46]TMB can be oxidized by peroxidase in the presence of H 2O 2,and a bluecharge-transfer complex (chromogen)is quickly formed and shows a maximum absorbance at 652nm (Scheme 1A).[47]Figure 7shows that all gold nanoparticles display catalytic ac-tivity toward oxidation of TMB in the presence of hydrogen peroxide,yet the peroxidase-like activity varies with thetypeof surfacemodification.Cationic amino-modified gold nano-particles show weak catalytic activity toward TMB due to theirlow affinity toward the positively charged substrate.In con-trast,citrate-capped gold nanoparticles,which are negatively charged,tend to attract amino groups of TMB electrostatically.These anionic nanoparticles will exhibit strong affinity withTMB as reaction substrate.Experimental data are in strong sup-port of this claim.Citrate-capped gold nanoparticles exhibit higher catalytic activity than amino-modified gold nanoparti-cles.Since the related standard derivation of each detection is less than 3%,this result is exact opposite to that of Jv et al.[42]different results may be ascribed to the concentration of peroxide used in the experiments.For citrate-gold nanoparticles,the intrinsic redox property of cit-rate,which has a negative effect on the peroxidase-like activityof gold nanoparticles,is an important factor that should betaken into account.The reduction activity of citrate could be observed when HAuCl 4was added to the citrate-capped gold nanoparticles.The SPR peak of the gold nanoparticles intensi-fied and shifted to longer wavelength (Figure 8).In addition,the peroxidase-like activity of the gold nanoparticles dimin-ished in the presence of excesscitrate (Figure 9).Therefore,the fabrication process of citrate-capped gold nanoparticles should be carefully controlled to duplicate the catalytic activity of the products.Although electrostatic affinity exerted by superficial charge could enhance catalytic activity,the physical presence of a coat-ing could also shield active do-mains on the surface of particles,weakening catalytic activity.[46]This effect can be observed when comparing the unmodified Figure 5.UV/Vis absorption spectra of the solution of 2-aminoethanethiol and HAuCl 4a)0and b)7h after adding unmodified gold nanoparticles.Figure 6.TEM images of unmodified (left),amino-modified (middle),and cit-rate-capped (right)gold nanoparticles.Scheme 1.Gold nanoparticle catalyzed reaction of A)TMB and B)ABTS with hydrogen peroxide.Figure parison of the peroxidase-like activity of a)amino-modified,b)citrate-capped,and c)unmodified gold nanoparticles toward TMB as per-oxidase substrate.The concentration of TMB was 1m m and the H 2O 2con-centration was varied.Inset (left to right):images of the catalysis product in the presence of amino-modified,citrate-capped,and unmodified gold nano-particles.The concentration of TMB was 1m m and the H 2O 2concentration was 750m m .Peroxidase-Like Activity of Gold Nanoparticlesand modified particles.In support of our claim,the unmodified gold nanoparticles exhibit much higher catalytic activity than both citrate-capped and amino-modified gold nanoparticles (Figure 7).This indicates that the superficial gold atoms are a contributing factor to observed peroxidase-like activity.To further confirm this,ABTS was chosen as another peroxi-dase substrate for activity comparison.In contrast to TMB,ABTS,which has two sulfo groups per molecule,is a negatively charged chromogenic substrate.ABTS can also be catalytically oxidized by H 2O 2,and an oxidized colored product is formed with an absorbance maximum at 420nm (Scheme 1B).The catalytic activities of the three types of gold nanoparticles are compared in Figure 10.The highest catalytic activity is again observed for unmodified gold pared to the TMB system,the amino-modified gold nanoparticles show higher catalytic activity than citrate-capped gold nanoparticles in the ABTS system,that is,charge characteristics of the nano-particles and the substrate also play an important role in the catalytic reactions.When mercaptoacetic acid modified gold nanoparticles are used instead of citrate-capped gold nanoparticles,similar re-sults can be obtained (see the Supporting Information).The catalytic activity of mercaptoacetic acid modified gold nano-particles is lower than that of unmodified gold nanoparticles but higher than that of amino-modified gold nanoparticles when TMB is used as substrate.However,the catalytic activity of mercaptoacetic acid modified gold nanoparticles is lowerthan that of amino-modified gold nanoparticles when ABTS is used as substrate.3.ConclusionsUnmodified gold nanoparticles have been shown to have in-trinsic peroxidase-like pared to amino-modified or citrate-capped gold nanoparticles,unmodified gold nanoparti-cles show significantly higher catalytic activity toward both TMB and ABTS,that is,the superficial gold atoms are a contri-buting factor to the observed peroxidase-like activity.Due to the absence of surface modifiers,unmodified gold nanoparti-cles could be conveniently labeled by biological molecules such as antigens,antibodies,and oligonucleotides.The differ-ent catalytic activities of amino-modified and citrate-capped gold nanoparticles toward TMB and ABTS show that the charge characteristics of the nanoparticles and the substrate also play an important role in the catalytic reactions.The influ-ence of superficial modifiers on the number of active gold atoms and the superficial charge is worthy of further studies to control the peroxidase-like activity of gold nanoparticles for different applications.Experimental SectionChemicals and Reagents:HAuCl 4·3H 2O,30%H 2O 2,and sodium cit-rate were purchased from Sinopharm Chemical Reagent Co.Ltd.3,3’,5,5’-Tetramethylbenzidine (TMB),sodium borohydride,2,2’-azino-bis(3-ethylbenzothiazoline-6-sulfonic acid)diammonium salt (ABTS),and 2-aminoethanethiol were purchased from Aladdin Re-agent Company (Shanghai).Other reagents and chemicals were of at least analytical reagent grade.Double-distilled water was used throughout experiments.Instrumentation:The UV/Vis spectra of gold nanoparticles were re-corded on a Shimadzu UV/2450spectrophotometer (Shimadzu,Japan).The TEM images were recorded on a FEI Tecnai-12trans-mission electron microscope with an accelerating voltage of 120kV.Figure 8.UV/Vis absorption spectra of citrate-capped gold nanoparticles a)0and b)7h after adding HAuCl 4.Figure 9.Influence of citrate ions on the peroxidase-like activity of unmodi-fied gold nanoparticles.Concentration of sodium citrate:a)0,b)0.5,c)0.75,d)1.25m m.Figure parison of the peroxidase-like activity of a)citrate-capped,b)amino-modified,and c)unmodified gold nanoparticles toward ABTS as peroxidase substrate.The concentration of TMB was 6m m and the H 2O 2concentration was varied.Inset (left to right):Solutions of the catalysis prod-uct in the presence of citrate-capped,amino-modified,and unmodified gold nanoparticles.The concentration of ABTS was 6m m and the H 2O 2concentra-tion was 2m .W.Chen,X.-H.Lin et al.Preparation of Gold Nanoparticles:Unmodified gold nanoparticles were synthesized by reduction of HAuCl4with NaBH4as sole reduc-ing agent.Briefly,HAuCl4solution(500m L,1%)was diluted with water(39.5mL),and NaBH4solution(1mL,1%)was injected under vigorous stirring(the injection time was about5min).An immedi-ate color change of the solution from pale yellow to a wine red was observed,indicating formation of gold nanoparticles.The un-modified gold nanoparticles can remain stable against aggregation in aqueous solution for at least two months.When stored in a re-frigerator at48C for two months,no change in catalytic activity could be found.Amino-modified gold nanoparticles were synthesized in aqueous solution by following a previous publication with some modifica-tions.[44]Briefly,HAuCl4solution(500m L,1%)was diluted with water(39.5mL),and2-aminoethanethiol solution(85m L,213m m) was added to the gold solution.After stirring for20min at room temperature,NaBH4solution(2.1m L,10m m)was added,and the mixture was stirred for more than10h in the dark.The final solu-tion was stored at48C in refrigerator.Citrate-capped gold nanoparticles were prepared according to pre-viously published protocols.[48]Briefly,HAuCl4solution(1mL,1%) was dissolved in water(100mL)and boiled.Trisodium citrate solu-tion(3mL,1%)was quickly added to the refluxing HAuCl4solution, and the resulting color change from pale yellow to deep red indi-cated formation of gold nanoparticles.After a continuously reflux-ing for an additional15min,the solution was slowly cooled to room temperature.The final solution was stored at48C in a refrig-erator.Influence of Citrate Ions on the Catalytic Activity of Gold Nanopar-ticles:A TMB solution was used to detect the influence of citric acid ions on the catalytic activity of gold nanoparticles.Experi-ments were carried out with280m L of unmodified gold nanoparti-cles in a reaction volume of4.0mL with different amounts of citric acid sodium(0.01m,from150to600m L)in the presence of1m m TMB as substrate.H2O2concentration was750m m.The mixed so-lution was incubated in a378C water bath for10min and used for adsorption spectroscopic measurements at652nm.Comparison of Catalytic Activity of Three Types of Gold Nanoparti-cles:Experiments on the TMB–H2O2catalytic reaction were carried out with280m L of unmodified and amino-modified gold nanopar-ticles or315m L of citrate-capped gold nanoparticles in a reaction volume of4.0mL in the presence of varying concentrations of H2O2(from25m m to2m).The concentration of TMB was5m m. The mixed solution was incubated in a378C water bath for10min and used for absorption spectroscopic measurements at652nm. The catalytic activity was quantified as the absorbance normalized by the total surface area of the gold nanoparticles.Experiments on the ABTS–H2O2catalytic reaction were carried out with280m L of unmodified and amino-modified gold nanoparticles or315m L of citrate-capped gold nanoparticles in a reaction volume of4.0mL in the presence of varying concentrations of H2O2(from25m m to2m).The concentration of ABTS was6m m. The mixed solution was incubated in a378C water bath for5min and used for absorption spectroscopic measurements at420nm. The catalytic activity was quantified as the absorbance normalized by the total surface area of the gold nanoparticles.AcknowledgementsWe acknowledge the financial support of the National Natural Science Foundation of China(21175023),the Medical Innovation Project of Fujian Province(2009-CX-17),the Natural Science Foundation of Fujian Province(2011J01034),Science and Technol-ogy Planning Project of Fujian Province(2011Y0030),and the Program for New Century Excellent Talents in Fujian Province University(W.C.,JA11102).Keywords:catalytic activity·enzyme mimics·gold·heterogeneous catalysis·nanoparticles[1]L.Gao,J.Zhuang,L.Nie,J.Zhang,Y.Zhang,N.Gu,T.Wang,J.Feng,D.Yang,S.Perrett,X.Yan,Nat.Nanotechnol.2007,2,577–583.[2]Z.Dai,S.Liu,J.Bao,H.Ju,Chem.Eur.J.2009,15,4321–4326.[3]Y.Song,K.Qu,C.Zhao,J.Ren,X.Qu,Adv.Mater.2010,22,2206–2210.[4]W.He,X.Wu,J.Liu,X.Hu,K.Zhang,S.Hou,W.Zhou,S.Xie,Chem.Mater.2010,22,2988–2994.[5]R.AndrØ,F.Natµlio,M.Humanes,J.Leppin,K.Heinze,R.Wever,H.C.Schrçder,W.E.G.Müller,W.Tremel,Adv.Funct.Mater.2011,21,501–509.[6]Y.Song,X.Wang,C.Zhao,K.Qu,J.Ren,X.Qu,Chem.Eur.J.2010,16,3617–3621.[7]M.Ma,Y.Zhang,N.Gu,Colloids Surf.A2011,373,6–10.[8]W.W.He,Y.Liu,J.S.Y,J.J.Yin,X.C.Wu,X.N.Hu,K.Zhang,J.B.Liu,C.Y.Chen,Y.L.Ji,Y.T.Guo,Biomaterials2011,32,1139–1147.[9]W.Chen,J.Chen,A.L.Liu,L.M.Wang,G.W.Li,X.H.Lin,ChemCatChem2011,3,1151–1154.[10]H.Wei,E.Wang,Anal.Chem.2008,80,2250–2254.[11]X.Q.Zhang,S.W.Gong,Y.Zhang,T.Yang,C.Y.Wang,N.Gu,J.Mater.Chem.2010,20,5110–5116.[12]W.Shi,Q.Wang,Y.Long,Z.Cheng,S.Chen,H.Zheng,Y.Huang,Chem.Commun.2011,47,6695–6697.[13]F.Qu,T.Li,M.Yang,Biosens.Bioelectron.2011,26,3927–3931.[14]N.Ding,N.Yan,C.Ren,X.Chen,Anal.Chem.2010,82,5897–5899.[15]L.Gao,J.Wu,S.Lyle,K.Zehr,L.Cao,D.Gao,J.Phys.Chem.C2008,112,17357–17361.[16]R.Cui,Z.Han,J.J.Zhu,Chem.Eur.J.2011,17,9377–9384.[17]J.Li,J.D.Qiu,J.J.Xu,H.Y.Chen,X.H.Xia,Adv.Funct.Mater.2007,17,1574–1580.[18]Y.Ding,G.Gu,X.H.Xia,J.Solid State Electrochem.2008,12,553–558.[19]X.Zuo,C.Peng,Q.Huang,S.Song,L.Wang,D.Li,C.Fan,Nano Res.2009,2,617–623.[20]W.Luo,L.Zhu,N.Wang,H.Tang,M.Cao,Y.She,Environ.Sci.Technol.2010,44,1786–1791.[21]H.Niu,D.Zhang,S.Zhang,X.Zhang,Z.Meng,Y.Cai,J.Hazard.Mater.2011,190,559–565.[22]H.Wang,Y.Huang,J.Hazard.Mater.2011,191,163–169.[23]J.Zhang,J.Zhuang,L.Gao,Chemosphere2008,73,1524–1528.[24]A.N.Shipway,hav,I.Willner,Adv.Mater.2000,12,993–998.[25]Q.H.Wei,K.H.Su,S.Durant,X.Zhang,Nano Lett.2004,4,1067–1071.[26]J.Becker,O.Schubert,C.Sçnnichsen,Nano Lett.2007,7,1664–1669.[27]K.Weber,L.Hockett,S.Creager,J.Phys.Chem.B1997,101,8286–8291.[28]R.Elghanian,J.J.Storhoff,R.C.Mucic,R.L.Letsinger,C.A.Mirkin,Sci-ence1997,277,1078–1081.[29]J.M.Nam,C.S.Thaxton,C.A.Mirkin,Science2003,301,1884–1886.[30]P.K.Jain,K.S.Lee,I.H.El-Sayed,M.A.El-Sayed,J.Phys.Chem.B2006,110,7238–7248.[31]X.Qian,X.H.Peng,D.O.Ansari,Q.Yin-Goen,G.Z.Chen,D.M.Shin,L.Yang,A.N.Young,M.D.Wang,S.Nie,Nat.Biotechnol.2008,26,83–90.[32]I.H.El-Sayed,X.Huang,M.A.El-Sayed,Nano Lett.2005,5,829–834.[33]Y.Cheng,A.C.Samia,J.D.Meyers,I.Panagopoulos,B.Fei,C.Burda,J.Am.Chem.Soc.2008,130,10643–10647.[34]B.K.Min,C.M.Friend,Chem.Rev.2007,107,2709–2724.[35]otti,W.C.Li,B.Spliethoff,F.Schüth,J.Am.Chem.Soc.2006,128,917–924.Peroxidase-Like Activity of Gold Nanoparticles[36]M.Turner,V.B.Golovko,O.P.H.Vaughan,P.Abdulkin,A.Berenguer-Murcia,M.S.Tikhov,B.F.G.Johnson,mbert,Nature2008,454, 981–983.[37]M.D.Hughes,Y.J.Xu,P.Jenkins,P.McMorn,ndon,D.I.Enache,A.F.Carley,G.A.Attard,G.J.Hutchings,F.King,E.H.Stitt,P.Johnston,K.Griffin,C.J.Kiely,Nature2005,437,1132–1135.[38]S.Rousseau,O.Marie,P.Bazin,M.Daturi,S.Verdier,V.Harle,J.Am.Chem.Soc.2010,132,10832–10841.[39]Z.F.Zhang,H.Cui,i,L.J.Liu,Anal.Chem.2005,77,3324–3329.[40]A.Safavi,G.Absalan,F.Bamdad,Anal.Chim.Acta2008,610,243–248.[41]H.Cui,Z.F.Zhang,M.J.Shi,J.Phys.Chem.B2005,109,3099–3103.[42]Y.Jv,B.Li,R.Cao,mun.2010,46,8017–8019.[43]G.Frens,Nat.Phys.Sci.1973,241,20–22.[44]T.Niidome,K.Nakashima,H.Takahashi,Y.Niidome,mun.2004,1978–1979.[45]J.Z.Guo,H.Cui,J.Phys.Chem.C2007,111,12254–12259.[46]F.Yu,Y.Huang,A.J.Cole,V.C.Yang,Biomaterials2009,30,4716–4722.[47]P.D.Josephy,T.E.Eling,R.P.Mason,J.Biol.Chem.1982,257,3669–3675.[48]C.P.Jia,X.Q.Zhong,B.Hua,M.Y.Liu,F.X.Jing,X.H.Lou,S.H.Yao,J.Q.Xiang,Q.H.Jin,J.L.Zhao,Biosens.Bioelectron.2009,24,2836–2841. Received:November11,2012Revised:January19,2012Published online on March2,2012W.Chen,X.-H.Lin et al.。