能源类外文翻译
新能源汽车外文文献翻译
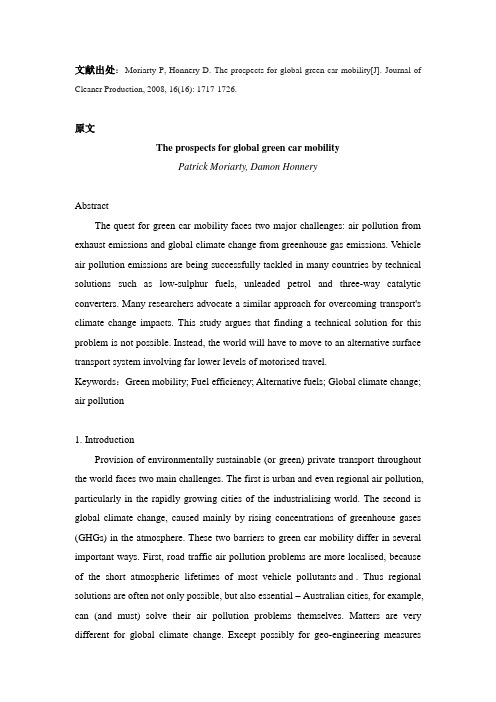
文献出处:Moriarty P, Honnery D. The prospects for global green car mobility[J]. Journal of Cleaner Production, 2008, 16(16): 1717-1726.原文The prospects for global green car mobilityPatrick Moriarty, Damon HonneryAbstractThe quest for green car mobility faces two major challenges: air pollution from exhaust emissions and global climate change from greenhouse gas emissions. Vehicle air pollution emissions are being successfully tackled in many countries by technical solutions such as low-sulphur fuels, unleaded petrol and three-way catalytic converters. Many researchers advocate a similar approach for overcoming transport's climate change impacts. This study argues that finding a technical solution for this problem is not possible. Instead, the world will have to move to an alternative surface transport system involving far lower levels of motorised travel.Keywords:Green mobility; Fuel efficiency; Alternative fuels; Global climate change; air pollution1. IntroductionProvision of environmentally sustainable (or green) private transport throughout the world faces two main challenges. The first is urban and even regional air pollution, particularly in the rapidly growing cities of the industrialising world. The second is global climate change, caused mainly by rising concentrations of greenhouse gases (GHGs) in the atmosphere. These two barriers to green car mobility differ in several important ways. First, road traffic air pollution problems are more localised, because of the short atmospheric lifetimes of most vehicle pollutants and . Thus regional solutions are often not only possible, but also essential – Australian cities, for example, can (and must) solve their air pollution problems themselves. Matters are very different for global climate change. Except possibly for geo-engineering measuressuch as placing large quantities of sulphate aerosols in the lower stratosphere or erecting huge reflecting mirrors in space, one country cannot solve this problem alone. Climate change is a global problem. Nevertheless, it is possible for some countries to ‘freeload’ if the majority of nations that are important GHG emitter。
能源专业外文翻译--来自太阳的能源
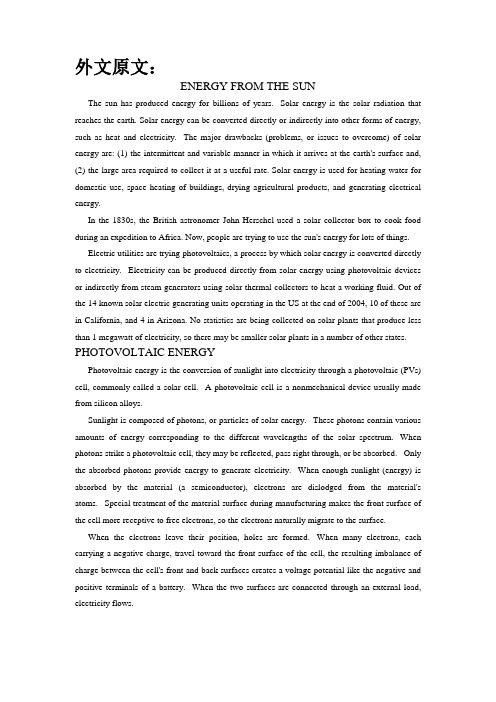
外文原文:ENERGY FROM THE SUNThe sun has produced energy for billions of years. Solar energy is the solar radiation that reaches the earth. Solar energy can be converted directly or indirectly into other forms of energy, such as heat and electricity. The major drawbacks (problems, or issues to overcome) of solar energy are: (1) the intermittent and variable manner in which it arrives at the earth's surface and, (2) the large area required to collect it at a useful rate. Solar energy is used for heating water for domestic use, space heating of buildings, drying agricultural products, and generating electrical energy.In the 1830s, the British astronomer John Herschel used a solar collector box to cook food during an expedition to Africa. Now, people are trying to use the sun's energy for lots of things.Electric utilities are trying photovoltaics, a process by which solar energy is converted directly to electricity. Electricity can be produced directly from solar energy using photovoltaic devices or indirectly from steam generators using solar thermal collectors to heat a working fluid. Out of the 14 known solar electric generating units operating in the US at the end of 2004, 10 of these are in California, and 4 in Arizona. No statistics are being collected on solar plants that produce less than 1 megawatt of electricity, so there may be smaller solar plants in a number of other states. PHOTOVOLTAIC ENERGYPhotovoltaic energy is the conversion of sunlight into electricity through a photovoltaic (PVs) cell, commonly called a solar cell. A photovoltaic cell is a nonmechanical device usually made from silicon alloys.Sunlight is composed of photons, or particles of solar energy. These photons contain various amounts of energy corresponding to the different wavelengths of the solar spectrum. When photons strike a photovoltaic cell, they may be reflected, pass right through, or be absorbed. Only the absorbed photons provide energy to generate electricity. When enough sunlight (energy) is absorbed by the material (a semiconductor), electrons are dislodged from the material's atoms. Special treatment of the material surface during manufacturing makes the front surface of the cell more receptive to free electrons, so the electrons naturally migrate to the surface.When the electrons leave their position, holes are formed. When many electrons, each carrying a negative charge, travel toward the front surface of the cell, the resulting imbalance of charge between the cell's front and back surfaces creates a voltage potential like the negative and positive terminals of a battery. When the two surfaces are connected through an external load, electricity flows.The photovoltaic cell is the basic building block of a PV system. Individual cells can vary in size from about 1 cm (1/2 inch) to about 10 cm (4 inches) across. However, one cell only produces 1 or 2 watts, which isn't enough power for most applications. To increase power output, cells are electrically connected into a packaged weather-tight module. Modules can be further connected to form an array. The term array refers to the entire generating plant, whether it is made up of one or several thousand modules. As many modules as needed can be connected to form the array size (power output) needed.The performance of a photovoltaic array is dependent upon sunlight. Climate conditions (e.g., clouds, fog) have a significant effect on the amount of solar energy received by a PV array and, in turn, its performance. Most current technology photovoltaic modules are about 10 percent efficient in converting sunlight with further research being conducted to raise this efficiency to 20 percent.The pv cell was discovered in 1954 by Bell Telephone researchers examining the sensitivity of a properly prepared silicon wafer to sunlight. Beginning in the late 1950s, pvs were used to power U.S. space satellites. The success of PVs in space generated commercial applications for pv technology. The simplest photovoltaic systems power many of the small calculators and wrist watches used everyday. More complicated systems provide electricity to pump water, power communications equipment, and even provide electricity to our homes.Photovoltaic conversion is useful for several reasons. Conversion from sunlight to electricity is direct, so that bulky mechanical generator systems are unnecessary. The modular characteristic of photovoltaic energy allows arrays to be installed quickly and in any size required or allowed.Also, the environmental impact of a photovoltaic system is minimal, requiring no water for system cooling and generating no by-products. Photovoltaic cells, like batteries, generate direct current (DC) which is generally used for small loads (electronic equipment). When DC from photovoltaic cells is used for commercial applications or sold to electric utilities using the electric grid, it must be converted to alternating current (AC) using inverters, solid state devices that convert DC power to AC. Historically, pvs have been used at remote sites to provide electricity. However, a market for distributed generation from PVs may be developing with the unbundling of transmission and distribution costs due to electric deregulation. The siting of numerous small-scale generators in electric distribution feeders could improve the economics and reliability of the distribution system.SOLAR THERMAL HEATThe major applications of solar thermal energy at present are heating swimming pools, heating water for domestic use, and space heating of buildings. For these purposes, the general practice is to use flat-plate solar-energy collectors with a fixed orientation (position).Where space heating is the main consideration, the highest efficiency with a fixed flat-plate collector is obtained if it faces approximately south and slopes at an angle to the horizon equal to the latitude plus about 15 degrees. Solar collectors fall into two general categories: nonconcentrating and concentrating.In the nonconcentrating type, the collector area (i.e. the area that intercepts the solar radiation) is the same as the absorber area (i.e., the area absorbing the radiation).In concentrating collectors, the area intercepting the solar radiation is greater, sometimes hundreds of times greater, than the absorber area. Where temperatures below about 200o F are sufficient, such as for space heating, flat-plate collectors of the nonconcentrating type are generally used.There are many flat-plate collector designs but generally all consist of (1) a flat-plate absorber, which intercepts and absorbs the solar energy, (2) a transparent cover(s) that allows solar energy to pass through but reduces heat loss from the absorber, (3) a heat-transport fluid (air or water) flowing through tubes to remove heat from the absorber, and (4) a heat insulating backing.Solar space heating systems can be classified as passive or active. In passive heating systems, the air is circulated past a solar heat surface(s) and through the building by convection (i.e. less dense warm air tends to rise while more dense cooler air moves downward) without the use of mechanical equipment. In active heating systems, fans and pumps are used to circulate the air or the heat absorbing fluid.SOLAR THERMAL POWER PLANTSSolar thermal power plants use the sun's rays to heat a fluid, from which heat transfer systems may be used to produce steam. The steam, in turn, is converted into mechanical energy in a turbine and into electricity from a conventional generator coupled to the turbine. Solar thermal power generation is essentially the same as conventional technologies except that in conventional technologies the energy source is from the stored energy in fossil fuels released by combustion. Solar thermal technologies use concentrator systems due to the high temperatures needed for the working fluid.PARABOLIC TROUGHThe parabolic trough is used in the largest solar power facility in the world located in the Mojave Desert at Kramer Junction, California. This facility has operated since the 1980 and accounted for the majority of solar electricity produced by the electric power sector in 2004.A parabolic trough collector has a linear parabolic-shaped reflector that focuses the sun's radiation on a linear receiver located at the focus of the parabola. The collector tracks the sun along one axis from east to west during the day to ensure that the sun is continuously focused on the receiver. Because of its parabolic shape, a trough can focus the sun at 30 to 100 times its normal intensity (concentration ratio) on a receiver pipe located along the focal line of the trough, achieving operating temperatures over 400 degrees Celcius.A collector field consists of a large field of single-axis tracking parabolic trough collectors. The solar field is modular in nature and is composed of many parallel rows of solar collectors aligned on a north-south horizontal axis. A working (heat transfer) fluid is heated as it circulates through the receivers and returns to a series of heat exchangers at a central location where the fluid is used to generate high-pressure superheated steam. The steam is then fed to a conventional steam turbine/generator to produce electricity. After the working fluid passes through the heat exchangers, the cooled fluid is recirculated through the solar field. The plant is usually designed to operate at full rated power using solar energy alone, given sufficient solar energy. However, all plants are hybrid solar/fossil plants that have a fossil-fired capability that can be used to supplement the solar output during periods of low solar energy. The Luz plant is a natural gas hybrid.SOLAR DISHA solar dish/engine system utilizes concentrating solar collectors that track the sun on two axes, concentrating the energy at the focal point of the dish because it is always pointed at the sun. The solar dish's concentration ratio is much higher that the solar trough, typically over 2,000, with a working fluid temperature over 750o C. The power-generating equipment used with a solar dish can be mounted at the focal point of the dish, making it well suited for remote operations or, as with the solar trough, the energy may be collected from a number of installations and converted to electricity at a central point. The engine in a solar dish/engine system converts heat to mechanical power by compressing the working fluid when it is cold, heating the compressed working fluid, and then expanding the fluid through a turbine or with a piston to produce work. The engine is coupled to an electric generator to convert the mechanical power to electric power.SOLAR POWER TOWERA solar power tower or central receiver generates electricity from sunlight by focusing concentrated solar energy on a tower-mounted heat exchanger (receiver). This system uses hundreds to thousands of flat sun-tracking mirrors called heliostats to reflect and concentrate the sun's energy onto a central receiver tower. The energy can be concentrated as much as 1,500times that of the energy coming in from the sun. Energy losses from thermal-energy transport are minimized as solar energy is being directly transferred by reflection from the heliostats to a single receiver, rather than being moved through a transfer medium to one central location, as with parabolic troughs. Power towers must be large to be economical. This is a promising technology for large-scale grid-connected power plants. Though power towers are in the early stages of development compared with parabolic trough technology, a number of test facilities have been constructed around the world.Last Revised: July 2008Sources: Energy Information Administration, Electric Power Annual,Form EIA-860, Annual Electric Generator Report database.中文译文:来自太阳的能源太阳产生能量已有数十亿年,太阳能是太阳辐射到地球的能量。
新能源经济【外文翻译】

(2011届)本科毕业设计(论文)外文翻译原文:THE NEW ENERGY ECONOMYEveryone agrees it's time, but the obstacles go well beyond matters of technology. Why real change will take nothing less than a new American revolutionTo describe what's needed to wean the country off fossil fuels, people often use the word transition. But transition is too smooth. It suggests steadiness, even inevitability, as if the endpoint is predetermined.The outcome of the tremendous push that's now underway to change how the United States and other countries obtain and consume energy is anything but predetermined. There are no definite answers to questions about the role one source of energy or another will play 15 or 20 years from now, no clear sense about the type of fuel (if any) people will put in their cars, no consensus on how quickly any of this can happen or at what cost.Nor is the change likely to be smooth and quiet. Instead, it will probably be disruptive, breaking down existing ways of thinking and acting. Not that disruption is bad: Joseph Schumpeter, the famous Austro-Hungarian economist, once spoke of "creative destruction," whereby new technologies and ideas replace old ones, which themselves are overthrown by newer, more progressive ones.Already, 2009 has been a year of visions, of prophecies. President Barack Obama's inaugural address offered one such vision: doubling alternative energy production in the next three years, updating and expanding the nation's energy infrastructure, saving billions of dollars in energy costs through improved energy efficiency. Think tanks, businesses, industry groups, and environmentalists have laid out their own plans, some more aggressive and some less so.The sheer number of these plans, not to mention the interest percolating up from nearly all corners of American life, suggests, as Energy Secretary Steven Chu puts it, that "the landscape is changing."Clean energy is, of course, a narrative that has been slowly developing in the UnitedStates over the past four decades or so, at least since President Jimmy Carter's administration. In the past, its visibility and its urgency have ebbed and flowed with the price of oil. Today, however, it's not just the wild fluctuation in oil prices that is driving the discussion. There is the economic crisis. There is the burgeoning climate crisis, with its implicit call for global cooperation. And there are fresh concerns about national security in an age of emboldened oil cartels and nuclear ambitions. Addressing each of these priorities raises its own set of questions. At the moment, there is no consensus on how aggressively the United States should reduce greenhouse gas emissions over the few next decades or, more broadly still, the proper role of fossil fuels and renewable energy.Meanw hile, almost every potential contributor to a “green energy economy"--wind and solar power, befouls, nuclear power, energy efficiency--faces hurdles well beyond the technology of each system. Regulatory policies or economic issues stand in the way of massive, quick deployment of any of these.And so, today, a new mentality is emerging among almost all the major energy players, from wind developers in the Dakotas to coal-plant operators in North Carolina: Energy issues can no longer be treated as piecemeal policy items left up to states and hodgepodge federal legislation to decide but instead must be addressed nationally, in a sweeping manner.Want the country running on flex-fuel vehicles? "It's just a few hundred dollars more per vehicles," says retired Gen. Wesley Clark, the 2004 presidential candidate and now cochairman of Growth Energy, a group representing several of the nation's largest ethanol producers. "What would encourage an automobile manufacturer to believe he should do it would be a government policy that says we are moving in that direction."Want more wind power? "The critical thing we are talking about here is national policy and the signals it sends to people," says Denise Bode, CEO of the American Wind Energy Association. "There is tremendous demand for wind power, but there is not enough transmission."The idea that a nation should have a clear-cut national energy policy sounds obvious enough. In the United States, however, the truth is that energy has not always beenconsidered a national issue, and in some ways it still isn't.Nowhere is this more obvious than with the transmission grid, a sprawling jumble of wires and mechanical connections dating back 50, 80, even 100 years in some places. Today, the grid is divided into more than 140 "balancing areas" to help manage the distribution of power. But some are so localized that they can't communicate with their next-door neighbors. As a result, extra power in one region is often wasted rather than being sent to a place that needs it.So if wind power, solar power, and plug-in electric vehicles are to be big players in the country's energy future, as many hope, this antiquated system for delivering electricity will have to change. The grid must be retooled, and new high-capacity power lines are needed to carry wind-generated electricity from the Midwest to the East and West coasts. To get those high-power lines approved, Bode and other advocates say, the federal government needs more authority to override nasty squabbles between states, environmentalists, and other interest groups that have typically stalled such efforts. The federal government, the thinking goes, already has the authority to build natural gas pipelines across state lines, and electricity should be no different. That sentiment seems to be gaining ground even among regulators who once opposed it, although there are many issues still to be worked out. As Chu says, "If we just take the view that we are going to cram something down someone's throat, this is not a constructive way of doing business" (interview, Page 32). Infrastructure is only one part of the battle to make national energy problems a national issue. Another is technology. Even though wind power technology is relatively mature--it was the country's largest provider of clean electricity last year--most other renewable sources still need work. Improvements to photovoltaic cells could reduce solar power costs significantly. New drilling technologies could help geothermal spread across a larger geographic range. Advancements in befouls, in particular to the enzymes needed to break down grasses and woods to produce ethanol, would have a major impact. Meanwhile, fossil fuels face their own technological challenges. If coal is to stay around for a while, it'll most likely be because of still-developing methods to capture carbon dioxide emissions before they enter the atmosphere.Scientific breakthroughs don't come cheap. The economic stimulus package set aside $21.5 billion for scientific research, signaling that Washington is taking a much more active role in basic energy issues after years of declining budgets at national labs. But this is just the groundwork. The most powerful force to remake the energy America uses could be government policies: climate change legislation, which would set a price on carbon dioxide emissions, and a national renewable-electricity standard, which would require the United States to get a certain portion of its electricity from renewable energy. Both rules could have far-reaching impacts, forcing industries to massively reconsider their operations, giving financial investor’s confidence to pump money into wind farms, solar fields, and other industries, and convincing the coal industry that it's worth investing billions in technology to reduce emissions.The consequences of climate change legislation, in fact, are expected to be so great that companies typically opposed to government regulation are asking Congress to go ahead and act just so that they can have some certainty about where to put their money.What Washington won't do--not in theory, anyway--is pick specific winners. "The market will decide what the mix will be," says Matt Rogers, a former director at McKinsey & Co. and now one of Chu's top advisers. "It will be interesting to see what the market brings forward." Of course, in reality, Congress's record is one of subsidizing some industries but not others. Even within the befoul world, corn-based ethanol is heavily supported, but some others, such as befoul made from algae, receive almost no backing.In this new era of national energy, one of the primary questions facing the country is that of timing. When should things happen? And how soon can they occur? "Broadly, what scares me is that we want to do this in an incremental fashion. We want this to come across as painlessly as possible," say Rich Wells, vice president of energy at Dow Chemical. "We need a breakthrough mentality."It has become a cliché to say that there is no "silver bullet" for the nation's energy and climate problems. Most experts prefer to think about energy solutions as a collection of options to be deployed in tandem. Perhaps the most widely quoted example is the "wedge model," developed by Princeton University professors Stephen Pocola andRobert Soochow in 2004. It outlined 15 wedges, each one representing a way to significantly reduce greenhouse gas emissions over the next 50 years. Among them: raising the fuel economy of 2 billion cars from 30 mpg to 60 mpg and doubling nuclear capacity worldwide.Only some of these, of course, are realistic in the shorter term. "If you're only going to do one, the top one is always energy efficiency," says Dow's Wells. "It is for the most part the easiest, cheapest fuel out there." Amory Loins, chief scientist at the nonprofit Rocky Mountain Institute, agrees, saying that energy savings from highly fuel-efficient cars would be equivalent to "finding a Saudi Arabia under Detroit." Perhaps the biggest barrier for buildings has been the upfront cost of doing retrofits, with the need to convince people that the costs can be quickly recouped by lower energy bills. The stimulus package is taking a stab at this, setting aside $5 billion for home weatherization.Meanwhile, everyone else is jockeying for position. The befoul industry wants Congress to lift the cap on how much ethanol can be blended into gasoline. The nuclear industry is asking Congress to cough up billions to insure new nuclear power plants; wind and solar industries are asking for transmission superhighways. Detroit wants more in government bailout money. And coal wants money to research carbon capture technology. The great energy nationalization is here.译文:新能源经济每个国家都希望开发新能晕,但局限于开发的障碍以及技术问题。
热能与动力工程专业外文翻译、中英对照、英汉互译

毕业设计外文翻译原文标题:Proposal for a high efficiency LNGpower-generation System utilizing wasteheat from the combined cycle中文标题:一个高效的利用液化天然气联合循环余热的发电系统学院名称:能源与动力工程学院专业名称:热能与动力工程Proposal for a high efficiency LNG power-generation system utilizing waste heat from the combined cycleY. Hisazumi*, Y. Yamasaki, S. SugiyamaEngineering Department, Osaka Gas Co., 1-2 Hiranomachi 4-chome Chuo-ku, Osaka 541, Japan Accepted 9 September 1998AbstractHigh-efficiency power-generation with an LNG vaporizing system isproposed: it utilizesthe LNG's cold energy to the best potential limit. This system can be applied to LNG vaporizers in gas companies or electric power companies and recovers the LNG's cold energy as electric power. The system consists of a Rankine cycle using a Freon mixture, natural-gas. Rankine cycle and a combined cycle with gas and steam turbines. The heat sources for this system are the latent heat from the steam-turbine's condenser and the sensible heat of exhaust gas from the waste-heat recovery boiler. In order to find out the optimal condition of the system, several factors, such as gas turbine combustion pressure, steam pressure, condensing temperature in combined cycle, composition of mixture Freon, and natural gas vaporizing pressure are evaluated by simulation. The results of these studies show that in the total system, about 400 kWh can be generated by vaporizing 1 ton of LNG, including about 60 kWh/LNG ton recovered from the LNG cold energy when supplying NG in 3.6 MPa.. About 8.2MWh can be produced by using 1 ton of LNG as fuel, compared with about 7 MWh by the conventional combined system. A net efficiency of over 53%HHV could be achieved by the proposed system. In the case of the LNG terminal receiving 5 million tons of LNG per year, this system can generate 240 MW and reduce the power of the sea water pump by more than 2MW. 1998 Elsevier Science Ltd. All rights reserved.1. IntroductionIn the fiscal year 1994, the amount of LNG imported to Japan reached about 43 million tons; of this 31 million tons were used as fuel for power generation. As shown in Fig. 1, about 20% of the LNG imported was used for power generation [2]. Fig. 2 shows the major LNG power generation systems now in operation and their outputs. Several commercial LNG power generation plants have been constructed since 1979, and their total output has reached approximately 73 MW. Among the new power-generation plants without CO2 emission, this value of 73 MW is second to the 450 MW input of geo-thermal power generation plants in Japan, with the exception of power generation by refuse incinerators, and is much larger compared with the 35 MW output of solar-power plants and the 14 MW output of wind-power stations.Table 1 shows the LNG power generation plants constructed in Japan. The economics of LNG power generation became worse as the appreciation of the yen madethe cost of energy kept constant but while raising the construction cost; the adoption of the combined cycle utilizing gas-turbine and steam turbine (hereafter called combined cycle) increased the gas send-out pressure and lowered the power output per ton of LNG. Therefore, no LNG powergeneration plants were constructed in the 1990s due to lower cost effectiveness of the systems.As for the thermal power plant using natural gas as fuel, the steam turbine produced only about 6 MWh of power output per ton of LNG. But recently, improvement in blade-cooling technology and materials of the gas turbine enabled a 1400℃class turbine to be designed and increased the combustion pressure up to 3 MPa. Therefore, as shown in Fig. 3, the heat efficiency of the combined cycle has been improved and the electrical output from 1 ton of LNG has reached about 7MWh.In this paper, a proposal is made for the high-efficiency LNG power generation system based on a new concept which fully utilizes the cold energy without discarding it into the sea. The system is composed of the combined cycle and the LNG power-generation plant.2. High-efficiency LNG power-generation system2.1. Basic componentsFig.4 shows the process flow diagram of the high-efficiency LNG power-generation system. This complex system consists of the combined cycle and the LNG power generation cycle. The combined cycle is composed of a gas turbine (GAS-T) and a steam turbine (ST-T) using natural gas (NG) as fuel, while the LNG power generation cycle is composed of a Freon (uorocarbon) mixture turbine (FR-T) and a natural-gas turbine (NG-HT, NG-LT) using the latent heat of condensation from the exhaust steam and the sensible heat of the exhaust gas as heat sources. The plate fin type heat exchanger can be used for the LNG/natural gas (LNG-CON) and LNG/ Freon mixture (FR-CON). The shell-and-tube type can be selected as exchangers for exhaust steam/natural gas (LNG-VAP),exhaust steam/Freon mixture(FR-VAP), and exhaust gas/natural gas (NG-SH) applications according to the operating conditions.Ice thickness on the surface of the heat-exchanger tubes becomes a problem as heat is exchanged between exhaust gas and cold natural gas or Freon mixture. The ice thickness can be estimated by the technology of heat transfer between LNG and sea water, thus enabling one to avoid blockages due to ice inside the tubes.In addition, stable and continuous send-out of gas is made possible by using a bypass system, even if turbines and pumps for the Freon mixture and natural gas circulating systems (FR-RP, LNG-RP) stop.2.2. Features of the systemThe practical use of the following existing technologies in combination shows the high feasibility of the proposed system:. Power generation using Freon or hydrocarbon type Rankine cycle,. Power generation by natural-gas direct expansion],. TRI-EX type vaporizer which vaporizes LNG by using an intermediate medium or vacuum type LNG vaporizer.The Freon mixture is made up of the HFC type, which is a fluorocarbon consisting of H, F, and C and has no adverse influence on the ozone layer; it enables reduction in exergy loss at the heat exchanger and increases itscirculating flow rate to be achieved.The effective recovery of cold exergy and pressure exergy is made possible by the combined system using natural gas and Freon mixture Rankine cycle.Fig. 5 shows the temperature-heat duty relation when vaporizing 1 kg of LNG in the system shown in Fig. 4. Separation of the condensed natural-gas in two sections enables an increase in the heat duty between Freon (FR) and LNG, and a reduction of difference in temperature of LNG and natural gas between the inlet and outlet of the heat exchanger.3. Evaluation of the characteristics of the proposed system3.1. Process simulationThe characteristics of this system were evaluated by using process simulator. The followings are the conditions used for the calculation:Effciencies of rotating machines LNG compositionGas turbine (GAS-T) 88% CH4 89.39%Steam turbine (ST-T) 85% C2H6 8.65%Natural-gas turbine (NG-HT, LT) 88% C3H8 1.55%Freon turbine (FR-T) 88% iC4H10 0.20%Air compressor (AIR-C) 85% nC4H10 0.15%LNG pump (LNG-MP, RP) 70% iC5H12 0.01%Freon pump (FR-RP) 70% N2 0.05%Natural gas gross heat-value: 10,510 kcal/Nm3AIR/NG flow ratio of gas turbine: 323.2. Effects of send-out pressure of the natural gasWhen natural-gas is sent out at 3.5 or 1.8 MPa, evaluations were made of the effects of send-out pressure of the LNG and change in superheating temperature of the natural gas on the total output of the high pressure (NG-HT) and the low pressure (NG-LT) natural-gas expansion-turbines. Fig. 6 shows the results of this calculation, where self consumption of power is calculated from the power, raising the pressure of the LNG up to the inlet pressure of the turbine minus the power required for the original send-out pressure. In both cases, the inlet pressure rise for the turbine causes an increase of self consumption power, but brings about a greater out-put. About 7 MPa of the inlet pressure of the turbine is appropriate considering the pressure tolerance of the heat exchangers.When the superheating temperature of the natural gas at the inlet to the turbine becomes high, the recovery of power increases, but the temperature of the exhaust gas from the outlet of the natural-gas super heater (NG-SH) declines, thus indicating that there is a limitation to superheating gas.3.3. Effects of combustion pressure of the gas turbineThe outputs of the gas turbine and the steam turbine, and the efficiency per gross heating value were evaluated by changing the combustion pressure of the gas turbine operating at 1300℃turbine-inlet temperature - see Fig. 7.If the combustion pressure of the gas turbine becomes high, the output of the gas turbine increases, but the output of the steam turbine decreases because the rise in combustion pressure causes a lowering of the exhaust-gas temperature at the outlet of the gas turbine and consequently a decline in the steam temperature at the inlet of the steam turbine. However, the overall efficiency of the turbines increases upon increasing the combustion pressure because the increment of gas-turbine output exceeds the decrement of steam turbine output. As a result, taking the pressure loss into account, it is appropriate to set the send-out pressure of the natural gas at the LNG terminal at 3.5 MPa.(FR-vap),3.4. Effects of Inlet pressure of the steam turbineFig. 8 shows the relations between the steam-turbines output and exhaust gas temperatures by changing the steam pressure in the range of 3-7 MPa. As the steam pressure increases, the output of the steam turbine rises and the temperature of the exhaust gases also increase. Besides, the power required for the water-supply pump increases with a rise in the steam pressure. Therefore, the current combined cycles operate at steam pressure of 7 MPa or more because the increment of the output of steam turbine exceeds the additional power required for the water-supply pump.3.5. Rankine cycle using a Freon-mixture refrigerant.The Freon refrigerant was selected from the HFC refrigerants on the basis of marketability, boiling point and freezing-point. Table 2 shows the physical properties of HFC Freon.When only HFC-23 is used as the medium, because of its low freezing-point it never freezes even if heat is exchanged between the LNG and HFC-23. But if HFC-23 is heated by the exhaust steam of the steam turbine, the pressure rises approximately up to the critical pressure. Therefore, the use of HFC-23 is not cost effective, because it is then necessary to set a high design pressure. To cope with this problem, we evaluated the compound refrigerant composed of HFC-134a (with high boiling point) and HFC-23.Fig. 9 shows saturated vapor pressure at various temperatures, the boiling point and the dew point at atmospheric pressure for mixtures of HFC-23 andHFC-134a of various compositions. The saturated pressure at each temperature rises with the increasing mole ratio of HFC-23: Hence, 40-45% of the mole ratio of HFC-23 is the optimal value considering the design pressure of the equipment.Fig. 10 shows the plots of the output of the Freon turbine versus the condensing temperature of the steam turbine when changing the composition of the HFC-23. In this figure, the turbine outlet pressure is determined in such a way that thedifference in temperature between the LNG and Freon mixture is not less than 5℃in the Freon condenser (FR-CON). The Freon turbine's inlet-pressure is set to the saturatedtemperature of the Freon mixture, i.e. less than 2℃from the steam-condensing temperature.This figure indicates that the output of the turbine scarcely correlates with the mole ratio of HFC-23. The higher the steam-condensing temperature becomes, the greater the output per ton of LNG the turbine produces, but in such a case, it is necessary to evaluate the system as a whole because more fuel is required, as described below. The result indicates that the optimal mole composition of HFC-23 and HFC-134a is 40%/60% considering both design pressure and the output of the turbine.3.6. Comprehensive evaluation from the viewpoint of the steam-condensing Temperature.As the dew point of the exhaust gas is 42℃, it is wise to set the exit temperature of the exhaust gas from the natural-gas super heater (NG-SH) to 80℃or more in order to prevent white smoke from the smoke stack. Table 3 shows the effect of the steam-condensing temperature on the generated output of the total system. The lower steam-condensing temperature brings about a higher efficiency of the total system, but also causes a lowering in the inlet temperature of natural-gas turbine. Therefore, it is appropriate to set the steam-condensing temperature at approximately 30℃.When the condensing temperature is 30C, the generated outputs per ton of LNG of the combined cycle and LNG power generation plant are 342.83 and67.55 kWh, respectively, resulting in 402.64 kWh of total generated output aftersubtracting the self-use power. As 48.94 kg of fuel is used for operating the system, the generated outputs of the combined cycle and the total system reach about 7 and 8.2 MWh, per ton of fuel respectively.3.7. Evaluation of exergyNatural-gas is liquefied at an LNG liquefaction terminal, with the consumption of about 380 kWh/LNG-ton: 1 ton of LNG having about 250 kWh of physical exergy as cold exergy and 13.5 MWh of chemical exergy. Fig. 11 shows the result of evaluating the exergy of the system shown in Fig. 4 under the optimal condition. The total output of Freon and natural gas turbines is 67.5 kWh, and the effective recovery percentage of cold exergy is 56%. As 90 kWh out of the pressure exergy can be recovered as output, about 157 kWh of net recovery can be obtained, which indicates the recovery percentage reaches about 63% for 250 kWh of LNG cold exergy. This conversion efficiency is higher than that achieved from chemical exergy to electric power.Most of the exergy loss occurs in the heat exchanger and the turbine, and in mixing with re-condensed LNG. As for the turbines, the loss of energy may be improved by using high-efficiency turbines. On the other hand, modification of the heat exchanger to reduce the energy loss may cause increased complexity of the system and is difficult to be done from the economic viewpoint. Though the recovery.percentage of cold energy in this system is low compared with the 80% in air-separation equipment, this system has the advantage of recovering a large amount of the available cold energy.4. ConclusionThe paper has proposed a high-efficiency LNG power generation system in combination with a combined-cycle power generation system fueled by natural-gas. The system utilizes LNG cold energy and it requires no sea water as a heat source.This system can be applied to LNG vaporization and send-out processes of gas companies or electric-power companies. The system recovers LNG coldenergy as an electric-power output without wasting it into sea water. The system consists of Rankine cycle with Freon mixture and a natural-gas Rankine cycle using the latent heat of exhaust steam from the steam turbine and the sensible heat of exhaust gas from the waste-heat recovery boiler. To improve the total efficiency of the system, a simulation was conducted to evaluate several factors, such as the composition of the Freon mixture, natural gas send-out pressure, as well as the combustion pressure steam inlet pressure, and steam-condensing temperature of the combined cycle. As a result, not less than 60 kWh/LNG-ton of output was generated even at a high natural-gas send-out pressure of 3.5 MPa. This value is considerably higher than the output generated at a LNG send-out pressure of 3 or 4 MPa, as given in Table 2.The system can produce about 400 kWh of net output when vaporizing 1 ton of LNG. While the conventional combined-cycle system in operation generates about 7 MWh when 1 ton of LNG is used as fuel, the system using the same amount of fuel generates about 8.2 MWh with a high degree of efficiency: a not-less-than 53% conversion efficiency was achieved per gross heat value.In the case of an LNG terminal receiving 5 million tons of LNG per year, this system can generate a power of about 240 MW when 600 t of LNG is used in an hour. With the elimination of about 24,000 tons per hour of sea water, which has been used for vaporizing 600 t/h of LNG in the conventional system, no less than 2 MW of electric power for operating sea water pumps can be saved.The proposed system emits no CO2, and can generate a large amount of electricity with high cost efficiency when incorporated into a combined cycle, with no use of sea water. Therefore, we consider that installation of this system is the one of the most favorable means of investment to put a new energy source or energy-saving equipment to practical use.To realize the full potential of this system, it is necessary to understand the heat characteristics of the Freon mixture, the icing and heat transfer characteristics of exhaust steam, the controllability of total system and the characteristics against partial load.References[1] The Center for Promotion of Natural gas Foundation. Research and development report of cold energy utilizing system, 1994[2] Japan's Energy and Economy Research Center. Energy and economy statistical data in 1995[3] Abe. Operating results and future prospect of a recent combined-cycle power generation plant. Thermal and Nuclear Power 1995;46(6):33-41[4] Maertens J. Design of Rankine cycles for power generation. Int. Refrig. 1986;9:137-43[5] Terada, Nakamoto. Power generation utilizing LNG cold. Thermal and Nuclear Power Generation 1986;37(10):66-71[6] Ooka, Ueda, Akasaka. Advanced LNG vaporizer and power generation utilizing LNG cold. Chemical Engineering 1981;45(3):187-90[7] Miura. The development of LNG vaporizer using vacuum steam heat (VSV). Journal of Japan Gas Society 1992;45:34-6[8] Nagai. Software-package and the usage. Chemical Equipment1994;August:31-7[9] Daikin Co. Ltd. Freon Data Sheet of HFC23一个高效的利用液化天然气联合循环余热的发电系统日本大阪541燃气有限公司工程部1-2平野町4肖梅中央谷,1998年9月概述本文提出了一个高效液化天然气气化发电系统,它是利用液化天然气冷能的最佳潜能极限。
能源类外文文献翻译(译文1)

太阳能蒸馏:一种有前途的供水代替技术,它使用免费的能源,技术简单,清洁Hassan E.S.Fath埃及,亚历山大,亚历山大大学机械学院工程系摘要:太阳能蒸馏为盐水淡化提供了一种替代技术,它使用免费的能源、技术简单、清洁,为人类提供所需的部分淡水。
太阳能蒸馏系统的发展已经证明:当天气情况良好,并且需求不太大时,比如少于200立方米/天,它在海水淡化过程中有一定的适用性。
太阳能蒸馏器的产量低这个问题迫使科学家研究许多提高蒸馏器产量和热效率,以此来降低产水的费用。
本文对许多最新发展的单效和多效太阳能蒸馏器进行了整体评论和技术评估。
同时,对蒸馏器构造的发展、各部件在运行过程中出现的问题、对环境的影响也进行了阐述。
关键词:太阳能;海水淡化1.简介在淡水需求超出了淡水资源所能满足的量的地方,对低质量的水进行去盐处理是一种合适的淡水来源途径。
对盐水或海水脱盐处理取得淡水满足了社会基本的需求。
一般说来,它不会对环境造成严重的损害作用。
因此,进行海水淡化的工序和工厂在数量上和能力上都有了巨大的进步。
许多不同的海水淡化技术被用来从盐水中分离淡水,包括有:多级闪蒸(MSF)、多效(ME)、蒸汽压缩(VC)、反渗透(RO)、离子交换、电渗析、相变和溶剂萃取。
但是,这些技术只能产生少量的淡水,因而是昂贵的。
另一方面,用来驱动这些技术的传统能源也会对环境产生消极的作用。
而太阳能蒸馏为盐水淡化技术提供了一种有前途的替代处理过程,它使用免费的能源,技术简单,清洁,并能为人类提供所需的部分淡水。
太阳能蒸馏系统的发展已经证明:当天气情况良好,并且需求不太大时,比如少于200立方米/天,它在海水淡化过程中有一定的适用性。
太阳能蒸馏器的产量和热效率,以此来最小化产水费用。
这些方法中包括被动的和主动的单效蒸馏器。
一些工作者也曾试图都产生的水蒸气在外部凝结(在额外的凝结表面上)。
另一方面,浪费的凝结潜热也被利用,从而增加馏出水的产量和提高效率。
能源专业外文翻译--燃料电池及其发展前景1

外文原文:Fuel Cells and Their ProspectsA fuel cell is an electrochemical conversion device. It produces electricity fromfuel (on the anode side) and an oxidant (on the cathode side), which react in the presence of an electrolyte. The reactants flow into the cell, and the reaction products flow out of it, while the electrolyte remains within it. Fuel cells can operate virtually continuously as long as the necessary flows are maintained.Fuel cells are different from electrochemical cell batteries in that they consume reactant from an external source, which must be replenished--a thermodynamically open system. By contrast batteries store electrical energy chemically and hence represent a thermodynamically closed system.Many combinations of fuel and oxidant are possible. A hydrogen cell uses hydrogen as fuel and oxygen (usually from air) as oxidant. Other fuels include hydrocarbons and alcohols. Other oxidants include chlorine and chlorine dioxide.Fuel cell designA fuel cell works by catalysis, separating the component electrons and protonsof the reactant fuel, and forcing the electrons to travel though a circuit, hence converting them to electrical power. The catalyst typically comprises a platinum group metal or alloy. Another catalytic process takes the electrons back in, combining them with the protons and oxidant to form waste products (typically simple compounds like water and carbon dioxide).A typical fuel cell produces a voltage from 0.6 V to 0.7 V at full rated load.Voltage decreases as current increases, due to several factors:•Activation loss•Ohmic loss (voltage drop due to resistance of the cell components and interconnects)•Mass transport loss (depletion of reactants at catalyst sites under high loads, causing rapid loss of voltage)To deliver the desired amount of energy, the fuel cells can be combined in series and parallel circuits, where series yield higher voltage, and parallel allows a stronger current to be drawn. Such a design is called a fuel cell stack. Further, the cell surface area can be increased, to allow stronger current from each cell.Proton exchange fuel cellsIn the archetypal hydrogen–oxygen proton exchange membrane fuel cell (PEMFC) design, a proton-conducting polymer membrane, (the electrolyte), separates the anode and cathode sides. This was called a "solid polymer electrolyte fuel cell" (SPEFC) in the early 1970s, before the proton exchange mechanism was well-understood. (Notice that "polymer electrolyte membrane" and "proton exchange mechanism" result in the same acronym.)On the anode side, hydrogen diffuses to the anode catalyst where it later dissociates into protons and electrons. These protons often react with oxidants causing them to become what is commonly referred to as multi-facilitated proton membranes (MFPM). The protons are conducted through the membrane to the cathode, but the electrons are forced to travel in an external circuit (supplying power) because the membrane is electrically insulating. On the cathode catalyst, oxygen molecules react with the electrons (which have traveled through the external circuit) and protons to form water — in this example, the only waste product, either liquid or vapor.In addition to this pure hydrogen type, there are hydrocarbon fuels for fuel cells, including diesel, methanol (see: direct-methanol fuel cells and indirect methanol fuel cells) and chemical hydrides. The waste products with these types of fuel are carbon dioxide and water.The materials used in fuel cells differ by type. In a typical membrane electrode assembly (MEA), the electrode–bipolar plates are usually made of metal, nickel or carbon nanotubes, and are coated with a catalyst (like platinum, nano iron powders or palladium) for higher efficiency. Carbon paper separates them from the electrolyte. The electrolyte could be ceramic or a membrane.Oxygen ion exchange fuel cellsIn a solid oxide fuel cell design, the anode and cathode are separated by an electrolyte that is conductive to oxygen ions but non-conductive to electrons. The electrolyte is typically made from zirconia doped with yttria.On the cathode side, oxygen catalytically reacts with a supply of electrons to become oxygen ions, which diffuse through the electrolyte to the anode side. On the anode side, the oxygen ions react with hydrogen to form water and free electrons. A load connected externally between the anode and cathode completes the electrical circuit.Fuel cell design issuesCostsIn 2002, typical cells had a catalyst content of US$1000 per-kilowatt of electric power output. In 2008 UTC Power has 400kw Fuel cells for $1,000,000 per 400kW installed costs. The goal is to reduce the cost in order to compete with current market technologies including gasoline internal combustion engines. Many companies are working on techniques to reduce cost in a variety of ways including reducing the amount of platinum needed in each individual cell. Ballard Power Systems have experiments with a catalyst enhanced with carbon silk which allows a 30% reduction (1 mg/cm2 to 0.7 mg/cm2) in platinum usage without reduction in performance.The production costs of the PEM (proton exchange membrane). The Nafion membrane currently costs €400/m². In 2005 Ballard Power Systems announced that its fuel cells will use Solupor, a porous polyethylene film patented by DSM.Water and air management (in PEMFC). In this type of fuel cell, the membrane must be hydrated, requiring water to be evaporated at precisely the same rate that it is produced. If water is evaporated too quickly, the membrane dries, resistance across it increases, and eventually it will crack, creating a gas "short circuit" where hydrogen and oxygen combine directly, generating heat that will damage the fuel cell. If the water is evaporated too slowly, the electrodes will flood, preventing the reactants from reaching the catalyst and stopping the reaction. Methods to manage water in cells are being developed like electroosmotic pumps focusing on flow control. Just as in a combustion engine, a steady ratio between the reactant and oxygen is necessary to keep the fuel cell operating efficiently.Temperature managementThe same temperature must be maintained throughout the cell in order to prevent destruction of the cell through thermal loading. This is particularly challenging as the 2H2 + O2 =2H2O reaction is highly exothermic, so a large quantity of heat is generated within the fuel cell.Durability, service life, and special requirements for some type of cells Stationary fuel cell applications typically require more than 40,000 hours of reliable operation at a temperature of -35°C to40°C, while automotive fuel cells require a 5,000 hour lifespan (the equivalent of 150,000 miles) under extreme temperatures. Automotive engines must also be able to start reliably at -30 °C and have a high power to volume ratio (typically 2.5 kW per liter).HistoryThe principle of the fuel cell was discovered by German scientist Christian Friedrich Schönbein in 1838 and published in one of the scientific magazines of thetime. Based on this work, the first fuel cell was demonstrated by Welsh scientist Sir William Robert Grove in the February 1839 edition of the Philosophical Magazine and Journal of Science, and later sketched, in 1842, in the same journal. The fuel cell he made used similar materials to today's phosphoric-acid fuel cell.In 1955, W. Thomas Grubb, a chemist working for the General Electric Company (GE), further modified the original fuel cell design by using a sulphonated polystyrene ion-exchange membrane as the electrolyte. Three years later another GE chemist, Leonard Niedrach, devised a way of depositing platinum onto the membrane, which served as catalyst for the necessary hydrogen oxidation and oxygen reduction reactions. This became known as the“Grubb-Niedrach fuel cell”. GE went on to develop this technology with NASA and McDonnell Aircraft, leading to its use during Project Gemini. This was the first commercial use of a fuel cell. It wasn't until 1959 that British engineer Francis Thomas Bacon successfully developed a 5 kW stationary fuel cell. In 1959, a team led by Harry Ihrig built a 15 kW fuel cell tractor for Allis-Chalmers which was demonstrated across the US at state fairs. This system used potassium hydroxide as the electrolyte and compressed hydrogen and oxygen as the reactants. Later in 1959, Bacon and his colleagues demonstrated a practical five-kilowatt unit capable of powering a welding machine. In the 1960s, Pratt and Whitney licensed Bacon's U.S. patents for use in the U.S. space program to supply electricity and drinking water (hydrogen and oxygen being readily available from the spacecraft tanks).United Technologies Corporation's UTC Power subsidiary was the first company to manufacture and commercialize a large, stationary fuel cell system for use as a co-generation power plant in hospitals, universities and large office buildings. UTC Power continues to market this fuel cell as the PureCell 200, a 200 kW system (although soon to be replaced by a 400 kW version, expected for sale in late 2009). UTC Power continues to be the sole supplier of fuel cells to NASA for use in space vehicles, having supplied the Apollo missions, and currently the Space Shuttle program, and is developing fuel cells for automobiles, buses, and cell phone towers; the company has demonstrated the first fuel cell capable of starting under freezing conditions with its proton exchange membrane automotive fuel cell.Fuel cell efficiencyThe efficiency of a fuel cell is dependent on the amount of power drawn from it. Drawing more power means drawing more current, which increases the losses in the fuel cell. As a general rule, the more power (current) drawn, the lower the efficiency.Most losses manifest themselves as a voltage drop in the cell, so the efficiency of a cell is almost proportional to its voltage. For this reason, it is common to show graphs of voltage versus current (so-called polarization curves) for fuel cells. A typical cell running at 0.7 V has an efficiency of about 50%, meaning that 50% of the energy content of the hydrogen is converted into electrical energy; the remaining 50% will be converted into heat. (Depending on the fuel cell system design, some fuel might leave the system unreacted, constituting an additional loss.)For a hydrogen cell operating at standard conditions with no reactant leaks, the efficiency is equal to the cell voltage divided by 1.48 V, based on the enthalpy, or heating value, of the reaction. For the same cell, the second law efficiency is equal to cell voltage divided by 1.23 V. (This voltage varies with fuel used, and quality and temperature of the cell.) The difference between these numbers represents the difference between the reaction's enthalpy and Gibbs free energy. This difference always appears as heat, along with any losses in electrical conversion efficiency.Fuel cells do not operate on a thermal cycle. As such, they are not constrained, as combustion engines are, in the same way by thermodynamic limits, such as Carnot cycle efficiency. At times this is misrepresented by saying that fuel cells are exempt from the laws of thermodynamics, because most people think of thermodynamics in terms of combustion processes (enthalpy of formation). The laws of thermodynamics also hold for chemical processes (Gibbs free energy) like fuel cells, but the maximum theoretical efficiency is higher (83% efficient at 298K) than the Otto cycle thermal efficiency (60% for compression ratio of 10 and specific heat ratio of 1.4). Comparing limits imposed by thermodynamics is not a good predictor of practically achievable efficiencies. Also, if propulsion is the goal, electrical output of the fuel cell has to still be converted into mechanical power with the corresponding inefficiency. In reference to the exemption claim, the correct claim is that the "limitations imposed by the second law of thermodynamics on the operation of fuel cells are much less severe than the limitations imposed on conventional energy conversion systems". Consequently, they can have very high efficiencies in converting chemical energy to electrical energy, especially when they are operated at low power density, and using pure hydrogen and oxygen as reactants.In practice, for a fuel cell operating on air (rather than bottled oxygen), losses due to the air supply system must also be taken into account. This refers to the pressurization of the air and dehumidifying it. This reduces the efficiency significantlyand brings it near to that of a compression ignition engine. Furthermore fuel cell efficiency decreases as load increases.The tank-to-wheel efficiency of a fuel cell vehicle is about 45% at low loads and shows average values of about 36% when a driving cycle like the NEDC (New European Driving Cycle) is used as test procedure. The comparable NEDC value for a Diesel vehicle is 22%. In 2008 Honda released a car with fuel stack claiming a 60% tank-to-wheel efficiency.Fuel cells cannot store energy like a battery, but in some applications, such as stand-alone power plants based on discontinuous sources such as solar or wind power, they are combined with electrolyzers and storage systems to form an energy storage system. The overall efficiency (electricity to hydrogen and back to electricity) of such plants (known as round-trip efficiency) is between 30 and 50%, depending on conditions. While a much cheaper lead-acid battery might return about 90%, the electrolyzer/fuel cell system can store indefinite quantities of hydrogen, and is therefore better suited for long-term storage.中文译文:燃料电池及其发展前景燃料电池是一种电化学转换装置。
生物质能源外文翻译外文文献英文文献中国的生物质能源

China’s Biomass EnergyChina leads the world in its energy reservation and is the second largest energy producer and consumer in the world. It is estimated that China has 4000 billion tons of potential primary energy reservation. However, per capita energy resource quantity and consuming quantity is far smaller than the world average level. The main characteristics of China’s energy exploration and utilization are as follows.1. Coal is the primary energy; exploration and utilization of renewable resources is supplementary. China’s explored reserves of coal resource accounts for over 90% of the primary energy total, such as coal, oil, natural gas, water energy and nuclear energy. Coal is dominant id China’s energy production and consumption.on.2. Energy consumption volume is increasing while energy utilization efficiency is comparatively low. As the economic scale expands, China’s energy consumption is on the constant increase. Under the influences of capital, technology, energy price, etc, China’s energy utilization efficiency is far lower than that of the developed countries. Energy comprehensive utilization efficiency is 32% and the overall energy systematic efficiency 9.3%. These numbers are only half of the developed country level.3. With the sustained increase of energy consumption, the coal-dominant energy structure has caused urban air pollution while with the excessive consumption of bio-mass energy giving rise to ecological destruction, the pressure on ecological environment is more and more severe. According to the World Bank, the economic loss caused by air and water pollution in China, approximately accounts for 3%--8% of GNP.4. The energy structure is getting more and more pluralized. By the middle of the 1990’s, energy self-sufficiency rate was 98%, while at present China is the second largest crude oil importer only after USA.The rapid development of China’s economy is based on the sufficient supply of energy, however, the higher-than-normal oil price on the international market together wit h China’s ever-increasing energy demand will be a serious subject faced withChina’s development road.More than 3200 places of terrestrial heat have been discovered in China, 225 of them could be used for power generation. It is estimated that the exploitable reserve of terrestrial heat in China is equal to the power of 462.65 billion tons of standard coal. In recent years, the use of terrestrial heat in our country has increased by 7%. We have usedChina will adopt the sustainable energy development str ategy of an “energy saving prioritized, plural structured, and environment friendly” nature in the coming 20 years. We will adopt the international energy strategy and strive to quadruple GDP with double energy consumption with the help of mechanism innovation and technological advancement. The following specific goals are expected to reach under the sustained energy development strategy: energy consumption of per unit GNP will be reduced by 20% on the level of the end of the 10th five-year plan; primary energy demand will be less than 2.5 billion tons of standard coal by 2020, saving 0.8 billion tons; coal consumption ratio is controlled under 60%, renewable energy utilization reaches 525 million standard coal (power generation by renewable energy stands at 100 million kilowatt); oil importing reliance is controlled under around 60%; the reduction rate of main pollutants is 45%--60%.The Development of New Energy in ChinaNew energy and renewable energy only started to develop in China. As pointed out in the Annual Report of China’s New Energy Industry released in January, 2007, China will largely increase the rate of new energy such as wind power, solar power, and biomass power in the overall energy consumption. The report says, our first step is; the new energy installed capacity will be 120 million kw by 2020, that is 12% of China’s total installed power capacity. We will have a boost of power generation by using wind, bio-energy and solar energy. The second step is; the percentage of new energy consumption will increased dramatically comparing to other kind of energies. By 2050, the new energy will account for 30%--40% of the total energy demand of China. Now l would like to give you a brief introduction on wind power, biomass energy, solar energy and terrestrial energy in China.Wind PowerThere are abundant wind power within our huge territory and along our long coastline. According to the survey of China meteorological Academy, based on the results of 900 weather stations, there is 253 million kw of wind power reserve 10 m below our earth. There is a great potential of wind power in the coastal area of eastern China 2---15 meters below sea level. Therefor ,we have a promising future in wind power generation there.Compiled by China Energy Comprehensive Use Association and European Wind Power Society, in a Report named Wind Force 12 in China, it is expected that China will have 40 million kw of wind power capacity in 2020, if is could develop fully. Then, the wind power will go beyond nuclear power and become the third largest power source in China. If that is true, China’s annual wind power generation would go up to 80 billion kwh, it could be enough for 80 million people. Meantime, it would reduce 48 million tons of CO2 emission.The report also says that China will need over 25 thousand of large wind generators by the year 2020, wind power sale will increase to morethan 300 billion yuan, that will create at least 150 thousand of job opportunities. It indicates that in the past 5 years, the cost of wind power will reduce by 20%, its technology cost is one of the lowest which can be decreased among the renewable energies.Biomass energyAccording to the Outline for Mid-- long Term Development of Renewable Energy in China, the goal of renewable energy development in the next 15 years is; by 2020, the renewable energy will occupy 16% in the energy structure of China. Among them, the production of ethanol is going up to 10 million tons; bio-diesel fuel 2 million tons; and the major part of the biomass energy development target are bio-energy power generation, Firedamp projects biomass liquid fuel and Biomass solid fuel. The biomass project in China has made great progress now, but it is still in an initial stages.According to the Supporting Policy of Bio-energy and Bio-chemistrydevelopment jointly promulgated by several ministries, China will give favorable treatments to support biomass energy development in the following aspects: setting up risk fund system, providing flexible loss subsidy; subsidies for raw material bases and demonstration projects; tax reduction is included. We can predict that the biomass energy in China will be developed rapidly in years to come.Terrestrial Energy12604.6 GWH of terrestrial heat till 2005 with an installed capacity of 3687 MWT, which rank number 1 and number 3 in the world respectively.We use the high heat to generate power and low middle heat to daily purposes. three thermo-power plants have been build in Tibet now, the total installed capacity in China is 29 mw. Terrestrial heat Floors have seen both economical and environmental benefit in the northern part of China as well as in big or medium cities such as: Beijing, Tianjin, Xi’an and Anshan. It is growing fast in agricultural areas like green house (terrestrial),breed aquatics, irrigation, in health care, recreation and tourism ,too China tops the world in utilization of terrestrial heat, but it is only 0.5% of our total energy. Terrestrial power is only 0.35% of the world total generation. We still have a long way to go in developing and utilizing the terrestrial heat.Ocean EnergyExperts have pointed out, while we are exploring petroleum, natural gas, coal and petrification energy, China ought to focus on the future, exploring the promising ocean energy. The clean, renewable ocean energy is the key source of solving the global energy problem, exploiting of ocean energy has been aroused much attention in USA, UK and Australia, some of the experiments have entered a phase of trial operation and evaluation. However, China, Japan and other eastern Asian counties have not realized the true meaning of ocean energy exploration.China’s Energy StrategyIn order to achieve the goal of building a well-off society and face the severe challenge of the long-term energy development, it is of overriding significance for China to adopt the right energy development strategy. Drawing upon the successful experiences of the developed countries and also proceeding from its nationalcondition, China will establish a long and middle term sustainable energy strategy that is in accordance with increasing energy efficiency and protecting the environment.中国的生物质能源中国的能源蕴藏量位居世界前列,同时也是世界第二大能源生产国与消费国。
外文资料及翻译

外文翻译ANALYSIS OF HVAC SYSTEM ENERGYCONSERVATIONIN BUILDINGSABSTRACTE conomic development and people's increasing demand for energy, but the nature of the energy is not inexhaustible. Environment and energy issues become increasingly acute, if no measures are taken, then the energy will limit the rapid economic development of the question.With the improvement of living standard, building energy consumption in the proportion of total energy consumption is increasing. In developed countries, building energy consumption accounts for 40% of total energy consumption of the community, while the country despite the low level of socio-economic development, but the building energy consumption has nearly 30% of total energy consumption, and still rising. Therefore, in western countries or in China, building energy consumption is affecting the socio-economic status of the overall development of the question. In building energy consumption, the energy consumption for HVAC systems has accounted for 30% of building energy consumption -50%, with the extensive application of HVAC, energy consumption for HVAC systems will further increase Great. HVAC systems are often coupled with high-quality electric energy, and our power and relatively tight in some areas, lack of energy supply and demand which is bound to lead to further intensification of contradictions. Therefore, energy-saving heating, higher professional requirements is inevitable across the board.KEYWORDS:energy-saving,HVAC1. Energy saving design measures should be takenRapid changes in science and technology today, area HVAC new technologiesemerge, we can achieve a variety of ways of energy saving HVAC systems.1.1 Starting from the design, selecting, designing HVAC systems, so that the efficient state of the economy running.Design is a leading engineering, system design will directly affect its performance. The building load calculation is an important part of the design, a common problem is that the current design of short duration, many designers to save time, wrong use of the design manual for the design or preliminary design estimates of cold, heat load with the unit construction area of cold, heat load index, direct construction design stage as hot and cold load to determine the basis, often making the total load is too large, resulting in heating equipment, air conditioning is too large, higher initial investment, operating costs, increased energy consumption.1.2 using the new energy-saving air-conditioning and heating comfort and healthy mannerAffect human thermal comfort environment of many parameters, different environmental parameters can get the same effect of thermal comfort, but for different heat and moisture parameters of the environment of its energy consumption air conditioning system is not the same.1.3 Actual situation of a reasonable choice of cold and heat sources, seek to achieve diversification of cold and heat sourceWith the extensive application of HVAC systems on non-renewable energy consumption also rose sharply, while the broken part of the ecological environment are becoming increasingly intensified. How to choose a reasonable heating sources, has caused widespread concern of all parties.1.4 to enhance the use of hot and cold recycling of the work, to achieve maximum energyHVAC systems to improve energy efficiency is one of the ways to achieve energy-saving air-conditioning. Heat recovery system installed mainly through energy recovery, with the air from wind energy to deal with new, fresh air can reduce the energy required for processing, reducing the load, to save energy. In the choice of heat recovery, the should be integrated with the local climate Tiao Jian, Jing Ji situation, Gong Cheng actual situation of harmful exhaust gases of the situation in avariety of factors Deng integrated to determine the Xuanyong suitable heat recovery, so as to achieve Hua Jiao Shao's investment, recovery of more heat (cold) the amount of purpose.1.5 focus on development of renewable energy, and actively promoting new energyAs the air-conditioning systems used in high-grade, non-renewable energy resources and environmental problems caused by the increasingly prominent, have to develop some reasonable and effective renewable energy to ease the current tensions. To heat (cold) and solar and other renewable resources used in air conditioning and refrigeration, has certain advantages, but also clean and pollution-free. Ground Source Heat Pump is a use of shallow and deep earth energy, including soil, groundwater, surface water, seawater, sewage, etc. as a cold source in winter and summer heat is not only heating but also a new central air-conditioning system cooling.2. Saving design problemsAchieve energy-saving HVAC systems, now has a lot of mature conditions, but in practical applications there are some problems:2.1 The issue of public awareness of energy conservationThe past is not enough public understanding of energy, and on the air conditioning is also very one-sided view. For a comfort of air conditioning system or heating system, should the human body has a very good comfort. But the prevailing view now is: the colder the better air-conditioning, heating the more heat the better. This is obviously we seek the comfort of air conditioning is contrary to the view. In fact, this not only greatly increase the energy consumption of air conditioning heating, indoor and outdoor temperature and because of the increase, but also to the human body's adaptability to different environmental decline, lowering the body immunity. Therefore, we need to improve advocacy efforts to change public to the traditional understanding of air conditioning and heating, vigorous publicity and promotion in accordance with building standards and the cold heat energy metering devices to collect tolls, raise public consciousness of energy.2.2 The design concept of the problemReasonable energy-saving design is a prerequisite. At present, some designers due to inadequate attention to design empirical value when applied blindly, resulting in the increase of the initial investment, energy consumption surprising, therefore recommended that the government functions and the energy-saving review body, to increase the monitoring of the HVAC air-conditioning energy saving efforts enhance staff awareness of energy conservation design, so that energy conservation is implemented.2.3 The promotion of new technologies issueNew technology in the HVAC system for energy conservation provides a new direction. Such as ground source heat pump systems, solar cooling and heating system, not only to achieve efficient use of renewable energy, and can bring significant economic benefits, is worth promoting. However, as with any new technology, these new technologies are often high in cost, and the geographical conditions of use have certain limitations, and technically there are still many areas for improvement to improve. Therefore, new energy-efficient technologies, we should be according to local conditions, sum up experience, and actively promote.3. ConclusionHVAC systems saving energy in the building occupies a very important position, should attract enough attention to the designer. Designers should be from a design point of view fully into account the high and strict compliance with energy standards energy saving ideas to run through all aspects of the construction sector. Energy-saving technologies and renewable energy recycling, the Government and other relevant departments should support and vigorously promoted. And the design, construction, supervision, quality supervision, municipal administration and other departments should cooperate closely and pay close attention to implementing a cold, heat metering devices to collect tolls, so people really get benefit from energy efficient building, energy-saving construction and non-heating energy efficient building can not have the same charge standard. At the same time to raise public awareness of energy conservation, and vigorously promote the development of new energy-saving technologies to achieve sustainable development of society.References[1] "residential design standard" DBJ14-037-2006.[2] "Public Buildings Energy Efficiency Design Standards" DBJ14-036-2006.[3] "Technical Specification for radiant heating" JGJ142-2004.析暖通空调系统在建筑中的节能问题摘要经济的发展使人们对能源的需求不断增加,但是自然界的能源并不是取之不尽,用之不竭的。
- 1、下载文档前请自行甄别文档内容的完整性,平台不提供额外的编辑、内容补充、找答案等附加服务。
- 2、"仅部分预览"的文档,不可在线预览部分如存在完整性等问题,可反馈申请退款(可完整预览的文档不适用该条件!)。
- 3、如文档侵犯您的权益,请联系客服反馈,我们会尽快为您处理(人工客服工作时间:9:00-18:30)。
二氧化碳跨临界热泵循环的优化同时制冷和制热应用J. Sarkar, Souvik Bhattacharyya, M. Ram Gopal机械工程学系,印度理工学院,印度,克勒格布尔7213022003年5月2日收到,在2004年2月2日收到修改版;于2004年3月9日接收摘要:在这篇文章中将会介绍能量和物质的分析,以及一个跨临界二氧化碳热泵系统的优化研究。
利用已开发的计算机代码,以获得亚临界和超临界的热力学和运送二氧化碳的性质。
估计系统的各个组件的不可逆性,以及可能导致性能改进的措施。
“公约”缔约方会议的趋势表明,这种系统是更适合高加热端温度和温和冷端温度的。
已得出的最佳循环参数为这些相关提供有用的指引,以获得最佳的系统设计和选择合适的工作条件。
关键字:优化;热泵;二氧化碳;热力循环;高压;建模;热力学性质1.引言早在1850年,二氧化碳蒸气压缩式制冷系统就获得专利,其次是其使用的几十年,主要集中在海洋应用。
早期面临的几个问题主要是由于低临界二氧化碳为基础的低温系统。
作为一个结果,相比于发明卤代烃制冷剂,二氧化碳是缓慢逐步淘汰制冷和空调应用阳离子。
然而,相比发现对环境有害影响合成卤代烃制冷剂,二氧化碳是一种新的兴起天然制冷剂。
Lorentzen和佩特森[1-3] Riffat 的等[4],通过他们的最新研究已经证明,在系统运行临界区,二氧化碳的临界温度低的问题可以有效地克服。
这导致二氧化碳跨临界循环冷凝器由气体冷却器来取代的发展。
据发现,气体冷却器散热使用一个不同寻常的地方昼夜温差大滑翔提供了一些独特的可能性,如同时制冷和热水加热/蒸汽生产简单的控制能力等。
一些理论和实验研究进一步激发以二氧化碳为基础的系统在不同应用中的兴趣。
环境友好,价格低廉,容易获得,不易燃,无毒,兼容各种常见的材料,压实度由于高工作压力,二氧化碳作为制冷剂具有良好的输运性质和可再生性。
过去的研究表明,以二氧化碳为基础的系统在在变频空调与热泵两个行业同时加热和冷却具有很大的潜力。
Nekas做了广泛应用二氧化碳热泵报告[5]。
Neksa[6]和yarrall等人[7]已进行了对跨临界二氧化碳热泵系统实验研究。
一些制造商(主要是日本)已经宣布计划将二氧化碳热泵热水器和一些实际上已推出的产品在市场上投放。
虽然一些研究相关的系统性能分析和优化的报告[8–10],这样的同时加热和冷却应用的系统理论优化研究是稀缺的文学。
在本研究中,已进行了二氧化碳系统同时冷却和加热应用的第一及第二定律分析。
评估二氧化碳在亚临界和超临界区域物理性质的计算机代码已经被开发出来。
在此基础上,进行了的性能系数和能源利用效率方面的系统的优化。
获得最佳的排气压力,与气体冷却器入口温度和性能系数在最佳条件的蒸发器和气体冷却器出口温度相关。
2.热力学性质评估在基于对二氧化碳热力学性质的实验数据的亥姆霍兹能源方程研究的基础上,Span and Wagner [11]已经开发出一种新的基本方程的形式。
基于这一开创性的计算机代码的“CO2PROP”评估二氧化碳热力学性质已经发展到了在亚临界和超临界区域。
该代码采用的技术是在亥姆霍兹自由能函数基础上衍生出来的。
有效的迭代程序已被用于预测各种状态属性。
根据状态方程的计算,一个比较系统发布的属性表[11]最多可产生0.1%的偏差。
值得一提的是,本代码比目前一些可用的商业软件代码在临界点周围区域的表现更好。
3.过程分析和模拟一个简化的二氧化碳加热和冷却系统草图的主要部件如图1所示。
相应的温---熵图,如图2所示,它是采用热力学属性代码CO2PROP绘制的。
如图所示,在内部热交换器里,饱和蒸气在状态6到状态1处于过热状态,然后在压缩机压缩到状态2。
处在状态2超临界二氧化碳通过气体冷却器向外部流体排热被冷却到状态3,(有效的加热效果)。
不同于冷凝器,气体冷却器的散热需要有温度的滑移.从状态3到4二氧化碳在高压下进一步在内部热交换器中被冷却。
经过换热器后,二氧化碳通过膨胀机膨胀至状态5,这是蒸发器入口。
制冷剂的变化,因为它在蒸发器中蒸发提取热量从外部流体(有用的冷却效果)5日至6的状态。
图。
2,进程1-2S是一个熵压缩过程,而过程1-2是实际压缩过程。
下面的过程2-3的虚线代表外部流体上述蒸发过程中的加热和虚线表示外部冷却液。
整个系统遵循已在模版基础的能源平衡系统的各个组成部分产生的守恒方程。
稳态的能量方程式根据热力学第一定律的方程受雇于每一种情况和使用特定的能源数量。
分析已作出以下假设:1.与周围环境的热传输可以忽略不计。
2.一直被认为是单相换热外部流体。
3.是绝热的,但非绝热压缩过程。
4.蒸发过程是恒压。
(i)蒸发器的制冷效果(ii)气体冷却器的效应(iii)压缩机的输入功(iv)内部热交换器的能量平衡(v)整个系统的能量平衡(vi)气体冷却器与外部被加热液体的能量平衡(vii)蒸发器与外部被冷却液体的能量平衡内部换热器的效率是:它可以指出,即使分析会完全是相同的其他类型压缩机,但是上述相关的是一特定类型的压缩机。
尽管真正的系统可以有压缩机等熵效率依赖于过热度,但这种相关性是独立的过热。
3.1 可用能量分析每个已执行的可用能量分析的基本稳定系统的组成部分状态方程:系统净可用能量传递=传热的可用能量传递+工作转移的可用能量传递+可用能量的流量改变(i)压缩机的不可逆(ii)膨胀过程的不可逆(iii)内部换热器的不可逆(iv)在蒸发器里总的可用能量的改变由于忽略了不可逆性的压降,蒸发器的不可逆是:蒸发器输出的有用能量是外部流体的热力学平均温度(v)在气体冷却器中总的有用能的变化不可逆性的百分比已估计代表各组分整体系统的不可逆性和给予完全不可逆性系统的个组件不可逆性的速率。
以上提出的热力学分析,成熟的模拟代码。
此代码综合属性的代码CO2PROP计算相关的热力学参数。
4、最佳流量压力研究[8,9,12]表明,空气冷却压力和非单调性对跨临界二氧化碳系统的性能系数有显著影响。
因此存在一个最佳压力系统产量的最好性能系数和最大性能系数条件下设计一个跨临界二氧化碳周期对应的最佳操作认识。
气体冷却器出口温度是取决于外部流体的入口温度,因此,在任何排放压力下,冷却器出口温度将被固定在一定的流体入口条件。
存在一个固定的最佳压力冷却器出口温度可以由以下参数确定。
以1-2-3-4-5-6-1(图3)为一周期,加热模式的性能系数:由于二氧化碳的特有性能周围的临界点、超临界点、斜坡等温线为一个特定的压力范围相当平缓;其他方面的压力高于和低于此范围内,等温线相当陡峭。
随着压力的增加,△h3增加量明显大于△h2,如图 3,这导致在改进的性能系数值可由式(26)观察。
在一个特定的压力下,性能系数达到一个最高值和相应的压力是称为周期的最佳压力。
随着压力的进一步增加,△h3没有超过△h2的必要产物,并且性能系数开始下降。
压力范围等温线相当平坦,这有利于增加不同冷却器出口温度的性能系数。
因此,气体冷却器出口温度担当着决定最佳周期的有力角色。
5 结果与讨论二氧化碳的加热和冷却系统性能系数,火用效率,各项工作流体冷热两端的温度和压力和不可逆转分数的各个组件是重要的设计和性能参数。
这些参数相应的绘制说明各种性能趋势和最佳操作点。
二氧化碳系统的性能研究同时加热和冷却的应用是在加热和冷却的性能系数基础上的评估,在各种操作条件下的评估,压缩机排气压力以0.5 bar的速率逐步增加。
结果显示组合系统的性能系数,是简单的加热和冷却方式的性能系数。
最大的系统的性能系数显示,相应最佳的各种冷却器出口温度为35℃的温度排气压力变化和60%的换热器内部的热量成效图如图 4。
在蒸发器温度由-10℃上升到10℃,系统性能系数急剧增加记录同比增长超过75%。
然而,最佳的压力蒸发温度变化是显着的要少得多。
虽然实验结果表明,随着蒸发器温度的增加,最佳的压力增加是由于在冷却器出口温度增加,这里最佳排出压力呈现出不同的性质是由于在冷却器出口温度的约束。
正如前面所说,由于在不同性质的等温线超临界地区,性能系数达到较低的蒸发温度排放压力的较高最大值如下图4所示。
在本研究中,被发现影响系统内部换热效率的性能系数和最佳压力是微不足道的。
同在冷却器出口温度变化的有效性35℃和蒸发器温度0℃,可以忽略不计最大的性能系数和最佳的压缩机排气压力所发生的变化。
因此,内部散热性能换热器性能在低系统优化的较小影响和适度的气体冷却器出口温度。
如前所述,在冷却器出口的变化温度上产生重大影响的优化设计条件。
最大的系统性能系数急剧增加伴随冷却器出口温度的下降是显而易见的,如图 5。
蒸发温度为0℃和60%的内部热交换效力,系统的性能系数几乎增加了一倍,出口温度由50℃下降至30℃和相应所需的最佳压力从122bar下降到74bar。
图11代表所有的不同的不可逆降压部件在蒸发器指定零摄氏度的条件下具有不同的百分比,气体冷却器入口的温度在35摄氏度并且内部热交换器的效率在60%。
据研究,不可逆性对换热效率的影响比较小。
可能观察的到,蒸发器和冷凝器曲线性质的不同是由于两种不同的假设。
附近最佳的排气压力是在压缩机和蒸发器不可逆性最大时但是却在气体冷却器不可逆性最小的时候。
随着排气压力的增加,空气冷却器不可逆性的增加时由于有效温差在气体冷却器和热交换器中不同并且蒸发器不可逆性的减少时由于蒸发量的增加。
压缩机的不可逆性主要取决于压缩机的等熵效率,因此适当的压缩机设计可以减少这种不可逆性。
为了减少蒸发器的不可逆性,蒸发器的换热装置被设计成这样,流体之间的温差尽可能保持的很小。
可以看出,在所有部件中膨胀阀的不可逆性的百分比是最高的。
因此,存在这样一个机会通过用涡轮代替等焓膨胀阀从膨胀过程中提取功(至少要在大容量系统中)。
对于最佳的系统优化,能量和用流的每单位工作的投入(或100%)在蒸发温度为280K,冷却器出口温度在310K,内部热交换器的效率为80%,最佳排气压力为90bar,环境温度为300K,如图12所示。
能量流图表示COP值为8.08。
如前所述,通过膨胀装置的拥损比较大是由于在两边的压差比较大同时也是由于二氧化碳独特的性质,在临界点附近的熵及其性质的突然改变(如压力突然从超临界下降至临界)。
在内部换热器的拥损大概在4%。
在上述条件下,与气体冷却器相比蒸发器的不可逆性更大,这是因为在现有的蒸发器中存在着比气体冷却器更大温差。
6 结论系统模型和跨临界二氧化碳计算机仿真同时加热和冷却的内部热交换器系统已经开发出来。
在系统的优化和结果的基础上,可以得出以下结论。
1、蒸发器温度效果和气体冷却器出口温度相比,更加突出在优化内部的热量交换效力系统的条件。
2、该系统可有效地用于工艺厂的地方从100℃加热到140℃(由于昼夜温差大滑行的气体冷却器)和同步冷却/冷凝的需要。