低热硅酸盐水泥改善大体积混凝土抗裂性能研究
外文翻译--低热硅酸盐水泥混凝土的抗裂性能

外文翻译Anti-Crack Performance of Low-HeatPortland Cement ConcreteAbstract: The properties of low-heat Portland cement concrete(LHC) were studied in detail. The experimental results show that the LHC concrete has characteristics of a higher physical mechanical behavior, deformation and durability. Compared with moderate-heat Portland cement(MHC), the average hydration heat of LHC concrete is reduced by about 17.5%. Under same mixing proportion, the adiabatic temperature rise of LHC concrete was reduced by 2 ℃-3℃,and the limits tension of LHC concrete was increased by 10×10-6-15×10-6than that of MHC. Moreover, it is indicated that LHC concrete has a better anti-crack behavior than MHC concrete. Key words: low-heat portland cement; mass concrete; high crack resistance; moderate-heat portland cement1 IntroductionThe investigation on crack of mass concrete is a hot problem to which attention has been paid for a long time. The cracks of the concrete are formed by multi-factors, but they are mainly caused by thermal displacements in mass concrete[1-3]. So the key technology on mass concrete is how to reduce thermal displacements and enhance the crack resistance of concrete.As well known, the hydration heat of bonding materials is the main reason that results in the temperature difference between outside and inside of mass concrete[4,5]. In order to reduce the inner temperature of hydroelectric concrete, several methods have been proposed in mix proportion design. These include using moderate-heat portland cement (MHC), reducing the content of cement, and increasing the Portland cement (OPC), MHC has advantages such as low heat of hydration, high growth rate of long-term strength, etc[6,7]. So it is more reasonable to use MHC in application of mass concrete.Low-heat portland cement (LHC), namely highbelite cement is currently attracting a great deal of interest worldwide. This is largely due to its lower energy consumption and CO2 emission in manufacture than conventional Portland cements.LHC has a lot of noticeable properties, such as low heat of hydration excellent durability, etc, so the further study continues to be important[8-10]. The long-term strength of C2S can approach to or even exceed that of C3S[11]. In addition, C2S has a series of characteristics superior to C3S. These include the low content of CaO, low hydration heat, good toughness, compact hydration products, excellent resistances to chemical corrosion, little dry shrinkage, etc[12,13].For hydroelectric concrete , the design requirements have some characteristics, such as long design age, low design strength, low hydration temperature rise, and low temperature gradient[14]. All these requirements agree with the characteristics of LHC. Furthermore, LHC has a high hydration activity at later ages, the effect of which can improve the inner micro-crack. Based on above-mentioned analyses, the properties of low-heat Portland cement concrete were studied in detail in this paper. Compared with the moderate-heat Portland cement (MHC) concrete, the anti-crack behavior of LHC concrete was analyzed.2 ExperimentalMHC was produced in Gezhouba Holding Company Cement Plant, China; and LHC was produced in Hunan Shimen Special Cement Co. Ltd., China. The chemical compositions and mineral compositions of cement are listed in Table 1 and Table 2 respectively, and the physical and mechanical properties of cement are listed in Table 3.In spite of a little difference in chemical compositions, there is an obvious dissimilarity between the mineral component of LHC and that of MHC because of the different burning schedule. The C3S (Alite) content of MHC is higher than that of LHC, and the C2S (Belite) content of LHC is higher than that of MHC. Alite is formed at temperatures of about 1 450 ℃, while Belite is formed at around 1 200 ℃. Therefore, LHC can be manufactured at lower kiln temperatures than MHC. And the amount of energy theoretically required to manufacture LHC is lower than that of MHC.Belite hydrates comparatively slowly, and the early compressive strengths of pastes, mortars, and concretes containing LHC are generally lower as a result. The long-term strength and durability of concrete made from LHC can potentially exceed those of MHC. The results from Table 3 show that the early strength of LHC pastes is lower than that of MHC pastes, and that the strength growth rate of LHC is higher than that of MHC.The hydration heat of bonding materials was tested. Class I fly ash of bonding materials came from Shandong Zhouxian Power Plant, China. The experimental results shown in Table 4 indicate that the hydration heat of LHC is much lower than that of MHC. The 1-day, 3-day and 7-day hydration heat of LHC without fly ash is 143 kJ/kg, 205 kJ/kg, 227 kJ/kg, respectively. The 1-day, 3-day and 7-day hydration heat of MHC without fly ash is 179 kJ/kg, 239 kJ/kg, 278 kJ/kg, respectively. Compared with MHC, the average hydration heat of LHC concrete is reduced by about 17.5%. Obviously, low hydration is of advantage to abate the pressure to temperature control, and to reduce the crack probability due to the temperature gradients. The adiabatic temperature of LHC concrete and MHC concrete was tested. As a result, the adiabatic temperature rise of LHC concrete is lower than that of MHC concrete and the different value ranges from 2 ℃to 3 ℃in general.After adding fly ash, all specimens show a lower hydration heat, and it decreases with increasing fly ash content. For MHC with 30% fly ash, the 1 d, 3 d, 7d accumulative hydration heat is reduced by 14.5%, 20.5%, 21.9%, respectively; and for LHC with 30% fly ash, the 1 d, 3 d, 7 d accumulative hydration heat is reduced by 21.7%, 26.3%, 23.3%, respectively. Obviously, the effect of fly ash on the hydration heat of LHC is more than that of MHC. It is well known that the fly ash activation could be activated by Ca(OH)2. LHC has a lower content of C3S and a higher content of C2S than MHC, so the Ca(OH)2, namely the exciter content in hydration products of LHC pastes is lower. Decreasing the hydration activation of fly ash reduces the hydration heat of bonding materials.3 Results and DiscussionIn this experiment, ZB-1A type retarding superplasticizer and DH9 air-entraining agent were used. The dosage of ZB-1 was 0.7% by the weight of the blending, and the dosage of DH9 was adjusted to give an air-containing of 4.5% to 6.0%. The parameters that affected the dosage included the composition and the fineness of thecement used, and whether the fly ash was used. Four gradations of aggregate were used, 120 mm-80 mm: 80 mm-40 mm: 40 mm-20 mm: 20 mm-5 mm=30:30:20:20.The term water-to-cementitious was used instead of water-to-cement, and the water-to-cementitious ratio was maintained at 0.50 for all the blending. The slump of concrete was maintained at about 40 mm, and the air content was maintained at about 5.0% in the experimental. After being demoulded, all the specimens were in a standard curing chamber. The mix proportion parameter of concrete is listed in Table 5.3.1 Physical and mechanical propertiesThe physical and mechanical properties include strength, elastic modulus, limits tension, and so on. The results of strength shown in Table 6 indicate the early strength (7 d curing ages) of LHC (odd samples) concrete increases slowly. The ratio between 7 d compressive strength and 28 d compressive strength of LHC concrete is about 0.4, while for MHC concrete the ratio is about 0.6. Compared with MHC concrete, the growth rate of strength of LHC concrete becomes faster after 7 d curing ages. The compressive strength for 28 d, 90 d, 180 d curing ages of LHC concrete containing 20% of fly ash is 30.2 MPa, 43.8 MPa, 48.5 MPa, respectively, while that of MHC concrete containing 20% of fly ash is 28.3 MPa, 35.6 MPa, 39.8 MPa, respectively. The content of C2S in LHC is higher than that in MHC, which results in the above-mentioned difference.Table 6 shows that the strength growth rate of concrete made with fly ash blended cements is higher than that of blank specimens; the more the dosage of fly ash, the higher the growth rate. Fly ash has a glassy nature, which can react with Ca(OH)2. Since Ca(OH)2 is a hydration product of cement, the reaction between fly ash and Ca(OH)2, called “secondary hydration”, will happen at latish ages. The magnitude of Ca(OH)2 is affected by some factors, such as the water-to-cementitious,the dosage of cement.The elastic modulus and the limits tension of concrete are given in Table 7. Under same mixing proportion, the elastic modulus of LHC concrete is approximately equal to that of MHC; the 28-day limits tension of LHC concrete is increased by 10×10-6 to 15 ×10-6 than that of MHC, and the 90-day limits tension of LHC concrete is increased by 12×10-6 than that of MHC concrete. The above results show that the use of LHC improves the limits tension of concrete. Increasing the limits tension of concrete will be benefit to the crack resistance of concrete.3.2 Deformation characteristicsDeformation characteristics of concrete include drying shrinkage, autogenous deformation, creep, etc. The drying shrinkage of concrete is shown in Fig.1. The drying shrinkage increases with age. At early ages a up to 90 days, all the LHCconcrete specimens show a lower drying shrinkage; and it decreases with increasing the fly ash content. When containing 30% of fly ash, the drying shrinkage of LHC concrete is 363 ×10-6 at 90 days, while for MHC concrete the value is 408×10-6. As a result, the volume stability of LHC concrete is better than that of MHC concrete in drying environment.Experiment results of autogenous deformation of concrete are given in Fig.2. There is an obvious difference between the development of autogenous deformation of LHC concrete and that of MHC concrete. The autogenous deformation of LHC concrete has an expansive tendency. At early ages up to 14 days, the autogenous deformation of pure LHC samples increases with age, and the 14-day value reaches a peak of 20×10-6. The autogenous deformation of pure LHC samples decreases with age at 14 days to 90 days, and the 90-day value is 10×10-6. After adding 30% of fly ash, the autogenous deformation of LHC concrete increases with age, and the 90-day value is 61×10-6. The autogenous deformation of MHC concrete has a tendency to shrink, especially without fly ash.3.3. DurabilityThe durability of concrete is evaluated by antipenetrability grade and frost-resistant level. Under the pressure of 1.2 MPa, the permeability height of pure LHC samples is 3.1 cm, while that of pure MHC samples is 2.0 cm. The test dataindicate that the LHC concrete has an excellent performance in anti-penetrability, as well as MHC concrete. The permeability of concrete increases somewhat with addition of fly ash. At the end of the 250 freezing and thawing cycling, there is a little difference in both mass and resonant frequency. Both LHC concrete and MHC concrete show an excellent frost-resistant behavior. The results of this work confirm that LHC concrete systems have an adequate anti-penetrability and frost-resistance to adapting design requirement.3.4 Analysis of crack resistanceIn order to control the crack phenomena, it is important to accurately evaluate the anti-crack behavior.As well known, concrete is a kind of typical brittle materials, and its brittleness is associated with the anti-crack behavior[15]. The brittleness is measured by the ratio of tension strength to compressive strength. With the increase of the ratio, concrete has a less brittleness, better crack resistance and toughness. It is indicated from the experiment results shown in Table 6 that the ratio of LHC concrete at all stages of hydration is higher than that of MHC concrete, which shows that LHC concrete has a better anti-crack behavior.In the crack control and design of hydroelectric mass concrete, the original evaluation of crack resistance behavior of concrete is using the utmost tensile strength which is shown in the following expression of Eq.1.σ=εP E (1)where, εP is the limits tension of concrete, and E is the elastic modulus of tension, which is assumed to be equal to the elastic modulus of compression[16].It is indicated from the calculation results shown in Table 8 that the utmost tensile strength of LHC concrete at all stages of hydration is higher than that of MHC cncrete.The research on materials crack resistance which is the basis for esign, construction and the choice of raw materials, has been popular in today’s world. Through a great deal of research, it is widely thought that concrete with a better crack resistance has a higher tension strength and limits tension, lower elastic odulus and adiabatic temperature rise and better volume stability[17,18].Based on above-mentioned results, the LHC concrete has a higher tension strength and limits tension, lower elastic modulus and adiabatic temperature rise, and lower drying shrinkage than MHC concrete. Compared with MHC concrete, the autogenous deformation of LHC concrete has an expansive tendency. Although the early strength of LHC concrete is lower than that of MHC concrete, its later strength has approached to or even exceed that of MHC concrete.4 Conclusionsa) The early compressive strength (7 d curing ages) of LHC is lower, but its later strength (28 d, 90 d curing ages) has approached to or even exceed that of MHC.b) Compared with MHC, the average hydration heat of LHC concrete is reduced by about 17.5%.c) Under the same mixing proportion, the elastic modulus of LHC concrete is approximately equal to that of MHC, and the limits tension of LHC concrete is increased by 10×10-6-15×10-6 than that of MHC.d) The drying shrinkage of LHC concrete is obviously smaller than that of MHC concrete, and the autogenous deformation of LHC concrete has a tendency to expand.e ) The LHC concrete has a better anti-penetrability and frost resistance, as well as the MHC concrete.f) At all stages of hydration, the anti-crack strength of LHC concrete is higher than that of MHC concrete, and the former has a higher ratio of tension strength to compressive strength.References[1] C X Yu, Z Kong. Research on the Causes of Cracks in Mass Concrete and Control Measures [J]. Low Temperature Architecture Technology (China), 2005 (5): 112-113 [2] A A Almusallam, M Maslehuddin. Effect of Mix Proportions on Plastic Shrinkage Cracking of Concrete in Hot Environments[J].Construction and Building Materials, 1998 (12): 353-358[3] Xu Jing’an, An Zhiwen. Countermeasure of Temperature Crack of Mass Concrete[J]. Journal of Hebie Institute of Architectural Engineering, 2005,23(3):36-40[4] Peng Weibing, Ren Aizhu. Effects and Evaluation on Cracking of Concrete Incorporating Supplementary Cementitious Materials[J]. Concrete (China), 2005 (6): 50-64[5] Xiao Reimin, Zhang Xiong. Effect of Binder on Drying Shrinkage of Concrete [J].China Concrete and Cement Products, 2002 (5): 11-13[6] Ye Qing, Chen Xin. Research on the Expansive Mechanism of Moderate Heat Portland Cement with Slight Expansion [J].Journal of the Chinese Ceramic Society, 2000, 128 (4):335-347[7] Shi Xun. Application of Slight Expansion Cement on Concrete of Stage II Works of the Three Gorges Project [J]. Cement (China). 2002 (5): 12-14[8] Nagaokas, Mizukosui M. Property of Concrete Using Beliterich Cement and Ternary Blended Cement [J]. Journal of the Society of Materials Science, Japan, 1994, 43 (491): 488-492[9] Ge Juncai. Technology Progress of Cement and Concrete [M]. Beijing: China Building Material Industry Press , 1993:275-276[10] Metha P K. Investigation on Energy-saving Cement[J]. World Cement Technology, 1980, 1(3): 166-177[11] Taylor. Cement Chemistry[M]. London: Academic Press, 1990:142-152[12] Sui Tongba, Liu Kezhong. A Study on Properties of High Belite Cement [J]. Journal of the Chinese Ceramic Society, 1999, 127 (4): 488-492[13] Yang Nanru, Zhong Baixi. Study on Active -C2S[C]. Symposium onCement,1983:180-185[14] Yang Huanquan, Li Wenwei. Research and Application of Hydroelectric Concrete[M]. Beijing, China Water Power Press,2004:393-394[15] E Ringot, A Bascoul. About the Analysis of Micro-cracking in Concrete[J]. Cement and Concrete Composites, 2001 (23):261-266[16] Li Guangwei. Assessment for Anti-Crack Performance of Concrete [J]. Advances in Science and Technology of Water Resources (China), 2001, 21 (2): 33-36[17] Liu Shuhua, Fang Kunhe. Summarization of Norm of Crack Resistance of Concrete[J]. Highway (China), 2004 (4): e[J] 105-107低热硅酸盐水泥混凝土的抗裂性能摘要:低热硅酸盐水泥混凝土(LHC)的特性详细地被研究。
低热硅酸盐水泥在闸墩混凝土中的应用研究
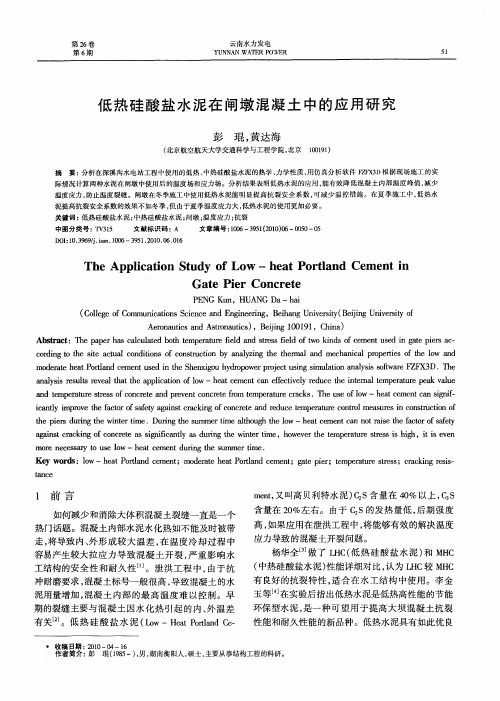
ia t mp o e t efco fsf t a an t r c ig o o ce ea d r d c mp r tr o t l a u e o sr ci f e l i rv tr e g is ca kn f n rt n u et eau c n r s r si c n t t n o n y h a o a y c e e e o me n u o
a d tmp r tr t s fc n r t d p e e tc n r t r m e e au ec a k n e eau e sr so o c e a rv n o cee f e e n o tmp rt r rc s.T e u e o w— h a e n a inf h s fl o e tc me tc n sg i-
D I1 .99jin 10 O :0 36 /. s.06—35 .00 0 .1 s 9 12 1 .6 06
Th p i a i n S u y o w — h a r l n m e t i e Ap l to t d fLo — e t Po t d Ce n n c a Ga e Pi r Co c e e t e n r t
第2 6卷 第 6期
云南水力发 电
YU NNAN W_TE P WER A R O 5 l
低 热硅 酸 盐 水 泥在 闸墩 混 凝 土 中的 应 用 研 究
低热膨胀水泥用于高强度混凝土中的性能研究
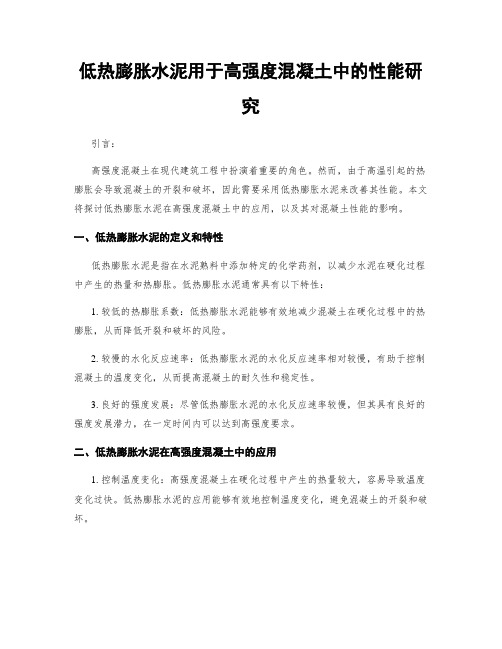
低热膨胀水泥用于高强度混凝土中的性能研究引言:高强度混凝土在现代建筑工程中扮演着重要的角色。
然而,由于高温引起的热膨胀会导致混凝土的开裂和破坏,因此需要采用低热膨胀水泥来改善其性能。
本文将探讨低热膨胀水泥在高强度混凝土中的应用,以及其对混凝土性能的影响。
一、低热膨胀水泥的定义和特性低热膨胀水泥是指在水泥熟料中添加特定的化学药剂,以减少水泥在硬化过程中产生的热量和热膨胀。
低热膨胀水泥通常具有以下特性:1. 较低的热膨胀系数:低热膨胀水泥能够有效地减少混凝土在硬化过程中的热膨胀,从而降低开裂和破坏的风险。
2. 较慢的水化反应速率:低热膨胀水泥的水化反应速率相对较慢,有助于控制混凝土的温度变化,从而提高混凝土的耐久性和稳定性。
3. 良好的强度发展:尽管低热膨胀水泥的水化反应速率较慢,但其具有良好的强度发展潜力,在一定时间内可以达到高强度要求。
二、低热膨胀水泥在高强度混凝土中的应用1. 控制温度变化:高强度混凝土在硬化过程中产生的热量较大,容易导致温度变化过快。
低热膨胀水泥的应用能够有效地控制温度变化,避免混凝土的开裂和破坏。
2. 提高抗裂性能:高强度混凝土对抗拉力的能力较弱,容易产生裂缝。
低热膨胀水泥可以减少混凝土的热膨胀,提高其抗裂性能,从而增加混凝土的耐久性和承载能力。
3. 改善耐久性:低热膨胀水泥的应用能够降低混凝土的温度变化幅度,避免由于温度变化引起的开裂和破坏,从而改善混凝土的耐久性。
三、低热膨胀水泥对混凝土性能的影响1. 抗压强度:研究显示,使用低热膨胀水泥的高强度混凝土具有较高的抗压强度。
这是由于低热膨胀水泥的水化产物具有较好的结晶状态,能够填充混凝土中的孔隙,提高其密实度和强度。
2. 抗裂性能:低热膨胀水泥的应用能够减少混凝土中的热膨胀,改善其抗裂性能。
研究发现,使用低热膨胀水泥的高强度混凝土在热循环和湿热环境下具有较好的耐久性,裂缝和微裂缝的发展速度较慢。
3. 水化反应速率:低热膨胀水泥的水化反应速率较慢,有助于控制混凝土的温度变化。
低热硅酸盐水泥用于大坝建设的研究
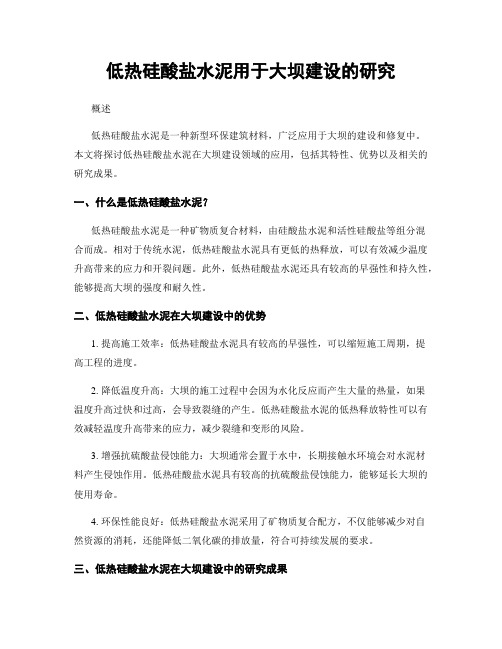
低热硅酸盐水泥用于大坝建设的研究概述低热硅酸盐水泥是一种新型环保建筑材料,广泛应用于大坝的建设和修复中。
本文将探讨低热硅酸盐水泥在大坝建设领域的应用,包括其特性、优势以及相关的研究成果。
一、什么是低热硅酸盐水泥?低热硅酸盐水泥是一种矿物质复合材料,由硅酸盐水泥和活性硅酸盐等组分混合而成。
相对于传统水泥,低热硅酸盐水泥具有更低的热释放,可以有效减少温度升高带来的应力和开裂问题。
此外,低热硅酸盐水泥还具有较高的早强性和持久性,能够提高大坝的强度和耐久性。
二、低热硅酸盐水泥在大坝建设中的优势1. 提高施工效率:低热硅酸盐水泥具有较高的早强性,可以缩短施工周期,提高工程的进度。
2. 降低温度升高:大坝的施工过程中会因为水化反应而产生大量的热量,如果温度升高过快和过高,会导致裂缝的产生。
低热硅酸盐水泥的低热释放特性可以有效减轻温度升高带来的应力,减少裂缝和变形的风险。
3. 增强抗硫酸盐侵蚀能力:大坝通常会置于水中,长期接触水环境会对水泥材料产生侵蚀作用。
低热硅酸盐水泥具有较高的抗硫酸盐侵蚀能力,能够延长大坝的使用寿命。
4. 环保性能良好:低热硅酸盐水泥采用了矿物质复合配方,不仅能够减少对自然资源的消耗,还能降低二氧化碳的排放量,符合可持续发展的要求。
三、低热硅酸盐水泥在大坝建设中的研究成果1. 抗渗性研究:使用低热硅酸盐水泥可以有效提高大坝的抗渗性能。
研究表明,在抗渗性能方面,低热硅酸盐水泥相比传统水泥具有更好的表现,可以降低溶解度和渗透系数,减少水分渗入的风险。
2. 抗冻融性研究:冻融循环是大坝建设中常见的问题,低热硅酸盐水泥在抗冻融性方面表现出色。
研究发现,采用低热硅酸盐水泥可以显著降低冻融循环对混凝土的损害程度,延长大坝的使用寿命。
3. 硅酸盐反应性研究:硅酸盐反应是大坝建设中一个重要的问题,可能导致混凝土膨胀和龟裂。
研究表明,低热硅酸盐水泥具有较低的硅酸盐反应性,能够减少膨胀和龟裂的风险。
结论低热硅酸盐水泥作为一种新型环保建筑材料,在大坝建设领域具有广泛应用前景。
白鹤滩水电站低热水泥混凝土技术研究

白鹤滩水电站低热水泥混凝土技术研究摘要:国内外水电站无全坝使用低热水泥混凝土浇筑的案例,无类似施工经验可供参考,采用灰岩骨料和低热水泥,通过原材料的选用及配合比的优化,以及低热水泥与中热水泥混凝土试验性能的比较,结果表明低热水泥具有水化热低、混凝土后期性能接近或超过中热硅酸盐水泥等特点,可改善大体积混凝土的抗裂性能,低热硅酸盐水泥在白鹤滩大坝主体混凝土中的全面应用,为后续低热水泥在大坝混凝土中的广泛应用积累经验。
关键词:低热水泥;混凝土;施工技术1引言近年来,低热硅酸盐水泥已在三峡、溪洛渡、向家坝等水电站局部工程部位得到使用,低热硅酸盐水泥在白鹤滩大坝主体混凝土中的全面应用,特别是在300m级特高双曲拱坝混凝土中的使用尚属首次。
如何面对混凝土方量巨大,施工强度高,干湿季分明,高温、大风、日照强、温差大等不利条件下确保混凝土施工质量,混凝土配合比优化及确定尤为重要。
2原材料及配合比设计2.1原材料试验研究分别采用四川嘉华“隆冠”牌P•LH42.5水泥和华新“堡垒”牌P•MH42.5水泥。
粉煤灰为曲靖Ⅰ级粉煤灰。
粗细骨料为旱谷地生产的灰岩人工碎石、砂。
外加剂分别采用JM-Ⅱ缓凝高效减水剂和GYQ-Ⅰ引气剂,以及浙江龙游ZB-1G引气剂。
检测结果表明均满足各相关标准要求。
通过试验可知嘉华低热水泥3d和7d龄期强度明显低于华新中热水泥,28d龄期抗压强度与中热水泥相近,而90d龄期抗压强度高于后者。
嘉华低热水泥3d龄期水化热较华新中热水泥低49kJ/kg,7d龄期低50kJ/kg。
2.2大坝混凝土配合比优化根据低热水泥混凝土配合比试验结果,初步确定的大坝混凝土四级配施工配合比C18040F90300W9015,水胶比为0.42,砂率为23%,粉煤灰35%,用水量为79kg/m3减水剂为0.6%,引气剂为0.023%;C18035F90300W9014,水胶比为0.46,砂率为24%,粉煤灰35%,用水量为80kg/m3减水剂为0.5%,引气剂为0.020%;C18030F90250W9013,水胶比为0.50,砂率为25%,粉煤灰35%,用水量为80kg/m3减水剂为0.5%,引气剂为0.020%;根据优化方案,通过试验确定的优化大坝混凝土施工配合比C18040F90300W9015,水胶比为0.42,砂率为24%,粉煤灰35%,用水量为81kg/m3减水剂为0.7%,引气剂为0.035%;C18035F90300W9014,水胶比为0.46,砂率为25%,粉煤灰35%,用水量为82kg/m3减水剂为0.7%,引气剂为0.035%;C18030F90250W9013,水胶比为0.50,砂率为26%,粉煤灰35%,用水量为82kg/m3减水剂为0.7%,引气剂为0.035%;3大坝混凝土设计指标及配制强度3.1混凝土设计指标根据设计单位提供的白鹤滩水电站大坝坝体四级配混凝土设计指标C18040F90300W9015最大水胶比为0.42,C18035F90300W9014最大水胶比为0.46,C18030F90250W9013最大水胶比为0.50,最大掺合料掺量35%,保证率为85%。
低热膨胀水泥用作超高性能混凝土中的性能研究
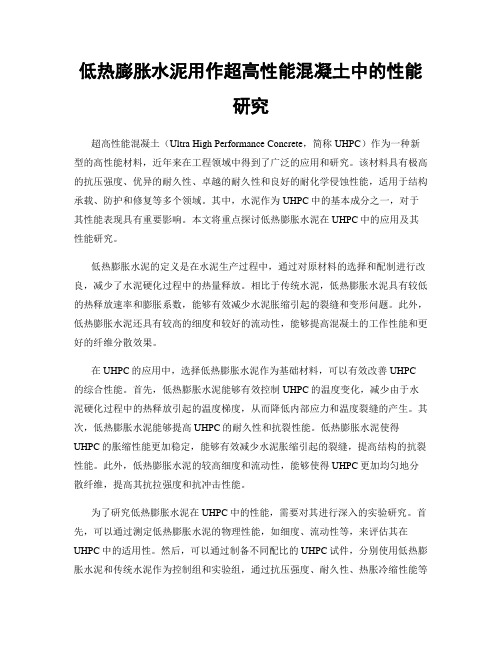
低热膨胀水泥用作超高性能混凝土中的性能研究超高性能混凝土(Ultra High Performance Concrete,简称UHPC)作为一种新型的高性能材料,近年来在工程领域中得到了广泛的应用和研究。
该材料具有极高的抗压强度、优异的耐久性、卓越的耐久性和良好的耐化学侵蚀性能,适用于结构承载、防护和修复等多个领域。
其中,水泥作为UHPC中的基本成分之一,对于其性能表现具有重要影响。
本文将重点探讨低热膨胀水泥在UHPC中的应用及其性能研究。
低热膨胀水泥的定义是在水泥生产过程中,通过对原材料的选择和配制进行改良,减少了水泥硬化过程中的热量释放。
相比于传统水泥,低热膨胀水泥具有较低的热释放速率和膨胀系数,能够有效减少水泥胀缩引起的裂缝和变形问题。
此外,低热膨胀水泥还具有较高的细度和较好的流动性,能够提高混凝土的工作性能和更好的纤维分散效果。
在UHPC的应用中,选择低热膨胀水泥作为基础材料,可以有效改善UHPC的综合性能。
首先,低热膨胀水泥能够有效控制UHPC的温度变化,减少由于水泥硬化过程中的热释放引起的温度梯度,从而降低内部应力和温度裂缝的产生。
其次,低热膨胀水泥能够提高UHPC的耐久性和抗裂性能。
低热膨胀水泥使得UHPC的胀缩性能更加稳定,能够有效减少水泥胀缩引起的裂缝,提高结构的抗裂性能。
此外,低热膨胀水泥的较高细度和流动性,能够使得UHPC更加均匀地分散纤维,提高其抗拉强度和抗冲击性能。
为了研究低热膨胀水泥在UHPC中的性能,需要对其进行深入的实验研究。
首先,可以通过测定低热膨胀水泥的物理性能,如细度、流动性等,来评估其在UHPC中的适用性。
然后,可以通过制备不同配比的UHPC试件,分别使用低热膨胀水泥和传统水泥作为控制组和实验组,通过抗压强度、耐久性、热胀冷缩性能等实验指标的对比分析,评估低热膨胀水泥对UHPC性能的影响。
此外,还可以对UHPC中低热膨胀水泥的微观结构进行观察和分析,以了解其对混凝土骨架结构、钢筋粘结性能等方面的影响。
低热硅酸盐水泥
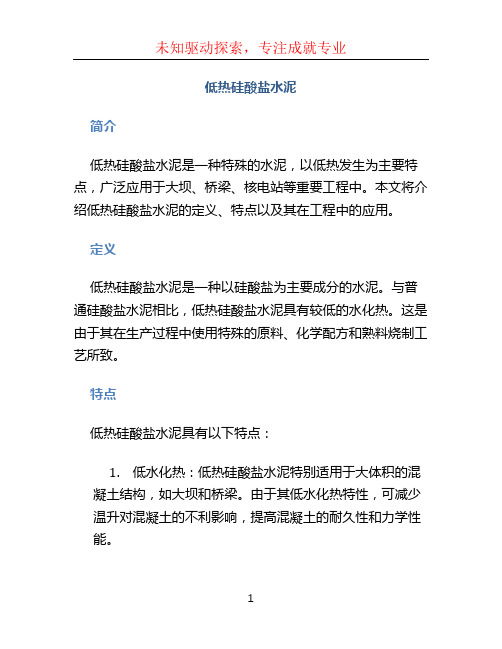
低热硅酸盐水泥简介低热硅酸盐水泥是一种特殊的水泥,以低热发生为主要特点,广泛应用于大坝、桥梁、核电站等重要工程中。
本文将介绍低热硅酸盐水泥的定义、特点以及其在工程中的应用。
定义低热硅酸盐水泥是一种以硅酸盐为主要成分的水泥。
与普通硅酸盐水泥相比,低热硅酸盐水泥具有较低的水化热。
这是由于其在生产过程中使用特殊的原料、化学配方和熟料烧制工艺所致。
特点低热硅酸盐水泥具有以下特点:1.低水化热:低热硅酸盐水泥特别适用于大体积的混凝土结构,如大坝和桥梁。
由于其低水化热特性,可减少温升对混凝土的不利影响,提高混凝土的耐久性和力学性能。
2.早强:低热硅酸盐水泥在水化过程中,能够产生更多的早期强度。
这使得低热硅酸盐水泥尤其适用于需要早期脱模或早期使用的工程项目。
3.抗裂性能:由于低热硅酸盐水泥具有较低的水化热,减少了温度应力的产生和累积,从而提高了混凝土的抗裂性能。
4.环境友好:低热硅酸盐水泥生产过程中采用特殊的原料和化学配方,减少了环境污染和资源消耗。
同时,由于其低热发生特性,也减少了对周围环境和工人的不良影响。
应用低热硅酸盐水泥广泛应用于以下工程项目中:1.大坝:大坝工程对混凝土的性能要求较高。
由于低热硅酸盐水泥具有低热发生和早强的特点,可避免大坝在水化过程中的温度应力积累,从而提高大坝的耐久性和稳定性。
2.桥梁:桥梁是承受车辆重载和气候变化等因素的工程项目。
低热硅酸盐水泥能够提供较高的早期强度,确保桥梁在早期使用阶段的安全性和稳定性。
3.核电站:核电站是对混凝土性能要求极高的工程项目。
低热硅酸盐水泥具有较低的水化热和优良的抗裂性能,能够减少混凝土在核电站运行期间的温度应力和裂缝产生,确保核电站的安全性和稳定性。
除了以上工程项目外,低热硅酸盐水泥还可以应用于需要较低水化热和高早期强度的其他工程项目中,如高速公路、隧道、海洋工程等。
结论低热硅酸盐水泥以其低水化热、早强、抗裂等特点,成为重要工程项目中的首选材料。
低热硅酸盐水泥在海工混凝土中的应用研究
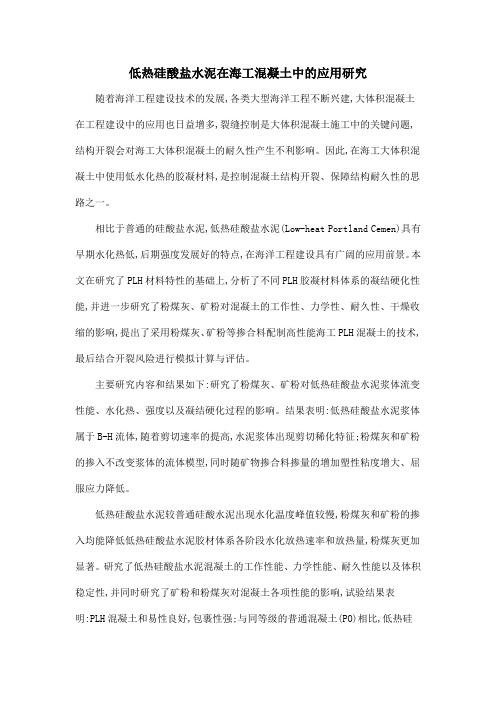
低热硅酸盐水泥在海工混凝土中的应用研究随着海洋工程建设技术的发展,各类大型海洋工程不断兴建,大体积混凝土在工程建设中的应用也日益增多,裂缝控制是大体积混凝土施工中的关键问题,结构开裂会对海工大体积混凝土的耐久性产生不利影响。
因此,在海工大体积混凝土中使用低水化热的胶凝材料,是控制混凝土结构开裂、保障结构耐久性的思路之一。
相比于普通的硅酸盐水泥,低热硅酸盐水泥(Low-heat Portland Cemen)具有早期水化热低,后期强度发展好的特点,在海洋工程建设具有广阔的应用前景。
本文在研究了PLH材料特性的基础上,分析了不同PLH胶凝材料体系的凝结硬化性能,并进一步研究了粉煤灰、矿粉对混凝土的工作性、力学性、耐久性、干燥收缩的影响,提出了采用粉煤灰、矿粉等掺合料配制高性能海工PLH混凝土的技术,最后结合开裂风险进行模拟计算与评估。
主要研究内容和结果如下:研究了粉煤灰、矿粉对低热硅酸盐水泥浆体流变性能、水化热、强度以及凝结硬化过程的影响。
结果表明:低热硅酸盐水泥浆体属于B-H流体,随着剪切速率的提高,水泥浆体出现剪切稀化特征;粉煤灰和矿粉的掺入不改变浆体的流体模型,同时随矿物掺合料掺量的增加塑性粘度增大、屈服应力降低。
低热硅酸盐水泥较普通硅酸水泥出现水化温度峰值较慢,粉煤灰和矿粉的掺入均能降低低热硅酸盐水泥胶材体系各阶段水化放热速率和放热量,粉煤灰更加显著。
研究了低热硅酸盐水泥混凝土的工作性能、力学性能、耐久性能以及体积稳定性,并同时研究了矿粉和粉煤灰对混凝土各项性能的影响,试验结果表明:PLH混凝土和易性良好,包裹性强;与同等级的普通混凝土(PO)相比,低热硅酸盐混凝土的早期强度(7d)偏低,但随着龄期的增长,强度不断提高,当养护龄期达56d、90d时,PLH的抗压强度比PO增长了103.7%、116.4%;单掺粉煤灰和矿粉时,早期强度较低,但后期强度增幅大;当10%粉煤灰+20%矿粉复掺时,混凝土工作性能和强度都得到了改善;PLH混凝土在早期氯离子扩散系数高于PO混凝土,但随着养护龄期增长,其氯离子扩散系数逐渐减小并低于PO混凝土;混凝土中掺入一定量的粉煤灰和矿粉时,可进一步降低混凝土氯离子扩散,后期效果显著;粉煤灰和矿粉的掺入均降低了混凝土各龄期的干缩率;当粉煤灰和矿粉复掺时,混凝土孔结构得到细化,硬化混凝土的密实度提高。
- 1、下载文档前请自行甄别文档内容的完整性,平台不提供额外的编辑、内容补充、找答案等附加服务。
- 2、"仅部分预览"的文档,不可在线预览部分如存在完整性等问题,可反馈申请退款(可完整预览的文档不适用该条件!)。
- 3、如文档侵犯您的权益,请联系客服反馈,我们会尽快为您处理(人工客服工作时间:9:00-18:30)。
金属 的体 积大 得多 , 铁锈 体积膨 胀 , 对周 围混凝 土产 生 挤压 , 使混 凝土 胀裂 。 ( )有 的混凝 土虽 然 设 计抗 裂 强 度 高 , 由于 原 8 但
超 出混凝 土 的抗 裂 能力 时 , 就会 出现 裂缝 。 ( )混凝 土塑性 塌落 引起 的裂缝 。 2
1 大 体 积 混 凝 土 出现 裂 缝 的 原 因及 处 理 措 施
1 1 出现 裂 缝 的原 因 。
( )温 度 应 力 产 生 的 裂 缝 。混 凝 土 在 硬 化 期 间 经 1
( )当结构 的基 础 出 现不 均 匀 沉 陷 , 有 可 能产 6 就
生 裂缝 , 随着 沉 陷的进一 步 发展 , 裂缝会 进 一步扩 大 。
第4 卷 第 l l 8期 2 0 10 年 9 月 文 章 编 号 :0 1— 1 9 2 1 ) 8— 0 3— 3 10 47 (0 0 1 0 7 0
人 民 长 江
Ya g z Rie n te vr
Vo . 1 41. No. 8 1 S p., e 2 0 01
( )混凝 土加 水 拌 和后 , 泥 中 的碱 性 物质 与 骨 3 水 料 中活性 氧化 硅 等起 反应 , 出 的胶 状 碱 一硅胶 从 周 析 围介 质 中吸水膨 涨 , 体积 增大 3倍 , 而使混 凝土涨 裂 从 产生 裂缝 。
复杂 、 资大 、 投 一旦 发 生 质 量 问题 , 果 严 重 。而 目前 后 大 体积混 凝土 由于施 工 中 的各种 原 因易 产 生 裂缝 , 严 重 危害工 程质 量 , 大体 积混凝 土施 工 的技 术难 点 。 是 本文结 合 四川大 渡 河 深溪 沟 水 电站 工程 , 大体 就
收 稿 日期 :0 0— 7— 0 2 1 0 3
作 者 简 介 : 建 国 , , 程 师 , 要 从 事水 泥及 外加 剂研 发 工作 。E— i hng9 5 13 cn 韩 男 工 主 ma :aj17 @ 6 .o l
7 4
人 民 长 江
材 料质地 不均 匀 、 灰 比不 稳定 以及 运 输 和浇 筑 过程 水 中的离析 现象 , 同一 块体 混 凝 土 中其 抗拉 强 度 存 在不 均匀 现象 , 存在 易 温 的温 度 变 化过 程 , 泥 产 生 水
( )当钢筋 混凝 土处 于不利 环境 中 , 如 , 蚀性 7 例 侵
水 , 于 混 凝 土 保 护 层 厚 度 有 限 , 别 是 当 混 凝 土 密 实 由 特
大量水化 热 , 内部温 度不 断上 升 , 在混 凝 土表面 引起拉 应 力 。后 期在 降温 过程 中 , 由于 受 到 基础 或 老 混 凝 土
低 热硅 酸 盐 水 泥 改 善 大体 积 混 凝 土 抗 裂 性 能 研究
韩 建 国 吕鹏 飞 阳 运 霞 吴 勇 , , ,
(. 1 四川 嘉 华 企 业 ( 团 ) 份 有 限 公 司 , 集 股 四川 乐 山 6 4 0 ; 2 国 电 大 渡 河 深 溪 沟 水 电 有 限 公 司 , 1 0 3 . 四川 汉源 6 5 0 ) 23 0
效作 初步探 讨 。
束 , 往往 产生 裂缝 。 也
( )构 件超 载产 生 的裂缝 , 如 , 5 例 构件 在超 出设 计 的均 布荷 载或集 中荷 载 作 用 下 产 生 内力 弯 矩 , 出现 垂 直 于构 件纵 轴 的裂缝 , 构件 在较 大剪 力作用 下 , 产生斜 裂 缝 , 向上 、 延伸 。 并 下
摘 要 : 结 分 析 了大 体 积 混 凝 土 易产 生 裂 缝 的 原 因 及 应 对 措 施 , 出使 用 低 热 硅 酸 盐 水 泥 可有 效 改 善 大体 积 总 指
混凝 土 的抗 裂性 能 。选 取 低 热 硅 酸 盐 水 泥 与 普 通 硅 酸 盐 水 泥 开 展 试 验 对 比分 析 , 水 化 热 、 压 强度 、 凝 土 从 抗 混 收 缩膨 胀 等 方面 阐述 了低 热 硅 酸 盐 水 泥 有 利 于提 高混 凝 土 抗 裂 能 力 的 原 因。 并 通 过 瀑 布 沟 水 电站 工 程 和 深 溪 沟水 电站 工程 的 实例 , 明 了将 低 热 水 泥 应 用 于 水 工 大 体 积 混 凝 土是 可 行 的 , 具 有 很 好 效 果 。 说 且
于 2 3k/ g 因此 , 了控制低 热硅 酸盐水 泥具 有较 9 J k 。 为
性不 良时 , 环境 中的氯 离 子 等 和溶 于 水 中的 氧会 使 混
凝 土 中 的 钢 筋 生 锈 , 成 氧 化 铁 , 化 铁 的 体 积 比原 来 生 氧
的约束 , 又会在 混凝 土 内部 出现 拉 应 力 。气 温 的 降低
也 会 在 混 凝 土 表 面 引 起 很 大 的 拉 应 力 , 这 些 拉 应 力 当
积 混凝土 出现裂 缝 的主要原 因及 现有 的抗裂 措施 进行
( )许多 混凝 土虽 然 内部湿度 变 化很小 或变 化较 4
慢 , 表 面湿度 可能 变化 较大 或发 生剧烈 变化 , 但 如养 护 不 当 、 干时湿 , 面干缩 变形 受到 内部 混凝 土体 的约 时 表
分析, 并结 合低 热硅 酸盐水 泥 的 自身性 能特 点 , 采用 对 低热 硅酸盐 水泥 配制 大体积 混凝 土减 少裂缝 危 害的成
关 键 词 : 热硅 酸 盐 水 泥 ;大 体 积 混 凝 土 ;抗 裂性 能 ;水 化 热 低 文 献 标 志 码 :A
中 图法 分 类 号 :T 4 V3
大体 积混 凝土 广泛应 用于 水 电 、 电 、 核 桥梁 、 洞 、 隧
国 防 建 筑 物 等 重 要 工 程 , 些 工 程 由 于 工 程 量 大 、 工 这 施