defects in ZnO nanorods prepared by a hydrothermal method
磷酸铁锂的制备及其电化学性能
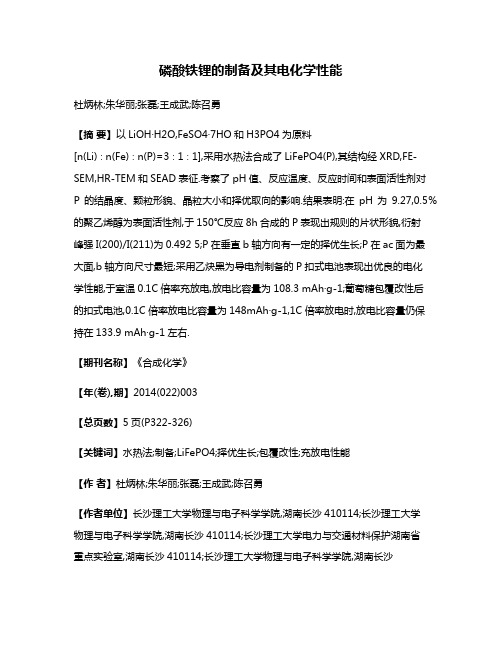
磷酸铁锂的制备及其电化学性能杜炳林;朱华丽;张磊;王成武;陈召勇【摘要】以LiOH·H2O,FeSO4·7HO和H3PO4为原料[n(Li)∶n(Fe)∶n(P)=3∶1∶1],采用水热法合成了LiFePO4(P),其结构经XRD,FE-SEM,HR-TEM和SEAD表征.考察了pH值、反应温度、反应时间和表面活性剂对P的结晶度、颗粒形貌、晶粒大小和择优取向的影响.结果表明:在pH为9.27,0.5%的聚乙烯醇为表面活性剂,于150℃反应8h合成的P表现出规则的片状形貌,衍射峰强I(200)/I(211)为0.492 5;P在垂直b轴方向有一定的择优生长;P在ac面为最大面,b轴方向尺寸最短;采用乙炔黑为导电剂制备的P扣式电池表现出优良的电化学性能,于室温0.1C倍率充放电,放电比容量为108.3 mAh·g-1;葡萄糖包覆改性后的扣式电池,0.1C倍率放电比容量为148mAh·g-1,1C倍率放电时,放电比容量仍保持在133.9 mAh·g-1左右.【期刊名称】《合成化学》【年(卷),期】2014(022)003【总页数】5页(P322-326)【关键词】水热法;制备;LiFePO4;择优生长;包覆改性;充放电性能【作者】杜炳林;朱华丽;张磊;王成武;陈召勇【作者单位】长沙理工大学物理与电子科学学院,湖南长沙410114;长沙理工大学物理与电子科学学院,湖南长沙410114;长沙理工大学电力与交通材料保护湖南省重点实验室,湖南长沙410114;长沙理工大学物理与电子科学学院,湖南长沙410114;长沙理工大学物理与电子科学学院,湖南长沙410114;长沙理工大学物理与电子科学学院,湖南长沙410114【正文语种】中文【中图分类】O614.8;O613.6针对磷酸铁锂(LiFePO4)的电导率和离子扩散率低两大缺陷,研究人员纷纷展开了大量深入的研究。
不同形貌的纳米氧化锌
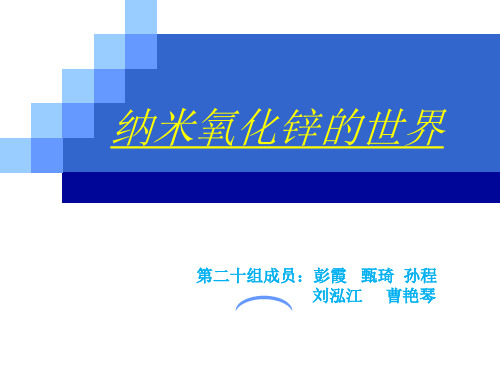
参考文献
1. Chan Yoon Jung, Jung Soo Kim etc. Journal of Colloid and Interface Science , 2012, 367: 257–263 2. Jing Ji, Masayashi Fuji, Hideo Watanabeb, Takashi Shirai. Colloids and Surfaces A: Physicochem Eng, 2012,393: 6– 10 3. SUN Ji-feng et al. Preparation of Bar-like Nano ZnO and Analysis on Its Photocatalytic Propert, J. Journal of Anhui Agri Sci, 2009, 37(27):12900-12901 4. Ko, S. H. et al. Nano-forest of hydrothermally grown hierarchical ZnO nanowires for a high efficiency dye-sensitized solar cell. Nano letters 11, 2011, 666-671 5. Law, M. Greene, L. E., Johnson, J. C. Saykally, R. & Yang, P. Nanowire dyesensitized solar cells. Nature materials 4, 2005, 455-459 6. Xiang Yang Kong, Zhong Lin Wang. Spontaneous Polarization-Induced Nanohelixes, Nanosprings, and Nanorings of Piezoelectric Nanobelts. Nano Letters, 2003, 3(12): 1625-1623 7.Maryam Movahedi, Elaheh Kowsari. A task specific basic ionic liquid for synthesis of flower-like ZnO by hydrothermal method [J]. Materials Letters, 2008, 62(23): 3856-3858
不同形貌硫化镍纳米材料的可控合成及电化学性能研究
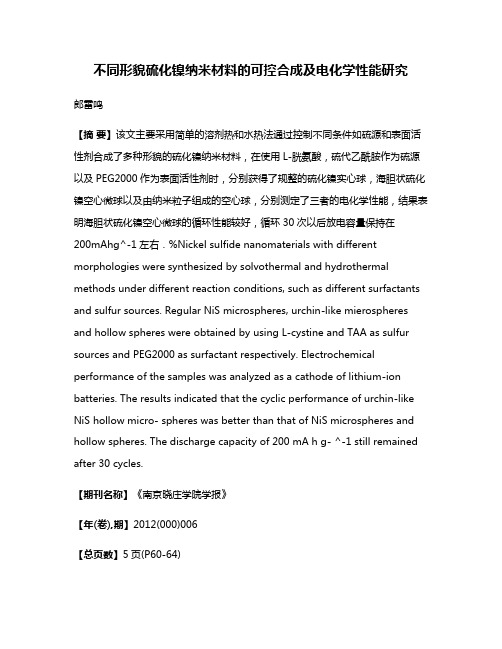
不同形貌硫化镍纳米材料的可控合成及电化学性能研究郎雷鸣【摘要】该文主要采用简单的溶剂热和水热法通过控制不同条件如硫源和表面活性剂合成了多种形貌的硫化镍纳米材料,在使用L-胱氨酸,硫代乙酰胺作为硫源以及PEG2000作为表面活性剂时,分别获得了规整的硫化镍实心球,海胆状硫化镍空心微球以及由纳米粒子组成的空心球,分别测定了三者的电化学性能,结果表明海胆状硫化镍空心微球的循环性能较好,循环30次以后放电容量保持在200mAhg^-1左右.%Nickel sulfide nanomaterials with different morphologies were synthesized by solvothermal and hydrothermal methods under different reaction conditions, such as different surfactants and sulfur sources. Regular NiS microspheres, urchin-like mierospheres and hollow spheres were obtained by using L-cystine and TAA as sulfur sources and PEG2000 as surfactant respectively. Electrochemical performance of the samples was analyzed as a cathode of lithium-ion batteries. The results indicated that the cyclic performance of urchin-like NiS hollow micro- spheres was better than that of NiS microspheres and hollow spheres. The discharge capacity of 200 mA h g- ^-1 still remained after 30 cycles.【期刊名称】《南京晓庄学院学报》【年(卷),期】2012(000)006【总页数】5页(P60-64)【关键词】硫化镍;可控合成;电化学【作者】郎雷鸣【作者单位】南京晓庄学院生物化工与环境工程学院,江苏南京211171【正文语种】中文【中图分类】O6140 引言低维结构的纳米材料由于具有独特的物理、化学和光电性能一直以来就受到学者们的关注.随着纳米材料的快速发展,已经成功合成出不同形貌的低维结构的纳米材料[1-6],这些材料在催化、药物传输、光学材料和电池材料等领域表现出极大的潜在应用价值[7-9].不同形貌和结构的材料在性能方面有很大的差异,进而表现出不同的实际应用价值,为了合成性能更为优越的纳米材料,实现对形貌和结构的可控合成是材料合成的关键,因此,材料的不同形貌和结构对其性能的影响一直是科研工作者关注的焦点.在众多的材料中,金属硫化物由于具有特殊的光学、磁学以及催化性质而成为研究的热点.硫化镍除了具有在临界温度时,高温相NiS由顺磁性的导体转变为反铁磁性的半导体这种特殊的性质外,它还在太阳能电池、加氢脱硫催化反应,以及光电导材料和锂电池电极材料等方面都有着广泛的应用[10],因而备受关注.目前,多种形貌的NiS纳米材料被相继合成出来,如纳米晶、纳米棒、三角状纳米棱柱、薄膜、空心球以及通过自组装方法获得的由纳米针或纳米片组成的三维花状或海胆状NiS微球[11-16].但以L-胱氨酸为硫源合成很规整的硫化镍纳米材料还未见报导,此外,系统研究各种因素对硫化镍形貌的影响以及对不同形貌硫化镍纳米材料电化学性能的研究都较为少见.本文主要采用简单的溶剂热法以L-胱氨酸为硫源成功合成了形貌规整的NiS微球,并研究了不同硫源、表面活性剂以及配体对硫化镍形貌的影响,以形貌较好的海胆状NiS空心微球,NiS实心微球和由纳米粒子组成的空心球为电极材料,对其进行了锂离子充放电性能测试,海胆状NiS微球显示出了较好的充放电性能和循环性能.1 实验部分1.1 硫化镍纳米材料的制备准确称取氯化镍和硫源各2 mmol,在磁力搅拌下溶于20 ml乙二醇中,待固体全部溶解后,加入2.0 ml乙二胺,搅拌片刻后将澄清透明溶液转移到高压釜中,将高压釜放入烘箱190℃加热反应24小时得尺寸均一的硫化镍纳米材料.自然冷却到室温,取出反应釜,在1000转/分的转速下离心分离产品并用无水乙醇洗涤产品3—4次.真空干燥后备用.在相同的实验方法下使用不同硫源和表面活性剂合成不同形貌的硫化镍微纳米材料.1.2 锂离子电池电极材料的制备和电化学性能测试将活性物质按NiS∶碳黑∶聚四氟乙烯(PTFE)=8∶1∶1(质量比)的比例均匀混合后,涂在宽度为8mm的铜箔表面,在100℃下真空干燥至少8 h,即可得工作电极.采用金属锂作为对电极,1 mol·L-1 LiPF6的碳酸乙烯(EC)、碳酸二甲酯(DMC)和碳酸二乙酯(DEC)的混合溶液(EC∶DMC∶DEC=1∶1∶1)作为电解液,在氩气保护的手套箱(Labconco glovebox)中进行电池组装,构筑锂离子电池进行充放电容量和循环性能测试.电化学性能测试时采用的是两电极体系,在充放电测试系统(Land CT2001)上进行充放电实验和循环性能测试,相应的充放电电流密度为0.2 mA/cm2,电势范围为3.0~0.1 V.图1 硫化镍微球的a-b)SEM图片,c)XRD和d)EDS谱图2 结果与讨论2.1 硫化镍微球SEM、XRD、EDS分析图1是以L-胱氨酸为硫源合成的NiS微球的扫描电镜图片、XRD以及EDS谱图,图1a是大面积的扫描电镜图,图中可以看到制备的NiS都为形貌规整的球形结构,大小均一,图1b是放大的SEM图片,可以清楚地看到NiS微球尺寸非常均一,平均直径为2—3 μm,从图中NiS微球某些破损处可以看到,所制备的产品有形成空心结构的趋势.物质的相结构通过XRD来进行表征.图1c是制备的硫化镍XRD 图,从图中可以看出所制备的产品为纯六方相(α)的 NiS,XRD 图谱中在2θ角为30.4°、34.7°、45.9°、53.7°处分别对应于α 相 NiS 的(100)、(101)、(102)、(110)特征晶面,与标准卡片(JCPDS 75-0613)完全一致.图1d是NiS的EDS谱图,图中显示产物中只含有硫和镍两种元素,两个小的杂峰来源于基底的碳和氧,镍和硫的原子个数比接近于1∶1,与硫化镍化学式中元素个数比相吻合.2.2 不同硫源、表面活性剂对硫化镍形貌的影响为了比较不同硫源对硫化镍纳米材料形貌的影响,我们使用硫代乙酰胺、硫代氨基脲、硫脲、硫代硫酸钠以及硫化钠替代L-胱氨酸,当使用硫代乙酰胺为硫源时,合成的产品全为大小比较均一的由针状纳米棒组成的海胆状球形结构,图2是海胆状NiS微球的扫描电镜图片和XRD谱图,图2a是大面积的扫描电镜图,图中可以看到制备的NiS微球大小非常均一,图2b是放大的SEM照片,可以清楚地看到NiS微球表面是由针状纳米棒组装而成的海胆状结构,平均直径为6 μm左右,将单个微球进行放大,针状纳米棒和空心结构清晰可见,纳米棒的直径为40 nm左右(图2c).图2d是制备的硫化镍XRD图,从图中可以看出硫化镍产物中存在两种相结构,斜方六面体相(β)的NiS和六方相(α)NiS,前者的2θ 角为18.5°、30.4°、32.8°、35.8°、40.6°、48.9°分别对应(110)、(101)、(300)、(021)、(211)、(131)特征晶面,与标准卡片(JCPDS 12-0041)相一致.后者的2θ角为30.4°、34.7°、45.9°、53.7°分别对应于α 相 NiS 的(100)、(101)、(102)、(110)特征晶面,与标准卡片(JCPDS 75-0613)完全一致.这与使用L-胱氨酸为硫源制备产品的XRD图有很大区别,说明硫源的不同对产品的相结构有很大的影响.图2 海胆状硫化镍空心球的a-c)SEM图片以及d)XRD谱图将硫源换为硫脲后,获得很多由较粗的纳米棒组成的花状结构(图3a),但大小不一,有部分其他不规整的形貌出现.当使用硫代氨基脲后,得到的则是杂乱无章、大小不一的粒子以及少量由针状纳米棒组成的海胆状结构(图3b),但都欠规整.使用硫代硫酸钠作为硫源时,得到的硫化镍则为长短不一、粗细不等的短棒(图3c),短棒平均直径在3微米左右,而使用硫化钠合成的产品都为杂乱无章的粒子(图3d),由此可见,硫源对硫化镍纳米材料的形貌有着极其重要影响.图3 使用不同硫源合成的NiS纳米材料的SEM图片:a)硫脲,b)硫代氨基脲,c)硫代硫酸钠,d)硫化钠表面活性剂在材料合成中常用来控制产品的形貌,不同表面活性剂的使用可以获得形貌相差很大的硫化镍产品,在使用L-胱氨酸作为硫源,水作为溶剂,用PVP和PEG2000作为表面活性剂时,得到了如图4两种形貌的硫化镍纳米材料,图4a是使用PVP作为表面活性剂合成产品的TEM图片,从图中可以看出制备的硫化镍为大小比较均一的球形粒子,平均尺寸在50 nm左右,而使用PEG2000合成的产品则为由许多小粒子组成的空心球结构(图4b),空心球球壁很薄,直径为100 nm左右.通过以上实验说明在相同条件下,使用不同的表面活性剂会得到形貌截然不同的产品,因此可以通过控制表面活性剂的种类来控制产品的形貌.2.3 电化学性能测试锂离子电池是20世纪90年代出现的绿色高能环保电池,由于具有突出的优点而有着广泛的应用.目前,锂离子电池的电极材料也发展非常迅猛,有许多不同物质的或新的结构的电极材料被研制出来,但以金属硫化物为电极材料的研究并不多见,而NiS由于其具有较高的理论容量在锂离子电池中也有着潜在的应用价值.图4 a)NiS纳米粒子和b)NiS空心球的TEM图片因此,我们分别以海胆状硫化镍空心微球(图2)、纳米粒子组成的硫化镍空心球(图4b)和硫化镍微球(图1)为工作电极,金属Li作为对电极,构筑了Li离子电池,测试了三者的锂离子充放电性能.图5a为海胆状硫化镍空心微球的循环性能图,图中显示首次放电容量超过900 mA h g-1,高于文献所报道的NiS电极材料的理论放电容量[17],但电池充放电容量衰减较快,循环四次以后容量衰减到350 mA h g-1左右,随着循环次数的增加逐渐趋于稳定,当循环到30次以后放电容量依然能保持在200 mA h g-1左右.而用硫化镍微球作为电极首次放电容量只有不到500 mA h g-1,循环30次以后稳定在150 mA h g-1左右(图5b),低于海胆状硫化镍空心微球,但相对比较稳定,衰减率不高.虽然NiS空心球首次放电容量也达到800 mA h g-1左右,但电池容量衰减也较快,循环30次以后容量只有不到80 mA h g-1(图5c),循环性能要明显差于前两者.性能出现以上差异主要是由于海胆状NiS空心微球具有空心的内腔和分级结构的壳,有较大的界面面积和方便的扩散通道,有利于电化学充放电过程的进行,因而循环性能较好,而NiS空心球球壁由许多小粒子组成,球壁较薄,结构比较疏松,循环过程中结构易遭破坏而导致充放电容量的显著衰减.NiS微球充放电容量不高主要是由于其实心结构阻碍了锂离子的嵌入与释放,但其结构相对比较稳定,所以放电容量衰减较慢.由此可见,材料的形貌对锂离子充放电性能有显著的影响,结构稳定、界面面积大的材料可获得更高的充放电容量和更好的循环性能.3 小结图5 a)海胆状NiS空心微球、b)NiS微球和c)NiS空心球的循环性能图(电流密度为0.2 mA/cm2,电势范围为3.0-0.1 V)本文主要通过简单的溶剂热法和水热法制备了多种形貌的硫化镍纳米材料,通过控制不同的硫源成功合成了海胆状空心微球、大小均一的实心微球以及纳米棒等形貌的硫化镍,使用不同的表面活性剂获得了球形粒子和空心球,实现了硫化镍纳米材料形貌的可控合成.分别对海胆状空心微球等三种形貌硫化镍进行了电化学性能测试,结果表明海胆状硫化镍空心微球首次放电容量和循环性能都要好于实心微球和由纳米粒子组成的空心球,循环30次以后放电容量保持在200 mAhg-1左右,显示了较好的循环性能,但放电容量相对还较低,如何通过改进实验条件获得形貌新颖,结构稳定,性能优越的纳米材料将是本课题进一步努力的方向.参考文献:【相关文献】[1]Zhu G,Xu Z.Controllable Growth of Semiconductor Heterostructures Mediated by Bifunctional Ag2S Nanocrystals as Catalyst or Source-Host[J].J.Am.Chem.Soc.,2011,133(1):148.[2]Hu J,Bando Y,Zhan J,et al.Fabrication of Silica-Shielded Ga-ZnS Metal-Semiconductor Nanowire Heterojunctions[J].Adv.Mater.,2005,17(16):1964.[3]Liu B,Zeng H C.Fabrication of ZnO“Dandelions”via a Modifi ed Kirkendall Process [J].J.Am.Chem.Soc.,2004,126(51):16744.[4]Zhou J,Ding Y,Deng S Z,et al.Three-Dimensional Tungsten Oxide Nanowire Networks[J].Adv.Mater.,2005,17(17):2107.[5]Xu L,Ding Y-S,Chen C-H,et al.3D Flowerlike α-Nickel Hydroxide with Enhanced Electrochemical Activity Synthesized by Microwave-Assisted Hydrothermal Method [J].Chem.Mater.,2008,20(1):308.[6]Cheng Y,Wang Y S,Jia C,et al.J.Phys.Chem.B,MnS Hierarchical Hollow Spheres with Novel Shell Structure[J].2006,110(48):24399.[7]Cao A M,Hu J S,Liang H P,et al.Self-Assembled VanadiumPentoxide(V2O5)Hollow Microspheres from Nanorods and Their Application in Lithium-Ion Batteries[J].Angew.Chem.Int.Ed.,2005,44(28):4391.[8]Hu J,Ren L,Guo Y,et al.Mass Production and High Photocatalytic Activity of ZnS Nanoporous Nanoparticles[J].Angew.Chem.Int.Ed.,2005,44(8):1269.[9]Lou X W,Deng D,Lee J Y,et al.Self-Supported Formation of NeedlelikeCo3O4Nanotubes and Their Application as Lithium-Ion Battery Electrodes[J].Adv.Mater.,2008,20(2):258.[10]Wong E,Sheeleigh C W,Rananvare S B.Proceedings of the Sixth Annual Conference on Fossil Energy Materials[A].Oak Ridge:Tennessee NETL Publications,1992.[11]Zhang B,Ye X,Dai W,Hou W,Xie Y.Biomolecule-Assisted Synthesis and Electrochemical Hydrogen Storage of Porous Spongelike Ni3S2Nanostructures Grown Directly on Nickel Foils[J].Chem.Eur.J.2006,12(8),2337.[12]Ghezelbash A,Sigman M B Jr,Korgel B A.Solventless Synthesis of Nickel Sulfide Nanorods and Triangular Nanoprisms[J].Nano Lett.,2004,4(4):537.[13]Yu S H,Yoshimura M.Fabrication Powders of Thin Films of Various Nickel Sulfides by Soft Solution-Processing Routes[J].Adv.Funct.Mater.,2002,12(4):277.[14]Zhang W Q,Xu L Q,Tang K B,et al.Solvothermal Synthesis of NiS 3D Nanostructures[J].Eur.J.Inorg.Chem.,2005(4):653.[15]Xu F,Xie Y,Zhang X,et al.From polymer-metal complex framework to 3D architectures:growth,characterization and formation mechanism of micrometer-sized α-NiS[J].New J.Chem.,2003,27(9):1331.[16]Wu Z,Pan C,Li T,Yang G,et al.Formation of Uniform Flowerlike Patterns of NiS by Macrocycle Polyamine Assisted Solution-Phase Route [J].Crystal Growth.Des.,2007,7(12):2454.[17]Han S C,Kim K W,Ahn H J,et al.Charge-discharge mechanism of mechanically alloyed NiS used as a cathode in rechargeable lithium batteries[J].J.Alloys Compd.,2003,361(1-2):247.。
Zn基柱状ZnO取向生长机制
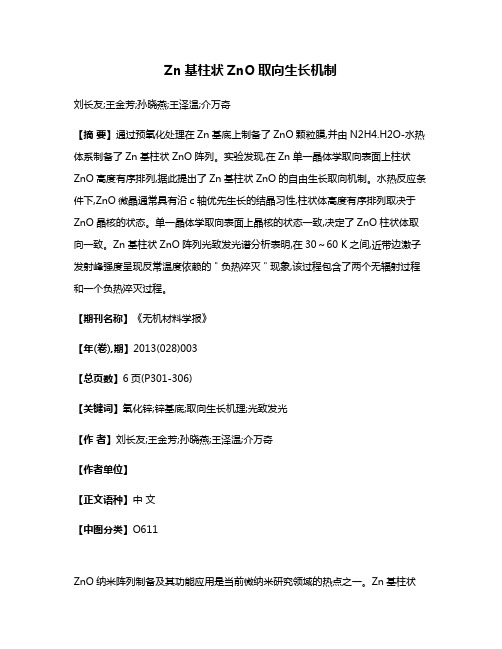
Zn基柱状ZnO取向生长机制刘长友;王金芳;孙晓燕;王泽温;介万奇【摘要】通过预氧化处理在Zn基底上制备了ZnO颗粒膜,并由N2H4.H2O-水热体系制备了Zn基柱状ZnO阵列。
实验发现,在Zn单一晶体学取向表面上柱状ZnO高度有序排列,据此提出了Zn基柱状ZnO的自由生长取向机制。
水热反应条件下,ZnO微晶通常具有沿c轴优先生长的结晶习性,柱状体高度有序排列取决于ZnO晶核的状态。
单一晶体学取向表面上晶核的状态一致,决定了ZnO柱状体取向一致。
Zn基柱状ZnO阵列光致发光谱分析表明,在30~60 K之间,近带边激子发射峰强度呈现反常温度依赖的"负热淬灭"现象,该过程包含了两个无辐射过程和一个负热淬灭过程。
【期刊名称】《无机材料学报》【年(卷),期】2013(028)003【总页数】6页(P301-306)【关键词】氧化锌;锌基底;取向生长机理;光致发光【作者】刘长友;王金芳;孙晓燕;王泽温;介万奇【作者单位】【正文语种】中文【中图分类】O611ZnO纳米阵列制备及其功能应用是当前微纳米研究领域的热点之一。
Zn基柱状ZnO阵列是以Zn片兼作基底和源,不仅能解决导电性问题,还能节省成本,且制备工艺简单,已为人们所关注。
Zn基柱状ZnO阵列的制备方法主要有水热法[1-11]、电化学沉积法[12-14]、热氧化法[15-16]、微波与放电氧化法[17-18]以及软化学法[19-23]等。
其中,水热法具有能耗低、原料廉价、易于控制和绿色环保等优点,倍受人们青睐。
目前,所谓的Zn基ZnO“阵列”,其柱状体在基底上排列并不规则,分布也不均匀。
分析表明,在ZnO柱状体和基底之间存在一个薄膜层,它为ZnO柱状体的生长提供晶核和支撑。
柱状体分布取决于Zn基片上ZnO形核的分布,而ZnO 柱状体的排列不仅与晶核有关,还与ZnO微晶的生长习性有关。
通常情况下,ZnO 沿晶格的c轴方向生长最快,往往呈棒状形态。
因此,ZnO在Zn基片上的成核就成了影响Zn基ZnO阵列形貌特征的主要因素,文献[5]和[19]在这方面作了有益的尝试。
三种纳米结构三氧化钨的气敏性研究

三种纳米结构三氧化钨的气敏性研究王新刚;郭一凡;田阳;刘丽丽;张怀龙【摘要】Utilizing ammonium metatungstate [(NH4)6W12O40] as raw material, we produced three kinds of nanostructured WO3 under the same reaction conditions by controlling the concentration of citric acid (C6H8O7). The nanostructured WO3 was characterized by XRD, SEM and TEM. Then, three kinds of gas sensors including WO 3 nanorod gas sensors, WO3 nanoplate gas sensors and WO3 nanoplate/nanorods mixing gas sensors were further manufactured. The sensitivity was measured for three kinds of nanostructured WO 3 gas sensors under the condition of acetone, ammonia and formaldehyde gas respectively. Experimental results show that the sensitivities of the three kinds of nanostructured WO3 firstly increase and then decrease with the increase of temperature at gas concentration of 1 000 ×10-6. In contrast, the sensitivity of WO3 nanoplate gas sensors is the highest among the three kinds of nanostructured WO3 for the three kinds of gases in the range of measuring temperature. The opt imum operating temperature of WO3 nanoplate gas sensor is 350 ℃, 300 ℃, 325 ℃, 250 ℃ and its maximum sensitivity is 25.4, 18.52, 30.29, 18.31 in acetone, ammonia and formaldehyde gas, respectively. The sensitivity of the three kinds of nanostructured WO3 firstly increases and then decreases with the increase of temperature in the acetone gas of 50 × 10-6 and the formaldehyde gas of 100×10-6, respectively. The sensitivityof WO3 nanoplate is obviously higher than that of other twonanostructured WO3. At the optimum operating temperature, the acetone and formaldehyde gas with lower concentration can be detected by using nanoplate WO 3 gas sensor.%试验以偏钨酸铵为钨源,采用水热法在相同的反应条件下,通过控制柠檬酸的加入量,合成了三种纳米结构的三氧化钨,并采用XRD、SEM和TEM对合成的WO3粉末进行分析。
AZO纳米线使用NAPLD技术

Fabrication of nanowires of Al-doped ZnO using nanoparticle assisted pulsed laser deposition (NAPLD)for deviceapplicationsS.Thanka Rajan a ,B.Subramanian a ,⇑,A.K.Nanda Kumar a ,M.Jayachandran a ,M.S.Ramachandra Rao ba ECMS Division,CSIR –Central Electrochemical Research Institute,Karaikudi 630006,India bDepartment of Physics,Indian Institute of Technology Madras,Chennai 600036,Indiaa r t i c l e i n f o Article history:Received 24June 2013Received in revised form 30August 2013Accepted 7September 2013Available online 26September 2013Keywords:NAPLD Thin films Al doped ZnO Nanowiresa b s t r a c tAluminium doped zinc oxide (AZO)nanostructures have been successfully synthesized on sapphire sub-strates by using nanoparticle assisted pulsed laser deposition (NAPLD)in Ar atmosphere without using any catalyst.The growth of the AZO nanowires has been investigated by varying the argon flow rates.The coatings have been characterized by X-ray diffraction (XRD),Field emission scanning electron microscopy (FESEM),Atomic force microscopy (AFM),Diffuse Reflectance Spectroscopy (DRS),Laser Raman spectroscopy and Photoluminescence spectroscopy.The results of XRD indicate that the deposited films are crystalline ZnO with hexagonal wurtzite structure with (002)preferred orientation.FESEM images also clearly reveal the hexagonal structure and the formation of nanowires with aspect ratios between 15and 20.The surface roughness value of 9.19nm was observed from AFM analysis.The optical properties of the sample showed that under excitation with k =325nm,an emission band was observed in UV and visible region.The characteristic Raman peaks were detected at 328,380,420,430cm À1.Ó2013Elsevier B.V.All rights reserved.1.IntroductionZinc oxide (ZnO)is one of the most important metal oxide semi-conductors.This material has good electrical and optical properties and it is chemically stable.It has a wide direct band gap of 3.37eV with a large binding energy of 60meV [1].Undoped ZnO thin films generally exhibit n-type conductivity due to intrinsic donors,such as oxygen vacancies and Zn interstitials [2].It has an open struc-ture,with a hexagonal close-packed lattice where Zn atoms occupy half of the tetrahedral sites,while all the octahedral sites are empty [3].This crystal structure offers plenty of sites to accommodate intrinsic defect and extrinsic dopants.The wurtzite structure of ZnO can be described as a series of alternating planes of tetrahe-drally coordinated O 2Àand Zn 2+ions stacked along the c -axis;this characteristic polarity of the surfaces gives rise to different nano-structures (nanorods,nanowires,nanobelts,nanotubes)under proper growth conditions [4].Such nanostructures show different and superior properties over their bulk counterparts due to their small size and large surface to volume ratio [5].Impurity-doped ZnO films also show stable electrical and optical properties.Aluminium doping in zinc oxide (AZO)is a promising material due to its high conductivity and good optical properties [6].The choice of Al as donor dopant for ZnO over higher valence ions is lar-gely owing to its ease of incorporation in the ZnO structure;more-over it decreases the resistivity of the host ZnO without impairing the optical transmission in thin film form [7].Both ZnO:Al and Al 2O 3are transparent to visible light,making them interesting can-didates for optical applications.Nowadays,pure ZnO or AZO are being actively investigated as alternative materials to indium tin oxide (ITO)because it is nontoxic,inexpensive and have long term environmental stability [8–10].Several deposition techniques have been routinely used to grow AZO nanostructures.The most common methods are magnetron sputtering [11,12],pulsed laser deposition (PLD)[13],chemical va-por deposition [14],and chemical spray [15].PLD is an attractive method,compared to magnetron sputtering and reactive RF sput-tering techniques,for deposition of ZnO thin films with high struc-tural homogeneity and crystalline quality [16].Compared with other techniques PLD has many advantages such as (i)the compo-sition of the films are close to that of the target,(ii)surface of the film is smooth and (iii)good quality film can be deposited [17].Nanoparticle assisted pulsed laser deposition (NAPLD)is a rela-tively new modification of the PLD technique in which nanowires can be grown at high temperatures and high pressures without using any catalyst.This technique also gives films with almost the same composition as that of the target.This advantage scores highly when compared to the other modified deposition tech-niques [18].In NAPLD the nanoparticles that are formed in the background gas by laser ablation are used for the subsequent growth of the nanowires [18].The initial nanoparticles formed in the laser ablation plume by the condensation are transported onto the substrate and act as starting materials for nano-crystal growth [19].In this work,we report the successful synthesis of AZO nano0925-8388/$-see front matter Ó2013Elsevier B.V.All rights reserved./10.1016/j.jallcom.2013.09.046Corresponding author.Tel.:+914565241538;fax:+914565227713.E-mail address:subramanianb3@ (B.Subramanian).wires on sapphire substrates by NAPLD at different argonflow rates without any catalyst.The structural and optical properties of the nanowires are also discussed in this paper.2.ExperimentalThe depositions of Aluminium doped ZnOfilms were carried out in the NAPLD system.The target preparation procedure is shown in Fig.1.The AZO target was prepared by mixing98mol%ZnO and2mol%Al2O3(99.99%pure,Sigma Aldrich). The sapphire substrate was cleaned byfirst boiling it in trichloroethylene and then ultrasonically cleaned in acetone for3min followed by milli pore water.AZO thin films were deposited on sapphire substrates using frequency triplet,Q switched Nd:YAG laser.Fig.2shows a schematic diagram of the NAPLD system.The target was loaded into the target holder and the substrate was stuck on the substrate holder using silver paste.The target and substrate holder are placed inside a fur-nace,so the whole atmosphere with target and substrate are heated.When the tem-perature reached1000°C,the laser was switched on for ablating the target and Ar gas was let in.The AZOfilms were deposited on sapphire substrate for different ar-gonflow rates(200,300,400and500sccm)for30min.The optimized deposition parameters and conditions are described in the Table1.Thefilms were characterized by Bruker D8Advance to study the phases and for grain size measurement.The surface morphology of the preparedfilms was studied using Quanta3D FESEM.Atomic force microscopy for topographic studies was done by Agliant technologies(model5500).PL spectral analyses were performed using Cary Eclipse(Varian)and Raman spectroscopy for micro-structural analysis was done by Renishaw in via laser Raman microscope.Hall Effect measurements(Eco-pia,HMS3000)were made on the AZOfilms at a constant magneticfield of 1.02T.Diffuse reflectance spectra of thefilms were recorded using Ocean Optics (USB2000)spectrophotometer.BaSO4powder compact was used as a standard ref-erence.The diffuse reflectance(R)was measured as a function of wavelength rang-ing from300to700nm.3.Results and discussion3.1.Structural and compositional analysisThe XRD patterns of the AZO thinfilm for different Argonflow rates(200–500sccm)on sapphire substrates are shown in Fig.3. It was observed that the wurtzite structure of the ZnO is unaffected by the doping of2mol%Aluminium.The diffraction patterns were equivalent,since the Al doping did not show any significant shifts shift is because of the decrease of the lattice constants a and c with increasing argonflow rate.At lowflow rates of the inert gas,the growth mechanism is lateral growth over the substrate surface with uniform coverage.Therefore,a strain can be induced in the lattice of the AZO owing to the strained interface.At higherflow rates,the mechanism varies to condensation in the vapor phase leading to perfect needle type nano wire,which are under signifi-cantly lower stress,with lattice parameters approaching that of an ideal single crystal,although not exactly,owing to the incorpora-tion of Al into the Zn sublattice.Therefore,due to the preferential growth along the[0001]direction,a strain can be induced in the lattice of the AZO by the strained substrate/film interface.This might have led to a change in lattice constants.High texture in (002)will determine the quality of the nano wire.At400sccm flow rate,the(004)line at72°is detected and intensity of the (002)also increased,indicating that the quality of thefilm was im-proved when the argonflow rate was increased.This shows that the crystallinity of the AZO thinfilms increased withflow rate. AZOfilms become polycrystalline,which means thefilm is com-posed of many grains with crystallographic orientations(100), (002),(101),(110),and(004)as indicated in Fig.3.The grain size(D)for various argonflow rates was calculated from the standard Scherer’s formula,expressed asD¼0:94kb cos hð1Þwhere k is the wavelength of Cu K a X-radiation(1.5406Å),b is the Full width at half maximum(FWHM)value for a particular orienta-tion calculated from the XRD pattern and h is the Braggs’angle.The calculated grain size for different argonflow rates(200–500sccm) for(002)peak were77.8,60,86.8and181.5nm respectively. The general trend seems to be that the crystallinity of thefilm also increases with the argonflow rate.Fig.4a–e compares the surface morphology of the ZnOfilms grown under different Arflow rates observed by FE-SEM.Films deposited at200sccm the lowflow rate regime–show a rather dense formation of ZnO grains growing laterally on the substrate surface with uniform coverage(Fig.4a).There is no evidence of the formation of thin ZnO wires.The wire-type morphology ap-pears only with increasingflow rates.Fig.4b shows the morphol-ogy offilm coated at300sccm.Some agglomeration is observed along with clusters randomly dispersed on the substrate.Interest-ingly,appearance of a thin needle-like structure is also seen.Fig.4c shows a SEM image of thefilm deposited at400sccm of Ar.While a number of vertically growing nano rods can be discerned in the microstructure,observation of the crystals along a direction nor-mal to{0001}shows afiner structure of the nano rods that consist of a layered arrangement of ZnO crystals,but neatly stacked along the c axis,suggestive of growth by oriented attachment mecha-nism[20].Such a layered growth produces corrugated10 10side walled nano rods as the layered structure is partially fused be-tween the stacks,probably by diffusional sintering.Clearly,this seems to be a preliminary step towards the perfect needle type nano structures observed at500sccm,shown in Fig.4d and e. Thefilm at500sccm,is homogeneous and comprises of nanowires and nanorods of diameters ranging from150to250nm and the lengths from3to10l m grown along the c-axis,clearly showing the hexagonal lattice of ZnO with wurtzite structure.They appear well aligned in a vertical plane of the substrate and have perfect hexagonal shape.Based on these observations,it is evident that the inert gas(Ar)flow rate plays a crucial role in con-trolling the morphology of the ZnOfilms.At lowflow rates,the partial pressure of Ar in the vapor is too low to produce any con-densation within the vapor and hence,uniform coverage of the substrate is seen.With increasingflow rate of the Ar gas,the ener-getic Zn and O species from the ablated target rapidly lose theirFig.1.Flow chart of target preparation.612S.Thanka Rajan et al./Journal of Alloys and Compounds584(2014)611–616energy by colliding with the colder Ar atoms and nucleate into nano particles within the vapor phase which subsequently act as seeds for the nanocrystalline growth.This mechanism is akin to the inert gas condensation technique and explains the formation of perfectly grown ZnO wire under optimized Ar flow rateconditions.The gradual change from uniformly grown films with lateral spread to vertical nano rods by varying the inert gas flow rate also introduces a slight straining of the lattice which is also reflected in changes in lattice parameter observed by XRD.The nano rods deposited at 500sccm have a low defect density and hence an unstrained lattice,whereas those deposited at 300sccm have a considerable strain due to bonding with the substrate.AFM studies were carried out to investigate the effect of Al dop-ing concentration on surface roughness of the films.Fig.5shows that the surface of the film is covered with grains of diameter about 50nm.The root mean square (rms)surface roughness of the film was measured to be 9.19nm.The elemental composition analyses were carried out on Al doped ZnO nanowires using Energy dispersive X-ray (EDX)analysis and is shown in Fig.6.The presence of Zn,Al and O was observed in the AZO samples.No impurity phases were detected on the surface of the film.The different Hall parameters such as Hall mobility (cm 2V À1s À1),resistivity (X cm)and carrier concentration (cm À3)have been measured for the films deposited at 500sccm.From the previous observation it is optimized that 500sccm shows high X-ray diffraction intensity and the SEM images also reveal that 500sccm is an apt parameter for our studies.Analysis of the equi-librium defect concentration in Al doped ZnO reveals that for each Al 3+that substitutes for one Zn 2+,a free electron is released into the crystal to enhance its conductivity,according to the relation (in Kröger–Vink notation):Al 2O 3ðZnO Þ 2Al Zn þ2O o þ2e 0þ12O 2ð2ÞIn this case,the Al dopant concentration has been fixed at 2mol%,and we assume that the O 2partial pressure does not vary significantly with the Ar flow rate.The electronic charge conduc-tion should control the net resistivity of the film at low frequen-cies.The carrier concentration of the AZO film determined by Hall effect measurement is 8.07Â1019.Hall mobility and resistiv-ity were measured as 25.28cm 2V À1s À1and 1.028Â10À2X cm,which agrees clearly with the values reported by Pin-Chuan Yao et al.[21].3.2.Optical propertiesRaman scattering is an effective technique to investigate the crystallization,structure and defects chemistry of ser Raman spectra of the nanostructured AZO films are shown in Fig.7.Wurtzite structure belongs to the space group C 46v with two formulae units per primitive cell,where all atoms occupy C 3v sites.Twelve vibrational modes exist in the ZnO unit cell;one longitudinal acoustic (LA),two transverse acoustic (TA),threeFig.2.Schematic set up of nanoparticle assisted pulsed laser deposition.Table 1Deposition parameters and conditions.SpecificationParameters LaserNd:YAG (355nm)Repetition rate 10Hz 4ns140mJ/pulse 2–4J cm À2Sintered AZO Sapphire 1000°C Argon $2mbar200–500sccmXRD pattern of AZO films grown on sapphire substrate at different flowlongitudinal-optical(LO),and six transverse optical(TO).A1and E1 symmetries are polar and split into LO and TO components with different frequencies[22].The Raman peaks near328,380,420,430and465cmÀ1can be attributed to the wurtzite crystal structure of AZO.The dominant peak at430cmÀ1indicates the Wurtzite structure of ZnO and is attributed to the high E2mode of non polar optical phonons.E2 high mode is for ZnO hexagonal structure with vibrations of O sub-lattice.The FWHM of the E2line is about8cmÀ1which is another indication of the high quality of the NAPLD synthesized nanocrys-talline ZnOfilms.The peak at380,420and465cmÀ1corresponds to A1(TO),E1(TO)and A1(LO)respectively.The peak A1measured at328cmÀ1is related to multiple phonon scattering process.A slight shift was also observed which was due to the doping of AlFig.4.FESEM images for AZOfilm at(a)200sccm,(b)300sccm,(c)400sccm,(d and e)500sccm.Fig.5.A representative AFM image of AZOfilm.Fig.6.Energy dispersive X-ray analysis of AZO nanowires on sapphire substrate.without introduction of any additional stress within the samples shown in XRD.Diffuse reflectance spectral studies in the UV–visible region were carried out to estimate the optical band gap of the nanostruc-turedfilm.The optical diffuse reflection spectra of AZO samples differentflow rates(200–500sccm)on sapphire substrate are dis-played in Fig.8.The energy band gap E g can be determined from onset of the linear increase in the diffuse reflectance.From2wefind that the band gap of the AZOfilm lies in the range 3.40–3.44eV using Einstein’s energy relation:1:24kðl mÞwhere E is the band gap and k is the wavelength.The band gap increases with increasing Arflow rate.The in-crease in E g may be due to the increase of electron concentration. Generally,the values for band gap of AZOfilms are slightly higher. The conduction electrons in the ZnOfilms are supplied from donor sites associated with oxygen vacancies or excess metal ions[23] The band gap of ZnO is in the near UV region which is3.37 The AZO reflection starts at about380nm and the samples exhib-ited absorption peaks in the visible region at about660nm in both spectra.The photo luminescent emission spectrum of AZOfilms for the various argonflow rates are shown in Fig.9.The PL peaks for all the samples are almost same in position but different in intensity.They show near-band edge emission around373nm which is the UV re-gion;this emission is due to ZnO.The visible emission was ob-served at around405nm,468nm and533nm due to defect emission.The blue peak at468nm comes from the electron transi-tion from Zn interstitial level to the top of the valance band.The green emission observed at533nm resulted from intrinsic defects. Deep level emissions are associated with intrinsic defects such as oxygen vacancies or zinc interstitials[24].From Table3wefind that the calculated band gaps are approx-imately equal to the band gap of ZnO.So the ZnO phase is con-firmed with the band gap in the UV absorption region.However, the UV emission intensity increases and the deep level emission intensity decreases as argonflow rate increases from200to 500sccm suggesting that the crystals grown at higherflow rates have lower defect densities in their lattice.This observation is in agreement with the XRD results suggesting that defect densities decrease with increasing Arflow rates,along with the correspond-ing change in the lattice parameter.ser Raman spectra of AZOfilms on sapphire substrates.Fig.8.DRS patterns of AZOfilms on sapphire substrates.Fig.9.PL emission spectra of AZOfilms on sapphire substrates.Table3Band gap values of AZOfilm on sapphire substrate determined from the PL spectrum.Rgonflow(sccm)Wavelength(nm)Band gap(eV)200364.2 3.32300365.9 3.32400364.9 3.31500355.7 3.334.ConclusionWe have used a relatively new technique,nanoparticle assisted pulsed laser deposition(NAPLD),to grow AZO nanostructures. Deposition of AZO on sapphire using NAPLD was done at high tem-perature and high pressure,without using any catalyst.XRD and EDAX characterization shows that AZO belongs to the most stable wurtzite type with the presence of Zn,Al and O on the surface of thefilm.SEM analysis reveals the formation of nanowires.Band gap of these nanostructures increases from3.37to3.41.Surface topology was studied by AFM imaging of the surfaces of AZOfilms deposited at high temperature and the RMS roughness was deter-mined.XRD and Raman measurements showed that Al ions were incorporated into the ZnO lattice.Photo luminescent emission spectrum of AZOfilms show near-band edge emission around 373nm in the UV region corresponds to ZnO.Increase in the inert gasflow rate seems to reduce defect densities and corresponding with a decrease in the defect level emission intensities in the PL spectra.AcknowledgementsOne of the authors(S.T)thanks Dr.E.Senthil Kumar and F.Bel-larmine for their help in doing the NAPLD process.References[1]S.Tewari,A.Bhattacharjee Pramana,J.Phys.76(1)(2011)153–163.[2]Kyong-Kook Kim,Hitoshi Tampo,June-O Song,Tae-Teon Seong,Seong-Ju Park,Ji-Myon Lee,Sang-Woo Kim,Shizuo Fujita,Shigeru Niki,Jpn.J.Appl.Phys.44 (7A)(2005)4776–4779.[3]Chundong Li,Jinpeng Lv,Bo Zhou,Zhiqiang Liang,Phys.Status Solidi A209(8)(2012)1538–1542.[4]Rodrigo Noriega,Jonathan Rivnay,Ludwig Goris,Daniel Kälblein,Hagen Klauk,Klaus Kern,Linda M.Thompson,Aaron C.Palke,Jonathan F.Stebbins,Jacob R.Jokisaari,Greg Kusinski,Alberto Salleo,in:J.Appl.Phys.107(2010)074312-1–074312-7.[5]M.Mozibur Rahman,M.K.R.Khan,M.Rafiqul Islam,M.A.Halim,M.Shahjahan,M.A.Hakim,Dilip Kumar Saha,Jasim Uddin Khan,J.Mater.Sci.Technol.28(4) (2012)329–335.[6]S.M.Park,G.H.Gu,C.G.Park,Phys.Status Solidi A208(2011)2688–2691.[7]H.Kim,J.S.Horwitz,S.B.Qadri,D.B.Chrisey,Thin Solid Films420–421(2002)107–111.[8]Sang moo Park,Tomoaki Ikegami,Kenji Ebihara,Jpn.J.Appl.Phys.45(2006)8453–8456.[9]S.Suzuki,T.Miyata,M.Ishii,T.Minami,Thin Solid Films434(2003)14–19.[10]B.Szyszka,Thin Solid Films351(1999)164–169.[11]Z.Le,W.Gao,Mater.Lett.58(2004)1363–1370.[12]Z.G.Yu,P.Wu,H.Gong,Appl.Phys.Lett.88(2006)132114–132116.[13]F.K.Shan,B.C.Shin,S.C.Kim,Y.S.Yu,J.Korean Phys.Soc.42(2003)S1374–S1377.[14]Y.Natsume,H.Sakata,T.Hirayama,H.Yanagada,J.Appl.Phys.72(1992)4203–4207.[15]H.Mondragon Suarez, A.Maldonaldo,M.de la,L.Olvera, A.Reyes,R.Castanedo-Perez,G.Torres-Delgado,R.Asomoza,Appl.Surf.Sci.193(2002) 52–59.[16]V.Cracium,J.Elders,J.G.E Gardeniers,I.W.Boyd,Appl.Phys.Lett.65(1994)2963–2965.[17]A.V.Singh,Manoj Kumar,R.M.Mehra,Akihiro Wakahara,Andakira Yoshida,J.Indian Inst.Sci.81(2001)527–533.[18]Y.N.Xia,P.D.Yang,Y.G.Sun,Y.Y.Wu,B.Mayers,B.Gates,Y.D.Yin,F.Kim,Y.Q.Yan,Adv.Mater.15(2003)353–389.[19]Tatsuo Okado,Ruqian Guo,Jun Nishimura,Masato Matsumoto,MitsuhiroHigashihata,Daisuke Nakamura,Thin Solid Films447–448(2004)33–39.[20]Shilei Xie TengZhai,Yexiang Tong,mun.14(2012)1850–1855.[21]Pin Chuan Yao,Shih Tse Hang,Yu Shuan Lin,Wen Tsai Yen,Yi Cheng Lin,Appl.Surf.Sci.257(2010)1441–1448.[22]A.Singh,A.Kumar,N.Suri,S.Kumar,M.Kumar,P.K.Khanna,D.Kumar,J.Optoelectron.Adv.Mater.11(6)(2009)790–793.[23]M.Suchea,S.Christoulakis,N.Katsarakis,T.Kitsopoulos,G.Kiriakidis,ThinSolid Films515(2007)6562–6566.[24]K.J.Chen,T.H.Fang,F.Y.Hung,L.W.Ji,S.J.Chang,S.J.Young,Y.J.Hsiao,Appl.Surf.Sci.254(2008)5791–5795.616S.Thanka Rajan et al./Journal of Alloys and Compounds584(2014)611–616。
一步水热法合成SiO2纳米棒
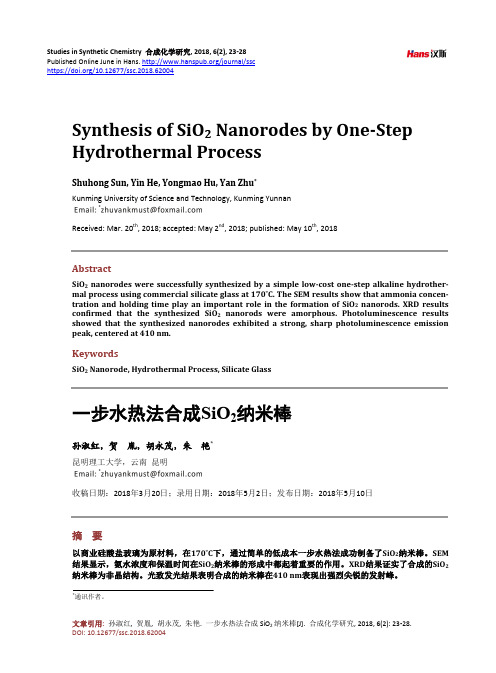
Studies in Synthetic Chemistry 合成化学研究, 2018, 6(2), 23-28Published Online June in Hans. /journal/sschttps:///10.12677/ssc.2018.62004Synthesis of SiO2 Nanorodes by One-StepHydrothermal ProcessShuhong Sun, Yin He, Yongmao Hu, Yan Zhu*Kunming University of Science and Technology, Kunming YunnanReceived: Mar. 20th, 2018; accepted: May 2nd, 2018; published: May 10th, 2018AbstractSiO2 nanorodes were successfully synthesized by a simple low-cost one-step alkaline hydrother-mal process using commercial silicate glass at 170˚C. The SEM results show that ammonia concen-tration and holding time play an important role in the formation of SiO2nanorods. XRD results confirmed that the synthesized SiO2nanorods were amorphous. Photoluminescence results showed that the synthesized nanorodes exhibited a strong, sharp photoluminescence emission peak, centered at 410 nm.KeywordsSiO2 Nanorode, Hydrothermal Process, Silicate Glass一步水热法合成SiO2纳米棒孙淑红,贺胤,胡永茂,朱艳*昆明理工大学,云南昆明收稿日期:2018年3月20日;录用日期:2018年5月2日;发布日期:2018年5月10日摘要以商业硅酸盐玻璃为原材料,在170˚C下,通过简单的低成本一步水热法成功制备了SiO2纳米棒。
翻译原文

Home Search Collections Journals About Contact us My IOPscienceThe synthesis and selective gas sensing characteristics of SnO2/α-Fe2O3 hierarchical nanostructuresThis article has been downloaded from IOPscience. Please scroll down to see the full text article.2008 Nanotechnology 19 205603(/0957-4484/19/20/205603)View the table of contents for this issue, or go to the journal homepage for moreDownload details:IP Address: 202.113.13.10The article was downloaded on 09/09/2012 at 03:11Please note that terms and conditions apply.IOP P UBLISHING N ANOTECHNOLOGY Nanotechnology19(2008)205603(5pp)doi:10.1088/0957-4484/19/20/205603The synthesis and selective gas sensing characteristics of SnO2/α-Fe2O3 hierarchical nanostructuresYujin Chen1,2,4,Chunling Zhu3,Xiaoling Shi2,Maosheng Cao2andHaibo Jin21College of Science,Harbin Engineering University,Harbin15001,People’s Republic ofChina2School of Materials Science and Engineering,Beijing Institute of Technology,Beijing100081,People’s Republic of China3College of Materials Science and Chemical Engineering,Harbin Engineering University,Harbin150001,People’s Republic of ChinaE-mail:chenyujin@Received28January2008,infinal form10March2008Published14April2008Online at /Nano/19/205603AbstractSnO2/α-Fe2O3hierarchical nanostructures,in which the SnO2nanorods grow on the sidesurface ofα-Fe2O3nanorods as multiple rows,were synthesized via a three-step process.Thediameters and lengths of the SnO2nanorods are6–15nm and about120nm.The growthdirection of SnO2nanorods is[001],significantly affected by that ofα-Fe2O3nanorods.Thehetero-nanostructures exhibit very good selectivity to ethanol.The sensing characteristics arerelated to the special heterojunction structures,confirmed by high-resolution transmissionelectron microscopy observation.Therefore,a heterojunction barrier controlled gas sensingmechanism is realized.Our results demonstrate that the hetero-nanostructures are promisingmaterials for fabricating sensors and other complex devices.1.IntroductionHierarchical hetero-nanostructures are expected to have novel or multifunctional properties and have applications in the fabrication of complex nanodevices.In recent years, great progress has been made in the synthesis of such nanocomposites[1–7].Hierarchical ZnO nanostructures were synthesized by a vapor transport and condensation technique. In the nanocomposites,the secondary ZnO nanorods grew either as a single row or multiple rows on the side surfaces of the core In2O3nanowires[1].Xie et al reported the epitaxial growth of T-ZnO/SnO2hetero-nanostructures and found that the new luminescence properties were induced by the epitaxial interfaces[2].Recently,Fen and his co-workers grew carbon nanotubes,ZnO nanowires and silicon nanowires on carbon cloth[3–5].These nanocomposites exhibited enhancedfield emission properties and are expected to befield emitters with practical applications in highly efficient lamps,field emission displays,and micro vacuum electron sources.4Author to whom any correspondence should be addressed.As two important kinds of fundamental material, SnO2[8–18]andα-Fe2O3[20–26]have attracted a lot of attention due to their applications as chemical catalysts and devices such as sensors[16–18],UV detectors and transistors[19],etc.In this paper,we report a three-step route to grow SnO2nanorod arrays on the side surfaces of porousα-Fe2O3nanorods.The gas sensing properties and sensing mechanism of the SnO2/α-Fe2O3hierarchical hetero-nanostructures are investigated.2.Experimental detailsSnO2/α-Fe2O3hierarchical hetero-nanostructures were synthe-sized via a three-step process.First,β-FeOOH nanorods were synthesized by a hydrothermal route.In a typical procedure, 40ml of0.5M FeCl3solution was transferred into a50ml Teflon-lined autoclave.The autoclave was heated to120◦C, and kept for12h.After cooling to room temperature,the resulting precipitates were washed several times with water and absolute ethanol,and then dried at80◦C for6h.AfterFigure1.(a)SEM image ofβ-FeOOH nanorods.(b)SEM image of α-Fe2O3nanorods.(c)XRD pattern of the products.Pattern1:β-FeOOH,pattern2:α-Fe2O3nanorods,pattern3:SnO2/α-Fe2O3 hierarchical hetero-nanostructures.(d)SEM image ofα-Fe2O3 nanorods.calcinations of the obtainedβ-FeOOH nanorods at500◦C for 2.5h,α-Fe2O3nanorods with porous structures were obtained. The SnO2/α-Fe2O3hierarchical hetero-nanostructures werefi-nally prepared usingα-Fe2O3nanorods and SnCl4as starting materials through a hydrothermal method as follows.0.015g α-Fe2O3nanorods were dispersed into a mixed solution con-sisting of heptane(10.2ml),hexanol(3.0ml),sodium dodecylsulfate(SDS,1.44g),Sn(OH)2−6(2.0ml;the volume ratio of0.5M SnCl4and5M NaOH solutions is1:3).After ultra-sonication for5min,the mixture was transferred into a25mlTeflon-lined autoclave and heated to220◦C for6h.After cool-ing to room temperature,the obtained precipitates were washedseveral times with absolute ethanol and distilled water.The fabrication process of the sensors based on the hetero-nanostructures has been described elsewhere[16,17,27–30].The sensitivity(S)in this paper is defined as S= R a/R g[16,17],where R a is the sensor resistance in air,and R g is the resistance in target–air mixed gas.During the measuredprocess the ambient relative humidity(RH)is about30%. 3.Results and discussion3.1.Morphology study of hetero-nanostructuresβ-FeOOH nanorods can be obtained at temperatures in the range from90to120◦C.Samples prepared at different synthesis temperatures have slightly different morphologies. Figure1(a)is a typical scanning electron microscopy(SEM) image of the as-synthesizedβ-FeOOH nanorods that were synthesized at120◦C.It indicates that the diameters and the lengths of the nanorods are50–180nm and∼2μm, respectively.Pattern1infigure1(c)shows corresponding x-ray diffraction(XRD)pattern from the pared with the data in JCPDS card(No.75-1594),all the diffraction peaks in the pattern can be indexed toβ-FeOOH.After calcinations at500◦C for2.5h,β-FeOOH nanorodswere Figure2.(a)Low-resolution TEM image of SnO2/α-Fe2O3 hierarchical hetero-nanostructures.Scale bar:100nm.(b)High-resolution TEM image of an individual SnO2nanorod grown onα-Fe2O3nanorods.Scale bar:5nm.(c)High-resolution TEM image of the interface of SnO2/α-Fe2O3hierarchicalhetero-nanostructures.transformed intoα-Fe2O3nanorods,proved by XRD pattern 2infigure1(c)(JCPDS No.33-0664).They remained in the original morphologies(figure1(b)).Pattern3is the measured XRD pattern of thefinal products.The asterisks indicate the peaks coming fromα-Fe2O3(JCPDS No.33-0664),while the others come from SnO2(JCPDS No.41-1445).The results indicate that thefinal products were composites ofα-Fe2O3and SnO2.Figure1(d)shows a typical SEM image of thefinal products.It can be clearly observed that thefinal products exhibit hierarchical heterostructures,in which the second nanorod arrays grow on the side surface of theα-Fe2O3 nanorods as multiple rows.According to the XRD results,the secondary phase in the structures is SnO2nanorods.The morphology and size of the hierarchical hetero-nanostructures were further characterized by transmission electron microscopy(TEM).Figure2(a)displays a typical TEM image of the heterostructures.It is found that the average diameters and lengths of the SnO2nanorods are6–15nm and about120nm,respectively.It is clear that the SnO2nanorods are parallel to each other at the same side surface of anα-Fe2O3nanorod,and the angle between the SnO2andα-Fe2O3 nanorods is about122◦or58◦.3.2.Crystallographic study of hetero-nanostructuresIn order to clarify how SnO2nanorods grow onα-Fe2O3 nanorods,high-resolution TEM(HRTEM)observations of hi-erarchical hetero-nanostructures were conducted.Figure2(b) shows the HRTEM image of an individual SnO2nanorod.The two adjacent spacings are0.246and0.237nm,correspond-ing to(101)and(200)planes of SnO2,respectively.This in-dicates the single-crystal nature of the nanorods with prefer-ential growth along the[001]direction.The result is differ-ent from the previous report by Zhang et al that[101]is thepreferential growth direction for SnO 2nanorods grown on α-Fe 2O 3nanotubes [11].This may be related to the growth direc-tion of α-Fe 2O 3nanorods because the growth of heterostruc-tures requires low lattice mismatch between different materi-als [2,11].Figure 2(c)is an HRTEM image of the interface of hetero-nanostructures.It is obvious that the SnO 2nanorods still grow along the [001]direction at the interface.For α-Fe 2O 3nanorods,the two almost identical spacings of 0.252nmare consistent with the d values of the (2¯10)and (110)planes,indicating that the α-Fe 2O 3nanorods grow preferentially along the [110]direction.Therefore,the interfacial orientation relationships between the SnO 2and α-Fe 2O 3nanorods are(101)SnO 2/(110)α-Fe 2O 3and (200)SnO 2/(2¯10)α-Fe 2O 3.The lat-tice mismatch is lower for such interfacial orientation relation-ships [11],thereby resulting in SnO 2nanorods with growth along the [001]rather than [101]direction.The result demon-strates that the growth direction of the second phase can be affected by the first phase in a heterogeneous system.This may be an effective route to synthesize heterostructures with controlled crystalline and physical characteristics because dif-ferent growth directions may lead to different physical proper-ties.For example,SnO 2nanoribbons with exposed (10¯1)and (010)surfaces have highly sensing characteristics to NO 2even at room temperature [31].The experimentally measured angle between the (101)and (200)planes of SnO 2is about 56.2◦,while it is about60◦between the (2¯10)and (110)planes of α-Fe 2O 3,as shown in figure 2(c).Both values are consistent with the theoretically calculated values,suggesting that the misfit angle between those planes is about 3.8◦.According to the HRTEMobservation (figure 2(c)),however,the (2¯10)α-Fe 2O 3plane is not completely parallel to the (200)SnO 2plane:the acute angle between them is about 2◦.Therefore,the misfit angle between the (110)α-Fe 2O 3plane and the (101)SnO 2plane is about 1.8◦.The results reveal that SnO 2nanorods grown on α-Fe 2O 3nanorods should be parallel to each other at the same side surface and the angle between SnO 2nanorods and α-Fe 2O 3nanorod should be 122◦or 58◦,which is consistent with the low-resolution TEM observation (figure 2(a)).In addition,lattice distortion induced by lattice mismatch is also observed,as indicated by the white frame in figure 2(c).The phenomenon is also observed by Zheng et al in the ZnO/SnO 2system [2].It should be noted that the synthesized conditions have an important effect on the formation of SnO 2/α-Fe 2O 3hierarchical hetero-nanostructures.When the volume ratio of SnCl 4and NaOH was changed to 1:1or 1:2,the hierarchical hetero-nanostructures mentioned above were difficult to obtain.In those cases,only SnO 2nanoparticles or short rods grew on the side surfaces of α-Fe 2O 3nanorods.The same results were also observed when the solvents were changed into a mixture of ethanol and water,or without the addition of SDS.Therefore,the volume ratio of SnCl 4and NaOH,the solvents and SDS are important factors for the growth of SnO 2/α-Fe 2O 3hierarchical hetero-nanostructures.3.3.Gas sensitivity of hetero-nanostructuresIt is well known that SnO 2and α-Fe 2O 3are important sensing materials.However,gas sensors for practicalapplicationsFigure 3.The sensitivities of SnO 2/α-Fe 2O 3hetero-nanostructures to ethanol at different working temperatures:curve 1,at 350◦C;curve 2,at 250◦C.The inset shows the sensitivities of SnO 2/α-Fe 2O 3hetero-nanostructures to H 2,CH 4and C 4H 10gases at a working temperature of 350◦C.require not only high sensitivities,but also very good selectivity to target molecules.The hierarchical hetero-nanostructures prepared in this work exhibited very good selectivity as well as high sensitivity to ethanol.Figure 3shows the sensitivities of the hetero-nanostructures to ethanol with various concentrations at different working temperature.At a working temperature of 350◦C,the sensitivity is 4.6–10ppm,as shown in curve 1,while the sensitivities are less than 2.3–1000ppm of H 2,CH 4and C 4H 10as shown in the inset of figure 3.As the working temperature was reduced to 250◦C,the hetero-nanostructures still kept high sensitivity.The sensitivity is up to 2.9–10ppm of ethanol,as shown in curve 2;however,the hetero-nanostructures have no response to the three other gases.The results demonstrate that the hetero-nanostructures are promising materials for fabricating gas sensors with good sensing characteristics.For a pure metal oxide semiconductor,the sensing mecha-nism is usually explained by the space–charge model [16,17].In this model,the sensitivity of the materials is strongly de-pendent on the change of the space–charge length under differ-ent gas atmospheres,resulting in higher sensitivity of the sens-ing materials with smaller size [16,17].However,the elec-tron transport mechanism of the nanocomposites synthesized in this work should be significantly different from that of the pure SnO 2or α-Fe 2O 3nanorods due to the formation of many semiconductor heterojunctions at their interfaces,observed by HRTEM,as shown in figure 2(c).Electron transport is thus expected to be strongly tuned by the heterojunction (HJ)bar-rier,which has been widely investigated for many HJ devices such as lasers,photodiodes and HJ bipolar transistors.It is well known that the band gaps of SnO 2and α-Fe 2O 3at room temperature are about 3.6eV and about 2.1eV [12,32,33].Thus the energy band structure of the SnO 2/α-Fe 2O 3HJ can be schematically depicted in figure 4,where φeff denotes the effective barrier height,considering the contribution of other factors such as temperature to the barrier height [34].There-fore,it requires that the transport of electrons should overcome the heterojunction barriers [34].At the high temperature re-gion,the electron motility μis expressed by [34]μ=μ0exp (−q φeff /k B T ),(1)Figure4.A schematic diagram of the energy band structure of the SnO2/α-Fe2O3hetero-nanostructures:(a)in air,(b)in ethanol. where q is the charge of an electron,k B is Boltzmann’s constant,and T is the absolute temperature.According to equation(1),the conductivity G of the heterostructures under different gas atmospheres can be given by[34]G=G0exp(−φeff/k B T).(2) In this equation,G0can be considered as a constant parameter.φeff will increase in air because electrons are trapped both in SnO2andα-Fe2O3induced by adsorbed oxygen species,as shown infigure4(a).In this case,the conductivity of the HJ is very low.When the heterostructures are exposed to ethanol,the reaction between the adsorbed oxygen species and the ethanol molecules leads to the release of the trapped electrons back simultaneously into the conduction bands of the SnO2and Fe2O3rods,resulting in a decrease in the width and height of the barrier potential at their interfaces,as shown infigure4(b).In the case,the conductivity of the HJ will consequently be greatly increased,resulting in high sensitivity of the SnO2/α-Fe2O3HJs to ethanol. Therefore,the barrier with adjustable height controls the transport of electrons in the heterostructures,and accordingly controls the sensing characteristics of the nanocomposites.As for the good selectivity of the hetero-nanostructures,it may be related to the following factors.First,for pure SnO2 sensors the sensitivity to H2exhibited significantly different characteristics,depending on the fabrication techniques of both the sensing materials and the sensors.For example, W¨o llenstein et al fabricated a micromachined micro-hotplate sensor array based on ZnO,WO3and V2O5,in which the sensitivity of SnO2to100ppm H2is less than3.0at a working temperature of400◦C[35].Salehi et al reported that SnO2 sensingfilms had a sensitivity to1000ppm H2less than4.0 at200◦C[36].But the sensitivity of sensors based on SnO2 thickfilms with porous structures were above400–800ppm H2at350◦C[37].The sensitivity was as high as1000–800ppm H2at350◦C after SnO2nanoparticles were calcined at600◦C[38].This phenomenon may result from the size and crystalline phase of SnO2sensing materials and the sensor configurations.Therefore,the sensors based on the hetero-nanostructures presumably exhibited low sensitivity to H2. Second,the sensing properties of composites are significantly different from those of the single-component counterparts. For instance,CeO/SnO2and SnO2/CuO composites had excellent selectivity to H2S[39,40].SnO2/ZnO[41]and SnO2/α-Fe2O3[42,43]composites exhibited greatly improved sensitivity to ethanol vapor compared to H2,CH4and CO molecules.The reason for this is that the sensing mechanism of the composites is different from that of the single-component counterparts[43,44].It has been reported that when the test gas has a complex molecular structure or a reactive functional group such as ethanol,the surface reactions could differ depending on the acid–base properties.The base properties are more helpful to the surface reactions between C2H5OH and surface O species.In this work,the hetero-nanostructures are composed of SnO2andα-Fe2O3nanorods.They are basic oxides,and thereby the basicity of the hetero-nanostructures will increase.Due to the fact mentioned above,the SnO2/α-Fe2O3hetero-nanostructures exhibited higher sensitivity to ethanol than to H2,CH4and C4H10gases.In addition,water vapor cross-sensitivity is important for gas sensors based on metal oxides.Thus the response of the sensors was also measured at the RH of∼30%and∼54%, respectively.Negligible resistance changes of the sensors with the present configuration at these humidities were observed. Therefore,the sensors based on the hetero-nanostructures can be used to detect ethanol vapor.4.ConclusionsIn summary,we synthesized SnO2/α-Fe2O3hierarchical hetero-nanostructures by a three-step process.The SnO2 nanorods with average diameters in the range6–15nm and lengths of120nm grow on the side surface of theα-Fe2O3 nanorods as multiple rows,resulting in the formation of hierarchical structures.It is observed that the preferential growth direction of SnO2nanorods is[001],significantly affected by the growth direction ofα-Fe2O3nanorods.This may offer an effective route to synthesize heterostructures with controlled crystalline and physical characteristics.The hetero-nanostructures exhibit high sensitivity and very good selectivity to ethanol.Heterojunction barrier controlled gas sensing characteristics were realized in SnO2/α-Fe2O3 hierarchical nanostructures.Our results demonstrate that SnO2/α-Fe2O3hetero-nanostructures are very promising for fabricating sensors as well as other complex devices. AcknowledgmentsThe authors acknowledge the support from the National Natural Science Foundation of China(50772025),the PhD Programs Foundation of the Ministry of Education of China(20070217002),China Postdoctoral Science Foundation (20060400042),and also the Innovation Foundation of Harbin City(RC2006QN017016).References[1]Lao J Y,Wen J G and Ren Z2002Nano Lett.21287[2]Kuang Q,Jiang Z Y,Xie Z X,Lin S C,Lin Z W,Xie S Y,Huang R B and Zheng L S2003J.Am.Chem.Soc.12511306[3]Banerjee D,Jo S H and Ren Z F2004Adv.Mater.162028[4]Jo S H,Wang D Z,Huang Z Y,Li W Z,Kempa K andRen Z F2004Appl.Phys.Lett.85801[5]Zeng B Q,Xiong G Y,Chen S,Wang W Z,Wang P Z andRen Z F2007Appl.Phys.Lett.90033112[6]Zhang D F,Sun L D,Jia C J,Yan Z G,You L P andYan C H2005J.Am.Chem.Soc.12713492[7]Wan Q,Wei M,Zhi D,MacManus-Driscoll J L andBlamire M G2006Adv.Mater.18234[8]Wu N L,Wang S Y and Rusakova I A1999Science2851375[9]Comini E,Faglia G,Sberveglieri G,Pan Z and Wang Z L2002Appl.Phys.Lett.811869[10]Law M,Kind H,Messer G,Kim F and Yang P D2002Angew.Chem.Int.Edn412405[11]Zhang D F,Sun L D,Yin J L and Yan C H2003Adv.Mater.151022[12]Dai Z R,Pan Z W and Wang Z L2003Adv.Funct.Mater.139[13]Chen Y J,Li Q H,Liang Y X,Wang T H,Zhao Q andYu D P2004Appl.Phys.Lett.855682[14]Cheng B,Russell J M,Shi W S,Zhang L andSamulski E T2004J.Am.Chem.Soc.1265972[15]Chowdhuri A,Gupta V,Sreenivas K,Kumar R,Mozumdar S and Patanjali P K2004Appl.Phys.Lett.841180[16]Chen Y J,Xue X Y,Wang Y G and Wang T H2005Appl.Phys.Lett.87233503[17]Chen Y J,Xue X Y,Wang Y G and Wang T H2006Appl.Phys.Lett.88083105[18]Kuang Q,Lao C S,Wang Z L,Xie Z X and Zheng L S2007J.Am.Chem.Soc.1296070[19]Dattoli E N,Wan Q,Guo W,Chen Y B,Pan X and Lu W2007Nano Lett.72463[20]Rockenberger J,Scher E C and Alivisatos A P1999J.Am.Chem.Soc.1211595[21]Park J,An K,Hwang Y,Park J G,Noh H J,Kim J Y,Park J H,Hwang N M and Hyeon T2004Nat.Mater.3891[22]Wang D S,He J B,Rosenzweig N and Rosenzweig Z2004Nano Lett.4409[23]Jia C J,Sun L D,Yan Z G,You L P,Luo F,Han X D,Pang Y C,Zhang Z and Yan C H2005Angew.Chem.Int.Edn444328[24]Xiong G,Joly A G,Holtom G P,Wang C M,McCready D E,Beck K M and Hess W P2006J.Phys.Chem.B11016937 [25]Wu J J,Lee Y L,Chiang H H and Wong D K P2006J.Phys.Chem.B11018108[26]Li L,Chu Y,Liu Y and Dong L H2007J.Phys.Chem.C1112123[27]Liang Y X,Chen Y J and Wang T H2004Appl.Phys.Lett.85666[28]Chen Y J,Zhu C L and Wang T H2006Nanotechnology173012[29]Chen Y J,Zhu C L and Xiao G2006Nanotechnology174537[30]Chen Y J,Zhu C L and Xiao G2008Sensors Actuators B129639[31]Maiti A,Rodriguez J A,Law M,Kung P,McKinney J R andYang P2003Nano Lett.31025[32]Preisinger M,Krispin M,Rudolf T,Horn S andStrongin D R2005Phys.Rev.B71165409[33]Gratzel M2001Nature414338[34]Weis T,Lipperheide R,Wille U and Brehme S2002J.Appl.Phys.921411[35]W¨o llenstein J,Plaza J A,Can´e C,Min Y,B¨o ttner H andTuller H L2003Sensors Actuators B93350[36]Salehi A and Gholizade M2003Sensors Actuators B89173[37]Sakai G,Baik N S,Miura N and Yamazoe N2001SensorsActuators B77116[38]Baik N S,Sakai G,Miura N and Yamazoe N2000SensorsActuators B6374[39]Fang G J,Liu Z L,Liu C Q and Yao K L2000SensorsActuators B6646[40]Chowdhuri A,Gupta V,Sreenivas K,Kumar R,Mozumdar S and Patanjali P K2004Appl.Phys.Lett.841180[41]de Lacy Costello B P J,Ewen R J,Guernion N andRatcliffe N M2002Sensors Actuators B87207[42]Gopal Reddy C V,Cao W,Tan O K and Zhu W2002SensorsActuators B81170[43]Tan O K,Cao W,Zhu W,Chai J W and Pan J S2003SensorsActuators B93396[44]Yamazoe N and Miura N1992Chemical Sensor Technologyvol4,ed S Yamauchi(Amsterdam:Elsevier)pp39–40。
- 1、下载文档前请自行甄别文档内容的完整性,平台不提供额外的编辑、内容补充、找答案等附加服务。
- 2、"仅部分预览"的文档,不可在线预览部分如存在完整性等问题,可反馈申请退款(可完整预览的文档不适用该条件!)。
- 3、如文档侵犯您的权益,请联系客服反馈,我们会尽快为您处理(人工客服工作时间:9:00-18:30)。
Defects in ZnO Nanorods Prepared by a Hydrothermal MethodK.H.Tam,†C.K.Cheung,†Y.H.Leung,‡A.B.Djurisˇic´,*,†C.C.Ling,†C.D.Beling,†S.Fung,†W.M.Kwok,‡W.K.Chan,‡D.L.Phillips,‡L.Ding,§and W.K.Ge§Department of Physics,The Uni V ersity of Hong Kong,Pokfulam Road,Hong Kong,Department of Chemistry,The Uni V ersity of Hong Kong,Pokfulam Road,Hong Kong,and Department of Physics,The Hong KongUni V ersity of Science and Technology,Clear Water Bay,Hong KongRecei V ed:May26,2006;In Final Form:July18,2006ZnO nanorod arrays were fabricated using a hydrothermal method.The nanorods were studied by scanningelectron microscopy,photoluminescence(PL),time-resolved PL,X-ray photoelectron spectroscopy,and positronannihilation spectroscopy before and after annealing in different environments and at different temperatures.Annealing atmosphere and temperature had significant effects on the PL spectrum,while in all cases thepositron diffusion length and PL decay times were increased.We found that,while the defect emission canbe significantly reduced by annealing at200°C,the rods still have large defect concentrations as confirmedby their low positron diffusion length and short PL decay time constants.1.IntroductionFabrication of ZnO nanostructures1-4and the optical proper-ties of ZnO3-15have been extensively studied.Among the various methods for fabrication of ZnO nanostructures,the hydrothermal method1-4is of particular interest since it is a low cost,environmentally friendly method with growth tem-peratures as low as90°C.As a natural consequence of solution-phase growth at low temperature,the as-grown nanorods have large defect concentrations and typically exhibit weak UV but prominent defect emission at room temperature.3,4While the morphology and orientation of the nanorods can be significantly improved by using the ZnO seeds prepared from zinc acetate solution instead of ZnO nanoparticles,1improvements of their optical quality have not been investigated in detail.While it has been shown that the room-temperature photoluminescence (PL)spectra of nanorods can be changed by annealing under different conditions,3,15other techniques have not been used to conduct a comprehensive investigation of the effects of anneal-ing on their properties.It is generally assumed that a large UV to visible emission ratio implies excellent quality of the ZnO nanostructures,but typically,no other techniques are used to verify whether the defect density is indeed low.In addition, the origin of the visible emission is highly controversial.16ZnO can exhibit different emissions in the visible range(violet,blue, green,yellow,and orange-red)which are associated with defects in the material.Green emission is the most commonly observed defect emission in ZnO.However,there is still no consensus on the origin of this emission,and a number of different hypotheses have been proposed.16The possibility that green emissions in different samples can have different origins despite similar position and width has also been proposed.16If this is indeed the case,then the origin of the defect emission cannot be postulated simply by noting the similarities with previously published spectra for ZnO prepared by other fabrication methods.Therefore,considerable interest exists in studying the defect emissions in ZnO in general and ZnO nanorod arrays in particular due to their great potential for practical applications. Since applications of nanorod arrays in optoelectronic devices require control of their emission properties,it is necessary to perform a comprehensive study of their optical properties. Here,we report an investigation of the properties of ZnO nanorods using field emission scanning electron microscopy (SEM),variable temperature PL,time-resolved PL,X-ray photoelectron spectroscopy(XPS),and positron annihilation spectroscopy(PAS).We show that PL spectra do not provide sufficient evidence of defect density in the material.Contrary to common assumption,it is possible to have strong UV emission and weak defect emission and still have considerable defect concentrations in the sample.Thus,to fully characterize sample properties,other measurements in addition to PL spectroscopy are necessary.2.Experimental SectionThe nanorod arrays were prepared from solutions of zinc nitrate hydrate and hexamethylene tetramine on Si substrates with a seed layer prepared from zinc acetate solution.1Poly-ethyleneimine was added to the solution to increase the nanorod aspect ratio.2The morphology of the nanorods before and after annealing was examined using a Leo1530field emission SEM. The rods were annealed in a tube furnace at temperatures200, 400and600°C.The annealing atmospheres were air(atmo-spheric pressure,relative humidity∼55-60%),argon,nitrogen, and forming gas(90%N2/10%H2).For annealing in a gas flow, the gas flow rate was0.1Lpm and the pressure was∼1Torr. For variable temperature PL measurements,samples were mounted at the coldfinger and placed in a closed-cycle He cryostat(APD Cryogenics,Inc.model HC-2).The excitation source was a HeCd laser(325nm).The spectra were dispersed by a spectrometer SPEX500M and recorded by a photo-multiplier tube R943and PDA-512-USB(Control Development Inc.)fiberoptic spectrometer.Time-resolved PL was measured by using the Kerr-gated fluorescence technique17with1ps*To whom correspondence should be addressed.Tel:+852********. E-mail:dalek@hkusua.hku.hk.†Department of Physics,The University of Hong Kong.‡Department of Chemistry,The Univeristy of Hong Kong.§Department of Physics,The Hong Kong University of Science and Technology.20865J.Phys.Chem.B2006,110,20865-2087110.1021/jp063239w CCC:$33.50©2006American Chemical SocietyPublished on Web09/20/2006excitation pulses at 267nm.The decay times have been determined by fitting the decay curves monitored at the peak position of the UV emission (∼380nm).The decay times of the visible emission were considerably longer,of the order of nanoseconds,and thus could not be reliably determined,but it should be noted that annealing may affect both UV and visible emission decay times.It should also be noted that the ratios of emission peaks measured in different PL systems may be different due to the dependence of UV to defect emission ratio on excitation intensity.However,excitation intensity does not affect the peak positions.XPS spectra were obtained using PAL 102XPS,and the position of the carbon C 1s peak (285.0eV)was taken as a reference.In the positron annihilation experiments,low-energy positrons were focused to a ∼1mm diameter spot on the sample under magnetic guidance and the implantation energy of the positrons was varied between 0and 25keV.The principles of PAS have been reviewed in detail in refs 18and 19.The positrons implanted into the sample bulk would be rapidly thermalized and undergo diffusion.The diffusing positrons can be trapped by the neutral or negatively charged open volume defects or by the positron shallow traps (i.e.,ionized acceptors or disloca-tions).The positron would thus finally annihilate at the delocalized state or the localized defect state with a surrounding electron and emit a pair of gamma photons (511keV),which contain information about the electronic environment in which the positron annihilates.The Doppler broadening technique used in the present PAS study monitored the Doppler broadening of the 511keV annihilation radiation to obtain the so-called S parameter,which was defined as the ratio of the fixed central region of the 511keV annihilation peak to the total area of the peak.The measured S parameter represents a linear combination of the corresponding annihilation states,that is,S )Σf i S i ,where f i and S i are the fraction and the characteristic S parameter of the positrons annihilating at a state i .Thus,the measured S parameter is related to the fraction of positrons annihilating with the valence electrons.Because a positron annihilating from the trapped vacancy state usually has a higher overlap with the valance electrons which possess higher momentum with respect to the core electrons,the S parameter corresponding to the positron annihilating in the defect state is higher than that in the delocalized bulk state.On the other hand,the positron annihilating from the shallow trap state experiences a very similar electronic environment to the bulk and thus the corre-sponding S parameter is indistinguishable from the bulk value.Effective positron diffusion length L eff +,which is related to the mobility of the positron,was another parameter that could offer information of the positron trapping process,since trapping in a vacancy state or a shallow trap state reduces the L eff +value.The experimental data for “low-momentum”S parameter S (E )were fitted by the computer program VEPFIT,20taking into account positron implantation,positron diffusion,and positron annihilating at different possible sites.Relevant parametersobtained by positron annihilation experiments for ZnO nanorods (as-grown and annealed at different conditions)are summarized in Table 1.3.Results and Discussions3.1.Electron Microscopy Characterization.Figure 1shows the morphology of ZnO nanorods.It can be observed that the rods are ∼800nm long with diameters in the range from 55to 70nm,while the rod density is of the order of several hundred rods per µm 2.SEM images do not indicate significant changes of the nanorod morphology after annealing.To obtain better information on the nanorod structure and morphology,TEM measurements have been performed and the obtained results are shown in Figure 2.All of the rods grow along the [0001]direction.It can be observed that annealing in an oxidative environment (air,oxygen)does not affect the morphology of the nanorods but annealing in a reducing environment (forming gas)causes some surface damage and thinning of the rods.3.2.Photoluminescence and Positron Annihilation Spec-troscopy.While electron microscopy can provide information on overall morphology and structure of the nanorods,other experimental techniques are needed to study point defects.Thus,PL,time-resolved PL,PAS,and XPS have been used to examine the defects in ZnO nanorod arrays before and after annealing.It can be observed that annealing significantly affects the optical properties of the nanorods.Photoluminescence spectra have been measured at different temperatures to obtain more information about the nature of point defects in the rods.At low temperature,ZnO typically exhibits sharp bound exciton lines,commonly labeled I 0-I 11,6which correspond to the excitons bound to different donor and/or acceptor defects.The low-temperature (12K)PL spectra of the as-grown nanorods and nanorodsTABLE 1:Comparison of Properties of ZnO Nanorods Annealed under Different Conditions aannealing conditions L eff +(nm)S PL decay times O 1/Zn O 1/O 2(O 1+O 2)/Znnone2.80.54726ps,30ps 0.440.69 1.07600°C,air 140.54626ps,49ps 0.410.64 1.04600°C,oxygen 150.55421ps,47ps 0.390.58 1.05600°C,nitrogen 390.55626ps,61ps 0.420.46 1.35600°C,Ar280.55431ps,63ps 0.460.55 1.31600°C,forming gas 200.55726ps,120ps 0.320.29 1.41200°C,forming gas 50.56824ps,74ps 0.410.58 1.11400°C,forming gas240.55423ps,57ps0.440.411.50aThe areas of XPS peaks have been adjusted by the sensitivity factors,0.733for oxygen and 2.768forZn.Figure 1.Representative SEM image of the ZnO nanorods:(a)top view and (b)side view.20866J.Phys.Chem.B,Vol.110,No.42,2006Tam et al.annealed in different atmospheres exhibit considerable differ-ences,as shown in Figure 3.The spectra have been normalized for easier comparison of peak positions and peak widths.It can be observed that the as-grown nanorods exhibit a broad,asymmetric,featureless emission with a maximum at 3.379eV and a full-width at half-maximum (fwhm)of 25meV.Broad,featureless emission is expected in the case of a large defect concentration since energy band instead of discrete defect levels would be formed.On the other hand,the annealed samples exhibit sharp and narrow donor-bound exciton peaks.For all annealing conditions,the spectra are dominated by donor-bound exciton peaks,but the peak positions and consequently the identities of the donors are different.The PL intensity of the UV emission is also considerably enhanced after annealing,in agreement with the increase in the decay time (see Table 1).For annealing in air,the main peak is located at 3.364eV (fwhm )6meV)with a small shoulder at 3.369eV.The forming gas-annealed sample exhibits a peak at 3.366eV (fwhm )7meV)and a shoulder at 3.371eV,while the sample annealed in Ar shows only a peak at 3.366eV (fwhm )6meV).The sampleannealed in nitrogen exhibits a slightly broader peak with a fwhm of 9meV,which is centered at 3.368eV.All of the observed peaks are in good agreement with previously reported donor-bound exciton lines.6The weak peak at ∼3.337eV is likely a two-electron satellite of the donor-bound exciton peak or possibly related to excitons bound to structural defects.5To obtain more information about the nature of defects in the nanorods,we performed detailed investigation on the influence of annealing in an oxidative (air)and a reducing (forming gas)environment.Figure 4shows the variable tem-perature PL spectra of as-grown nanorods (parts a and b of Figure 4),nanorods annealed in air (parts c and d of Figure 4),and nanorods annealed in forming gas (parts e and f of Figure 4).It can be observed that the UV emission intensity is the lowest for as-grown nanorods and the highest fornanorodsFigure 2.Representative TEM images of ZnO nanorods:(a)as-grown,(b)annealed in forming gas at 600°C,(c)annealed in air at 600°C,and (d)annealed in oxygen at 600°C.The insets show corresponding HRTEMimages.Figure 3.12K PL spectra of ZnO nanorods annealed in different atmospheres.The spectra have been normalized for easier comparison of peakpositions.Figure 4.Temperature dependence of the UV and defect emission for ZnO nanorods (a and b)no annealing,(c and d)annealed in air,and (e and f)annealed in forming gas.ZnO Nanorods Prepared by a Hydrothermal Method J.Phys.Chem.B,Vol.110,No.42,200620867annealed in forming gas.The PL intensity in the UV spectral range at12K in the case of as-grown nanorods is∼67times smaller than that of nanorods annealed in forming gas(600°C) and∼41times smaller than that of nanorods annealed in air (600°C).We can also clearly observe different behavior of defect emissions with temperature.As-grown nanorods exhibit a peak shift and decreasing intensity of the defect emission with increasing temperature.After annealing in air at600°C,red and green emission peaks can be observed.The red emission decreases significantly with increasing temperature,while green emission intensities remain similar at all temperatures.After annealing in forming gas,there is no change in green emission intensity up to∼150K and then there is a small increase at 200and300K.Both green and red emissions show a negligible peak shift with increasing temperature.A different dependence on measurement temperature of the defect emissions observed indicates different origins of these emissions.It should also benoted that although emergence of the red band has been previously reported for ZnO single crystals annealed in air,16 the temperature dependence of the green and red bands was different from the one observed in nanorods.While the green emission in air-annealed ZnO single crystal quenched rapidly above200K,16this was not the case for ZnO nanorods.In addition,the dependence of green emission intensity on tem-perature cannot be described by the commonly used expressions since it does not decrease with increasing temperature.On the other hand,red and yellow defect emission intensities decrease with increasing temperature as expected.The PL emission intensity temperature dependence is usually described with21,22where R is the process rate parameter,E T is the activation energy,k is the Boltzmann constant,and T is temperature.The expression with two different activation energies E T1and E T221-23is also commonly used.Equation2can be used to describe two competitive nonradiative channels21or to describe different behaviors in low-temperature and high-temperature regions.22,23 The value of the activation energy dominant at low temperatures, E T1,is commonly very low.22,23One possible interpretation of this is that such a low value would be expected to occur as a result of donor ionization in the case of a donor-acceptor pair emission.22However,it has been shown that the small values of E T1are a consequence of T-2temperature dependence of the capture section for carriers at recombination centers.22 Figure5shows the temperature dependence of the emission intensity of the yellow and red bands(yellow for as-grown rods, red for rods annealed in air and forming gas).The temperature dependences can be fitted with eq2.For E T1,small values(∼6 meV for as-grown and rods annealed in air and16meV for rods annealed in forming gas)are obtained,in agreement with the literature.22Thermal activation energies E T2were253meV for yellow emission,47meV for red emission of samples annealed in air,and209meV for red emission of samples annealed in forming gas.For both annealing atmospheres,the emission in the visible spectral range could be decomposed into two Gaussian peaks,one in the red and one in the green spectral region.However,different activation energies indicate that despite the similarities of peak positions,the samples annealed in different atmospheres have very different nonradiative recombination rates and consequently different types and concentrations of point defects.The same conclusion can be reached from the comparison of the decay times of the UV emission(Table1).It should also be noted that,for the sample annealed in forming gas,there is an increase in green emission after the red emission is quenched(opposite to the air-annealed ZnO single crystals,where quenching of green emission leads to the rise in red emission16)which indicates possible competi-tion for holes between two different acceptor levels.16A similar phenomenon is also observed for blue and yellow defect emission bands in GaN.24The difference in temperature behavior of two different GaN samples(increase vs no increase of emission from one channel after the decrease of emission from the other channel),similar to that observed in air-and forming gas-annelaed ZnO nanorods,was explained by the differences in nonradiative recombination rates between the two samples.24 We have also investigated the influence of annealing tem-perature on the PL spectra.The room-temperature PL spectra for nanorods annealed in air and forming gas flow are shown in parts a and b of Figure6,while the comparisons between different annealing atmospheres at200and600°C are shown in parts c and d of Figure6.As-grown nanorods exhibit the UV emission peak and the broad yellow defect emission.They also have short PL decay times and a very short effective positron diffusion length.Since the effective positron diffusion length represents a measure of how much positrons trap into all of the defects,including both trapping and scattering defects, the short diffusion length for the as-grown sample is in good agreement with its poor luminescent properties.On the other hand,the S parameter is sensitive only to certain types of trapping defects,which in ZnO are Zn vacancy and vacancy clusters or complexes because O vacancy represents a weakly bound state similar to the ionized acceptor and the dislocation.25 Thus,the corresponding S parameter would not differ signifi-cantly from the bulk state.25This implies that a large measured S parameter would be associated with more positron events originating from the Zn vacancy and/or the vacancy cluster.On another hand,a low value of L eff+implies that more positrons are trapped in the various kinds of defects in ZnO,namely,O vacancy,Zn vacancy,vacancy cluster,and ionized acceptors. The high value of the S parameter and the short diffusion length in ZnO nanorods as compared with that of the ZnO epilayer grown on sapphire(measured to be0.502(0.002and76(1I(T))I1+R exp(-ET/kT)(1)I(T))I1+R1exp(-ET1/kT)+R2exp(-ET2/kT)(2)Figure5.Temperature dependence of the intensities of different visibleluminescence lines in ZnO.20868J.Phys.Chem.B,Vol.110,No.42,2006Tam et al.nm)indicate that there is a large concentration of the vacancy type defects in the samples.With the increase of annealing temperature,the positron diffusion length L eff +increases and so do the decay times obtained from time-resolved measurements.All of the samples exhibit biexponential decay,and the slow component exhibited a substantial increase after annealing,as shown in Table 1.After annealing at 200°C,the ratio of UV to visible emission is improved,with samples annealed in forming gas showing negligible defect emission,which is commonly considered as an indication of excellent crystal quality and low defect concentration.However,although L eff +has been increased after annealing at 200°C,it is still considerably lower than that of samples annealed at higher temperatures which exhibit larger defect emission.Also,annealing the samples in N 2,Ar,oxygen,or forming gas results in a higher value of the S parameter implying more Zn vacancy or vacancy cluster formation.However,the increase of the S parameter upon annealing in these samples was accompanied by the increase of L eff +,which could only be explained by the decrease in concentrations of weakly binding positron states such as O vacancy,ionized acceptor,or dislocations.It was plausible to conclude that annealing the samples in N 2,Ar,oxygen,and forming gas would have the effect of increasing the concentrations of Zn vacancy and/or vacancy cluster and decreasing the concentrations of O-vacancy,ionized acceptor,and/or dislocation.For the case of annealing in air,the S parameter had no significant change and L eff +increased with respect to the as-grown one,which implied no change in Zn vacancy/vacancy cluster concentration,while the concentration of weakly binding positron traps was slightly decreased.However,there is no obvious,direct relation-ship between the L d +and PL decay times,in agreement with a previous study on ZnO epitaxial layers.26Positron annihilation experiments also exhibit a lack of obvious correlation with visible emission,in agreement with a previous study which assigned visible emission to interstitial defects (zinc for green and oxygen for yellow emissions).27Imperfect correlation between L d +and radiative lifetime may indicate that crystal imperfections play a significant role in obtained results,similar to GaN where imperfect correlation between L d +and dislocation density,as well as the radiative lifetime and dislocation density,was found.28The lack of obvious relationship between L d +and the PL decay times is likely due to the large number of possible defects in ZnO.While L d +is a measure of total defect concentration,UV emission PL decay times are sensitive to defects which participate in carrier relaxation following optical excitation.While for all annealing atmospheres defect emission is reduced by annealing at 200°C,the UV to visible emission ratio worsens for annealing at 400°C and then improves again for 600°C.The defect peak shape is strongly dependent on the annealing atmosphere.After annealing in air at 400°C and 600°C,green and orange-red components can be observed.On the other hand,the samples annealed in forming gas show almost no visible emission for annealing at 200°C,while green emission appears for annealing at 400and 600°C.The samples annealed at 400°C exhibit longer L d +but shorter PL decay times and stronger defect emissions compared with samples annealed at 200°C.One possible explanation for this is that the nonradiative defect concentration is lower,but the inferior UV emission is caused by an increased career transfer to deep defect levels followed by their radiative relaxation and conse-quent decrease in the intensity ratio of UV and defect emissions.3.3.XPS Characterization.To clarify the issue of the possible origin of the observed defect emissions,XPS measure-ments were performed.The results obtained are shown in Figure 7and summarized in Table 1.The curves for samples annealed in air at 200°C (similar result as forming gas annealing at 200°C)and forming gas at 400°C (similar result as forming gas annealing at 600°C)have been omitted to improve the image clarity.The lower energy peak in the O 1s spectrum can be attributed to oxygen ions in the wurtzite ZnO structure,and the position of this peak in different reports was from 529to 530.7eV.29-31The higher energy peak,at 531-532eV,29-31is typically assigned to loosely bound oxygen on the surface,such as OH groups,30,31or O 2-ions in oxygen deficient regions.29Two peaks (O 1and O 2)are sufficient to achieve a good fit for O 1s spectra in all cases,although the shift of the higherenergyFigure 6.Room-temperature PL spectra of ZnO nanorods for:different annealing temperatures for samples annealed in the following:(a)air and (b)forming gas,(c)different annealing atmospheres for annealing at 200°C,and (d)different annealing atmospheres for annealing at 600°C.Figure 7.XPS spectra of ZnO nanorods annealed under different conditions:(a)O 1s (b)Zn 2p 2/3.ZnO Nanorods Prepared by a Hydrothermal Method J.Phys.Chem.B,Vol.110,No.42,200620869O2peak position for samples annealed in forming gas indicates a possible small contribution of an additional peak at higher energy(peak at∼533eV,attributed to water30,31).The peak shift of the O1s peak can also occur due to band bending.31As for the Zn2p3/2peak,small shifts to higher energy can be observed for all annealed samples.The O1/Zn ratio in all samples is lower than0.5,indicating oxygen deficiency in the bulk of the rods.3.4.Origins of Defect Emissions.The origin of the visible emission in ZnO is highly controversial.It has been shown that different defect centers are responsible for green,yellow,and red emissions.9,32Yellow and red emissions also exhibited different decay properties,which were attributed to different initial states(conduction band and shallow donors).8For the origin of green emission,a number of hypotheses have been proposed,such as singly ionized oxygen vacancy V o+,10V o2+ center,11oxygen antisite,12and zinc vacancy V Zn.13It has been suggested that the green emission is associated with oxygendeficiency,while the orange-red emission is associated with excess oxygen.5On the other hand,the red emission was also attributed to the interstitial zinc Zn i.14Yellow emission is commonly attributed to interstitial oxygen defects O i,3,4although some impurities such as Li could also contribute to this emission.4Unlike the green emission,the yellow emission was unaffected by surface modifications,4and assignment of the emission to O i has been confirmed by the reduction of this emission after annealing in a reducing environment.3,15Visible (green and yellow)emission in ZnO has also been attributed to the presence of Zn(OH)2on the surface.33,34Enhancement of the UV emission due to water desorption has also been demonstrated.35Hydrogen impurities also play a significant role in ZnO,36-38and the desorption temperatures of hydrogen and OH groups are different.35As-grown nanorods exhibit yellow defect emission,while after annealing typically red and green components are present in the emission spectra,and for some annealing conditions, defect emission becomes negligible.The yellow emission was reduced with annealing in all cases after annealing at temper-atures as low as200°C,although the decrease was lower in air.The desorption rate of hydroxyl groups peaks at∼150°C,35 and this desorption is likely responsible for the reduction of yellow defect emission after annealing at200°C.The assign-ment of this emission to the presence of hydroxyl groups is in agreement with previous reports on visible luminescence in ZnO nanocrystals.33,34Studies of the O-H local vibrational modes in ZnO also confirm that annealing at150°C results in the removal of OH groups,while H does not leave the sample for annealing at this temperature for30min.36However,O-H is only one type of hydrogen-related defect in ZnO,and the other defect has been shown to be thermally stable up to∼500°C.36 The study of hydrogen treatment of ZnO nanorods followed by annealing at400°C demonstrated that the presence of hydrogen improves the UV to visible emission ratio,while annealing at 400°C restores the original UV to visible emission ratio.37Thus, we can conclude that a decrease in the UV to visible emission ratio after annealing at400°C is most likely due to the removal of hydrogen.It should be noted that an increase in the defect (green)emission after hydrogen plasma treatment was also reported in ZnO,which was attributed to surface damage of the samples,similar to our results for forming gas annealing.38 Therefore,the yellow emission of the as-grown sample is likely due to the presence of OH groups,while worsening of the UV to visible emission ratio can be attributed to the removal of hydrogen.The green component of the emission(centered at∼2.47eV) is present in all annealed samples which exhibit defect emission. It is prominent in the samples annealed in forming gas,which exhibit evidence of surface damage(Figure2b).A similar observation has been reported for hydrogen plasma treatment of ZnO.38Thus,the green emission likely originates from surface defects,in agreement with previous reports.4,32The appearance of a green component of the emission is in most cases observed together with an increased positron diffusion length and S parameter,as well as lower O1/O2ratios.The increase in the S parameter with the appearance of green emission,as well as a decrease of O1/O2ratio,are in agreement with the involvement of V Zn14or V Zn complexes,as well as surface trapping by chemisorbed oxygen.11It should be noted that samples annealed in oxygen and argon at600°C also have similar values of the S parameter and thus similar concentrations of zinc vacancy related defects,but they exhibit a higher O1/O2ratio.Also, calculations of the energy levels of different defects predict that a transition involving V Zn would result in a blue emission.12,39 The summary of calculated energy levels from different studies12,39-41are shown in Figure8.It should be noted that the majority of calculations only predict energies of single point defects.While the energy of the V o Zn i complex has been calculated to be2.16eV below the conduction band minimum,39 energy levels of other defect complexes are unknown.Calcula-tions also predict that V Zn and V o have low formation energies,42 while Zn i is the most likely shallow donor candidate but it has a higher formation energy and it is a fast diffuser and thus not likely to be stable.42Electron irradiation experiments have shown that V Zn are a part of two different defect complexes and that one of those complexes anneals out simultaneously with neutral V O after annealing at∼550K.43Since all samples which exhibit green emission also exhibit a large S parameter value,while the opposite is not the case(i.e.,a large S parameter does not imply the existence of strong green emission),the likely origins of this emission are defect complexes related to V Zn and these defect complexes are mainly located at the surface.The assignment of this emission to a defect complex is also supported by the fact that single point defects,such as V Zn43and Zn i,42 are expected to be mobile at relatively low temperatures and thus would anneal out below600°C.Concerning red emission,it should be noted that there is a difference in the peak positions of the red components for samples annealed in forming gas(∼2.0eV)and samples annealed in air(∼1.8eV).A different peak position,together with different behaviors with increasing temperature,indicates Figure8.Energy levels of different defects in ZnO from different studies in the literature:(a)ref12,(b)ref40,(c)ref39,and(d)ref 41.V Zn,V Zn-,and V Zn2-denote neutral,singly charged,and doubly charged zinc vacancy,respectively.Zn i°and Zn i indicate neutral zinc interstitial while Zn i+denotes singly charged zinc interstial.V O°and V O denote neutral oxygen vacancy while V O+denotes singly charged oxygen vacancy.O i and H i represent oxygen and hydrogen interstitial, respectively.V O Zn i denotes a complex of oxygen vacancy and zinc interstitial.20870J.Phys.Chem.B,Vol.110,No.42,2006Tam et al.。