醇水混合溶剂中沉淀无机晶体的研究
乙醇和水分子形成配合物与荧光光谱特性研究
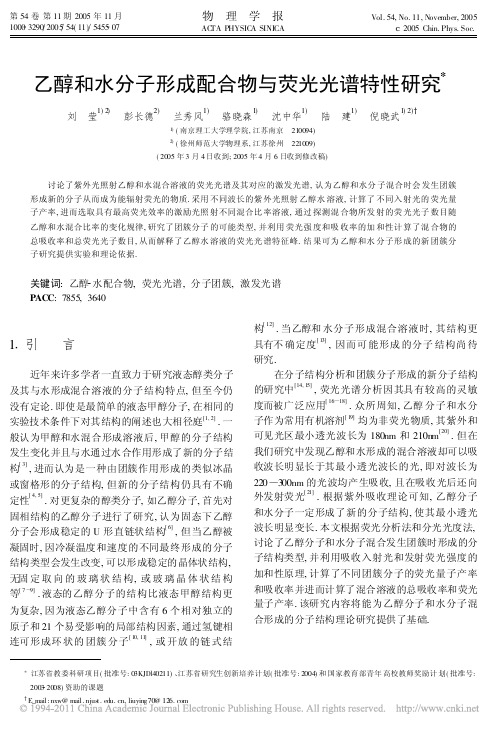
5458
物
理
学
报
54 卷
图4
236nm 的入射光激励不同浓度溶液的荧光光谱两维 ( a) 、 三维 ( b) 图
度, 则当入射光照射乙醇水混合溶液时 , 溶液对不同
4 讨
论
波长的入射光的总吸收度可以计算如下: A
1
1,
1
2, 2, 2,
1
3, 3, 3,
1
bc1 bc2 , bc3
1
4 1 利用激发光谱及光吸收度的加和性讨论三种 可能的配合物 由于乙醇和水混合后 , 对较长波长的紫外光产 生吸收并向外辐射荧光, 显然溶液中一定含有新的 物质 , 它是由乙醇和水分子缔合形成的配合物 . 此时 溶液中含有多种物质: 乙醇, 水 , 乙醇和水分子的配 合物等. 根据吸收光强度的加和性可知 , 在多组分体 系中 , 假设各种吸光物质间没有相互作用, 体系的总 吸收度等于各组分吸光度之和 , 即满足吸光度的加 [ 9] 和性 : A总 = A1 + A2 + + 光度; +
该激励光照射则没有谱峰出现. 从实验结果可以看 出, 当溶液的浓度变化时 , 体积百分比为 60% 左右 的乙醇水溶液的荧光发射强度最大 , 当体积百分比 小于 60% 时 , 荧 光光 子 数目 减少 , 但在 体 积比 为 40% 左右, 荧光光子数目出现锐减, 明显少于体积比 为 30% 的溶液所发射的荧光光子数目, 即此时出现 了荧光猝灭的现象 ; 同样当溶液中乙醇的体积百分 比大于 60% 时 , 溶液所发射的荧光光子数目总体趋 势减少, 但在体积比为 70% 80% 时荧光光子数目 锐减 , 其数目少于体积比为 90% 的溶液所发射的荧 光光 子 数 目, 即 当 溶 液 中 乙 醇 溶 液 的 体 积 比 为 70% 80% 时也出现了荧光猝灭现象.
各类成分性质实验报告(3篇)
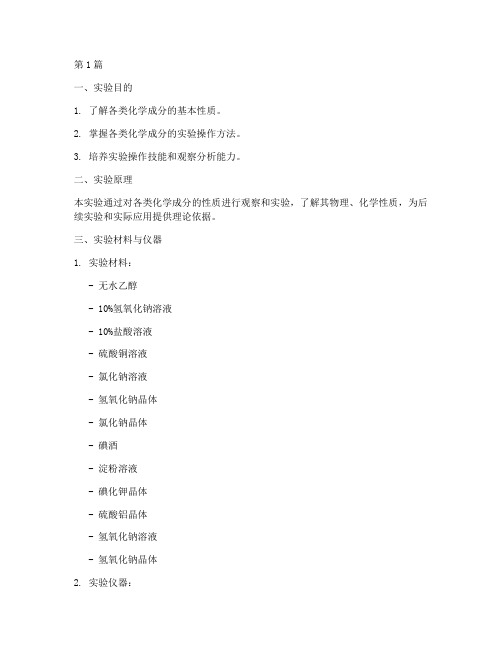
第1篇一、实验目的1. 了解各类化学成分的基本性质。
2. 掌握各类化学成分的实验操作方法。
3. 培养实验操作技能和观察分析能力。
二、实验原理本实验通过对各类化学成分的性质进行观察和实验,了解其物理、化学性质,为后续实验和实际应用提供理论依据。
三、实验材料与仪器1. 实验材料:- 无水乙醇- 10%氢氧化钠溶液- 10%盐酸溶液- 硫酸铜溶液- 氯化钠溶液- 氢氧化钠晶体- 氯化钠晶体- 碘酒- 淀粉溶液- 碘化钾晶体- 硫酸铝晶体- 氢氧化钠溶液- 氢氧化钠晶体2. 实验仪器:- 试管- 烧杯- 胶头滴管- 酒精灯- 玻璃棒- 滴定管- pH计- 显微镜四、实验步骤1. 无水乙醇的性质实验- 取少量无水乙醇于试管中,观察其颜色、气味、沸点等物理性质。
- 将无水乙醇与水混合,观察溶解度、分层现象等。
2. 氢氧化钠溶液的性质实验- 取少量氢氧化钠溶液于试管中,用pH计测定其pH值。
- 将氢氧化钠溶液与盐酸溶液混合,观察中和反应现象。
3. 硫酸铜溶液的性质实验- 取少量硫酸铜溶液于试管中,观察其颜色、溶解度等物理性质。
- 将硫酸铜溶液与氢氧化钠溶液混合,观察沉淀反应现象。
4. 氯化钠溶液的性质实验- 取少量氯化钠溶液于试管中,用pH计测定其pH值。
- 将氯化钠溶液与碘酒混合,观察颜色变化。
5. 淀粉溶液的性质实验- 取少量淀粉溶液于试管中,用碘酒滴定,观察颜色变化。
- 将淀粉溶液与碘化钾晶体混合,观察颜色变化。
6. 硫酸铝晶体的性质实验- 取少量硫酸铝晶体于试管中,观察其颜色、溶解度等物理性质。
- 将硫酸铝晶体与氢氧化钠溶液混合,观察沉淀反应现象。
五、实验结果与分析1. 无水乙醇:无色液体,具有特殊的气味,沸点为78.4℃。
与水混合后,溶解度较好,不分层。
2. 氢氧化钠溶液:无色液体,具有腐蚀性,pH值为14。
与盐酸溶液混合后,产生中和反应,溶液变中性。
3. 硫酸铜溶液:蓝色溶液,溶解度较好。
与氢氧化钠溶液混合后,产生蓝色沉淀。
硫酸铜和氨水反应生成深蓝色晶体加乙醇的原理
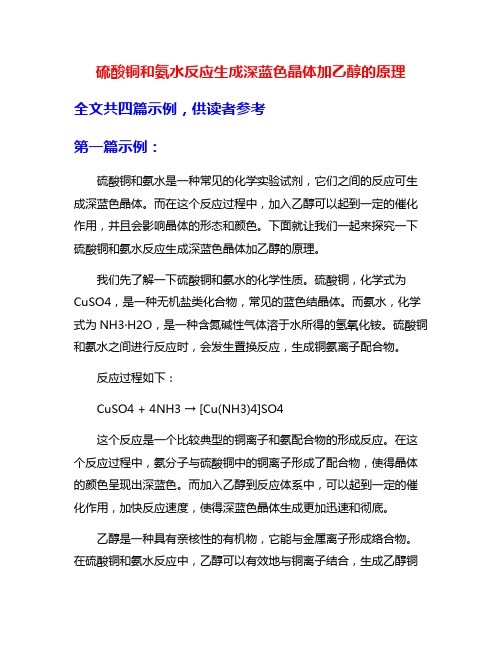
硫酸铜和氨水反应生成深蓝色晶体加乙醇的原理全文共四篇示例,供读者参考第一篇示例:硫酸铜和氨水是一种常见的化学实验试剂,它们之间的反应可生成深蓝色晶体。
而在这个反应过程中,加入乙醇可以起到一定的催化作用,并且会影响晶体的形态和颜色。
下面就让我们一起来探究一下硫酸铜和氨水反应生成深蓝色晶体加乙醇的原理。
我们先了解一下硫酸铜和氨水的化学性质。
硫酸铜,化学式为CuSO4,是一种无机盐类化合物,常见的蓝色结晶体。
而氨水,化学式为NH3·H2O,是一种含氮碱性气体溶于水所得的氢氧化铵。
硫酸铜和氨水之间进行反应时,会发生置换反应,生成铜氨离子配合物。
反应过程如下:CuSO4 + 4NH3 → [Cu(NH3)4]SO4这个反应是一个比较典型的铜离子和氨配合物的形成反应。
在这个反应过程中,氨分子与硫酸铜中的铜离子形成了配合物,使得晶体的颜色呈现出深蓝色。
而加入乙醇到反应体系中,可以起到一定的催化作用,加快反应速度,使得深蓝色晶体生成更加迅速和彻底。
乙醇是一种具有亲核性的有机物,它能与金属离子形成络合物。
在硫酸铜和氨水反应中,乙醇可以有效地与铜离子结合,生成乙醇铜络合物,促进铜氨离子配合物的形成反应。
乙醇还可以提供一定的缓冲作用,调节反应体系的pH值,使得反应更加平稳和高效。
在实际实验操作中,通常将硫酸铜和氨水按一定比例混合在一起,然后加入少量的乙醇,搅拌均匀后便会观察到深蓝色晶体的生成。
这些深蓝色晶体正是由铜氨离子配合物组成的,其颜色深浅和形态大小会受到乙醇的影响。
硫酸铜和氨水反应生成深蓝色晶体的过程是一个有机物和无机物相互作用的过程。
而加入乙醇可以起到催化作用和调节作用,促进反应的进行并影响晶体的形态。
这一过程不仅展示了化学反应的奇妙之处,也为我们提供了一种学习和研究的良好范例。
希望通过对这一反应原理的深入了解,能够帮助大家更好地掌握化学实验技巧和知识。
【2000字到此结束,如有不足还请谅解】。
第二篇示例:硫酸铜和氨水是一种常见的化学试剂,它们在反应中能够生成深蓝色的晶体。
采用基于乙醇体系的一步草酸共沉淀法制备层状富锂锰基正极材料
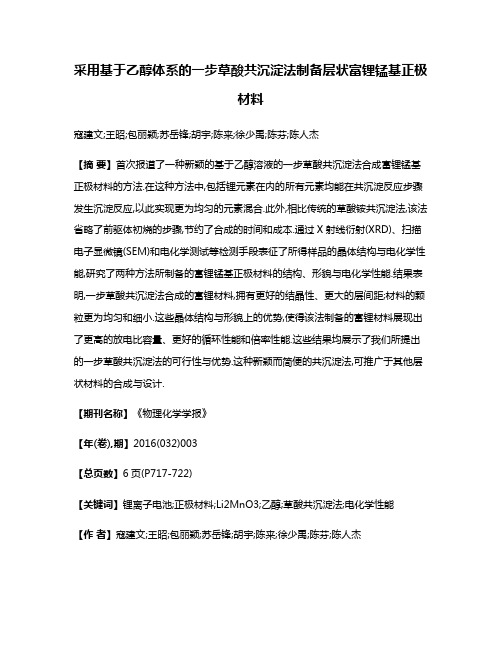
采用基于乙醇体系的一步草酸共沉淀法制备层状富锂锰基正极材料寇建文;王昭;包丽颖;苏岳锋;胡宇;陈来;徐少禹;陈芬;陈人杰【摘要】首次报道了一种新颖的基于乙醇溶液的一步草酸共沉淀法合成富锂锰基正极材料的方法.在这种方法中,包括锂元素在内的所有元素均能在共沉淀反应步骤发生沉淀反应,以此实现更为均匀的元素混合.此外,相比传统的草酸铵共沉淀法,该法省略了前驱体初烧的步骤,节约了合成的时间和成本.通过X射线衍射(XRD)、扫描电子显微镜(SEM)和电化学测试等检测手段表征了所得样品的晶体结构与电化学性能,研究了两种方法所制备的富锂锰基正极材料的结构、形貌与电化学性能.结果表明,一步草酸共沉淀法合成的富锂材料,拥有更好的结晶性、更大的层间距;材料的颗粒更为均匀和细小.这些晶体结构与形貌上的优势,使得该法制备的富锂材料展现出了更高的放电比容量、更好的循环性能和倍率性能.这些结果均展示了我们所提出的一步草酸共沉淀法的可行性与优势.这种新颖而简便的共沉淀法,可推广于其他层状材料的合成与设计.【期刊名称】《物理化学学报》【年(卷),期】2016(032)003【总页数】6页(P717-722)【关键词】锂离子电池;正极材料;Li2MnO3;乙醇;草酸共沉淀法;电化学性能【作者】寇建文;王昭;包丽颖;苏岳锋;胡宇;陈来;徐少禹;陈芬;陈人杰【作者单位】北京理工大学材料学院,北京100081;北京理工大学材料学院,北京100081;北京理工大学材料学院,北京100081;北京电动车辆协同创新中心,北京100081;北京理工大学材料学院,北京100081;北京电动车辆协同创新中心,北京100081;北京理工大学材料学院,北京100081;北京理工大学材料学院,北京100081;中国北方车辆研究所,北京100072;中国北方车辆研究所,北京100072;北京理工大学材料学院,北京100081;北京电动车辆协同创新中心,北京100081【正文语种】中文【中图分类】O646Transportable devices driven by Li-ion cells such as cell phones, laptop computers,and uninterrupted power supplies(UPS)have become indispensable parts in our daily life for the pastdecades. To fulfill the requests of these applications,efficient cathode materials are required to fabricate high performance Li-ion batteries w ith low mass density,reliable safety,and high energy density1.Recently,layered lithium-rich(LR)cathodematerials, Li2MnO3-LiMO2(M=Mn,Ni,Co,etc.)have been extensively studied as a new generation of prom ising cathodematerials for advanced Li-ion batteries thanks to theirhigher reversible capacity (ca250mAh∙g-1),lower cost,and lessenvironmental impactor2-7.The electrochemical performance of thesematerials strongly dependsupon the selected syntheticmethod,which determines the uniform and homogeneousdegree of finalproducts8.In previous researches,hydroxide coprecipitation instead of solid state reaction is w idely used to produce uniform and homogeneous transition metal precursors9-12.However,thismethod is less competitive when producing precursors with high manganese contentbecause Mn2+can be easily oxidized to Mn3+athigh pH value,formingmanganese oxyhydroxide(MnOOH)and leading to a deteriorative performance13.Carbonate coprecipitation,asan alternativemethod,could keep the stateof the cationsas+2 forall transitionmetals14-18.But the samples synthesized by thismethod usually have a low er tap density than those by hydroxide coprecipitation.More recently,Zheng et al.19reported the direct correlation between voltage fadeand differentsyntheticmethods,and they found that thematerialsprepared by the coprecipitation and sol-gelmethodsexhibita faster voltage fade and poorer cycling stability than thematerials prepared by the hydrothermal assisted method.This strongly implied that the electrochemical characteristics of LRmaterialsare prone to be affected by preparation condition.In our previous research,ammonium oxalate was applied to serve as the precipitator to replace the hydroxide and carbonate in aweak-acidic aqueoussolution20.Theexperimental conditionsof thismethod aremuch less harsh,and the oxidation state of the cations can be easily keptat+2 for all transitionmetals.However, such an ammonium oxalate coprecipitationmethod,like other coprecipitationmethods,stillneed to prepare the precursors firstly, and thenmixed with lithium salts followed by calcination treatment.Such complicated process is costive,time consuming,and may further cause a propensity of nonuniform ly distribution of transitionmetals.Herein,we presentanovelethanol-basedonestep oxalate coprecipitationmethod for precipitating all theelements including Liduring the coprecipitation reaction,to realize a more homogeneous particle morphology and give a better electrochemistry performance.Li1.2Mn0.54Ni0.13Co0.13O2is used here asan example to verify the feasibility of thenew method.Ourapproach isshown in Fig.1.All the raw reagents employed here w ere of analytical purity grade.The ammonium oxalate coprecipitationmethod is the same as reported in our previous research20.In this ammonium oxalate coprecipitation method, Ni(NO3)2(Aladdin),Co(NO3)2(Aladdin),andMn(NO3)2(Aladdin, 50%aqueoussolution)with amole ratio of 1:1:4.15and stoichiometric(NH4)2C2O4were dissolved in deionizedwater,respectively.Both of the solutionswere dropped into a strongly stirred beaker.Precipitate w as separated from the solution by vacuum filtration and dried at80°C for 12 h.A ftermixingw ith a stoichiometric amountof lithium hydroxide,the dried powder w aspresintered at450°Cfor5h,and ground after cooling.Finally,the decomposedm ixturewas pressed into pelletsand calcined at900°C for12 h to obtainLi1.2Mn0.54Ni0.13Co0.13O2(hereafter named assampleA).While in the one-step oxalate coprecipitation method,Li(NO3)2(A laddin)w as simultaneously added into the transition solution,and H2C2O4was chosen as the precipitator. Both of these two solutionswere dissolved in ethanoland dropped into a strongly stirred beaker to synthesize the precursors.After filtration and drying,the obtained pow derwas directly pressed into pellets and calcined at900°C for 12 h to obtain the finalproduct(hereafternamed assample B).A structural analysis was carried out using X-ray diffraction (XRD,Rigaku Ultima IV-185)with a Cu Kαradiation source.The sampleswere scanned from 2θ=20°to 2θ=80°ata scan rateof 2(°)∙m in-1.To analyze the samplemorphology,FEIQUANTA 250field em ission scanning electronm icroscope(FESEM)w as used.Electrochem icalperformancesof the sampleswere examined with CR2025coin type cells.The positive electrodeswere made of 80%(w,mass fraction)as-prepared materials pow der, 10%(w)acetylene black,and 10%(w)polyvinylidene fluoride. The electrolytewas 1mol∙L-1LiPF6dissolved in amixture of ethylene carbonate and ethylmethyl carbonate(1:1,volume ratio).The coin-type half cells,which chose lithium metalas the negativeelectrode and Celgard2400membraneasseparator,were assembled in aglove box filled with argon.Electrochemical tests were performed by CT2001A Land instrument(Wuhan,China)atpotential range of 2.0-4.8 V.The currentdensity of 250m A∙g-1wasdefined as 1C rate during thetest.Electrochemical impedance spectra(EIS)of the cellswerealso conducted using the CHI660electrochemicalworkstation at frequencies from 105Hzto0.01Hz w ith an AC perturbation signal of 5mV.And the resultswere analyzed using ZSimpW in softw are.The X-ray powder diffraction(XRD)patternsof samplesA and B,aswellas their lattice parameters are shown in Fig.2.Clearly, XRD patternsof both the samplesA and B arewell in dexedwith α-NaFeO2layered structure(R3ˉm space group)except for some weak superlattices reflectionsaround 2θ=20°-25°caused by Li+/ Mn4+cation ordering in the transitionmetallayers5,21.The ratio of c/a of both samples A and B is higher greater than 4.9,which indicated the formation of ahighly ordered lamellar structure22. While the(003)and(104)peak intensity ratio of I(003)/I(104)(bigger than 1.2)means that the materials have less cation disorder23. Besides,for sample A,themain XRD peaks corresponding to the LiMnO3-like super latticesarea slightly weaker andw ider than that thoseof sample B.In conclusion,theabsenceof presintered at 450°C in one-step oxalate coprecipitation method will not influence the crystallinity of sample B.On the contrary,sample B showsahigher crystallinity from the ratio of c/a and I(003)/I(104).Fig.3 compares SEMimagesof the Li[Li0.2Mn0.54Ni0.13Co0.13]O2particles synthesized by the two coprecipitationmethods.Itcan be clearly seen from the SEMimages thatbothof thematerialsare quite homogeneous in particle sizes with inerratic bulk morphology.However,particles in sample B areof smaller sizes.The significantly reduced dimensionsof smaller particlesof sample B may largely increase the rate of lithium insertion/removal;and their higher surface area could perm it a higher contactareaw ith the electrolyte and hence a high lithium-ion flux across the interface24. Fig.4shows electrochem ical performancesof samples A and B. Fig.4a shows the initial and 80th charge-discharge curves at25mA∙g-1(0.1C rate)of the samplesA and B.Both of them present a typicalpotentialplateau of LRmaterials atabout4.5V regions at the initial cycle,which is originated from the electrochemical activation of Li2MnO3phase25-27.This plateauatabout4.5V of sample B is longer than that of sample A,indicating amore completeactivation of Li2MnO3phase,whichmay benefit from its smaller particle sizes.For the same reason,sample B also shows a lowerelectrode polarization than sample A.Naturally,sample B deliversamuch higherdischarge c apacity(286.1mAh∙g-1)than sample A(242.5mAh∙g-1).Both of them suffer from obvious capacity drop and voltagedecay after80cycles,whichmay be due to the increased interface reaction impedanceand layered-to-spineltransformation.However,theattenuation trend in sample B is less obvious.The corresponding discharge differential capacity(d Q/ d V)plotsof Fig.4a are illustrated in Fig.4b.The reduction peaks atabout3.75V isgenerally considered as the reduction of Ni4+orCo4+ions in the rhombohedralphase,while the peaksatabout3.3 V areassociatedwith reduction ofMn4+from LiMO2phase28.It is clearer in Fig.4b thatsample B presents lessened voltage decay.Cycling performance is shown in Fig.4c.A fter 80cycles,a reversible capacity of 231.3m Ah∙g-1of sam ple B could still be maintained,w hich ismuch higher than that of samp le A(186.1 mAh∙g-1).Herewe choose3.5V asademarcation line to separate the discharge capacity into two parts for comparison:one is the capacity from the reduction of Ni4+orCo4+above3.5V,and the other is from the reduction of Mn4+below3.5V29,30.Due to the layered-to-spinel transformation,the discharge capacity of both the samplesabove3.5V decreasesmonotonously during cycling as shown in Fig.4c.Before about50cycles,this decrease isaccompanied by an increase of discharge capacity below 3.5V forsamples A and B due to increased formation of the spinel-like phase.While after 50cycles,sample A exhibits continuous capacity decay below 3.5V,imp lying a deteriorated structure for lithium ion storage.On thecontrary,sample B exhibits a capacity increase in the region below3.5V,indicating excellentcapacityretention.These advances in discharge capacity and cycling performance of sample B shows the advantages of the novel method we presented here.Fig.4d compares the rate performances of the two samples.Itcan be seen that the discharge capacity of the sample B atany rates isalwayshigher than thatof the samplebinedw ith the lattice parameters in Fig.2 and results ofFig.3,thebetter rate performanceof sample Bmay partly own to its higher c value and smaller sizes,which indicates thatsam ples synthesized by one-step oxalate coprecipitation method could providemoreopen and fasterLi+transportchannels.To gain a better understanding of factors leading to the differences in rate capabilitiesof the two samples,EISmeasurements have been carried out after 3 cyclesas shown in Fig.5.A possible equivalent circuit is also proposed,and the fitting data of the sampleA and the sample B are shown in Table 1.Before the EIS measurements,all the sampleshavebeen charged to 50%stateof charge(SOC)to reach an identicalstatus.The Nyquistplots for the electrodes in Fig.5shows sim ilar shapes.They are com posed of two semicircles in thehigh frequency,which isassociatedwith the resistance for lithium ion diffusion in solid electrolyte interface (SEI)layer(Rs)and the charge transfer resistance(Rct)31,32,and a quasi-straight line in the lowfrequency corresponding toWarburg im pedance(Zw).As seen in Table1,the Rsof the sam ple B is slightly larger than thatof thesampleA.However,the sample B showsamuch smaller Rctcompared to the sampleA.The lower surface charge-transfer resistance of samp le B should also be responsible for itsbetterhigh-rate performance.Layered lithium-rich cathodematerialsnamed as samplesA and B were successfully synthesized by conventional ammonium oxalate coprecipitationmethod and anovelone-step oxalate coprecipitationmethod,respectively.XRD results shows thatsample B owns better crystallinity and larger interlayer spacing.SEMimages shows thatparticlesof sample B aremorehomogeneous andsmaller.Electrochemical tests reveal that samples synthesized by one-step oxalate coprecipitationmethod exhibitmuch higher discharge capacity,better cycle performance,better rate performance,and smaller Rct.And these enhancements in electrochem ical performances indicate the advantagesof one-step oxalate coprecipitation method,which may realize amore uniform ity cation distribution in sample B.This novel and convenient coprecipitation method we developed here introduces a possible strategy to realize amore uniform transitionmetal distribution in layered materials,which may open a great opportunity form itigating voltage fade in lithium-rich cathodematerials.References(1)Dunn,B.;Kamath,H.;Tarascon,J.M.Science2011,334(18),928.doi:10.1126/science.1212741(2)Chen,L.;Su,Y.F.;Chen,S.;Li,N.;Bao,L.Y.;Li,W.K.;Wang,Z.;Wang,M.;Wu,F.Adv.Mater.2014,26(39),6756.doi:10.1002/adma.v26.39(3)Lu,Z.;Beaulieu,L.;Donaberger,R.;Thomas,C.;Dahn,J.J.Electrochem.Soc.2002,149(6),A778.doi:10.1149/ 1.1471541(4)Lu,Z.;MacNeil,D.;Dahn,J.Electrochem.Solid ST.2001,4(6),A191.doi:10.1149/1.1407994(5)Thackeray,M.M.;Kang,S.H.;Johnson,C.S.;Vaughey,J.T.;Benedek,R.;Hackney,S.J.Mater.Chem.2007,17(30),3112.doi:10.1039/B702425H(6)Wu,F.;Li,N.;Su,Y.F.;Shou,H.F.;Bao,L.Y.;Yang,W.;Zhang,L.J.;An,R.;Chen,S.Adv.Mater.2013,25(27),3722.doi:10.1002/adma.201300598(7)Xu,B.;Fell,C.R.;Chi,M.;Meng,Y.S.Energ.Environ.Sci.2011,4(6),2223.doi:10.1039/c1ee01131f(8)Lee,M.H.;Kang,Y.J.;Myung,S.T.;Sun,Y.K.Electrochim. Acta2004,50,939.doi:10.1016/j.electacta.2004.07.038(9)Van Bommel,A.;Dahn,J.Chem.Mater.2009,21(8),1500.doi:10.1021/cm803144d(10)Liang,L.;Du,K.;Peng,Z.;Cao,Y.;Duan,J.;Jiang,J.;Hu,G. Electrochim.Acta 2014,130,82.doi:10.1016/j. electacta.2014.02.100(11)Kim,H.J.;Jung,H.G.;Scrosati,B.;Sun,Y.K.J.PowerSources2012,203,115.doi:10.1016/j.jpowsour.2011.11.076(12)Lim,J.H.;Bang,H.;Lee,K.S.;Amine,K.;Sun,Y.K. J.PowerSources2009,189,571.doi:10.1016/j. jpow sour.2008.10.035(13)Zhou,F.;Zhao,X.;van Bommel,A.;Rowe,A.W.;Dahn,J.Chem.Mater.2009,22(3),1015.doi:10.1021/cm9018309(14)Wang,D.;Belharouak,I.;Koenig,G.M.;Zhou,G.;Amine,K.J.Mater.Chem.2011,21(25),9290.doi:10.1039/c1jm11077b(15)Zhang,S.;Deng,C.;Fu,B.;Yang,S.;Ma,L.Powder Technol.2010,198(3),373.doi:10.1016/j.pow tec.2009.12.002(16)Lee,D.K.;Park,S.H.;Amine,K.;Bang,H.;Parakash,J.;Sun,Y.K.J.PowerSources2006,162,1346.doi:10.1016/j.jpowsour.2006.07.064 (17)Zhang,S.;Deng,C.;Yang,S.;Niu,pd.2009,484(1),519.doi:10.1016/j.jallcom.2009.04.149(18)Park,S.H.;Kang,S.H.;Belharouak,I.;Sun,Y.;Am ine,K. J.PowerSources2008,177,177.doi:10.1016/j. jpowsour.2007.10.062(19)Zheng,J.;Gu,M.;Genc,A.;Xiao,J.;Xu,P.;Chen,X.;Zhu,Z.;Zhao,W.;Pullan,L.;Wang,C.Nano Lett.2014,14(5),2628.doi:10.1021/nl500486y(20)Wu,F.;Lu,H.Q.;Su,Y.F.;Li,N.;Bao,L.Y.;Chen,S.J.Appl.Electrochem.2010,40(4),783.(21)Thackeray,M.M.;Johnson,C.S.;Vaughey,J.T.;Li,N.;Hackney,S.A.J.Mater.Chem.2005,15(23),2257.doi: 10.1039/b417616m (22)Kim,J.H.;Park,C.;Sun,Y.K.Solid StateIonics2003,164(1),43.doi:10.1016/j.ssi.2003.08.003(23)Ohzuku,T.;Ueda,A.;Nagayama,M.;Iwakoshi,Y.;Komori,H.Electrochim.Acta 1993,38,1159.doi:10.1016/0013-4686(93)80046-3(24)Bruce,P.G.;Scrosati,B.;Tarascon,J.M.Angew.Chem.Int.Edit.2008,47(16),2930.doi:10.1002/anie.200702505(25)Yabuuchi,N.;Yoshii,K.;Myung,S.T.;Nakai,I.;Komaba,S.J.Am.Chem.Soc.2011,133(12),4404.doi:10.1021/ ja108588y(26)Johnson,C.S.;Li,N.;Lefief,C.;Vaughey,J.T.;Thackeray,M.M.Chem.Mater.2008,20(19),6095.doi:10.1021/ cm801245r(27)Gu,M.;Genc,A.;Belharouak,I.;Wang,D.;Amine,K.;Thevuthasan,S.;Baer,D.R.;Zhang,J.G.;Browning,N.D.;Liu,J.Chem.Mater.2013,25(11),2319.doi:10.1021/ cm4009392(28)A runkumar,T.;Wu,Y.;Manthiram,A.Chem.Mater.2007,19(12),3067.doi:10.1021/cm070389q(29)Kou,J.W.;Chen,L.;Su,Y.F.;Bao,L.Y.;Wang,J.;Li,N.;Li,W.K.;Wang,M.;Chen,S.;Wu,F.ACSAppl.Mater.Inter.2015,7(32),17910.doi:10.1021/acsami.5b04514(30)Chen,L.;Chen,S.;Hu,D.Z.;Su,Y.F.;Li,W.K.;Wang,Z.; Bao,L.Y.;Wu,F.Acta Phys.-Chim.Sin.2014,30(3),467. [陈来,陈实,胡道中,苏岳锋,李维康,王昭,包丽颖,吴锋.物理化学学报,2014,30(3),467.]doi:10.3866/PKU. WHXB201312252(31)Yu,H.;Wang,Y.;Asakura,D.;Hosono,E.;Zhang,T.;Zhou,H.S.RSCAdv.2012,2(23),8797.doi:10.1039/c2ra20772a(32)Liu,X.;Li,H.;Yoo,E.;Ishida,M.;Zhou,H.S.Electrochim. Acta2012,83,253.doi:10.1016/j.electacta.2012.07.111。
MNO3(M=Na,K,Rb,Cs)在多元醇水混合溶剂中的溶解度、溶液密度和折光率比较研究
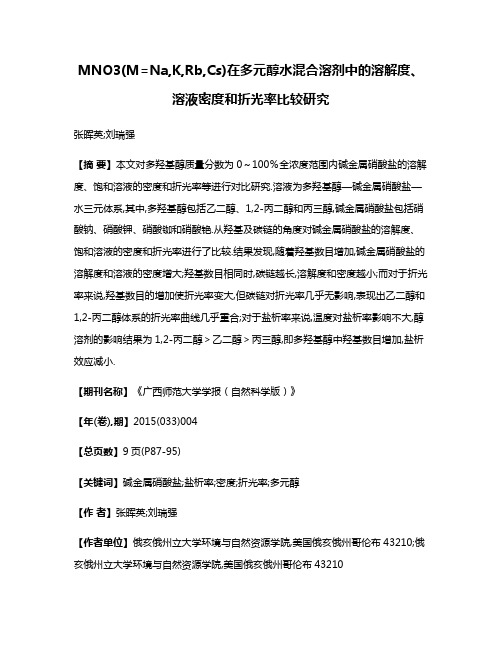
MNO3(M=Na,K,Rb,Cs)在多元醇水混合溶剂中的溶解度、溶液密度和折光率比较研究张晖英;刘瑞强【摘要】本文对多羟基醇质量分数为0~100%全浓度范围内碱金属硝酸盐的溶解度、饱和溶液的密度和折光率等进行对比研究.溶液为多羟基醇—碱金属硝酸盐—水三元体系,其中,多羟基醇包括乙二醇、1,2-丙二醇和丙三醇,碱金属硝酸盐包括硝酸钠、硝酸钾、硝酸铷和硝酸铯.从羟基及碳链的角度对碱金属硝酸盐的溶解度、饱和溶液的密度和折光率进行了比较.结果发现,随着羟基数目增加,碱金属硝酸盐的溶解度和溶液的密度增大;羟基数目相同时,碳链越长,溶解度和密度越小;而对于折光率来说,羟基数目的增加使折光率变大,但碳链对折光率几乎无影响,表现出乙二醇和1,2-丙二醇体系的折光率曲线几乎重合;对于盐析率来说,温度对盐析率影响不大,醇溶剂的影响结果为1,2-丙二醇>乙二醇>丙三醇,即多羟基醇中羟基数目增加,盐析效应减小.【期刊名称】《广西师范大学学报(自然科学版)》【年(卷),期】2015(033)004【总页数】9页(P87-95)【关键词】碱金属硝酸盐;盐析率;密度;折光率;多元醇【作者】张晖英;刘瑞强【作者单位】俄亥俄州立大学环境与自然资源学院,美国俄亥俄州哥伦布43210;俄亥俄州立大学环境与自然资源学院,美国俄亥俄州哥伦布43210【正文语种】中文【中图分类】O616自20世纪中期以来, 随着新的分离方法—萃取结晶法的开发[1],在萃取和结晶的理论和应用方面国内外学者相继开展了大量的研究工作[2-3]。
目前,萃取结晶法在生物物质分离[4-6]、分析检测[7-9]、煤的溶剂萃取[10-11]、金属分离[12-16]、环境保护[17-19]以及溶剂回收[20-23]等众多领域已得到广泛应用。
如:利用萃取结晶法将紫杉醇从红豆杉细胞两液相体系中分离出来[4],用混合溶剂法测定水中的铜[12],络合萃取法处理癸二酸生产中含酚废水[18]等。
乙醇溶液中碱沉淀制γ-Al2O3催化剂载体复习课程

乙醇溶液中碱沉淀制γ-A l2O3催化剂载体Article history:Received 17 November 2007Accepted 20 March 2008Available online 26 March 2008在乙醇中沉淀合成γ-Al2O3的研究Shaoyan Wang a,b, Xiaoan Li b, Shaofeng Wang c, Yang Li b, Yuchun Zhai摘要:用溶胶 - 凝胶法在乙醇中沉淀,然后经洗涤、干燥和煅烧处理制备纳米γ-Al2O3粉末。
控制反应物浓度,反应物的摩尔比和煅烧温度,用BET,TEM,XRD和IR对产品进行表征。
实验结果表明,用乙醇沉淀过程中难以避免结块,决定最终产物的表面积,其直径和相位组成的主要因素为焙烧温度。
当煅烧温度控制在686-1029°C之间,得到无团聚的纳米尺寸为5-9纳米的γ-Al2O3粒子。
关键词:制备,纳米γ-氧化铝,溶胶 - 凝胶沉淀,乙醇1引言氧化铝在技术和工业上具有巨大的应用。
其中存在多种亚稳态结构,包括γ,η,δ,θ,κ和χ-Al2O3,以及作为其稳定相的α-氧化铝 [1],这些相转变中,γ-Al2O3是其中一种极其重要的纳米材料。
它已被用于在汽车和石油工业中作为催化剂和催化剂载体,航天器的结构复合材料和磨料和热磨损涂层材料[2]。
最近的研究显示,当达到临界表面积时γ-氧化铝热力学稳定性接近α-氧化铝[3],且纳米γ-氧化铝粉末,可促进氧化铝和碳化硅纤维的烧结行为[4],与γ-和χ-Al2O3样品相比,使用单相的γ-氧化铝粉末使致密化温度降低 [5]。
这些结果可以开辟γ-氧化铝应用的无限可能性,所以这就是研究γ-氧化铝制备的重要意义。
迄今为止,大量的法已被用于制备γ-氧化铝。
如煅烧勃姆石的溶胶 - 凝胶合成法[2],多羟基铝聚乙烯醇[5]在氧气气氛中铝靶的激光烧蚀法[6],醇铝盐的水解法[7],硫酸铝盐热解法 [8],有机金属化合物Al(CH 3)3的化学汽相沉积法[9]。
乙醇自然结晶实验报告(3篇)

第1篇一、实验目的1. 了解乙醇在不同温度下的溶解度特性。
2. 通过自然结晶的方法,观察乙醇晶体的形成过程。
3. 掌握乙醇晶体的提纯方法。
4. 熟悉实验操作技能,提高实验操作规范。
二、实验原理乙醇的溶解度随温度的变化而变化,当温度降低时,乙醇在水中的溶解度会减小,从而析出晶体。
本实验通过逐渐降低乙醇溶液的温度,使乙醇从溶液中析出,形成乙醇晶体。
三、实验仪器与试剂仪器:1. 容量瓶(250mL)2. 烧杯(250mL)3. 温度计4. 玻璃棒5. 滤纸6. 烘箱试剂:1. 乙醇(95%)2. 纯净水四、实验步骤1. 准备250mL的乙醇溶液,加入烧杯中。
2. 将烧杯放置在室温下,用温度计测量乙醇溶液的温度,记录初始温度。
3. 将烧杯放入冰箱中,逐渐降低温度,每隔5分钟记录一次温度和乙醇溶液的浑浊程度。
4. 当观察到乙醇溶液出现浑浊时,继续降低温度,直至乙醇晶体析出。
5. 将烧杯从冰箱中取出,用玻璃棒轻轻搅拌乙醇溶液,观察晶体形态。
6. 用滤纸过滤乙醇晶体,收集晶体。
7. 将收集到的乙醇晶体放入烘箱中,于60℃下干燥1小时,得到纯净的乙醇晶体。
五、实验结果与分析1. 在室温下,乙醇溶液的初始温度约为20℃。
2. 随着温度的降低,乙醇溶液逐渐变得浑浊,最终在约10℃时,乙醇晶体开始析出。
3. 晶体形态为无色透明,呈针状或针簇状。
4. 干燥后的乙醇晶体质量约为1.2g。
六、讨论1. 实验结果表明,乙醇在不同温度下的溶解度不同,当温度降低时,乙醇的溶解度减小,从而析出晶体。
2. 通过自然结晶的方法,可以观察到乙醇晶体的形成过程,了解晶体的生长规律。
3. 本实验成功地将乙醇从溶液中析出,并得到了纯净的乙醇晶体。
4. 在实验过程中,应严格控制温度,避免温度波动过大,影响晶体的形成。
七、结论1. 本实验通过自然结晶的方法,成功地将乙醇从溶液中析出,并得到了纯净的乙醇晶体。
2. 实验结果表明,乙醇在不同温度下的溶解度不同,当温度降低时,乙醇的溶解度减小,从而析出晶体。
乙醇水混合溶剂沉淀制备纳米BaF2及粒径控制(论文)
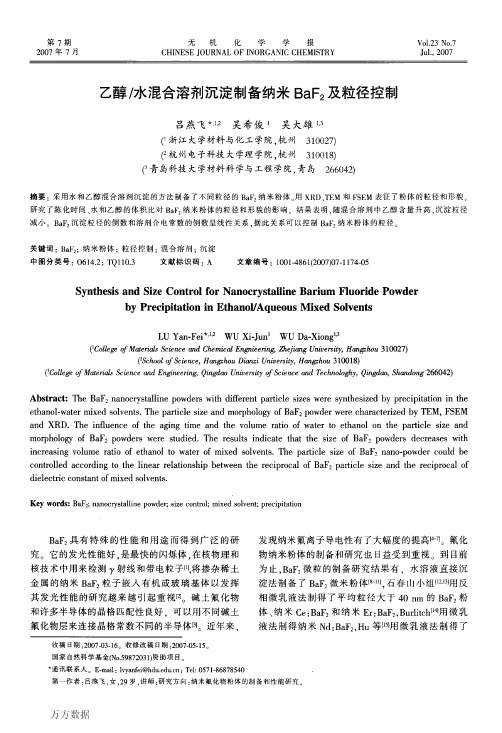
关键词:BaF:;纳米粉体;粒径控制;混合溶剂;沉淀
中图分类号:0614.2:TQll0.3
文献标识码:A
文章编号:1001—4861(2007)07—1174—05
Synthesis and Size Control for Nanocrystalline Barium Fluoride Powder
2结果与讨论
2.1 陈化时间对沉淀粒径的影响 水和乙醇体积比1:1的混合溶剂.反应物混合
后一生成沉淀.在2 min内很快制成电镜观测样品. 其TEM观察结果如图l(a1所示.可以看出颗粒非常 均匀.粒径均不超过10 nm.对照片中的观察区域进 行衍射,有稍弥散的衍射环,如图1(a1左上角所示, 表明小颗粒结晶良好。溶液中的沉淀经陈化23 h 后,电镜观测结果如图1fb)所示,小粒子已经消失, 长成平均50 nm左右较大的方形粒子。可见在陈化
80
70
暑
三60
冀
∞
●
善50
‰ 懿
f
g 40 毒
l
30
20 0
10
20
30
40
50
60
Agingtime/h
图2水/乙醇体积比为1:l的混合溶剂中获得的BaF, 粉体的晶粒粒径与陈化时间的关系
Fig.2
Variation of grain size with aging time for BaF2 powder prepared in ethanol|water mixed solvent with volume ratio of 1:1
所获得的干燥BaF,沉淀粉体.用乙醇分散.超 声40 min.滴一滴样品在铜网上制成电镜观察样 品.采用日本JEM200CX透射电镜进行观察.工作