低维材料报告
低维材料的制备及应用
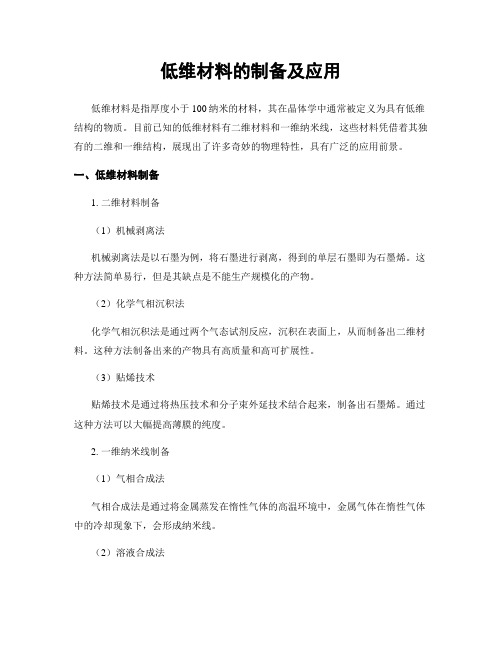
低维材料的制备及应用低维材料是指厚度小于100纳米的材料,其在晶体学中通常被定义为具有低维结构的物质。
目前已知的低维材料有二维材料和一维纳米线,这些材料凭借着其独有的二维和一维结构,展现出了许多奇妙的物理特性,具有广泛的应用前景。
一、低维材料制备1. 二维材料制备(1)机械剥离法机械剥离法是以石墨为例,将石墨进行剥离,得到的单层石墨即为石墨烯。
这种方法简单易行,但是其缺点是不能生产规模化的产物。
(2)化学气相沉积法化学气相沉积法是通过两个气态试剂反应,沉积在表面上,从而制备出二维材料。
这种方法制备出来的产物具有高质量和高可扩展性。
(3)贴烯技术贴烯技术是通过将热压技术和分子束外延技术结合起来,制备出石墨烯。
通过这种方法可以大幅提高薄膜的纯度。
2. 一维纳米线制备(1)气相合成法气相合成法是通过将金属蒸发在惰性气体的高温环境中,金属气体在惰性气体中的冷却现象下,会形成纳米线。
(2)溶液合成法溶液合成法是把原料物质溶解在有机溶剂中,通过在溶液中添加稳定剂或表面活性剂对溶液中的某种物质进行还原反应,从而合成出纳米线。
二、低维材料应用1. 光电子学低维材料的电子结构得到了广泛的研究,这种电性使其在光电子学领域具有广泛应用。
例如,二维材料石墨烯、过渡金属二硫化物等材料在太阳能电池、激光器和LED中的应用。
2. 催化剂低维材料在化学催化剂领域中应用广泛,这是由于低维材料具有很高的比表面积。
例如,纳米线材料在化学传感器和分析器件中的应用。
3. 生物医学低维材料在生物医学领域中的应用越来越广泛,主要用于生物医学成像和生物检测。
例如,纳米线材料可以用于医学成像领域,例如,检测DNA和蛋白质。
总之,低维材料具有很高的潜力和发展前景。
其制备方法可持续发展,未来将有更多应用场景。
随着材料科学及化学研究的不断发展,低维材料的应用领域将会更广。
低维材料
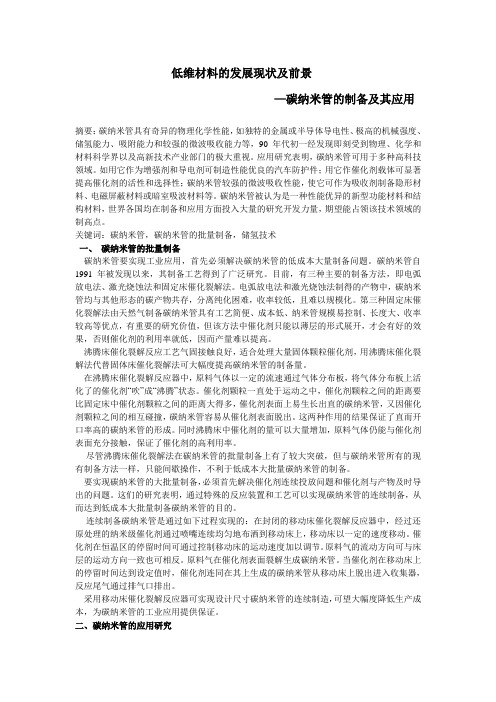
低维材料的发展现状及前景—碳纳米管的制备及其应用摘要:碳纳米管具有奇异的物理化学性能,如独特的金属或半导体导电性、极高的机械强度、储氢能力、吸附能力和较强的微波吸收能力等,90年代初一经发现即刻受到物理、化学和材料科学界以及高新技术产业部门的极大重视。
应用研究表明,碳纳米管可用于多种高科技领域。
如用它作为增强剂和导电剂可制造性能优良的汽车防护件;用它作催化剂载体可显著提高催化剂的活性和选择性;碳纳米管较强的微波吸收性能,使它可作为吸收剂制备隐形材料、电磁屏蔽材料或暗室吸波材料等。
碳纳米管被认为是一种性能优异的新型功能材料和结构材料,世界各国均在制备和应用方面投入大量的研究开发力量,期望能占领该技术领域的制高点。
关键词:碳纳米管,碳纳米管的批量制备,储氢技术一、碳纳米管的批量制备碳纳米管要实现工业应用,首先必须解决碳纳米管的低成本大量制备问题。
碳纳米管自1991年被发现以来,其制备工艺得到了广泛研究。
目前,有三种主要的制备方法,即电弧放电法、激光烧蚀法和固定床催化裂解法。
电弧放电法和激光烧蚀法制得的产物中,碳纳米管均与其他形态的碳产物共存,分离纯化困难,收率较低,且难以规模化。
第三种固定床催化裂解法由天然气制备碳纳米管具有工艺简便、成本低、纳米管规模易控制、长度大、收率较高等优点,有重要的研究价值,但该方法中催化剂只能以薄层的形式展开,才会有好的效果,否则催化剂的利用率就低,因而产量难以提高。
沸腾床催化裂解反应工艺气固接触良好,适合处理大量固体颗粒催化剂,用沸腾床催化裂解法代替固体床催化裂解法可大幅度提高碳纳米管的制备量。
在沸腾床催化裂解反应器中,原料气体以一定的流速通过气体分布板,将气体分布板上活化了的催化剂“吹”成“沸腾”状态。
催化剂颗粒一直处于运动之中,催化剂颗粒之间的距离要比固定床中催化剂颗粒之间的距离大得多,催化剂表面上易生长出直的碳纳米管,又因催化剂颗粒之间的相互碰撞,碳纳米管容易从催化剂表面脱出。
磁性材料与低维材料的研究报告
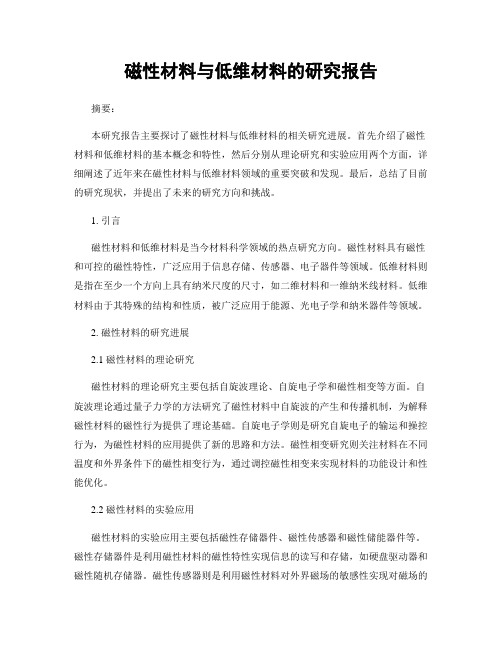
磁性材料与低维材料的研究报告摘要:本研究报告主要探讨了磁性材料与低维材料的相关研究进展。
首先介绍了磁性材料和低维材料的基本概念和特性,然后分别从理论研究和实验应用两个方面,详细阐述了近年来在磁性材料与低维材料领域的重要突破和发现。
最后,总结了目前的研究现状,并提出了未来的研究方向和挑战。
1. 引言磁性材料和低维材料是当今材料科学领域的热点研究方向。
磁性材料具有磁性和可控的磁性特性,广泛应用于信息存储、传感器、电子器件等领域。
低维材料则是指在至少一个方向上具有纳米尺度的尺寸,如二维材料和一维纳米线材料。
低维材料由于其特殊的结构和性质,被广泛应用于能源、光电子学和纳米器件等领域。
2. 磁性材料的研究进展2.1 磁性材料的理论研究磁性材料的理论研究主要包括自旋波理论、自旋电子学和磁性相变等方面。
自旋波理论通过量子力学的方法研究了磁性材料中自旋波的产生和传播机制,为解释磁性材料的磁性行为提供了理论基础。
自旋电子学则是研究自旋电子的输运和操控行为,为磁性材料的应用提供了新的思路和方法。
磁性相变研究则关注材料在不同温度和外界条件下的磁性相变行为,通过调控磁性相变来实现材料的功能设计和性能优化。
2.2 磁性材料的实验应用磁性材料的实验应用主要包括磁性存储器件、磁性传感器和磁性储能器件等。
磁性存储器件是利用磁性材料的磁性特性实现信息的读写和存储,如硬盘驱动器和磁性随机存储器。
磁性传感器则是利用磁性材料对外界磁场的敏感性实现对磁场的检测和测量,如磁力计和磁敏传感器。
磁性储能器件则是利用磁性材料的磁性能量实现能量的存储和释放,如磁性电感和磁性电容。
3. 低维材料的研究进展3.1 二维材料的研究二维材料是指具有二维结构的材料,如石墨烯和二硫化钼等。
石墨烯是由一个碳原子层组成的二维材料,具有高度的导电性和热导性,被广泛应用于电子器件和能源存储领域。
二硫化钼则是一种具有层状结构的二维材料,具有优异的光电性能,被广泛应用于光电子学和传感器领域。
低维材料与器件的研究报告
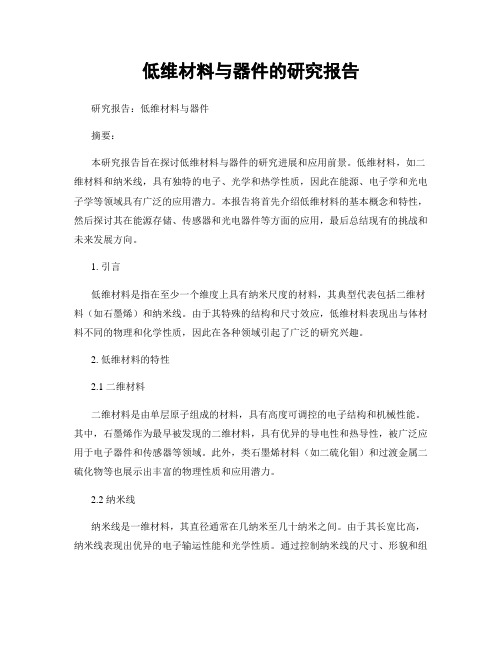
低维材料与器件的研究报告研究报告:低维材料与器件摘要:本研究报告旨在探讨低维材料与器件的研究进展和应用前景。
低维材料,如二维材料和纳米线,具有独特的电子、光学和热学性质,因此在能源、电子学和光电子学等领域具有广泛的应用潜力。
本报告将首先介绍低维材料的基本概念和特性,然后探讨其在能源存储、传感器和光电器件等方面的应用,最后总结现有的挑战和未来发展方向。
1. 引言低维材料是指在至少一个维度上具有纳米尺度的材料,其典型代表包括二维材料(如石墨烯)和纳米线。
由于其特殊的结构和尺寸效应,低维材料表现出与体材料不同的物理和化学性质,因此在各种领域引起了广泛的研究兴趣。
2. 低维材料的特性2.1 二维材料二维材料是由单层原子组成的材料,具有高度可调控的电子结构和机械性能。
其中,石墨烯作为最早被发现的二维材料,具有优异的导电性和热导性,被广泛应用于电子器件和传感器等领域。
此外,类石墨烯材料(如二硫化钼)和过渡金属二硫化物等也展示出丰富的物理性质和应用潜力。
2.2 纳米线纳米线是一维材料,其直径通常在几纳米至几十纳米之间。
由于其长宽比高,纳米线表现出优异的电子输运性能和光学性质。
通过控制纳米线的尺寸、形貌和组分,可以实现对其电子结构和能带工程的调控,从而拓展其在能源存储和传感器等领域的应用。
3. 低维材料的应用3.1 能源存储低维材料在能源存储领域具有巨大的潜力。
例如,石墨烯被广泛应用于锂离子电池和超级电容器,因其高电导率和大比表面积。
此外,纳米线也被用作锂离子电池的电极材料,以提高能量密度和循环稳定性。
3.2 传感器低维材料在传感器领域的应用也备受关注。
二维材料的高灵敏度和快速响应速度使其成为理想的气体传感器和生物传感器材料。
纳米线的高比表面积和可调控的表面化学性质使其成为优异的化学传感器和生物传感器。
3.3 光电器件由于低维材料具有特殊的光学性质,如量子限域效应和表面等禁带调制效应,它们在光电器件中的应用也备受关注。
特色研究报告:低维电磁功能材料研究进展
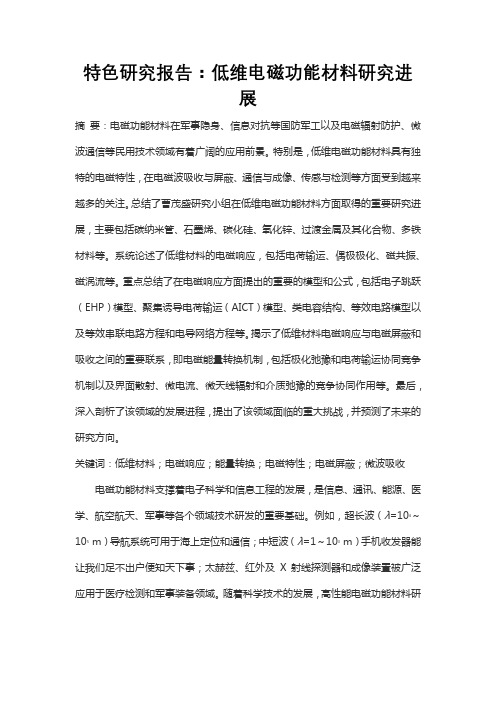
特色研究报告:低维电磁功能材料研究进展摘要:电磁功能材料在军事隐身、信息对抗等国防军工以及电磁辐射防护、微波通信等民用技术领域有着广阔的应用前景。
特别是,低维电磁功能材料具有独特的电磁特性,在电磁波吸收与屏蔽、通信与成像、传感与检测等方面受到越来越多的关注。
总结了曹茂盛研究小组在低维电磁功能材料方面取得的重要研究进展,主要包括碳纳米管、石墨烯、碳化硅、氧化锌、过渡金属及其化合物、多铁材料等。
系统论述了低维材料的电磁响应,包括电荷输运、偶极极化、磁共振、磁涡流等。
重点总结了在电磁响应方面提出的重要的模型和公式,包括电子跳跃(EHP)模型、聚集诱导电荷输运(AICT)模型、类电容结构、等效电路模型以及等效串联电路方程和电导网络方程等。
揭示了低维材料电磁响应与电磁屏蔽和吸收之间的重要联系,即电磁能量转换机制,包括极化弛豫和电荷输运协同竞争机制以及界面散射、微电流、微天线辐射和介质弛豫的竞争协同作用等。
最后,深入剖析了该领域的发展进程,提出了该领域面临的重大挑战,并预测了未来的研究方向。
关键词:低维材料;电磁响应;能量转换;电磁特性;电磁屏蔽;微波吸收电磁功能材料支撑着电子科学和信息工程的发展,是信息、通讯、能源、医学、航空航天、军事等各个领域技术研发的重要基础。
例如,超长波(λ=104~105 m)导航系统可用于海上定位和通信;中短波(λ=1~103 m)手机收发器能让我们足不出户便知天下事;太赫兹、红外及X射线探测器和成像装置被广泛应用于医疗检测和军事装备领域。
随着科学技术的发展,高性能电磁功能材料研发将成为今后科学界新的研究热点之一,未来电磁功能材料和器件的创新将给人类带来更多意想不到的惊喜。
低维电磁功能材料的研发推动了全球高新技术领域的进步。
新的物理效应,新的电磁响应机制和电磁性能,新的低维材料以及多元化、微小型化和智能化的新型电磁器件,为电磁波吸收与屏蔽、探测与传感、成像、开关与滤波、光学与光电等领域的发展带来了无限的活力。
低维材料范文

低维材料范文低维材料是一种结构维度较低的材料,通常由单层或少数层的原子或分子构成。
它的特殊的结构和性质使得低维材料在纳米技术和电子学等领域展现出巨大的潜力。
本文将介绍几种常见的低维材料及其应用。
首先,石墨烯是最为知名的低维材料之一、它由单层的碳原子构成,具有高度的导电性和导热性。
石墨烯的独特结构使得它成为开展纳米电子学研究的理想材料。
石墨烯的电子可移动性极高,因此在纳米电子器件中可以实现高速传输和处理数据的能力。
此外,由于石墨烯可以通过拉伸形成纳米尺度的孔洞,因此在纳米过滤器和储能设备等领域也有广泛应用。
第二,碲化二维材料具有较高的载流子迁移率和光吸收能力。
其中,二硒化钼和二硫化钼是最常见的碲化二维材料。
它们的晶体结构中,金属离子与硫(或硒)原子形成一个二维的平面,有利于电子的传输。
碲化二维材料在光电子器件中具有重要的应用潜力,如光电探测器和光催化剂等。
此外,碲化二维材料还可以通过控制其结构或化学成分实现各种特殊性质的调控,如磁性和拓扑绝缘等。
第三,过渡金属二维材料也是一类重要的低维材料。
它们由过渡金属原子形成的二维平面结构构成,具有多种功能性能。
其中,二硫化钴和二硒化钴是常见的过渡金属二维材料。
这些材料具有媲美石墨烯的导电性和机械稳定性,同时还具有独特的磁性和光学性质。
过渡金属二维材料在存储器件和传感器等领域有广泛的应用潜力。
最后,有机-无机杂化低维材料是一类由有机和无机组分通过化学键形成的材料。
在这类材料中,有机分子通过与无机原子团簇相互作用,形成稳定的二维平面结构。
有机-无机杂化低维材料具有丰富的光电性质,可以用于制备光电器件和光电转换材料。
此外,有机-无机杂化低维材料还具有良好的可加工性和机械性能,因此在柔性电子学和可穿戴设备等领域也有广泛应用。
综上所述,低维材料是具有特殊结构和性质的一类材料。
石墨烯、碲化二维材料、过渡金属二维材料和有机-无机杂化低维材料都是重要的低维材料。
它们在纳米电子学、光电子学和柔性电子学等领域具有广泛的应用潜力,对于推动纳米技术和电子学的发展具有重要意义。
低维功能材料的制备与应用研究
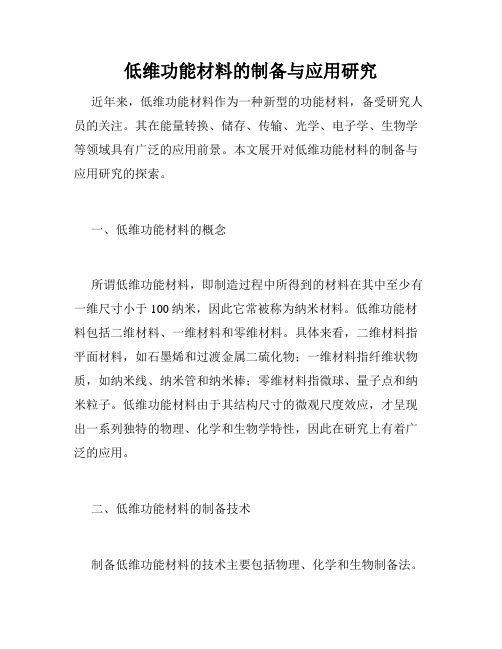
低维功能材料的制备与应用研究近年来,低维功能材料作为一种新型的功能材料,备受研究人员的关注。
其在能量转换、储存、传输、光学、电子学、生物学等领域具有广泛的应用前景。
本文展开对低维功能材料的制备与应用研究的探索。
一、低维功能材料的概念所谓低维功能材料,即制造过程中所得到的材料在其中至少有一维尺寸小于100纳米,因此它常被称为纳米材料。
低维功能材料包括二维材料、一维材料和零维材料。
具体来看,二维材料指平面材料,如石墨烯和过渡金属二硫化物;一维材料指纤维状物质,如纳米线、纳米管和纳米棒;零维材料指微球、量子点和纳米粒子。
低维功能材料由于其结构尺寸的微观尺度效应,才呈现出一系列独特的物理、化学和生物学特性,因此在研究上有着广泛的应用。
二、低维功能材料的制备技术制备低维功能材料的技术主要包括物理、化学和生物制备法。
1. 物理制备法物理制备法主要通过物理方法获得纳米级别的材料,包括机械剥离法、溅射法、热蒸发法、物理气相沉积法等。
这些方法制备的低维功能材料通常具有较高的可以重复生长的特点,而且具有较高的质量和晶体结构完整性。
例如,机械剥离法是通过剥离超薄的材料获得二维材料,如石墨烯。
而溅射法主要用于制备金属和半导体薄膜。
热蒸发法则主要用于制备薄膜。
物理气相沉积法通常用于制造一维纳米线和纳米管。
2. 化学制备法化学制备法主要通过化学反应来获得材料,包括溶剂沉淀法、水热法、微波合成法等。
这些方法制备的低维功能材料通常具有更高的比表面积和更大的反应活性,从而可以被广泛应用于分析、催化和生物学等领域。
例如,溶剂沉淀法常用于合成纳米粒子和量子点。
水热法常用于制备金属氧化物、金属磷酸盐等材料。
微波合成法则常用于合成金属氧化物、纳米碳管等。
3. 生物制备法生物制备法是指使用细胞或分子进行合成,通过这种方式获得的低维功能材料通常也具有更大的比表面积和更大的反应活性。
生物制备法也是一种低成本的制备方式。
例如,利用微生物等细胞进行生物制备,可以获得纳米材料。
低维材料的制备与性质
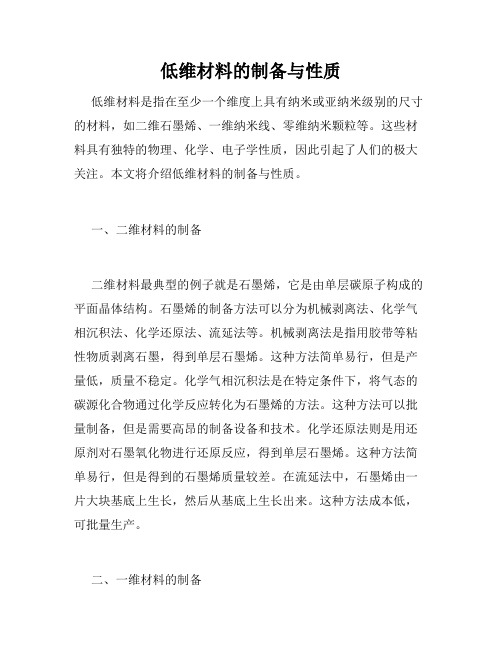
低维材料的制备与性质低维材料是指在至少一个维度上具有纳米或亚纳米级别的尺寸的材料,如二维石墨烯、一维纳米线、零维纳米颗粒等。
这些材料具有独特的物理、化学、电子学性质,因此引起了人们的极大关注。
本文将介绍低维材料的制备与性质。
一、二维材料的制备二维材料最典型的例子就是石墨烯,它是由单层碳原子构成的平面晶体结构。
石墨烯的制备方法可以分为机械剥离法、化学气相沉积法、化学还原法、流延法等。
机械剥离法是指用胶带等粘性物质剥离石墨,得到单层石墨烯。
这种方法简单易行,但是产量低,质量不稳定。
化学气相沉积法是在特定条件下,将气态的碳源化合物通过化学反应转化为石墨烯的方法。
这种方法可以批量制备,但是需要高昂的制备设备和技术。
化学还原法则是用还原剂对石墨氧化物进行还原反应,得到单层石墨烯。
这种方法简单易行,但是得到的石墨烯质量较差。
在流延法中,石墨烯由一片大块基底上生长,然后从基底上生长出来。
这种方法成本低,可批量生产。
二、一维材料的制备一维纳米线可以作为电子学器件、高灵敏度感知器、高效催化剂等材料。
纳米线的制备方法包括溶液法、气液固相生长法、气-液界面法等。
其中溶液法是纳米线制备的简单有效方法,被广泛研究。
通过将显微粒子、无机盐等成分溶解于适当的溶剂中,通过控制溶液中各种成分的浓度和温度等条件,可以得到晶体生长方向与大小相适应的纳米线结构。
气液固相生长法则是通过加热气体,从稀薄气体中将原子或分子沉积在基底表面形成纳米线。
气-液界面法则是由于溶液的挥发,固液界面上的成核几率增加,因而直接在这个界面上合成纳米线。
三、零维材料的制备零维材料是指尺寸在纳米级别的球形纳米粒子,这些材料具有很高的表面积,因而具有很好的催化、传感等性能。
纳米粒子的制备方法包括化学合成法、激光等离子体法、物理气相沉积法等。
化学合成法是将金属盐等化合物在一定条件下还原为金属纳米粒子。
这种方法操作简单,可以批量制备,但是粒子形状和大小控制较难。
激光等离子体法则是将激光束聚焦在金属表面,形成等离子体,通过化学反应从而得到金属纳米粒子。
- 1、下载文档前请自行甄别文档内容的完整性,平台不提供额外的编辑、内容补充、找答案等附加服务。
- 2、"仅部分预览"的文档,不可在线预览部分如存在完整性等问题,可反馈申请退款(可完整预览的文档不适用该条件!)。
- 3、如文档侵犯您的权益,请联系客服反馈,我们会尽快为您处理(人工客服工作时间:9:00-18:30)。
Development of integrated circuit industry and currenttechnological challengesThe integrated circuit is a small chip that can function as an amplifier, oscillator, timer, microprocessor, or even computer memory. And it is a small wafer, usually made of silicon, that can hold anywhere from hundreds to millions of transistors, resistors, and capacitors. These extremely small electronics can perform calculations and store data, and are used in almost all electronic equipment today. The integrated circuits have two main advantages over discrete circuits, cost and performance. Cost is low because the components of chips are printed as a unit by photolithography rather than being constructed one transistor at a time. Furthermore, packaged integrated circuits use much less material than discrete circuits. And performance is high because the integrated circuits’components switch quickly and consume little power compared to their discrete components, as a result of the small size and close arrangement of the components. Integrated circuits are used in virtually all electronic equipment today and have revolutionized the world [1].Early developments of the integrated circuit date back to 1949, when German engineer Werner Jacobi applied for a patent for an integrated-circuit-like semiconductor amplifying device showing five transistors on a common substrate in a 3-stage amplifier arrangement [2]. Jacobi disclosed small and cheap hearing aids as typical industrial applications of his patent. But, an immediate commercial use of his patent has not been reported. And the idea of the integrated circuit was conceived by Geoffrey W.A. Dummer (1909–2002), a radar scientist working for the Royal Radar Establishment of the British Ministry of Defense. Dummer presented the idea to the public at the Symposium on Progress in Quality Electronic Components in Washington, D.C. on 7 May 1952. He did many to propagate his ideas, and unsuccessfully attempted to build such a circuit in 1956. Otherwise, at that time a precursor idea to the integrated circuit was to create small ceramic squares or wafers, each containing a single miniaturized component. Components could then be integrated and wired into a bidimensional or tridimensional compact grid. This idea, which seemed very promising in 1957, was proposed to the US Army by Jack Kilby and led to the short-lived Micromodule Program. However, as the project was overwhelming, Kilby came up with a new, revolutionary design: the IC [3].Since the first piece of integrated circuit chip invented by Jack Kilby in 1958 and Noyce in 1959 it has significantly change the electronic industry and the world. Just as the Moore’s law s ays that the number of transistors on a chip doubles about every 18 month, the development of integrated circuit was very fast and our life and society also changed rapidly. In the very beginning of integrated circuits there only a few transistors could be placed on a chip, and the scale was large because of the contemporary technology. The first integrated circuits contained only a few transistors, which called Small-Scale Integration (SSI) [4]. And SSI circuits were crucial to early aerospace projects, and vice-versa. As we all know, both the Minuteman missile and Apollo program need lightweight digital computers for their inertial guidance systems. Therefore, the Apollo guidance computer led and motivated the integrated-circuit technology, while the Minuteman missile forced it into mass-production. The demand by the government supported the initial integrated circuit market until costs fell enough to allow companies to enter the industrialand eventually the consumer markets.In the late 1960s, the next development stage of integrated circuits which contains hundreds of transistors on each chip, called "Medium-Scale Integration" (MSI). Apparently, there were attractive economically because while they cost little more to produce than SSI devices, they allowed more complex systems to be produced using smaller circuit boards, less assembly work because of fewer separate components, and so on. And further development, driven by the same economic factors, led to "Large-Scale Integration" (LSI) in the mid 1970s, with tens of thousands of transistors per chip. At this stage integrated circuits such as 1000 bit RAMs, calculator chips, and the first microprocessors, that began to be manufactured in the early 1970s, had fewer than 4000 transistors. And for true LSI circuits, which approaching 10000 transistors began to be produced around 1974 and used as computer main memories as well as second-generation microprocessors. And with the developing of science and technology, the final step starting from the early 1980s to the present was "very large-scale integration" (VLSI). In 1986 the first one megabit RAM chips were introduced, which contained more than one million transistors. Microprocessor chips passed the million transistor mark in 1989 and the billion transistor mark in 2005[5]. And the chips introduced in 2007 containing tens of billions of memory transistors [6], and the trend which cannot be halted. What’s more, to refl ect further growth of the complexity, the term ULSI that stands for "ultra-large-scale integration" was proposed for chips of more than 1 million transistors [7].And to further reducing costs and improving integration there need some new technologies to make the integration circuits, such as wafer-scale integration, system-on-a-chip as well as three-dimensional integrated circuit. Wafer-scale integration [8] (WSI) is a method to make very large integrated circuits that uses an entire silicon wafer to produce a single superchip. Through a combination of large size and reduced packaging, WSI could result in dramatically reduced costs for some systems, especially massively parallel supercomputers. The current state of the art of WSI is being developed. A system-on-a-chip (SOC) is an integrated circuit in which all the components needed for a computer or other system is included on one single chip [9]. The design of such a device can be complex and costly, and building disparate components on a single piece of silicon may compromise the efficiency of some elements. However, these drawbacks can be offset by lower manufacturing and assembly costs and by a greatly reduced power budget. And a three-dimensional integrated circuit [10][11][12] (3D-IC) has two or more layers of active electronic components that are integrated both vertically and horizontally into a single circuit. Communication between layers uses on-die signaling, so power consumption is much lower than in equivalent separate circuits.The feature sizes of integrated circuits have continuously getting smaller and smaller over the years, and the degree of integration keep increasing which allow more circuitry to be packed on each chip. In general, with the shrinking of feature size, almost everything improves, such as the cost per unit and the switching power consumption go down and the speed goes up. However, integrated circuits with nanometer-scale devices have some problems, main among which is leakage current, bad size effect of this kind of nano material. After decades of reengineering repeatedly, silicon transistors began to reach its development limit, and this is a severe problem and a big challenge. Apparently, use silicon as material of integrated circuit cannot meet our requirement, and we need new material or technologies. Fortunately, with the development material science and technology, we found that with the aid of ferroelectric materials [13], thecalculation of computer can no longer use digital logic. By researching the researchers found that we use the ferroelectric material in which the charge can switch rapidly among four different states to store the datum. And the transistors made of this kind of ferroelectric material can use different charge states to express more digital logic than one and zero, and it can hold the charge state without external power. So, these kinds of material can both processes information and store datum. And I think in the future the development of integrated circuits will focus on ferroelectric materials and this kind of material will further improve integrated circuits.What’s more, recently IBM researchers have built the first integrated circuit (IC) based on a graphene transistor [14], another step toward overcoming the limits of silicon and a potential path to flexible electronics. The circuit, built on a wafer of silicon carbide, consists of field-effect transistors (FETs) made of graphene, a highly conductive chicken-wire-like arrangement of carbon that's a single atomic layer thick. The integrated circuit also includes metallic structures, such as on-chip inductors and the transistors' sources and drains. The work is described in this week's issue of Science. Researchers say that graphene, which has the potential to make transistors that operate at terahertz speeds, could one day supplant silicon as the basis for computer chips. Otherwise, one remarkable feature is that the performance of the device didn't change very much when its temperature went from about 27 °C to 127 °C. And that means a graphene circuit won't have to be overdesigned to compensate for temperature changes, potentially leading to a less-complex and less-expensive circuit. But, there are two main difficulties [15]: One is that the metals used to make other parts of the circuit, such as aluminum, gold, and palladium, don't adhere very well to the graphene. The other is the fact that graphene, being only a single atom thick, is easily damaged by standard semiconductor etching processes.Finally, the developments of integrated circuits have pushed the development of electronic industry and still play a vital role in our society. Although, it seemed reach its limitation in silicon material, with the development of material science and technology, new materials, grapheme and ferroelectric material, have developed and researched to make new type integrated circuits. And them will further promote the development of electronic industry and change our society and life largely.Reference________________[1]"Integrated circuits help Invention". . Retrieved 2012-08-13.[2]DE 833366 W. Jacobi/SIEMENS AG: …Halbleiterverstärker“ priority filing on 14 April 1949,published on 15 May 1952.[3]Briddock D. The Integrated Circuit[J]. Micro Mart, 2013.[4]Mark Melliar-Smith C, Haggan D E, Troutman W W. Key steps to the integrated circuit[J].Bell Labs Technical Journal, 1997, 2(4):15–28.[5]Havemann R H, Hutchby J A. High-performance interconnects: An integration overview[J].Proceedings of the IEEE, 2001, 89(5):586--601.[6]Peter Clarke, EE Times: Intel enters billion-transistor processor era, 14 November 2005.[7]Meindl, J.D. "Ultra-large scale integration". . IEEE. Retrieved 21 September 2014.[8]LEIGHTON T. Wafer-Scale Integration of Systolic Arrays[J]. Computers IEEE Transactions on,1985, 34(5):448--461.[9]PATTI R S. Three-Dimensional Integrated Circuits and the Future of System-on-Chip Designs[J].Proc IEEE, 2006, 94(6):1214 - 1224.[10]Chan M, Ko P K. Development of a viable 3D integrated circuit technology[J]. Science in China,2001, 44(4):241-248.[11]Chan M, Ko P K. Development of a viable 3D integrated circuit technology[J]. Science in China,2001, 44(4):241-248.[12]Topol, A.W.; Tulipe, ; Shi, L; et., al. "Three-dimensional integrated circuits". .International Business Machines Corporation (IBM). Retrieved 21 September 2014.[13]Calzada M , Bretos I, Jiménez R, et al. Low‐Temperature Processing of FerroelectricThin Films Compatible with Silicon Integrated Circuit Technology[J]. Advanced Materials, 2004, 16(18):1620–1624.[14]YM L, A V, SJ H, et al. Wafer-scale graphene integrated circuit.[J]. Science, 2011,332(6035):1294-.[15]Wu Y, Farmer D B, Xia F, et al. Graphene Electronics: Materials, Devices, and Circuits[J].Proceedings of the IEEE, 2013, 101(7):1620 - 1637.。