有机键吸收
pd-s键红外光谱位置 -回复
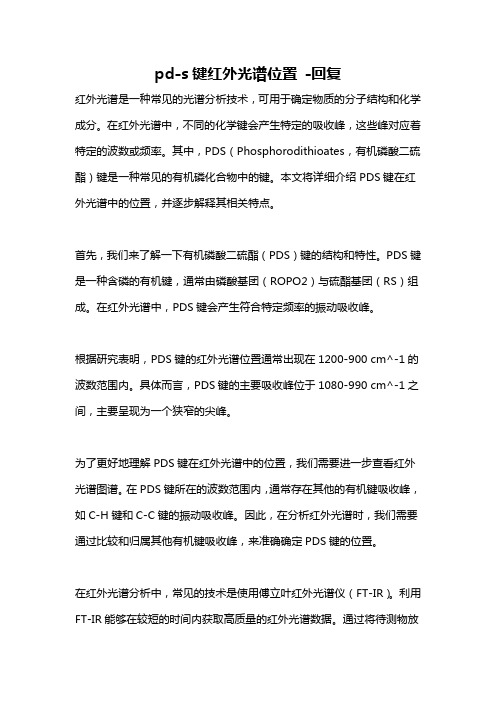
pd-s键红外光谱位置-回复红外光谱是一种常见的光谱分析技术,可用于确定物质的分子结构和化学成分。
在红外光谱中,不同的化学键会产生特定的吸收峰,这些峰对应着特定的波数或频率。
其中,PDS(Phosphorodithioates,有机磷酸二硫酯)键是一种常见的有机磷化合物中的键。
本文将详细介绍PDS键在红外光谱中的位置,并逐步解释其相关特点。
首先,我们来了解一下有机磷酸二硫酯(PDS)键的结构和特性。
PDS键是一种含磷的有机键,通常由磷酸基团(ROPO2)与硫酯基团(RS)组成。
在红外光谱中,PDS键会产生符合特定频率的振动吸收峰。
根据研究表明,PDS键的红外光谱位置通常出现在1200-900 cm^-1的波数范围内。
具体而言,PDS键的主要吸收峰位于1080-990 cm^-1之间,主要呈现为一个狭窄的尖峰。
为了更好地理解PDS键在红外光谱中的位置,我们需要进一步查看红外光谱图谱。
在PDS键所在的波数范围内,通常存在其他的有机键吸收峰,如C-H键和C-C键的振动吸收峰。
因此,在分析红外光谱时,我们需要通过比较和归属其他有机键吸收峰,来准确确定PDS键的位置。
在红外光谱分析中,常见的技术是使用傅立叶红外光谱仪(FT-IR)。
利用FT-IR能够在较短的时间内获取高质量的红外光谱数据。
通过将待测物放置在样品舱中,将红外光束透过样品进行衍射和干涉,最终得到具有吸收峰的红外光谱图谱。
为了解释PDS键在红外光谱中的位置,我们需要了解红外光谱的波数标度。
红外光谱的横坐标通常使用波数(cm^-1)表示,波数越大,对应的波长越短,反之亦然。
在红外光谱图谱中,通常以1000 cm^-1附近的C-H 键振动吸收峰作为参考峰。
一旦我们获得了包含PDS键吸收峰的红外光谱图谱,我们可以开始解释其位置。
首先,我们需要考虑红外光谱图谱中的其他峰的位置和形状。
例如,如果在1080-990 cm^-1范围内有明显的其他吸收峰存在,我们可以进一步检查这些峰的形状和相对强度,以确定PDS键的位置。
红外光谱1000左右的吸收峰

红外光谱1000左右的吸收峰
红外光谱中,吸收峰的位置通常与特定的化学键或者分子振动模式有关。
在1000 cm-1左右的区域,常见的吸收峰主要与以下几种化学键或分子振动有关:
C-O键的拉伸振动:在醇、醚或酯等含有C-O键的有机化合物中,C-O键的拉伸振动通常会在1050-1150 cm-1的区域产生吸收峰。
C-N键的拉伸振动:在胺或酰胺等含有C-N键的有机化合物中,C-N键的拉伸振动通常会在1080-1360 cm-1的区域产生吸收峰。
S=O键的拉伸振动:在磺酰或亚磺酰等含有S=O键的有机化合物中,S=O键的拉伸振动通常会在1000-1300 cm-1的区域产生吸收峰。
C-Cl键的拉伸振动:在含有C-Cl键的有机化合物中,C-Cl键的拉伸振动通常会在600-800 cm-1的区域产生吸收峰,但在某些情况下也可能出现在1000 cm-1左右。
以上只是一些常见的情况,实际上在1000 cm-1左右的吸收峰可能与其他类型的化学键或分子振动有关。
具体的判断需要结合化合物的结构和其他谱图信息。
ti-o键红外吸收峰

ti-o键红外吸收峰
Ti-O键红外吸收峰是一种特殊的红外吸收峰,它可以用来识别和
分类多种有机物质。
它主要来源于Ti-O键,它是由一个金属原子和一
个氧原子组成的化学键。
在晶体表面上,Ti-O键会形成多个分子形式,这种键被称为键桥。
由于Ti-O键特殊的化学特性,它可以在低温下保
持其结构稳定,而且还能影响光的吸收波长,这就是Ti-O键红外吸收
峰的来源。
Ti-O键红外吸收峰的特征是吸收波长为3.6μm左右,它与表明该
物质结构中存在Ti-O键的特征有关。
Ti-O键红外吸收峰的典型应用包
括有机分子的鉴别,例如聚苯乙烯、卤代烃和丙烷等。
此外,Ti-O键
红外吸收峰也常用作检测有机物质浓度及粒径分布的定量工具。
Ti-O键红外吸收峰除了可以用于物质鉴别外,还在许多其它领域
有所应用。
比如,它可以用作检测溶剂、液体、气体以及超级质子的
定量方法;用于测量样品表面上构造单元的结构变化,在无机硅催化
剂表面上Ti-O键红外吸收峰可以反映其化学结构变化和催化活性变化。
Ti-O键红外吸收峰有着广泛的应用,也被广泛用于化学反应研究
和表面物理研究。
Ti-O键红外吸收峰可以帮助我们深入了解有机物质
的结构及功能性,从而进一步探究自然界不同物质之间的关系。
碳硫键的红外吸收峰

碳硫键的红外吸收峰
碳硫键是一种重要的化学键,它是由碳原子和硫原子之间共享一对电子形成的。
在有机化合物中,碳硫键通常出现在含有硫基团的化合物中,例如硫醇、硫醚、硫酸酯等。
这些化合物在红外光谱中通常会显示出特征性的吸收峰,这些吸收峰可以用来确定化合物中碳硫键的存在和数量。
在红外光谱图中,碳硫键通常显示为一个强烈的吸收峰,在1350-650 cm^-1范围内。
这个范围内的吸收峰被称为“C-S拉伸振动”,因为它们是由分子中碳-硫键拉伸引起的。
C-S拉伸振动在不同类型的化合物中表现出不同的特征性吸收峰。
例如,在含有烷基或芳香基取代基的烷基硫醇和烷基硫醚中,C-S拉伸振动通常显示为一个强烈而窄的吸收峰,在约 667-663 cm^-1 范围内。
这个吸收峰通常被称为“主要C-S拉伸振动”,它的强度和位置可以用来确定化合物中硫原子的取代基类型和数量。
另一方面,在含有芳香环的硫醚和硫酚中,C-S拉伸振动通常显示为两个吸收峰,在约 700-550 cm^-1 范围内。
这些吸收峰被称为“次要C-S拉伸振动”,它们的位置和相对强度可以用来确定芳香环的取代基类型和数量。
此外,在含有双键或三键的硫醇、硫酚或硫醚中,C-S拉伸振动通常显示为一个相对较弱的吸收峰,在约 1000-600 cm^-1 范围内。
这个吸收峰通常被称为“共轭C=S或C≡S拉伸振动”,它可以用来确定化合物中双键或三键硫原子的存在。
总之,碳硫键是一种重要的化学键,它在有机化合物中表现出不同的特征性红外吸收峰。
这些红外吸收峰可以用来确定化合物中碳硫键的存在和数量,并提供有关分子结构和取代基类型等信息。
碳氮双键的红外吸收峰

碳氮双键的红外吸收峰1 碳氮双键的红外吸收碳氮双键是氮原子和碳原子之间的双锯齿型化学键,是各种有机物质中最为普遍的结构。
碳氮双键的红外吸收特性主要取决于其相邻分子间的键强度,键型以及键长等。
2 吸收频谱碳氮双键表现出宽频宽的红外吸收频谱。
其最大的吸收峰是介于3000cm-1 与2800cm-1 之间的芳香C=N双键的扭曲振动(bend)和共振振动(anti-symmetric-stretch),其吸收度为1200~1800。
其次是介于1700cm-1到1600cm-1之间,由芳香C=N双键的普通共振振动和N-H极化振动构成,其吸收强度为600~800。
3 吸收峰特征碳氮双键的红外吸收峰位于3000cm-1-2800cm-1之间,由芳香C=N 双键的弯曲振动(bend)和共振振动(asymmetric-stretch)所构成。
C=N双键弯曲振动的振动曲线中吸收应变是凸线型,而发射应变是以圆弧形状呈现,而共振振动的振动曲线中发射应变是凸线型,而吸收应变则以圆弧形状出现。
碳氮双键在芳香C=N双键共振振动(asymmetric-stretch)时具有较强的分子结构扭曲应变,因此这种结构下的吸收峰具有强度较强的特征,是一种十分重要的分子结构特征。
4 利用红外吸收信号分析利用碳氮双键的红外吸收信号可以分析出分子本身的结构,而其氮可以从信号中识别出这种结构,比如介分子中各种氮原子以及不同氮原子的器件氮的位置信息或构型信息等,同时可以研究其氢键力以及其他化学键的分布情况等。
因此,碳氮双键的红外吸收信号是分子研究的重要材料。
5 总结碳氮双键的红外吸收峰特性取决于相邻分子间的键强度、键型以及键长等,它可以实现宽频宽的红外吸收频谱表现,吸收度高达1200-1800。
因此,通过对碳氮双键红外吸收信号的分析可以用来判断有机分子的结构和化学键的相互作用情况,为我们的有机分子研究工作提供了很多有价值的信息。
亚胺键的红外吸收峰

亚胺键的红外吸收峰
亚胺键是有机化合物中最常见的一种键。
它的存在使得许多有机分子都表现出了独特的化学性质。
在红外光谱中,亚胺键的吸收峰通常出现在1450-1650 cm^-1的范围内。
这个范围是因为亚胺键的振动频率与分子中氮原子的电子密度和碳氮键的键阶有关。
通常情况下,亚胺键的吸收峰会出现在1450-1550 cm^-1的范围内。
这个峰位通常被称为“亚胺吸收峰”,是有机化合物红外光谱中最常
见的吸收峰之一。
亚胺吸收峰的强度可以提供关于分子中亚胺键数量的信息,因为每个亚胺键都会对应一个吸收峰。
除了亚胺吸收峰外,还有一个范围为1600-1650 cm^-1的“亚胺酰吸收峰”。
这个峰位通常对应着分子中的酰基亚胺键(即分子中含有两
个羰基和一个氮原子的键)。
需要注意的是,亚胺吸收峰的位置和强度可以受到环境的影响。
比如,分子中的各种官能团的共存可能导致亚胺吸收峰的位置发生位移或
分裂,这种现象被称为“峰展宽”。
此外,分子中的氢键也可能对亚
胺吸收峰的位置和形状产生影响。
总之,亚胺键是有机化合物中非常常见的键,其在红外光谱中的吸收峰可以提供关于分子结构和化学性质的重要信息。
在分析红外光谱时,
需要综合考虑亚胺键所处的环境、共存的官能团以及化合物本身的特性。
碳氮双键 紫外吸收
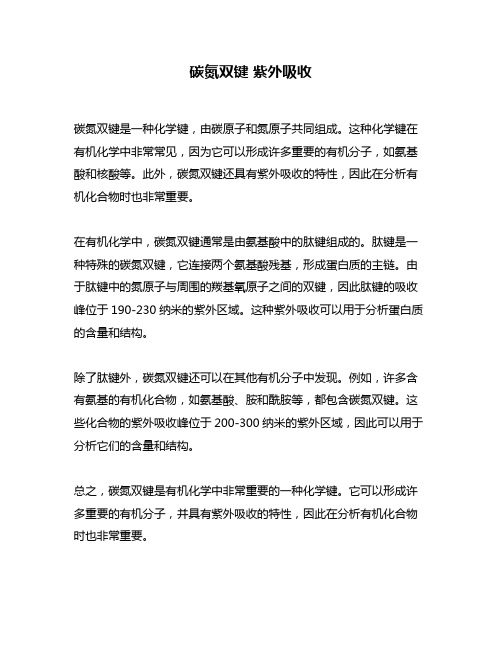
碳氮双键紫外吸收
碳氮双键是一种化学键,由碳原子和氮原子共同组成。
这种化学键在有机化学中非常常见,因为它可以形成许多重要的有机分子,如氨基酸和核酸等。
此外,碳氮双键还具有紫外吸收的特性,因此在分析有机化合物时也非常重要。
在有机化学中,碳氮双键通常是由氨基酸中的肽键组成的。
肽键是一种特殊的碳氮双键,它连接两个氨基酸残基,形成蛋白质的主链。
由于肽键中的氮原子与周围的羰基氧原子之间的双键,因此肽键的吸收峰位于190-230纳米的紫外区域。
这种紫外吸收可以用于分析蛋白质的含量和结构。
除了肽键外,碳氮双键还可以在其他有机分子中发现。
例如,许多含有氨基的有机化合物,如氨基酸、胺和酰胺等,都包含碳氮双键。
这些化合物的紫外吸收峰位于200-300纳米的紫外区域,因此可以用于分析它们的含量和结构。
总之,碳氮双键是有机化学中非常重要的一种化学键。
它可以形成许多重要的有机分子,并具有紫外吸收的特性,因此在分析有机化合物时也非常重要。
聚硅氧烷材料中Si-O键的红外吸收特性研究

聚硅氧烷材料中Si-O键的红外吸收特性研究聚硅氧烷材料(Polydimethylsiloxane,PDMS)是一种特殊的有机硅材料,其主要结构由硅原子与氧原子之间的键连接而成。
在PDMS 中,Si-O键是材料中最重要的键结构之一,它决定了PDMS的性质与应用。
为了深入了解聚硅氧烷材料中Si-O键的特性,本文系统地研究了其红外光谱吸收特性。
首先,通过红外光谱分析仪对PDMS样品进行测试,记录并分析了其红外光谱图谱。
在红外光谱中,我们观察到了多个特征峰,其中Si-O键的吸收峰位于约1100 cm-1和950 cm-1的波数范围内。
这些特征峰的出现是由于Si-O键的振动引起的,因此可以通过红外光谱来研究和分析Si-O键的性质。
进一步,我们利用红外光谱的峰位强度信息来评估Si-O键的结构特性。
在红外光谱中,特征峰的峰位强度与键的振动频率和键的强度有关。
通过对PDMS样品进行一系列实验研究,我们发现Si-O键的峰位强度与PDMS样品中Si-O键的含量和键的结构有关。
在PDMS样品中,Si-O键的含量越高,其红外光谱中的特征峰峰位强度就越高,反之亦然。
此外,不同结构的Si-O键在红外吸收谱图中可能表现出不同的峰位移特性,这也影响了特征峰的位置和强度。
除了红外光谱分析,我们还通过其他表征手段来研究聚硅氧烷材料中Si-O键的特性。
例如,核磁共振波谱(NMR)可以提供有关Si-O 键化学位移和键长的信息。
通过NMR实验,我们可以进一步验证红外光谱对Si-O键特性的分析,并确定其精确的结构特性。
此外,PDMS材料中的Si-O键在不同环境条件下可能有不同的红外吸收特性。
例如,当PDMS与其他材料相互作用或受到外界条件的影响时,Si-O键的振动频率和强度可能发生变化。
因此,针对不同条件下的PDMS材料,我们可以通过红外光谱分析来研究Si-O键与其它键的相互作用以及材料性能的变化。
综上所述,聚硅氧烷材料中Si-O键的红外吸收特性研究对于深入理解PDMS材料的性质和应用具有重要意义。
- 1、下载文档前请自行甄别文档内容的完整性,平台不提供额外的编辑、内容补充、找答案等附加服务。
- 2、"仅部分预览"的文档,不可在线预览部分如存在完整性等问题,可反馈申请退款(可完整预览的文档不适用该条件!)。
- 3、如文档侵犯您的权益,请联系客服反馈,我们会尽快为您处理(人工客服工作时间:9:00-18:30)。
Mid-and near-infrared spectroscopic assessment of soil compositional parameters and structural indices in two FerralsolsBeáta E.Madari a,⁎,James B.Reeves III b ,Pedro L.O.A.Machado a ,Cleber M.Guimarães c ,Eleno Torres d ,Gregory W.McCarty eaEmbrapa Solos,Rua Jardim Botânico,1024,22460-000Rio de Janeiro,RJ,BrazilbEMBUL,ANRI,ARS,USDA,BARC East,Beltsville,MD 20705,USAcEmbrapa Rice and Bean,P .O.Box 179,75375-000Santo Antônio de Goiás,GO,BrazildEmbrapa Soybean,P .O.Box 231,86001-970Londrina,PR,Brazil eHRSL,ARS,USDA,BARC West,Beltsville,MD 20705,USAReceived 23November 2004;received in revised form 14December 2005;accepted 24March 2006Available online 15June 2006AbstractBulk soil samples (BS)(n =120)from long-term tillage experiments and areas covered by secondary natural forest were collected in the Brazilian savanna region (Cerrado)at Santo Antônio de Goiás,and southern Atlantic forest region at Londrina.Bulk soils were separated into 8aggregate size fractions (ASF)by wet sieving (n =700).Bulk soil samples (BS)and ASF were analyzed for total C,N by dry combustion.BS were also analyzed for sand,silt,and clay by densitometry,and several measures of soil aggregation were determined.All samples were scanned in the mid-and near-infrared (Mid-IR,NIR)by diffuse reflectance of ground,non-KBr diluted samples using a Digilab FTS-7000FTIR spectrometer equipped with a Pike Auto-Diff auto-sampler.Quantitative calibrations for the various analytes were performed separately for the original set of 120BS samples and the set of 700ASF samples using partial least squares regression (PLSR).Results for the set of 120BS for total C and N were excellent using either spectral range with R 2>0.97,except for C by Mid-IR (R 2=0.93).Similar results were obtained for determinations of sand and clay (R 2>0.94).Silt,determined by difference (100%−%sand −%clay),gave much lower R 2(0.80and 0.63for Mid-IR and NIR,respectively).For aggregation indices,R 2of ∼0.80were achieved in the Mid-IR,NIR results were not as good (R 2<0.67).Efforts with the 700ASF samples resulted in R 2of 0.98and 0.96for C,and 0.96and 0.97for N,for Mid-IR and NIR calibrations,respectively.Calibration efforts to determine the values for the set of 700ASF samples using set of 120BS from which they were fractionated gave R 2=0.90and 0.87in the Mid-IR and NIR,respectively,when 5%of the ASF were added to the set of 120BS.Spectral discrimination of sample source was easily and very accurately determined for all data sets examined.©2006Elsevier B.V .All rights reserved.Keywords:Mid-infrared;Near-infrared;Spectroscopy;Carbon;Chemometrics;PLSR;Soil1.IntroductionIn many research endeavors,large numbers of samples are either required or generated.These samples likely then need to be analyzed for one or more analytes of interest which is often time consuming and expensive.An important example of the need for largescaleGeoderma 136(2006)245–259⁎Corresponding author.Current address:Embrapa Rice and Bean,P.O.Box 179,75375-000Santo Antônio de Goiás,GO,Brazil.E-mail address:madari@cnpaf.embrapa.br (B.E.Madari).0016-7061/$-see front matter ©2006Elsevier B.V .All rights reserved.doi:10.1016/j.geoderma.2006.03.026analyses would be soil C inventory assessments that become mandated under international climate change agreements that include accounting for soil carbon sequestration as may occur under future enforcement periods of the Kyoto Protocol(1997).As an effect of this, the ability to measure carbon sequestration in soils and assess the role of soil in carbon fluxes is becoming more relevant.The Conference of the Parties(COP)of the United Nations Framework Convention on Climate Change(UNFCCC)is making efforts to implement the Kyoto Protocol and to make the necessary modifications required from the International Panel on Climate Change (IPCC)on a special report on the principal aspects of an analytical procedure related to carbon(Watson et al., 2000).It is thus highly probable that there will soon be a strong demand for determination of C in soils for the evaluation of agricultural production and forestry systems in assessment of their role in global climate change mitigation.If this occurs,large numbers of samples will need to be analyzed by fast,accurate and inexpensive means.Among the presently available methods for soil carbon quantification combustion is considered sufficiently accurate(Watson et al.,2000), however,rather expensive.Loss-on-ignition and wet digestion(Nelson and Sommers,1996;Embrapa,1997) are time consuming and require the use of harsh chemicals and are prone to bias(Watson et al.,2000).In many areas of agriculture both commercial and research spectroscopic methods based on near-infrared reflectance spectroscopy(NIRS)have come to be used to reduce the need for chemical base and even other instrumentally based methods of sample analysis (Williams and Norris,1987,2001;Roberts et al., 2004).In NIRS,calibrations are developed using a representative sample set to relate spectral information to the analyte in question(calibrations),for example protein in wheat(Tkachuk,1987),using a process known as chemometrics(Williams and Norris,1987; Beebe et al.,1998;Williams and Norris,2001;Naes et al.,2002;Roberts et al.,2004).While NIRS has come to dominate many areas of analysis in agriculture,the analysis of soils using NIRS has only relatively recently been examined for quantitative analysis(Reeves et al., 1999;Reeves and McCarty,2001;Reeves et al.,2002).While NIRS uses electromagnetic radiation in the 25,000to4000cm−1(400–2500nm)range,diffuse reflectance Fourier Transform mid-infrared(Mid-IR) spectroscopy(DRIFTS)uses radiation in the range from 4000to400cm−1(2500–25,000nm).Virtually all agricultural applications using diffuse reflectance(illu-mination of a ground,non-mirror like sample)are based on NIRS because it was thought that quantitative analysis using DRIFTS was impossible unless samples are diluted with KBr to a concentration of5%or less.The dilution being needed to avoid spectral distortions caused by mirror-like reflection known as specular reflection which does not occur in the near-infrared (NIR)where absorptions are weaker(Reeves,2003a,b). However,efforts have demonstrated that quantitative analysis can be performed using DRIFTS generated spectra in conjunction with chemometrics and they provide strong indications that DRIFTS can outperform NIRS for soils(Reeves,1994,1996;Janik et al.,1998; Reeves et al.,2001;McCarty et al.,2002;Reeves et al., 2002).The objective of this work was to examine the usefulness of DRIFTS and NIRS in the quantitative determination of total carbon and nitrogen in soil samples collected under a case study assessing the mechanism of soil organic carbon accumulation in Ferralsols as affected by soil structural dynamics under traditional and conservation tillage systems and under secondary natural forest(Madari et al.,2005a).In addition,the capability of DRIFTS and NIRS was also examined in assessing the particle size distribution of the soil and the indicators that describe soil aggregate distribution as a measure of soil structural properties.2.Materials and methods2.1.Field sitesThe tillage experiments were located in Londrina (23°23′S;51°11′W)and Santo Antônio de Goiás (16°28″S;49°17″W).In Londrina(southern Atlantic Forest biome),the mean annual temperature is20.7°C with monthly means between11°C in July and31°C in February,and annual rainfall1622mm,two-thirds occurring during the warm season(October to March). The soil type was a Rhodic Ferralsol[WRB(FAO, ISRIC,ISSS,1994)]or Latossolo Vermelho eutroférrico according to the Brazilian Soil Classification System (Embrapa,1999)or Rhodic Hapludox according to the USDA Soil Taxonomy(Soil Survey Staff,1999).The dominant species at the forest site,which is at the same altitude and on the same soil type,were Trichilia clausenii,Euterpe edulis and Andidosperma polyneuron (Oliveira-Filho and Ratter,1995).It is assumed that the same species characterized the site prior to forest clearance ca.1940.The intermediate vegetation at the field site in Londrina comprised pasture grasses (Brachiaria spp.and Penisetum clandestinum)and coffee plantation.The field experiment was established in1987.In Santo Antônio de Goiás,the mean annual246 B.E.Madari et al./Geoderma136(2006)245–259temperature is 23°C,and annual rainfall 1500mm.There are two distinct seasons in this Cerrado biome:the rainy season from September to May and the dry season from June to August.Cultivation of cash crops is limited to the rainy season,but dry spells in the rainy season are also common (Assad et al.,1994).The soil type was a Haplic Ferralsol (WRB)(Latossolo Vermelho-Amarelo distrófico according to the Brazilian Soil Classification System;or Typic Haplustox accord-ing to the USDA Soil Taxonomy).The Cerrado vegetation in this region is characterized by plant species,such as Magonia pubescens ,Dilodendrum bipinnatum and Hirtella glandulosa .Forest was cleared in the 1960s and prior to the establishment of the experiment in 1995the area was covered by Andropo-gum sp.for 6years and lastly by maize –common beans rotation.The dominant mineral of the clay-size fraction (<2μm)was kaolinite in both soils,the soil in Santo Antônio de Goiás showing relatively stronger signs of gibbsite and hematite.Some physico-chemical charac-teristics of the two soils are described in Table 1.In Londrina,the tillage treatments were conventional tillage (disc plough at 18cm depth followed by harrowing twice with light discs at 10cm depth,CT)and no-tillage (no disturbance to the soil other than the sowing operation,NT).Two rotations were a crop succession (SUC)of wheat (Triticum aestivum L.)followed by soybean (Glycine max L.)and a crop rotation (ROT)including white lupine (Lupinus albus L.)/maize (Zea mays L.)–black oat (Avena strigosaSchieb.)/soybean –wheat/soybean –wheat/soybean.In Santo Antônio de Goiás,the tillage treatments (main plots)were conventional tillage (mouldboard plow followed by harrowing twice with light discs,CT)and no-tillage (NT).The subplots were crop succession (SUC),which included fallow/rice (Oriza sativa )–fallow/soybean (G.max )and the rotation (ROT)with sunhemp (Crotalaria juncea )/rice-millet (Pennisetum glaucum L.R.Br.)/soybean.All plots were in triplicate.2.2.Soil samplesBoth tillage experiments were sampled in a similar way.Bulk soil samples were collected from 4different tillage-rotation treatments.Each treatment was sampled in triplicate in the field and at 4depths (0–5,5–10,10–20,20–30cm).In addition soil samples were similarly taken from the nearby forests (4depths from three pits positioned 50m apart),resulting in a total of 120bulk soil (BS)samples [(2areas×4treatments×3replicates×4depths)+(forest:2areas×3replicates×4depths)].2.3.Sample preparationEach bulk soil sample from the 0–5,5–10,10–20cm sampling layer was fractionated into 8aggregate size fractions (ASF)(8–19,4–8,2–4,1–2,0.5–1,0.25–0.5,0.125–0.25,0.053–0.125mm diameter)by wet sieving (Madari et al.,2005a )resulting in 700ASF samples.The ASF samples were dried,and ground to pass an 80-Table 1Chemical properties (A)and particle size distribution (B)of the Rhodic Ferralsol,Londrina,and of the Haplic Ferralsol,Santo Antônio de Goiás(A)Chemical properties pH H 2OAl 3+(cmol c .dm −3)H+Al(cmol c .dm −3)Ca 2+(cmol c .dm −3)Mg 2+(cmol c .dm −3)K +(cmol c .dm −3)P available (mg.kg −1)Rhodic Ferralsol (Londrina)Forest 6.30.1 4.514.9 2.60.5830Experiment 5.5–6.80.1–0.24.4–6.74.2–7.61.5–3.60.23–0.56260–800Haplic Ferralsol (Santo Antônio de Goiás)Forest 4.8 1.28.80.50.20.11Experiment 5.7–6.10.0–0.13.1–4.1 1.3–2.80.9–1.20.2–0.43–9(B)Particle size distribution Sand (g kg −1)Silt (g kg −1)Clay (g kg −1)Rhodic Ferralsol Forest 63211726Experiment 40178782Haplic Ferralsol Forest 310110580Experiment 380127493247B.E.Madari et al./Geoderma 136(2006)245–259mesh sieve for analysis,as was in the same manner for an aliquot of the BS samples.2.4.Conventional sample analysisTotal C and N concentrations of all samples were measured on finely ground samples(80mesh)by dry combustion(Nelson and Sommers,1996;Anonymous, 1999)using a Perkin Elmer2400Series II CHNS/O Analyzer(Perkin Elmer Life and Analytical Sciences, Inc.,Boston,MA,USA).Coefficient of variation of the method was3%.The particle size distribution of the bulk soil samples was determined by densitometry:the sand and clay fractions were separated based on their specific gravity,silt was obtained by deducting sand and clay from the total{silt[g kg−1]=1000−(sand +clay)}(Embrapa,1997).The parameters describing soil aggregate distribution(soil aggregation indices: Meanweight Diameter—MWD,Mean Geometric Di-ameter—MGD and Aggregate Stability Index—AS)of the BS samples were calculated using the results obtained by aggregate size fractionation and particle size analysis as follows:MWD of aggregates(Kemper and Rosenau,1986) MWD¼X ni¼1x i w iwhere w i=proportion of each aggregate class in relation to the whole,x i=mean diameter of the classes(mm).MGD of aggregates(Kemper and Rosenau,1986)MGD¼expP ni¼1w i log x iP ni¼1w i26643775where,w i=weight of aggregates(g)in a size class with an average diameter x i.AS of soils(Castro Filho et al.,1998)AS¼Weight of the dry sampleÀwp25Àsand Weight of the dry sampleÀsandÂ100where wp25=weight of aggregates<0.25mm(g), sand=weight of particles between2.0and0.053mm(g).2.5.Fourier-transformed diffuse reflectance Mid-IR spectroscopy(DRIFTS)Samples were scanned in the Mid-IR on a Digilab (Bio-Rad,Randolph,MA)FTS-7000Fourier Transform spectrometer equipped with a KBr beam splitter and a DGTS(deuterated glycine trisulfate)detector.Samples were scanned from4000to400cm−1(2500to 25,000nm)at4cm−1resolution with64co-added scans per spectrum.Scanning was performed using ground,but non-KBr diluted(Reeves,2003a,b),samples using a Pike Autodiff autosampler/reflectance accessory (Pike Technologies,Madison,WI,USA)with KBr used as the reference standard.2.6.Near-infrared spectroscopySamples were scanned in the near-infrared(NIR)on a Digilab(Bio-Rad,Randolph,MA)FTS-7000Fourier Transform spectrometer equipped with a quartz beam splitter and an InSb liquid nitrogen cooled detector. Samples were scanned from9091to4000cm−1(1100–2500nm)at4cm−1resolution with64co-added scans per spectrum.Scanning was performed using ground samples using the Pike Autodiff autosampler/reflectance accessory with S used as the reference standard. Samples were scanned as originally ground for reference analyses(to pass the80mesh=0.177mm sieve),no further grinding was done prior to DRIFTS and NIRS analysis.2.7.Statistical analysisSummary statistics and correlation analysis were performed using SAS(SAS Institute,1988)PROC Means and FREQ version8.2.8.ChemometricsCalibration development was carried out using SAS PLS with a custom made program(Reeves and Delwiche,2003,2004).All spectra were mean centered and variance scaled.In addition,spectral pre-treatments using first and second gap derivatives with gaps of4–64 data points with and without multiplicative scatter correction(MSC,Martens et al.,1983)were tested.In all,22different spectral pre-treatments were tested for all analytes.Initial testing was performed using different spectral ranges and data point averaging to determine the optimal spectral range to use in the final PLSR calibration development(final calibrations based on average of4data points with entire spectral range used for both Mid-IR and NIR spectra).Calibrations were developed using the one-out cross validation procedure with and without an independent test set.Calibrations were also examined using GRAMS PLSPlus V2.1G (Galactic Industries,Salem,NH,USA).248 B.E.Madari et al./Geoderma136(2006)245–2593.Results and discussion3.1.Sample compositionThe elemental composition(C,N)for the bulk soil(BS) samples and for the aggregate size fractions(ASF)samples are shown in Table2.The particle size distribution and the soil aggregation indices for the BS samples are also shown in Table2.The range of analyte values,even in the BS samples,was fairly wide providing a good data set for the development of NIR or Mid-IR calibrations.It is worthwhile noting that values for silt were sometimes reported as negative due to the method of computation (silt=1000−sand−clay).These values represent the range of accuracy of the method in question and converting them to0could bias calibration equations,thus they were used as computed.The mean C and N values of the BS samples and of the set of all700ASF samples are in the same range.The maximum C and N values however are higher for the set of 700aggregate size fractions.The reason for this is that under certain circumstances,in the present case especially under the natural forest vegetation,more soil organic matter,and consequently more C and N,is accumulated in the microaggregates(the smallest aggregate size fractions) than in the other size fractions(Madari et al.,2005a). 3.2.Mid-IR and NIR spectraShown in Fig.1are representative Mid-IR and NIR spectra of the700aggregate size fractions.Examination shows that both the absolute magnitude and range of the Mid-IR absorptions are much greater than those found for the corresponding NIR spectrum.It was because of this that quantitative analysis using DRIFTS spectra was held to be impossible unless samples were diluted with KBr,an expensive and time consuming practice not required for NIRS(Reeves,2003a,b).The Mid-IR spectrum generally shows more distinctive spectral features than the NIR spectrum.Despite this,with the proper chemometric analysis,NIR spectra can be used to accurately determine the composition of many agricultural products(Roberts et al.,2004;Williams and Norris,1987,2001).Fig.1shows representative Mid-IR and NIR spectra by location(Londrina and Santo Antônio de Goiás).The two areas have two different soil types with difference in mineralogy that is indicated by the Mid-IR spectra.The predominant clay-mineral in the Rhodic Ferralsol of the Atlantic forest biome(Londrina)was kaolinite.The peaks between 3695and3620cm−1and various peaks below 1100cm−1are characteristic for kaolinite.There is also indication for the presence of other clay minerals, such as gibbsite(peaks between3610and3395cm−1, and various peaks below1200cm−1)(White and Roth, 1986).X-ray analysis confirmed the presence of these, and other clay minerals like hematite and vermiculite with interlayer hydroxyl-Al in trace amounts(Madari et al.,2005a),but the identification of the two latter ones was not evident in the Mid-IR spectra due to over-lapping of absorption bands.The Haplic Ferralsol of the Cerrados biome(Santo Antonio de Goiás)had gibbsite and kaolinite as principal constituents of the mineral matrix,and traces of hematite and vermiculite with interlayer hydroxyl-Al,the latter two being difficult to identify in the Mid-IR spectra.X-ray analysis also confirmed the presence of these clay minerals(unpub-lished data).Soil organic matter also has characteristic absorption bands in the Mid-IR range(Table3), however,due to its low concentration in the soil samples (≤89.5g kg−1),and their overlapping with mineral peaks,most of these could not readily be identified by simple visual evaluation in spectra of bulk soil samples. The small bands around2920–2930and2855cm−1 indicates the presence of organic aliphatic C–H stretchings(Fig.1).This band is more evident in the spectra of soil samples having higher concentrations of organic carbon.Other organic peaks are overlapping with the mineral peaks.Viscarra Rossel et al.(2006),in a study on the usefulness of Mid-IR,NIR and visible diffuse reflectance spectroscopy examining Partial Least Squares Regression(PLSR)factor loadings weights, showed that frequencies in the Mid-IR rangeTable2Elemental composition(C,N),particle size distribution,and aggregation indices of the120bulk soil and700aggregate size fraction samplesVariable/analyte Mean StandarddeviationMinimum Maximum(g kg−1)120Bulk soil(bs)samplesCarbon18.25 6.447.9048.00 Nitrogen 1.30 1.360.04 5.60 Sand203.22162.3228.00460.00 Silt145.2955.59−102.00304.00 Clay651.49128.65469.00851.00 MWD a 5.33 2.760.6512.51 MGD b 1.110.090.92 1.31 AS c88.848.5055.5199.76 700Aggregate size fraction(ASF)samplesCarbon20.638.999.4089.50 Nitrogen 1.76 1.030.608.90a Mean weight diameter.b Mean geometric diameter.c Aggregate stability index.249B.E.Madari et al./Geoderma136(2006)245–259corresponding to the absorption of organic compounds.like organic acids.or acidic functional groups,like alkyl,amide and aromatic groups,were indicators of correlation (showed positive peaks)between organic carbon concentrations in the bulk soil samples and the Mid-IR spectra.Interfering factors were kaolin and quartz (showed negative peaks).However it seems that certain parts of the spectra have more usefulinformationFig.1.Representative Mid-IR (DRIFTS)and NIR (NIRS)spectra of the bulk soil (BS)and aggregate size fraction (ASF)samples of the Rhodic Ferralsol (Atlantic forest biome —Londrina)and Haplic Ferralsol (Cerrados biome —Santo Antônio de Goiás)with high and low total C content.250 B.E.Madari et al./Geoderma 136(2006)245–259for the calibration for a certain analyte;efforts with many sample sets using selected spectral ranges have demonstrated that information exists across the whole spectral range used in the ing only parts of the Mid-IR spectra(e.g.leaving out the<1200cm−1 range;unpublished data)resulted in less accurate calibrations than using the whole spectra.Even leaving out ranges where no apparent bands were present(e.g. 2500–2000cm−1)has been shown to degrade PLSR calibrations.The shift in overall baseline in the NIR spectra is likely caused by the overall difference in the particle size distribution versus composition of the samples of the two locations(Fig.2).As it is shown in Table1, the soil from Londrina has a finer texture compared to the other location,which results in a higher baseline.For most types of samples,smaller particle size results in higher reflectance, e.g.lower absor-bances,but for these soils,the higher absorption coefficients for the clay and silt fraction apparently dominates the particle size effect resulting in higher absorbances for the Londrina samples.The NIR spectra features absorption peaks associated with the bending(7075cm−1)and stretching(5219cm−1)of the O–H bonds of free water,and in lattice minerals at around4523cm−1.The peak at4413cm−1in the spectra of the Cerrado soil(Santo Antônio de Goiás) may indicate the difference in the mineral composi-tion compared to the soil from the Atlantic forest biome(Londrina).3.3.Calibrations for the bulk soil(BS)samplesTable4contains the calibration results using Mid-IR(Table4a)and NIR(Table4b)spectral ranges. Calibrations for C,N,sand,clay and location produced R2above0.90using spectra from either spectral range.It is notable however that for theseTable3Absorption bands of carbon in organic bonds in the Mid-IR spectral rangeAbsorptionband,cm−1Assignments Overlapping with mineral peaks3380a O–H stretching of phenolic OH OH-stretching frequency for water molecules(Vermiculite) 3400–3300b O–H stretching(H bonded OH groups),N–H stretching OH-stretching frequency for water molecules(Halloysite,Montmorillonite,Nontronite,Gibbsite)3030a Aromatic C–H stretching2940–2900b Aliphatic C–H stretching2600a O–H stretching of H-bonded–COOH1725–1720b C f O stretching of–COOH and ketones1660–1630b C f O stretching of amide groups(amide I band),quinine C f O and/or C f O of H-bonded conjugated ketones OH-bending frequency for water molecules (Halloysite,Montmorillonite,Nontronite,Vermiculite)1620–1600b Aromatic C f C stretching and/or asymmetric–COO stretching1590–1517b COO–symmetric stretching,N–H deformation+C f Nstretching(amide II band)1525a Aromatic C f C stretching1460–1450b Aliphatic C–H1400–1390b OH deformation and C–O stretching of phenolic OH,C–Hdeformation of CH2and CH3goups,COO–asymmetric stretching1350a Symmetric COO–stretching and/or–CH bending of aliphatics1270a C–OH stretching of phenolic OH1280–1200b C–O stretching and OH deformation of COOH,C–O stretching of aryl ethers1225a C–O stretching and OH deformation of COOH1170–950b C–O stretching of polysaccharides orpolysaccharide-like substancesSi–O of silicate impurities(various clay minerals) 1170a C–OH stretching of aliphatic OH1170c Polysaccharides1070a C–C stretching of aliphatic groups Si–O of silicate impurities(various clay minerals) 1050c Polysaccharides Si–O of silicate impurities(various clay minerals) 830a Aromatic CH out of plane bending Nontronite,Muscovite775a Aromatic CH out of plane bendinga Baes and Bloom(1989).b Stevenson(1994).c Niemeyer et al.(1992).251B.E.Madari et al./Geoderma136(2006)245–259samples,neither spectral range consistently produced the best calibration.In the Mid-IR range,the R 2value was 0.93and the number of factors for the C calibration model with the lowest RMSD (1.73g kg −1)was 4.In the NIR the R 2was 0.90,however,the number of PLS factors for the lowest RMSD (0.721g kg −1)was 10.In general,the fewer the factors used,the better,as the calibration is more apt to be applicable to new samples.These results for Mid-IR corroborate with those found in the literature (0.95by McCarty et al.,2002;0.81–0.95by Madari et al.,2005b ),however for NIR R 2values reported elsewhere were a bit lower (0.86by McCarty et al.,2002;0.96by Reeves et al.,1999;0.74–0.96by Madari et al.,2005b )than our findings.Past efforts have shown that calibrations for C are in general better when using Mid-IR spectra (Reeves et al.,2001;McCarty et al.,2002;Reeves et al.,2002),but,concerning accuracy,that was not the case here.In Fig.3A and C the Mid-IR and NIR calibrations for C are shown,respectively.While the NIR calibration for C show all the data points close to the 1:1line,the data for C by Mid-IR shows more scatter with even a few samples clearly not being determined as well as the others.Since the same sample was scanned and the same chemistries used,the reason for the poorer performance for C by Mid-IR is not readily explained.In the Mid-IR range,the R 2value was 0.99and the number of factors for the N calibration model with the lowest RMSD (0.148g kg −1)was 12.In the NIR the R 2was 0.97,however,the number of PLSR factors for the lowest RMSD (0.233g kg −1)was 3.The accuracy of the calibration with NIR was a bit higher,but in theorder of magnitude,compared with those found in the literature (0.92by Dalal and Henry,1986;0.94by Reeves and McCarty,2001;0.95by Reeves et al.,1999).No comparative results were found in the literature for Mid-IR.Own results,for a heterogeneous sample population (n =1135)in terms of soil type and soil texture (Madari et al.,2005b ),showed R 2=0.95.In Fig.3B the Mid-IR calibration for N is shown.The Mid-IR calibration for N also show little dispersion of data points from the 1:1line,such as the NIR calibration for C (Fig.3C).The ability to discern the location of the samples from the spectra was found to be very easy (R 2=1.00by Mid-IR and 0.999by NIR).Considering the differences in the spectra from the two soils as shown in Fig 1,these results are not surprising.The two locations represent two different soil types with different mineralogy and particle size distribution (Table 1).It is worthwhile noting that the NIR calibration did not use multiplicative scatter correction (MSC)which is designed to remove baseline effects (Martens et al.,1983).ThisindicatesFig.2.Average near-infrared spectra of aggregate size fraction (ASF)samples by location.Table 4AnalyteDerivative Gap a ScatterCorrectionNo.of factors bRMSD c R 2(a)Final calibration results using 120bulk soil (BS)samples and mid-infrared spectra Carbon 2nd 4MSC 4 1.730.93Nitrogen 1st 4MSC 120.1480.99Sand None 0MSC 1115.40.99Silt None 0MSC 1124.70.80Clay None 0MSC 1124.70.96MWD d 2nd 8MSC 5 1.270.79MGD e 2nd 8None 50.04320.79AS f 2nd 8None 5 3.830.80Location 1st 4MSC 130.0186 1.00(b)Final calibration results using 120bulk soil (BS)samples and near-infrared spectra Carbon 2nd 8MSC g100.7210.99Nitrogen 2nd 32MSC 30.2330.97Hydrogen 1st 16None 6 2.410.87Sand 1st 8MSC 517.10.99Silt 1st 4MSC 233.50.64Clay 2nd 64None 332.40.94MWD a2nd 8None 3 1.610.66MGD b2nd 8None 30.05440.67AS c1st 8None 9 2.410.92Location 1st 16None110.02490.99a Number of data points skipped for derivatives.b Number of partial least squares factors used in calibration.c Rootmean squared deviation.d Mean weight diameter.e Mean geometric diameter.f Aggregate stability index.gMultiplicative scatter correction.252 B.E.Madari et al./Geoderma 136(2006)245–259。