Ab Initio Study of Aqueous Hydrochloric Acid
迎浪船舶参数横摇的理论研究

1.2 参数横摇研究进展
long-crest waves,wave group
VII
上海交通大学硕士学位论文
上海交通大学 学位论文原创性声明
本人郑重声明:所呈交的学位论文,是本人在导师的指导下,独立 进行研究工作所取得的成果。除文中已经注明引用的内容外,本论文不 包含任何其他个人或集体已经发表或撰写过的作品成果。对本文的研究 做出重要贡献的个人和集体,均已在文中以明确方式标明。本人完全意 识到本声明的法律结果由本人承担。
4
上海交通大学硕士学位论文
时也导致了船舶在波浪上的稳性特征值的变化。其中,船舶横摇恢复力矩作为保证 船舶安全的最为重要的参数受此变化影响最为严重。传统理论对船舶各个运动模态 的数值估计和预报是在船舶线性运动理论框架下进行的,适应于微幅运动,对于船 舶发生大幅度运动时所呈现强烈的非线性运动无法适用。参数横摇的存在揭示了船 舶海上客货安全和航行效率上存在的危险隐患.其影响强度是船舶频域幅值理念下 安全预报的盲区,因此正确预报船舶参数横摇的发生范围和危险程度势在必行。 1.1.2 研究目的
手段保存和汇编本学位论文。
保密□,在 本学位论文属于
不保密□。
年解密后适用本授权书。
(请在以上方框内打“√”)
学位论文作者签名:常永全
日期: 年 月 日
指导教师签名:缪国平
日期: 年 月
IV
上海交通大学硕士学位论文
水凝胶 英语作文
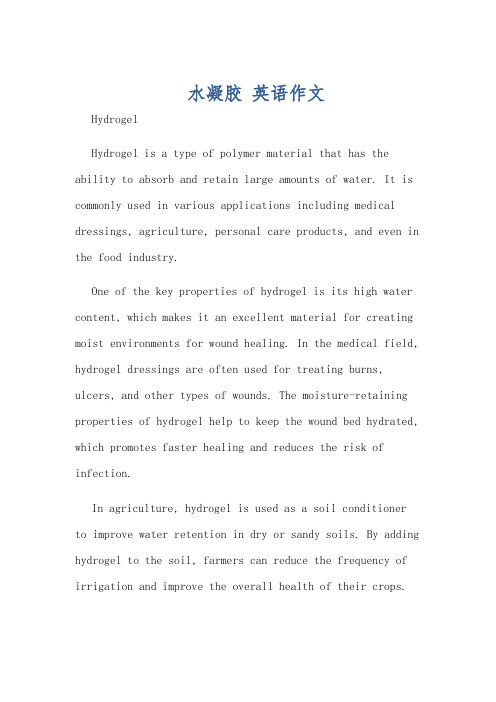
水凝胶英语作文HydrogelHydrogel is a type of polymer material that has the ability to absorb and retain large amounts of water. It is commonly used in various applications including medical dressings, agriculture, personal care products, and even in the food industry.One of the key properties of hydrogel is its high water content, which makes it an excellent material for creating moist environments for wound healing. In the medical field, hydrogel dressings are often used for treating burns, ulcers, and other types of wounds. The moisture-retaining properties of hydrogel help to keep the wound bed hydrated, which promotes faster healing and reduces the risk of infection.In agriculture, hydrogel is used as a soil conditionerto improve water retention in dry or sandy soils. By adding hydrogel to the soil, farmers can reduce the frequency of irrigation and improve the overall health of their crops.This is particularly beneficial in regions with limited water resources or in areas that are prone to drought.In personal care products, hydrogel is commonly found in products such as contact lenses, facial masks, and moisturizing creams. Its ability to retain water makes it an ideal ingredient for products designed to hydrate and nourish the skin.In the food industry, hydrogel is used as a thickening agent and stabilizer in various food products. It can be found in a wide range of items including sauces, dressings, and bakery items. Its ability to absorb and retain water makes it useful for creating desired textures and improving the shelf life of food products.Overall, hydrogel is a versatile and useful material with a wide range of applications. Its unique properties make it an important component in various industries, contributing to advancements in healthcare, agriculture, personal care, and food technology.水凝胶水凝胶是一种聚合物材料,具有吸收和保持大量水分的能力。
hydrolysis词根 -回复
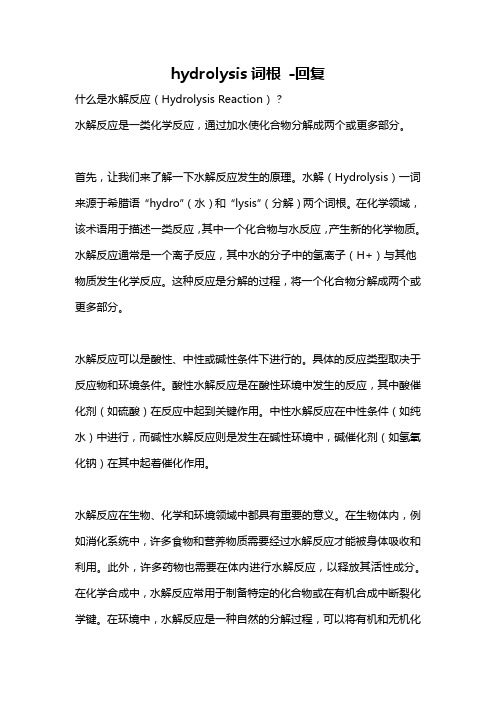
hydrolysis词根-回复什么是水解反应(Hydrolysis Reaction)?水解反应是一类化学反应,通过加水使化合物分解成两个或更多部分。
首先,让我们来了解一下水解反应发生的原理。
水解(Hydrolysis)一词来源于希腊语“hydro”(水)和“lysis”(分解)两个词根。
在化学领域,该术语用于描述一类反应,其中一个化合物与水反应,产生新的化学物质。
水解反应通常是一个离子反应,其中水的分子中的氢离子(H+)与其他物质发生化学反应。
这种反应是分解的过程,将一个化合物分解成两个或更多部分。
水解反应可以是酸性、中性或碱性条件下进行的。
具体的反应类型取决于反应物和环境条件。
酸性水解反应是在酸性环境中发生的反应,其中酸催化剂(如硫酸)在反应中起到关键作用。
中性水解反应在中性条件(如纯水)中进行,而碱性水解反应则是发生在碱性环境中,碱催化剂(如氢氧化钠)在其中起着催化作用。
水解反应在生物、化学和环境领域中都具有重要的意义。
在生物体内,例如消化系统中,许多食物和营养物质需要经过水解反应才能被身体吸收和利用。
此外,许多药物也需要在体内进行水解反应,以释放其活性成分。
在化学合成中,水解反应常用于制备特定的化合物或在有机合成中断裂化学键。
在环境中,水解反应是一种自然的分解过程,可以将有机和无机化合物分解为其原始构成部分。
让我们以生物降解为例来具体探讨水解反应的步骤和机制。
生物降解是指生物体通过生物代谢将复杂有机化合物降解为简单物质的过程。
许多生物降解过程涉及到水解反应。
例如,纸张在湿润环境中逐渐分解成纤维素,这是因为纤维素分子被水解为单糖单位。
这个过程涉及一个水解反应,其中纤维素被水分解为较小的分子。
水解反应的机制可以分为两个主要步骤:断裂和形成。
首先,水分子中的氢离子(H+)与化合物中的化学键之一发生反应,导致这个化合物的分子被断裂。
例如,在酸性环境中,水的氢离子与酯连接反应,分解成羧酸和醇。
这个断裂过程通常需要一定的能量输入。
亚硫酸氢铵 低温结晶

亚硫酸氢铵低温结晶英文回答:Ammonium bisulfite is a white crystalline solid that is soluble in water. It is used as a reducing agent, an antioxidant, and a preservative. It is also used in the production of paper, textiles, and dyes.When ammonium bisulfite is cooled to low temperatures, it crystallizes into a white solid. The crystals are typically small and needle-shaped. The crystallization process can be accelerated by seeding the solution with a small amount of ammonium bisulfite crystals.The crystallization of ammonium bisulfite is a reversible process. When the temperature is raised, the crystals will dissolve and the solution will become clear again.中文回答:亚硫酸氢铵是一种白色晶体固体,可溶于水。
它用作还原剂、抗氧化剂和防腐剂。
它也用于造纸、纺织和染料的生产。
当亚硫酸氢铵冷却至低温时,会结晶成白色固体。
晶体通常很小且呈针状。
通过向溶液中加入少量亚硫酸氢铵晶体,可以加速结晶过程。
亚硫酸氢铵的结晶是一个可逆的过程。
当温度升高时,晶体会溶解并且溶液将再次变为透明。
有研粉末新材料股份有限公司上交所科创板上市
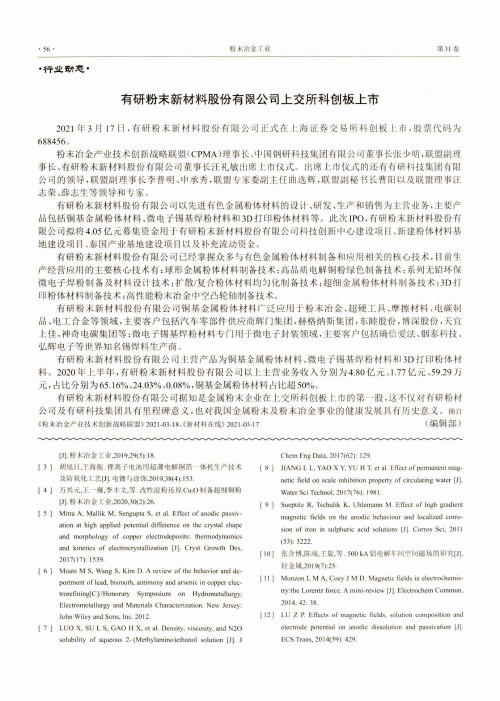
• 56 •粉末冶金工业第31卷•行业动灸*有研粉末新材料股份有限公司上交所科创板上市2021年3月17日,有研粉末新材料股份有限公司正式在上海证券交易所科创板上市,股票代码为 688456=粉末冶金产业技术创新战略联盟(CPMA)理事长、中国钢研科技集团有限公司董事长张少明,联盟副理 事长、有研粉末新材料股份有限公司董事长汪礼敏出席上市仪式。
出席上市仪式的还有有研科技集团有限 公司的领导,联盟副理事长李普明、申承秀,联盟专家委副主任曲选辉,联盟副秘书长曹阳以及联盟理事汪 志荣、薛志生等领导和专家。
有研粉末新材料股份有限公司以先进有色金属粉体材料的设计、研发、生产和销售为主营业务,主要产 品包括铜基金属粉体材料、微电子锡基焊粉材料和3D打印粉体材料等。
此次1PO,有研粉末新材料股份有 限公司拟将4.05亿元募集资金用于有研粉末新材料股份有限公司科技创新中心建设项目、新建粉体材料基 地建设项目、泰国产业基地建设项目以及补充流动资金。
有研粉末新材料股份有限公司己经掌握众多与有色金属粉体材料制备和应用相关的核心技术,目前生 产经营应用的主要核心技术有:球形金属粉体材料制备技术;高品质电解铜粉绿色制备技术;系列无铅环保 微电子焊粉制备及材料设计技术;扩散/复合粉体材料均匀化制备技术;超细金属粉体材料制备技术;3D打 印粉体材料制备技术;高性能粉末冶金中空凸轮轴制备技术。
有研粉末新材料股份有限公司铜基金属粉体材料广泛应用于粉末冶金、超硬工具、摩擦材料、电碳制 品、电工合金等领域,主要客户包括汽车零部件供应商辉门集团,赫格纳斯集团,东睦股份,博深股份,天宜 上佳,神奇电碳集团等;微电子锡基焊粉材料专门用于微电子封装领域,主要客户包括确信爱法、铟泰科技、弘辉电子等世界知名锡焊料生产商。
有研粉末新材料股份有限公司主营产品为铜基金属粉体材料、微电子锡基焊粉材料和3D打印粉体材 料。
2020年上半年,有研粉末新材料股份有限公司以上主营业务收入分别为4.80亿元、丨.77亿元、59.29万元,占比分别为65.16%、24.03%、0.08%,铜基金属粉体材料占比超50%。
AQUEOUS SOLUBILITY OF INORGANIC COMPOUNDS AT VARIOUS TEMPERATURES
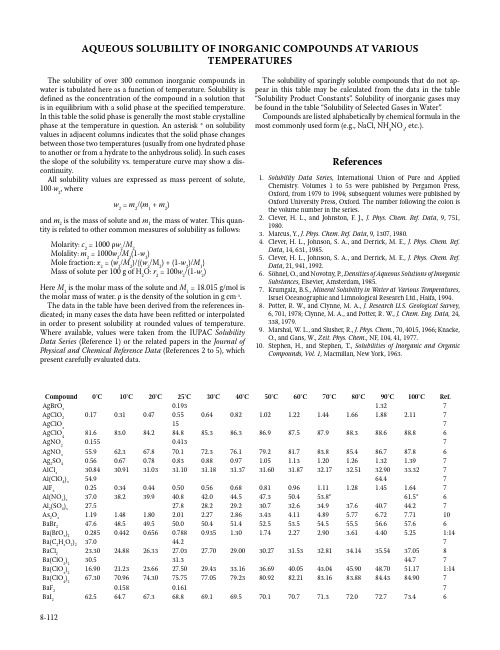
AQUEOUS SOLUBILITY OF INORGANIC COMPOUNDS AT VARIOUSTEMPERATURESThe solubility of over 300 common inorganic compounds in water is tabulated here as a function of temperature. Solubility is defined as the concentration of the compound in a solution that is in equilibrium with a solid phase at the specified temperature. In this table the solid phase is generally the most stable crystalline phase at the temperature in question. An asterisk * on solubility values in adjacent columns indicates that the solid phase changes between those two temperatures (usually from one hydrated phase to another or from a hydrate to the anhydrous solid). In such cases the slope of the solubility vs. temperature curve may show a dis-continuity.All solubility values are expressed as mass percent of solute, 100⋅w2, wherew2 = m2/(m1 + m2)and m2 is the mass of solute and m1 the mass of water. This quan-tity is related to other common measures of solubility as follows: Molarity: c2 = 1000 ρw2/M2Molality: m2 = 1000w2/M2(1-w2)Mole fraction: x2 = (w2/M2)/{(w2/M2) + (1-w2)/M1}Mass of solute per 100 g of H2O: r2 = 100w2/(1-w2)Here M2 is the molar mass of the solute and M1 = 18.015 g/mol is the molar mass of water. ρ is the density of the solution in g cm-3. The data in the table have been derived from the references in-dicated; in many cases the data have been refitted or interpolated in order to present solubility at rounded values of temperature. Where available, values were taken from the IUPAC Solubility Data Series (Reference 1) or the related papers in the Journal of Physical and Chemical Reference Data (References 2 to 5), which present carefully evaluated data.The solubility of sparingly soluble compounds that do not ap-pear in this table may be calculated from the data in the table “Solubility Product Constants”. Solubility of inorganic gases may be found in the table “Solubility of Selected Gases in Water”. Compounds are listed alphabetically by chemical formula in the most commonly used form (e.g., NaCl, NH4NO3, etc.).References1. Solubility Data Series, International Union of Pure and AppliedChemistry. Volumes 1 to 53 were published by Pergamon Press, Oxford, from 1979 to 1994; subsequent volumes were published by Oxford University Press, Oxford. The number following the colon is the volume number in the series.2. Clever, H. L., and Johnston, F. J., J. Phys. Chem. Ref. Data, 9, 751,1980.3. Marcus, Y., J. Phys. Chem. Ref. Data, 9, 1307, 1980.4. Clever, H. L., Johnson, S. A., and Derrick, M. E., J. Phys. Chem. Ref.Data, 14, 631, 1985.5. Clever, H. L., Johnson, S. A., and Derrick, M. E., J. Phys. Chem. Ref.Data, 21, 941, 1992.6. Söhnel, O., and Novotny, P., Densities of Aqueous Solutions of InorganicSubstances, Elsevier, Amsterdam, 1985.7. Krumgalz, B.S., Mineral Solubility in Water at Various Temperatures,Israel Oceanographic and Limnological Research Ltd., Haifa, 1994.8. Potter, R. W., and Clynne, M. A., J. Research U.S. Geological Survey,6, 701, 1978; Clynne, M. A., and Potter, R. W., J. Chem. Eng. Data, 24, 338, 1979.9. Marshal, W. L., and Slusher, R., J. Phys. Chem., 70, 4015, 1966; Knacke,O., and Gans, W., Zeit. Phys. Chem., NF, 104, 41, 1977.10. Stephen, H., and Stephen, T., Solubilities of Inorganic and OrganicCompounds, Vol. 1, Macmillan, New York, 1963.Compound0°C10°C20°C25°C30°C40°C50°C60°C70°C80°C90°C100°C Ref. AgBrO30.193 1.327 AgClO20.170.310.470.550.640.82 1.02 1.22 1.44 1.66 1.88 2.117 AgClO3157 AgClO481.683.084.284.885.386.386.987.587.988.388.688.86 AgNO20.1550.4137 AgNO355.962.367.870.172.376.179.281.783.885.486.787.86 Ag2SO40.560.670.780.830.880.97 1.05 1.13 1.20 1.26 1.32 1.397 AlCl330.8430.9131.0331.1031.1831.3731.6031.8732.1732.5132.9033.327 Al(ClO4)354.964.47 AlF30.250.340.440.500.560.680.810.96 1.11 1.28 1.45 1.647 Al(NO3)337.038.239.940.842.044.547.350.453.8*61.5*6 Al2(SO4)327.527.828.229.230.732.634.937.640.744.27 As2O3 1.19 1.48 1.80 2.01 2.27 2.86 3.43 4.11 4.89 5.77 6.727.7110 BaBr247.648.549.550.050.451.452.553.554.555.556.657.66 Ba(BrO3)20.2850.4420.6560.7880.935 1.30 1.74 2.27 2.90 3.61 4.40 5.251:14 Ba(C2H3O2)237.044.27 BaCl223.3024.8826.3327.0327.7029.0030.2731.5332.8134.1435.5437.058 Ba(ClO2)230.531.344.77 Ba(ClO3)216.9021.2323.6627.5029.4333.1636.6940.0543.0445.9048.7051.171:14 Ba(ClO4)267.3070.9674.3075.7577.0579.2380.9282.2183.1683.8884.4384.907 BaF20.1580.1617 BaI262.564.767.368.869.169.570.170.771.372.072.773.468-112Compound0°C10°C20°C25°C30°C40°C50°C60°C70°C80°C90°C100°C Ref. Ba(IO3)20.01820.02620.03420.03960.045*0.058*0.0730.0900.1090.1310.1560.1821:14 Ba(NO2)231.136.641.844.346.851.656.260.564.668.572.175.610 Ba(NO3)2 4.7 6.38.29.310.212.414.717.019.321.523.525.56 Ba(OH)2 1.67 4.688.4193352741007 BaS 2.79 4.78 6.978.219.5812.6716.1820.0524.1928.5533.0437.617 Ba(SCN)262.67 BaSO30.00111:26 BeCl240.541.77 Be(ClO4)259.57 BeSO426.6927.5828.6129.2229.9031.5133.3935.5037.7840.2142.7245.287 CaBr2555659616368717310 CaCl236.7039.1942.1344.83*49.12*52.85*56.05*56.7357.4458.2159.0459.948 Ca(ClO3)263.264.265.566.367.269.071.073.275.5*77.4*77.778.01:14 Ca(ClO4)265.37 CaF20.00130.001610 CaI264.666.067.668.369.070.872.474.076.078.079.681.07 Ca(IO3)20.0820.1550.2430.3050.384*0.517*0.5900.6520.811*0.665*0.6681:14 Ca(NO2)238.639.544.548.67 Ca(NO3)250.153.156.759.060.965.477.878.178.278.378.478.56 CaSO30.00590.00540.00490.00410.00350.00300.00260.00230.00200.00191:26 CaSO40.1740.1910.2020.2050.2080.2100.2070.2010.1930.1840.1730.1639 CdBr236.043.049.953.456.460.3*60.3*60.560.760.961.361.66 CdC2O40.00605 CdCl247.250.153.254.656.3*57.3*57.557.858.158.5158.9859.56 Cd(ClO4)258.766.97 CdF2 5.82 4.65 4.18 3.765 CdI244.144.945.846.346.847.949.050.251.552.754.155.46 Cd(IO3)20.0915 Cd(NO3)255.457.159.661.062.866.570.686.186.586.887.187.46 CdSO443.143.143.243.443.644.143.542.541.440.238.536.76 CdSeO442.0440.5939.0238.1837.2935.3533.1530.6527.8424.6921.2417.495 Ce(NO3)357.9959.8061.8963.0564.31*67.0*68.671.1*74.9*79.280.983.11:13 CoCl230.3032.6034.8735.9937.1039.2741.3843.4645.5047.5149.5151.507 Co(ClO4)250.053.07 CoF2 1.47 CoI258.0061.7865.3566.9968.5171.1773.4175.2976.8978.2879.5280.707 Co(NO2)20.0760.497 Co(NO3)245.547.049.450.852.456.060.162.664.967.76 CoSO419.923.026.127.729.232.334.435.935.533.230.627.86 Co(SCN)250.77 CrO362.262.362.662.863.063.564.164.765.566.267.167.96 CsBr55.27 CsBrO3 1.16 1.93 3.01 3.69 4.46 6.328.6011.3214.4517.9621.8325.981:30 CsCl61.8363.4864.9665.6466.2967.5068.6069.6170.5471.4072.2172.961:47 CsClO3 2.40 3.87 5.947.228.6912.1516.3321.1426.4532.1037.8943.421:30 CsClO40.79 1.01 1.51 1.96 2.57 4.28 6.559.2912.4115.8019.3923.077 CsI30.937.243.245.948.653.357.360.763.665.967.769.26 CsIO3 1.08 1.58 2.21 2.59 3.02 3.96 5.06 6.297.709.2010.7912.451:30 CsNO38.4613.018.621.825.132.039.045.751.957.362.166.26 CsOH757 Cs2SO462.663.464.164.564.865.566.166.767.367.868.368.86 CuBr255.87 CuCl240.841.742.643.143.744.846.047.248.549.951.352.76 Cu(ClO4)254.359.37 CuF20.0757 Cu(NO3)245.249.856.359.261.162.063.164.565.967.569.271.06 CuSO412.414.416.718.019.322.225.428.832.436.340.343.56 CuSeO410.616.07 Dy(NO3)358.7959.9961.4962.3563.2965.4368.0471.581:13 Er(NO3)361.5863.1564.8465.7566.6968.7070.9673.6477.751:13 Eu(NO3)355.256.758.559.460.462.564.61:13Compound0°C10°C20°C25°C30°C40°C50°C60°C70°C80°C90°C100°C Ref. FeBr254.664.8*7 FeCl233.2*39.4*48.7*7 FeCl342.744.947.947.751.674.876.784.684.384.384.484.76 Fe(ClO4)263.3967.767 FeF3 5.597 Fe(NO3)340.1546.577 Fe(NO3)241.4446.677 FeSO413.517.020.822.824.828.832.835.533.630.427.124.06 Gd(NO3)356.357.759.260.161.062.965.267.971.51:13 HIO373.4574.1074.9875.4876.0377.2078.4679.7881.1382.4883.8285.141:30 H3BO3 2.61 3.57 4.77 5.48 6.278.1010.312.915.919.323.127.36 HgBr20.260.370.520.610.720.96 1.26 1.63 2.08 2.61 3.23 3.954 Hg(CN)2 6.577.839.3310.211.113.115.518.221.224.628.332.36 HgCl2 4.24 5.05 6.17 6.817.629.5312.0215.1819.1624.0629.9036.624 HgI20.00410.00550.00720.01220.01994 Hg(SCN)20.0704 Hg2Cl20.00043 Hg2(ClO4)273.879.8*85.3*7 Hg2SO40.0380.0430.0480.0510.0540.0590.0650.0700.0760.0820.0880.0934 Ho(NO3)363.81:13 KBF40.280.340.450.550.75 1.38 2.09 2.82 3.58 4.34 5.12 5.9010 KBr35.037.339.440.441.443.244.846.247.648.849.850.86 KBrO3 2.97 4.48 6.427.558.7911.5714.7118.1421.7925.5729.4233.281:30 KC2H3O268.4070.2972.0972.9273.7075.0876.2777.3178.2279.0479.8080.557 KCl21.7423.6125.3926.2227.0428.5930.0431.4032.6633.8634.9936.051:47 KClO3 3.03 4.67 6.747.939.2112.0615.2618.7822.6526.8831.5336.651:30 KClO40.70 1.10 1.67 2.04 2.47 3.54 4.94 6.748.9911.7114.9418.676 KF30.9039.847.350.4153.260.07 KHCO318.6221.7324.9226.628.1331.3234.4637.5140.456 KHSO427.129.732.333.635.037.840.543.446.249.0251.8254.66 KH2PO411.7414.9118.2519.9721.7725.2828.9532.7636.7540.9645.4150.121:31 KI56.057.659.059.760.461.662.863.864.865.766.667.46 KIO3 4.53 5.967.578.449.3411.0913.2215.2917.4119.5821.7824.031:30 KIO40.160.220.370.510.70 1.24 1.96 2.83 3.82 4.89 6.027.177 KMnO4 2.74 4.12 5.967.068.2811.1114.4218.166 KNO273.774.675.375.776.076.777.478.078.579.179.680.16 KNO312.017.624.227.731.338.645.752.258.063.067.370.86 KOH48.750.853.254.756.157.958.659.560.661.863.164.66 KSCN63.866.469.170.471.674.176.578.981.183.385.387.36 K2CO351.351.752.352.753.154.054.956.057.258.459.661.06 K2CrO437.138.138.939.439.840.541.341.942.643.243.844.36 K2Cr2O7 4.307.1210.913.115.520.826.331.736.941.545.548.96 K2HAsO448.5*63.6*79.8*7 K2HPO457.059.161.562.764.167.7*72.7*1:31 K2MoO464.766.57 K2SO351.3051.3951.4951.5551.6251.7651.9352.1152.3252.5452.7953.061:26 K2SO47.118.469.9510.711.412.914.215.516.717.718.619.36 K2S2O349.0*62.3*75.7*7 K2S2O522.126.731.133.135.239.042.646.049.152.054.61:26 K2SeO368.4*68.5*68.5*7 K2SeO452.7052.9353.1753.3053.4353.7053.9954.3054.6154.9455.2655.607 K3AsO451.5*55.6*73*7 K3Fe(CN)623.927.631.132.834.337.239.641.743.545.046.147.06 K3PO444.351.47 K4Fe(CN)612.517.322.023.925.629.232.535.538.240.641.443.16 LaCl349.048.548.648.949.350.552.154.056.358.961.76 La(NO3)355.056.958.960.061.163.666.369.9*74.1*1:13 LiBr58.460.162.764.465.967.868.369.069.870.771.772.86 LiBrO361.0362.6264.4465.4466.5168.9071.68*73.24*74.4375.6676.9378.321:30 LiC2H3O223.7626.4929.4231.0232.7236.4840.6545.1549.9354.9160.0465.267 LiCl40.4542.46*45.29*45.8146.2547.3048.4749.7851.2752.9854.98*56.34*1:47Compound0°C10°C20°C25°C30°C40°C50°C60°C70°C80°C90°C100°C Ref. LiClO373.275.6*80.8*82.183.485.9*87.1*88.289.691.393.495.71:30 LiClO430.132.635.537.038.641.945.549.253.257.261.371.46 LiF0.1200.1260.1310.1347 LiH2PO455.87 LiI59.460.561.762.363.064.365.867.368.881.381.782.66 LiIO343.81:30 LiNO24145495153566063666810 LiNO334.837.642.750.557.960.162.264.065.767.268.569.76 LiOH10.810.811.011.111.311.712.212.713.414.215.116.16 LiSCN54.57 Li2CO3 1.54 1.43 1.33 1.28 1.24 1.15 1.070.990.920.850.780.727 Li2C2O4 5.877 Li2HPO39.078.407.777.477.18 6.64 6.16 5.71 5.30 4.91 4.53 4.167 Li2SO426.325.925.625.525.325.024.824.524.324.023.823.66 Li3PO40.0271:31 Lu(NO3)371.11:13 MgBr249.349.850.350.650.951.552.152.853.554.255.055.76 Mg(BrO3)243.045.248.049.451.054.357.961.665.369.0*70.9*71.71:14 Mg(C2H3O2)236.1837.5538.9239.617 MgC2O40.0387 MgCl233.9634.8535.5835.9036.2036.7737.3437.9738.7139.6240.7542.158 Mg(ClO3)253.3554.4056.8158.6660.91*65.46*67.3369.2771.0172.4473.481:14 Mg(ClO4)247.848.749.650.150.551.352.16 MgCrO432.06*35.39*7 MgCr2O758.967.07 MgF20.0137 MgI254.756.158.259.460.863.965.065.065.065.065.165.26 Mg(IO3)2 3.19* 6.70*7.928.529.1110.4511.9913.715.617.619.61:14 Mg(NO2)2477 Mg(NO3)238.439.540.841.642.444.145.947.950.052.270.672.06 MgSO30.320.370.460.520.610.87*0.85*0.760.690.640.620.601:26 MgSO418.221.725.126.328.230.933.435.636.935.934.733.36 MgS2O330.734.17 MgSeO431.4*35.7*47*7 MnBr256.0057.7259.3960.1960.9662.4163.7565.0166.1967.3268.4269.507 MnCl238.740.642.543.644.747.049.454.154.755.255.756.16 MnF20.80* 1.01*0.487 Mn(IO3)20.270.347 Mn(NO3)250.561.77 MnSO434.637.338.638.938.937.736.334.632.830.828.826.76 NH4Br37.540.242.743.945.147.349.451.353.054.656.157.47 NH4Cl22.9225.1227.2728.3429.3931.4633.5035.4937.4639.4041.3343.241:47 NH4ClO410.814.117.819.721.725.829.833.637.340.743.846.66 NH4F41.743.244.745.546.347.849.350.952.554.17 NH4HCO310.613.717.619.922.427.934.241.449.358.167.678.07 NH4H2AsO425.229.032.734.536.339.743.146.249.352.255.07 NH4H2PO417.822.026.428.831.236.241.647.253.059.265.772.47 NH4I60.762.163.464.064.665.866.867.868.769.670.471.16 NH4IO3 3.70 4.20 5.647.631:30 NH4NO255.759.064.968.87 NH4NO354.060.165.568.070.374.377.780.883.485.888.290.36 NH4SCN64.481.17 (NH4)2C2O4 2.31 3.11 4.25 4.94 5.737.569.7312.215.118.321.825.77 (NH4)2HPO436.438.240.041.042.044.146.248.550.953.355.958.67 (NH4)2S2O565.567.969.870.571.372.372.973.11:26 (NH4)2S2O837.0040.4543.8445.4947.1150.2553.2856.2359.1362.007 (NH4)2SO332.234.937.739.140.643.747.050.654.558.91:26 (NH4)2SO441.342.142.943.343.844.745.646.647.548.549.550.56 (NH4)2SeO349.051.153.454.756.058.962.065.469.17 (NH4)2SeO454.027 (NH4)3PO415.57Compound0°C10°C20°C25°C30°C40°C50°C60°C70°C80°C90°C100°C Ref. NaBr44.445.947.748.649.651.653.754.154.354.554.754.96 NaBrO320.023.2226.6528.2829.8632.8335.5538.0540.3742.521:30 NaCHO230.837.945.748.750.652.053.555.06 NaC2H3O226.528.831.833.535.539.945.158.359.360.561.762.96 NaCl26.2826.3226.4126.4526.5226.6726.8427.0327.2527.5027.7828.051:47 NaClO22.744.47 NaClO297.0*95.3*7 NaClO344.2746.6749.350.151.253.655.557.058.560.563.367.11:30 NaClO461.964.166.267.268.370.472.574.174.775.476.176.76 NaF 3.52 3.72 3.89 3.97 4.05 4.20 4.34 4.46 4.57 4.66 4.75 4.826 NaHCO3 6.487.598.739.329.9111.1312.4013.7015.0216.3717.7319.107 NaHSO422.233.310 NaH2PO436.5441.0746.0048.6851.5457.89*61.7*62.3*65.968.71:31 NaI61.262.463.964.865.767.769.872.074.774.874.975.16 NaIO3 2.43 4.407.78*8.65*9.6011.6713.9916.5219.25*21.1*22.924.71:30 NaIO412.627 NaNO241.943.445.145.946.848.750.752.855.057.259.561.86 NaNO342.244.446.647.748.851.053.255.357.559.661.763.86 NaOH30394650535863677174767910 NaSCN52.957.160.262.763.564.265.065.966.967.969.06 Na2B4O7 1.23 1.71 2.50 3.07 3.82 6.029.714.917.119.923.528.06 Na2CO3 6.4410.817.923.528.732.832.231.731.331.130.930.96 Na2C2O4 2.62 2.95 3.30 3.48 3.65 4.00 4.36 4.71 5.06 5.41 5.75 6.086 Na2CrO422.632.344.646.746.948.951.053.455.355.555.856.16 Na2Cr2O762.163.164.465.266.168.070.172.374.677.079.680.76 Na2HAsO4 5.6*29.3*67*7 Na2HPO4 1.66 4.197.5110.5516.34*35.17*44.64*45.2046.8148.7850.5251.531:31 Na2MoO430.638.839.439.439.840.341.041.742.643.544.545.56 Na2S11.113.215.717.118.622.126.728.130.233.036.441.06 Na2SO312.016.120.923.526.3*27.3*25.924.823.722.822.121.51:26 Na2SO416.1321.9429.22*32.35*31.5530.9030.3930.0229.7929.678 Na2S2O333.136.340.643.345.952.062.365.768.869.470.171.06 Na2S2O538.439.540.040.641.843.044.245.546.848.149.51:26 Na2SeO347.3*45*7 Na2SeO411.736.9*42.1*7 Na2WO441.641.942.342.642.943.644.445.346.247.348.449.56 Na3PO4 4.287.3010.812.614.116.622.928.432.437.640.443.56 Na4P2O7 2.23 3.28 4.81 6.627.0010.1014.3820.0727.3136.0332.3730.676 NdCl349.049.349.750.050.451.252.253.354.555.857.158.56 Nd(NO3)355.7657.4959.3760.3861.4363.6966.2769.471:13 NiCl234.736.138.540.341.742.143.245.046.146.246.446.66 Ni(ClO4)251.152.87 NiF2 2.50 2.527 NiI255.4057.6859.7860.6961.5062.8063.7364.3864.8065.0965.307 Ni(NO3)244.146.048.449.851.354.658.361.063.165.667.969.06 NiSO421.424.427.428.830.3*32.0*34.135.837.739.942.344.86 Ni(SCN)235.487 NiSeO421.626.2*45.6*7 PbBr20.4490.6200.8410.966 1.118 1.46 1.892 PbCl20.660.810.98 1.07 1.17 1.39 1.64 1.93 2.24 2.60 2.99 3.422 Pb(ClO4)281.57 PbF20.06030.06490.06700.06932 PbI20.0410.0520.0670.0760.0860.1120.1440.1870.2430.3152 Pb(IO3)20.00257 Pb(NO3)228.4632.1335.6737.3839.0542.2245.1747.9050.4252.7254.8256.752 PbSO40.00330.00380.00420.00440.00470.00520.00582 PrCl348.048.148.649.049.550.852.354.156.158.36 Pr(NO3)357.5059.2061.1662.2463.40*65.7*67.870.273.41:13 RbBr47.450.152.653.854.957.058.860.662.163.564.865.96 RbBrO30.97 1.55 2.36 2.87 3.45 4.87 6.648.7811.2914.1517.3220.761:30 RbCl43.5845.6547.5348.4249.2750.8652.3453.6754.9256.0857.1658.151:47Compound0°C10°C20°C25°C30°C40°C50°C60°C70°C80°C90°C100°C Ref. RbClO3 2.10 3.38 5.14 6.227.4510.3513.8517.9322.5327.5732.9638.601:30 RbClO41 1.5177 RbF757 RbHCO353.77 RbI55.858.661.162.363.465.467.268.870.371.672.773.86 RbIO3 1.09 1.53 2.07 2.38 2.74 3.52 4.41 5.42 6.527.749.0010.361:30 RbNO316.425.034.639.444.253.160.867.272.276.179.081.26 RbOH63.47 Rb2CrO438.2743.267 Rb2SO427.330.032.533.734.836.938.740.341.843.044.144.96 SbCl385.790.87 SbF379.483.17 Sc(NO3)357.059.361.662.863.966.268.51:13 Sm(NO3)354.8356.3358.0859.0560.0862.3865.05*68.1*70.874.21:13 SmCl348.048.248.448.649.250.06 SnCl246647 SnI20.97 3.877 SrBr246.048.350.651.752.955.257.659.962.364.666.869.06 Sr(BrO3)218.5322.0025.3927.0228.5931.5534.2136.5738.64*40.2*40.841.01:14 SrCl231.9432.9334.4335.3736.4338.9341.9445.44*46.81*47.6948.7049.878 Sr(ClO2)213.013.614.114.314.514.915.315.615.97 Sr(ClO3)263.2963.4263.6463.7763.9364.2964.7065.1665.6566.1866.7467.311:14 Sr(ClO4)270.04*75.35*78.44*7 SrF20.0110.0217 SrI262.562.863.563.964.565.867.369.070.872.774.779.26 Sr(IO3)20.1020.1260.1520.1650.1790.2060.2330.2590.2840.3070.3280.3461:14 Sr(MnO4)2 2.57 Sr(NO2)241.944.358.67 Sr(NO3)228.234.641.044.547.047.447.948.448.949.550.150.76 Sr(OH)20.9 2.27 SrSO30.00151:26 SrSO40.01357 SrS2O38.813.217.720.022.226.87 Tb(NO3)360.661.021:13 Tl2SO4 2.65 3.56 4.61 5.19 5.807.098.469.8911.3312.7714.1815.536 Tm(NO3)367.91:13 UO2(NO3)249.5251.8254.4255.8557.5561.5967.071:55 Y(NO3)355.5756.9358.7559.8661.11*63.3*64.967.972.51:13 Yb(NO3)370.51:13 ZnBr279.380.181.883.084.185.685.886.186.386.686.887.16 ZnC2O40.00100.00190.00265 ZnCl276.679.080.381.481.882.483.083.784.485.286.06 Zn(ClO4)244.29*46.27*48.707 ZnF2 1.535 ZnI281.181.281.381.481.581.782.082.382.683.083.383.76 Zn(IO3)20.580.640.690.770.825 Zn(NO3)247.850.854.454.658.579.180.187.589.96 ZnSO30.17860.17900.17940.18030.18125 ZnSO429.132.035.036.638.241.343.042.141.039.938.837.66 ZnSeO433.0634.9837.3838.7940.345。
协同视域下高职水产类专业人才培养模式研究与实践
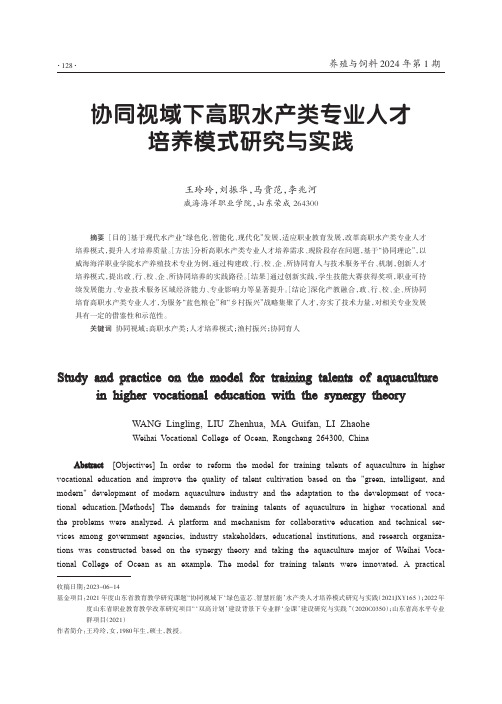
协同视域下高职水产类专业人才培养模式研究与实践王玲玲,刘振华,马贵范,李兆河威海海洋职业学院,山东荣成 264300摘要[目的]基于现代水产业“绿色化、智能化、现代化”发展,适应职业教育发展,改革高职水产类专业人才培养模式,提升人才培养质量。
[方法]分析高职水产类专业人才培养需求、现阶段存在问题,基于“协同理论”,以威海海洋职业学院水产养殖技术专业为例,通过构建政、行、校、企、所协同育人与技术服务平台、机制,创新人才培养模式,提出政、行、校、企、所协同培养的实践路径。
[结果]通过创新实践,学生技能大赛获得奖项,职业可持续发展能力、专业技术服务区域经济能力、专业影响力等显著提升。
[结论]深化产教融合,政、行、校、企、所协同培育高职水产类专业人才,为服务“蓝色粮仓”和“乡村振兴”战略集聚了人才,夯实了技术力量,对相关专业发展具有一定的借鉴性和示范性。
关键词协同视域;高职水产类;人才培养模式;渔村振兴;协同育人Study and practice on the model for training talents of aquaculture in higher vocational education with the synergy theoryWANG Lingling, LIU Zhenhua, MA Guifan, LI ZhaoheWeihai Vocational College of Ocean, Rongcheng 264300, ChinaAbstract[Objectives] In order to reform the model for training talents of aquaculture in higher vocational education and improve the quality of talent cultivation based on the "green, intelligent, and modern" development of modern aquaculture industry and the adaptation to the development of voca‐tional education.[Methods] The demands for training talents of aquaculture in higher vocational and the problems were analyzed. A platform and mechanism for collaborative education and technical ser‐vices among government agencies, industry stakeholders, educational institutions, and research organiza‐tions was constructed based on the synergy theory and taking the aquaculture major of Weihai Voca‐tional College of Ocean as an example. The model for training talents were innovated. A practical收稿日期:2023-06-14基金项目:2021 年度山东省教育教学研究课题“协同视域下‘绿色蓝芯、智慧匠能’水产类人才培养模式研究与实践(2021JXY165 );2022年度山东省职业教育教学改革研究项目“‘双高计划’建设背景下专业群‘金课’建设研究与实践”(2020C0350);山东省高水平专业群项目(2021)作者简介:王玲玲,女,1980年生,硕士,教授。
hypotheses
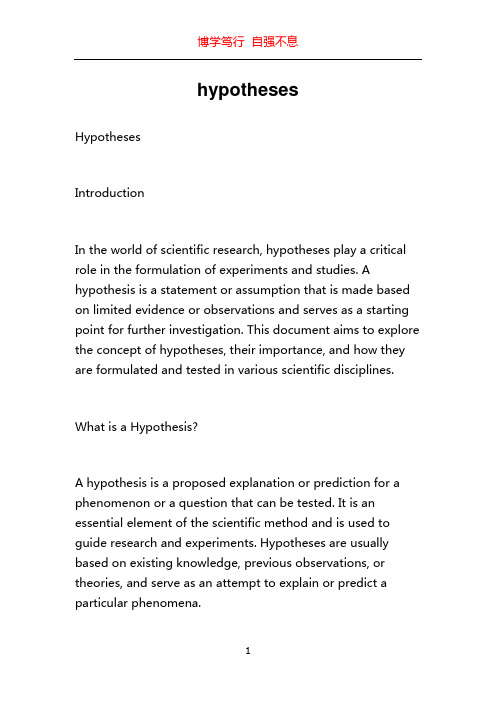
hypothesesHypothesesIntroductionIn the world of scientific research, hypotheses play a critical role in the formulation of experiments and studies. A hypothesis is a statement or assumption that is made based on limited evidence or observations and serves as a starting point for further investigation. This document aims to explore the concept of hypotheses, their importance, and how they are formulated and tested in various scientific disciplines.What is a Hypothesis?A hypothesis is a proposed explanation or prediction for a phenomenon or a question that can be tested. It is an essential element of the scientific method and is used to guide research and experiments. Hypotheses are usually based on existing knowledge, previous observations, or theories, and serve as an attempt to explain or predict a particular phenomena.Formulating a HypothesisThe process of formulating a hypothesis requires careful consideration of the existing knowledge and evidence. To develop a hypothesis, researchers typically follow a few key steps:1. Identify the research question: The first step in formulatinga hypothesis is to clearly identify the research question or problem that needs to be addressed. This question should be specific and focused to provide a clear direction for the research.2. Review existing knowledge: Once the research question is identified, it is important to review the existing knowledge and literature related to the topic. This helps in understanding previous findings and theories that can inform the formulation of a hypothesis.3. Generate possible explanations: Based on the existing knowledge, researchers generate possible explanations or predictions for the research question. These explanations are known as hypotheses and should be testable and falsifiable.4. Refine the hypothesis: After generating the initial hypotheses, researchers refine and narrow down the options to develop a more focused and specific hypothesis. This is done by considering factors such as feasibility, relevance, and available resources.Testing a HypothesisOnce a hypothesis is formulated, it needs to be tested through experimentation or observation. The process of testing a hypothesis involves the following steps:1. Design the experiment: The researcher designs an experiment or study that will allow them to collect data and test the hypothesis. The design of the experiment should be carefully planned to ensure that it provides valid and reliable results.2. Collect and analyze data: During the experiment, data is collected and analyzed to determine whether the results support or refute the hypothesis. Statistical analysis is often used to evaluate the significance of the findings and to draw meaningful conclusions.3. Draw conclusions: Based on the analysis of the data, the researcher draws conclusions about the hypothesis. If the results support the hypothesis, it is considered to be validated. On the other hand, if the results contradict the hypothesis, it may be necessary to revise the hypothesis or develop new ones for further investigation.Importance of Hypotheses in Scientific ResearchHypotheses are a fundamental aspect of scientific research for several reasons:1. Guiding research: Hypotheses provide a clear direction for research, allowing researchers to focus their efforts on specific questions or problems. They help in organizing the research process and ensuring that it is purposeful and systematic.2. Promoting objectivity: Hypotheses help in maintaining objectivity in scientific research by providing a framework for testing and evaluating ideas. They prevent bias and ensure that the research is based on evidence and logic rather than personal opinions or beliefs.3. Advancing knowledge: By formulating hypotheses and testing them through rigorous experimentation, researchers contribute to the advancement of knowledge in their respective fields. Hypotheses that are supported by evidence can lead to new discoveries and insights.4. Identifying limitations: Hypotheses allow researchers to identify and address the limitations of existing knowledge and theories. They highlight the gaps in understanding and provide opportunities for further investigation and refinement of theories.ConclusionHypotheses are a critical component of scientific research. They provide a starting point for investigation, guide research efforts, and contribute to the advancement of knowledge. By formulating and testing hypotheses, researchers can better understand the world around us and make meaningful contributions to their respective fields.。
- 1、下载文档前请自行甄别文档内容的完整性,平台不提供额外的编辑、内容补充、找答案等附加服务。
- 2、"仅部分预览"的文档,不可在线预览部分如存在完整性等问题,可反馈申请退款(可完整预览的文档不适用该条件!)。
- 3、如文档侵犯您的权益,请联系客服反馈,我们会尽快为您处理(人工客服工作时间:9:00-18:30)。
Ab Initio Study of Aqueous Hydrochloric AcidKari asonen†and Michael L.Klein*Center for Molecular Modeling and Department of Chemistry,Uni V ersity of Pennsyl V ania,Philadelphia,Pennsyl V ania19104-6323Recei V ed:August15,1996;In Final Form:No V ember1,1996XAb initio molecular dynamics simulations have been carried out for solutions of hydrochloric acid in water,under ambient conditions,over a wide range of concentrations.For acid-to-water mole ratios of1:31and1:7the hydrochloric acid molecules readily ionize to form hydrated chloride and hydronium ions.However,in a typical laboratory strength1:3.6solution,the textbook picture of separated solvated ions breaks downwith the formation of a hydrogen-bonded species,Cl-H‚‚‚Cl-.The presence of this species should beobservable in experiment and its detection and characterization could provide a stringent test of the currentab initio simulation methodology.1.IntroductionIn the last few years,the development of powerful ab initio simulation algorithms1and their implementation on parallel computers2has lead to important insights into the behavior of condensed phase systems.These ab initio simulation tech-niques,based on density functional theory,have already had great success when applied to the study of covalently bonded atomic solids and liquids3,4and molecular systems such as hydrogen-bonded ice and water.5-7The technique has been used to study surface chemical reactions8,9and,more recently, ultradense high-temperature solid hydrogen.10The great ad-vantage of an ab initio approach to condensed phase simulations is that it obviates the need for empirical interatomic potential functions.Herein,we report an application of the ab initio molecular dynamics methodology to a problem in“wet chem-istry”;namely,we explore the nature of hydrochloric acid,one of the most common reagents found in any chemistry laboratory. Anticipating our results,we will see that,in addition to hydrated chloride and hydronium ions,which are present in dilute solutions,reagent strength hydrochloric acid is found to contain the bifluoride-like associated ionic species,Cl-H‚‚‚Cl-.The predictions of our ab initio results on hydrochloric acid could be tested by a new generation of complementary spectroscopic and diffraction experiments.Acid dissociation is one of the simplest and most important solvation reactions.One of the guiding chemical principles, found in standard texts,is that a strong acid such as HCl(p K a )-7)readily dissociates in dilute aqueous solution to form hydrated chloride and hydronium ions,whereas,under the same conditions,a weak acid such as HF(p K a)3)barely ionizes.11,12 These expectations have been borne out by numerous ingenious experimental studies spanning more than a century.13,15How-ever,the behavior of the hydracids in concentrated aqueous solutions is somewhat less clear.For example,water has only a very limited solubility in concentrated HCl,but the solubility of HCl in water saturates at around40wt%.13Indeed,the HCl/water system is recognized as a classic nonideal liquid mixture.13Powerful physical techniques,such as the diffraction of X-rays and neutrons,and spectroscopic probes have con-firmed the presence of hydronium in hydrochloric acid solutions and have also established that significant structural changes occur in the solution with increasing acid concentration.14-16 To further understand the nature of aqueous solutions,we extend earlier work on water7to include the ab initio molecular dynamics simulation of aqueous hydracids.17The present study is designed to gain some insight into the possible microscopic mechanisms that might limit acid solubility in water.Our investigation complements topical and ongoing research into the ionization of HCl on the surface of ice crystals.18,19In contrast to the latter works,the present study employs the Car-Parrinello(CP)density functional theory(DFT)based molecular dynamics(MD)method.6,7An ab initio approach is helpful because the dissociation of a water molecule is difficult to model with empirical potentials.20An advantage of the CP-MD technique is that it is able to describe both the bond-breaking ionization process and the dynamics of the system in a self-consistent fashion,subject to the limitation of DFT and the adiabatic propagation of nuclear degrees of freedom.1The present article reports the salient results of CP-MD simulations carried out for solutions of DCl in heavy water(D2O)with acid-to-water mole ratios1:31,1:7,and1:3.6.These particular values were chosen to be close to those employed in previous experimental studies.14-16The1:3.6system is near the satura-tion limit for aqueous solution.132.Technical DetailsAt the start of the CP-MD calculations,the simulation systems consist of solute DCl and solvent D2O molecules.The computational details are very similar to those employed in earlier CP-MD calculations(see,for example,refs7and17). The valence electronic wave functions are developed in plane waves,and their interactions with nuclei and the core electrons are described using ultrasoft pseudopotentials.21The psudo-potentials were Vandebilt type,and the cutoffs were1.4au for oxygen,0.7au for hydrogen,and1.5au for Cl.The exchange-correlation was the LDA form parametrized by Perdew and Zunger,22and the gradient-corrected exchange term is taken from the work of Becke22with no gradient correction applied to the correlation terms.(The accuracy of this approximation is extensively discussed in ref7.)Even though the size of our system is relatively small(in this case32molecules),the inclusion of periodic boundary conditions and Ewald summation makes the CP-MD approach more relevant for the discussion*To whom correspondence should be addressed:Laboratory forResearch on the Structure of Matter,University of Pennsylvania,3231Walnut Street,Philadelphia,PA19104-6202.Phone:(215)898-8571.FAX:(215)898-8296.Email:klein@.†asonen@helsinki.fi.Present address:Department of Chemistry,University of Oulu,Oulu,Finland.X Abstract published in Ad V ance ACS Abstracts,December15,1996.98J.Phys.Chem.A1997,101,98-102S1089-5639(96)02513-3CCC:$14.00©1997American Chemical Societyof the behavior of concentrated solutions than,for example,a cluster calculation.For the Ewald summation in r -space,the Gaussians employed (exp(-[r /r c ]2)were very narrow with r c )1.1au.In k -space,the cutoff is the same as used for the density,namely,k c 2<96Ry.We have replaced all of the hydrogen atoms in the periodic MD cell with deuterium so thatFigure 1.Snapshots of the ab initio CP-MD simulations.(A)A dilute (mole ratio 1:31)solution of DCl in D 2O showing hydrated chloride ion (blue)with four D 2O ligands and hydronium D 3O +(O red and D gray).Hydrogen bonds are shown as green lines.(B)A concentrated (mole ratio 1:3.6)solution.The large and small blue,red,and gray spheres are Cl,O,and D atoms,respectively.The bichloride [Cl -D ‚‚‚Cl -]species are shown with enlarged Cl and D radii.Ab Initio Study of Aqueous Hydrochloric Acid J.Phys.Chem.A,Vol.101,No.1,199799the simulation actually refers to DCl in D 2O.This has the advantage that we can use a larger time step (0.145fs)for integrating the equations of motion.The CP-MD runs started from an equilibrated 32molecule liquid water configuration 17in which either one,four,or seven of the water molecules were replaced by DCl molecules and,simultaneously,the MD box was dilated to yield the desired density of the solution.13Although the DCl positions were chosen to maximize Cl -Cl distances,in the most concentrated solution there was actually one Cl -D ‚‚‚Cl -D near-neighbor pair (solute dimer)in the initial configuration.The starting Cl -D distances were set to the gas-phase value (1.27Å).At the begining of the CP-MD calculation the system temperature intially increases.It was then gradually cooled over a time period of about 1ps (i.e.7000time steps)to the desired value,which in the present case was 320K.The CP-MD results discussed herein were obtained from subsequent trajectories of 10ps for the most concentrated solution and 4ps each for the other two runs.3.Results and DiscussionIn all of the simulations the DCl molecules typically dissociated to form Cl -and hydronium,D 3O +,ions (Figure 1A).However,in the case of the most concentrated solution (Figure 1B),not all of the DCl molecules in the simulation system ionized during the CP-MD run.Indeed,two DCl molecules remained intact at the end of the 10ps run and formed hydrogen-bonded Cl -D ‚‚‚Cl -species,analogous to a hydrated bifluoride ion.11One of these complexes began as a Cl -D ‚‚‚Cl -D dimer in the initial configuration of the simulation and persisted after ionization of one of the DCl molecules.The other complex formed spontaneously from initially well separated DCl mol-ecules and stayed as such for the remainder of the run.The anion Cl -D ‚‚‚Cl -species is known;it has been studied in the gas phase 23and in matrix isolation,24and its structure has been predicted by ab initio methods,25but as far as we are aware,it has not been reported heretofore in solution.In the dilute solutions (Figure 1A),the chloride ion has a coordination number that fluctuates between 4and 5,where the coordination number is determined here by counting the number of “bonds”with a D ‚‚‚Cl distance shorter than 2.90Å.However,in the most concentrated solution,such a high coordination number is only possible if the hydrated Cl -ions share solvation sheath water molecules (Figure 1B).Another possibility 14is the formation of Cl --Cl -contact-ion pairs,with a common water solvation sheath.Although this latter sug-gestion would seem to be electrostatically unfavorable,such ion-pairing structures have been reported in classical MD simulations based on empirical potential functions 26and possibly seen in neutron diffraction studies of concentrated LiCl solu-tions.27However,in the present CP-MD simulations,no Cl --Cl -contact-ion pairs were observed.In the concentrated solution,Cl -ions participating in the bichloride-like Cl -D ‚‚‚Cl -complex can be regarded as being surrounded by a hydration sphere that includes an undissociated DCl molecule.This view is supported by a more detailed analysis of the solvent structure.The coordination number of the Cl -ion in the complex is ∼4,which is similar to the other Cl -ions in the system,whereas the Cl in the undissociated DCl molecule has a significantly lower coordination number,typi-cally between 1and 2;see Figure 1B and Figure 2.In principle,the proton in the hydrated bichloride species Cl -D ‚‚‚Cl -can migrate from one Cl to the other without causing dissociation of the bound complex,i.e.Cl -D ‚‚‚Cl -S Cl -‚‚‚DCl.We have observed such jumps and verified that they are indeed correlated with variations in the coordination numbers of the Cl atoms (see Figure 2).Analysis of the electronic density also confirms the ionicity change.Figure 2.Evolution with time of the Cl -D distance,d ,and Cl coordination number,N ,for the [Cl -D ‚‚‚Cl -]species.(A)The species exhibiting proton migration at t )2.6,4.6,and 5.8ps.(B)The species that shows fluctuations but does not show an actual proton jump.The curves are running averages,with 60fs averaging,of the actual CP-MD data.100J.Phys.Chem.A,Vol.101,No.1,1997Laasonen andKleinThe sensitivity of the Cl-D‚‚‚Cl-structure to the local environment likely also explains its apparent stability.If we link the dissociation of the DCl with the formation of a higher coordination state of Cl,the stability of the hydrated bichloride-like complex is enhanced by the fact that even though the DCl molecule ionizes,the structure of the complex is still preserved. Dissociation will likely happen only when both of the chlorine atoms in the complex become fully(four)coordinated.Un-fortunately,due to the short duration of the MD run,we cannot rule out the possibility that the bichloride species will e V entually dissociate.However,as evidence for the likely stability of this complex,we note that we did actually observe the spontaneous formation of one of the Cl-D‚‚‚Cl-complexes during the MD run.Thus,taken at face value,the simulation suggests that hydrated bichloride-like species should exist in a concentrated solution.29We have used atomic radial distribution functions(RDFs)to explore,more quantitatively,the structure of the solutions.27 The RDFs(see Figure3and Figure4)confirm that the saturated solution is really a multicomponent mixture of water(D2O), chloride(Cl-),hydrated hydronium(as in ref7,we observe both D3O+and D5O2+structures),and bichloride(Cl-D‚‚‚Cl-), with distinct coordination numbers for atoms associated with the different species.The calculated hydronium O(D3O+)-O(D2O)RDF has a sharp first peak at2.5Å,which is in excellent accord with the diffraction data(see the dashed line in Figure4A).The solvent O(D2O)-O(D2O)first peak occurs at∼2.7Å,as expected for the model.7In the most concentrated solution studied herein,the Cl-Cl RDF(Figure3)has a first peak at3.2Å(Figure3A),which is related to the bichloride species,plus a broad peak between 3.8and4.8Åarising from the Cl--Cl-interactions.Typically, Cl-ions form Cl--H3O+-Cl-clusters,with Cl-O distances of∼3Å(Figure3B)and a Cl-O-Cl angle of∼90°.This finding is in good qualitative agreement with the structural features present in the trihydrate crystal.30One conclusion to emerge from the RDF structural analysis of the CP-MD simulations is that no Cl--Cl-contact-ion pairs formed during the simulation.For aqueous LiCl and NiCl2the ion-ion RDFs have been measured.27The reported Cl-Cl RDFs suggest anion pairing in the former case but not the latter.Also,in a recent study of the more dilute system LiCl:6H2O,the Cl-ions were reported to be completely solvated and no evidence was presented concerning Cl--Cl-ion pairs.28The experimental values reported in refs27and28for the coordination number of the chlorine in LiCl were∼5.3and∼5.8,respectively,which compare to the average value of∼4.6calculated here from consideration of the Cl-D coordination and∼5.1from the Cl-O in the concentrated solution.At high concentration the experimental X-ray RDF(the dashed line in Figure4A)shows a small peak at2.52Å, identified as due to hydronium(O-O),and a peak at3.13Å, which has been attributed to Cl-O(see Figure3B).14Accord-ing to our simulations,the latter peak might well be interpreted as a combination of the first Cl--O hydration peak(recallFigure3.Radial distribution functions(RDFs)for the concentrated solution calculated from the last8ps of the simulation.(A)Cl-Cl, Cl--Cl,Cl(DCl)-Cl,and(B)Cl-O,Cl--O,Cl(DCl)-O.The total Cl-Cl RDF is drawn with a bold solid line,Cl--Cl,as a thin solid line,and Cl(DCl)-Cl,as a thin dashed line.The latter two have been scaled by a factor of1/3.Similarly,the total Cl-O RDF is drawn with a bold solid line,Cl--O,as a thin solid line,and Cl(DCl)-O,as a thin dashed line.The latter two RDFs have also been scaled by a factor of1/parison between the CP-MD X-ray(A)and neutron (B)RDFs,G n,x(R),and the measurements of ref14.Here,G n,x(R))∑cic j b i b j g ij(R)/∑c i c j b i b j,where,g ij(R)is the atom-atom RDF,c i the concentration of species i,and b i the relative value of the X-ray or neutron scattering length:b x,O)10.0,b x,Cl)18.0,b x,D)0.0and b n,O )0.58,b n,Cl)0.96,b n,D)0.667,respectively.Ab Initio Study of Aqueous Hydrochloric Acid J.Phys.Chem.A,Vol.101,No.1,1997101Figure3B)plus the Cl(DCl)-Cl bichloride peak.The neutron RDF data14show two new peaks at high concentration,one at 1.6Åand the other at2.1Å(the dashed line in Figure4B). The latter is likely related to Cl‚‚‚D interactions from chloride hydration,but if this is the only contribution,then its measured peak height should increase with acid concentration,which it does not.14The reported relative constancy of this peak height, as the acid concentration increases,could perhaps be explained by increasing amounts of undissociated HCl and a reduction in the Cl-ion coordination number(from,say,∼5to∼4).The neutron peak observed at1.6Å(Figure4B)is mostly likely related to hydronium,i.e.O(D3O+)‚‚‚D,interactions.14To make explicit comparison with X-ray and neutron data,we have constructed the appropriately weighted RDFs,which are shown in Figure4.It should be noted that the intramolecular H-H and O-H contributions have been subtracted from the experi-mental neutron data.The CP-MD technique can also probe the short time dynami-cal behavior of the acid solutions.Indeed,we have identified frequencies related to the different species in the solution.7For example,the DCl stretching frequency(recall that all of the H atoms have been replaced with D)is calculated to be∼1400 cm-1,which is near a broad feature in the infrared spectrum attributed to hydronium D3O+vibrations.15The low-frequency bichloride Cl-Cl breathing vibration falls in the water“lattice-mode”region,around150cm-1,where peaks are seen in the Raman spectrum.164.ConclusionThe present ab initio MD simulations confirm that for aqueous hydrochloric acid the conventional picture of solvated chloride and hydronium is obtained at mole ratios of acid-to-water of1:31and1:7.However,according to our calculation, there is likely to be undissociated,but complexed,HCl present in more concentrated solutions of aqueous HCl.Thus,in a bottle of reagent grade HCl some of the acid molecules will likely be undissociated;they are stabilized by participating in the first coordination shell of hydrated Cl-ions,thereby forming a hydrated bichloride-like species Cl-H‚‚‚Cl-.To date the available experimental data14-16have been interpreted without invoking the presence of the Cl-D‚‚‚Cl-species.The experi-mental situation is sufficiently unclear that a new generation of diffraction experiments,focused on aqueous hydracids,would seem to be worthwhile.Individual RDFs can now be measured using neutron diffraction with isotopic substitution.27If our prediction is valid,the signal arising from Cl-D‚‚‚Cl-should be quite clear in the diffraction data.New spectroscopic15,16 and NMR studies should also give valuable additional informa-tion on this species,which has already been studied in matrix isolation and in the gas phas.23,24Acknowledgment.We thank Michele Parrinello and Michiel Sprik for sharing with us details of their related studies on aqueous systems before publication and Kechuan Tu and Preston Moore for useful discussions.This research was supported by the National Science Foundation under Grant CHE96-23017 and benefited,in part,from facilities provided under DMR91-20668.Some of the calculations were conducted with allocation MCA93S020on the IBM SP2parallel machine at the Cornell Theory Center.References and Notes(1)Car,R.;Parrinello,M.Phys.Re V.Lett.1985,55,2471.(2)Clarke,L.C.;Stich,I.;Payne,mun.1992, 72,14.(3)Sugino,O.;Car,R.Phys.Re V.Lett.1995,74,1823.(4)Sarnthein,J.;Pasquarello,A.;Car,R.Phys.Re V.Lett.1995,74, 4682.(5)Lee,C.;Vanderbilt,D.;Laasonen,K.;Car,R.;Parrinello,M.Phys. Re V.Lett.1992,69,462;Phys.Re V.B1993,47,10142.(6)Laasonen,K.;Sprik,M.;Parrinello,M.;Car,R.J.Chem.Phys. 1993,99,9080.(7)Tuckerman,M.;Laasonen,K.;Sprik,M.;Parrinello,M.J.Phys. Chem.1995,99,5749.Sprik,M.;Hutter,J.;Parrinello,M.J.Chem.Phys. 1996,105,1142.(8)DeVita,A.;Stich,I.;Gillan,M.J.;Payne,M.C.;Clarke,L.C. Phys.Re V.Lett.1993,71,1276.(9)Langel,W.;Parrinello,M.J.Chem.Phys.1995,103,3240.(10)Alavi,A.;Parrinello,M.;Frenkel,D.Science1995,269,1252.(11)Gillespie,R.J.;Humphreys,D.A.;Baird,N.C.;Robinson,E.A. Chemistry;Allyn and Bacon:New York,1986;p480.(12)Oxtoby,D.W.;Nachtrieb,N.H.Modern Chemistry;Saunders: New York,1990;p205.(13)Rupert,F.F.J.Am.Chem.Soc.1909,31,851.See also:Rowlinson, J.S.;Swinton,F.L.Liquids and Liquid Mixtures;Butterworths:London, 1982;p210.(14)Triolo,R.;Narten,A.H.J.Chem.Phys.1975,63,3624.(15)Giguere,P.A.;Turrell,S.J.Am.Chem.Soc.1980,102,5473.(16)Walrafen,G.E.;Chu,Y.C.;Carlon,H.R.In Proton Transfer in Hydrogen-Bonded Systems;Bountis,T.,Ed.;Plenum Press:New York, 1992;pp297-311.(17)Laasonen,K.;Klein,M.L.J.Am.Chem.Soc.1994,116,11640; Mol.Phys.1996,88,135.(18)Robinson,S.H.;Clary,D.C.J.Chem.Soc.,Faraday Trans.1995, 100,309.(19)Gertner,B.J.;Hynes,J.T.Science1996,271,1563.Clary,D.C. Ibid.1996,271,1509.Ando,K.;Hynes,J.T.J.Mol.Liq.1995,64,25.(20)Stillinger,F.H.;David,C.W.J.Chem.Phys.1978,69,1473.(21)Vanderbilt,D.Phys.Re V.B1990,41,7892.(22)Perdew,J.;Zunger,A.Phys.Re V.B1981,23,5048.Becke,A.D. J.Chem.Phys.1992,96,2155.(23)Metz,R.B.;Kitsoploulos,T.;Weaver,A.;Newmark,D.M.J. Chem.Phys.1988,88,1463.(24)Wight,C.A.;Ault,B.S.;Andrews,L.J.Chem.Phys.1976,65, 1244.(25)Almlof,J.J.Mol.Struct.1981,85,179.(26)Smith,D.E.;Dang,L.X.J.Chem.Phys.1994,100,3757.(27)Neilson,G.W.;Broadbent,R.D.;Howell,I.;Tromp,R.H.J.Chem. Soc.,Faraday Trans.1993,89,2927.Tromp,R.H.;Neilson,G.W.;Soper,A.K.J.Chem.Phys.1992,96,8460.(28)Yamagami,M.;Wakita,H.;Yamaguchi,T.J.Chem.Phys.1995, 103,8174.(29)In the gas phase,Cl-binds HCl more strongly than H2O:Larson, J.W.;McMahon,T.B.J.Am.Chem.Soc.1984,106,517.(30)Lundgren,J.-O.;Olovsson,I.Acta.Crystallogr.1967,23,971. Yoon,Y.K.;Carpenter,G.B.Ibid.1959,12,17.102J.Phys.Chem.A,Vol.101,No.1,1997Laasonen and Klein。