Absorbing Boundary Conditions for Hyperbolic Systems
Fin–tube junction effects on flow and heat transfer in flat tube multilouvered heat exchangers

Fin–tube junction effects on flow and heat transfer in flat tubemultilouvered heat exchangersD.K.Taftia,*,J.CuibaMechanical Engineering Department,Virginia Tech,105Randolph Hall,Blacksburg,VA 24061,USAbMechanical Engineering Department,Center for Electric Power,Tennessee Technological University,Cookeville,TN 38505,USAReceived 2July 2002;received in revised form 17October 2002AbstractThree-dimensional simulations of four louver–tube junction geometries are performed to investigate the effect on louver and tube friction and heat transfer characteristics.Three Reynolds numbers,300,600and 1100,based on bulk velocity and louver pitch are calculated.Strong three-dimensionality exists in the flow structure in the region where the angled louver transitions to a flat landing adjoining the tube surface,whereas the flow on the angled louver far from the tube surface is nominally two-dimensional.Due to the small spatial extent of the transition region,its overall impact on louver heat transfer is limited,but the strong unsteady flow acceleration on the top louver surface augments the heat transfer coefficient on the tube surface by over 100%.In spite of the augmentation,the presence of the tube lowers the overall Nusselt number of the heat exchanger between 25%and 30%.Comparisons with correlations derived from experiments on full heat exchanger cores show that computational modeling of a small subsystem can be used reliably to extract performance data for the full heat exchanger.Ó2003Elsevier Science Ltd.All rights reserved.1.IntroductionFlat tube corrugated multilouvered fins are used in many compact heat exchanger applications to enhance the air-side heat transfer performance.Louvers reduce the average thermal boundary-layer thickness by inter-rupting its growth and by enhancing mixing through large-scale instabilities,hence increasing the average heat transfer coefficient.Previous experimental and numerical studies have established that the heat transfer in multi-louvered fins is influenced by three factors:(a)flow di-rection [1,2];(b)thermal wake interference [3];(c)flow instabilities and transport of coherent vorticity in the vicinity of the louver surface [4].These three mechanisms have mostly been studied with a louver-centric view,i.e,heat transfer enhancement on a nominally two-dimen-sional louver,with the assumption that louvers contri-bute a significant portion to the overall heat transfersurface.For the most part this assumption is well justi-fied.However,in exchangers with large fin pitches and small fin heights or tube pitch,the tube surface can contribute substantially to the total heat transfer.For example for a fin pitch of 1.5–2.0times the louver pitch,and a tube pitch of 5louver pitches,the tube surface area contributes between 20%and 30%of the total heat transfer area.This,coupled with the fact that the tube is the primary heat transfer surface with the largest po-tential for heat transfer,requires that attention be paid to the heat transfer from the tube surface.Our specific geometry of interest is a flat tube multi-louvered exchanger with corrugated rectangular chan-nels.In order to gain some insight into what influences tube heat transfer,in this study we focus our attention on the region of the louver near the junction with the tube surface.In this region,along the height of the fin,the louver transitions from an angle h to 0°into a flat landing adjoining the tube surface as shown in Fig.1(d).1Cui*Corresponding author.Tel.:+1-540-231-9975;fax:+1-540-231-9100.E-mail address:dtafti@ (D.K.Tafti).1The corrugated fin curvature near the tube wall is neglected.0017-9310/03/$-see front matter Ó2003Elsevier Science Ltd.All rights reserved.doi:10.1016/S0017-9310(02)00509-4International Journal of Heat and Mass Transfer 46(2003)2027–2038/locate/ijhmtand Tafti[5]numerically investigated the geometry in Fig.1(d)at a Reynolds number of1100,based on louver pitch and bulk velocity.They found that although the flow on the angled portion of the louver was nominally two-dimensional with self-sustainedflow oscillations characterized by spanwise vortices,theflow was stronglyputational domain for four louver geometries:(a)periodic louver;(b)straight louver;(c)transition without landing;(d)transition with landing.Shaded areas are the louver and tube surface.2028 D.K.Tafti,J.Cui/International Journal of Heat and Mass Transfer46(2003)2027–2038three-dimensional and unsteady in the transition region. An energetic unsteady vortex jet formed at the leading edge,which was drawn under the louver.The jet was complemented by a region of strong unsteadyflow ac-celeration in the vicinity of the top louver surface.Evi-dence was presented that the temporal evolution of the two was correlated,which had a significant impact on local heat transfer coefficients.In spite of the high heat transfer in this region,the overall effect on mean louver heat transfer was found to be small because of the small spatial extent of the transition region.However,it was found that the strong acceleration near the junction with theflat landing had a significant effect on tube heat transfer.Our research objective is to study the three-dimen-sionalflow and temperaturefields generated in compact heat exchangers and to determine whether these largely unknown characteristics can be used to further augment heat transfer by slight modifications to the base geom-etries.The objective of this paper is to extend the pre-vious three-dimensional unsteady simulations to study three Reynolds numbers,1100,600and300.In addition to the Reynolds number effect,simulations are carried out on four variations of the transitional louver geo-metry to study the incremental effect of geometry at the junction with the tube.The heat transfer and friction results are presented separately for the louver and tube,and combined to estimate the overall eff-parisons are also made with existing louver-and-tube correlations in the literature to determine whether com-putational modeling of a subsystem can be used reliably to predict full heat exchanger core performance.The paper is organized as follows:the numerical and computational method is presented briefly in the next section,followed by the description of the louver ge-ometries.In the section on results,the generalflow features,louver and tube friction and heat transfer characteristics are discussed.Finally comparisons are made with experimental correlations.This is followed by concluding remarks.2.Numerical formulationWe solve the non-dimensional,time-dependent,in-compressible Navier–Stokes and energy equations in conservative form in generalized curvilinear coordinates. The governing equations for momentum and energy are discretized with a conservativefinite volume formulation using a second-order central difference scheme on a non-staggered mesh.The Cartesian velocities,pressure,and temperature are calculated and stored at the cell center, whereas contravariantfluxes are stored and calculated at the cell faces.A projection method[6]is used for the time integration of the discretized continuity and mo-mentum equations.The louveredfin geometry is approximated by aninfinite array of louvers in both streamwise and cross-stream directions,which results in a simpler system with periodic repetition of the basic unit.Periodic boundary conditions for velocity,modified pressure and tempera-ture are applied in the streamwise and cross-stream directions since theflow is assumed to be both hydro-dynamically and thermally fully developed without any entrance or exit effects.No-slip,no-penetration bound-ary conditions for velocity and constant heatflux con-ditions are enforced on the louver and tube surface.More details of the numerical algorithm,treatment of the boundary conditions,verification and validation of the computer program and strategies for parallel com-puting can be found in Tafti et al.[7–9]and Cui and Tafti[5].3.Description of four louver geometriesFour louver geometries are considered in this paper (see Fig.1):(1)periodic louver;the louver is assumed periodic in the spanwise direction with no tube.This simulation isolates any intrinsic three-dimensional ef-fects brought about by secondary three-dimensional in-stabilities[10];(2)straight louver;the angled louver extends all the way to the tube;this serves as a baseline case to study the effect of louver geometry transition;(3) louver with transition without landing;the angled louver directly transitions to the tube surface;(4)louver with transition andflat landing,which has been studied in detail by Cui and Tafti[5]at Re¼parison of (3)and(4),highlights the role of theflat landing.For all four geometries,the unit computational do-main has a dimension of1(normalized by louver pitch LÃp)in streamwiseðxÞ-direction,fin pitch1(in this par-ticular case,fin pitch FÃpis same as LÃp)in cross-stream ðyÞ-direction,and2.5in spanwiseðzÞ-direction along the fin height.Along the spanwise direction in geometry4 (hereafter referred as transition with landing),the louver can be divided into three parts:angled louver(length, 1.75),transition part(length,0.5),andflat landing (length,0.25).A linear transition profile is prescribed between the angled louver and theflat landing with a small radii of curvature at the junction with the louver [11].For geometry3(hereafter referred to as transition without landing),the angled louver part is extended to a length of2.0,and the transition part is unchanged,but theflat landing between the transition and the tube surface is removed.Geometry2,referred to as a straight louver,has a spanwise extent of2.5.Finally,geometry1 is referred to as a periodic louver and has a spanwise extent of2.5.In all cases,the thickness of the angled louver is0.1 times the louver pitch with25°louver angle.For the last three geometries,symmetry boundary conditions areD.K.Tafti,J.Cui/International Journal of Heat and Mass Transfer46(2003)2027–20382029imposed at a distance of2.5from the tube surface along thefin height,assuming that theflow is sufficiently re-moved from the extrinsic three-dimensional effects of the tube wall region and is nominally two-dimensional.This also assumes implicitly that thefin height is5.0louver pitches.For the periodic louver,periodic boundary conditions are implemented in the spanwise direction since theflow is homogeneous along this direction.The computational domain surrounding each louver is resolved by98Â98Â128computational cells in the x-,y-and z-directions,respectively for the transitioning geometries.For the periodic and straight louver,96 computational cells are used in the z-direction along the fin height.A veryfine,nearly orthogonal mesh,is used in the vicinity of the louver and tube surface,and in the transition region[5].A posteriori extraction of the mean wall shear stress for Re¼1100shows that thefirst grid point near the louver surface falls between0.1and0.3in local wall units based on the local shear stress.In the region with the largest shear stress(in the transitionregion),there arefive grid points within10wall units normal to the surface,with thefirst at0.3.Along the streamwise direction,the grid is nearly uniform with spacing of5–7wall units.Along thefin height or spanwise direction,the mesh is coarsest in the two-dimensional region of the geometry with the maximum spacing of60wall units andfinest at the beginning and end of transition,and near the tube wall with spacing around3wall units.Spectral analyses show that the spatial and temporal resolution isfine enough to capture all the relevant scales in these calculations[5].4.ResultsIn each of the calculations,a mean non-dimensional pressure gradient of unity is imposed in the streamwise direction to drive theflow.As the calculation proceeds, theflow rate,in response to the frictional and pressure drag losses in the calculation domain,adjusts to the mean pressure gradient and reaches a stationary(or steady state,in the case of low Reynolds number steady flow).Time signals offlow variables are recorded and a stationaryflow is assumed when a near constant mean value or a quasi-periodicfluctuation in time is observed. Fig.2shows the temporal evolution of the spatially averaged Nusselt number for four louver geometries at a nominal Reynolds number of1100.It is clear that all flows have adjusted to the mean pressure gradient and reached a statistically stationary state.Similar plots at nominal bulk Reynolds number of600and300also show that theflow has reached a stationary or steady state.To characterize the heat transfer,we define a local instantaneous Nusselt number over the louver/tube surface based on the louver pitch as Nu¼LÃpq00Ã=ðTÃsÀTÃrefÞjIn terms of non-dimensional quantities the above can be re-written asNu¼1s refwhere h s2is the modified non-dimensional surface temperature and h ref is the reference modified non-dimensional bulk temperature,which is defined as:h ref¼R Rj u j h d A xR Rj u j d A xThe surface-averaged Nusselt number is obtained by integration over the louver or tube surface as:Nu¼R RXd XR RXðh sÀh refÞd Xwhere X denotes the louver or tube surface.The Colburn j-factor as a measure of heat transfer is calculated as: j¼NuRePr0:4The Fanning friction coefficient is calculated as:f¼D pÃ12q VÃcDÃh4Fd¼D h21V2cwhere DÃhis the hydraulic diameter,D pÃ=FÃdis the pre-scribed pressure gradient across the calculation domain (unity non-dimensional value in present calculations),and VÃcis the calculated maximum mean velocity.2Tðx;y;z;tÞ¼Tinþc xþhðx;y;z;tÞ,where c is the mean temperature gradient.2030 D.K.Tafti,J.Cui/International Journal of Heat and Mass Transfer46(2003)2027–20384.1.Generalflow featuresIn the study of the louver with transition andflat landing[5],it is shown thatflow on the angled louver portion is characterized by periodic spanwise vortex shedding at the Reynolds number of1100.The spanwise vortices are nominally two-dimensional in nature with weak three-dimensionality across thefin height.The time signal at a location above the top louver surface exhibits a nearly periodic pattern,and the frequency spectrum shows a clear peak at1.8(non-dimensionalized by bulk velocity and louver pitch),which corresponds to the frequency of the spanwise vortex shedding.At this Reynolds number of1100,all four louver geometries exhibit the same vortex shedding characteristic fre-quency.Although there is considerable geometry varia-tion near the tube surface,its effects on theflowfield on the louver away from the tube is minimal.Because of these similarities at the angled louver part,nearly iden-ticalflow and heat transfer behavior is expected for the four louver geometries.Any observable differences would come from the area near the tube surface.At Reynolds number of600,theflow unsteadiness becomes much weaker at the angled louver part.The time signals do not show a periodic pattern,and vortex shedding only occurs in an occasional manner,and there is no clear characteristic frequency.At Reynolds number of300,theflow is completely steady and remains at-tached on the louver surface and there is no evidence of vortex shedding for all louver geometries.These results are in agreement with a previous two-dimensional in-vestigation on the onset of instabilities for developing flow in a louver bank[4].To facilitate our understanding of the unsteady na-ture of theflow and the associated vorticity dynamics, the r u[12]vortex identification technique is used.This frame-invariant method identifies vortical structures as regions of large vorticity,where rotation dominates over strain to cause the rate-of-deformation tensor r u(ve-locity gradient tensor)to have complex eigenvalues (one real and two conjugate complex eigenvalues).The complex eigenvalues imply that the local streamline pattern is closed or spiral,thus correctly eliminating near-wall shear layers.This methodology can also be separately applied in the x-,y-,or z-planes in order to identify streamwise,cross-flow,and spanwise vortices [10],respectively.The strength of the vortex is measured in terms of the imaginary part of the eigenvalue of the velocity gradient tensor and is denoted by k i.The strength of its three subsets,streamwise,cross-flow,and spanwise vortices is measured in terms of the imaginary part of the eigenvalue of the velocity gradient on the x-, y-,and z-planes,respectively,and is denoted by k i;x,k i;y, and k i;z,respectively.Fig.3(a)–(d)shows the volume-averaged vortical strength k i;x;y;z distribution along thefin height at an arbitrary instant at Reynolds number of1100.3Only the volumes with non-zero eigenvalues are included in the volume averaging.For the periodic case(Fig. 3(a)),the lines for streamwise(k i;x)and cross-flow(k i;y) vorticity are identically zero throughout the louver height.The only contribution to the total vorticity is from the spanwise vorticity(k i;z).Hence at Re¼1100, for the given louver geometry,theflow is strictly two-dimensional and intrinsic three-dimensional secondary instabilities have not developed.4For the straight lou-ver(Fig.3(b)),the spanwise vorticity dominates.How-ever,there are small components of both streamwise (k i;x)and cross-stream(k i;y)vorticity present along the louver height.This implies that the three-dimensionality introduced by the presence of the tube wall permeates into theflow away from the wall and introduces weak three-dimensionality in a nominally two-dimensional flow.The spanwise vorticity(k i;z)is damped consider-ably by the viscous presence of the wall which is felt up to one louver pitch away from it,implying very thick boundary layers on the tube wall.Approaching the tube surface,there is a noticeable but slight increase for both streamwise(k i;x)and cross-flow(k i;y)vorticity as the spanwise and total vorticity decrease.For the louver with transition andflat landing(Fig. 3(d)),on the angled louver,k i essentially maintains a constant value,with a dominant contribution from spanwise vorticity.However,in the transition region the flow is strongly three-dimensional.k i increases,with increasing contributions from streamwise and cross-stream vorticity,with a drop in contributions from spanwise vorticity.k i reaches a maximum in the center of the transition region and then decreases as the louver approaches theflat landing and the tube surface.The increase in the streamwise and cross-stream components of vorticity is related to the formation of an unsteady vortex jet under the bottom louver surface,which is described in detail in Cui and Tafti[5].Not reflected in these plots,but related to the vortex jet,is the formation of a highly unsteady region of acceleratedflow velocities on the top surface of the louver.For transition without landing(Fig.3(c)),it is seen that the magnitude of co-herent vorticity in the transition region is reduced.This is because,in the presence of theflat landing thefluid acceleration on the top surface and the vortex jet feed offthe streamwiseflow along theflat landing.In the ab-sence of theflat landing,when the louver transitions directly to the tube surface,there is reduced access to 3To obtain the distribution,the volume averaging is performed in domains defined by decompositions used for parallel computation along thefin height.4The nominally2-Dflow was perturbed by3-D disturbances to seed any intrinsic three-dimensional secondary instabilities, but the perturbations were not self-sustaining.D.K.Tafti,J.Cui/International Journal of Heat and Mass Transfer46(2003)2027–20382031fluid mass,which results in the weakening of theseflow structures.4.2.Pressure and friction drag on louver and tubeFig.4(a)–(d)plots the fractional variation of mean form and friction drag per unit length along thefin height or spanwise direction at a nominal Re¼1100.5 For all four geometries,at the angled louver portion,the form drag dominates the friction drag and is almost unchanged throughout the angled louver.This is best exemplified by the two-dimensionalflow over the peri-odic louver in Fig.4(a),in which the form drag con-tributes80%to the overall pressure loss.For transition with landing(Fig.4(d)),and transition without landing (Fig.4(c)),the magnitude of pressure and friction drag is similar at the angled louver part.For the straight louver,although the form drag loss is four times the friction losses away from the tube surface,which is similar to other geometries,the contribution to total losses is dominated by the presence of the tube.Both frictional and form losses increase substantially in the vicinity of the tube surface because of viscous effects.As theflow approaches the tube,it slows down,and theflow angle reduces substantially,which leads to the increased con-tribution to form drag.For the transitioning geometries in Fig.4(c)and(d),the trends are completely different. In the transition region,the form drag increases slightly and eventually vanishes at theflat landing.On the other hand,friction drag increases sharply in the transition region and reaches its largest value near theflat landing due to the accelerated high velocity boundary layer in that region before decreasing again on theflat landing. Similar,albeit weaker,distributions at the transition region are found for the transitioning geometry without the landing(Fig.4(c)).Fig.5(a)–(c)plots the mean drag force distribution as a function of thefin height for the transition with landing geometry at three Reynolds numbers:1100,600, and300.As the Reynolds number decreases,the con-tribution of pressure drag decreases while that of friction5The form and friction drag are plotted as a fraction of thetotal losses.Since the mean pressure gradient isfixed at unity,the integrated area under the curves should add up toapproximately(barring tube frictional losses)2.5,the pressureloss expressed as a force on the computational domain.2032 D.K.Tafti,J.Cui/International Journal of Heat and Mass Transfer46(2003)2027–2038drag increases at the angled louver part.At a nominal Reynolds number of300,the two drag forces are nearly equal.The distribution at the transition region andflat landing follow the same trend as the Reynolds number decreases.Overall the changes in Reynolds number do not change the salient features of the drag distribution throughout the louver.This is also true for the other three louver geometries.Fig.6plots the fractional contribution of friction losses on the tube surface to the total losses.For all three geometries,the contribution of the tube to overall losses is less than8%of the total.The louvers with transition exhibit a higher contribution because of the increased shear stress on the tube surface as a result of the un-steady accelerating boundary layer in the vicinity.4.3.Time-averaged heat transfer coefficientFig.7(a)–(d)plots the time mean thermalfield (modified temperature,h)on the top surface of the louver.Because the heatflux isfixed on the louver and tube surface,a high surface temperature implies low heat transfer.In all cases,at a nominal Re¼1100,the shear layer at the leading edge of the louver separates and sheds vortices.Very near the leading edge,the heat transfer coefficients are high,but decrease in the recir-culation zone which forms downstream of the leading edge.In the reattachment region,at half the louver length,the vorticity generated by the separated shear layer increases the heat transfer coefficient by increasing mixing.For the periodic geometry,in the absence of any extrinsic three-dimensionality,the surface temperature does not show any variations in the z-direction.For the straight louver,the thick thermal boundary layer on the tube surface dominates the temperature distribution on the top surface.For transition with landing,in the transition region,the low temperature/high heat transfer region on the top surface near theflat landing is a result of the unsteady accelerating boundary layer on the louver surface.Similar trends are observed for the transitioning louver with no landing.Temperature contours on the lower surface are shown in Fig.8(a)–(d).For the periodic louver the heat transfer coefficient is a maximum at the leading edge and decreases thereafter till near the trailing edge where it increases again.A high temperature/low heat transfer region formed in the transition region in Fig.8(c)and (d)results from the presence of the vortex jet.The jet is detached from the louver surface and a stagnant recir-culating region is formed underneath the jet.Similar to the top surface,a thick boundary layer near the tube surface exists for the straight louver on the bottomD.K.Tafti,J.Cui/International Journal of Heat and Mass Transfer46(2003)2027–20382033paring temperature contours on the top and bottom louver surfaces for both the transitional geometries in the vicinity of the tube clearly shows the positive effect of the accelerating boundary layer on the top surface.Temperature contours have lower values in the immediate vicinity of the tube on the top louver surface than on the bottom surface.In Fig.9(a)and(b),the average(time and spatial) Nusselt number on the louver,and tube surface is plotted separately.In general,thefirst-order effect of the angled louver transitioning to0°and aflat landing is to reduce the heat transfer coefficient.Also,the presence of the tube surface further reduces the heat transfer coef-ficient on the louver surface because of the presence of thick thermal boundary layers at the junction between fin and tube.These effects can either be countered or reinforced further by other non-linear effects as observed (unsteady boundary layer acceleration on louver top surface and vortex jet on bottom surface,separation)in the current study.The unsteady boundary layer accel-eration on the top surface has a positive impact on louver heat transfer,whereas the formation of the vortex jet at the bottom has a neutral to negative impact.The results in Fig.9(a)are consistent with these observa-tions.The periodic louver exhibits the highest heat transfer coefficient,whereas the straight louver and the transitioning louver with aflat landing exhibit heat transfer coefficients which are between15%and25% lower.The transitioning louver without a landing lies between the two extremes and is between6%and15% lower.These results indicate that to maintain a high heat2034 D.K.Tafti,J.Cui/International Journal of Heat and Mass Transfer46(2003)2027–2038transfer coefficient on a transitioning louver,theflat landing should be as small as physically possible.On the other hand,the enhancement provided by the transitioning louver with aflat landing on the tube sur-face is quite strong.The tube Nusselt number is lowest for the straight louver because there is nothing that can break the thick thermal boundary layer that forms at the fin–tube junction.With the transitional louver,the un-steady boundary layer acceleration on the top surface and to some extent the vortex jet under the louver,help to perturb and thin the thermal boundary layer on the tube and increase the heat transfer coefficient.Without theflat landing,the unsteady nature of theflow is con-siderably weakened as noted in Fig.3,and the augmen-tation on the tube surface is not as high.Transition with flat landing provides an augmentation of over a100% over a straight louver,whereas with no landing,the augmentation is reduced to between30%and40%.4.4.Overall friction and heat transfer coefficient forflat tube louvered heat exchangerIn this section,the overall heat transfer and friction factors for an equivalent duct of aspect ratio5,bounded by louveredfins and the tube surface are presented. These are compared to theoreticalflow results for fully developed laminarflow in ducts.Fig.10(a)compares the calculated friction coefficient(f),and Fig.10(b)plots the equivalent Nusselt number(Nu Dh)versus Re Dh.The friction coefficient increases by a factor between 4and9when compared to a fully developed laminar flow in a duct of aspect ratio5.On the other hand the Nusselt number is augmented by factors varying from2 to3.5.The tube surface results in approximately a25–30%reduction in the overall Nusselt number.Hence,for small tube pitches and largefin pitches,tube surface heat transfer becomes critical to the performance of the heat exchanger.In fact,in spite of the louver heat transfer being highest for the geometry without a landing,the overall Nusselt number is highest for the geometry with aflat landing because of a larger heat transfer coefficient on the tube surface.Between the three geometries,the louver with transition andflat landing exhibits the lowest friction coefficient,whereas the friction coefficient is highest for the straight louver.The result goes against ReynoldÕs analogy,but is consistent with the fact that losses are dominated by louver form drag,which is re-duced substantially in the transition region andvanishes。
Boundary Conditions

If Bn ≠ 0, B n must also be specified. t
Aerospace & Energetics Research Program
Plasma Dynamics Group
Boundary Conditions for Insulators
Consider the case of an insulating, permeable wall. The integrated induction equation becomes
Expressing the MHD equations in compact form,
Q·F t
Sp
Integrating over the domain,
tdVQdSn· FdVSp
For ideal MHD, RHS vanishes.
Consistent boundary values must be specified for the normal fluxes in the surface integral.
Aerospace & Energetics Research Program
Plasma Dynamics Group
Boundary Conditions for Ideal MHD
For the energy equation, the boundary conditions give
n ? e p B 22 v B ? v B e p B 22 v n B t? v tB n
Aerospace & Energetics Research Program
Plasma Dynamics Group
多孔跳跃边界条件
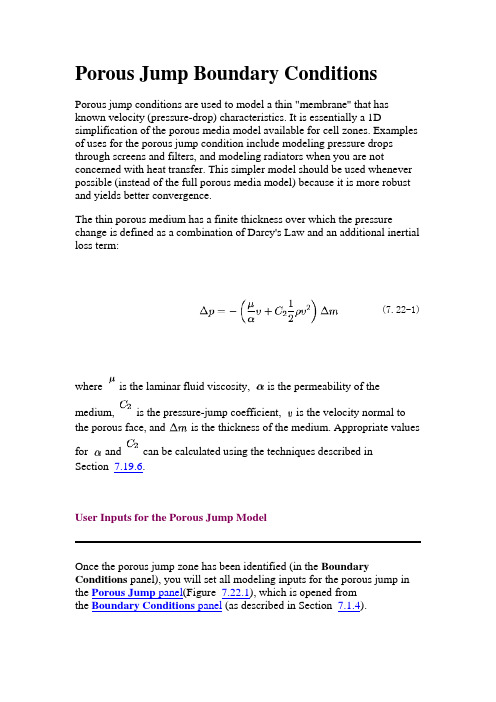
Porous Jump Boundary Conditions Porous jump conditions are used to model a thin "membrane'' that has known velocity (pressure-drop) characteristics. It is essentially a 1D simplification of the porous media model available for cell zones. Examples of uses for the porous jump condition include modeling pressure drops through screens and filters, and modeling radiators when you are not concerned with heat transfer. This simpler model should be used whenever possible (instead of the full porous media model) because it is more robust and yields better convergence.The thin porous medium has a finite thickness over which the pressure change is defined as a combination of Darcy's Law and an additional inertial loss term:(7.22-1)where is the laminar fluid viscosity, is the permeability of the medium, is the pressure-jump coefficient, is the velocity normal to the porous face, and is the thickness of the medium. Appropriate values for and can be calculated using the techniques described inSection 7.19.6.User Inputs for the Porous Jump ModelFigure 7.22.1:The Porous Jump PanelThe inputs required for the porous jump model are as follows:1. Identify the porous-jump zone.2. Set the Face Permeability of the medium ( in Equation 7.22-1).3. Set the Porous Medium Thickness ( ).4. Set the Pressure-Jump Coefficient ( ).5. Define the discrete phase boundary condition for the porous jump (for discrete phase calculations).Identifying the Porous Jump ZoneSince the porous jump model is a 1D simplification of the porous media model, the porous-jump zone must be modeled as the interface between cells, rather than a cell zone. Thus the porous-jump zone is a type of internal face zone (where the faces are line segments in 2D ortriangles/quadrilaterals in 3D). If the porous-jump zone is not identified as such by default when you read in the grid (i.e., if it is identified as another type of internal face zone), you can use the Boundary Conditions panel to change the appropriate face zone to a porous-jump zone.Define Boundary Conditions...The procedure for changing a zone's type is described in Section 7.1.3. Once the zone has been changed to a porous jump, you can open the Porous Jump panel (as described in Section 7.1.4) and specify the porous jump parameters listed above.Defining Discrete Phase Boundary Conditions for the Porous JumpIf you are modeling a discrete phase of particles, you can set the fate of particle trajectories at the porous jump. See Section 22.13 for details.Postprocessing for the Porous JumpPostprocessing suggestions for a problem that includes a porous jump are the same as for porous media problems. See Section 7.19.9.。
机械工程学专业词汇 A E
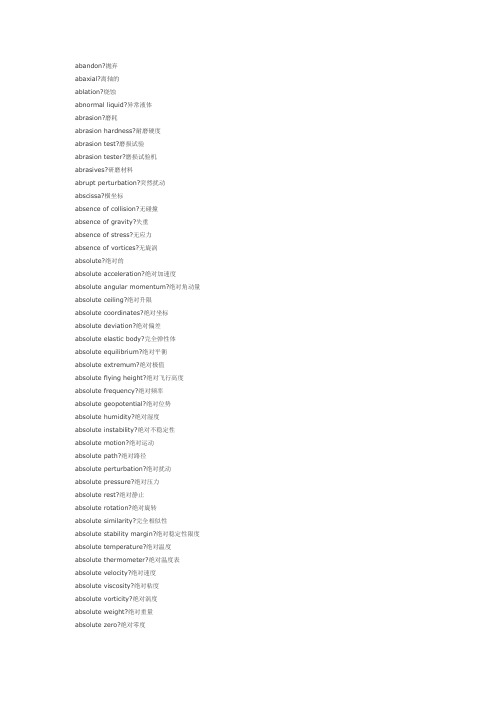
abandon?抛弃abaxial?离轴的ablation?烧蚀abnormal liquid?异常液体abrasion?磨耗abrasion hardness?耐磨硬度abrasion test?磨损试验abrasion tester?磨损试验机abrasives?研磨材料abrupt perturbation?突然扰动abscissa?横坐标absence of collision?无碰撞absence of gravity?失重absence of stress?无应力absence of vortices?无旋涡absolute?绝对的absolute acceleration?绝对加速度absolute angular momentum?绝对角动量absolute ceiling?绝对升限absolute coordinates?绝对坐标absolute deviation?绝对偏差absolute elastic body?完全弹性体absolute equilibrium?绝对平衡absolute extremum?绝对极值absolute flying height?绝对飞行高度absolute frequency?绝对频率absolute geopotential?绝对位势absolute humidity?绝对湿度absolute instability?绝对不稳定性absolute motion?绝对运动absolute path?绝对路径absolute perturbation?绝对扰动absolute pressure?绝对压力absolute rest?绝对静止absolute rotation?绝对旋转absolute similarity?完全相似性absolute stability margin?绝对稳定性限度absolute temperature?绝对温度absolute thermometer?绝对温度表absolute velocity?绝对速度absolute viscosity?绝对粘度absolute vorticity?绝对涡度absolute weight?绝对重量absolute zero?绝对零度absolute zero point?绝对零度absorbability?吸收性absorbing agent?吸收剂absorbing medium?吸收媒质absorbing resistance?吸收阻抗absorptiometer?吸收率计absorption?吸收;缓冲absorption curve?吸收曲线absorption dynamometer?吸收功率计absorption heat?吸收热absorption loss?吸收损失absorption model?吸收模型absorption potential?吸收势absorption tube?吸收管absorption wave meter?吸收式波长计absorptive?吸收的absorptive power?吸收本领absorptivity?吸收率accelerated creep?加速蠕变accelerated motion?加速运动accelerating ability?加速能力accelerating field?加速场accelerating gradient?加速梯度accelerating impact?加速撞击accelerating period?加速周期accelerating tube?加速管accelerating unit?加速装置acceleration?加速度acceleration diagram?加速度图acceleration energy?加速度能量acceleration field?加速度场acceleration meter?加速度计acceleration nozzle?加速喷嘴acceleration of creep?蠕变加速度acceleration of gravity?重力加速度acceleration of moving space?牵连加速度acceleration potential?加速势acceleration pressure?加速压acceleration time graph?加速度时间图acceleration vector?加速度矢量acceleration wave?加速波acceleration work?加速功accelerationless?无加速的accelerograph?加速度记录仪accelerometer?加速度计accessibility?可达性accessible point?可达点accidental?偶然的accidental coincidence?偶然符合accidental error?随机误差accidental resonance?偶然共振accommodation?适应accommodation coefficient?适应系数accumulated error?累积误差accumulator?蓄压器储压器accuracy?准确度accuracy of measurement?测量准确度accuracy of readings?读数准确度accurate adjustment?精确蝶accurate measurement?精确测量accurate model?准确模型accurate similarity?准确相似accurate simulation?准确模拟acicular structure?针状组织acoustic?声的acoustic absorption?声吸收acoustic absorptionsound absorption?声吸收acoustic absorptivity?声吸收率acoustic admittance?声导纳acoustic analysis?声学分析acoustic baffle?隔音板acoustic conductivity?声导率acoustic dispersion?声弥散acoustic disturbance?声音干扰acoustic efficiency?声效率acoustic energy?声能acoustic field?声场acoustic filter?滤声器acoustic frequency?音频acoustic impedance?声阻抗acoustic load?声负载acoustic material?隔声材料acoustic mechanical efficiency?声机械效率acoustic power?声功率acoustic pressure?声压acoustic radiation pressure?声辐射压acoustic reactance?声抗acoustic resistance?声阻acoustic resonance?共鸣acoustic velocity?声速acoustical?声的acoustical wave?声波acting force?织力action at distance?超距酌action center?活动中心action integral?酌积分action of deflagration?爆燃酌action of force?力的酌action principle?酌原理action sphere?酌区action turbine?冲唤涡轮action variable?酌变量action wave?酌波activation?活化active earth pressure?织土压active flight?织飞行active force?织力active gas?活性气体active load reaction?有功负载反酌active pressure?织压力activity of force?力功率actual load?实际载荷actual stress?实际应力actual value?实际值actuator?执行器acyclic?非循环的adaptability?适应性adapter?转接器adaptometer?适应计addition?附加additional drag?附加阻力additional load?附加载荷additional mass?附加质量additional pressure?附加压力adhere?粘着adherence?附着adhesion?附着adhesion coefficient?粘着系数adhesive force?粘附力adhesive tension?粘附张力adiabat?绝热线adiabatic?绝热的adiabatic approximation?绝热近似adiabatic atmosphere?绝热大气adiabatic calorimeter?绝热式量热器adiabatic change?绝热变化adiabatic change of state?态的绝热变化adiabatic compression?绝热压缩adiabatic cooling?绝热冷却adiabatic curve?绝热线adiabatic efficiency?绝热效率adiabatic ellipse?绝热椭圆adiabatic equilibrium?绝热平衡adiabatic expansion?绝热膨胀adiabatic factor?绝热因子adiabatic heating?绝热加热adiabatic hypothesis?绝热假设adiabatic invariant?绝热不变量adiabatic invariant of eddy?涡旋的绝热不变量adiabatic invariant of vortex?涡旋的绝热不变量adiabatic perturbation?绝热扰动adiabatic principle?绝热原理adiabatic process?绝热过程adiabatic pulsations?绝热脉动adjoint operator?伴算子adjustable guide vane?可导叶adjusting device?蝶装置adjusting spring?蝶弹簧adjusting valve?蝶阀adjusting wedge?蝶楔块adjustment?蝶adjustment mark?照准标志admissible concentration?容许浓度admissible crror?容许误差admissible load?容许荷载admissible pressure?容许压力admissible stress?容许应力admissible value?容许值admission?进气admission pipe?进气管admission pressure?进气压力admixture?混合物adsorb?吸附adsorbent?吸附剂adsorption?吸附adsorption force?吸附力adsorption layer?吸附层advancing rate?超前速率advancing wave?前进波advection?平流advection fog?平另advective?平聊aeration?充气aerator?通风机aerial?空气的aerial ropeway?架空死aeroballistics?航空弹道学aerodonetics?滑翔学aerodromics?滑翔力学aerodromometry?空气速度测量法aerodynamic action?空气动力酌aerodynamic analogy?空气动力比拟aerodynamic angle of attack?气动迎角aerodynamic angle of incidence?气动迎角aerodynamic balance?风洞天平aerodynamic center?气动中心aerodynamic coefficient?气动力系数aerodynamic derivative?空气动力导数aerodynamic drag?气动阻力aerodynamic force?气动力aerodynamic heating?气动力加热aerodynamic lift?气动升力aerodynamic resistance?气动阻力aerodynamic stability?空气动力稳定性aerodynamical?空气动力学的aerodynamical moment?气动力矩aerodynamics?空气动力学aerodyne?重航空器aeroelasticity?气动弹性aeroelastics?气动弹性力学aeroengine?飞机发动机aerohydrodynamics?空气铃动力学aerology?高空气象学aeromechanics?航空力学aerometer?气体比重计aeromotor?飞机发动机aeronautical?航空的aeronautics?航空学aeroplane?飞机aeropulse engine?脉动式喷气发动机aerosol?气溶胶aerospace engineering?航空航天技术aerostat?轻航空器aerostatics?气体静力学aerothermodynamics?气动热力学aerothermoelasticity?空气热弹性学;气动热弹性aerotonometry?气体张力测量法affine transformation?仿射变换affinity?亲合力after effect?后效after effect function?后效函数afterbody?后部afterburner?加力燃烧室aftershock wave?余震波age hardening?时效硬化ageing?应变时效ageing test?老化试验agglomeration?凝聚aileron?副翼air amount?空气量air brake?空气制动器air bubble?气泡air buffer?空气缓冲器air burst?空中爆炸air cap?空气冠air circulation?空气环流air column?空气柱air compressor?空气压缩机air condenser?空气电容器air conveying?气龄送air coolant?空气冷却剂air cooling?空气冷却air coordinates?空间坐标air current?气流air cushion?气垫air cylinder?压气缸air damper?空气阻尼器air damping?空气阻尼air density?空气密度air drag?空气阻力air duct?风道air eddy?空气涡旋air flow?气流空气量air flow meter?气疗air friction?空气摩擦air gap?空隙air gas?风煤气air hammer?空气锤air hardening?空气淬火air hoist?风动起重滑车air humidity?空气湿度air inflating?充气air injection diesel engine?空气喷射柴油发动机air inrush?空气侵入air intake valve?进气阀air jet?空气喷射air pipe?气管air plasma?空气等离子体air pressure gage?空气压力计air pressure head?空气压头air pressure reducer?空气减压器air proof?气密的air pump?空气泵air register?空气活门air relief cock?放气塞air reservoir?储气筒air resistance?空气阻力air shock wave?空气激波air speed?空气速率air speed indicator?空气速率指示器air streamline?空气吝air tank?空气箱air temperature?气温air tight?气密的air to air missile?空对空导弹air trajectory?空气轨迹air wave?空气波air wedge?空气楔aircraft dynamics?飞机动力学airfoil?机翼airfoil profile?翼型airfoil section?翼剖面airfoil theory?机翼理论airless injection?无气喷射airline?飞行航线airlock?气锁airmechanics?空气力学airplane?飞机airscrew?空气螺旋桨airship?飞艇airway?航路airy stress function?爱里应力函数algebraic stress model?代数应力模型aligned composite material?定向复合材料aligned structure?定向结构allocation?分配allotropic change?同素异形变化allotropic transformation?同素异形变化allowable clearance?容许间隙allowable deflection?容许挠度allowable deviation?容许偏差allowable error?容许误差allowable load?容许荷载allowable pressure?容许压力allowable stress?容许应力allowable temperature?容许温度allowed cross section?容许截面allowed energy band?容许能带allowedness?容许度alternate immersion test?反复浸没试验alternating bending?交变弯曲alternating bending stress?交变弯曲应力alternating bending test?交变弯曲试验alternating direction?交替方向alternating hysteresis?交变滞后alternating impact bending test?交变冲讳曲试验alternating impact test?交变冲辉验alternating load?交替载荷alternating load deformation?交变负载变形alternating pressure?交变压力alternating stress?交替应力alternation?交变alternation of load?负载交变altimeter?高度表altitude?高度altitude circle?等高圈altitude difference?高度差altitude front?高空锋面ambient air?周围空气ambient medium?周围介质ambient pressure?周围压力ambient temperature?周围温度ambipolar diffusion?两极性扩散amendment?修正amoeboid movement?阿米巴式运动amorphous state?无定形状态amount of energy?能量amount of evaporation?蒸发量amplification?放大amplification limit frequency?放大极限频率amplifier?放大器amplitude?振幅amplitude clipping?脉冲幅度限幅amplitude cosine?幅角余弦amplitude discriminator?振幅甄别器amplitude distortion?波幅畸变amplitude frequency diagram?幅频图amplitude function?振幅函数amplitude locus?幅值轨迹amplitude modulation?爹amplitude resonance?振幅共振amplitude response?振幅响应amplitude selector?振幅选择器amplitude spectrum?振幅谱amplitude swing?幅度变动anabatic front?上升锋面anabatic wind?上坡风anafront?上滑锋anallobar?气压上升区analog?模拟analog digital computer?模拟数字计算机analog method?模拟法analogue?模拟analogue computer?模拟计算机analogue method?相似法analogy?模拟analogy of magnetic field?磁场相似analysis?分析学analysis of the oscillation?振荡分析analytic?解析的analytic transformation?解析变换analytical mechanics?分析力学anchoring?系泊anelastic material?滞弹性材料anemogram?风力自记曲线anemograph?风速计anemometer?风速表anemoscope?风向仪angle modulation?相角灯angle of advance?超前角angle of arrival?到达角angle of attack?迎角angle of bank?倾斜角angle of capillarity?毛细角angle of contact?接触角angle of contingence?切线角angle of crossing?交叉角angle of declination?偏斜角angle of departure?起飞角angle of distortion?畸变角angle of downwash?下洗角angle of elevation?仰角angle of emergence?出角angle of flap deflection?襟翼偏转角angle of flow?燎angle of friction?摩擦角angle of impact?碰撞角angle of inclination?倾角angle of internal friction?内摩擦角angle of repose?静止角angle of rotation?转动角angle of sideslip?侧滑角angle of slope?倾斜角angle of twist?扭转角angle of yaw?偏航角angle pipe?弯管angle preserving mapping?保角映射angle straggling?角离散angle variable?角变量angular acceleration?角加速度angular coefficient?角系数angular coordinates?角坐标angular correlation?角相关angular derivation loss?角偏向损失angular derivative?角微商angular dispersion?角分散angular displacement?角位移angular distance?角距angular distribution?角分布angular division?角分度angular frequency?角频率angular impulse?角冲量angular momentum?角动量angular momentum conservation law?角动量守恒定律angular momentum operator?角动量算符angular momentum tensor?角动量张量angular momentum theorem?角动量定理angular motion?角运动angular separation?方向夹角angular speed?角速度angular unit?角单位angular variable?角变量angular velocity?角速度anharmonic?非低的anharmonic oscillation?非谐振动anharmonic oscillator?非谐振子anharmonic ratio?非低比anisentropic flow?非等熵怜anisothermal porous flow?非等温渗透流anisotropic?蛤异性的anisotropic body?蛤异性体anisotropic liquid?蛤异性液体anisotropic material?蛤异性材料anisotropic rock?蛤异性岩石anisotropic turbulence?蛤异性湍流anisotropy?蛤异性anisotropy constant?蛤异性常数anisotropy ratio?蛤异性比annular flow?环流annular focus?环形焦点annular mist flow?环形雾状流anomalous absorption?异常吸收anomalous diffusion?反常扩散anomalous scattering?反常散射anti diffusion?反扩散anticlockwise rotation?反时针方向旋转anticoincidence?反重合anticoincidence method?反符合法anticyclolysis?反气旋消散anticyclonic vorticity?反气旋涡度antinode?波腹antipodal space?对映空间antiresonance?反共振antiresonance frequency?反共振频率antisymmetric tensor?反对称张量antisymmetrical state?反对称态antisymmetry?反对称antitriptic wind?减速风aperiodic?非周期的aperiodic damping?非周期衰减aperiodic motion?非周期运动aperiodicity?非周期性aperture?孔径aperture ratio?孔径比aphelion?远日点apocenter?远心点apogee?远地点apolar?无极的apolune?远月点apparent absorption coefficient?表观吸收系数apparent cohesion?表观粘力apparent diffusivity?表观扩散率apparent elastic limit?表观弹性极限apparent energy?表观能量apparent equilibrium?表观平衡apparent expansion?表观膨胀apparent force?表观力apparent modulus of elasticity?表观弹性模量apparent phase angle?表观相角apparent velocity?表观速度apparent viscosity?表观粘度apparent work?表观功appearance of fatigue?疲劳现象appell equation?阿佩尔方程application point?酌点applied elasticity theory?应用弹性学applied mechanics?应用力学approach velocity?驶近速度approximate analysis?近似解析approximate model?近似模型approximate similarity?近似相似approximate simulation?近似模拟approximate solution?近似解approximation?近似approximation calculus?近似计算approximation of lagrange function?拉格朗日函数近似approximation theory?近似理论approximative value?近似值arbitrary unit?任意单位arch?拱arch bridge?拱桥arch dam?拱坝arch gravity dam?拱形重力坝arch structure?拱形结构arch truss?拱形桁架arched beam?拱形梁arched girder?拱形梁archimedes principle?阿基米德原理area curve?面积曲线area load?表面负载area moment?面积矩area of contact?接触面积area ratio?面积比areal acceleration?面加速度areal coordinates?面积坐标areal velocity?面积速度areometer?比重计arm?边arm of couple?力偶臂arrest?制动器arrhenius equation?阿雷尼厄斯方程arrhenius law?阿雷尼厄斯定律artesian head?自廉头artesian pressure?自廉压artesian water?自廉artesian well?自廉artificial compression?人工压缩artificial earth's satellite?人造地球卫星artificial earthquake?人工地震artificial gravity?人造重力artificial perturbation?人工扰动artificial satellite?人造卫星artificial ventilation?人工通风ascending air current?向上气流ascending motion?上升运动ascending stroke?上行冲程ascension?升起aseismic?耐震的ash ejector?吹灰器排灰器aspect ratio?展弦比assembly?装配associate cumulation?结合累积associated mass?附加质量associated wave?缔合波astromechanics?天体力学astronavigation?天文导航astronomical azimuth?天文方位角astronomical unit?天文单位asymmetric?非对称的asymmetrical distribution?非对称性分布asymmetrical load?非对称负载asymmetry?非对称性asymptotic model?渐近模型athodyd?冲压喷气发动机atmosphere?大气atmospheric boundary layer?大气边界层atmospheric flight?大气飞行atmospheric humidity?大气湿度atmospheric layer?大气层atmospheric moisture?大气湿度atmospheric perturbation?大气扰动atmospheric pressure?大气压atmospheric pressure chart?气压图atmospheric subsidence?大气下沉atmospheric temperature?大气温度atmospheric turbulence?大气湍流atmospheric wave?大气波atom collision factor?原子碰撞因子atomic blast?原子爆炸atomic crack?原子龟裂atomic distance?原子间距atomic energy?原子能atomic energy level?原子能级atomic hardening?原子硬化atomization?喷雾化atomization pressure?雾化压力attachment?附着attenuation?衰减attenuation vibration?衰减振动attenuator?衰减器attitude?姿态attraction?吸引attraction potential?引力势attractive center?引力中心attractive force?引力audibility?可听性augmentation?增加augmenter?加速室auto spectrum?自乘谱autocontrol?自动控制autocorrelation?自相关autoexcitation?自激autogeneous ignition?自点火autogiro?自动陀螺仪automatic control system?自动控制系统automatic control theory?自动控制理论automatic design?计算机辅助设计automatic frequency control?自动频率控制automatic programming?自动编程序automatic regulator?自动第器autonomous system?自治系统autonomous vibration?自激振荡autopilot?自动导航装置autorotation?自转available energy?有效能available head?有效水头available heat?有效热average?平均;平均的average acceleration?平均加速度average curvature?平均曲率average deviation?平均加速度average diameter?平均直径average error?平均误差average gradient?平均坡度average life?平均寿命average load?平均负载average potential?平均势average power?平均功率average pressure?平均压力average quantity?平均量average speed?平均速度average temperature?平均温度average value?平均值average velocity?平均速度averaged orbital element?平均轨迹元averaging?取平均值averaging method?平均方法avertence?偏斜axes of coordinates?座标轴axial application of force?轴向施加力axial compression?轴向压缩axial elongation?轴向伸长axial flow?轴流轴向气流axial flow pump?轴撩axial force?轴向力axial impact?轴向碰撞axial load?轴向荷载axial magnification?轴向放大率axial mode?轴向模axial moment?轴向力矩axial moment of inertia?轴惯性矩axial pitch?轴向节距axial pressure?轴向压力axial pressure load?轴向压力负载axial stress?轴向应力axial symmetry?轴对称axial tension?轴向拉力axial thrust?轴向推力axial turbine?轴两涡轮axial vector?轴矢量axially symmetric flow?轴对称流轴对称怜axiom of constraint?约束公理axiomatics?公理系统axipetal?向心的axis of abscissas?横坐标轴axis of center of bending?弯曲中心轴axis of curvature?曲率轴axis of ordinates?纵坐标轴axis of revolution?回转轴axis of rotation?转动轴axis of symmetry?对称轴axis of traction?牵引轴axisymmetric?轴对称的axisymmetric element?轴对称单元axisymmetric flow?轴对称流axisymmetric problem?轴对称问题axle load?轴负载azimuth angle?方位角back flow?回流逆流back mixing?反混back pressure?背压力back reaction?逆反应back substitution?回代backpressure?反向压力;反压力backscattering technique?逆散射法backstreaming?逆流backward difference?后向差分backward motion?逆向运动backward precession?逆旋进backward thrust?逆推力backward wave?逆向行波backwash?后涡流backwater?回水backwater curve?回水曲线backwater distance?回水距离backwater function?回水函数backwater surface?回水面backwater surge?回水浪baer law?巴尔定律baeyer strain theory?贝耶尔应变理论balance?平衡balance condition?平衡条件balance equation?平衡方程balance force?平衡力balance weight?平衡锤balanced load?平衡负载balanced system of force?平衡力系balancing?平衡balancing force?补偿力balancing in situ?本机平衡balancing machine?平衡试验机balancing method?平衡法balancing speed?平衡速率ball support?球面支座ballast?压载物ballistic?弹道的ballistic coefficient?弹道系数ballistic constant?弹道常数ballistic curve?弹道曲线ballistic deflection?弹道偏差ballistic error?冲惑差ballistic pendulum?冲悔ballistic rocket?弹道火箭ballistic trajectory?弹道轨迹ballistic wave?弹道波ballistics?弹道学balloon?气球balloon borne rocket?气球发射火箭banded structure?带状结构bandwidth?带宽bank?侧向倾斜bar?巴bar construction?棒构造baric topography?气压形势baric wave?气压波barocline?斜压baroclinic fluid?斜压铃baroclinity?斜压性barodynamics?重结构力学barograph?气压计barometer?气压计barometric altitude?气压高度barometric column?气压柱barometric gradient?气压梯度barometric height?气压高度barosphere?气压层barotropic equation?正压方程barotropic equilibrium?正压平衡barotropic flow?正压流barotropic fluid?正压铃barotropic instability?正压不稳定barotropy?正压性barrel vault?圆柱壳barrier?障碍barycenter?重心barycentric?重心的barycentric coordinates?重心坐标barycentric system?重心系barycentric velocity?重心速度base flow?底流base pressure?底面压力basic equation?基本方程basic load?基本载荷basin?硫basset force?巴塞特力bathyal region?半深海区bauschinger effect?包辛格效应beach?海岸beam?梁beam axle?梁式轴beam balance?杠杆秤beam column?梁杆beam structure?梁结构beam supported of both ends?简支梁beam truss?桁架梁beam with varying section?变截面梁bearing?方位;轴承bearing capacity?承载力bearing friction?支承摩擦bearing friction loss?轴承摩擦损失bearing line?方位线bearing load?轴承荷载bearing power?承重能力bearing pressure?支承压力bearing strain?承载应变bearing strength?承载强度bearing stress?承载应力bearing surface?支承面beat?差拍beat frequency?拍频beat period?拍频周期bed load?推移质beginning of curve?曲线起点behavior?行为bell pressure gage?钟型压力计bell type manometer?钟型压力计bellows?波纹管belt tension?皮带张力bend test?弯曲试验bending?弯曲bending center?弯曲中心bending fatigue limit?弯曲疲劳极限bending line?弯曲线bending load?弯曲载荷bending moment?弯矩bending moment density?弯矩密度bending moment diagram?弯矩图bending radius?弯曲半径bending rigidity?抗弯刚度bending strain?弯曲应变bending strength?抗弯强度bending stress?弯曲应力bending test?弯曲试验bending vibration?弯曲振动bending wave?弯曲波beneding stress?挠应力bernoulli constant?伯努利常数bernoulli equation?伯努利方程bessel function?贝塞耳函数beta ratio?比压biaxial stress?双轴向应力bifurcated shock?分岔激波bifurcation?分支bifurcation point?分支点biharmonic function?双谐函数bilateral?双向的bilateral constraint?双边约束billow?大浪bimoment?双力矩bimotor?双发动机飞机binary collision?双碰撞binary diffusion coefficient?二元扩散系数binder?结合剂binding agent?结合剂binding energy?结合能binding force?结合力bingham body?宾汉物体bingham flow?宾汉怜bingham fluid?宾汉铃bingham model?宾汉模型binodal seiche?双节点驻波振荡biological similarity?生物学相似性biomechanics?生物力学biomechanics of bone?骨力学biomechanics of sports?运动生物力学biorheology?生物龄学biot savart law?毕奥萨伐尔定律biplane?双翼飞机bipolar coordinates?双极坐标birefringence?双折射bivector?双矢black body?绝对黑体;黑体black body radiation?黑体辐射blade?叶片blade efficiency?叶片效率blade exit angle?叶片出口角blade grid?叶栅blade inlet angle?叶片入口角blade loss?叶片损失blade outlet angle?叶片出口角blade pitch?叶片距blading?叶片装置blast?送风blast nozzle?喷气嘴blast of wind?阵风blast pressure?风压blast tuyere?风口blast volume?风量blasting?爆破blasting chamber?起爆室blasting efficiency?爆破效率blimp?软式飞艇blocking phenomenon?堵塞现象blood flow?血流blood visco elasticity?血液粘弹性blood viscosity?血液粘度blower?吹风器blown flap?吹气襟翼bluff body?钝体blunt body?钝头体body centroide?本体极迹body fixed system?物体固定坐标系body force?体力body of revolution?旋转体boger fluid?保格铃boiling?沸腾boiling point?沸点boiling temperature?沸点温度boltzmann constant?玻耳兹曼常数boltzmann distribution?玻耳兹曼分布boltzmann factor?玻耳兹曼因子boltzmann h theorem?玻耳兹曼h 定理boltzmann law?玻耳兹曼定律boltzmann transport equation?玻耳兹曼输运方程bolus flow?团流bond energy?结合能bond strength?附着强度bonding force?耦合力boost?升压booster?加速器bootstrap dynamics?靴袢动力学border?边缘borderline?边线bore?内径borehole?钻孔born green equation?玻陡窳址匠眺born von kormon boundary condition?玻斗肟呓缣跫bottom chord?底弦bottom current?底流bottom standing wave?底层驻波bottom velocity?底层速度bouguer wave number?布格波数bounce?回跳bound energy?束缚能bound state?束缚态bound vector?束缚矢量bound vortex?约束涡boundary?边界boundary collocation?边界配置boundary condition?边界条件boundary effect?边界效应boundary element method?边界元法boundary friction?边界摩擦boundary layer?边界层boundary layer control?边界层控制boundary layer equation?边界层方程boundary layer flow?边界层怜boundary layer method?边界层法boundary layer region?边界层区域boundary layer separation?边界层分离boundary layer thickness?边界层厚度boundary layer transition?边界层转捩boundary method?边界解法boundary of the air mass?气团的边界boundary part?边界部分boundary point?边界点boundary surface?边界面boundary value?边界值boundary value problem?边界值问题boundary wave?界面波bourdon gage?布尔登压力计boussinesq approximation?布辛涅斯克近似boussinesq equation of motion?布辛涅斯克运动方程bow?船首bow shock wave?头波bow wave?头波brachistochrone?最速降线bracket?悬臂梁brake?制动器brake horsepower?制动马力brake parachute?制动伞brake power?制动功率brake pressure?闸压力brake test?闸试验braking?制动braking distance?制动距离braking force?制动功率braking moment?制动力矩braking rocket?制动火箭break through?穿透breakaway?分离breakdown test?断裂试验breaker?破浪breaking angle?断裂角breaking elongation?断裂伸长breaking load?断裂负截breaking of wave?海浪断裂breaking point?断点breaking strain?断裂应变breaking strength?裂断强度breaking stress?破坏应力breaking test?致断试验breaking waves?碎波breaking weight?断裂负截bridge truss?桥桁架brinell hardness?布氏硬度brinell hardness test?布里涅耳硬度试验brittle?脆的brittle behavior?脆性行为brittle coating?脆性涂层brittle creep?脆性蠕变brittle fracture?脆裂brittle material?脆性材料brittle strength?抗脆裂强度brittleness?脆性broken line?折线brownian motion?布朗运动brunt vaisala frequency?布伦特韦伊塞拉频率bubble?气泡bubble center?气泡中心bubble cloud?气泡云bubble density?气泡密度bubble domain?泡畴bubble flow?泡状流bubble formation?气泡形成bubble of turbulence?湍联bubble pressure?泡压bubbling?气泡形成bubbling fluidized bed?鼓泡怜床bucket?叶片buckingham potential?伯金汉姆势buckling?屈曲buckling behavior?翘曲行为buckling coefficient?屈曲系数buckling load?屈曲负载buckling of plate?板的翘曲buckling of shell?壳的皱损buckling strength?抗屈曲强度buckling stress?屈曲应力buckling test?纵弯试验buffer?缓冲器减震器buffer action?缓冲酌buffer beam?缓冲梁buffer solution?缓冲溶液buffer spring?缓冲弹簧buffeting?扰炼震built in arch?固定拱bulge?隆起bulk acceleration?牵连加速度bulk forces?体积力bulk modulus?体积弹性横量bulk modulus of elasticity?体积弹性横量bulk motion?牵连运动bulk potential?体积势bulk resonance?体积共振bulk scattering?体积散射bulk strain?体积应变bulk temperature?总体温度bulk velocity?牵连速度bulk viscosity?体积粘度buoyancy?浮力buoyant frequency?浮力频率burgers material?伯格斯材料burning?燃烧burning load?燃烧负荷burning point?燃点burning temperature?燃点burning velocity?燃烧速度bursting?爆裂bursting pressure?爆炸压力bursting strength?破裂强度bursting stress?破裂应力bursting test?爆破试验busemann relation?布泽曼关系式cable stayed bridge?斜拉桥cad?计算机辅助设计calculator?计算机calculus of approximation?近似计算calculus of finite differences?差分演算calibration?校准calibrator?校准器calorific capacity?热容量calorimetric measurement?量热测量camber changing flap?改变机翼弯度的襟翼canal?管道canal vortex?沟渠涡旋canal wave?沟渠波canard?鸭翼canonical coordinate?正则坐标canonical distribution?正则分布canonical equation of motion?正则运动方程canonical equations?正则方程canonical form?正则形式canonical momentum?正则动量canonical transformation?正则变换canonical variable?正则变量cantilever?悬臂梁caoutchouc elasticity?橡胶弹性capacitive transducer?电容传感器capacity?功率capacity measure?容积量度capacity strain gage?电容应变计capillarity?毛细现象capillary absorption?毛细管吸收capillary action?毛细酌capillary attraction?毛细引力capillary condensation?毛细凝缩capillary constant?毛细常数capillary energy?表面张力能capillary fissure?毛细裂纹capillary flow?毛管流capillary force?毛细力capillary gravity wave?毛细重力波capillary level oscillation?毛细面振动capillary phenomenon?毛细现象capillary pressure?毛细压力capillary rise?毛细升高capillary tension?毛细张力capillary tube?毛细管capillary viscosimeter?毛细管粘度计capillary waves?毛细波capture?俘获carbon fiber?碳纤维cardan angle?卡登角cardan rings?卡登环cardiac dynamics?心脏动力学cardiac work?心脏的工作cargo?货物carrier gas?气体载体carrier inertial force?牵连惯性力carrier liquid?载体液体carrier oscillation?载波振荡carrier rocket?运载火箭carrier velocity?牵连速度carrying capacity?负荷量cartesian coordinates?笛卡儿坐标cartesian vector?笛卡儿矢量cascade excitation?级联激发cascade flow?翼栅怜cascade of aerofoil?翼型叶栅cascade tunnel?叶栅风洞case depth?渗碳层深度case hardening?表面硬化casing?外壳castigliano theorem?卡斯蒂利亚诺定理casting stress?铸造应力catapult?弹射器catenary?悬链线catenoid?悬链曲面cauchy deformation tensor?柯挝变张量cauchy equation of motion?柯嗡动方程cauchy integral theorem?柯锡分定理cauchy law of similarity?柯梧似性定律cauchy residue theorem?柯涡数定理cauchy riemann equations?柯卫杪匠眺cauchy stress tensor?柯桅力张量caudad acceleration?尾向加速度causality?因果律caving?空泡形成cavitating flow?气穴流涡空流cavitation?气蚀现象cavitation bubble?空泡cavitation damage?空化损坏cavitation effect?空化效应cavitation erosion?空蚀cavitation nucleus?空化核cavitation number?空化数cavitation parameter?空化参数cavitation phenomenon?气蚀现象cavitation shock?气蚀冲击cavitation tunnel?空泡试验筒cavity?空腔cavity collapse?空泡破裂cavity drag?空泡阻力cavity flow?气穴流涡空流cavity flow theory?空泡另论cavity formation?空泡形成cavity pressure?空腔压力cavity resonator?空腔共振器cavity vibration?共振腔振动ceiling?上升限度celestial mechanics?天体力学cell model?笼子模型cell reynolds number?网格雷诺数cellular grid?网状栅格cellular structure?栅格结构center?中心center line?中心线center line average height?中线平均高度center line of the bar?杆件轴线center of area?面积中心center of buoyancy?浮心center of curvature?曲率中心center of gravity?重心center of inertia?惯性中心center of inertia system?惯性中心坐标系。
分析化学专业英语词汇总结

专业英语词汇-----分析化学第一章绪论分析化学:analytical chemistry定性分析:qualitative analysis定量分析:quantitative analysis物理分析:physical analysis物理化学分析:physico-chemical analysis仪器分析法:instrumental analysis流动注射分析法:flow injection analysis;FIA顺序注射分析法:sequentical injection analysis;SIA化学计量学:chemometrics第二章误差的分析数据处理绝对误差:absolute error相对误差:relative error系统误差:systematic error可定误差:determinate error随机误差:accidental error不可定误差:indeterminate error准确度:accuracy精确度:precision偏差:debiation,d平均偏差:average debiation相对平均偏差:relative average debiation标准偏差(标准差):standerd deviation;S相对平均偏差:relatibe standard deviation;RSD变异系数:coefficient of variation误差传递:propagation of error有效数字:significant figure置信水平:confidence level显著性水平:level of significance合并标准偏差(组合标准差):pooled standard debiation 舍弃商:rejection quotient ;Q化学定量分析第三章滴定分析概论滴定分析法:titrametric analysis滴定:titration容量分析法:volumetric analysis化学计量点:stoichiometric point等当点:equivalent point电荷平衡:charge balance电荷平衡式:charge balance equation质量平衡:mass balance物料平衡:material balance质量平衡式:mass balance equation第四章酸碱滴定法酸碱滴定法:acid-base titrations 质子自递反应:auto protolysis reaction质子自递常数:autoprotolysis constant质子条件式:proton balance equation酸碱指示剂:acid-base indicator指示剂常数:indicator constant变色范围:colour change interval混合指示剂:mixed indicator双指示剂滴定法:double indicator titration第五章非水滴定法非水滴定法:nonaqueous titrations质子溶剂:protonic solvent酸性溶剂:acid solvent碱性溶剂:basic solvent两性溶剂:amphototeric solvent无质子溶剂:aprotic solvent均化效应:differentiatin g effect区分性溶剂:differentiating solvent离子化:ionization离解:dissociation结晶紫:crystal violet萘酚苯甲醇: α-naphthalphenol benzyl alcohol奎哪啶红:quinadinered百里酚蓝:thymol blue偶氮紫:azo violet溴酚蓝:bromophenol blue第六章配位滴定法配位滴定法:compleximetry乙二胺四乙酸:ethylenediamine tetraacetic acid,EDTA 螯合物:chelate compound金属指示剂:metal lochrome indcator第七章氧化还原滴定法氧化还原滴定法:oxidation-reduction titration碘量法:iodimetry溴量法:bromimetry ]溴量法:bromine method铈量法:cerimetry高锰酸钾法:potassium permanganate method条件电位:conditional potential溴酸钾法:potassium bromate method硫酸铈法:cerium sulphate method偏高碘酸:metaperiodic acid高碘酸盐:periodate亚硝酸钠法:sodium nitrite method重氮化反应:diazotization reaction重氮化滴定法:diazotization titration亚硝基化反应:nitrozation reaction亚硝基化滴定法:nitrozation titration外指示剂:external indicator外指示剂:outside indicator重铬酸钾法:potassium dichromate method 第八章沉淀滴定法沉淀滴定法:precipitation titration容量滴定法:volumetric precipitation method 银量法:argentometric method第九章重量分析法重量分析法:gravimetric analysis挥发法:volatilization method引湿水(湿存水):water of hydroscopicity 包埋(藏)水:occluded water吸入水:water of imbibition结晶水:water of crystallization组成水:water of composition液-液萃取法:liquid-liquid extration溶剂萃取法:solvent extration反萃取:counter extraction分配系数:partition coefficient分配比:distribution ratio离子对(离子缔合物):ion pair沉淀形式:precipitation forms称量形式:weighing forms仪器分析概述物理分析:physical analysis物理化学分析:physicochemical analysis仪器分析:instrumental analysis第十章电位法及永停滴定法电化学分析:electrochemical analysis电解法:electrolytic analysis method电重量法:electrogravimetry库仑法:coulo metry库仑滴定法:coulo metric titration电导法:conductometry电导分析法:conductometric analysis电导滴定法:conductometric titration电位法:potentiometry直接电位法:dirext potentiometry电位滴定法:potentiometric titration伏安法:voltammetry极谱法:polarography溶出法:stripping method电流滴定法:amperometric titration化学双电层:chemical double layer相界电位:phase boundary potential 金属电极电位:electrode potential化学电池:chemical cell液接界面:liquid junction boundary原电池:galvanic cell电解池:electrolytic cell负极:cathode正极:anode电池电动势:eletromotive force指示电极:indicator electrode参比电极:reference electroade标准氢电极:standard hydrogen electrode一级参比电极:primary reference electrode饱和甘汞电极:saturated calomel electrode银-氯化银电极:silver silver-chloride electrode液接界面:liquid junction boundary不对称电位:asymmetry potential表观PH值:apparent PH复合PH电极:combination PH electrode离子选择电极:ion selective electrode敏感器:sensor晶体电极:crystalline electrodes均相膜电极:homogeneous membrance electrodes非均相膜电极:heterogeneous membrance electrodes非晶体电极:non- crystalline electrodes刚性基质电极:rigid matrix electrode流流体载动电极:electrode with a mobile carrier气敏电极:gas sensing electrodes酶电极:enzyme electrodes金属氧化物半导体场效应晶体管:MOSFET离子选择场效应管:ISFET总离子强度调节缓冲剂:total ion strength adjustment buffer,TISAB永停滴定法:dead-stop titration双电流滴定法(双安培滴定法):double amperometric titration 第十一章光谱分析法概论普朗克常数:Plank constant电磁波谱:electromagnetic spectrum光谱:spectrum光谱分析法:spectroscopic analysis原子发射光谱法:atomic emission spectroscopy质量谱:mass spectrum质谱法:mass spectroscopy,MS第十二章紫外-可见分光光度法紫外-可见分光光度法:ultraviolet and visible spectrophotometry;UV-vis肩峰:shoulder peak末端吸收:end absorbtion生色团:chromophore助色团:auxochrome红移:red shift长移:bathochromic shift短移:hypsochromic shift蓝(紫)移:blue shift增色效应(浓色效应):hyperchromic effect减色效应(淡色效应):hypochromic effect强带:strong band弱带:weak band吸收带:absorption band透光率:transmitance,T吸光度:absorbance谱带宽度:band width杂散光:stray light噪声:noise暗噪声:dark noise散粒噪声:signal shot noise闪耀光栅:blazed grating全息光栅:holographic grating光二极管阵列检测器:photodiode array detector 偏最小二乘法:partial least squares method ,PLS褶合光谱法:convolution spectrometry褶合变换:convolution transform,CT离散小波变换:wavelet transform,WT多尺度细化分析:multiscale analysis供电子取代基:electron donating group吸电子取代基:electron with-drawing group第十三章荧光分析法荧光:fluorescence荧光分析法:fluorometryX-射线荧光分析法:X-ray fluorometry原子荧光分析法:atomic fluorometry分子荧光分析法:molecular fluorometry振动弛豫:vibrational relaxation内转换:internal conversion外转换:external conversion体系间跨越:intersystem crossing激发光谱:excitation spectrum荧光光谱:fluorescence spectrum斯托克斯位移:Stokes shift荧光寿命:fluorescence life time荧光效率:fluorescence efficiency荧光量子产率:fluorescence quantum yield荧光熄灭法:fluorescence quenching method散射光:scattering light瑞利光:R a yleith scattering light拉曼光:Raman scattering lightAbbe refractometer 阿贝折射仪absorbance 吸收度absorbance ratio 吸收度比值absorption 吸收absorption curve 吸收曲线absorption spectrum 吸收光谱absorptivity 吸收系数accuracy 准确度acid-dye colorimetry 酸性染料比色法acidimetry 酸量法acid-insoluble ash 酸不溶性灰分acidity 酸度activity 活度第十四章色谱法additive 添加剂additivity 加和性adjusted retention time 调整保留时间adsorbent 吸附剂adsorption 吸附affinity chromatography 亲和色谱法aliquot (一)份alkalinity 碱度alumina 氧化铝ambient temperature 室温ammonium thiocyanate 硫氰酸铵analytical quality control(AQC)分析质量控制anhydrous substance 干燥品anionic surfactant titration 阴离子表面活性剂滴定法antibiotics-microbial test 抗生素微生物检定法antioxidant 抗氧剂appendix 附录application of sample 点样area normalization method 面积归一化法argentimetry 银量法arsenic 砷arsenic stain 砷斑ascending development 上行展开ash-free filter paper 无灰滤纸(定量滤纸)assay 含量测定assay tolerance 含量限度atmospheric pressure ionization(API) 大气压离子化attenuation 衰减back extraction 反萃取back titration 回滴法bacterial endotoxins test 细菌内毒素检查法band absorption 谱带吸收baseline correction 基线校正baseline drift 基线漂移batch, lot 批batch(lot) number 批号Benttendorff method 白田道夫(检砷)法between day (day to day, inter-day) precision 日间精密度between run (inter-run) precision 批间精密度biotransformation 生物转化bioavailability test 生物利用度试验bioequivalence test 生物等效试验biopharmaceutical analysis 体内药物分析,生物药物分析blank test 空白试验boiling range 沸程British Pharmacopeia (BP) 英国药典bromate titration 溴酸盐滴定法bromimetry 溴量法bromocresol green 溴甲酚绿bromocresol purple 溴甲酚紫bromophenol blue 溴酚蓝bromothymol blue 溴麝香草酚蓝bulk drug, pharmaceutical product 原料药buret 滴定管by-product 副产物calibration curve 校正曲线calomel electrode 甘汞电极calorimetry 量热分析capacity factor 容量因子capillary zone electrophoresis (CZE) 毛细管区带电泳capillary gas chromatography 毛细管气相色谱法carrier gas 载气cation-exchange resin 阳离子交换树脂ceri(o)metry 铈量法characteristics, description 性状check valve 单向阀chemical shift 化学位移chelate compound 鳌合物chemically bonded phase 化学键合相chemical equivalent 化学当量Chinese Pharmacopeia (ChP) 中国药典Chinese material medicine 中成药Chinese materia medica 中药学Chinese materia medica preparation 中药制剂Chinese Pharmaceutical Association (CPA) 中国药学会chiral 手性的chiral stationary phase (CSP) 手性固定相chiral separation 手性分离chirality 手性chiral carbon atom 手性碳原子chromatogram 色谱图chromatography 色谱法chromatographic column 色谱柱chromatographic condition 色谱条件chromatographic data processor 色谱数据处理机chromatographic work station 色谱工作站clarity 澄清度clathrate, inclusion compound 包合物clearance 清除率clinical pharmacy 临床药学coefficient of distribution 分配系数coefficient of variation 变异系数color change interval (指示剂)变色范围color reaction 显色反应colorimetric analysis 比色分析colorimetry 比色法column capacity 柱容量column dead volume 柱死体积column efficiency 柱效column interstitial volume 柱隙体积column outlet pressure 柱出口压column temperature 柱温column pressure 柱压column volume 柱体积column overload 柱超载column switching 柱切换committee of drug evaluation 药品审评委员会comparative test 比较试验completeness of solution 溶液的澄清度compound medicines 复方药computer-aided pharmaceutical analysis 计算机辅助药物分析concentration-time curve 浓度-时间曲线confidence interval 置信区间confidence level 置信水平confidence limit 置信限congealing point 凝点congo red 刚果红(指示剂)content uniformity 装量差异controlled trial 对照试验correlation coefficient 相关系数contrast test 对照试验counter ion 反离子(平衡离子)cresol red 甲酚红(指示剂)crucible 坩埚crude drug 生药crystal violet 结晶紫(指示剂)cuvette, cell 比色池cyanide 氰化物cyclodextrin 环糊精cylinder, graduate cylinder, measuring cylinder 量筒cylinder-plate assay 管碟测定法daughter ion (质谱)子离子dead space 死体积dead-stop titration 永停滴定法dead time 死时间decolorization 脱色decomposition point 分解点deflection 偏差deflection point 拐点degassing 脱气deionized water 去离子水deliquescence 潮解depressor substances test 降压物质检查法derivative spectrophotometry 导数分光光度法derivatization 衍生化descending development 下行展开desiccant 干燥剂detection 检查detector 检测器developer, developing reagent 展开剂developing chamber 展开室deviation 偏差dextrose 右旋糖,葡萄糖diastereoisomer 非对映异构体diazotization 重氮化2,6-dichlorindophenol titration 2,6-二氯靛酚滴定法differential scanning calorimetry (DSC) 差示扫描热量法differential spectrophotometry 差示分光光度法differential thermal analysis (DTA) 差示热分析differentiating solvent 区分性溶剂diffusion 扩散digestion 消化diphastic titration 双相滴定disintegration test 崩解试验dispersion 分散度dissolubility 溶解度dissolution test 溶出度检查distilling range 馏程distribution chromatography 分配色谱distribution coefficient 分配系数dose 剂量drug control institutions 药检机构drug quality control 药品质量控制drug release 药物释放度drug standard 药品标准drying to constant weight 干燥至恒重dual wavelength spectrophotometry 双波长分光光度法duplicate test 重复试验effective constituent 有效成分effective plate number 有效板数efficiency of column 柱效electron capture detector 电子捕获检测器electron impact ionization 电子轰击离子化electrophoresis 电泳electrospray interface 电喷雾接口electromigration injection 电迁移进样elimination 消除eluate 洗脱液elution 洗脱emission spectrochemical analysis 发射光谱分析enantiomer 对映体end absorption 末端吸收end point correction 终点校正endogenous substances 内源性物质enzyme immunoassay(EIA) 酶免疫分析enzyme drug 酶类药物enzyme induction 酶诱导enzyme inhibition 酶抑制eosin sodium 曙红钠(指示剂)epimer 差向异构体equilibrium constant 平衡常数equivalence point 等当点error in volumetric analysis 容量分析误差excitation spectrum 激发光谱exclusion chromatography 排阻色谱法expiration date 失效期external standard method 外标法extract 提取物extraction gravimetry 提取重量法extraction titration 提取容量法extrapolated method 外插法,外推法factor 系数,因数,因子feature 特征Fehling’s reaction 费林反应field disorption ionization 场解吸离子化field ionization 场致离子化filter 过滤,滤光片filtration 过滤fineness of the particles 颗粒细度flame ionization detector(FID) 火焰离子化检测器flame emission spectrum 火焰发射光谱flask 烧瓶flow cell 流通池flow injection analysis 流动注射分析flow rate 流速fluorescamine 荧胺fluorescence immunoassay(FIA) 荧光免疫分析fluorescence polarization immunoassay(FPIA) 荧光偏振免疫分析fluorescent agent 荧光剂fluorescence spectrophotometry 荧光分光光度法fluorescence detection 荧光检测器fluorimetyr 荧光分析法foreign odor 异臭foreign pigment 有色杂质formulary 处方集fraction 馏分freezing test 结冻试验funnel 漏斗fused peaks, overlapped peaks 重叠峰fused silica 熔融石英gas chromatography(GC) 气相色谱法gas-liquid chromatography(GLC) 气液色谱法gas purifier 气体净化器gel filtration chromatography 凝胶过滤色谱法gel permeation chromatography 凝胶渗透色谱法general identification test 一般鉴别试验general notices (药典)凡例general requirements (药典)通则good clinical practices(GCP) 药品临床管理规范good laboratory practices(GLP) 药品实验室管理规范good manufacturing practices(GMP) 药品生产质量管理规范good supply practices(GSP) 药品供应管理规范gradient elution 梯度洗脱grating 光栅gravimetric method 重量法Gutzeit test 古蔡(检砷)法half peak width 半峰宽[halide] disk method, wafer method, pellet method 压片法head-space concentrating injector 顶空浓缩进样器heavy metal 重金属heat conductivity 热导率height equivalent to a theoretical plate 理论塔板高度height of an effective plate 有效塔板高度high-performance liquid chromatography (HPLC) 高效液相色谱法high-performance thin-layer chromatography (HPTLC) 高效薄层色谱法hydrate 水合物hydrolysis 水解hydrophilicity 亲水性hydrophobicity 疏水性hydroscopic 吸湿的hydroxyl value 羟值hyperchromic effect 浓色效应hypochromic effect 淡色效应identification 鉴别ignition to constant weight 灼烧至恒重immobile phase 固定相immunoassay 免疫测定impurity 杂质inactivation 失活index 索引indicator 指示剂indicator electrode 指示电极inhibitor 抑制剂injecting septum 进样隔膜胶垫injection valve 进样阀instrumental analysis 仪器分析insulin assay 胰岛素生物检定法integrator 积分仪intercept 截距interface 接口interference filter 干涉滤光片intermediate 中间体internal standard substance 内标物质international unit(IU) 国际单位in vitro 体外in vivo 体内iodide 碘化物iodoform reaction 碘仿反应iodometry 碘量法ion-exchange cellulose 离子交换纤维素ion pair chromatography 离子对色谱ion suppression 离子抑制ionic strength 离子强度ion-pairing agent 离子对试剂ionization 电离,离子化ionization region 离子化区irreversible indicator 不可逆指示剂irreversible potential 不可逆电位isoabsorptive point 等吸收点isocratic elution 等溶剂组成洗脱isoelectric point 等电点isoosmotic solution 等渗溶液isotherm 等温线Karl Fischer titration 卡尔·费歇尔滴定kinematic viscosity 运动黏度Kjeldahl method for nitrogen 凯氏定氮法Kober reagent 科伯试剂Kovats retention index 科瓦茨保留指数labelled amount 标示量leading peak 前延峰least square method 最小二乘法leveling effect 均化效应licensed pharmacist 执业药师limit control 限量控制limit of detection(LOD) 检测限limit of quantitation(LOQ) 定量限limit test (杂质)限度(或限量)试验limutus amebocyte lysate(LAL) 鲎试验linearity and range 线性及范围linearity scanning 线性扫描liquid chromatograph/mass spectrometer (LC/MS) 液质联用仪litmus paper 石蕊试纸loss on drying 干燥失重low pressure gradient pump 低压梯度泵luminescence 发光lyophilization 冷冻干燥main constituent 主成分make-up gas 尾吹气maltol reaction 麦牙酚试验Marquis test 马奎斯试验mass analyzer detector 质量分析检测器mass spectrometric analysis 质谱分析mass spectrum 质谱图mean deviation 平均偏差measuring flask, volumetric flask 量瓶measuring pipet(te) 刻度吸量管medicinal herb 草药melting point 熔点melting range 熔距metabolite 代谢物metastable ion 亚稳离子methyl orange 甲基橙methyl red 甲基红micellar chromatography 胶束色谱法micellar electrokinetic capillary chromatography(MECC, MEKC) 胶束电动毛细管色谱法micelle 胶束microanalysis 微量分析microcrystal 微晶microdialysis 微透析micropacked column 微型填充柱microsome 微粒体microsyringe 微量注射器migration time 迁移时间millipore filtration 微孔过滤minimum fill 最低装量mobile phase 流动相modifier 改性剂,调节剂molecular formula 分子式monitor 检测,监测monochromator 单色器monographs 正文mortar 研钵moving belt interface 传送带接口multidimensional detection 多维检测multiple linear regression 多元线性回归multivariate calibration 多元校正natural product 天然产物Nessler glasses(tube) 奈斯勒比色管Nessler’s r eagent 碱性碘化汞钾试液neutralization 中和nitrogen content 总氮量nonaqueous acid-base titration 非水酸碱滴定nonprescription drug, over the counter drugs (OTC drugs) 非处方药nonproprietary name, generic name 非专有名nonspecific impurity 一般杂质non-volatile matter 不挥发物normal phase 正相normalization 归一化法notice 凡例nujol mull method 石蜡糊法octadecylsilane chemically bonded silica 十八烷基硅烷键合硅胶octylsilane 辛(烷)基硅烷odorless 无臭official name 法定名official specifications 法定标准official test 法定试验on-column detector 柱上检测器on-column injection 柱头进样on-line degasser 在线脱气设备on the dried basis 按干燥品计opalescence 乳浊open tubular column 开管色谱柱optical activity 光学活性optical isomerism 旋光异构optical purity 光学纯度optimization function 优化函数organic volatile impurities 有机挥发性杂质orthogonal function spectrophotometry 正交函数分光光度法orthogonal test 正交试验orthophenanthroline 邻二氮菲outlier 可疑数据,逸出值overtones 倍频峰,泛频峰oxidation-reduction titration 氧化还原滴定oxygen flask combustion 氧瓶燃烧packed column 填充柱packing material 色谱柱填料palladium ion colorimetry 钯离子比色法parallel analysis 平行分析parent ion 母离子particulate matter 不溶性微粒partition coefficient 分配系数parts per million (ppm) 百万分之几pattern recognition 模式识别peak symmetry 峰不对称性peak valley 峰谷peak width at half height 半峰宽percent transmittance 透光百分率pH indicator absorbance ratio method? pH指示剂吸光度比值法pharmaceutical analysis 药物分析pharmacopeia 药典pharmacy 药学phenolphthalein 酚酞photodiode array detector(DAD) 光电二极管阵列检测器photometer 光度计pipeclay triangle 泥三角pipet(te) 吸移管,精密量取planar chromatography 平板色谱法plate storage rack 薄层板贮箱polarimeter 旋光计polarimetry 旋光测定法polarity 极性polyacrylamide gel 聚丙酰胺凝胶polydextran gel 葡聚糖凝胶polystyrene gel 聚苯乙烯凝胶polystyrene film 聚苯乙烯薄膜porous polymer beads 高分子多孔小球post-column derivatization 柱后衍生化potentiometer 电位计potentiometric titration 电位滴定法precipitation form 沉淀形式precision 精密度pre-column derivatization 柱前衍生化preparation 制剂prescription drug 处方药pretreatment 预处理primary standard 基准物质principal component analysis 主成分分析programmed temperature gas chromatography 程序升温气相色谱法prototype drug 原型药物provisions for new drug approval 新药审批办法purification 纯化purity 纯度pyrogen 热原pycnometric method 比重瓶法quality control(QC) 质量控制quality evaluation 质量评价quality standard 质量标准quantitative determination 定量测定quantitative analysis 定量分析quasi-molecular ion 准分子离子racemization 消旋化radioimmunoassay 放射免疫分析法random sampling 随机抽样rational use of drug 合理用药readily carbonizable substance 易炭化物reagent sprayer 试剂喷雾器recovery 回收率reference electrode 参比电极refractive index 折光指数related substance 有关物质relative density 相对密度relative intensity 相对强度repeatability 重复性replicate determination 平行测定reproducibility 重现性residual basic hydrolysis method 剩余碱水解法residual liquid junction potential 残余液接电位residual titration 剩余滴定residue on ignition 炽灼残渣resolution 分辨率,分离度response time 响应时间retention 保留reversed phase chromatography 反相色谱法reverse osmosis 反渗透rider peak 驼峰rinse 清洗,淋洗robustness 可靠性,稳定性routine analysis 常规分析round 修约(数字)ruggedness 耐用性safety 安全性Sakaguchi test 坂口试验salt bridge 盐桥salting out 盐析sample applicator 点样器sample application 点样sample on-line pretreatment 试样在线预处理sampling 取样saponification value 皂化值saturated calomel electrode(SCE) 饱和甘汞电极selectivity 选择性separatory funnel 分液漏斗shoulder peak 肩峰signal to noise ratio 信噪比significant difference 显著性差异significant figure 有效数字significant level 显著性水平significant testing 显著性检验silanophilic interaction 亲硅羟基作用silica gel 硅胶silver chloride electrode 氯化银电极similarity 相似性simultaneous equations method 解线性方程组法size exclusion chromatography(SEC) 空间排阻色谱法sodium dodecylsulfate, SDS 十二烷基硫酸钠sodium hexanesulfonate 己烷磺酸钠sodium taurocholate 牛璜胆酸钠sodium tetraphenylborate 四苯硼钠sodium thiosulphate 硫代硫酸钠solid-phase extraction 固相萃取solubility 溶解度solvent front 溶剂前沿solvophobic interaction 疏溶剂作用specific absorbance 吸收系数specification 规格specificity 专属性specific rotation 比旋度specific weight 比重spiked 加入标准的split injection 分流进样splitless injection 无分流进样spray reagent (平板色谱中的)显色剂spreader 铺板机stability 稳定性standard color solution 标准比色液standard deviation 标准差standardization 标定standard operating procedure(SOP) 标准操作规程standard substance 标准品stationary phase coating 固定相涂布starch indicator 淀粉指示剂statistical error 统计误差sterility test 无菌试验stirring bar 搅拌棒stock solution 储备液stoichiometric point 化学计量点storage 贮藏stray light 杂散光substituent 取代基substrate 底物sulfate 硫酸盐sulphated ash 硫酸盐灰分supercritical fluid chromatography(SFC) 超临界流体色谱法support 载体(担体)suspension 悬浊液swelling degree 膨胀度symmetry factor 对称因子syringe pump 注射泵systematic error 系统误差system model 系统模型system suitability 系统适用性tablet 片剂tailing factor 拖尾因子tailing peak 拖尾峰tailing-suppressing reagent 扫尾剂test of hypothesis 假设检验test solution(TS) 试液tetrazolium colorimetry 四氮唑比色法therapeutic drug monitoring(TDM) 治疗药物监测thermal analysis 热分析法thermal conductivity detector 热导检测器thermocouple detector 热电偶检测器thermogravimetric analysis(TGA) 热重分析法thermospray interface 热喷雾接口The United States Pharmacopoeia(USP) 美国药典The Pharmacopoeia of Japan(JP) 日本药局方thin layer chromatography(TLC) 薄层色谱法thiochrome reaction 硫色素反应three-dimensional chromatogram 三维色谱图thymol 百里酚(麝香草酚)(指示剂)thymolphthalein 百里酚酞(麝香草酚酞)(指示剂)thymolsulfonphthalein ( thymol blue) 百里酚蓝(麝香草酚蓝)(指示剂)titer, titre 滴定度time-resolved fluoroimmunoassay 时间分辨荧光免疫法titrant 滴定剂titration error 滴定误差titrimetric analysis 滴定分析法tolerance 容许限toluene distillation method 甲苯蒸馏法toluidine blue 甲苯胺蓝(指示剂)total ash 总灰分total quality control(TQC) 全面质量控制traditional drugs 传统药traditional Chinese medicine 中药transfer pipet 移液管turbidance 混浊turbidimetric assay 浊度测定法turbidimetry 比浊法turbidity 浊度ultracentrifugation 超速离心ultrasonic mixer 超生混合器ultraviolet irradiation 紫外线照射undue toxicity 异常毒性uniform design 均匀设计uniformity of dosage units 含量均匀度uniformity of volume 装量均匀性(装量差异)uniformity of weight 重量均匀性(片重差异)validity 可靠性variance 方差versus …对…,…与…的关系曲线viscosity 粘度volatile oil determination apparatus 挥发油测定器volatilization 挥发法volumetric analysis 容量分析volumetric solution(VS) 滴定液vortex mixer 涡旋混合器watch glass 表面皿wave length 波长wave number 波数weighing bottle 称量瓶weighing form 称量形式weights 砝码well-closed container 密闭容器xylene cyanol blue FF 二甲苯蓝FF(指示剂)xylenol orange 二甲酚橙(指示剂)zigzag scanning 锯齿扫描zone electrophoresis 区带电泳zwitterions 两性离子zymolysis 酶解作用簡體書目錄Chapter 1 Introduction 緒論1.1 The nature of analytical chemistry 分析化學的性質1.2 The role of analytical chemistry 分析化學的作用1.3 The classification of analytical chemistry分析化學的分類1.4 The total analytical process分析全過程Terms to understand重點內容概述Chapter 2 Errors and Data Treatment in Quantitative Analysis 定量分析中的誤差及數據處理2.1 Fundamental terms of errors誤差的基本術語2.2 Types of errors in experimental data實驗數據中的誤差類型2.2.1 Systematic errors 系統誤差2.2.2 Random errors偶然誤差2.3 Evaluation of analytical data分析數據的評價2.3.1 Tests of significance顯著性檢驗2.3.2 Rejecting data可疑值取捨2.4 Significant figures有效數字ProblemsTerms to understand重點內容概述Chapter 3 Titrimetric Analysis滴定分析法3.1 General principles基本原理3.1.1 Relevant terms of titrimetric analysis滴定分析相關術語3.1.2 The preparation of standard solution and the expression of concentration 標準溶液的配製與濃度表示方法3.1.3 The types of titrimetric reactions滴定反應類型3.2 Acid-base titration酸鹼滴定3.2.1 Acid-base equilibria 酸鹼平衡3.2.2 Titration curves滴定曲線3.2.3 Acid-base indicators酸鹼指示劑3.2.4 Applications of acid-base titration酸鹼滴定的應用3.3 Complexometric titration配位滴定3.3.1 Metal-chelate complexes金屬螯合物3.3.2 EDTA 乙二胺四乙酸3.3.3 EDTA titration curves EDTA滴定曲線3.3.4 Metal Ion indicators金屬離子指示劑3.3.5 Applications of EDTA titration techniques EDTA滴定方法的應用3.4 Oxidation-reduction titration氧化還原滴定3.4.1 Redox reactions氧化還原反應3.4.2 Rate of redox reactions氧化還原反應的速率3.4.3 Titration curves滴定曲線3.4.4 Redox indicators氧化還原指示劑3.4.5 Applications of redox titrations氧化還原滴定的應用3.5 Precipitation titration沉澱滴定3.5.1 Precipitation reactions沉澱滴定反應3.5.2 Titration curves滴定曲線3.5.3 End-point detection終點檢測ProblemsTerms to understand重點內容概述Chapter 4 Potentiometry 電位分析法4.1 Introduction簡介4.1.1 Classes and characteristics分類及性質4.1.2 Definition定義4.2 Types of potentiometric electrodes電極種類4.2.1 Reference electrodes 參比電極4.2.2 Indicator electrodes指示電極4.2.3 Electrode response and selectivity電極響應及選擇性4.3 Potentiometric methods and application電位法及應用4.3.1 Direct potentiometric measurement 直接電位法4.3.2 Potentiometric titrations電位滴定4.3.3 Applications of potentiometry 電位法應用ProblemsTerlns to understand重點內容概述Chapter 5 Chromatography色譜法5.1 An introduction to chromatographic methods色譜法概述5.2 Fundamental theory of gas chromatography氣相色譜基本原理5.2.1 Plate theory塔板理論5.2.2 Kinetic theory(rate theory) 速率理論5.2.3 The resolution Rs as a measure of peak separation 分離度5.3 Gas chromatography 氣相色譜5.3.1 Components of a gas chromatograph 氣相色譜儀的組成5.3.2 Stationary phases for gas-liquid chromatography 氣液色譜固定相5.3.3 Applications of gas-liquid chromatography 氣液色譜的應用5.3.4 Adsorption chromatography 吸附色譜5.4 High performance liquid chromatography 高效液相色譜5.4.1 Instrumentation 儀器組成5.4.2 High-performance partition chromatography 高效分配色譜5.5 Miscellaneous separation methods 其他分離方法5.5.1 High-performance ion-exchange chromatography 高效離子交換色譜5.5.2 Capillary electrophoresis 毛細管電泳5.5.3 Planar chromatography 平板色譜ProblemsTerms to understand重點內容概述Chapter 6 Atomic Absorption Spectrometry原子吸收光譜分析法6.1 Introduction 概述6.2 Principles 原理6.2.1 The process of AAS,resonance line and absorption line 原子吸收光譜法的過程,共振線及吸收線6.2.2 The number of ground atom and the temperature of flame 基態原子數與光焰溫度6.2.3 Quantitative analysis of AAS原子吸收光譜定量分析6.3 Instrumentation 儀器6.3.1 Primary radiation sources 光源6.3.2 Atomizer 原子儀器6.3.3 Optical dispersive systems 分光系統6.3.4 Detectors 檢測器6.3.5 Signal measurements 信號測量6.4 Quantitative measurements and interferences 定量測定及干擾6.4.1 Quantitative measurements 定量測定6.4.2 Interferences 干擾6.4.3 Sensitivity6.5 Applications of AAS原子吸收光譜法的應用ProblemsTerms to understand重點內容概述Chapter 7 Ultraviolet and Visible Spectrophotometry 紫外-可見分光光度法7.1 Introduction簡介7.2 Ultraviolet and visible absorption spectroscopy 紫外-可見吸收光譜7.2.1 Introduction for radiant energy 輻射能簡介7.2.2 Selective absorption of radiation and absorbance spectrum 物質對光的選擇性吸收和吸收光譜7.2.3 Absorbing species and electron transition 吸收物質與電子躍遷7.3 Law of absorption吸收定律7.3.1 Lambert-Beer's law朗伯-比爾定律7.3.2 Absorptivity吸光係數7.3.3 Apparent deviations from Beer's law對比爾定律的明顯偏離7.4 Instruments儀器7.5 General types of spectrophotometer分光光度計種類7.6 Application of UV-Vis absorption spectroscopy 紫外-可見吸收光譜的應用7.6.1 Application of absorption measurement to qualitative analysis 光吸收測定在定性分析上的應用7.6.2 Quantitative analysis by absorption measurements 光吸收測量定量分析法7.6.3 Derivative spectrophotometry 導數分光光度法ProblemsTerms to understand重點內容概述Chapter 8 Infrared Absorption Spectroscopy紅外吸收光譜8.1 Theory of infrared absorption紅外吸收基本原理8.1.1 Dipole changes during vibrations and rotations 振轉運動中的偶極距變化8.1.2 Mechanical model of stretching vibrations 伸縮振動機械模型8.1.3 Quantum treatment of vibrations 振動的量子力學處理、8.1.4 Types of molecular vibrations分子振動形式8.2 Infrared instrument components紅外儀器組成8.2.1 Wavelength selection波長選擇8.2.2 Sampling techniques 採樣技術8.2.3 Infrared spectrophotometers for qualitative analysis 定性分析用紅外分光光度計8.2.4 Other techniques其他技術8.3 The group frequencies of functional groups in organic compounds 有機化合物官能團的特徵頻率8.4 The factors affecting group frequencies 影響基團特徵吸收頻率的因素8.4.1 Adjacent groups 鄰近基團的影響。
molecular-dynamics simulations

Pressure-transmitting boundary conditions formolecular-dynamics simulationsCarsten Sch€a fer a,Herbert M.Urbassek a,*,Leonid V.Zhigilei b,Barbara J.Garrison ca Fachbereich Physik,Universit€a t Kaiserslautern,Erwin-Schr€o dinger-Straße,D-67663Kaiserslautern,Germanyb Department of Materials Science and Engineering,University of Virginia,Charlottesville,VA22903,USAc Department of Chemistry,152Davey Laboratory,Pennsylvania State University,University Park,PA16802,USAReceived10October2001;accepted20November2001AbstractA scheme for establishing boundary conditions in molecular-dynamics simulations that prevent pressure wave reflection out of the simulation volume is formulated.The algorithm is easily implemented for a one-dimensional geometry.Its efficiency is tested for compressive waves in Cu.Ó2002Elsevier Science B.V.All rights reserved. PACS:02.70.Ns1.IntroductionMolecular-dynamics calculations are generally used to simulate macroscopic systems containing a large number(in the order of Avogadro’s number, 1023)of atoms.By necessity,the simulation con-centrates on a small part of the system,on the order of several hundreds[1]to a maximum[2] of nowadays5Â109atoms.This reduction in sys-tem size is possible,if convenient boundary con-ditions are applied to the simulation volume;these boundary conditions are chosen to mimic the re-sponse of the surrounding material to the pro-cesses occurring in the simulation volume itself.The boundary conditions applicable to simula-tions in thermodynamic equilibrium(static re-sponse)have been well studied in the past[3–6]. However,nowadays,often non-equilibrium pro-cesses are to be simulated.Then the formulation of the appropriate boundary conditions poses a larger challenge,as they need to incorporate the dynamic response of the surroundings to the pro-cesses occurring in the simulation volume.Thus, for example,if heat is produced in the simulation volume,the boundaries have to dispense of it in a realistic way,mimicking the natural heat con-duction of the solid.This can be achieved using so-called energy-dissipating boundary conditions [7,8].This problem arises, e.g.,when energetic processes,such as ion or laser irradiation of a solidComputational Materials Science24(2002)421–429*Corresponding author.E-mail address:urbassek@rhrk.uni-kl.de(H.M.Urbassek).URL:http://www.physik.uni-kl.de/urbassek.0927-0256/02/$-see front matterÓ2002Elsevier Science B.V.All rights reserved. PII:S0927-0256(01)00263-4surface,are simulated[7,9].Analogously,stress produced in the simulation volume has to be re-laxed at the boundaries in a way compatible with the elastic properties of the solid.Again,this is quite a commonly encountered phenomenon in irradiation or impact simulations[10,11].The latter class of boundary conditions is more difficult to formulate.In fact,a number of schemes have been published recently to solve this problem that are based on a generalized Langevin approach [12–14],in which the reaction of the surrounding is incorporated into the dynamical equations of the simulation volume via(delayed)forces[15,16].It turns out,however,that the calculation of these boundary conditions is rather time consuming. For this reason,we propose in this paper a simple phenomenological scheme to handle pressure re-laxation and pressure wave transmission,which is easy to implement and adds virtually no extra computational costs to the simulation.This scheme was introduced in Ref.[17],and used re-peatedly for applications in laser ablation of solids [11,18,19].We shall validate the scheme for a me-tallic system by investigating its ability to transmit pressure waves.2.Formulation of boundary conditions2.1.Passage of a pressure wave through matterIn order to set the stage,we remind the reader of the basic properties of a pressure wave in a solid.Fig.1exemplifies the conditions in a mate-rial through which a pressure wave passes.The figure was taken from a molecular-dynamics sim-ulation of Cu;the wave was started by applying a sudden force perpendicular to the surface(for de-tails see Section3below).The quantities discussed below denote local averages;they were determined in the simulation as averages over a distance of r c¼6:2 A,the cutoffradius of our potential.The pressure wave runs with a velocity of55 A/ ps,which is25%above the speed of sound,which is c0¼44 A/ps for a longitudinal wave in Cu in the [100]direction;it is hence a(moderately)weak pressure wave[20].In the wave itself,the density n is increased by around10%above the nominal crystal density n0¼0:085 AÀ3.In the region of enhanced density––between27and35 A,roughly ––the atom velocity u is non-vanishing;its values, however,are small compared to c0.The pressure p, however,starts to become already strong in the leading part of the wave,before atoms have reached sizable velocities.This is in contrast to a well-known relationship[21],which has pressure to be proportional to the atom velocityp¼Àmn0c0u¼ÀZu;ð1Þwhere m is the atom mass,and Z¼mn0c0is called the impedance of the system;in our case,Z¼3:96 GPa/( A ps)¼24:7meVps/ A4.Inspection of Fig.1b and c shows that this simple proportionality does not hold on the microscopic(atomistic)scale.It is the purpose of the following section to show that a closely related proportionality does indeed hold for a well-defined part of the forces acting on an atom,viz.Eq.(4)below.2.2.Boundary forcesAs soon as such a pressure wave reaches the boundary of the simulation volume,it will be re-flected.This happens for both types of boundary conditions commonly applied,namely free and fixed boundaries.In order to prevent reflection, one has to mimic the action of the surrounding medium on the atoms close to the boundary.We do this in the following way:we define as bound-ary zone the part of the simulation volume adja-cent to the boundary(cf.Fig.2);its width D z must be(slightly)larger than r c.Atoms in the rest of the simulation volume(the MD zone)obey the origi-nal MD equations of motion with forces stemming from the neighboring atoms.Thus in Fig.2,atom i experiences a force F stemming from all atoms j surrounding it.Let us write F asF¼F topþF bottom;ð2Þwhere F top is the force originating from all atoms above i(i.e.,away from the boundary),and F bottom is the force originating from all atoms below it(i.e., closer to the boundary).Here and in the following we shall be interested only in the force components422 C.Sch€a fer et al./Computational Materials Science24(2002)421–429perpendicular to the boundary;hence we can ab-stain from using vector notation.Fig.1d shows F and its two components F top and F bottom in a pressure pulse.We observe that F top and F bottom have a simple unimodal shape,while the total force F shows the more complex structure typical of a pressure pulse,consisting of an accelerating and a decelerating part.While using interatomic interaction to determine both F top and F bottom in themolecular-dynamicszone,in the boundary zone we model F bottom by a simple expression F bc such that atoms in the boundary zone experience a total forceF¼F topþF bc;ð3Þin analogy to Eq.(2).F top is calculated from the interatomic potentials in the same way as in the MD zone.The boundary force,F bc,is directed perpendicular to the boundary.Its magnitude is determined asF bc¼F0Àa u:ð4ÞHere,F0is a static contribution,which is present even if atoms do not move.It is needed to keep the crystal in equilibrium,to counter the force of the top atoms and to eliminate the surface tension forces acting on the atoms in the boundary region. The second part of the force,Eq.(4)is propor-tional to the velocity u of the atoms in the boundary.For the purposes of this paper––short pulses and low ambient temperatures––it is ap-propriate to take u as the velocity of the individual boundary atom.More generally,this procedure would induce an artificial cooling of the boundary, and u should be taken as the average velocity (center-of-mass velocity)of all atoms in the boundary[17].According to Eq.(1),we choose the proportionality constant a asa¼ZA¼mn0c0A;ð5Þwhere A is the cross-sectional area of an atom.Thus,Eq.(4)makes sure that the atoms in the boundary zone are subject to the pressure,Eq.(1), that they need to feel under the passage of a pressure pulse.By prescribing the correct imped-ance to the boundary we prevent acoustic waves from reflecting.We shall hence refer to a as the impedance coefficient in this paper.Note that f¼a=m may be interpreted as a friction coeffi-cient in Eq.(4).Since A is of the order of nÀ2=3,fis proportional to the Debye frequency x D¼ffiffiffiffiffiffiffiffiffiffiffiffi6p2n03pÁ c,where c is an appropriately averaged speed of sound.A friction of f AD¼px D=6was indeed derived by Adelman and Doll[12]in their generalized Langevin approach by using a Brown-ian approximation.We emphasize that the boundary zone must be determined dynamically during the simulation,to keep it a width D z above the bottommost atom layer;its absolute position and even the identity of its atoms can change during the simulation. 2.3.Determination of parametersIn the following,we shall discuss the determi-nation of the parameters necessary to implement the boundary conditions.We shall do this specifi-cally for a many-body potential as it is adequate for a metal,such as the EAM potential[22].The implementation for two-body potentials can be viewed as a special case of this more general type of potentials by setting the many-body embedding function equal to zero.We shall establish the pa-rameters here for the many-body potential defined in Ref.[23].For many-body forces,the partitioning of the (well-defined)total force into F top and F bottom needs an explication.We proceed as follows:let us write the total potential energy of the crystal asE tot¼Xi<jUðr ijÞþXiGðq iÞ;ð6Þwithqi¼Xj¼igðr ijÞ:ð7ÞHere U is a pair potential,G the embedding function,and g the atomic contribution to q i,theso-called electron density.Then the total force on atom i isF i¼Xj¼iF ij;ð8ÞwhereF ij¼Àf U0ðr ijÞþg0ðr ijÞ½G0ðq iÞþG0ðq jÞ g e ij:ð9ÞHere the dash denotes differentiation with re-spect to the argument and e ij is the unit vector pointing from atom j to atom i.F ij may be inter-preted as the force contributed by atom j to the total force on atom i;this makes the partitioning of F i into F top and F bottom by the position of atom j with respect to atom i obvious.We note that these formulae allow us to calcu-late F0,and hence the static part of the boundary force,analytically.Alternatively,F0can be con-veniently determined from a static simulation of the crystal in equilibrium(pressure p¼0).Both procedures yield F0¼0:302eV/ A.The dynamical part of the boundary force is governed by the parameter ing Eq.(5),it could be determined from the impedance of the crystal and the atomic cross-section,which(for the (100)surface)may be set equal to A¼a2=2¼6:53 A2.This gives a value of a theor¼0:162eVps/ A2,corresponding to a friction coefficient f¼2c=a¼24:4/ps.As an alternative procedure,we may determine a by performing a dynamical simulation of a pressure wave travelling through a crystal.The dependence of F bottom on the velocity u in a region far away from all boundaries can thus be deter-mined.The result of such a simulation is shown in Fig.3a.It is seen that F bottom correlates quite well with the individual velocity of each atom.A linear fit gives a simul¼0:193eVps/ A2,in quite good agreement with the estimate a theor given above.We plotted F bc according to Eq.(4)with parameters as discussed above also in Fig.1d.Good agreement with the simulated values of F bottom is observed. Note,however,that in the leading part of the pulse,F bc overestimates F bottom,while it underesti-mates it in the trailing part.We note that we performed the samefit for a soft material,condensed Ar.The results,obtained for a Lennard–Jones pair potential[24],are shown in Fig.3b.Here for negative velocities,a bi-valued result is obtained.This sort of hysteresis behavior is analogous––but more pronounced––to the be-havior found for Cu and discussed above:in the leading(trailing)part of the wave smaller(larger) forces than predicted by Eq.(4)occur.A linearfit is then useful only as an average or for one part of the hysteresis loop.3.ValidationWe shall use in this paper Cu as a model system to exemplify and test our scheme.The molecular-dynamics simulation employs the many-body po-tential defined in Ref.[23];this is of the so-called embedded-atom[22]or tight-binding[25]form. The simulation employs a Cu crystallite at0K with a(100)surface and a cross-sectional area of ð6aÞ2,where a¼3:615 A is the Cu latticeconstant.While the surface is left free,lateral periodic boundary conditions are applied at all four sides. At the bottom of the simulation volume,we shall employ the pressure-transmitting boundary con-ditions,which are the subject of this paper.A convenient way to test our scheme is to measure to which degree the boundary conditions are able to prevent pressure waves from reflection. To this end we prepare a pressure wave by ap-plying a force K for a short period of time(here: D t¼11fs)on each surface atom in the direction perpendicular to the surface.Fig.1showed the form of the resulting pressure wave in the material for a value of K¼7:5eV/ A.In order to quantify wave reflection,we intro-duce the reflection coefficientR¼E refl=E in;ð10Þwhere E in is the(constant)kinetic energy in the wave before reaching the boundary and E refl is the kinetic energy in the crystal after wave reflection. We measured E refl some time after wave reflection, when it had become constant.Let usfirst test whether the parameters F0and a appearing in the boundary force,Eq.(4),were optimal in preventing wave reflection.For this test,wefixed the wave strength to K¼7:5eV/ A, and let the wave interact with a boundary withvarying values of F0and a in the boundary force. Fig.4displays the variation of the reflection co-efficient with F0and a.The optimum reflectivity R opt reaches a value of only3.15%,which is suffi-ciently small for many applications.Note that our pressure wave is very sharp;as was shown in Ref.[15],it can be assumed that the reflection of waves with less steep boundaries will be even more re-duced than the sharp wave studied by us.R opt occurs for the value of F0determined in Section2.3;however,the corresponding value of a¼0:21eVps/ A2is somewhat larger than that found in Section2.3from Fig.3,a simul¼0:193 eVps/ A2.The reason for this increase in the opti-mum value of a may lie in the strength of our pressure pulse.We therefore performed another determination of F0and a for a really weak wave, excited with a force K¼0:75eV/ A.Then the parameter dependence is even more pronounced. Furthermore,the optimum value of a has de-creased from0.21to a value of0.19eVps/ A2,i.e., the value determined from Fig.3in Section2.3. This is due to the fact that for the weak wave,the wave speed is identical to the speed of sound c0. We note that also the scatter in the F bottom vs u correlation is reduced(not shown).Note that R is not very sensitive to changes of the impedance coefficient a in the order of10%; this is helpful,since the determination of a oc-curred either by the determination of crystal pa-rameters like c0,n0,A,which may not be known to an accuracy better than10%(note that in par-ticular the wave speed may increase with wave strength!);or by afitting procedure as displayed in Fig.3,which again is subject to some error.On the other hand,the dependence of R on F0is quite pronounced,and changes of F0by a few%change R distinctly.As outlined above,however,F0can be safely determined with high accuracy analytically or by a staticsimulation.Fig.5shows how R varies with the strength of the pressure wave.Here the optimum value of a simul¼0:193eVps/ A2as determined in Section2.3 has been kept.In particular that we did not opti-mize a for each value of the force K.Note that R is between3%and4%for a wide range of wave strengths,and only increases towards very weak waves.The reason hereto lies in the fact that these quite weak waves become very delocalized during the simulation,and look more like an acoustic wave rather than a pressure pulse.These longer wave trains transmit less efficiently through the boundary zone than a well localized pulse.Thus, the boundary impedance seems to workfine even for stronger waves,where the wave speed is defi-nitely larger than c0.4.Summary•We formulated a conceptually simple and easy-to-implement method which allows to minimize pressure wave reflection at the boundary of a molecular-dynamics simulation volume.•The method is easily adapted to a variety of ma-terials,including those with many-body interac-tion forces.•Two parameters need to be determined for im-plementing the boundary force.We discuss sev-eral ways to determine these,and measure the sensitivity of the method to the accuracy of choosing the parameters.•Wefind pressure wave reflection to be sup-pressed by our method to a level of3–4%which is adequate for many applications. AcknowledgementsThe authors are grateful to M.Moseler and G. Williams for discussions.They thank the computer center RHRK,University of Kaiserslautern,for making available computer time for this study. Appendix A.Microscopic processes during pulse passageIn this appendix,we shall study how the kinetic, potential and total energies summed over all atoms in the crystal change when a pressure pulse im-pinges on the boundary zone.Throughout this appendix,we shall study a pulse excited with a force of7.5eV/ A.Let usfirst study(Fig.1e)the spatially resolved energy content of the pressure pulse displayed in Fig.1.E pot has been set to zero for a crystal in equilibrium.We observe again the rather pronounced sharp front,followed by a rather long,but considerably weaker tail.Note that the front part of the pulse has negative potential energy.This is a feature common to all realistic potentials stretching out further than the nearest-neighbor distance:atoms in front of the pulse feel approaching atoms,and hence the bonding(at-traction)increases.Only when nearest-neighbor atoms come close,does repulsion set in,and the potential energy becomes positive.As a reference case,in Fig.6,we display the temporal changes of the kinetic and potential en-ergies in a crystal of30ML length when the pressure pulse impinges on a free surface.The pressure pulse needs about0.1ps to equilibrate potential and kinetic energy.Note that E kin>E pot, since the pulse is strong enough that the crystal forces become anharmonic.At1ps,the pulse is reflected:at the free surface the crystal almost completely relaxesðE pot¼0Þwhile all energy is converted to kinetic energy.The pulse shape changes upon reflection;hence the oscillations visible afterreflection.Fig.7shows pulse transmission through an ‘ideal boundary’:this was realized by using a large crystal(60ML)and measuring the energies only in thefirst30ML.Upon passage the kinetic energy drops very sharply(within50fs)to about5%of its initial value.The later decrease to0%is quite slow, and takes more than1ps;this is due to the pulse tail shown in Fig.1e.The potential energy shows somewhat more structure:immediately before pulse passage it increases;this occurs when the front part of the pulse possessing negative poten-tial energy(Fig.1e)has passed the ideal boundary. Immediately after passage,it decreases(even below0!);this is due to the oscillatory nature of the pulse visible in Fig.1e.Note that the total energy within thefirst30ML slightly increases immediately before the pulse leaves the zone.This happens when the attractive part of the pulse (cf.Fig.1e)just has passed into the lower30ML.Finally,Fig.8shows what happens when the pulse impinges on the boundary zone presented in this paper.The strong decrease of the energy at1.1 ps proves that this boundary zone essentially acts like the ideal boundary.However,several differ-ences are seen in detail.When the pulse enters the boundary zone,atfirst atoms are decelerated due to the action of the boundary force,Eq.(4).Then, at tffi1ps,atoms are accelerated,in close analogy to what happens at a free boundary,Fig. 6. However,while there the crystal relaxed almost completely to E pot¼0,E pot increases in the bound-ary zone,since atom motion is hindered,and hence repulsive forces dominate.Also after pulse passage,t>1ps,the energies show a temporal dependence different from that of the ideal bound-ary,Fig.7;and in particular not all energy leaves the crystal.References[1]B.J.Alder,T.E.Wainwright,J.Chem.Phys.27(1957)1208.[2]u,MRS Bull.25(9)(2000)5.[3]M.P.Allen,D.J.Tildesley(Eds.),Computer simulation ofliquids,Clarendon,Oxford,1987.[4]J.M.Haile,Molecular dynamics simulation:elementarymethods,Wiley,New York,1992.[5]D.C.Rapaport,The Art of Molecular Dynamics Simula-tion,Cambridge University Press,Cambridge,1995. [6]D.Raabe,Computational Materials Science,Wiley-VCH,Weinheim,1998.[7]J.R.Beeler Jr.,Radiation effects computer experiments,North-Holland,Amsterdam,1983.[8]Y.Wu,R.J.Friauf,J.Appl.Phys.65(1989)4714.[9]R.Smith(Ed.),Atomic and ion collisions in solids and atsurfaces,Cambridge University Press,Cambridge,1997.[10]H.Haberland,Z.Insepov,M.Moseler,Phys.Rev.B51(1995)11061.[11]L.V.Zhigilei,B.J.Garrison,J.Appl.Phys.88(2000)1281.[12]S.A.Adelman,J.D.Doll,J.Chem.Phys.64(1976)2375.[13]J.C.Tully,J.Chem.Phys.73(1980)1975.[14]D.J.Diestler,M.E.Riley,J.Chem.Phys.83(1985)3584.[15]M.Moseler,J.Nordiek,H.Haberland,Phys.Rev.B56(1997)15439.[16]W.Cai,M.de Koning,V.V.Bulatov,S.Yip,Phys.Rev.Lett.85(2000)3213.[17]L.V.Zhigilei,B.J.Garrison,Mat.Res.Soc.Symp.Proc.538(1999)491.[18]L.V.Zhigilei,B.J.Garrison,SPIE Proc.Series3254(1998)135.[19]L.V.Zhigilei, B.J.Garrison,Proceedings of the Inter-national Conference on Modeling and Simulation of Microsystems,Semiconductors,Sensors,and Actuators (MSM’99),Computational Publications,Boston,1999, p.138.[20]Y.B.Zel’dovich,Y.P.Raizer,Physics of Shock Waves andHigh-Temperature Hydrodynamic Phenomena,Vol.2, Academic Press,New York,1967,p.688.[21]Y.B.Zel’dovich,Y.P.Raizer,Physics of Shock Waves andHigh-Temperature Hydrodynamic Phenomena,Vol.2, Academic Press,New York,1967,p.742.[22]M.S.Daw,S.M.Foiles,M.Baskes,Mat.Sci.Rep.9(1993)251.[23]H.Gades,H.M.Urbassek,Nucl.Instrum.Meth.B69(1992)232.[24]L.Verlet,Phys.Rev.159(1967)98.[25]M.W.Finnis,J.E.Sinclair,Philos.Mag.A50(1984)45.C.Sch€a fer et al./Computational Materials Science24(2002)421–429429。
Numerical dispersion and absorbing boundary conditions

INTERNATIONAL JOURNAL OF NUMERICAL MODELLING:ELECTRONIC NETWORKS,DEVICES AND FIELDS Int.J.Numer.Model.2000;13:483}498Numerical dispersion and absorbing boundary conditionsPeter G.Petropoulos* RDepartment of Mathematics,New Jersey Institute of Technology,Uni v ersity Heights,Newark,NJ07102,U.S.A.SUMMARYPredictions of performance of exact and approximate absorbing boundary conditions(ABCs)do not take into account the fact that in an actual simulation it is numerical waves that are incident on the computa-tional domain boundary where they are imposed.Via a model problem in rectangular co-ordinates we identify and examine this issue.Then,we study the re#ection produced by discrete local ABCs in cylindrical co-ordinates using the"rst-order Bayliss}Turkel operator as a model.We"nd the analytical re#ection coe$cient of this ABC signi"cantly underestimates the actual re#ection on the grid.Also,we identify the source of the additional error and show it decays slowly with increasing resolution.Implications for other ABCs are discussed.Copyright 2000John Wiley&Sons,Ltd.1.INTRODUCTIONAbsorbing boundary conditions are required for the numerical solution of electromagneticscattering problems governed by Maxwell's equations formulated on unbounded regions.Theyare imposed at the outer edge(* )of a"nite-sized computational region(denoted as herein) obtained from the original unbounded domain by truncation.Their main function is to absorb alloutgoing waves from the region of interest,and to provide boundary values to complete thenumerical scheme used in in the vicinity of* .A local approximation of the appropriate non-local Dirichlet-to-Neumann map that describesoutgoing waves on* is typically employed as an ABC;a multitude of such conditions have been derived and used ever since the seminal works of Engquist and Majda[1]and Bayliss and Turkel[2].In-depth reviews of this area are available in References[3,4].Exact formulations have recently been implemented and show much promise[5,6].Also,a recently introduced alternative to ABCs surrounds with a re#ectionless wave absorbing region[7,8]to eliminate outgoing waves.The performance of an ABC,or an absorbing layer,is predicted by calculating the re#ectioncoe$cient that results when it is illuminated by model waves exiting .The ideal case is obtained*Correspondence to:Peter G.Petropoulos,Department of Mathematics,New Jersey Institute of Technology,University Heights,Newark,NJ07102,U.S.A.R E-mail:peterp@Contract/grant sponsor:AFOSR;contract/grant number:F49620-99-1-0072Recei v ed30August1998 Copyright 2000John Wiley&Sons,Ltd.Re v ised27January1999when this re #ection coe $cient is zero for all such waves.For local conditions this is possible only for a small subset of all outgoing waves on * ,while for exact conditions (and semi-in "nite absorbing regions [9],except for grazing waves)it holds for all outgoing waves.For example,the "rst-order Higdon operator,B "*R #c *V [10],is an exact ABC for the scalar wave equation in two dimensions,u RR !c (u VV #u WW )"0,when plane waves impinge normally from the left on * which here is the boundary of the left half-plane at x "0.Further,the exact ABC in spherical co-ordinates [5,6]exhibits an analytical re #ection coe $cient that is zero for the "rst N spherical harmonics of a scattered wave "eld,while the re #ectionless wave absorbing region [7,8]exhibits an exponentially small analytical re #ection coe $cient when it is terminated by applying a bound-ary condition some distance from * to obtain a layer for use as an ABC [9].In discrete space,the above-described best performance of each of the three types of ABCs is obtained only in "nely resolved computations due to the phase error introduced by the numerical dispersion inherent in all schemes used in .In Section 2we analyse the discrete B Higdon operator in order to motivate the work herein.For such local operators we also determine how one may alter them to suppress the deleterious e !ect of the interior scheme 's numerical disper-sion.To date,little has been done to analyse how discretization a !ects the performance of exact ABCs in any co-ordinate system,while the performance of discrete wave absorbing layers has not been analysed in cylindrical and spherical co-ordinates (see Reference [11]for the case of rectangular co-ordinates).We now outline the remainder of the paper.In Section 3we consider Maxwell 's equations onthe !plane of the cylindrical co-ordinate system.In Section 5we derive the numerical dispersion relation for a typical second-order accurate "nite-di !erence discretization (which is detailed in Section 4)of the hyperbolic Maxwell 's equations on a staggered polar grid;it provides the complex numerical frequency for the m th cylindrical harmonic on the grid as a function of the real wavenumber (k ),the Courant number ( M )and grid points per wavelength (N N )in the radial direction,the number of grid points in the angular direction (N (),and the electrical distance from the origin ( "k ).The distance from the origin enters since,for "xed N M ,the actual wave resolution varies with radius on the grid due to the compression/expansion of the underlying co-ordinate system.Additionally,the order of the cylindrical harmonic appears now as the equivalent of the rectangular-co-ordinates concept of angle of propagation of a plane wave.In Section 6, is used to determine and investigate the re #ection of outgoing cylindrical waves on the grid by the B Bayliss }Turkel local ABC operator;other ABCs can be similarly analysed.The main results are summarized and discussed in Section 7.2.MOTIVATIONWe consider the following two-dimensional scalar boundary-value wave problem involving the Babsorbing operator in rectangular co-ordinates:u RR !c (u VV #u WW)"0,(x ,y )3(!R ,0);(!R ,R )(1)u R #cu V"0,(x ,y )3[0];(!R ,R )where c is the wave speed.Time-harmonic plane waves with wavenumber k ,propagating at an angle w.r.t.the x -axis,are solutions of the wave equation when the dispersion relation "ck 484P.G.PETROPOULOSCopyright 2000John Wiley &Sons,Ltd.Int .J .Numer .Model .2000;13:483}498holds.Substitutingu (x ,y ,t )"e IV F >IW F \S R #R e \IV F >IW F \S R(2)in the boundary condition at x "0we obtain its analytical re #ection coe $cient,R ( )"!1!cos 1#cos(3)Thus,the B operator is an exact ABC for normally incident ( "03)plane waves.Next,the standard,second-order-accurate discretization of (1)is considered:R !cV # W h u L H J"0,(j ,l )3(!R ,1);(!R ,R ) \R V t #c \V R h u L> H J "0,(j ,l )3[0];(!R ,R )(4)where h is the mesh size, t is the timestep,and T , \T and T are,respectively,the central second di !erence,the backward di !erence and backward averaging operators in the v -direction.The numerical dispersion relation for plane wave solutions in the computational domain is [12]sin t 2 " sin k cos h 2 #sin k sin h 2 (5)where "c t /h is the Courant number ()1/(2for stability).Note that for h , t P 0we obtain P .Substitutingu L H J "e HIF F >JIF F \S L R #R e \ IF F >JIF F >S L R (6)in the discrete boundary condition at j "0we obtain its re #ection coe $cient,R ( ,kh , )"!e \ I F F sin( t /2)cos(k cos h /2)! sin(k cos h /2)cos( t /2)sin( t /2)cos(k cos h /2)# sin(k cos h /2)cos( t /2)(7)Making use of (5)and setting "03(normally incident waves),(7)reduces toR (03,kh , )"!e \ IF cos(kh /2)!(1! sin (kh /2)cos(kh /2)#(1! sin (kh /2)(8)Contrary to the analytical prediction (3),(8)shows the discrete B operator is not exact for normally incident plane waves encountered in a computation with (4).In Figure 1we plot the magnitude of R (03,kh , )vs the points per wavelength N"2 /kh for two typical values of ,485DISPERSION AND ABCs Copyright 2000John Wiley &Sons,Ltd.Int .J .Numer .Model .2000;13:483}498Figure1.Magnitude of the re#ection coe$cient of the B Higdon operator.one corresponding to the stability limit(when the interior scheme introduces the least dispersion),the other being a value used in actual computations in two dimensions.For example,ina simulation with N "10the discretization is seen to introduce signi"cant error for normal incidence as the theoretical re#ection coe$cient is exactly zero and the relative error is in"nite.InFigure2we plot the magnitude of(3)and(7)as a function of13) )903for N "10and "0.5;the performance of the discrete ABC for plane waves near normal incidence is much worse than that expected from(3).The form of(7)suggests the e!ect of numerical dispersion on discrete ABC performance can besuppressed.If,instead of the wavespeed c,one uses in the discrete boundary condition(secondequation in(4))the group velocity(not the phase velocity as erroneously proposed in Reference[13])of discrete waves propagating in the "03direction,c (03,kh, )"cos(kh/2)((9)it follows that R (03,kh, )"0,and then R is recovered in the discrete problem(third curve in Figure2at N "10)for all other angles of incidence.Figure3is for N "30.Note that while R is recovered by using the modi"ed ABC,the uncorrected discrete ABC is still inaccurate for discrete waves exiting the half-space near normal incidence even for a highly resolved computa-tion.The approach used above can be straightforwardly applied to other discrete local ABCs in order to determine their actual performance in a computation and correct their implementation to account for the phase error introduced by the interior scheme.For example,one can suppress the e!ect of numerical discretization in the M th-order Higdon ABC(a composition of one-way 486P.G.PETROPOULOSCopyright 2000John Wiley&Sons,Ltd.Int.J.Numer.Model.2000;13:483}498Figure parison of the continuous and discrete re #ection coe $cients of theB Higdon operator at N"10for 13) )903.Figure 3.Same as Figure 2for N"30;the "rst and third curves overlap.wave equations),B +"+ O**t #c O **x ,M *2(10)487DISPERSION AND ABCs Copyright 2000John Wiley &Sons,Ltd.Int .J .Numer .Model .2000;13:483}498where O"cos O are the cosines of the angles of perfect absorption for plane waves incident at an angle O on the boundary at x"0as they exit the computational ing the interior scheme's group velocity c E( O,kh, )instead of c in(10)will result in the analytical re#ection coe$cients computed in Reference[10]to be observed in the discrete setting.In the remainder of the paper we will use the above approach to analyse one member of a representative class of local ABCs in cylindrical co-ordinates.As the approach hinges on the availability of the numerical dispersion relation of the interior scheme,we must"rst determine this relation for the discrete wave equation in that co-ordinate system.3.MODEL EQUATIONS IN CYLINDRICAL CO-ORDINATESIn the typical scattering problem a TM-polarized plane wave illuminates an impenetrable circular obstacle of radius " embedded in the computational domain in which the permitivity and permeability is and ,respectively.The boundary condition on the obstacle is E X"E X#E X"0and the ABC is applied to E X on* (at " ).Maxwell's equations for the electromagnetic"eld components(E X,H M,H()are*E X*t"1** ( H()!*H M**H M*t"!1*E X* (11)*H(*t"*E X*The"elds are divergence free,i.e.*E X/*z"0,which is automatically satis"ed,and M()H"0, where M(is the gradient in polar co-ordinates.Eliminating the magnetic"elds from(11)one obtains the scalar wave equation for E X,* E X*t !c 1 ** *E X* #1 * E X* "0(12) where c"1/( is the speed of light in the dielectric.Away from the obstacle,and provided that the dispersion relation k" /c holds,Equation(12)has the time-harmonic solutionsE X( , ,t)"K \+A K H K(k )#B K H K(k ),e K(\ S R,(13)where A K,B K are complex-valued constants,m3(!R,R)is an integer and HK(k )is the Hankel function of the"rst(second)-kind for outgoing(incoming)waves.The Bayliss}Turkel local ABC[2]is employed herein as our model condition in cylindrical co-ordinates.It is a one-dimensional operator,independent of the angular variables,and in 488P.G.PETROPOULOSCopyright 2000John Wiley&Sons,Ltd.Int.J.Numer.Model.2000;13:483}498a numerical simulation is applied to the scattered "eld E X "E X !E X at "B +[E X ]M M "+ O **t #c ** #c 2 (4q !3) E X "M M "0,M *1(14)The analytical re #ection coe $cient of the ABC is obtained by applying (14)to the m th cylindrical mode of the time-harmonic scattered "eld,including the re #ection generated by the ABC,E X "+H K (k )#R +H K(k ),e \ S R (15)and solving for R +at ".We obtain R +(m ,k )"!B +[H K (k )e \ S R ]B +[H K (k )e \ S R ] M M (16)and note that the re #ection R +does not depend explicitly on since "ck .In Section 6,we derive the numerical re #ection coe $cient induced by using the discrete analog of (14),and we compare that coe $cient to (16).4.DISCRETIZATION OF MODEL EQUATIONSA cylindrical grid is placed on the domain of interest and the mesh points l " #l h ,where h "( ! )/N 0and 0)l )N 0,and H "j ,where "2 /N (and 0)j )N (,are de-"ned.Time is discretized as t L "n t ,n *0.The dependent variables on the grid are staggered.The time derivative of E X is centred at t "(n #1/2) t ,while those of H M and H (are centred at t "n t .In this fashion,centred di !erences across one timestep result in second-order accuracy in ing cell-and edge-centred di !erences for */* and */* on the cylindrical stencil,a second-order-accurate discretization of (11)isE L> X (l # ,j # )"E L X (l # ,j # )# t (l #)h +(l #1)H L> ((l #1,j # ),!l H L> ((l ,j # )!H L> M (l # ,j #1)!H L> M (l # ,j )H L> M (l # ,j )"H L \ M (l # ,j )# t (l #)h +E L X (l # ,j ! )!E L X (l # ,j # ),(17)H L> ((l ,j # )"H L \ ((l ,j # )# t h +E L X(l # ,j # )!E L X (l ! ,j # ),for 1)l )N 0!1and 0)j )N (!1.To complete the numerical scheme we impose non-homogeneous Dirichlet data on l "0;0)j )N (!1,and the periodicity condition 489DISPERSION AND ABCs Copyright 2000John Wiley &Sons,Ltd.Int .J .Numer .Model .2000;13:483}498Figure 4.Dual mesh cell used to prove )B "0is enforced on the !plane.e.g.,E L X (l ,0)"E L X (l ,N (),0)l )N 0.The scheme is closed with the discretized ABC to obtain udates of the "elds near/on l "N 0;0)l )N (!1.The dependent variables are then identi "ed as the scattered "eld components.To show (17)corresponds to (11),we must prove that the divergence-free properties of the continuous electromagnetic "eld are also enforced on the numerical grid.Clearly,*E X /*z "0is enforced as only z -independent "elds are considered.To show that M ()H "0is enforced we must determine "rst whether the divergence theorem,Z !H (t ))d C "0where C is the surface of the dual mesh cell whose trace on the !plane is depicted in Figure 4,holds for all time if it does so at t "0.Taking into account the z -invariance of the problem we compute**t !H (t ))d C "**t+( l #1/2H M (l # ,j ,t )! l !1/2H M (l ! ,j ,t )) #(H ((l ,j # ,t )!H ((l ,j ! ,t )) ,(18)Next,letting t P 0in (17),i.e.considering the semi-discrete problem,we substitute the time derivatives in (18)in terms of grid values of E X at the four corners of the dual mesh cell and "nd that*!H (t ))d C "0P!H (t ))d C "!H (t "0))d C "0(19)i.e.(17)computes divergence-free "elds.Straightforward manipulation of the (l ,j ,n )integer indices in (17)allows the elimination of the H M and H (grid functions.The results is the second-order-accurate discretization of (12):E L> X (l ,j )"2E L X (l ,j )!E L \ X(l ,j )# M l+( l #1/2[E L X (l #1,j )!E L X (l ,j )]! l !1/2[E L X (l ,j )!E L X (l !1,j )])#h l (E L X(l ,j #1)!2E L X (l ,j )#E L X (l ,j !1)),(20)where M "c t /h is the Courant number in the direction.490P.G.PETROPOULOSCopyright 2000John Wiley &Sons,Ltd.Int .J .Numer .Model .2000;13:483}498We discretize (14)with the box scheme.Each operator is centred at " ,0\ ,t "(n # ) t and,using the di !erences introduced in Section 2,a single member of the product (14)isdiscretized to second-order accuracy**t #c ** #c (4q !3)2 + \R M t #c \M R h #c (4q !3) R M 2 ,M \ (21)The composition of M terms (21),one for each q ,is then applied to E L> X (N 0,j );0)j )N (!1,to complete (17)and cycle to the next timestep.5.NUMERICAL DISPERSION ANALYSIS OF DISCRETE MODEL EQUATIONS To determine the complex numerical frequency on the cylindrical grid we apply (20)to an outgoing cylindrical waveE L X (l ,j )"H K (k l )e KH (\ S L R ,(22)where k is the real wavenumber.Employing the de "nitions kh "2 /N N , "k l ,h / l "2 /( N M ),and noting that H K ( )does not exhibit zeros on the real axis,we obtain the numerical dispersion relationsin t 2" M 12!14 H K ( #2 /N M )#H K ( !2 /N M )H K( )# N M H K ( #2 /N M )!H K ( !2 /N M )H K ( ) #N ( N M sin m N ( .(23)It is easy to determine that P in the limit of in "nite resolution.The exact dispersion relation is the real function t /2" M /N M ;any non-zero imaginary part of is a spurious spatial e !ect.We computed the relative phase error E ("" ! "/ as a function of the local resolution in the direction at "2.5,N ("720and M "0.25,for the m "0,3,6cylindrical modes.It was found that unresolved waves in the -direction are una !ectedby more resolution in the-direction,and that in order to incur (1per cent phase error for the "rst six cylindrical modes one must have N M +100at "2.5.It follows that the highly compressed grid near the obstacle will then dictate a very small timestep.Figure 5shows the absolute error,E ("" ! ",and the spurious imaginary part of ,for the symmetric (m "0)mode on the grid.Similar information is given in Figure 6for the m "7mode.We see that for m '0the phase error exhibits a minimum at some when N M is "xed.Therefore,there is an optimum distance from the origin as far as the phase error is concerned.As increases from the value at the minimum the phase error increases since the grid expands and the Hankel functions become more oscillatory,while as decreases the phase error increases again as now the Hankel functions vary rapidly due to the co-ordinate singularity at the origin being approached.491DISPERSION AND ABCs Copyright 2000John Wiley &Sons,Ltd.Int .J .Numer .Model .2000;13:483}498Figure5.Phase error vs for the symmetric mode m"0:(a)E("" ! ";and(b)spurious Im( t/2).6.NUMERICAL REFLECTION FROM MODEL LOCAL ABCsTo"nd the re#ection coe$cient of(14)on the grid we applyB +"+O \R M t#c \M R h#c(4q!3) R M2 ,0\(24)492P.G.PETROPOULOSCopyright 2000John Wiley&Sons,Ltd.Int.J.Numer.Model.2000;13:483}498Figure6.Phase error vs for the mode m"7:(a)E(toE L X(l,j)"H K(k l)#R +H K(k l)e KH (\ S L R(25)(" )and obtainat l" ,R + m,k ,0, t2 "!B +[H K(k l)e\ S L> R]B +[H K(k l)e\ S l" ,0.(26) Even though(24)is a one-dimensional operator,we note R +implicitly depends on N(.Copyright 2000John Wiley&Sons,Ltd.Int.J.Numer.Model.2000;13:483}498Figure7.Error introduced into R by discretization for m"0.Figure8.Same as8for m"1.For M"1,Figures7and8show the relative error"R +(m,k , t/2)! R +(m,k )"/"R +(m,k )"as a function of the electrical distance k to* ,for the cylindrical modes m"0,1.For reference,Figure9shows"R +(m,k )"for the same modes.The ABC works well when the assumptions used in its derivation are satis"ed in the numerical setting,i.e.lowCopyright 2000John Wiley&Sons,Ltd.Int.J.Numer.Model.2000;13:483}498Figure9.Magnitude of B re#ection coe$cient.Figure10.Error introduced into R by discretization at k "2.5.m and large k .In this regime,we see the discretization introduces additional re#ection that is strongly dependent on the local resolution N M(which is the number of points per wavelength at the particular k ).For the most favourable case,m"0,it is only for well-resolved waves (N N'200)that the ABC is acceptable.Copyright 2000John Wiley&Sons,Ltd.Int.J.Numer.Model.2000;13:483}498Figure11.Same as11for k "5.Figure12.Magnitude of B re#ection coe$cient.Figures10and11show the relative error as a function of the cylindrical mode index and resolution at various distances to the absorbing boundary,while Figure12shows the continuous re#ection coe$cient as a reference.We see there that it is only for well-resolved waves at k "5 that the discretization adds on the average about10per cent re#ection in addition to the) 10per cent already exhibited by R +(m,k )for m)6.Copyright 2000John Wiley&Sons,Ltd.Int.J.Numer.Model.2000;13:483}4987.SUMMARY AND DISCUSSIONUsing a model problem in rectangular co-ordinates to motivate the work herein we have studied the re#ection coe$cient of discrete local ABCs in cylindrical co-ordinates.This was achieved by using the numerical frequency,obtained by applying the discrete wave equation to an outgoing cylindrical mode sampled on the cylindrical grid.We found that the numerical discretization limits the usefulness of the analytical re#ection coe$cients as predictors of ABC performance. However,for the model problem in rectangular co-ordinates we showed how a simple modi"ca-tion of the discrete ABC operator restores the usefulness of the continuous re#ection coe$cient as a predictor of ABC performance.The issue dealt with in the present paper is pivotal for predictions of performance of exact ABC procedures.Those exact ABCs are also derived by considering analytical rather than numerical waves to be incident on the boundary where they are imposed.It is interesting to note that the left hand side of all existing exact ABCs is a one-way wave equation appropriate for the underlying co-ordinate system;it is the B Higdon-operator in rectangular co-ordinates,the B Bayliss} Turkel operator in polar co-ordinates,and the B Bayliss}Turkel operator in spherical co-ordinates.We expect that the numerical dispersion of the interior scheme will limit the perfor-mance of such exact conditions,and we are presently extending the procedure developed herein to study the actual error.DISCLAIMERThe ernment is authorized to reproduce and distribute reprints for governmental purposes notwithstanding any copyright notation thereon.The views and conclusions contained herein are those of the author and should not be interpreted as necessarily representing the o$cial policies or endorsements,either expressed or implied,of the Air Force O$ce of Scienti"c Research or the ernment.REFERENCES1.Engquist B,Majda A.Absorbing boundary conditions for the numerical simulation of waves.Mathematics ofComputation1977;31:629}651.2.Bayliss A,Turkel E.Radiation boundary conditions for wave-like munications in Pure and AppliedMathematics1980;XXXIII:707}725.3.Givoli D.Non-re#ecting boundary conditions.Journal of Computational Physics1991;94:1}29.4.Tsynkov SV.Numerical solution of problems on unbounded domains.Applied Numerical Mathematics1998;27:465}532.5.Grote MJ,Keller JB.Nonre#ecting boundary conditions for Maxwell's equations.Journal of Computational Physics1998;139:327}342.6.Sofronov IL.Condition of absolute transparency on the sphere for the three-dimensional wave equation.DokladyMathematics1993;46:397}401.7.Zhao L,Cangellaris AC.GT-PML:generalized theory of perfectly matched layers and its application to there#ectionless truncation of"nite-di!erence time-domain grids.IEEE¹ransactions on Microwave¹heory¹echnology 1996;44:2555}2563.8.Petropoulos PG.Re#ectionless sponge layers as absorbing boundary conditions for the numerical solution ofmaxwell's equations in rectangular,cylindrical and spherical co-ordinates.SIAM Journal of Applied Mathematics 2000;60:1037}1058.Copyright 2000John Wiley&Sons,Ltd.Int.J.Numer.Model.2000;13:483}4989.Petropoulos PG.On the termination of the perfectly matched layer with local absorbing boundary conditions.Journal of Computational Physics1998;143:665}673.10.Higdon RL.Radiation boundary conditions for elastic wave propagation.SIAM Journal of Numerical Analysis1990;27:831}870.11.Zhao L,Cangellaris AC.The generalized theory of perfectly matched layers(GT-PML):numerical re#ection analysisand optimization.In Proceedings of the IEEE Antennas and Propagation Society International Symposium,1997;III:1896}1899.12.Strikwerda JC.Finite Di+erence Schemes and Partial Di+erential Equations.Wadsworth and Brooks/Cole:BelmontCA,1989.13.Ta#ove putational Electrodynamics:¹he Finite-Di+erence¹ime-Domain Method.Artech House:Boston,MA,1995.Copyright 2000John Wiley&Sons,Ltd.Int.J.Numer.Model.2000;13:483}498。
线上音乐会评论英语作文

线上音乐会评论英语作文全文共3篇示例,供读者参考篇1Online Music Gigs: The New Norm?It was just another Friday night during lockdown when my notifications went crazy with posts from friends sharing the link for an upcoming "Living Room Concert" by one of my favorite indie bands. Like many other events, their scheduled tour had been cancelled due to the pandemic, so they decided to go digital and live stream a full concert set from the lead singer's apartment.At first, I was pretty skeptical about the whole idea. How could a online concert even come close to replicating that incredible energy and atmosphere you get from a real live show? There's just something magic about being physically present in the same space as the musicians, feeling the rhythms pulsating through your body, and feeding off the collective exhilaration of the crowd. Not to mention the absence of mosh pits, concession stands, and overpriced band merch.But with not much else to do that night, I rallied some friends on Messenger and we agreed to virtually "attend" together. We each pitched in a few bucks to purchase our access passes, which I suppose was the new modern equivalent of buying concert tickets. As showtime approached, I cracked open a beer, connected my laptop to the TV, and prepared to see how this trendy new experience would unfold.When the stream kicked off, I was instantly blown away by the production quality. The band had clearly invested a ton of effort into ensuring this would be a performance to remember despite the limitations. There were multiplemultiple camera angles that offered some really cool shots, cutting between tight close-ups of the singers/instrumentalists and wider vantage points that revealed the basement setting. The lighting was on point too, with moody atmospheric bulbs surrounding them in their makeshift "home studio."Most importantly though, the audio mix was absolutely pristine. I could hear every single lick, riff, and lyrical line with amazing clarity and separation of the instruments. No fighting with crowd chatter or less-than-stellar venue acoustics. Just pure, crisp sound beaming straight into my living room speakers.As for the performance itself, it was extremely energetic and passionate right from the start. The musicians clearly fed off each other's presence even without a roaring audience in front of them. Song after song, they brought the heat and played their hearts out as if it was a massive arena show. In between tracks, they even told some fun backstories and did a little informal banter and Q&A by keeping an eye on the scrolling live chat.While the online concert was a very different experience from their normally raucous live sets, it managed to recreate a really cool intimate lounge vibe. It sort of felt like you were a guest getting treated to this exclusive private showcase in their basement. I could have done without some of the awkward silences between songs where they'd scroll through the comments, but overall it was impressive how they were able to improvise and adapt so seamlessly to this unique format.The wildest moment came halfway through the stream, when their drummer suddenly kicked into one of their biggest hit singles that everyone was waiting for. Within seconds, my friends and I could see comments exploding from fans all around the world who were just as psyched for this slapper of a track. Reading all those streams of emojis and hype messages inreal-time, a powerful sense of global togetherness andcommunity started to spark between everyone tuning in simultaneously. Even separated in our individual homes by both distances and screens, we were all sharing this electrifying moment at the exact same instant—and it felt absolutely unifying.By the time they finally reached the climactic crescendo of that song, I couldn't resist leaping off the couch, raising my drink in the air, and shamelessly singing/shouting along at the top of my lungs. My roommates probably thought I was losing it, but the truth is I was as alive and present as I'd felt in months. For those few euphoric minutes, the isolation and mental toll of the pandemic got left behind. My mind was completely untamed, unleashed, and in the moment.Of course, nothing can ever quite replicate the majesty of an in-person show. There were certainly things I missed that night—like the sound reverberating through my entire body instead of just my audio setup, or that indescribable magic of communally bearing witness alongside hundreds of others. Not to mention the shenanigans of concert rituals, whether it's trying to snag one of the guitar picks or fighting your way to the front row.At the end of the day though, this online concert accomplished something that I had written off as impossible—it temporarily freed me from my confined pandemic existence and filled me with an authentically cathartic euphoria that music alone can provide. For those couple of hours, I was emotionally unleashed and spiritually elevated in a way I hadn't felt in forever. It served as a poignant reminder that music is so much deeper than simply being entertainment. It's a universal language that transcends physical barriers and connects people across the globe through deeply human expression and shared experiences.The stream may have cut off after their encore, but the reverberations of that night continued to echo through me for awhile. As I reflected, it dawned on me that perhaps these online concerts shouldn't be regarded as a pandemic novelty, but rather an enduring complement to live shows moving forward. Think about the possibilities—artists could seamlessly broadcast their shows to fans anywhere on the planet in real-time. No more selling out or restricting tickets due to venue capacities. No more touring costs or carbon emissions from equipment transportation. And fans with disabilities or financial restrictions could still get intimate access without mobility or budget constraints.Much like how movie theatres and VOD/streaming services have learned to co-exist, maybe the future holds a similar symbiotic relationship between these dual concert mediums and experience options. Because if this pandemic life taught us anything, it's that music is far too essential to the human spirit to deprive us of it through any situation. And while we should absolutely cherish and support the return of live music with every fibre of our beings, perhaps we shouldn't dismiss the unique magic and accessibility that even a "virtual" concert can provide either.One thing's for sure—after that unforgettable night streaming from my living room, I'll never look at online gigs the same way again. If artists continue putting in that level of care and quality, it has the potential to pioneer a whole new way of absorbing and appreciating music on a larger scale than we ever imagined possible. If the past year was defined by universal struggles and heartaches, maybe the next era could be defined by a new frontier of communion through the uniting power of song. And I'll be ready—controllers charged, brews cooled, singing voices warmed up.篇2Virtual Vibes: An Online Music ExtravaganzaAs a music lover and avid concert-goer, the pandemic put a major damper on my live music experiences. With venues shut down and tours cancelled, I thought my days of rocking out were over. That is, until online concerts became the new norm. While nothing can truly replicate the electric atmosphere of a real show, these virtual events have given music fans like me amuch-needed fix during these strange times.Last weekend, I tuned in for Cobra Remix's "Quarantune" online festival. As someone who typically prefers smaller, intimate gigs, I was a bit skeptical about how an onlinemega-show of this scale would play out. Boy, was I in for a welcome surprise!Right from the start, I was blown away by the high production value. The festival had clearly invested a pretty penny into their virtual set-up, with top-notch sound quality, múltiple high-def camera angles, and seamless audio-video syncing. It was like I had a front-row seat to the whole shebang from the comfort of my bedroom!The line-up was also hugely impressive, with a wonderfully eclectic mix of musical acts. They ran the gamut from mainstream pop titans to underground indie gems I'd never even heard of before. While part of me missed the serendipitousvibe of stumbling across new artists at a physical festival, I loved how the online format allowed me to easily hop between "stages" and sample acts I may have overlooked otherwise.Things really kicked into high gear once the headliners started playing in the evening. Up first was alt-rock royalty The Neon Walls, who absolutely blew the (virtual) roof off with a scorching set of fan favorites and surprise deep cuts. Despite being worlds away, beamed straight to my laptop screen, their raw energy and passion translated beautifully. I was pogoing around my bedroom like a crazed teen!The night continued with a series of equally iconic performers, from pop diva Rina Del Mar's shimmering dance explosion to hip-hop legend Verb-X's smoldering rhymes. Each act brought their A-game, rising to the uniquechallenge of making an online show as electrifying as the real deal. The roaring (virtual) crowds and non-stop influx of fan comments definitely helped fuel that adrenaline-pumping ambiance.Of course, even the fanciest technology is bound to hit a few snags here and there. A couple of the artists seemed to struggle with their audio mixing at times, resulting in slightly muddied vocals. There were also a handful of brief video hiccups that frustrated some fans in the comments. But overall, the premiumstreaming quality ensured these were mere minor blips in what was otherwise a stunningly seamless experience.Speaking of the comments, that's where the virtual shows have created an entirely new dimension to the live music experience. Rather than just passively observing, I found myself actively engaging with the worldwide community of fans throughout the performances. We'd cheer each other on through dazzling guitar solos, bond over shared favorite tracks, and trade wildly different perspectives from our respective home studios, apartments, and basement setups. Seeing endless viewers from across the globe spam the comment threads with the heart-eye emoji in real-time was such a beautiful reminder that music remains an unstoppable, universal force for human connection.At one point, I even had the chance to briefly hop on my webcam and peer over the virtual shoulders of a group of friends from Sweden who were co-streaming the event. Despite being separated by an entire ocean, we managed to have a funcross-continental singalong and bond over our mutual love for the bands on the bill. Connections like these have made every virtual show feel like its own tight-knit global gathering.In the end, what struck me most about "Quarantune" was its ability to tap into the pure, unbridled euphoria of live music in such unprecedented circumstances. Sure, there were no sweaty mosh pits, overflowing beer lines, or 3 AM parking lot DJ sets to chase down. But in that singular, electrifying moment when the beats dropped and I surrendered to the music, none of that seemed to matter. My room became a sacred space for sublime sonic transportation.As the final chords reverberated through my headphones, I'd never felt such immense gratitude for the incredible technological feats enabling not just this event, but live music's resilience at large. In a time of anxiety, uncertainty, and social confinement, moments like these are powerful reminders of the indomitable human spirit and our stunning capacity for transcendent shared experiences.While I eagerly await the return of in-person showspost-pandemic, "Quarantune" proved that online concerts have truly come into their own as a spectacular art form worth celebrating. They've set a bold new precedent for how we can consume and commune around music in our digital age. Even as life gradually returns to normal, I have a feeling these uniquely intimate yet impossibly vast virtual gatherings will remain corepieces of the live music landscape for years to come. If they continue delivering unforgettable evenings like this one, count me in as a loyal attendee.篇3An Electrifying Online Music ExperienceAs the COVID-19 pandemic raged on, forcing cancellations of live events worldwide, musicians had to get creative to connect with fans. One brilliant solution was to take concerts online through livestreaming platforms. While nothing truly compares to the energy and atmosphere of a live in-person show, these online music events provided a lifeline for artists and audiences alike during an incredibly difficult time.Last weekend, I had the chance to attend one of these virtual concerts, and I was absolutely blown away by the experience. The headliner was one of my favorite indie rock bands, The Neon Drifters. Even though I was sitting in my living room staring at a laptop screen, I felt utterly transported by their high-energy performance.From the second the livestream began, I could feel the electric anticipation buzzing through the online crowd. A constant stream of comments flooded the chatbox as thousandsof diehard fans from across the globe checked in. There were hellos and song requests from Tokyo, Paris, Rio de Janeiro, and seemingly every pocket of the world. Despite our physical separations, we were all united through our love of music in that virtual space.When the first opening chords of the set blasted through my speakers, an instantaneous wave of goosebumps rippled across my skin. Seeing the bandmates take their places on stage triggered a sensory rush of excitement that I never expected to experience through a screen. Their iconic frontman, Jake, looked right into the camera lens with those signature brooding eyes, and I swear he stared straight into my soul. "What's up, world? Are you ready to rock?" His rallying cry elicited a deafening roar of cheers in the comments.For the next ninety minutes, I was utterly mesmerized watching The Neon Drifters tear through their setlist of hits and deep cuts. Sure, the video quality was nowhere near 4K resolution and the audio was a bit compressed through my laptop speakers. But you know what? It didn't matter one single bit. The furious energy from the band transported me to the front row of an arena show. I was banging my head, singingalong at the top of my lungs, and furiously smashing the applause emoji in the chatbox between every tune.At one point during the fast and furious rendition of their signature anthem "Stardust Soul," the wailing guitars and crashing cymbals reached such a transcendent fever pitch that I'm pretty sure my neighbors started pounding on my walls. But I couldn't find a single care in the entire universe to give. I was absolutely lost in the thrashing rhythms, captivating stage presence, and raw musical passion pouring through my screen.While the whole show felt like an adrenaline-soaked religious experience, my most treasured moment was when the band launched into "Kaleidoscope Heart," a lesser-known deep cut from early in their catalogue. It's one of those rare gems that has carried profound emotional resonance for me through various profound life experiences and transitions. As Jake's impassioned vocals flooded my room, the unmistakable sting of happy tears welled up in my eyes. I realized in that moment how much I'd truly missed that electrifying connection between artist and audience in the absence of live music.Although I was sitting alone, I felt a profound sense of communion with my fellow fans across the globe. We were all fervently belting along with the lyrics:"When the kaleidoscope's turningAnd your world is realigningDon't let the storm clouds keep youFrom the rainbow on the other side"In the comments, there was an overwhelming outpouring of hearts, flames, and teary-eyed emojis as others expressed feeling that same profoundly human bond. Despite our disparate physical locations spreading across thousands of miles, we were spiritually united in that instant by the awesome healing power of music to uplift and inspire.Once the stream finally concluded after a blisteringthree-song encore, I had to sit in awe for several long moments trying to process the depths of emotion I had just experienced. While there's certainly an argument that online concerts lack a certain sort of larger-than-life grandeur and intimacy you can only get from a real show, there's also something incredibly special and even democratizing about this format.After all, how many fans from Nairobi or Bangkok could realistically afford the travel costs and tickets to see their favorite band perform on tour? With online shows, anyone anywhere can basically get a front-row seat and that same catharticlife-affirming experience. Sharing a powerful artistic performance through a virtual portal still facilitates those same profound human connections that make live music so magical and transcendent.In the weeks since attending The Neon Drifters' show, I've become absolutely ravenous to experience more of these online concerts. I've already got my virtual tickets lined up for upcoming streams from some of my other beloved artists. While I desperately hope we can return to conventional touring as soon as safely possible, I have a newfound appreciation for this innovative format.At the end of the day, the energy exchange between musicians pouring their heart out on stage and moved listeners is what really matters, pandemic or not. As long as that sacred bond between artist and audience can still spark through our digital connections, I'll be the first to log on and let the awesome power of live music wash over me. From the comfort of my living room, I've discovered a newfound way to experience some incandescent magic when my favorite bands have the whole world tuning in and turning up.。