点阵LED外文文献+翻译
LED点阵书写显示屏

LED点阵书写显示屏摘要本设计运用了基于 Nios II 嵌入式处理器的 SOPC 技术。
系统以 ALTERA 公司的 Cyclone II 系列 FPGA 为数字平台,将微处理器、Avalon 总线、LED 点阵扫描控制器、存储器和人机接口控制器等硬件设备集中在一片 FPGA 上,利用片内硬件来实现 LED 点阵的带地址扫描,降低系统总功耗和简化 CPU 编程的同时,提高了系统的精确度、稳定性和抗干扰性能。
关键词:SOPC FPGA 带地址扫描AbstractThis design using the Nios II based on embedded processor SOPC technology.ALTERA system to the company for the Cyclone II FPGA digital platform、 series、Will microprocessor、 Avalon bus of LED dot matrix scanning controller、memory and human-computer interface controller hardware device focused on such a FPGA,Using the piece of hardware to achieve inside of LED dot matrix with address scanning, reduce the total power of the programming and simplify the CPU, improve the precision and stability of the system and the anti-jamming performance.Keyword: SOPC FPGA Address scanning1目录1 引言 (3)2 系统方案 (3)2.1主控器选择方案论证 (3)2.2点阵驱动方案论证 (3)3 理论分析与计算 (4)3.1 光笔选取与参数设计 (5)3.2 LED点阵屏驱动参数设计........................................ (5)3.3 屏亮自动调节设计 (6)3.4 超时关显示节电设计 (6)4 系统电路设计 (7)4.1 系统工作原理 (7)4.2 系统工作时序 (7)5 系统程序设计 (8)5.1 系统流程概述 (8)5.2系统总流程图 (8)6 系统测试与结果 (9)7 结论 (9)参考文献 (10)附录: (10)附1:电路原理图 (10)附2:扫描电路硬件描述 (11)附2:软核NIOS II程序 (17)附4:完整的测试结果 (47)21 引言LED点阵显示屏被用到很多领域,随着电子技术的发展,LED点阵书写显示屏的广泛应用是一种趋势。
关于LED的外文文献和中文译文

多个LED发光装置的新型采集系统作为光源的一种,发光二极管(LED)有很多优点。
LED集成度更高,颜色种类多,使用寿命更长,而且工作电压较低。
但是,它仍有一个非常大的缺陷:一只LED的光照强度还是比较低。
这个缺点导致显示屏上的光通量不会很高。
但是无论如何,LED还是以其出色的性能在低电压装置中普遍应用。
因此,利用此系统采集多个LED的光,集成为更高强度的照明装置。
本设计提出三种采集系统,来实现增强光强的功能。
效率最好的一种采集系统可以达到96%。
同时,还分析了本系统的制造误差以及预算。
1 简介利用传统的光源来设计一个便携式探照灯,尺寸和能耗会很大。
而利用LED 来设计将会避免这些问题。
LED有很多优点:节能、体积较小、使用寿命长(约100,103小时)等,尤其是LED的光很适合环境工作。
Carel Zeiss和Philips打算用LED光源设计两种便携式探照灯。
尽管LED有诸多优点,可以让他们设计出的探照灯更加便携和小巧,但是由于光学元件的转换效率问题,导致系统有很多困难。
解决这个困难将是本文研究的重点。
通常,用一种合成非线性集中器(CPC)来减小分散度。
但是,这种传统的CPC采集效率仅为72%,必须要改善采集效率来提高光的利用率。
本文中将解决分散度和采集效率两个问题。
为实现这个目标,设计了三种不同的采集系统,以提高效率,下面逐一介绍。
2 仿真部分利用光学仿真软件和标签查找模块(BRO),来设计并分析采集系统的性能。
LED光源部分来自Osram-Opical半导体。
远程LED光源是一种Lambertian模式,LED的规格见表1。
在采集系统的底部有四个LED。
系统各个LED之间的位置关系如图1。
通光部分为2.1×2.1mm2,孔径3.26mm。
LED阵列对称的分布于系统的底部。
采集系统的第一个光学元件为均质器。
这个均质器的受光角度是12.5°。
因此,这个系统就是要把LED的受光角度的范围控制在±60°到±12.5°之间。
led点阵显示原理

led点阵显示原理LED点阵显示原理。
LED点阵是一种常见的显示设备,它由许多小的LED灯组成,可以显示数字、字母、符号等信息。
它在各种电子产品中广泛应用,如电子钟、电子表、电子游戏机等。
那么,LED点阵显示的原理是什么呢?首先,我们来了解一下LED的基本原理。
LED全称为“Light Emitting Diode”,即发光二极管。
它是一种半导体器件,具有正向导通特性,当正向电压施加到LED两端时,电子和空穴在PN结复合,释放出能量,产生光。
LED点阵就是将许多这样的LED灯排列在一起,通过控制不同的LED灯点亮或熄灭,从而显示出所需的图形或文字。
LED点阵的显示原理可以分为两部分,控制和驱动。
首先是控制部分。
LED点阵的控制通常采用行列扫描的方式。
即将LED按行和列的方式排列,通过控制各行和列的电平信号来控制LED的点亮和熄灭。
控制部分通常由微控制器或者专门的LED控制芯片来实现,通过发送相应的控制信号来控制LED点阵的显示。
其次是驱动部分。
LED点阵的驱动通常采用常流驱动的方式。
LED是一种电流驱动的器件,为了保证LED的亮度稳定,需要对LED施加恒定的电流。
因此,在LED点阵的驱动电路中通常会加入恒流源,以保证LED的亮度稳定。
此外,还需要考虑LED的正向电压和功率等参数,来设计合适的驱动电路。
除了控制和驱动,LED点阵的显示原理还涉及到LED的亮度和色彩控制。
LED的亮度可以通过控制LED的通电时间来实现,即通过调节LED的占空比来控制LED的亮度。
而LED的色彩控制则需要使用不同颜色的LED,并通过控制不同颜色LED的点亮组合来实现。
总的来说,LED点阵显示的原理是通过控制和驱动LED点阵中的各个LED灯,来实现所需的图形或文字显示。
控制部分通过行列扫描的方式来控制LED的点亮和熄灭,驱动部分采用常流驱动的方式来保证LED的亮度稳定,同时还需要考虑LED的亮度和色彩控制。
LED点阵显示原理的了解对于电子产品的设计和应用具有重要意义,希望本文能够帮助大家更好地理解LED点阵显示的原理和应用。
LED点阵的外文翻译---纳米结构InGaN发光Diodesfor固态照明

(要求翻译与毕业设计(论文)相关的外文文献两篇,且3000单词以上/ 篇,将译文附在原文之后)第一篇:[ 所译外文资料:①作者:Taeil Jung②书名(或论文题目):Nano-structured InGaN Light-Emitting Diodesfor Solid-State Lighting③出版社(或刊物名称或可获得地址):All Rights Reserved.④出版时间(或卷期号):2009.⑤所译起止页码:Nano-structured InGaN Light-Emitting Diodesfor Solid-State LightingSolid-state lighting can potentially reduce the electricity consumption by 25%. It requires high efficiency light-emitting diodes across the visible spectrum. GaN and related materials have direct band gap across the entire visible spectrum and are ideal for future solid-state lighting applications. However, materials defects, polarization charges, and total internal reflection have thus far limited the efficiencies of InGaN LEDs, in particular InGaN LEDs in the green/yellow wavelength range, which are critical in achieving highly efficient LED luminaries with an excellent color-rendering indexIn this Thesis, we have developed and demonstrated that novel in situ nanostructured GaN processes in MOCVD are effective in improving the efficiencies of InGaN LEDs. InGaN LEDs grown on quasi-planar semi-polar GaN templates were proven to exhibit three times higher internal quantum efficiencies and negligible quantum confined Stark effect using selective area epitaxy. InGaN LEDs grown on nanostructured semi-polar GaN templates are also effective to improve the internal quantum efficiency by 31%. The same in situ processes are also effective in reducing the defect density by an order of magnitude and increasing the photon extraction efficiency as a factor of two.The in situ processes include in situ silane treatment and high temperature overgrowth. Both processes require only standard MOCVD tools and hence are cost effective and suitable for mass-production. In situ silane treatment treatsc-plane GaN samples with silane under ammonia environment, generating nano-scale truncated cone structures with up to 200 nm scale. These truncated cone structures can be subsequently transformed into pyramidal nanostructures comprising of only (10-11) and (11-22) semipolar planes using high temperature overgrowth. These processes were applied to both InGaN active region and the LED surface to improve the internal quantum efficiency and the photon extraction efficiency, respectively. Extensive materials, device, and optical characterizations have been carried out in this research.1.1 Gallium Nitride Materials for Optoelectronic ApplicationsGallium nitride based materials, including GaN, AlN, InN, and their alloys, are excellent candidates for short-wavelength optoelectronic applications. Their direct bandgaps extend from ultraviolet to near-infrared. In addition, they exhibit high mechanical and thermal stabilities compared to other III-Vsemi-conductors, making them especially suitable for high-power andhigh-temperature operations. In recent years, breakthroughs in p-type doping and defect reduction have led to the commercialization of GaN based laser diodes, light-emitting diodes (LEDs), high electron mobility transistors (HEMT) and hydrogen detectors. Despite these advances, many technological challenges such as green gap and substrate growths still remain.Perhaps one of the most important applications for GaN based materials is solidstate lighting (SSL). Worldwide, lighting constitutes 20% of electricity consumption while its efficiency is much lower than 25%. In contrast, efficiency of space heating has exceeded 90%. To this end, the development of highly efficient and reliable LEDs for solid-state lighting has been very active in both industry and academia in the past few years. It is projected by the US Department of Energy that by 2015, if successful, solidstate lighting can reduce the overall electricity consumption by 25%.Unlike GaAs and InP based semi-conductors, GaN based materials have suffered from a high density of defects due to very limited availability of lattice-matched GaN substrates. Up to now, most GaN based optoelectronic devices have been fabricated using hetero-epitaxy on foreign substrates such as sapphire (Al2O3), silicon carbide (SiC), and aluminum nitride (AlN), and in a very small percentage on silicon. Because of large lattice mismatch, GaN grown on these substrates often exhibits a high density of threading dislocations, typically on the order of 108 – 1010 /cm2. These defects are still one of the major limiting factors for the performance of GaN based optoelectronic devices, acting as non-radiative recombination and scattering centers. Achievement of lower defect density would also improve device reliability, resulting in a longer lifetime. Various defect reduction approaches, such as epitaxial lateral over-growth (ELOG), have been demonstrated and some of the details will be discussed in Chap.1.3.1. As part of this thesis, we have explored a novel approach to using nano-structured GaN to effectively lower the threading dislocation density.Among various epitaxial techniques that have been developed for GaN based materials, metal-organic chemical vapor deposition (MOCVD) is the leading technology. The typical growth temperature for GaN materials is around 1000 to 1200°C. This high growth temperature is necessary to improve the crystal quality and is a result of low cracking efficiency of the nitrogen source, ammonia (NH3), at a low temperature. In Chapter 2, I will summarize my contributions to successfully ramp up an MOCVD tool for the epitaxial growth of GaN LEDs for this research.1.2 InGaN LEDs for Solid-State LightingThe basic component for SSL is a white-light LED. As shown in Figure 1-1, itcan be achieved by mixing various color components, which can be generated either from the direct output of individual LEDs or from color-conversion materials, such as phosphor. To date, commercially available white-light LEDs usually consist of a blue emitter and a yellow phosphor plate. It has been shown that InGaN based blue LEDs could achieve external quantum efficiency in excess of 70% [1, 2]. However, this di-chromatic configuration typically has a poor color rendering index due to the lack of green and red components. The phosphor conversion process also limits the overall luminous efficiency due to energy loss during downconversion. To achieve luminous efficiency in excess of 200 lm/W and a color rendering index (CRI) in excess of 90, which is required for general illumination, a further improvement in blue LED efficiency and the use of tetra-chromatic configuration (blue + green + yellow + red) is necessary [3].* Unfortunately, the efficiency of both InGaN and AlInGaP LEDs decreases significantly in the green-yellow (500 - 580 nm) range. This efficiency gap is also known as “green gap”. Because AlInGaP materials have indirect bandgaps in this wavelength range, to achieve high-efficiency SSL, it is crucial to significantly improve the luminousNote that a trichromatic (e.g. blue + green + red) source cannot achieve a CRI > 90. efficiency of green and yellow InGaN LEDs. In this thesis, we will address these challenges using nano-structured GaN.Figure 1-1. Illustration of various potential white-light LEDs configurations (after Ref. [4]).1.3Limiting Factors for InGaN LEDs EfficiencyTo date, the efficiencies of InGaN LEDs are still limited by materials defects, polarization charges, and photon trapping. In this Section, we will briefly review the state of theart and overview how this research helps address these limitations.1.3.1 Materials DefectsAs mentioned before, the high defect density in GaN based materials grown on foreignsubstrates increases the non-radiative recombination rate and lowers the radiative efficiency. To date, several techniques have been demonstrated to improve the crystal quality and reduce the threading dislocation (TD) density of the GaN layer. Substrate pretreatmentat the growth temperature in an ammonia environment, also known as nitridation [5-7], has been shown to be critical for high quality GaN epilayers. The TD density of a typical GaN layer grown on c-plane sapphire substrate can be reduced to 108/cm2 [8] by employing the combination of a low temperature (LT; 450 - 600 °C)nucleation layer (NL) and a short annealing at the growth temperature to change the phase of the as-grown NL from cubic to hexagonal [9-11]. As will be discussed in Chapter 2, careful optimization of these low temperature growth sequences can significantly alter the subsequent GaN template growth. To this end, a home-made optical in situ monitoring tool (reflectometry) was established and will be discussed extensively in Chapter 2.In addition low temperature buffer growth, epitaxial lateral overgrowth (ELOG) which is a variation of selective area epitaxy (SAE) has been introduced [12, 13] to further lower the TD density by an order of magnitude to below 107/cm2. Variations of ELOG including pendeo- (from the Latin : hang on or suspended from) epitaxy (PE) [14] and multi-step ELOG are also effective to further reduce the TD density. Additional techniques such as TiN nano-porous network [15] and anodic aluminum oxide nano-mask [16] have also been proposed and demonstrated. All these methods, however, require ex situ processing and hence will add complexity and cost to the manufacturing. In this thesis, we will explore and generalize an in situ silane treatment approach to effectively lowering the TD density by an order of magnitude.1.3.2 Polarization ChargesDue to the non-cubic symmetry of GaN materials, compressively-strained active regions in InGaN LEDs exhibit both spontaneous and piezoelectric polarization charges. These polarization charges induce a strong internal electric field (IEF), typically on the order of MV/cm, in the active region, resulting in both efficiency droop at a high injection current density and the decrease of radiative efficiency with an increasing emission wavelength. The IEF can separate electrons from holes and increase electron leakage, resulting in low internal quantum efficiency (IQE) and efficiency droop [17], respectively. The suppression of the IEF, which is expected to increase IQE and the current density at which efficiency droop occurs, can be achieved by reducing the lattice mismatch in hetero-structures or growing them on semi-polar (e.g. {10-11} and {11-22}) and non-polar (e.g. a-plane and m-plane) surfaces. Because indium incorporation is more difficult on non-polar planes than on semi-polar planes, it is more advantageous to fabricate long-wavelength green-yellow LEDs on semi-polar planes to suppress the IEF.At least three approaches to fabricating semi-polar InGaN LEDs have been reported thus far. These include the growth of a GaN epilayer on spinel substrates [18], on bulk GaN substrates [19-27], and on the sidewalls of pyramidal or ridge GaN structures created on planar polar GaN surfaces using SAE [28-35]. GaN grown on spinel substrates have so far exhibited a high density of threading dislocations and stacking faults, thereby compromising the potential improvement of efficiency from the lowering of IEF. The use of bulk semi-polar GaN substrates has demonstrated the advantage of a lower IEF for the enhanced efficiency of green and yellow LEDs [25, 26]. However, limitations such as prohibitively high wafer cost and small substrate size need to be resolved before this approach can become more practical. On the other hand, the SAE technique can create semi-polar planes on polar GaN surfaces.High quality polar GaN films have been fabricated from a variety of substrates including sapphire, 6H-SiC, and bulk GaN by MOCVD. Using growth rate anisotropy and three-dimensional growth, different semi-polar and non-polar GaN planes can be generated on c-plane GaN [13]. In Chapter 3, we will show that high quality InGaN multiple quantum wells (MQWs) which exhibit IQE as large as a factor of three compared to polar MQWs can be grown on pyramidal GaN microstructures. This approach, however, requires ex situ patterning processes and does not easily produce a planar structure for electrical contacts. In this thesis, a new semi-polar LED structure is investigated, which is enabled by a novel epitaxial nanostructure, namely the nanostructured semi-polar (NSSP) GaN, which can be fabricated directly on c-plane GaN but without the issues of the SAE technique mentioned above [36]. NSSP GaN also eliminates the issues of excessive defects for GaN grown on spinel substrates and lowers the cost of using bulk semi-polar GaN substrates. As we will show later, the surface of NSSP GaN consists of two different semi-polar planes: (10-11) and (11-22). Therefore it is expected that InGaN active regions fabricated on NSSP GaN can exhibit a low IEF, and hence much improved IQE.1.3.3 Photon ExtractionAfter photons are generated from the active region in LEDs, they need to escape the device in order to be useful. When light travels from a medium with a higher refractive index to a medium with a lower refractive index, total internal reflection (TIR) occurs at the interface. In InGaN LEDs, photons experiencing TIR at LED surfaces can be re-absorbed by the active region or trapped in the device due to a wave-guiding effect as shown in Figure 1-2. In a simple InGaN LED, only 4% of photons generated from the active region can escape from each device surface. It has been shown that surface textures on LED surfaces can greatly reduce TIR and improve photon extraction efficiency as illustrated in Figure 1-2. To date, many surface texturing techniques such as photonic crystal structures [37] and photo-electrochemical etching of GaN surfaces [38] have been introduced. Notably, the photo-electrochemical etching of nitrogen-terminated GaN surface has been successfully implemented into commercial blue LEDs [2]. However, these approaches all require additional ex situ patterning processes which add significant costs.In this thesis, we investigate an in situ process to fabricate nano-structured GaN surfaces on LEDs which effectively improves the photon extraction efficiency. Figure 1-2. Light traveling within waveguides (left) with a smooth interface and (right) with a rough interface (after [39]).1.4 Organization of the ThesisThe objective of this thesis is to investigate cost-effective nanofabrication techniques that can significantly improve the efficiency of the state-of-the-art InGaN LEDs in both blue and green/yellow ranges for high performance solid-state lighting. The organization of this thesis is as follows.In Chapter 2, a summary of the MOCVD techniques for InGaN LEDs is given. In Chapter 3, we study the dependence of InGaN LED IQE on {10-11} semi-polar planes using SAE. In Chapter 4, fabrication and characterization of novel andcost-effective nano-structured GaN templates will be described. Using in situ silane treatment (ISST) and high temperature overgrowth (HTO), the formation of nano-scale inverted cone structures and nano-structured semi-polar (NSSP) templates has been obtained. In Chapter 5, we study InGaN semi-polar LEDs based on NSSP templates. An improvement of internal quantum efficiency is demonstrated.A green semi-polar InGaN LED grown on a c-plane substrate is also demonstrated. In Chapter 6, current spreading in NSSP InGaN LEDs will be discussed. In Chapter 7, the application of ISST for theimprovement of photon extraction efficiency of an InGaN LED will be discussed. In Chapter 8, we will summarize and make suggestions for future work.2.1 Gallium Nitride GrowthAs mentioned in the Introduction, gallium nitride (GaN) and related alloys are excellent candidates for future solid-state lighting. To date, III-nitride epitaxial growth has been limited by the lack of sufficiently large single crystal substrate for homoepitaxial growth. Therefore, the growth of GaN and related materials has been largely based on hetero-epitaxy using hydride vapor phase epitaxy (HVPE), metal organic chemical vapor deposition (MOCVD), and molecular beam epitaxy (MBE). Among these techniques, MOCVD is the leading technology due to the advantages on material quality, scalability, and cost [40]. The material quality of GaN grown by MOCVD has been excellent owing to its relatively high growth temperature (1000 - 1200°C) [41, 42].To date, various substrate materials including sapphire (Al2O3), silicon carbide (SiC), and silicon have been studied for GaN growth (Table 2-1). Although GaN substrates have been recently introduced in markets through bulk material growth on foreign substrates using HVPE and laser cutting along specific crystal planes, the cost has been prohibitively high. On the other hand, GaN grown on c-plane (0001) sapphire substrate exhibits stable growth over a wide range of growth conditions despite high dislocation density at the interface between thesubstrate and epitaxial layer. In this research, I have helped ramping up an MOCVD system together with Dr. Hongbo Yu. In this Chapter, I will summarize the MOCVD technologies and defect reduction strategies for InGaN light-emitting diodes (LEDs) epitaxy that will be used throughout this Thesis.2.1.1 GaN Growth Using MOCVDDue to a large lattice mismatch between GaN and sapphire, it is important to contain the defects near the GaN/sapphire interface such that the defect density can be minimized in the device region. Such optimization is achieved using in situ reflectometry [44, 45]. A home-made reflectometry system shown in Figure 2-1 was established in our 3 x 2” Thomas-Swan Close-Coupled Showerhead (CCS) MOCVD system. White light is reflected from the sample surface and monitored by a spectrometer during the growth. The reflectivity is sensitive to both the surface morphology and the epitaxial layer structure.Figure 2-1. Illustration of a home-made in situ reflectometry system integrated into the MOCVD system.Figure 2-2. Typical growth conditions for GaN templates used in this research.Typical growth conditions for GaN templates used in this research are summarized in Figure 2-2 and Table 2-2. Unless otherwise mentioned, c-plane sapphire substrates were used. The five steps outlined in Table 2-2, including high temperature (HT) cleaning, nitridation, low temperature (LT) nucleation, annealing of LT nucleation layer, and HT GaN growth, are crucial for high quality GaN epilayer.Figure 2-3 and Table 2-3 show the corresponding in situ reflectometry signal.In the following, we will describe how the reflectometry signal can be used to optimize the GaN template growth. Unless otherwise mentioned, we will refer to the reflectometry signal shown in Figure 2-3.Figure 2-3. In situ reflectometry trace of GaN template growth (Sample ID : UM-S07- 254). The highlighted areas correspond to important sub-steps during the epitaxy.2.1.1.1 High Temperature CleaningInitially, as the sample temperature is ramped up, the reflectivity increases due to the increase of the refractive index of the sample. Kim et al. has thoroughly studied the effect of initial thermal cleaning on the sapphire substrate andexperimentally demonstrated that this thermal treatment can effectively reduce the surface roughness of the substrate [46]. Generally, the flat surface is preferred for the GaN nuclei to be formed uniformly, which is critical to the crystal quality of the final GaN epilayer. The specific condition for the HT cleaning should be optimized by examining the treatment temperature and time. In our GaN growth, the optimal treatment temperature and time were set to be 1075 °C and 5 minutes, respectively. Moreover, HT surface annealing can effectively eliminate surface moisture.2.1.1.2 NitridationNitridation [5, 7] is the process of NH3 preflow under hydrogen (H2) ambient to prepare the surface for growth. During nitridation, NH3 reacts with the surface oxygen atoms on the sapphire substrate. Due to the replacement of the oxygen atoms by the nitrogen atoms and the diffusion of the nitrogen atoms into a certain depth, the exposed surface becomes a smooth amorphous state. Because this change of surface morphology is on the order of tens of angstrom, the corresponding reflectivity change is not significant. It has been shown that with a proper nitridation condition, GaN epilayers with lower dislocation density and better electrical and optical properties can be achieved [7]. However, as mentioned above, suitable combination of reactor conditions such as temperature, treatment time, and NH3 flow rate must be considered. In our GaN growth, the nitridation was optimized at 530 °C for a total of 210 seconds under 3 slm of NH3 flow.2.1.1.3 Low Temperature NucleationAs mentioned in Section 1.3.1, several approaches have been introduced to reduce the threading dislocation (TD) density in growing the GaN template. Specifically, the use of low temperature nucleation layer (LT NL) has been shown to be simple yet effective. A threading dislocation density as low as 108/cm2 has been reported [8].As GaN is nucleated on sapphire, the cubic phase islands are first formed at a temperature of 450 - 600 °C. These islands are subsequently transformed into the wurtzite phase [8]. The increase of the reflectivity during the LT NL growth is attributed to the increase of reflection from the flat top surfaces of nuclei. Basically, we know that the reflection from GaN is about twice stronger than that from sapphire due to the difference in refractive indices. As the islands become denser (i.e. the growth time of LT NL becomes longer), total reflection from the top surface of nuclei becomes up to 200% of reflection from sapphire substrate assuming that the entire surface is covered by GaN islands. Even though the islands are not coalesced completely to form a crystalline layer, this is still possible because the distances between the adjacent islands are too small compared to the optical wavelength. Once the reflectance exceeds twice that of the sapphire (as shown in Figure 2-3), the islands continue to coalesce further, which results in larger GaN grains and a thicker NL. Here, the size of the nucleation islands and the thickness of the NL are critical to obtain high quality GaN epilayer. To show that, we have compared a series of GaN templates with different NL conditions. All conditions were kept the same† except the growth time of the LT NL was varied,resulting in different LT NL thicknesses. The thickness of the LT NL was extrapolated by analyzing the reflectometry data as the reflection ratio at the end of LT NL growth to the sapphire substrate (RLT NL / RSapphire). The qualities of the GaN templates were characterized using photoluminescence (PL) and x-ray diffraction (XRD). From these results, the best GaN template quality can be obtained when RLT NL / RSapphire is around 2.6 which corresponds to a 40nm thick NL, at the given growth conditions.† LT NL growth temperature = 530°C, V/III = 9140, LT NL annealing time = 420 seconds, HT GaN growth temperature = 1040°C, V/III = 1230, growth time = 4300 seconds.Figure 2-4. The comparison of GaN template qualities with respect to the reflection ratio between the LT NL surface and the sapphire substrate.2.1.1.4 Annealing of Low Temperature Nucleation LayerIn GaN hetero-epitaxy with a large lattice mismatch, the initial growth on the surface follows the Volmer Weber model [47], i.e. GaN island growth dominates. In order to obtain smooth GaN templates, these islands need to be transformed into the layer-by-layer growth mode using an NL annealing process. During annealing, the substrate temperature is gradually increased up to around 1030 - 1050 °C under NH3 overpressure. Temperature ramping rate, reactor pressure, and NH3 flow can control the NL decomposition rate, which determines the surface roughness at the end of the annealing process [48, 49]. In Figure 2-3, after point (h) at which LT NL annealing begins, slight increase of reflectance is normally observed. The increase continues until around 800 °C at which GaN decomposition process starts to occur. Once the reflection intensity peaks, it begins to drop due to the increase in surface roughness. Initially randomly distributed islands start to be transformed into relatively uniform islands due to the decomposition of the NL and the migration of the gallium ad-atoms.During the annealing process, the reflectivity first decreases due to the increase of surface roughness. Further annealing results in a slight increase of reflectivity because at a higher temperature, the surface morphology becomes smoother. However, if we anneal the surface even further, the surface roughness increases again, which results in the decrease of reflection intensity [48, 49]. This phenomenon can be explained by considering the volume of the GaN islands. At the transition point ((k) in Figure 2-3), the volume of the islands per unit area becomes the highest which is preferable for the subsequent HT GaN growth. As a rule of thumb, the position of this (reflectometry trace) shoulder is dominated by the highest temperature of the annealing process [50]. In summary, the goal of the low temperature nucleation and the subsequent annealing is to achieve a surface morphology with proper density and sizes of the islands for the following HT GaN growth.As shown in Figure 2-5, even a slight change of the island distribution caused by a slight difference of the NL thickness and temperature ramping rate (Table 2-4) can result in a significant difference in the following HT GaN growth under the same conditions. In general, it takes longer for an NL with a rougher surface and smaller islands to be transformed into the 2D growth mode. The conditions to achieve high crystal quality GaN on sapphire are mostly related to the growth and annealing of the LT NL.2.1.1.5 HT GaN GrowthAs soon as the sapphire surface is covered with suitable volume, uniformity, thickness, and density of GaN islands, HT GaN growth can be followed. This HT GaN itself can be divided into two parts (Figure 2-6). Part I corresponds to the initial stage of HT GaN growth when the growth mode is transitioned from 3D to 2D, which affects the crystal quality significantly. In part II, GaN epilayer becomes thicker because the growth mode as well as growth condition is stabilized for 2Dmode. Several strategies to control the GaN growth in each regime will be briefly discussed in the following.The growth in part I is a buffer step to prepare a surface suitable for HT GaN growth. During this step, the oscillation of the reflectometry signal becomes increasingly obvious. Initially, the reflectivity continues to drop due to the increase of surface roughness induced by the coagulations of the islands, i.e. 3D growth. As time goes by, the 3D growth mode is suppressed and the 2D growth mode is enhanced. Once the surface becomes flattened due to the enhanced 2D growth, layer by layer growth of GaN begins, which causes the reflectivity to increase. The duration of this part of growth can be optimized by tweaking the reactor pressure, V/III ratio, and growth rate [51, 52]. For example, in the case of a low V/III ratio, it takes longer to recover the reflection intensity, which implies that the change of the growth mode (3D 2D) occurs more slowly. The reflectivity recovery time is critical to oscillation amplitude in part II. In general, a larger oscillation amplitude corresponds to a better crystal quality.The part II of the HT GaN growth is stable in a wide range of growth conditions because the growth occurs in a mass transfer limited region. Nevertheless, several key factors will still affect the crystalline structure, including the growth temperature, trimethyl-gallium (TMG) flow, NH3 flow, V/III ratio, and reactor pressure. As shown in Figure 2-7, the growth rate increases as the group III flow increases but decreases as the V/III ratio and growth temperature increase. The。
期末论文16×32点阵LED电子显示屏的设计模块

期末电子设计论文16×32点阵LED电子显示屏的设计The 16×32 lattice LED electron display monitor design论文书组长姓名:学号:指导教师;所在院系:计算机科学与技术所学专业:电子信息科学与技术山东财经大学中国·济南2013年6月一、课题名称16×32点阵LED电子显示屏的设计二、课题内容设计一个室内用的16×32点阵LED图文显示屏。
三、课题任务要求在目测条件下16×32点阵LED显示屏各点亮度均匀、充足,可显示图形和文字,显示图形或文字应稳定、清晰无串扰。
图形或文字显示有静止、移入移出等显示方式。
四、主要参考文献[1] 郭天祥主编:《51单片机C语言教程》北京:电子工业出版社,2013[2]张毅刚主编:《单片机原理及应用》.北京:高等教育出版社,2006指导教师签字教研室主任签字年月日摘要本设计是一16×32点阵LED电子显示屏的设计。
整机以美国ATMEL 公司生产的40脚单片机AT89C52为核心,介绍了以它为控制系统的LED点阵电子显示屏的动态设计和开发过程。
通过该芯片控制两个个行驱动器74HC138和两个列驱动器74HC595来驱动显示屏显示。
该电子显示屏可以显示各种文字或单色图像,全屏能显示2个汉字,采用8块8×8点阵LED显示模块来组成16×32点阵显示模式。
显示采用动态显示,使得图形或文字能够实现静止、移入移出等多种显示方式。
文中详细介绍了LED点阵显示的硬件设计思路、硬件电路各个部分的功能及原理、相应软件的程序设计,以及使用说明等。
单片机控制系统程序采用单片机C语言进行编辑,通过编程控制各显示点对应LED阳极和阴极端的电平,就可以有效的控制各显示点的亮灭。
所显示字符的点阵数据可以自行编写(即直接点阵画图)。
LED显示以其组构方式灵活、显示稳定、功耗低、寿命长、技术成熟、成本低廉等特点在车站、证券所、运动场馆、交通干道及各种室内/外显示场合的信息发布,公益宣传,环境参数实时,重大活动倒计时等等得到广泛的应用。
LED点阵显示屏毕业设计文献综述
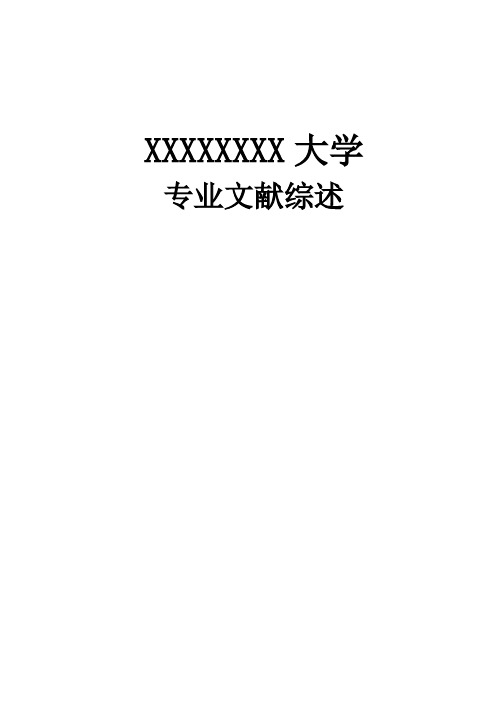
XXXXXXXX大学专业文献综述LED点阵电子显示屏系统的设计作者:xxx 指导老师:xxx摘要:本设计拟采用一种由单片机控制的8*8点阵发光模块组成的16*64发光点阵,探讨简单的汉字、英文字母和动态图象显示技术,以及实时的温度测量显示。
该系统具有设计简单、字符清晰、可靠性高等特点。
关键词:LED;单片机;点阵;Design of LED Dot Matrix Electronic Display SystemAuthor: Song Jian—lei Instruct teacher: Li Xue—lianAbstract:The paper introduces a kind of 16*64 dot matrix consists of 8*8 dot matrix module,we discuss the display technology of Chinese character, English letter,dynamic image and real time temperature。
It is simple in design,cheap in cost and high in reliability.Key words: LED;Single Chip Microcomputer;Dot Matrix;引言LED(Light Emitting Diode,LED)电子显示屏是随着计算机及相关的微电子、光电子技术的迅速发展而形成的一种新型信息显示媒体。
LED点阵显示器作为一种新兴的显示器件,它是由多个独立的LED发光二极管封装而成。
发光二极管LED具有体积小、抗冲击和抗震性能好、高可靠性、寿命长、工作电压低、功耗小及响应速度等优点[1].由于电子显示屏具有显示内容信息量大,外形美观大方,操作使用方便灵活,用户可随时任意自行编辑修改显示内容,显示方式图文并茂等优点,因此被广泛应用于商场、学校、银行、邮局、机场、车站、码头、金融证券市场、文化中心、信息中心休息设施等公共场所[2].本文主要介绍一种用于值班室外等场合的公告牌的LED点阵电子显示屏。
LED点阵全彩屏的设计【文献综述】
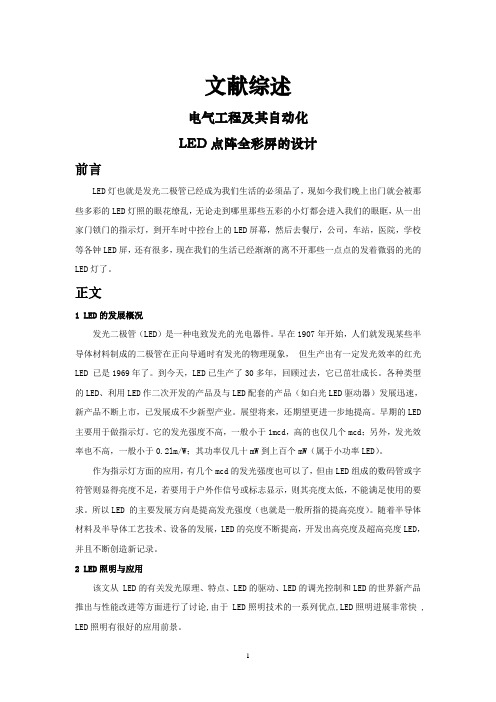
文献综述电气工程及其自动化LED点阵全彩屏的设计前言LED灯也就是发光二极管已经成为我们生活的必须品了,现如今我们晚上出门就会被那些多彩的LED灯照的眼花缭乱,无论走到哪里那些五彩的小灯都会进入我们的眼眶,从一出家门锁门的指示灯,到开车时中控台上的LED屏幕,然后去餐厅,公司,车站,医院,学校等各钟LED屏,还有很多,现在我们的生活已经渐渐的离不开那些一点点的发着微弱的光的LED灯了。
正文1 LED的发展概况发光二极管(LED)是一种电致发光的光电器件。
早在1907年开始,人们就发现某些半导体材料制成的二极管在正向导通时有发光的物理现象,但生产出有一定发光效率的红光LED 已是1969年了。
到今天,LED已生产了30多年,回顾过去,它已茁壮成长。
各种类型的LED、利用LED作二次开发的产品及与LED配套的产品(如白光LED驱动器)发展迅速,新产品不断上市,已发展成不少新型产业。
展望将来,还期望更进一步地提高。
早期的LED 主要用于做指示灯。
它的发光强度不高,一般小于1mcd,高的也仅几个mcd;另外,发光效率也不高,一般小于0.2lm/W;其功率仅几十mW到上百个mW(属于小功率LED)。
作为指示灯方面的应用,有几个mcd的发光强度也可以了,但由LED组成的数码管或字符管则显得亮度不足,若要用于户外作信号或标志显示,则其亮度太低,不能满足使用的要求。
所以LED 的主要发展方向是提高发光强度(也就是一般所指的提高亮度)。
随着半导体材料及半导体工艺技术、设备的发展,LED的亮度不断提高,开发出高亮度及超高亮度LED,并且不断创造新记录。
2 LED照明与应用该文从 LED的有关发光原理、特点、LED的驱动、LED的调光控制和LED的世界新产品推出与性能改进等方面进行了讨论,由于 LED照明技术的一系列优点,LED照明进展非常快 , LED照明有很好的应用前景。
1963年2月, LED的发明者N. HOLONY AK在《读者文摘》(美)上发表了“我们坚信LED 会发展成实用的白色光源”一文 ,并作了明确的预言:“将来的灯可以是铅笔尖大小的一块合金 ,它实用且不易破碎、决不烧毁 ,比起今天通用的灯泡来说,其转换效率至少大10倍。
LED显示屏中英文对照外文翻译文献

LED显示屏中英文对照外文翻译文献(文档含英文原文和中文翻译)译文:大屏幕显示系统的研究LED的发展随着计算机技术的高速发展,LED屏幕显示系统作为继电视、广播、报纸、杂志之后的“第五大媒体”正快速步入社会生活的各个方面。
它集微电子技术、计算机技术、信息处理技术于一体,可以将信息通过文字、图案、动画及视频四种形式显示出来。
与电视墙、磁翻板等媒体相比,LED大屏幕显示系统具有图案美观、色彩亮丽;图案、色彩变化丰富、快速;低功耗、长寿命、使用成本低、工作稳定可靠等特点。
它显示的图文视角大、视距远,因而已广泛应用于大型广场、商业广告、体育场馆、信息传播、新闻发布、证券交易;它还应用于工业控制和工业调动系统,便于把各种参数、报警点、工艺流程显示得更加清晰完美,可以满足不同环境的需要。
LED显示屏是一种利用计算机和复杂数字信号处理的电子广告宣传屏。
它的屏体部分由微处理器(主要是单片机)和驱动电路控制运行,显示的图像或文字由计算机编辑软件编辑获得。
由于LED显示屏这种新一代信息显示设备具有显示图案稳定、功耗低、寿命长等特点,而且它综合了各种信息显示设备的长处,并且克服了自身的不足,特别是由于一幅显示屏可以显示不同的内容,显示方式丰富。
所以在公共场合,它具有强烈的广告宣传和信息传递效果,日趋在固体显示中占主导地位。
LED显示屏的发展前景极为广阔,目前正朝着更高亮度、更高耐气候性、更高的发光密度、更高的发光均匀性、可靠性、全色化方向发展。
由不同材料的半导体组成能发出不同色彩的LED晶点。
目前应用最广的是红色、绿色、黄色LED。
而蓝色和纯绿色LED的开发已经达到了实用阶段。
LED显示屏的分类LED显示屏是多种技术综合应用的产品,涉及光电子学、半导体器件、数字电子电路、大规模集成电路、单片机及微机等各个方面,既有硬件又有软件。
LED 显示屏是作为广播、电视、报纸、杂志之后的又一新传播媒体。
目前LED显示屏根据使用场所不同,可以分为室外屏和室内屏两种,其主要区别是发光管的发光亮度不同。
- 1、下载文档前请自行甄别文档内容的完整性,平台不提供额外的编辑、内容补充、找答案等附加服务。
- 2、"仅部分预览"的文档,不可在线预览部分如存在完整性等问题,可反馈申请退款(可完整预览的文档不适用该条件!)。
- 3、如文档侵犯您的权益,请联系客服反馈,我们会尽快为您处理(人工客服工作时间:9:00-18:30)。
基于AT89C52的LED概述LED(Light Emitting Diode),发光二极管,是一种固态的半导体器件,它可以直接把电转化为光。
LED的心脏是一个半导体的晶片,晶片的一端附在一个支架上,一端是负极,另一端连接电源的正极,使整个晶片被环氧树脂封装起来。
半导体晶片由两部分组成,一部分是P型半导体,在它里面空穴占主导地位,另一端是N型半导体,在这边主要是电子。
但这两种半导体连接起来的时候,它们之间就形成一个“P-N结”。
当电流通过导线作用于这个晶片的时候,电子就会被推向P区,在P区里电子跟空穴复合,然后就会以光子的形式发出能量,这就是LED发光的原理。
而光的波长也就是光的颜色,是由形成P-N结的材料决定的。
LED历史50年前人们已经了解半导体材料可产生光线的基本知识,第一个商用二极管产生于1960年。
LED是英文light emitting diode(发光二极管)的缩写,它的基本结构是一块电致发光的半导体材料,置于一个有引线的架子上,然后四周用环氧树脂密封,即固体封装,所以能起到保护内部芯线的作用,所以LED的抗震性能好。
发光二极管的核心部分是由P型半导体和N型半导体组成的晶片,在P 型半导体和N型半导体之间有一个过渡层,称为P-N结。
在某些半导体材料的PN结中,注入的少数载流子与多数载流子复合时会把多余的能量以光的形式释放出来,从而把电能直接转换为光能。
PN结施加反向电压时,少数载流子难以注入,故不发光。
这种利用注入式电致发光原理制作的二极管叫发光二极管,通称LED。
当它处于正向工作状态时(即两端加上正向电压),电流从LED阳极流向阴极时,半导体晶体就发出从紫外到红外不同颜色的光线,光的强弱与电流有关。
最初LED用作仪器仪表的指示光源,后来各种光色的LED在交通信号灯和大面积显示屏中得到了广泛应用,产生了很好的经济效益和社会效益。
以12英寸的红色交通信号灯为例,在美国本来是采用长寿命、低光效的140瓦白炽灯作为光源,它产生2000流明的白光。
经红色滤光片后,光损失90%,只剩下200流明的红光。
而在新设计的灯中,Lumileds公司采用了18个红色LED光源,包括电路损失在内,共耗电14瓦,即可产生同样的光效。
汽车信号灯也是LED光源应用的重要领域。
对于一般照明而言,人们更需要白色的光源。
1998年白光的LED 开发成功。
这种LED是将GaN芯片和钇铝石榴石(YAG)封装在一起做成。
GaN芯片发蓝光(λp=465nm,Wd=30nm),高温烧结制成的含Ce3+的YAG荧光粉受此蓝光激发后发出黄色光射,峰值550nm。
蓝光LED基片安装在碗形反射腔中,覆盖以混有YAG的树脂薄层,约200-500nm。
LED基片发出的蓝光部分被荧光粉吸收,另一部分蓝光与荧光粉发出的黄光混合,可以得到得白光。
现在,对于InGaN/YAG白色LED,通过改变YAG荧光粉的化学组成和调节荧光粉层的厚度,可以获得色温3500-10000K的各色白光。
这种通过蓝光LED得到白光的方法,构造简单、成本低廉、技术成熟度高,因此运用最多。
上个世纪60年代,科技工作者利用半导体PN结发光的原理,研制成了LED发光二极管。
当时研制的LED,所用的材料是GaASP,其发光颜色为红色。
经过近30年的发展,现在大家十分熟悉的LED,已能发出红、橙、黄、绿、蓝等多种色光。
然而照明需用的白色光LED仅在近年才发展起来,这里向读者介绍有关照明用白光。
LED显示屏分为图文显示屏和视频显示屏,均由LED矩阵块组成。
图文显示屏可与计算机同步显示汉字、英文文本和图形;视频显示屏采用微型计算机进行控制,图文、图像并茂,以实时、同步、清晰的信息传播方式播放各种信息,还可显示二维、三维动画、录像、电视、VCD节目以及现场实况。
LED显示屏显示画面色彩鲜艳,立体感强,静如油画,动如电影,广泛应用于金融、税务、工商、邮电、体育、广告、厂矿企业、交通运输、教育系统、车站、码头、机场、商场、医院、宾馆、银行、证券市场、建筑市场、拍卖行、工业企业管理和其它公共场所。
LED显示屏可以显示变化的数字、文字、图形图像;不仅可以用于室内环境还可以用于室外环境,具有投影仪、电视墙、液晶显示屏无法比拟的优点。
LED之所以受到广泛重视而得到迅速发展,是与它本身所具有的优点分不开的。
这些优点概括起来是:亮度高、工作电压低、功耗小、小型化、寿命长、耐冲击和性能稳定。
LED的发展前景极为广阔,目前正朝着更高亮度、更高耐气候性、更高的发光密度、更高的发光均匀性,可靠性、全色化方向发展。
LED显示屏的分类 1、按颜色基色可以分为,单基色显示屏:单一颜色(红色或绿色)。
双基色显示屏:红和绿双基色,256级灰度、可以显示65536种颜色。
全彩色显示屏:红、绿、蓝三基色,256级灰度的全彩色显示屏可以显示一千六百多万种颜色。
2、按显示器件分类LED数码显示屏:显示器件为7段码数码管,适于制作时钟屏、利率屏等,显示数字的电子显示屏。
LED点阵图文显示屏:显示器件是由许多均匀排列的发光二极管组成的点阵显示模块,适于播放文字、图像信息。
LED视频显示屏:显示器件是由许多发光二极管组成,可以显示视频、动画等各种视频文件。
3、按使用场合分类,室内显示屏:发光点较小,一般Φ3mm--Φ8mm,显示面积一般几至十几平方米。
室外显示屏:面积一般几十平方米至几百平方米,亮度高,可在阳光下工作,具有防风、防雨、防水功能。
4、按发光点直径分类,室内屏:Φ3mm、Φ3.75mm、Φ5mm。
室外屏:Φ10mm、Φ12mm、Φ16mm、Φ19mm、Φ20mm、Φ21mm、Φ22mm、Φ26mm 。
室外屏发光的基本单元为发光筒,发光筒的原理是将一组红、绿、蓝发光二极管封在一个塑料筒内共同发。
5.显示方式有静态、横向滚动、垂直滚动和翻页显示等。
单块模块控制驱动12块(最多可控制24块)8X8点阵,共16X48点阵(或32X48点阵),是单块MAX7219(或PS7219、HD7279、ZLG7289及8279等类似LED显示驱动模块)的12倍(或24倍)!可采用“级联”的方式组成任意点阵大显示屏。
显示效果好,功耗小,且比采用MAX7219电路的成本更低。
LED显示屏检验方法,一看屏体规格,外观,平整度,屏内的连线等。
二看屏点亮后坏点,在不在不范围之内,(一般来说现在的屏基本上没有了)色差一致性,显示文字是否正常,显示屏图片等,全彩的要全屏打白色,红,绿,蓝。
技术优势评述。
现有常见的室内全彩方案的比较:1.点阵模块方案:最早的设计方案,由室内伪彩点阵屏发展而来。
优势:原材料成本最有优势,且生产加工工艺简单,质量稳定。
缺点:色彩一致性差,马赛克现象较严重,显示效果较差。
2.单灯方案:为解决点阵屏色彩问题,借鉴户外显示屏技术的一种方案,同时将户外的像素复用技术(又叫像素共享技术,虚拟像素技术)移植到了室内显示屏。
优势:色彩一致性比点阵模块方式的好。
缺点:混色效果不佳,视角不大,水平方向左右观看有色差。
加工较复杂,抗静电要求高。
实际像素分辨率做到10000点以上较难。
主要性能●与MCS-51单片机产品兼容●8K字节在系统可编程Flash存储器●1000次擦写周期●全静态操作:0Hz~33Hz●三级加密程序存储器●32个可编程I/O口线●三个16位定时器/计数器●八个中断源●全双工UART串行通道●低功耗空闲和掉电模式●掉电后中断可唤醒●看门狗定时器●双数据指针●掉电标识符功能特性描述AT89C52是一种低功耗、高性能CMOS8位微控制器,具有8K在系统可编程Flash 存储器。
使用Atmel公司高密度非易失性存储器技术制造,与工业80C51产品指令和引脚完全兼容。
片上Flash允许程序存储器在系统可编程,亦适于常规编程器。
在单芯片上,拥有灵巧的8位CPU和在系统可编程Flash,使得AT89C52为众多嵌入式控制应用系统提供高灵活、超有效的解决方案。
AT89C52具有以下标准功能:8k 字节Flash,256字节RAM,32位I/O口线,看门狗定时器,2个数据指针,三个16位定时器/计数器,一个6向量2级中断结构,全双工串行口,片内晶振及时钟电路。
另外,AT89C52可降至0Hz静态逻辑操作,支持2种软件可选择节电模式。
空闲模式下,CPU停止工作,允许RAM、定时器/计数器、串口、中断继续工作。
掉电保护方式下,RAM内容被保存,振荡器被冻结,单片机一切工作停止,直到下一个中断或硬件复位为止。
ST: 复位输入。
晶振工作时,RST脚持续2个机器周期高电平将使单片机复位。
看门狗计时完成后,RST脚输出96个晶振周期的高电平。
特殊寄存器AUXR(地址8EH)上的DISRTO位可以使此功能无效。
DISRTO默认状态下,复位高电平有效。
ALE/PROG:地址锁存控制信号(ALE)是访问外部程序存储器时,锁存低8位地址的输出脉冲。
在flash编程时,此引脚(PROG)也用作编程输入脉冲。
在一般情况下,ALE 以晶振六分之一的固定频率输出脉冲,可用来作为外部定时器或时钟使用。
然而,特别强调,在每次访问外部数据存储器时,ALE脉冲将会跳过。
如果需要,通过将地址为8EH的SFR的第0位置“1”,ALE操作将无效。
这一位置“1”,ALE 仅在执行MOVX 或MOVC指令时有效。
否则,ALE 将被微弱拉高。
这个ALE 使能标志位(地址为8EH的SFR的第0位)的设置对微控制器处于外部执行模式下无效。
PSEN:外部程序存储器选通信号(PSEN)是外部程序存储器选通信号。
当 AT89S52从外部程序存储器执行外部代码时,PSEN在每个机器周期被激活两次,而在访问外部数据存储器时,PSEN将不被激活。
EA /VPP:访问外部程序存储器控制信号。
为使能从0000H 到FFFFH的外部程序存储器读取指令,EA必须接GND。
为了执行内部程序指令,EA应该接VCC。
在flash编程期间,EA也接收12伏VPP电压。
XTAL1:振荡器反相放大器和内部时钟发生电路的输入端。
XTAL2:振荡器反相放大器的输出端。
存储器结构:MCS-51器件有单独的程序存储器和数据存储器。
外部程序存储器和数据存储器都可以64K寻址。
程序存储器:如果EA引脚接地,程序读取只从外部存储器开始。
对于89C52,如果EA接VCC,程序读写先从内部存储器(地址为0000H~1FFFH)开始,接着从外部寻址,寻址地址为:2000H~FFFFH。
中断 AT89C52有6个中断源:两个外部中断(INT0和INT1),三个定时中断(定时器0、1、2)和一个串行中断。
这些中断每个中断源都可以通过置位或清除特殊寄存器IE中的相关中断允许控制位分别使得中断源有效或无效。