Search for R-parity Violating SUSY in Run 2 at D0
PACSnumbers1215Ff,1130Hv,1210Dm,1125Mj…
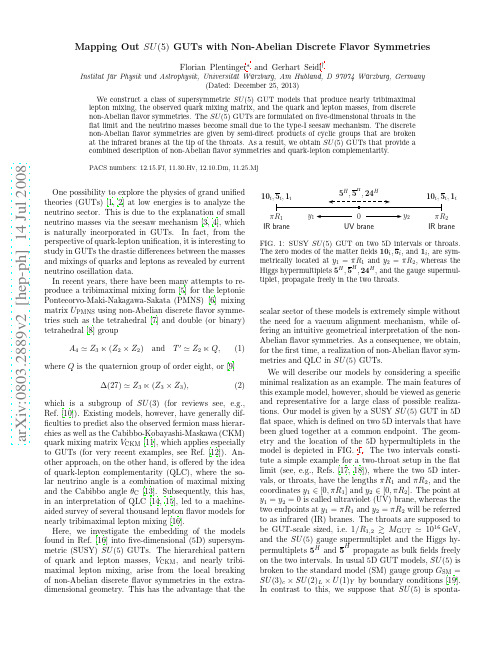
a r X i v :0803.2889v 2 [h e p -p h ] 14 J u l 2008Mapping Out SU (5)GUTs with Non-Abelian Discrete Flavor SymmetriesFlorian Plentinger ∗and Gerhart Seidl †Institut f¨u r Physik und Astrophysik,Universit¨a t W¨u rzburg,Am Hubland,D 97074W¨u rzburg,Germany(Dated:December 25,2013)We construct a class of supersymmetric SU (5)GUT models that produce nearly tribimaximal lepton mixing,the observed quark mixing matrix,and the quark and lepton masses,from discrete non-Abelian flavor symmetries.The SU (5)GUTs are formulated on five-dimensional throats in the flat limit and the neutrino masses become small due to the type-I seesaw mechanism.The discrete non-Abelian flavor symmetries are given by semi-direct products of cyclic groups that are broken at the infrared branes at the tip of the throats.As a result,we obtain SU (5)GUTs that provide a combined description of non-Abelian flavor symmetries and quark-lepton complementarity.PACS numbers:12.15.Ff,11.30.Hv,12.10.Dm,One possibility to explore the physics of grand unified theories (GUTs)[1,2]at low energies is to analyze the neutrino sector.This is due to the explanation of small neutrino masses via the seesaw mechanism [3,4],which is naturally incorporated in GUTs.In fact,from the perspective of quark-lepton unification,it is interesting to study in GUTs the drastic differences between the masses and mixings of quarks and leptons as revealed by current neutrino oscillation data.In recent years,there have been many attempts to re-produce a tribimaximal mixing form [5]for the leptonic Pontecorvo-Maki-Nakagawa-Sakata (PMNS)[6]mixing matrix U PMNS using non-Abelian discrete flavor symme-tries such as the tetrahedral [7]and double (or binary)tetrahedral [8]groupA 4≃Z 3⋉(Z 2×Z 2)and T ′≃Z 2⋉Q,(1)where Q is the quaternion group of order eight,or [9]∆(27)≃Z 3⋉(Z 3×Z 3),(2)which is a subgroup of SU (3)(for reviews see, e.g.,Ref.[10]).Existing models,however,have generally dif-ficulties to predict also the observed fermion mass hierar-chies as well as the Cabibbo-Kobayashi-Maskawa (CKM)quark mixing matrix V CKM [11],which applies especially to GUTs (for very recent examples,see Ref.[12]).An-other approach,on the other hand,is offered by the idea of quark-lepton complementarity (QLC),where the so-lar neutrino angle is a combination of maximal mixing and the Cabibbo angle θC [13].Subsequently,this has,in an interpretation of QLC [14,15],led to a machine-aided survey of several thousand lepton flavor models for nearly tribimaximal lepton mixing [16].Here,we investigate the embedding of the models found in Ref.[16]into five-dimensional (5D)supersym-metric (SUSY)SU (5)GUTs.The hierarchical pattern of quark and lepton masses,V CKM ,and nearly tribi-maximal lepton mixing,arise from the local breaking of non-Abelian discrete flavor symmetries in the extra-dimensional geometry.This has the advantage that theFIG.1:SUSY SU (5)GUT on two 5D intervals or throats.The zero modes of the matter fields 10i ,5H,24H ,and the gauge supermul-tiplet,propagate freely in the two throats.scalar sector of these models is extremely simple without the need for a vacuum alignment mechanism,while of-fering an intuitive geometrical interpretation of the non-Abelian flavor symmetries.As a consequence,we obtain,for the first time,a realization of non-Abelian flavor sym-metries and QLC in SU (5)GUTs.We will describe our models by considering a specific minimal realization as an example.The main features of this example model,however,should be viewed as generic and representative for a large class of possible realiza-tions.Our model is given by a SUSY SU (5)GUT in 5D flat space,which is defined on two 5D intervals that have been glued together at a common endpoint.The geom-etry and the location of the 5D hypermultiplets in the model is depicted in FIG.1.The two intervals consti-tute a simple example for a two-throat setup in the flat limit (see,e.g.,Refs.[17,18]),where the two 5D inter-vals,or throats,have the lengths πR 1and πR 2,and the coordinates y 1∈[0,πR 1]and y 2∈[0,πR 2].The point at y 1=y 2=0is called ultraviolet (UV)brane,whereas the two endpoints at y 1=πR 1and y 2=πR 2will be referred to as infrared (IR)branes.The throats are supposed to be GUT-scale sized,i.e.1/R 1,2 M GUT ≃1016GeV,and the SU (5)gauge supermultiplet and the Higgs hy-permultiplets 5H and2neously broken to G SM by a 24H bulk Higgs hypermulti-plet propagating in the two throats that acquires a vac-uum expectation value pointing in the hypercharge direc-tion 24H ∝diag(−12,13,15i ,where i =1,2,3is the generation index.Toobtainsmall neutrino masses via the type-I seesaw mechanism [3],we introduce three right-handed SU (5)singlet neutrino superfields 1i .The 5D Lagrangian for the Yukawa couplings of the zero mode fermions then readsL 5D =d 2θ δ(y 1−πR 1) ˜Y uij,R 110i 10j 5H +˜Y d ij,R 110i 5H +˜Y νij,R 15j5i 1j 5H +M R ˜Y R ij,R 21i 1j+h.c. ,(3)where ˜Y x ij,R 1and ˜Y x ij,R 2(x =u,d,ν,R )are Yukawa cou-pling matrices (with mass dimension −1/2)and M R ≃1014GeV is the B −L breaking scale.In the four-dimensional (4D)low energy effective theory,L 5D gives rise to the 4D Yukawa couplingsL 4D =d 2θ Y u ij 10i 10j 5H +Y dij10i 5H +Y νij5i ∼(q i 1,q i 2,...,q i m ),(5)1i ∼(r i 1,r i 2,...,r im ),where the j th entry in each row vector denotes the Z n jcharge of the representation.In the 5D theory,we sup-pose that the group G A is spontaneously broken by singly charged flavon fields located at the IR branes.The Yukawa coupling matrices of quarks and leptons are then generated by the Froggatt-Nielsen mechanism [21].Applying a straightforward generalization of the flavor group space scan in Ref.[16]to the SU (5)×G A represen-tations in Eq.(5),we find a large number of about 4×102flavor models that produce the hierarchies of quark and lepton masses and yield the CKM and PMNS mixing angles in perfect agreement with current data.A distri-bution of these models as a function of the group G A for increasing group order is shown in FIG.2.The selection criteria for the flavor models are as follows:First,all models have to be consistent with the quark and charged3 lepton mass ratiosm u:m c:m t=ǫ6:ǫ4:1,m d:m s:m b=ǫ4:ǫ2:1,(6)m e:mµ:mτ=ǫ4:ǫ2:1,and a normal hierarchical neutrino mass spectrumm1:m2:m3=ǫ2:ǫ:1,(7)whereǫ≃θC≃0.2is of the order of the Cabibbo angle.Second,each model has to reproduce the CKM anglesV us∼ǫ,V cb∼ǫ2,V ub∼ǫ3,(8)as well as nearly tribimaximal lepton mixing at3σCLwith an extremely small reactor angle 1◦.In perform-ing the group space scan,we have restricted ourselves togroups G A with orders roughly up to 102and FIG.2shows only groups admitting more than three valid mod-els.In FIG.2,we can observe the general trend thatwith increasing group order the number of valid modelsper group generally increases too.This rough observa-tion,however,is modified by a large“periodic”fluctu-ation of the number of models,which possibly singlesout certain groups G A as particularly interesting.Thehighly populated groups would deserve further system-atic investigation,which is,however,beyond the scopeof this paper.From this large set of models,let us choose the groupG A=Z3×Z8×Z9and,in the notation of Eq.(5),thecharge assignment101∼(1,1,6),102∼(0,3,1),103∼(0,0,0),52∼(0,7,0),52↔4FIG.3:Effect of the non-Abelian flavor symmetry on θ23for a 10%variation of all Yukawa couplings.Shown is θ23as a function of ǫfor the flavor group G A (left)and G A ⋉G B (right).The right plot illustrates the exact prediction of the zeroth order term π/4in the expansion θ23=π/4+ǫ/√2and the relation θ13≃ǫ2.The important point is that in the expression for θ23,the leading order term π/4is exactly predicted by thenon-Abelian flavor symmetry G F =G A ⋉G B (see FIG.3),while θ13≃θ2C is extremely small due to a suppression by the square of the Cabibbo angle.We thus predict a devi-ation ∼ǫ/√2,which is the well-known QLC relation for the solar angle.There have been attempts in the literature to reproduce QLC in quark-lepton unified models [26],however,the model presented here is the first realization of QLC in an SU (5)GUT.Although our analysis has been carried out for the CP conserving case,a simple numerical study shows that CP violating phases (cf.Ref.[27])relevant for neutri-noless double beta decay and leptogenesis can be easily included as well.Concerning proton decay,note that since SU (5)is bro-ken by a bulk Higgs field,the broken gauge boson masses are ≃M GUT .Therefore,all fermion zero modes can be localized at the IR branes of the throats without intro-ducing rapid proton decay through d =6operators.To achieve doublet-triplet splitting and suppress d =5pro-ton decay,we may then,e.g.,resort to suitable extensions of the Higgs sector [28].Moreover,although the flavor symmetry G F is global,quantum gravity effects might require G F to be gauged [29].Anomalies can then be canceled by Chern-Simons terms in the 5D bulk.We emphasize that the above discussion is focussed on a specific minimal example realization of the model.Many SU (5)GUTs with non-Abelian flavor symmetries,however,can be constructed along the same lines by varying the flavor charge assignment,choosing different groups G F ,or by modifying the throat geometry.A de-tailed analysis of these models and variations thereof will be presented in a future publication [30].To summarize,we have discussed the construction of 5D SUSY SU (5)GUTs that yield nearly tribimaximal lepton mixing,as well as the observed CKM mixing matrix,together with the hierarchy of quark and lepton masses.Small neutrino masses are generated only by the type-I seesaw mechanism.The fermion masses and mixings arise from the local breaking of non-Abelian flavor symmetries at the IR branes of a flat multi-throat geometry.For an example realization,we have shown that the non-Abelian flavor symmetries can exactly predict the leading order term π/4in the sum rule for the atmospheric mixing angle,while strongly suppress-ing the reactor angle.This makes this class of models testable in future neutrino oscillation experiments.In addition,we arrive,for the first time,at a combined description of QLC and non-Abelian flavor symmetries in SU (5)GUTs.One main advantage of our setup with throats is that the necessary symmetry breaking can be realized with a very simple Higgs sector and that it can be applied to and generalized for a large class of unified models.We would like to thank T.Ohl for useful comments.The research of F.P.is supported by Research Train-ing Group 1147“Theoretical Astrophysics and Particle Physics ”of Deutsche Forschungsgemeinschaft.G.S.is supported by the Federal Ministry of Education and Re-search (BMBF)under contract number 05HT6WWA.∗********************************.de †**************************.de[1]H.Georgi and S.L.Glashow,Phys.Rev.Lett.32,438(1974);H.Georgi,in Proceedings of Coral Gables 1975,Theories and Experiments in High Energy Physics ,New York,1975.[2]J.C.Pati and A.Salam,Phys.Rev.D 10,275(1974)[Erratum-ibid.D 11,703(1975)].[3]P.Minkowski,Phys.Lett.B 67,421(1977);T.Yanagida,in Proceedings of the Workshop on the Unified Theory and Baryon Number in the Universe ,KEK,Tsukuba,1979;M.Gell-Mann,P.Ramond and R.Slansky,in Pro-ceedings of the Workshop on Supergravity ,Stony Brook,5New York,1979;S.L.Glashow,in Proceedings of the 1979Cargese Summer Institute on Quarks and Leptons, New York,1980.[4]M.Magg and C.Wetterich,Phys.Lett.B94,61(1980);R.N.Mohapatra and G.Senjanovi´c,Phys.Rev.Lett.44, 912(1980);Phys.Rev.D23,165(1981);J.Schechter and J.W. F.Valle,Phys.Rev.D22,2227(1980);zarides,Q.Shafiand C.Wetterich,Nucl.Phys.B181,287(1981).[5]P.F.Harrison,D.H.Perkins and W.G.Scott,Phys.Lett.B458,79(1999);P.F.Harrison,D.H.Perkins and W.G.Scott,Phys.Lett.B530,167(2002).[6]B.Pontecorvo,Sov.Phys.JETP6,429(1957);Z.Maki,M.Nakagawa and S.Sakata,Prog.Theor.Phys.28,870 (1962).[7]E.Ma and G.Rajasekaran,Phys.Rev.D64,113012(2001);K.S.Babu,E.Ma and J.W.F.Valle,Phys.Lett.B552,207(2003);M.Hirsch et al.,Phys.Rev.D 69,093006(2004).[8]P.H.Frampton and T.W.Kephart,Int.J.Mod.Phys.A10,4689(1995); A.Aranda, C. D.Carone and R.F.Lebed,Phys.Rev.D62,016009(2000);P.D.Carr and P.H.Frampton,arXiv:hep-ph/0701034;A.Aranda, Phys.Rev.D76,111301(2007).[9]I.de Medeiros Varzielas,S.F.King and G.G.Ross,Phys.Lett.B648,201(2007);C.Luhn,S.Nasri and P.Ramond,J.Math.Phys.48,073501(2007);Phys.Lett.B652,27(2007).[10]E.Ma,arXiv:0705.0327[hep-ph];G.Altarelli,arXiv:0705.0860[hep-ph].[11]N.Cabibbo,Phys.Rev.Lett.10,531(1963);M.Kobayashi and T.Maskawa,Prog.Theor.Phys.49, 652(1973).[12]M.-C.Chen and K.T.Mahanthappa,Phys.Lett.B652,34(2007);W.Grimus and H.Kuhbock,Phys.Rev.D77, 055008(2008);F.Bazzocchi et al.,arXiv:0802.1693[hep-ph];G.Altarelli,F.Feruglio and C.Hagedorn,J.High Energy Phys.0803,052(2008).[13]A.Y.Smirnov,arXiv:hep-ph/0402264;M.Raidal,Phys.Rev.Lett.93,161801(2004);H.Minakata andA.Y.Smirnov,Phys.Rev.D70,073009(2004).[14]F.Plentinger,G.Seidl and W.Winter,Nucl.Phys.B791,60(2008).[15]F.Plentinger,G.Seidl and W.Winter,Phys.Rev.D76,113003(2007).[16]F.Plentinger,G.Seidl and W.Winter,J.High EnergyPhys.0804,077(2008).[17]G.Cacciapaglia,C.Csaki,C.Grojean and J.Terning,Phys.Rev.D74,045019(2006).[18]K.Agashe,A.Falkowski,I.Low and G.Servant,J.HighEnergy Phys.0804,027(2008);C.D.Carone,J.Erlich and M.Sher,arXiv:0802.3702[hep-ph].[19]Y.Kawamura,Prog.Theor.Phys.105,999(2001);G.Altarelli and F.Feruglio,Phys.Lett.B511,257(2001);A.B.Kobakhidze,Phys.Lett.B514,131(2001);A.Hebecker and J.March-Russell,Nucl.Phys.B613,3(2001);L.J.Hall and Y.Nomura,Phys.Rev.D66, 075004(2002).[20]D.E.Kaplan and T.M.P.Tait,J.High Energy Phys.0111,051(2001).[21]C.D.Froggatt and H.B.Nielsen,Nucl.Phys.B147,277(1979).[22]Y.Nomura,Phys.Rev.D65,085036(2002).[23]H.Georgi and C.Jarlskog,Phys.Lett.B86,297(1979).[24]H.Arason et al.,Phys.Rev.Lett.67,2933(1991);H.Arason et al.,Phys.Rev.D47,232(1993).[25]D.S.Ayres et al.[NOνA Collaboration],arXiv:hep-ex/0503053;Y.Hayato et al.,Letter of Intent.[26]S.Antusch,S.F.King and R.N.Mohapatra,Phys.Lett.B618,150(2005).[27]W.Winter,Phys.Lett.B659,275(2008).[28]K.S.Babu and S.M.Barr,Phys.Rev.D48,5354(1993);K.Kurosawa,N.Maru and T.Yanagida,Phys.Lett.B 512,203(2001).[29]L.M.Krauss and F.Wilczek,Phys.Rev.Lett.62,1221(1989).[30]F.Plentinger and G.Seidl,in preparation.。
Reservoir Computing Approaches toRecurrent Neural Network Training
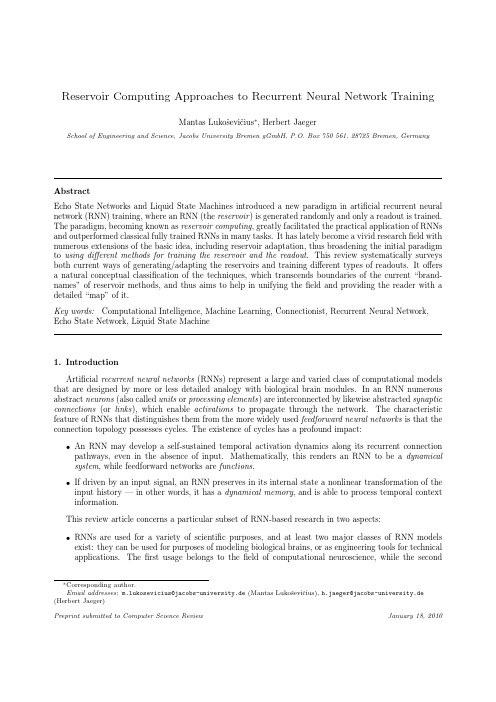
1. Introduction Artificial recurrent neural networks (RNNs) represent a large and varied class of computational models that are designed by more or less detailed analogy with biological brain modules. In an RNN numerous abstract neurons (also called units or processing elements ) are interconnected by likewise abstracted synaptic connections (or links ), which enable activations to propagate through the network. The characteristic feature of RNNs that distinguishes them from the more widely used feedforward neural networks is that the connection topology possesses cycles. The existence of cycles has a profound impact: • An RNN may develop a self-sustained temporal activation dynamics along its recurrent connection pathways, even in the absence of input. Mathematically, this renders an RNN to be a dynamical system, while feedforward networks are functions. • If driven by an input signal, an RNN preserves in its internal state a nonlinear transformation of the input history — in other words, it has a dynamical memory, and is able to process temporal context information. This review article concerns a particular subset of RNN-based research in two aspects: • RNNs are used for a variety of scientific purposes, and at least two major classes of RNN models exist: they can be used for purposes of modeling biological brains, or as engineering tools for technical applications. The first usage belongs to the field of computational neuroscience, while the second
paritycheck函数 -回复
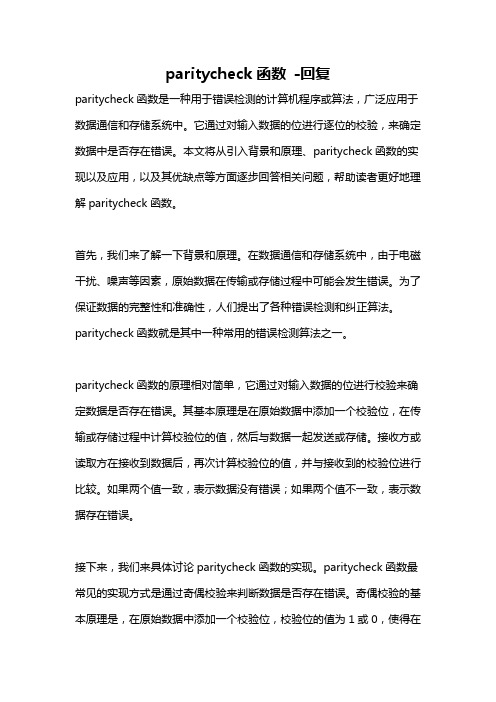
paritycheck函数-回复paritycheck函数是一种用于错误检测的计算机程序或算法,广泛应用于数据通信和存储系统中。
它通过对输入数据的位进行逐位的校验,来确定数据中是否存在错误。
本文将从引入背景和原理、paritycheck函数的实现以及应用,以及其优缺点等方面逐步回答相关问题,帮助读者更好地理解paritycheck函数。
首先,我们来了解一下背景和原理。
在数据通信和存储系统中,由于电磁干扰、噪声等因素,原始数据在传输或存储过程中可能会发生错误。
为了保证数据的完整性和准确性,人们提出了各种错误检测和纠正算法。
paritycheck函数就是其中一种常用的错误检测算法之一。
paritycheck函数的原理相对简单,它通过对输入数据的位进行校验来确定数据是否存在错误。
其基本原理是在原始数据中添加一个校验位,在传输或存储过程中计算校验位的值,然后与数据一起发送或存储。
接收方或读取方在接收到数据后,再次计算校验位的值,并与接收到的校验位进行比较。
如果两个值一致,表示数据没有错误;如果两个值不一致,表示数据存在错误。
接下来,我们来具体讨论paritycheck函数的实现。
paritycheck函数最常见的实现方式是通过奇偶校验来判断数据是否存在错误。
奇偶校验的基本原理是,在原始数据中添加一个校验位,校验位的值为1或0,使得在传输或存储过程中原始数据中的1的个数是奇数或偶数。
接收方在接收到数据后,再次计算数据中1的个数,如果是奇数,则校验位的值与原始数据一致,数据没有错误;如果是偶数,则校验位的值与原始数据不一致,数据存在错误。
通过实现paritycheck函数,我们可以很容易地判断数据中是否存在错误。
以下是一个简单的paritycheck函数的实现示例:pythondef paritycheck(data):# 计算原始数据中1的个数count = 0for bit in data:if bit == 1:count += 1# 判断校验位的值if count 2 == 0:return "No error"else:return "Error detected"以上示例中,我们使用一个循环来计算原始数据中1的个数,然后通过计算结果来判断校验位的值,从而确定数据是否存在错误。
文献外部特征的检索语言

文献外部特征的检索语言外部特征的检索语言是指在文献检索中使用的特定术语或关键词,用于寻找与特定主题或研究领域相关的外部特征的文献。
这些外部特征可能涉及物体表面的形态、纹理、颜色、特定结构或其他性质,或者涉及人体的某些特定特征或行为。
以下是一些与外部特征相关的常用检索语言的示例:1. 表面形态特征:- 均匀性(uniformity)- 曲率(curvature)- 平滑度(smoothness)- 粗糙度(roughness)- 几何形状(geometric shape)- 表面形貌(surface topography)- 表面形态(surface morphology)2. 表面纹理特征:- 纹理特征(texture features)- 纹理描述(texture descriptors)- 纹理分析(texture analysis)- 纹理判别(texture discrimination)- 纹理识别(texture recognition)- 纹理提取(texture extraction)- 纹理模型(texture model)- 纹理分类(texture classification)3. 表面颜色特征:- 颜色特征(color features)- 颜色分布(color distribution)- 颜色模型(color model)- 颜色空间(color space)- 颜色直方图(color histogram)- 颜色特征提取(color feature extraction)- 颜色描述符(color descriptor)4. 特定结构特征:- 细胞结构(cellular structure)- 晶格结构(lattice structure)- 分子结构(molecular structure)- 生物组织结构(biological tissue structure)- 表表面结构(surface structure)- 微观结构(microstructure)5. 人体特征:- 人脸特征(facial features)- 身体形态(body shape)- 手部特征(hand features)- 骨骼结构(skeletal structure)- 步态分析(gait analysis)- 视觉注意(visual attention)- 姿势识别(pose recognition)- 表情识别(facial expression recognition)以上仅是外部特征检索语言的示例,实际应用中需要根据具体的研究领域和研究目的进行调整和进一步扩展。
写作业不正确的方式英语

1.Procrastination:Putting off the task until the last minute,which can lead to rushed and poorly thoughtout work.ck of Preparation:Starting the assignment without fully understanding the topic or requirements,resulting in confusion and errors.3.Distraction:Allowing external factors such as social media,television,or noisy environments to divert attention from the task at hand.4.Poor Time Management:Not allocating sufficient time for research,drafting,and revising the work,leading to incomplete or hastily done assignments.5.Copying from Others:Plagiarizing or copying work from classmates or online sources, which is unethical and can lead to academic penalties.6.Irrelevant Information:Including information in the assignment that does not pertain to the topic or question,which can dilute the focus and quality of the work.ck of Citation:Failing to properly cite sources,which can lead to accusations of plagiarism and a lack of credibility in the work.8.Poor Grammar and Spelling:Submitting work with numerous grammatical and spelling errors,which can detract from the overall quality and professionalism of the assignment.9.Inadequate Research:Relying on superficial or insufficient research,which can result in a lack of depth and understanding in the assignment.10.Not Following Instructions:Ignoring specific instructions given by the teacher or professor,such as formatting requirements,word count,or specific topics to be covered.11.Rushing the Conclusion:Ending the assignment abruptly or without a proper conclusion,which can leave the reader with an incomplete understanding of the argument or analysis.12.Overloading with Jargon:Using too much technical language or jargon without proper explanation,which can make the work difficult to understand for the intended audience.13.Not Proofreading:Failing to proofread the work for errors or inconsistencies beforesubmission,which can lead to a lower grade due to avoidable mistakes.ck of Personal Insight:Writing an assignment that lacks original thought or personal interpretation,making it generic and less engaging.15.Inappropriate Tone:Using an informal or overly casual tone in an academic assignment,which can undermine the seriousness and credibility of the work.。
实例讲解一篇大牛制定的检索策略
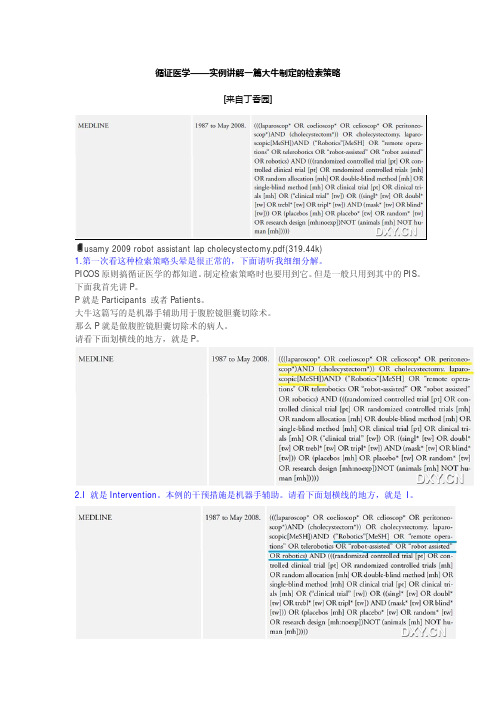
循证医学——实例讲解一篇大牛制定的检索策略[来自丁香园]us2.I 就是Intervention。
本例的干预措施是机器手辅助。
请看下面划横线的地方,就是I。
3. S 就是Study Design。
Cochrane规定一般只能纳入RCTs进行分析,所以要用到筛查RCTs 的过滤器(search filter)请看下面划横线部分就是S。
是不是头晕嘛,不急,待会教你它是怎么来的,不难的。
4.不经要问,大牛能做出这个来,但是假如我是初学者,我怎么做得出这么复杂的检索策略。
其实不难,我们再细细分解。
首先分解P。
((laparoscop* OR coelioscop* OR celioscop* OR peritoneoscop*)AND (cholecystectom*)) OR cholecystectomy, laparoscopic[MeSH])。
上述是怎么来的呢?首先我要讲讲自由词、关键词[tiab] title 和 abstract 的缩写、主题词[MeSH]。
laparoscop*这种就是自由词,后面没有加限制,至于*就是后面跟随意字母的意思。
cholecystectomy[tiab] 这种就是关键词,表示 cholecystectomy要在标题和摘要里有。
cholecystectomy, laparoscopic[MeSH]就是主题词,主题词是PubMed的专家给每篇文章标志的。
有人给我说这么麻烦,我就用关键词检索就行了,何必主题词呢?我反问了一句,那这些专家是不是吃饱了撑的,给每篇标主题词?主题词最大的好处是更容易查全查准,系统评价就是要集合目前世界上所有相关证据,查得越全越好。
查的全就是敏感性要高,但是要牺牲特异性,查得太多结果出来,以后筛查工作量大死你。
主题词就是协调这个的,查得既全又准,何乐而不为呢!5.又有人要问了,主题词这么好,那大牛为什么还要用自由词呢?以前我也不知道,最近才明白。
Peters (2010) Episodic Future Thinking Reduces Reward Delay Discounting
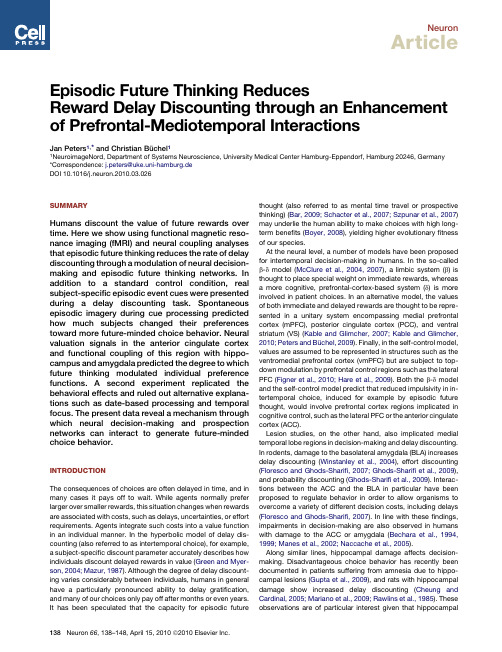
NeuronArticleEpisodic Future Thinking ReducesReward Delay Discounting through an Enhancement of Prefrontal-Mediotemporal InteractionsJan Peters1,*and Christian Bu¨chel11NeuroimageNord,Department of Systems Neuroscience,University Medical Center Hamburg-Eppendorf,Hamburg20246,Germany*Correspondence:j.peters@uke.uni-hamburg.deDOI10.1016/j.neuron.2010.03.026SUMMARYHumans discount the value of future rewards over time.Here we show using functional magnetic reso-nance imaging(fMRI)and neural coupling analyses that episodic future thinking reduces the rate of delay discounting through a modulation of neural decision-making and episodic future thinking networks.In addition to a standard control condition,real subject-specific episodic event cues were presented during a delay discounting task.Spontaneous episodic imagery during cue processing predicted how much subjects changed their preferences toward more future-minded choice behavior.Neural valuation signals in the anterior cingulate cortex and functional coupling of this region with hippo-campus and amygdala predicted the degree to which future thinking modulated individual preference functions.A second experiment replicated the behavioral effects and ruled out alternative explana-tions such as date-based processing and temporal focus.The present data reveal a mechanism through which neural decision-making and prospection networks can interact to generate future-minded choice behavior.INTRODUCTIONThe consequences of choices are often delayed in time,and in many cases it pays off to wait.While agents normally prefer larger over smaller rewards,this situation changes when rewards are associated with costs,such as delays,uncertainties,or effort requirements.Agents integrate such costs into a value function in an individual manner.In the hyperbolic model of delay dis-counting(also referred to as intertemporal choice),for example, a subject-specific discount parameter accurately describes how individuals discount delayed rewards in value(Green and Myer-son,2004;Mazur,1987).Although the degree of delay discount-ing varies considerably between individuals,humans in general have a particularly pronounced ability to delay gratification, and many of our choices only pay off after months or even years. It has been speculated that the capacity for episodic future thought(also referred to as mental time travel or prospective thinking)(Bar,2009;Schacter et al.,2007;Szpunar et al.,2007) may underlie the human ability to make choices with high long-term benefits(Boyer,2008),yielding higher evolutionaryfitness of our species.At the neural level,a number of models have been proposed for intertemporal decision-making in humans.In the so-called b-d model(McClure et al.,2004,2007),a limbic system(b)is thought to place special weight on immediate rewards,whereas a more cognitive,prefrontal-cortex-based system(d)is more involved in patient choices.In an alternative model,the values of both immediate and delayed rewards are thought to be repre-sented in a unitary system encompassing medial prefrontal cortex(mPFC),posterior cingulate cortex(PCC),and ventral striatum(VS)(Kable and Glimcher,2007;Kable and Glimcher, 2010;Peters and Bu¨chel,2009).Finally,in the self-control model, values are assumed to be represented in structures such as the ventromedial prefrontal cortex(vmPFC)but are subject to top-down modulation by prefrontal control regions such as the lateral PFC(Figner et al.,2010;Hare et al.,2009).Both the b-d model and the self-control model predict that reduced impulsivity in in-tertemporal choice,induced for example by episodic future thought,would involve prefrontal cortex regions implicated in cognitive control,such as the lateral PFC or the anterior cingulate cortex(ACC).Lesion studies,on the other hand,also implicated medial temporal lobe regions in decision-making and delay discounting. In rodents,damage to the basolateral amygdala(BLA)increases delay discounting(Winstanley et al.,2004),effort discounting (Floresco and Ghods-Sharifi,2007;Ghods-Sharifiet al.,2009), and probability discounting(Ghods-Sharifiet al.,2009).Interac-tions between the ACC and the BLA in particular have been proposed to regulate behavior in order to allow organisms to overcome a variety of different decision costs,including delays (Floresco and Ghods-Sharifi,2007).In line with thesefindings, impairments in decision-making are also observed in humans with damage to the ACC or amygdala(Bechara et al.,1994, 1999;Manes et al.,2002;Naccache et al.,2005).Along similar lines,hippocampal damage affects decision-making.Disadvantageous choice behavior has recently been documented in patients suffering from amnesia due to hippo-campal lesions(Gupta et al.,2009),and rats with hippocampal damage show increased delay discounting(Cheung and Cardinal,2005;Mariano et al.,2009;Rawlins et al.,1985).These observations are of particular interest given that hippocampal138Neuron66,138–148,April15,2010ª2010Elsevier Inc.damage impairs the ability to imagine novel experiences (Hassa-bis et al.,2007).Based on this and a range of other studies,it has recently been proposed that hippocampus and parahippocam-pal cortex play a crucial role in the formation of vivid event repre-sentations,regardless of whether they lie in the past,present,or future (Schacter and Addis,2009).The hippocampus may thus contribute to decision-making through its role in self-projection into the future (Bar,2009;Schacter et al.,2007),allowing an organism to evaluate future payoffs through mental simulation (Johnson and Redish,2007;Johnson et al.,2007).Future thinking may thus affect intertemporal choice through hippo-campal involvement.Here we used model-based fMRI,analyses of functional coupling,and extensive behavioral procedures to investigate how episodic future thinking affects delay discounting.In Exper-iment 1,subjects performed a classical delay discounting task(Kable and Glimcher,2007;Peters and Bu¨chel,2009)that involved a series of choices between smaller immediate and larger delayed rewards,while brain activity was measured using fMRI.Critically,we introduced a novel episodic condition that involved the presentation of episodic cue words (tags )obtained during an extensive prescan interview,referring to real,subject-specific future events planned for the respective day of reward delivery.This design allowed us to assess individual discount rates separately for the two experimental conditions,allowing us to investigate neural mechanisms mediating changes in delay discounting associated with episodic thinking.In a second behavioral study,we replicated the behavioral effects of Exper-iment 1and addressed a number of alternative explanations for the observed effects of episodic tags on discount rates.RESULTSExperiment 1:Prescan InterviewOn day 1,healthy young volunteers (n =30,mean age =25,15male)completed a computer-based delay discounting proce-dure to estimate their individual discount rate (Peters and Bu ¨-chel,2009).This discount rate was used solely for the purpose of constructing subject-specific trials for the fMRI session (see Experimental Procedures ).Furthermore,participants compiled a list of events that they had planned in the next 7months (e.g.,vacations,weddings,parties,courses,and so forth)andrated them on scales from 1to 6with respect to personal rele-vance,arousal,and valence.For each participant,seven subject-specific events were selected such that the spacing between events increased with increasing delay to the episode,and that events were roughly matched based on personal rele-vance,arousal,and valence.Multiple regression analysis of these ratings across the different delays showed no linear effects (relevance:p =0.867,arousal:p =0.120,valence:p =0.977,see Figure S1available online).For each subject,a separate set of seven delays was computed that was later used as delays in the control condition.Median and range for the delays used in each condition are listed in Table S1(available online).For each event,a label was selected that would serve as a verbal tag for the fMRI session.Experiment 1:fMRI Behavioral ResultsOn day 2,volunteers performed two sessions of a delay dis-counting procedure while fMRI was measured using a 3T Siemens Scanner with a 32-channel head-coil.In each session,subjects made a total of 118choices between 20V available immediately and larger but delayed amounts.Subjects were told that one of their choices would be randomly selected and paid out following scanning,with the respective delay.Critically,in half the trials,an additional subject-specific episodic tag (see above,e.g.,‘‘vacation paris’’or ‘‘birthday john’’)was displayed based on the prescan interview (see Figure 1)indicating which event they had planned on the particular day (episodic condi-tion),whereas in the remaining trials,no episodic tag was pre-sented (control condition).Amount and waiting time were thus displayed in both conditions,but only the episodic condition involved the presentation of an additional subject-specific event tag.Importantly,nonoverlapping sets of delays were used in the two conditions.Following scanning,subjects rated for each episodic tag how often it evoked episodic associations during scanning (frequency of associations:1,never;to 6,always)and how vivid these associations were (vividness of associa-tions:1,not vivid at all;to 6,highly vivid;see Figure S1).Addition-ally,written reports were obtained (see Supplemental Informa-tion ).Multiple regression revealed no significant linear effects of delay on postscan ratings (frequency:p =0.224,vividness:p =0.770).We averaged the postscan ratings acrosseventsFigure 1.Behavioral TaskDuring fMRI,subjects made repeated choices between a fixed immediate reward of 20V and larger but delayed amounts.In the control condi-tion,amounts were paired with a waiting time only,whereas in the episodic condition,amounts were paired with a waiting time and a subject-specific verbal episodic tag indicating to the subjects which event they had planned at the respective day of reward delivery.Events were real and collected in a separate testing session prior to the day of scanning.NeuronEpisodic Modulation of Delay DiscountingNeuron 66,138–148,April 15,2010ª2010Elsevier Inc.139and the frequency/vividness dimensions,yielding an‘‘imagery score’’for each subject.Individual participants’choice data from the fMRI session were then analyzed byfitting hyperbolic discount functions to subject-specific indifference points to obtain discount rates (k-parameters),separately for the episodic and control condi-tions(see Experimental Procedures).Subjective preferences were well-characterized by hyperbolic functions(median R2 episodic condition=0.81,control condition=0.85).Discount functions of four exemplary subjects are shown in Figure2A. For both conditions,considerable variability in the discount rate was observed(median[range]of discount rates:control condition=0.014[0.003–0.19],episodic condition=0.013 [0.002–0.18]).To account for the skewed distribution of discount rates,all further analyses were conducted on the log-trans-formed k-parameters.Across subjects,log-transformed discount rates were significantly lower in the episodic condition compared with the control condition(t(29)=2.27,p=0.016),indi-cating that participants’choice behavior was less impulsive in the episodic condition.The difference in log-discount rates between conditions is henceforth referred to as the episodic tag effect.Fitting hyperbolic functions to the median indifference points across subjects also showed reduced discounting in the episodic condition(discount rate control condition=0.0099, episodic condition=0.0077).The size of the tag effect was not related to the discount rate in the control condition(p=0.56). We next hypothesized that the tag effect would be positively correlated with postscan ratings of episodic thought(imagery scores,see above).Robust regression revealed an increase in the size of the tag effect with increasing imagery scores (t=2.08,p=0.023,see Figure2B),suggesting that the effect of the tags on preferences was stronger the more vividly subjects imagined the episodes.Examples of written postscan reports are provided in the Supplemental Results for participants from the entire range of imagination ratings.We also correlated the tag effect with standard neuropsychological measures,the Sensation Seeking Scale(SSS)V(Beauducel et al.,2003;Zuck-erman,1996)and the Behavioral Inhibition Scale/Behavioral Approach Scale(BIS/BAS)(Carver and White,1994).The tag effect was positively correlated with the experience-seeking subscale of the SSS(p=0.026)and inversely correlated with the reward-responsiveness subscale of the BIS/BAS scales (p<0.005).Repeated-measures ANOVA of reaction times(RTs)as a func-tion of option value(lower,similar,or higher relative to the refer-ence option;see Experimental Procedures and Figure2C)did not show a main effect of condition(p=0.712)or a condition 3value interaction(p=0.220),but revealed a main effect of value(F(1.8,53.9)=16.740,p<0.001).Post hoc comparisons revealed faster RTs for higher-valued options relative to similarly (p=0.002)or lower valued options(p<0.001)but no difference between lower and similarly valued options(p=0.081).FMRI DataFMRI data were modeled using the general linear model(GLM) as implemented in SPM5.Subjective value of each decision option was calculated by multiplying the objective amount of each delayed reward with the discount fraction estimated behaviorally based on the choices during scanning,and included as a parametric regressor in the GLM.Note that discount rates were estimated separately for the control and episodic conditions(see above and Figure2),and we thus used condition-specific k-parameters for calculation of the subjective value regressor.Additional parametric regressors for inverse delay-to-reward and absolute reward magnitude, orthogonalized with respect to subjective value,were included in theGLM.Figure2.Behavioral Data from Experiment1Shown are experimentally derived discount func-tions from the fMRI session for four exemplaryparticipants(A),correlation with imagery scores(B),and reaction times(RTs)(C).(A)Hyperbolicfunctions werefit to the indifference points sepa-rately for the control(dashed lines)and episodic(solid lines,filled circles)conditions,and thebest-fitting k-parameters(discount rates)and R2values are shown for each subject.The log-trans-formed difference between discount rates wastaken as a measure of the effect of the episodictags on choice preferences.(B)Robust regressionrevealed an association between log-differences indiscount rates and imagery scores obtained frompostscan ratings(see text).(C)RTs were signifi-cantly modulated by option value(main effectvalue p<0.001)with faster responses in trialswith a value of the delayed reward higher thanthe20V reference amount.Note that althoughseven delays were used for each condition,somedata points are missing,e.g.,onlyfive delay indif-ference points for the episodic condition areplotted for sub20.This indicates that,for the twolongest delays,this subject never chose the de-layed reward.***p<0.005.Error bars=SEM.Neuron Episodic Modulation of Delay Discounting140Neuron66,138–148,April15,2010ª2010Elsevier Inc.Episodic Tags Activate the Future Thinking NetworkWe first analyzed differences in the condition regressors without parametric pared to those of the control condi-tion,BOLD responses to the presentation of the delayed reward in the episodic condition yielded highly significant activations (corrected for whole-brain volume)in an extensive network of brain regions previously implicated in episodic future thinking (Addis et al.,2007;Schacter et al.,2007;Szpunar et al.,2007)(see Figure 3and Table S2),including retrosplenial cortex (RSC)/PCC (peak MNI coordinates:À6,À54,14,peak z value =6.26),left lateral parietal cortex (LPC,À44,À66,32,z value =5.35),and vmPFC (À8,34,À12,z value =5.50).Distributed Neural Coding of Subjective ValueWe then replicated previous findings (Kable and Glimcher,2007;Kable and Glimcher,2010;Peters and Bu¨chel,2009)using a conjunction analysis (Nichols et al.,2005)searching for regions showing a positive correlation between the height of the BOLD response and subjective value in the control and episodic condi-tions in a parametric analysis (Figure 4A and Table S3).Note that this is a conservative analysis that requires that a given voxel exceed the statistical threshold in both contrasts separately.This analysis revealed clusters in the lateral orbitofrontal cortex (OFC,À36,50,À10,z value =4.50)and central OFC (À18,12,À14,z value =4.05),bilateral VS (right:10,8,0,z value =4.22;left:À10,8,À6,z value =3.51),mPFC (6,26,16,z value =3.72),and PCC (À2,À28,24,z value =4.09),representing subjective (discounted)value in both conditions.We next analyzed the neural tag effect,i.e.,regions in which the subjective value correlation was greater for the episodic condi-tion as compared with the control condition (Figure 4B and Table S4).This analysis revealed clusters in the left LPC (À66,À42,32,z value =4.96,),ACC (À2,16,36,z value =4.76),left dorsolateral prefrontal cortex (DLPFC,À38,36,36,z value =4.81),and right amygdala (24,2,À24,z value =3.75).Finally,we performed a triple-conjunction analysis,testing for regions that were correlated with subjective value in both conditions,but in which the value correlation increased in the episodic condition.Only left LPC showed this pattern (À66,À42,30,z value =3.55,see Figure 4C and Table S5),the same region that we previously identified as delay-specific in valuation (Petersand Bu¨chel,2009).There were no regions in which the subjective value correlation was greater in the control condition when compared with the episodic condition at p <0.001uncorrected.ACC Valuation Signals and Functional Connectivity Predict Interindividual Differences in Discount Function ShiftsWe next correlated differences in the neural tag effect with inter-individual differences in the size of the behavioral tag effect.To this end,we performed a simple regression analysis in SPM5on the single-subject contrast images of the neural tag effect (i.e.,subjective value correlation episodic >control)using the behavioral tag effect [log(k control )–log(k episodic )]as an explana-tory variable.This analysis revealed clusters in the bilateral ACC (right:18,34,18,z value =3.95,p =0.021corrected,left:À20,34,20,z value =3.52,Figure 5,see Table S6for a complete list).Coronal sections (Figure 5C)clearly show that both ACC clusters are located in gray matter of the cingulate sulcus.Because ACC-limbic interactions have previously been impli-cated in the control of choice behavior (Floresco and Ghods-Sharifi,2007;Roiser et al.,2009),we next analyzed functional coupling with the right ACC from the above regression contrast (coordinates 18,34,18,see Figure 6A)using a psychophysiolog-ical interaction analysis (PPI)(Friston et al.,1997).Note that this analysis was conducted on a separate first-level GLM in which control and episodic trials were modeled as 10s miniblocks (see Experimental Procedures for details).We first identified regions in which coupling with the ACC changed in the episodic condition compared with the control condition (see Table S7)and then performed a simple regression analysis on these coupling parameters using the behavioral tag effect as an explanatory variable.The tag effect was associated with increased coupling between ACC and hippocampus (À32,À18,À16,z value =3.18,p =0.031corrected,Figure 6B)and ACC and left amygdala (À26,À4,À26,z value =2.95,p =0.051corrected,Figure 6B,see Table S8for a complete list of activa-tions).The same regression analysis in a second PPI with the seed voxel placed in the contralateral ACC region from the same regression contrast (À20,34,22,see above)yielded qual-itatively similar,though subthreshold,results in these same structures (hippocampus:À28,À32,À6,z value =1.96,amyg-dala:À28,À6,À16,z value =1.97).Experiment 2We conducted an additional behavioral experiment to address a number of alternative explanations for the observed effects of tags on choice behavior.First,it could be argued thatepisodicFigure 3.Categorical Effect of Episodic Tags on Brain ActivityGreater activity in lateral parietal cortex (left)and posterior cingulate/retrosplenial and ventro-medial prefrontal cortex (right)was observed in the episodic condition compared with the control condition.p <0.05,FWE-corrected for whole-brain volume.NeuronEpisodic Modulation of Delay DiscountingNeuron 66,138–148,April 15,2010ª2010Elsevier Inc.141tags increase subjective certainty that a reward would be forth-coming.In Experiment 2,we therefore collected postscan ratings of reward confidence.Second,it could be argued that events,always being associated with a particular date,may have shifted temporal focus from delay-based to more date-based processing.This would represent a potential confound,because date-associated rewards are discounted less than delay-associated rewards (Read et al.,2005).We therefore now collected postscan ratings of temporal focus (date-based versus delay-based).Finally,Experiment 1left open the question of whether the tag effect depends on the temporal specificity of the episodic cues.We therefore introduced an additional exper-imental condition that involved the presentation of subject-specific temporally unspecific future event cues.These tags (henceforth referred to as unspecific tags)were obtained by asking subjects to imagine events that could realistically happen to them in the next couple of months,but that were not directly tied to a particular point in time (see Experimental Procedures ).Episodic Imagery,Not Temporal Specificity,Reward Confidence,or Temporal Focus,Predicts the Size of the Tag EffectIn total,data from 16participants (9female)are included.Anal-ysis of pretest ratings confirmed that temporally unspecific and specific tags were matched in terms of personal relevance,arousal,valence,and preexisting associations (all p >0.15).Choice preferences were again well described by hyperbolic functions (median R 2control =0.84,unspecific =0.81,specific =0.80).We replicated the parametric tag effect (i.e.,increasing effect of tags on discount rates with increasing posttest imagery scores)in this independent sample for both temporally specific (p =0.047,Figure 7A)and temporally unspecific (p =0.022,Figure 7A)tags,showing that the effect depends on future thinking,rather than being specifically tied to the temporal spec-ificity of the event cues.Following testing,subjects rated how certain they were that a particular reward would actually be forth-coming.Overall,confidence in the payment procedure washighFigure 4.Neural Representation of Subjective Value (Parametric Analysis)(A)Regions in which the correlation with subjective value (parametric analysis)was significant in both the control and the episodic conditions (conjunction analysis)included central and lateral orbitofrontal cortex (OFC),bilateral ventral striatum (VS),medial prefrontal cortex (mPFC),and posterior cingulate cortex(PCC),replicating previous studies (Kable and Glimcher,2007;Peters and Bu¨chel,2009).(B)Regions in which the subjective value correlation was greater for the episodic compared with the control condition included lateral parietal cortex (LPC),ante-rior cingulate cortex (ACC),dorsolateral prefrontal cortex (DLPFC),and the right amygdala (Amy).(C)A conjunction analysis revealed that only LPC activity was positively correlated with subjective value in both conditions,but showed a greater regression slope in the episodic condition.No regions showed a better correlation with subjective value in the control condition.Error bars =SEM.All peaks are significant at p <0.001,uncorrected;(A)and (B)are thresholded at p <0.001uncorrected and (C)is thresholded at p <0.005,uncorrected for display purposes.NeuronEpisodic Modulation of Delay Discounting142Neuron 66,138–148,April 15,2010ª2010Elsevier Inc.(Figure 7B),and neither unspecific nor specific tags altered these subjective certainty estimates (one-way ANOVA:F (2,45)=0.113,p =0.894).Subjects also rated their temporal focus as either delay-based or date-based (see Experimental Procedures ),i.e.,whether they based their decisions on the delay-to-reward that was actually displayed,or whether they attempted to convert delays into the corresponding dates and then made their choices based on these dates.There was no overall significant effect of condition on temporal focus (one-way ANOVA:F (2,45)=1.485,p =0.237,Figure 7C),but a direct comparison between the control and the temporally specific condition showed a significant difference (t (15)=3.18,p =0.006).We there-fore correlated the differences in temporal focus ratings between conditions (control:unspecific and control:specific)with the respective tag effects (Figure 7D).There were no correlations (unspecific:p =0.71,specific:p =0.94),suggesting that the observed differences in discounting cannot be attributed to differences in temporal focus.High-Imagery,but Not Low-Imagery,Subjects Adjust Their Discount Function in an Episodic ContextFor a final analysis,we pooled the samples of Experiments 1and 2(n =46subjects in total),using only the temporally specific tag data from Experiment 2.We performed a median split into low-and high-imagery participants according to posttest imagery scores (low-imagery subjects:n =23[15/8Exp1/Exp2],imagery range =1.5–3.4,high-imagery subjects:n =23[15/8Exp1/Exp2],imagery range =3.5–5).The tag effect was significantly greater than 0in the high-imagery group (t (22)=2.6,p =0.0085,see Figure 7D),where subjects reduced their discount rate by onaverage 16%in the presence of episodic tags.In the low-imagery group,on the other hand,the tag effect was not different from zero (t (22)=0.573,p =0.286),yielding a significant group difference (t (44)=2.40,p =0.011).DISCUSSIONWe investigated the interactions between episodic future thought and intertemporal decision-making using behavioral testing and fMRI.Experiment 1shows that reward delay dis-counting is modulated by episodic future event cues,and the extent of this modulation is predicted by the degree of sponta-neous episodic imagery during decision-making,an effect that we replicated in Experiment 2(episodic tag effect).The neuroi-maging data (Experiment 1)highlight two mechanisms that support this effect:(1)valuation signals in the lateral ACC and (2)neural coupling between ACC and hippocampus/amygdala,both predicting the size of the tag effect.The size of the tag effect was directly related to posttest imagery scores,strongly suggesting that future thinking signifi-cantly contributed to this effect.Pooling subjects across both experiments revealed that high-imagery subjects reduced their discount rate by on average 16%in the episodic condition,whereas low-imagery subjects did not.Experiment 2addressed a number of alternative accounts for this effect.First,reward confidence was comparable for all conditions,arguing against the possibility that the tags may have somehow altered subjec-tive certainty that a reward would be forthcoming.Second,differences in temporal focus between conditions(date-basedFigure 5.Correlation between the Neural and Behavioral Tag Effect(A)Glass brain and (B and C)anatomical projection of the correlation between the neural tag effect (subjective value correlation episodic >control)and the behav-ioral tag effect (log difference between discount rates)in the bilateral ACC (p =0.021,FWE-corrected across an anatomical mask of bilateral ACC).(C)Coronal sections of the same contrast at a liberal threshold of p <0.01show that both left and right ACC clusters encompass gray matter of the cingulate gyrus.(D)Scatter-plot depicting the linear relationship between the neural and the behavioral tag effect in the right ACC.(A)and (B)are thresholded at p <0.001with 10contiguous voxels,whereas (C)is thresholded at p <0.01with 10contiguousvoxels.Figure 6.Results of the Psychophysiolog-ical Interaction Analysis(A)The seed for the psychophysiological interac-tion (PPI)analysis was placed in the right ACC (18,34,18).(B)The tag effect was associated with increased ACC-hippocampal coupling (p =0.031,corrected across bilateral hippocampus)and ACC-amyg-dala coupling (p =0.051,corrected across bilateral amygdala).Maps are thresholded at p <0.005,uncorrected for display purposes and projected onto the mean structural scan of all participants;HC,hippocampus;Amy,Amygdala;rACC,right anterior cingulate cortex.NeuronEpisodic Modulation of Delay DiscountingNeuron 66,138–148,April 15,2010ª2010Elsevier Inc.143。
不对称信息下的主题搜索信息服务策略——村镇信息主题搜索引擎的实验
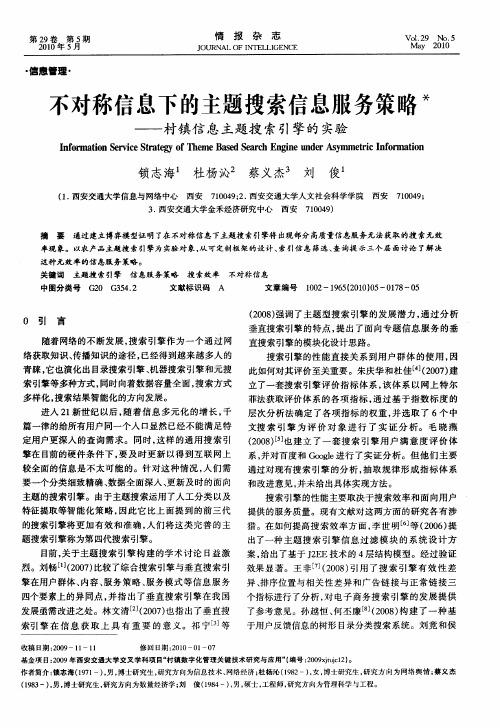
基金 项 目:0 9年 西安 交 通 大 学 交 叉 学 科项 目“ 镇 数宇 化 管 理 关 键 技术 研 究 与 应 用 ” 编 号 :0 9ju 1 ) 20 村 ( 2 0 xtj 2 。 c
作者简介 : 锁志海 (9 1 , , 17 一) 男 博士研究生 , 研究方 向为信息技术、 网络经济 ; 杜杨沁(9 2一)女 。 18 , 博士研究生 , 研究 方向为网络舆情 ; 蔡义杰
第
29 期 2 1 5月 0 年 0
情
报
杂
志
J OURN N L I NC AL OF I TE L GE E
Vo . 9 No 5 12 . M a 2 1 y 00
・
信息 管理 ・
不对称信息下的主题搜索信息服务策略 *
— —
村镇 信 息主题搜 索 引 擎的 实验
( . 安交通大学信息与网络中心 西安 1西 704 ;. 10 9 2 西安交通大学人文社 会科学学 院 西安 7 0 4 ; 10 9
西安 704 ) 1 09 3 西安交通大学金禾 经济研究 中心 .
摘
要
通过建立博 弈模型证 明了在不对称信 息下主题搜 索引擎将 出现部 分高质量信息服务无法获取 的搜 索无效
烈 。刘畅u (0 7 比较了综合搜索 引擎 与垂直搜 索引 2 0 ) J
擎在用户群体、 内容、 服务 策略 、 服务模式等信 息服 务 异 、 排序位置 与相关性差 异和广告链 接 与正常 链接三
四个要素上 的异 同点 , 并指 出 了垂 直搜索 引擎 在我 国 发展亟需改进之处 。林 文清_ (0 7 也指 出了垂主要 og e
随着网络的不断发展 , 索 引擎 作为一 个通过 网 搜 络获取知识 、 传播知识的途径 , 已经得到越来越 多人 的 青 睐, 它也演化 出 目录搜索引擎 、 机器搜索 引擎 和元搜 索 引擎等多种方式 , 同时向着数据容量全面 , 搜索方式 多样化 , 搜索结果智能化的方 向发展 。 篇一律的给所有用户同一个人 口显然 已经不能满足特 定用户更深入 的查询需求 。同时 , 这样 的通 用搜 索引
- 1、下载文档前请自行甄别文档内容的完整性,平台不提供额外的编辑、内容补充、找答案等附加服务。
- 2、"仅部分预览"的文档,不可在线预览部分如存在完整性等问题,可反馈申请退款(可完整预览的文档不适用该条件!)。
- 3、如文档侵犯您的权益,请联系客服反馈,我们会尽快为您处理(人工客服工作时间:9:00-18:30)。
a r X i v :h e p -p h /9904397v 1 19 A p r 1999Search for R-parity Violating SUSY in Run 2at DØS.Banerjee,N.K.Mondal,V.S.Narasimham,and N.ParuaTata Institute of Fundamental Research,Bombay,IndiaMarch 25,1999Abstract.We present a study of sensitivity of the R-parity violating SUSY searches with the upgraded DØdetector in Run II of the Fermilab Tevatron,within a SUGRA framework.We considered the lightest neutralino as an LSP that decays into a lepton and slepton (R-parity violating decay),resulting in 2e +≥4jets or 2µ+≥4jets final state.The analysis,based on scaling of the Run I results,shows that squarks and gluinos with masses up to about 0.6TeV could be probed with 2fb −1of Run II data.This work has been done in the context of the BTMSSM Working Group of the Run II SUSY/Higgs Workshop at Fermilab.I PHYSICS MOTIVATION Recent interest in R-parity violating (RPV)SUSY decay modes is motivated by the possible high-Q 2event excess at HERA [1].When interpretation of the excess through first-generation leptoquarks was excluded by the DØ[2]and CDF [3]experiments,it was suggested [4]that such an effect could be explained via the s -channel production of a charm or top squark decaying into the e +jet final state.Both the production and the decay vertices would thereby violate R-parity.Although more recent data has not confirmed the previous event excess,and despite the combined analysis showed that the anomalous events reported by the H1and ZEUS experiments were unlikely to originate from the production of a single s -channel narrow resonance [5],interest in RPV signatures has not abatted.The CDF and DØCollaborations have recently performed searches for RPV SUSY [7,8],and have set new mass limits on the RPV SUSY particles.Both experiments focussed their searches on the λ′couplings,as motivated by the high-Q 2HERA event excess.The results of the DØsearches are extended to the Run 2case and the expected sensitivity to the RPV couplings is discussed.II DØSEARCH FOR RPV NEUTRALINO DECAYS The DØsearch for RPV SUSY considered the case of neutralino LSP which decays into a lepton and two quarks due to a finite RPV λ′coupling (see Fig.1).Both the electron and muon decay channels were considered,corresponding to what commonly referred to as λ′1ij and λ′2ij couplings,respectively.The corresponding final states contain either2e or 2µand at least four accompanying jets.Unlike at HERA,this search is not sensitive to the value of the RPV coupling,as long as it is large enough so that the neutralino decays within the DØdetector.That corresponds to λ′≥10−3,which gives a lot of room,given current indirect constraints [6].χ10∼l–l ∼qq ′λFIGURE 1.RPV decay of a neutralino LSP into a lepton and two quarks.We assume that the neutralino(LSP)pairs are produced in cascade decays of other supersymmetric particles and use all SUSY pair production mechanisms when generating signal events.Signal events were generated within the SUGRA framework with the following values of SUSY parameters:A0=0,µ<0and tanβ=2(the results are not sensitive to the value of A0.)Center of mass energy of the colliding beams was taken to be2TeV.ISAJET[9]was used for event generation.The acceptance and resolution of the DØdetector√were parametrized using the following resolutions:δE/E=2%⊕15%/E[GeV](jets)and found consistent with the full detector simulation based on GEANT[10].FIGURE2.Points in the(m0,m1/2)SUGRA parameter space used to generate RPV events in the ee+4jets channel.Figure2shows the points in the(m0,m1/2)SUGRA parameter space where signal Monte Carlo events were generated for the electron channel.Similar points were studied for the muon-decay channel.III SELECTION CRITERIA FOR THE DIELECTRON CHANNELA multijet trigger was used for the analysis of Run1data.It was found to be nearly100%efficient for the typical RPV signal.Since Run2trigger list will include a similar trigger,we assume trigger efficiency of100%and do not perform any trigger simulations for the Run2.The following offline selections were used:•At least two good electrons,the leading one with E T(e)>15GeV and the other one with E T(e)>10GeV;•Rapidity range|η|≤1.1(central calorimeter),or1.5≤|η|≤2.5(end calorimeters)for all the electrons;•Energy isolation for the electrons:the EM energy in the R=0.2cone about the center of gravity of the EM cluster,subtracted from the total energy in R=0.4cone,should not exceed15%of the EM energy in the R-0.2 cone.•At least four jets with E T(j)>15GeV and|η|<2.5;•The dielectron invariant mass(M ee)should not be in the Z-mass interval,ie,|M ee−M Z|>15GeV/c2.In the present analysis we have dropped the requirement on H T= E T(e)+ E T(j),but retained all other offline criteria that were used in the previous analysis of data from Run I[8].IV SELECTION IN THE DIMUON CHANNELThe following event selection requirements were used for the muon decay channel:•Two muons,the leading one with p T>15GeV,and the other one with p T>10GeV.•Rapidity range|η|<2.3for both muons.•Energy isolation requirement for both muons,i.e.the calorimeter energy accompanying the muon in a(ηφ) cone of0.4should be consistent with that from a minimum ionising particle.•At least four jets with E T(j)>15GeV and|η|<2.5;V SIGNAL EFFICIENCIESThe number of signal events expected can be written as: N =L·σ·ǫ,where N is the expected number of events for luminosity L,σis the cross-section,andǫis the overall efficiency.The efficiencyǫcan be split into three terms:ǫ=ǫtrig·ǫkin·ǫid.Hereǫtrig is the trigger efficiency for the events that pass the offline cuts(assumed to be 100%),ǫkin is the efficiency for offline criteria,which includes kinematic,fiducial and topological requirements,and ǫid is the electron/jet identification efficiency.The efficiency for identifying jets is very high(>95%)and is expected to stay the same in Run2.Electron identification efficiencies in Run1were80±7%in the central(|η|<1.1)and71±7%in the forward (1.5<|η|<2.5)regions[8].These efficiencies were calculated for electrons with E T(e)>25GeV,It drops by about 30%for electrons with E T(e)=10GeV.The muon identification efficiencies used in Run1were62±2%in the central(|η|<1.0)and24±4%in the forward(1.0<|η|<1.7)regions[11].These were calculated for muons with p T>15GeV.For muons with 10GeV<p T<15GeV the efficiencies were80%smaller on average[12].In the present analysis we have taken the overall particle identification efficiency to be0.90±0.09in each channel, independent of lepton E T,primarily due to the expectation of a better tracker and muon spectrometer for the upgraded DØexperiment.VI BACKGROUNDSThe main backgrounds are expected to arise from Drell-Yan production in association with four or more jets, dilepton top-quark events,and QCD multijet events.The latter is the dominant background for the electron channel (followed by the Drell-Yan background).In the case of muons,the background is dominated by the Drell-Yan and top pair production.We used Monte Carlo to calculate background from thefirst two sources,and data to estimate background from QCD jets.Background for the Run1analysis was estimated to be1.8±0.2±0.3(with1.27±0.24from QCD and0.42±0.15±0.16from the other processes)for∼100pb−1of data.To extrapolate this number to the data set from Run2, we have simply multiplied it by the ratio of luminosities to obtain36±4±6events.However,it is expected that due to the central magneticfield in the upgraded DØdetector,the probability of jets to be misidentified as electrons will be reduced by a factor of∼2in Run2.We have therefore considered a second scenario with the smaller expected background of15±1.5±1.5events.For the muon channel,the expected background has been scaled directly from the Run1analysis.We expect 10±1±1background events in Run2.VII RESULTSIn order to obtain the sensitivity of Run2in to RPV decays,we calculated the efficiency for signal for all the mass points shown in Fig.2.Typical efficiencies,the signal cross section in the ee+4jets channel,and the expected event yield in2fb−1of data,for several representative(m0,m1/2)points,are given in Table VII.Similar numbers are obtained for the muon channel.We use these efficiencies to obtain exclusion limits in the(m0,m1/2)plane at95%CL,assuming that no excess of events will be observed above the predicted background.The exclusion contours for the electron and muon channel are shown in Fig.3and4,respectively.Numerical values of the limits are summarized in Table VII.TABLE1.Efficiency×BR(%),signal cross section and the expectedevent yield in2fb−1of data,at various(m0,m1/2)parameter space points.m0Efficiency×BR(%) N(GeV)(in2fb−1)2350.162450.082550.062200.102300.082400.052400.05TABLE2.Lower limits on the squark and gluino masses from Run2.Lower limit on m˜g(For any m˜g)Run1232GeV430GeV490GeV Run2(Scenario II)575GeVMuons560GeV665GeVp Collisions at√FIGURE3.Estimated exclusion contour for Run2in the(m0,m1/2)plane for tanβ=2,A0=0,µ<0,from the ee+4jets channel.Scenario I corresponds to a background of36±4±6events(direct scaling from Run1);scenario II uses thebackground of15±1.5±1.5events(scaling,but with improvements in the detector taken into account).FIGURE4.Estimated exclusion contour for Run2in the(m0,m1/2)plane for tanβ=2,A0=0,µ<0,from theµµ+4jets channel for background of10±1.0±1.0(direct scaling from Run1).。