Unusual thermal stability of nano-structured ferritic alloys
微纳结构沸腾表面构建及传热性能的研究进展
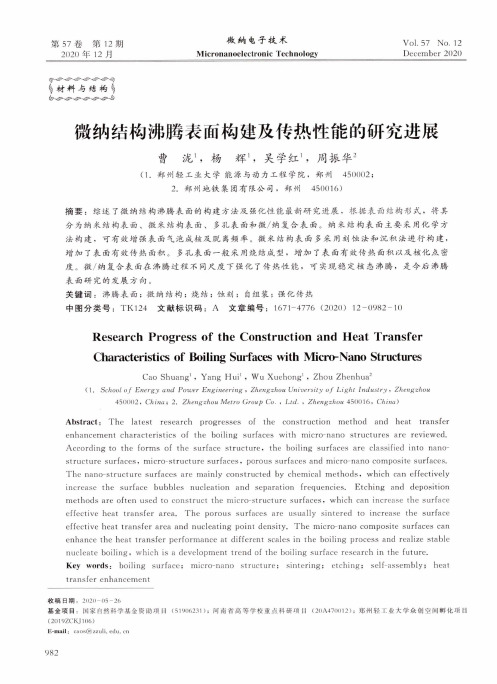
第57卷第12期 2020年12月撳纳电子技术Micronanoelectronic TechnologyVol.57 No. 12December 2020微纳结构沸腾表面构建及传热性能的研究进展曹泷1,杨辉S吴学红\周振华2(1.郑州轻工业大学能源与动力工程学院,郑州 450002;2.郑州地铁集团有限公司,郑州 450016)摘要:综述了微纳结构沸腾表面的构建方法及强化性能最新研究进展,根据表面结构形式,将其 分为纳米结构表面、微米结构表面、多孔表面和微/纳复合表面。
纳米结构表面主要采用化学方法构建,可有效增强表面气泡成核及脱离频率。
微米结构表面多采用刻蚀法和沉积法进行构建,增加了表面有效传热面积。
多孔表面一般采用烧结成型,增加了表面有效传热面积以及核化点密 度。
微/纳复合表面在沸腾过程不同尺度下强化了传热性能,可实现稳定核态沸腾,是今后沸腾 表面研究的发展方向。
关键词:沸腾表面;微纳结构;烧结;蚀刻;自组装;强化传热中图分类号:T K124 文献标识码:A文章编号:1671-4776 (2020) 12-(>982-10Research Progress of the Construction and Heat TransferCharacteristics of Boiling Surfaces with Micro-Nano StructuresCao Shuang1 ,Yang Hui1 ,Wu Xuehong1 ,Zhou Zhenhua2(1. School o f E nergy and Puzver E ngineering, Zhengzhou U niversity o f L ig h t Industry Zhengzhou450002, C hina;2. Zhengzhou M etro Group Co., Ltd., Zhengzhou450016, China)Abstract:The latest research progresses of the construction method and heat transfer enhancement characteristics of the boiling surfaces with micro-nano structures are reviewed.According to the forms of the surface structure,the boiling surfaces are classified into nanostructure surfaces,micro-structure surfaces,porous surfaces and micro-nano composite surfaces.The nano-structure surfaces are mainly constructed by chemical m ethods,which can effectively increase the surface bubbles nucleation and separation frequencies.Etching and deposition methods are often used to construct the micro-structure surfaces,which can increase the surface effective heat transfer area.The porous surfaces are usually sintered to increase the surface effective heat transfer area and nucleating point density.The micro-nano composite surfaces can enhance the heat transfer performance at different scales in the boiling process and realize stable nucleate boiling,which is a development trend of the boiling surface research in the future.Key words:boiling surface;micro-nano structure;sintering;etching;self-assembly;heat transfer enhancement收稿日期:2020-05-26基金项目:国家自然科学基金资助项目(51(川6231);河南省髙等学校重点科研项目(2()A47()(n2);郑州轻工业大学众创空间孵化项目(2019ZCK J106)E-mail :caos@982曹泷等:微纳结构沸腾表面构建及传热性能的研究进展DOI:10. 13250/ki.wndz.2020. 12. 005 PACC:4430()引百沸腾传热作为一种伴随着气液相变的高效能量 传递方式,具有传热温差小和热流密度大等特点,由于微电子器件小型化及集成度高的发展特点,其 对高效散热方式也提出了较高的要求,相应地沸腾 传热的应用将具有巨大的优势。
二硫化钛纳米片材料用于光声成像指导下的肿瘤光热治疗研究
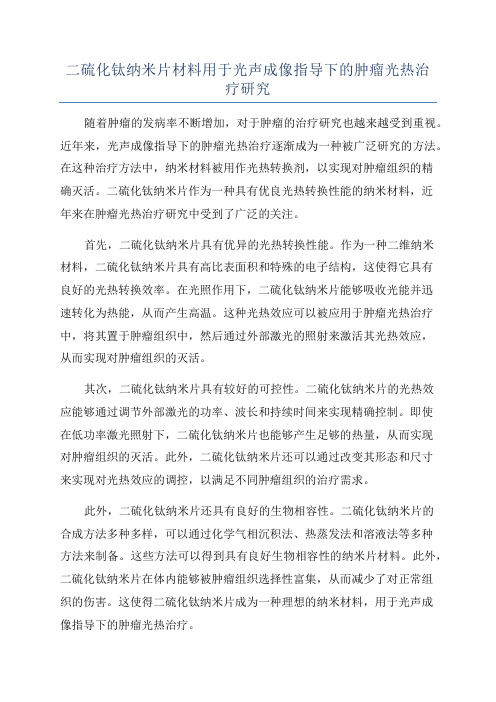
二硫化钛纳米片材料用于光声成像指导下的肿瘤光热治疗研究随着肿瘤的发病率不断增加,对于肿瘤的治疗研究也越来越受到重视。
近年来,光声成像指导下的肿瘤光热治疗逐渐成为一种被广泛研究的方法。
在这种治疗方法中,纳米材料被用作光热转换剂,以实现对肿瘤组织的精确灭活。
二硫化钛纳米片作为一种具有优良光热转换性能的纳米材料,近年来在肿瘤光热治疗研究中受到了广泛的关注。
首先,二硫化钛纳米片具有优异的光热转换性能。
作为一种二维纳米材料,二硫化钛纳米片具有高比表面积和特殊的电子结构,这使得它具有良好的光热转换效率。
在光照作用下,二硫化钛纳米片能够吸收光能并迅速转化为热能,从而产生高温。
这种光热效应可以被应用于肿瘤光热治疗中,将其置于肿瘤组织中,然后通过外部激光的照射来激活其光热效应,从而实现对肿瘤组织的灭活。
其次,二硫化钛纳米片具有较好的可控性。
二硫化钛纳米片的光热效应能够通过调节外部激光的功率、波长和持续时间来实现精确控制。
即使在低功率激光照射下,二硫化钛纳米片也能够产生足够的热量,从而实现对肿瘤组织的灭活。
此外,二硫化钛纳米片还可以通过改变其形态和尺寸来实现对光热效应的调控,以满足不同肿瘤组织的治疗需求。
此外,二硫化钛纳米片还具有良好的生物相容性。
二硫化钛纳米片的合成方法多种多样,可以通过化学气相沉积法、热蒸发法和溶液法等多种方法来制备。
这些方法可以得到具有良好生物相容性的纳米片材料。
此外,二硫化钛纳米片在体内能够被肿瘤组织选择性富集,从而减少了对正常组织的伤害。
这使得二硫化钛纳米片成为一种理想的纳米材料,用于光声成像指导下的肿瘤光热治疗。
综上所述,二硫化钛纳米片作为一种具有优异光热转换性能、可控性和生物相容性的纳米材料,有望在光声成像指导下的肿瘤光热治疗中发挥重要的作用。
未来的研究可以进一步探索二硫化钛纳米片的制备方法和生物效应,以期实现其在临床肿瘤治疗中的应用。
倏逝波模式下修饰化电极的太阳电池层厚优化

电转 化效 率 姗。此外 , A l 电极 涂 布 HF上 能提 高 电 池填 充 因子 和稳 定 开路 电压 , 修饰 层 厚度 不 同会 产 生不 同的效 果 [ 5 , 7 , 8 , 1 3 1 。对 比 L i F和 N a F发 现 。 N a F修 饰 层 对 电池 光 电 转 换 效 率 提 高 更 为 理 想 3 1 , 而 且 薄膜 层 厚 组 合 也会 影 响 到有 机 太 阳 电池 光 电 转换
间光 强 分 布 理 论 模 型 。 根 据 多层 薄 膜 结 构 的 有机 太 阳 能 电 池 的材 料 及 光 学特 性 . 采 用 绒 化 入 射 面 的 方 法 引入 朗伯 漫
反 射 ,并 结 合 对 倏 逝 波 模 式 下 有 机 太 阳能 电池 光 捕 获 率 的 探 讨 ,通 过 数 值 计 算研 究 了 Na F修 饰 层 对 光 场 分布 的影 响 ,提 高 了模 型 的 光 捕 获 并得 出 了有机 太 阳能 电池 捕 获 可 见 太 阳 光 能 最 大 值 时各 层 厚 参 数 G l m s( 1 m m) / I T O( 1 2 0 mn ) / P E DOT: P S S ( 2 0 n m) / MDMO— P P V : P C B M( 1 2 0 n m) / Na F ( 1 n m) /  ̄ f 1 1 0 i r m) 和 电池的短路 电流为 2 . 7 6 mA / c m
效率 【 。然 而 , 这 些研 究 主要集 中在 电学 性 能上 的
机 聚 合 物 和 富勒 烯 构 成 的 本 体 异 质 结 太 阳 电池 的 激 子分 离率 更 高,光 电转换 效 率也 相应 的高 些 。但
是, 这 种 电池 会 在 有 机 活性 材 料 与 阴极之 间形 成 富
超疏水涂层防覆冰技术研究进展

㊀第43卷㊀第4期2024年4月中国材料进展MATERIALS CHINAVol.43㊀No.4Apr.2024收稿日期:2022-04-23㊀㊀修回日期:2022-07-15基金项目:新疆维吾尔自治区自然科学青年基金项目(2021D01C100);天山青年计划项目(2020Q012);天池百人计划项目(TCBR202106)第一作者:陈小东,男,1997年生,硕士研究生通讯作者:胡丽娜,女,1986年生,副教授,硕士生导师,Email:hulina@DOI :10.7502/j.issn.1674-3962.202204022超疏水涂层防覆冰技术研究进展陈小东,胡丽娜,杜一枝(新疆大学电气工程学院,新疆乌鲁木齐830017)摘㊀要:覆冰现象时刻威胁着电力系统的安全运行,过去几十年里,研究人员采用各种措施来预防电力设备表面覆冰,但这些措施都无法从根本上解决该问题㊂超疏水涂层由于具有独特的微纳米结构及低表面能物质,在低温环境下,能够延缓结冰且降低表面的冰附着力㊂从超疏水涂层的防覆冰机理入手,重点综述国内外超疏水涂层防覆冰的实验研究现状,并将影响防覆冰性能的因素分为环境因素和基底因素,分析当前方案的局限性,同时阐述提高超疏水涂层机械鲁棒性的设计与制备方面的最新进展,最后提出超疏水涂层在电力系统应用中存在的问题以及未来的发展方向㊂该综述有助于研究人员建立评估超疏水涂层的防覆冰性能的试验规范,并推进超疏水涂层防覆冰技术在电力系统中的应用㊂关键词:电力系统;超疏水涂层;防覆冰机理;环境因素;基底因素中图分类号:TG174.4㊀㊀文献标识码:A㊀㊀文章编号:1674-3962(2024)04-0301-10引用格式:陈小东,胡丽娜,杜一枝.超疏水涂层防覆冰技术研究进展[J].中国材料进展,2024,43(4):301-310.CHEN X D,HU L N,DU Y Z.Research Progress of Anti-Icing Technology of Superhydrophobic Coating[J].Materials China,2024,43(4):301-310.Research Progress of Anti-Icing Technology ofSuperhydrophobic CoatingCHEN Xiaodong,HU Lina,DU Yizhi(School of Electrical Engineering,Xinjiang University,Urumqi 830017,China)Abstract :Icing always threatens the safe operation of power system.In the past few decades,researchers have taken vari-ous measures to prevent the icing on the surface of power equipments,but these measures can not fundamentally solve this problem.Due to its unique micro-nano structure and low surface energy materials,superhydrophobic coating can delay icing and reduce the adhesion ability of surface ice at low temperature.Therefore,started with the anti-icing mechanism of super-hydrophobic coating,this paper focuses on the experimental research status of anti-icing of superhydrophobic coating at home and abroad,divides the factors affecting the anti-icing performance into environmental factors and substrate factors,analyzes the limitations of the current schemes,and expounds the latest design and preparation progress on improving the mechanical robustness of superhydrophobic coating.Finally,the problems existing in the application of superhydrophobic coating in pow-er system and the future development direction are put forward.This review is helpful for researchers to establish test specifi-cations for evaluating the anti-icing performance of superhydrophobic coatings and promote their application in power system.Key words :power system;superhydrophobic coating;anti-icing mechanism;environmental factors;substrate factors1㊀前㊀言随着特高压直流输电技术的突破和新能源并网需求的增多,电力设备数量激增,设备表面凝露[1,2]㊁覆冰现象降低了电网供电能力,给电网的检修维护提出巨大挑战㊂在国内外学者的不断探索下,目前主要有2种思路来应对电力设备的防覆冰问题:除冰和防冰[3,4]㊂除冰方法包括:机械除冰法[5,6]㊁热力除冰法[7-9]㊁电磁除冰法和超声波除冰法[10]㊁化学除冰法[11,12]㊂防冰方法包括被动除冰法以及其它方法[13]㊂被动除冰方法是指涂覆电热防冰材料[14,15]和光热防冰材料[16,17],这种方法会导致电线中有泄露电流,且增加线路损耗㊂近10年来,随着仿生涂层材料的发展[18],研究人员从单一的除冰或防中国材料进展第43卷冰开始走向 防-除并举 ,着重于防㊂受 荷叶效应 启发,1996年Onda等[19]在玻璃板上用烷基烯二聚体制备粗糙表面,并在其上涂覆低表面能材料,首次获得了人工超疏水表面,为超疏水涂层的制备提供重要思路㊂该涂层使液滴难以附着于表面,在很大程度上减少了表面结冰概率和结冰量㊂图1列举了自然界中超疏水涂层的例子[20-23]㊂全力挖掘超疏水涂层在防覆冰领域的潜力,对我国 双碳 目标的实现具有重大意义㊂图1㊀自然界中超疏水涂层的例子:(a)莲叶[20],(b)鼠尾草表面[21],(c)蝴蝶翅膀[22],(d)壁虎足底[23] Fig.1㊀Examples of superhydrophobic coatings in nature:(a)lotus leaf[20],(b)sage surface[21],(c)butterfly wings[22],(d) gecko foot[23]㊀㊀目前研究现状表明,还没有一种材料可以完全解决如低温高湿等的复杂环境中的积冰问题,现有关于超疏水涂层的研究多数处于实验室阶段㊂因此,本文从超疏水涂层的防覆冰机理着手,重点综述超疏水涂层防覆冰性能的主要影响因素,探讨与工程实际应用环境的差距,总结当前设计方案的局限,同时针对超疏水涂层机械稳定性差这一问题,阐述提高涂层鲁棒性的设计与制备方法的最新进展㊂本综述希望为适应复杂环境的超疏水涂层的设计提供支撑,加快工业化进程㊂2㊀超疏水涂层防覆冰机理表面结冰从宏观上可分为3个阶段:首先是水蒸气或小液滴在冷表面凝结;其次是过冷液滴结冰;最后是液滴完全冻结,固态冰继续增长[24,25]㊂超疏水涂层的防覆冰机理可从3个方面阐释:一是超疏水涂层表面的过冷液滴滑落[26];二是超疏水涂层表面可延缓液滴结冰过程[27,28];三是超疏水涂层的低表面能可降低冰与基底的粘附力[29,30]㊂2.1㊀过冷液滴滑落超疏水涂层具有微纳米粗糙结构及低表面能物质,液滴在表面呈Cassie-Baxter状态[31],此状态下,液滴与表面粗糙结构之间存在 空气垫 ,这些空气垫起到 托举 作用,减小了液滴与表面的接触面积㊂相较于亲水和疏水表面,当液滴撞击到超疏水表面或液滴受到外力时,在-25ħ左右液滴出现明显的收缩和反弹行为[32],且最大限度地缩短过冷水与表面的接触时间㊂但是在高湿度㊁接近露点温度时,超疏水表面的接触角降低,滚动角增加,这种液滴的弹跳效应可能无效[33],因此在表面试验时,要仔细考虑环境因素㊂除了 空气垫 作用,冷凝形成的小水珠在超疏水涂层纳米结构的毛细管力作用下逃逸出纳米间隙,随后与其他水珠结合成小水滴,该过程释放的能量使得水滴发生自迁移[34]㊂由于 空气垫 作用和自迁移现象,液滴在结冰之前会从超疏水表面滑落,从而大大降低表面的结冰概率和结冰量㊂图2为液滴在超疏水表面的各种状态㊂图2㊀液滴在超疏水表面的各种可能状态:(a)Cassie-Baxter状态,(b)液滴在表面滑落,(c)液滴弹跳Fig.2㊀Possible states of droplets on superhydrophobic coatings:(a)Cassie-Baxter state,(b)droplets sliding on the surface,(c)droplet bouncing2.2㊀延缓结冰时间经典成核理论中,均相成核能垒由式(1)计算: 203㊀第4期陈小东等:超疏水涂层防覆冰技术研究进展ΔGhomo c=16πγ33(ΔG V )2(1)式中,γ为冰和水的界面张力,ΔG V 为单位体积冰和水的自由能之差㊂考虑到外界因素对成核的促进作用,此时结冰为异相成核过程,成核能垒为[35]:ΔG c =ΔGhomo cf (m ,x )(2)式(2)中,系数f (m ,x )取值在0到1之间㊂对于表面的结冰现象,在一定的驱动力下,主要考虑表面形貌对成核能垒的影响[35],即:f (m ,x )=12+121-mx w ()+x 322-3x -m w ()+(x -m )3w éëêùûú+3mx 32x -m w -1()(3)式(3)中,w =(1+x 2-2xm )1/2,m =cos θ,x =r /r c ,θ为冰核与表面的接触角,r 为成核促进粒子半径,r c 为结冰的临界成核半径㊂研究表明,在一定的驱动力下,液滴在凸面成核时,基底曲率半径越小,成核能垒越高;而在凹面成核时,刚好相反[36,37]㊂最近,作者课题组最新研究成果也证实了这一点[38]㊂除此之外,作者团队从理论上确定了圆柱体表面液滴成核所需能垒,成核能垒处在平面㊁球面液滴成核能垒之间[38]㊂由于超疏水表面微纳米粗糙结构的存在,使液滴的成核能垒高于普通表面,从而导致液滴结冰过程得到延缓㊂成核基底的形貌也影响成核速率,研究发现20nm 的颗粒尺寸设计比100nm 的颗粒尺寸设计具有更低的冰核形成速率[39]㊂从传热角度分析,超疏水涂层粗糙结构中的空气起到了 隔离 和 热障 作用,导致传热速率大大降低(图3[40]),减缓液滴在冷表面的成核以及成核后冻结峰的传播[41]㊂图3㊀冻结液滴在不同表面的散热过程示意图[40]:(a)亲水表面,(b)疏水表面Fig.3㊀Heat dissipation process schematics of frozen droplets on differ-ent surfaces [40]:(a)hydrophilic surface,(b)hydrophobic surface超疏水涂层边缘结冰现象也是表面结冰速率减缓的原因之一,由于超疏水涂层边缘处热力学相变驱动力大于中间,因此超疏水表面结冰是由边缘逐渐向中间蔓延,减缓了整个表面结冰过程㊂图4为超疏水铜表面的边缘结冰现象[42]㊂图4㊀超疏水铜表面边缘结冰现象[42]Fig.4㊀Icing on the edge of superhydrophobic copper surface [42]2.3㊀降低冰与基底的粘附力冰的粘附力是衡量超疏水涂层防覆冰性能的重要指标㊂从根本上说,冰与固体表面之间的相互作用包括长程的范德华力㊁短程静电作用和界面微观凸起的机械联锁[29],水在表面上的吸附由粘合力和内聚力之间的平衡造成,水分子之间氢键以及水分子和衬底之间氢键的相对强度决定了吸附力的大小㊂超疏水表面具有键合强度较低的氢键位点,导致水分子之间的内聚力大于水对基体的粘合力,使得液滴与超疏水表面的接触角较大,接触面积较小[43],从而降低表面冰的粘附力,许多研究也证实了这一点[44-46]㊂但也有学者发现,超疏水涂层在经过多次结冰 融冰实验后,表面防覆冰能力减303中国材料进展第43卷弱,原因是液滴体积膨胀破坏了表面微观结构[47]㊂图5为结冰导致超疏水表面微观结构被破坏的示意图㊂因此,对于超疏水涂层是否真正有利于减小冰的粘附有待进一步研究㊂图5㊀结冰导致超疏水表面微观结构被破坏[47]Fig.5㊀Microstructure of superhydrophobic surface being destroyedby freezing [47]除了上述3个方面,还要综合考虑液滴中的杂质㊁表面化学性质㊁环境因素(温度㊁湿度㊁风速)的协同作用,这样问题也更加复杂,需要国内外学者展开更深入的研究㊂3㊀超疏水涂层防覆冰性能的实验研究超疏水涂层在低温高湿条件下是否具有良好的防覆冰性能,如较长的液滴冻结延迟时间和较低的冰粘附力,以及是否存在浸润性的转变,如由表面超疏水变为疏水,在学术界仍存在争议,原因在于各个研究之间实验条件不同㊂成冰方式可分为:静态结冰,即水蒸气在基底表面冷凝结冰;动态结冰,即液滴撞击冷基底表面凝结结冰㊂多数实验研究以一定条件下超疏水涂层表面液滴结冰速度㊁结霜量以及冰附着力的大小,作为防覆冰性能的评判依据㊂总结近几年国内外文献发现,将防覆冰性能影响因素可分为2类:一类是环境因素,即温度㊁湿度㊁液滴撞击速度㊁风速;另一类是基底因素,即粗糙度㊁浸润性㊁机械鲁棒性㊂3.1㊀环境因素环境因素对超疏水涂层防覆冰性能的影响可分为三方面:一是对液滴结冰时间延迟的影响,多数研究表明超疏水涂层可以延迟液滴结冰;二是对基底上液滴润湿状态改变的影响,这直接关系超疏水涂层防冰㊁除冰性能;三是对动态结冰中液滴撞击表面后动力学行为的影响㊂3.1.1㊀温度液滴结冰过程伴随着液滴与环境之间的热传递,温度不仅影响热传递速率,也是构成成核能垒的关键因素㊂2010年,周艳艳[48]开展了-7.5,-11.8,-21.1,-28及-35ħ环境温度下普通铝表面㊁疏水铝表面㊁超疏水铝表面结霜试验,结果显示随着温度的降低,3种表面的结霜量都不断增加,但在同一温度下,超疏水铝表面的结霜量相较于普通铝表面有很大程度的减少,说明超疏水铝表面具有很好的防覆冰性能㊂2011年,徐文骥等[49]测量了基体温度为-5.2,-10.1及-14.2ħ时普通铝片和超疏水铝片表面结霜质量和边缘处结霜高度,发现随着温度降低,二者边缘处霜高都相应增加且差异不大,但是处于同一基体温度时超疏水铝片表面的结霜质量较少,当完成50次结霜除霜实验后,超疏水性能仍能保持㊂2014年,Hao 等[50]探究了温度对超疏水铜表面结冰㊁结霜行为的影响,发现基底温度越低,样品表面结冰㊁结霜速度越快㊂2015年,Ou 等[51]在不同温度下测量了亲水㊁疏水和超疏水表面冰的粘附力,结果显示随着温度的降低,冰在3种表面的粘附力均有所增加㊂但是对于超疏水样品,其表面冰粘附力增加幅度比亲水和疏水样品更为明显,这是因为温度较低时,液滴渗透到微结构内部,与表面形成机械联锁㊂2015年,Shen 等[52]研究了不同样品表面液滴结冰时冰层生长速度与温度的关系,结果表明冰层生长速度随着温度降低而增大,但是超疏水表面的冰层生长速度随温度降低的变化幅度相对较小,这归因于超疏水表面缓慢增加的冰成核速率㊂2017年,Emelyanenko 等[53]记录了不同温度下超疏水表面液滴弹跳效率,发现-17ħ㊁湿度为75%时,超疏水橡胶表面的反弹效率达到100%;当温度在-20ħ下,弹跳效率达到70%,主要原因是随着温度的降低接触面积和液滴扩散反冲时间显著增加㊂3.1.2㊀湿度环境湿度对超疏水涂层表面结冰有促进作用且会增大表面冰的附着力,原因是当环境湿度较大,微小液滴在表面凝结成较大液滴,此时液滴压力大于毛细管力,导致表面由原来Cassie-Baxter 状态过渡为Wenzel 状态,这一点被许多文献提及[54,55]㊂图6为湿度对表面液滴浸润状态的影响示意图㊂图6㊀湿度增加促使液滴浸润状态改变Fig.6㊀Increase of humidity causing the change of droplet infiltra-tion state卢津强[56]报道了在相对湿度分别为50%,70%和90%条件下超疏水铜表面的结冰情况,发现环境湿度对超疏水涂层表面边缘的结冰行为几乎没有影响,但随着403㊀第4期陈小东等:超疏水涂层防覆冰技术研究进展环境湿度增大,超疏水铜表面的结冰量逐渐增多,在与普通表面㊁亲水表面的对照实验中,超疏水表面在延迟结冰时间和减少结冰量方面都具有显著优势㊂Yin等[57]关注了在温度为-10~30ħ,湿度为10%,30%,60%及90%时自然荷叶与超疏水涂层表面接触角和滚动角的变化,发现当表面温度接近露点温度且湿度较高(>60%)时,接触角减小㊁滚动角增加,此时表面液滴状态从Cassie-Baxter状态变为Wenzel状态,超疏水表面的浸润性增加,当表面凝结水消失,超疏水性得到恢复㊂Wang 等[33]发现当温度为-10ħ时,相对湿度从10%变化到90%,接触角和滚动角从163ʎ和6ʎ变为138ʎ和20ʎ,这种变化必然与超疏水表面微纳米结构中水的冷凝有关;除此之外,还探究了不同湿度条件下,10μL过冷水滴从5mm高度撞击10ʎ倾斜超疏水表面的动态行为,结果表明随着湿度增加,回弹高度急剧下降,当相对湿度超过95%时,液滴无法在超疏水表面反弹㊂3.1.3㊀液滴撞击速度和风速液滴撞击速度直接影响超疏水表面Cassie状态的稳定性㊁液滴与表面接触后的动力学过程以及传热过程,如果液滴的速度较快,接触超疏水表面时获得的动能大,克服了表面微结构产生的毛细管力,从而穿透微结构中滞留的空气,此时表面浸润性将大大增加[58]㊂同时,撞击速度越快,液滴在表面的扩散系数越大,结冰越迅速[59],而且与底层固体的接触面积增加,传热增强,导致更多非均质冰核形成㊂Han等[60]探究了不同直径的超疏水圆柱体弯曲表面上液滴撞击速度对液滴铺展直径以及液滴与表面接触时间的影响,如图7所示,液滴铺展直径随着液滴撞击速度增大而增大,但液滴与曲面接触时间随之减少㊂Zhu等[61]关注了风场条件下超疏水表面的除冰性能,发现当风速为7m/s时,吹落光滑基体表面冰珠大约需要12s,但吹落经过氟化修饰处理后的超疏水表面的冰珠仅需7s,说明超疏水涂层拥有较强风场除冰能力,该研究有望推动超疏水涂层在实际工程中的应用㊂以上研究成果多数是在实验室特定环境下开展试验获得的,然而在实际工作环境中,温度㊁湿度㊁风速等因素多变,而且积冰形成的方式不同,如雪㊁霜冻㊁冻雨等㊂因此在户外复杂环境中开展超疏水涂层的抗结冰试验应引起重视㊂3.2㊀基底因素超疏水涂层对水的粘附力较低,但是对冰是否具有低粘附力,学者们的观点并不一致,原因在于冰与水粘附机制不同[62]㊂对冰的粘附力是评价超疏水涂层防覆冰性能的重要指标,探究与水浸润性相关的参数(接触角㊁图7㊀水滴以不同速度撞击超疏水圆柱体表面的图像[60] Fig.7㊀Dynamic images of droplet impingement on superhydrophobic cylindrical surface at different velocities[60]滚动角等)如何影响表面对冰的粘附力,将直接影响超疏水/冰涂层的设计㊂3.2.1㊀接触角和滚动角接触角和滚动角是表征超疏水涂层的重要指标㊂关于接触角如何影响表面冰的粘附力,目前的研究结果仍存在争议㊂1997年,Saito等[63]制备了聚四氟乙烯含量为30%~ 90%的超疏水材料,并通过实验发现聚四氟乙烯含量增加使得表面接触角增加㊁表面能降低,而表面能的降低进而导致表面冰的粘附力减小,因此超疏水表面接触角的增加会导致表面冰粘附力的减小㊂同年,该团队发现聚四氟乙烯超疏水材料表面冰的粘附力和由接触角计算得出的表面自由能之间为线性关系[64]㊂2009年,Dotan 等[65]通过离心测力装置测试了亲水㊁疏水㊁超疏水等5种材料表面冰的粘附力,结果显示冰附着力随着接触角的增大而减小㊂在前人基础上,Ozbay等[66]在金属㊁橡胶和聚合物表面进行结冰实验,结果表明表面润湿性和由表面接触角计算得出的表面能之间具有显著的相关性,且二者共同影响表面冰的粘附力㊂随着研究的深入,人们发现冰的粘附力和接触角的相关性并非简单的线性关系㊂研究人员将更多的注意力放到接触角的滞后性对表面冰的粘附力的影响㊂Kulinich 等[67]利用离心装置测量了6种材料表面冰的粘附力,发现粗糙疏水表面冰的粘附力与表面接触角无关,而与接触角滞后密切相关㊂Meuler等[68]制备了21种不同润湿性的涂层,发现冰的粘附力和后退接触角具有很强的相关性,因此可以通过测量表面后退接触角对表面的 憎冰503中国材料进展第43卷性 进行预测㊂与前人得出的结论不同,Wu 等[29]制备了37种不同表面形貌的超疏水涂层,发现冰的粘附强度与表面接触角㊁接触角滞后不存在简单的相关性,不能直接作为防冰超疏水涂层的结构设计参数,而应结合表面浸润性以及结冰过程中传热传质特性㊂3.2.2㊀浸润性关于超疏水表面浸润性的研究表明,超疏水表面并不一定具有降低冰粘附力的作用,这一点与超疏水表面的理论研究所推断的结果大相径庭㊂2010年,Varanasi 等[69]通过光刻工艺获得一系列疏水硅柱,然后喷涂低表面能物质获得超疏水表面㊂利用扫描电镜记录了霜在超疏水表面的形成过程,如图8所示㊂图片显示霜在超疏水表面形成时,基底的部分微观结构已经被水浸润且逐渐形成霜晶,这将对超疏水表面后续防覆冰性能产生影响㊂图8㊀霜在超疏水表面微观结构中逐渐形成[69]Fig.8㊀Gradually formed frost in the microstructure of superhydrophobic surface [69]㊀㊀2011年,Kulinich 等[47]分别利用浸涂㊁旋涂㊁喷涂方法制备了3种不同浸润性的涂层,并测量了3种涂层表面冰的粘附力,结果显示浸涂法制备的涂层表面冰的粘附力最小,且在结冰 除冰实验中,该涂层也展现出了更加优异的机械稳定性㊂2012年,Chen 等[70]探究了表面形貌和表面化学性质对冰粘附强度的影响,结果显示粗糙表面冰的粘附强度高于光滑表面,原因是冰与超疏水表面的粗糙结构形成机械联锁㊂与其结论相反,2014年Bharathidasan 等[71]的研究成果表明,亲水涂层表面冰的粘附力高于疏水涂层,并将疏水表面冰的低粘附力归因于低表面能物质㊂除了影响表面冰的粘附力,一些学者关注了浸润性对表面液滴冻结过程的影响㊂Liu [72]等研究了表面润湿性对液滴撞击曲面后动态特性的影响,结果表明,当曲率比一定时,较差的表面润湿性会阻碍液滴的扩散,但会促进液滴的收缩和回弹㊂张青等[73]在导线表面制备了超憎水涂层,探究表面浸润性对导线表面覆冰的影响㊂实验结果表明,超憎水性涂层不利于过冷水滴在导线上粘附和冻结,可以显著抑制和缓解铝导线表面覆冰的形成和增长㊂Liao 等[74]发现与普通表面相比,超疏水涂层可以有效延迟表面液滴结冰,原因在于超疏水涂层粗糙结构中的空气起到 隔离 和 热障 作用,另外由于液滴自迁移现象,部分液滴会在结冰之前滚落下来,减少了结冰概率㊂图9所示为裸铝表面和超疏水铝表面结冰情况㊂3.2.3㊀粗糙度表面粗糙度是冰粘附力的一个重要影响因素,增加粗糙度,可以提高界面拉普拉斯力,阻碍液滴从Cassie 状态向Wenzel 状态转变㊂Satio 等[64]探究了表面粗糙度对疏水表面和亲水表面冰粘附力的影响,发现这2种材料呈现出截然相反的结果㊂对于疏水表面,表面粗糙度的增加导致表面冰粘附力的减小,而对于亲水表面,表面粗糙度的增加导致表面冰粘附力的增加㊂与其结论不同,Tarquini 等[75]开展了直升机桨叶表面超疏水涂层的脱冰性能研究,发现冰粘附力随表面粗糙度增加而增加,认为冰和固体表面之间的有效接触面积增加导致脱冰所需的力增加㊂603㊀第4期陈小东等:超疏水涂层防覆冰技术研究进展图9㊀裸铝和超疏水铝表面上形成釉冰的情况[74]Fig.9㊀Glaze ice on the surfaces of bare aluminum and superhydrophobic aluminum [74]㊀㊀粗糙度除了影响表面冰附着力,还会影响表面的结霜行为㊂张友法等[76]对比研究了单级纳米结构和二级复合结构对表面除冰㊁融冰的影响,如图10所示㊂结果表明微纳米复合结构在防覆冰性能方面并不逊色于单级纳米结构,关键在于经过多次结冰 融冰试验后,微纳米复合结构表面的防霜抗冰性能仍得到保持㊂3.2.4㊀机械鲁棒性微纳米结构的机械强度弱是目前超疏水涂层面临的最大问题,因此设计出坚固耐用的超疏水涂层成为近几年学者们的研究重点㊂Groten 等[77]通过实验论证了微纳米复合结构在构建机械性能稳定的超疏水表面中的重要性,尤其当涂层抵抗外界较大剪切应力时,微米结构更是起到决定性作用㊂在Balordi 等[78]的研究中,这一点同样被证明㊂Kondrash-ov 等[79]通过刻蚀工艺制备了 微骨和纳米草 复合结构表面,经过氟化处理获得超疏水表面,该表面显示出极大的机械耐久性,尤其是抗剪切性㊂Zhang 等[80]通过刻蚀法和喷涂法制备了机械稳定性强的铝合金超疏水涂层,图11所示为涂层抗磨损示意图㊂该涂层能够抵抗循环水喷射㊁砂粒冲击和砂粒剪切磨损以及手指摩擦,图12所图10㊀可控阵列微纳复合结构表面结冰及结霜情况对比[76]:(a)条纹阵列结构,(b)方柱阵列结构,(c)四棱锥阵列结构,M代表微结构表面,S 代表光滑表面,N 代表 纳米草 ,MN 代表具有微结构和 纳米草 的表面Fig.10㊀Comparison of icing and frosting on the surfaces of controllable array micro-nano composite structure [76]:(a)striped array struc-ture,(b)square column array structure,(c)quadrangular prism array structure;M represents microstructured surface,S is smooth surface,N is nanograss,MN represents the surface with microstructure and nanograss703。
快速热退火对铌:二氧化钛多层电极插入有机太阳能电池的纳米银层的电学,光学,结构性能的影响

附件1:外文资料翻译译文快速热退火对铌:二氧化钛多层电极插入有机太阳能电池的纳米银层的电学,光学,结构性能的影响我们研究了铌的电学,光学,结构,界面和表面特性的快速热退火RTA的影响:二氧化钛NTO-Ag-NTO多层电极插入一个应用于纳米银层有机太阳能电池的OSCs退火温度为500℃,“NTO银NTO电极的电阻率和光透过率由于银向外扩散的情况下保持稳定。
在NTO层的铌有效激活导致在电阻率略有下降,并在伯斯坦- 莫斯效应引起的带隙增加。
然而,提高温度超过600°C等导致退化严重NTO-Ag-NTO多层电极由于银扩散。
基于同步加速器x射线散射和x射线光电子能谱分析结果,电气性能的相关NTO-Ag-NTO电极材料的显微结构和各层界面扩散。
此外,研究发现是批判的性能是依赖于区域温度NTO-Ag-NTO电极。
焊后的NTO-Ag-NTO电极虽然经历了一个相当低电阻率。
这表明的激活在顶部注搀杂剂粉末层起着重要作用的载体提取有机层NTO-Ag-NTO阳极电极。
©2010美国物理协会。
一.导言聚合物体相异质结有机OSCS太阳能电池已作为被深入研究的高效的新一代环保型太阳能电池,由于其具有独特的优势,结构简单,成本低,重量轻,简单的印刷制程。
硅基薄膜太阳能电池竞争,应采用更划算高效的过程和更便宜的材料OSCS。
特别是,制作成本效益OSCS,这是当务之急,以取代铟锡氧化物ITO 电极的OSCS。
铟短缺,这是ITO中的主要元素,增加了OSCS总成本。
出于这个原因,各种低成本负极材料如Ga-ZnO,Al-ZnO,InZnSnOx聚3,4- 乙烯基聚苯乙烯PEDOT:PSS的单壁碳纳米管和石墨已得到了考察以取代昂贵ITO电极。
近日,Nb掺杂的TiO2的NTO已作为一种很有前途的铟透明导电氧化物TCO的材料引起关注,由于其2*10^-4Ω每厘米的低电阻率,90%高可见透明度,而Ti元素相对丰富。
虽然NTO对钛酸锶STO和LaAlO3单晶劳衬底外延膜低电阻和高透明度,是不可取的特殊单晶STO和LAO基板上生长外延NTO薄膜为低成本OSCS。
温度对钛酸钠纳米管光电及光催化性能的影响

塔
里
木
大
学
学
报
第2 4卷
成、 结构和形成机理等方面 , 同时还对其光电性质进
行 了研 究 。而焙烧 温度 对钛 酸钠 纳米管 光 电性能 及
光 催化性 能 影响 的报 道很少 。表 面光 电压 ( P ) S S 技
纳 米颗粒 ; 析纯级 甲醛 ; 分 蒸馏 水 。 主要 仪 器 : 日本 JM 一10 XI 10 V) 射 E 0C 0K 透 I( 电子显微 镜 ( E ;hlsX, et r T M) P ip P rPoX射 线 衍射 i
2 结 果 与讨 论
2 1T M 表 征 . E
图 1 样 品 A、 c、 、 F的透射 电镜 图 。 由 是 B、 D E、
塔
里
木
大
学
学
报
第2 4卷
2 3 光 电压 分析 .
管 烧结在 一起 成 为近 似 纳 米棒 的结 构 , 使 纳 米 管 致 管 径增 大 。管 径变 大 引 起 管 的禁 带 宽 度 变 小 , 而 从
这是 因为焙烧 引起钛 酸钠纳米管的形貌和 晶体类 型发生变 化 。所制 样 品对 甲醛 的降解 特性测试 结果 表明 , 焙烧温度 对样 品
的光催化活性有一定程 度的影响。 关键 词 钛酸钠纳米管 ;表面光 电压 ; 光催化 文献标识码 : A D I1 .9 9ji n 10 0 6 .0 2 0 .0 O :0 3 6 /. s.0 9— 58 2 1. 20 5 s 中图分类号 : 6 44 0 1 .
温度对钛酸钠纳 米管光 电及光 催化性 能的影 响
张红美 孔德 国 胡芸莎 唐玉荣 陈明鸽
( 1塔里 木 大学 机械 电气 化工 程学 院 , 新疆 阿拉 尔 8 30 ) 4 30
霍夫梅斯特效应 超分子水凝胶的机械强度
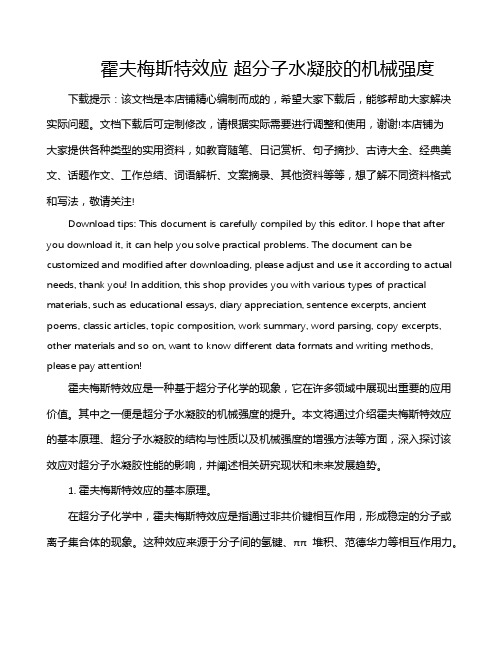
霍夫梅斯特效应超分子水凝胶的机械强度下载提示:该文档是本店铺精心编制而成的,希望大家下载后,能够帮助大家解决实际问题。
文档下载后可定制修改,请根据实际需要进行调整和使用,谢谢!本店铺为大家提供各种类型的实用资料,如教育随笔、日记赏析、句子摘抄、古诗大全、经典美文、话题作文、工作总结、词语解析、文案摘录、其他资料等等,想了解不同资料格式和写法,敬请关注!Download tips: This document is carefully compiled by this editor. I hope that after you download it, it can help you solve practical problems. The document can be customized and modified after downloading, please adjust and use it according to actual needs, thank you! In addition, this shop provides you with various types of practical materials, such as educational essays, diary appreciation, sentence excerpts, ancient poems, classic articles, topic composition, work summary, word parsing, copy excerpts, other materials and so on, want to know different data formats and writing methods, please pay attention!霍夫梅斯特效应是一种基于超分子化学的现象,它在许多领域中展现出重要的应用价值。
研究人员首次发现光悬浮纳米金刚石扭动模式运动

了富含含氧官能团的碳量子点 ,该方法制备碳量子点产量高、 操作简便 、成 本低廉。合成 的碳量 子点在磷酸二 氢钠 的辅 助
作用及高温热处理下通过 自组装可形成大面积的磷掺杂碳纳米 片。该 团队系统探究 了由碳量子点到碳纳米片的转化过程及其
潜力 的新 型治疗手段 ,该 类技术能有效 增加化疗药物 在靶部 式 。 研究论文 《 悬浮非球形纳米粒子的扭动光力学 ( T o r s i o n a l 位的浓度 同时降低其在健 康组织器 官内的蓄积 ,最终提 高化 O p t o me c h a n i c s o f a L e v i t a t e d No n s p h e r i c a l Na n o p a r t i c l e ) 疗的疗效和安全性 。 日前发表在 物理评论快 报》( P h y s i c a l Re v i e w L e t t e r s ) 上,
影响 因素 ,并深入研究了磷掺杂碳纳米片的电化学储钠行为。
该材 料 展现 了优异 的储 钠 性 能 ,其 可逆 比容量 高 、循
环 寿 命 长。 在 0 . I Ag 的 电流 密 度 下 ,其 可 逆 比容 量 为 3 2 8 mA h・ g ~,在 2 0 A g 的超大 电流密 度 下 ,其 可逆 容 量
池碳 负极材料 方面的研究取得 重要进展 ,创新性 地提 出了一
种批量 制备碳量 子点及其衍 生碳 材料 的方法 。 该团队以乙醛和氢氧化钠为原料 ,通过室温静置反应制各
聚合物与血液 内各种成分 的相 互作用 ,其结构往 往会遭到破 坏并导致所包 封的药物释放 至血液 内。另外 ,药物还可 以从 聚合物胶束 的内核转移至血 液循环 内导致药 物突释效应 。上 述两个过程单 独或综合起来 均可极大地 降低 聚合 物胶束所 包 封的药物在血 液循环 中的半 衰期 ,因而难 以充分 利用肿瘤 的 高通透性 和滞 留效应 ( E P R e f e c t ) 达到满意的肿瘤靶 向效果 。 该专栏文章综 述 了用于解决 这一 问题最有 效的几种物理 作用力 ( 丌一丌堆积 、 立构复合、 氢 键、 主客体包络 、 配位作用 ) 及化学方法 (自由基 聚合 、点 击化学 、双硫 键、腙 键 ) 。 以上 方法可显著提 高聚合物 胶束的肿瘤靶 向效率 ,并 已促成近 十
- 1、下载文档前请自行甄别文档内容的完整性,平台不提供额外的编辑、内容补充、找答案等附加服务。
- 2、"仅部分预览"的文档,不可在线预览部分如存在完整性等问题,可反馈申请退款(可完整预览的文档不适用该条件!)。
- 3、如文档侵犯您的权益,请联系客服反馈,我们会尽快为您处理(人工客服工作时间:9:00-18:30)。
Journal of Alloys and Compounds 529 (2012) 96–101Contents lists available at SciVerse ScienceDirectJournal of Alloys andCompoundsj o u r n a l h o m e p a g e :w w w.e l s e v i e r.c o m /l o c a t e /j a l l c omUnusual thermal stability of nano-structured ferritic alloysX.L.Wang a ,∗,C.T.Liu b ,c ,U.Keiderling d ,A.D.Stoica a ,L.Yang a ,ler b ,C.L.Fu b ,D.Ma a ,K.An aaNeutron Scattering Science Division,Oak Ridge National Laboratory,Oak Ridge,TN 37831,USAbMaterials Science and Technology Division,Oak Ridge National Laboratory,Oak Ridge,TN 37831,USA cDepartment of System Engineering and Engineering Management,City University of Hong Kong,83Tat Chee Avenue,Kowloon Tong,Hong Kong dHelmholtz Center Berlin for Materials and Energy,Glienicker Strasse 100,D-14109Berlin,Germanya r t i c l ei n f oArticle history:Received 18October 2011Received in revised form 23February 2012Accepted 24February 2012Available online 7 March 2012Keywords:NanostructureSmall angle neutron scattering Neutron diffractionHigh temperature deformation Atom probe tomography (APT)a b s t r a c tA scientific question vitally important to the materials community is whether there exist “self-assembled”nanoclusters that are thermodynamically stable at elevated ing in situ neutron scat-tering,we have characterized the structure and thermal stability of a nano-structured ferritic alloy.Nanometer sized nanoclusters were found to persist up to ∼1400◦C,providing direct evidence of a thermodynamically stable alloying state for the nanoclusters.High-temperature neutron diffraction measurements show a stable ferritic matrix,with little evidence of recrystallization or grain growth at temperatures up to 1300◦C.This result suggests that thermally stable nanoclusters and the oxygen-vacancy interaction limit the diffusion of Fe atoms and hence the mobility of grain boundaries,stabilizing the microstructure of the ferritic matrix at high temperatures.© 2012 Published by Elsevier B.V.1.IntroductionEmergent behaviors are found in many complex material sys-tems,where a small adjustment in chemical composition can cause a dramatic change in properties.Excellent mechanical properties are obtained,for example,by mechanical alloying of ferritic steel with only minor addition of Ti and Y 2O 3(≤0.5wt.%)[1–13].At temperatures between 650and 900◦C,the creep rate of Fe–14Cr–3W +0.25Y 2O 3+0.4Ti (wt.%)(hereafter designated as 14YWT)ferritic alloy is six orders of magnitude lower as compared to the similar cast materials [5].This level of improvement repre-sents a major breakthrough in the design of ferritic alloys for high temperature structural applications.Similarly,significant improve-ment in creep resistance (by two orders of magnitude)was reported by introducing carbonitride precipitates with minor addition of N and C [14].It has been suggested that the unusually low creep rate in these micro-alloyed steel is related to the nanoclusters that formed dur-ing processing.Here,by nanoclusters,we mean fine scale particles with less well defined boundaries.Indeed,transmission electron microscopy (TEM)carried out on 14YWT demonstrated high-density stable nanoclusters with diameters of 2–5nm,and atom probe tomography indicates that these nanoclusters are distinctly enriched with Ti,O and Y,as compared to the matrix composition∗Corresponding author.Tel.:+18655749164;fax:+18655746080.E-mail address:wangxl@ (X.L.Wang).[15].Similarly,atom probe tomography studies on a related 12YWT (with 12wt.%Cr)revealed stable clusters with a diameter of ∼4nm [3].Small angle neutron scattering (SANS)is another powerful probe to characterize nanoscale structures [12,13,16–19].For 14YWT,Alinger et al.[13]reported a particle size of 2–3nm,but it is clear from the SANS intensity data [12]that more than one particle size exists.A detailed comparison between TEM measurements and SANS analysis was given for MA957[11],a Mo containing alloy with a nomination composition of Fe–14Cr–0.9Ti–0.3Mo–0.25Y 2O 3.From SANS data,a particle size distribution was estimated with a peak around 4nm.On the other hand,TEM work revealed larger particles with a particle size distribution centered around 10–20nm.The combination of SANS and TEM suggests that two particle sizes exist in MA957.Unlike most engineered or artificially created nano-materials which are meta-stable in nature,the self-assembled clusters in the 14YWT alloy appear to be extremely thermally stable.Microscopy and atom probe tomography analyses on quenched 14YWT samples after extensive heat treatments at 1380◦C still show high-density nanoclusters,without significant coarsening [15].There are two schools of thoughts as to why these nanoclusters are still present in the quenched samples.One possibility is that these nanoclusters remain during annealing at high temperatures.It is also conceiv-able,however,that the nanoclusters dissolve at high temperatures and re-precipitate during quenching,so long as the quenching rate is not exceedingly high.In situ observation is the only way to0925-8388/$–see front matter © 2012 Published by Elsevier B.V.doi:10.1016/j.jallcom.2012.02.143X.L.Wang et al./Journal of Alloys and Compounds529 (2012) 96–10197Fig.1.High-temperature furnace and sample mount used in the in situ small angle scattering experiments.unambiguously determine the exact mechanism.However,in situ scattering experiments are difficult.This is not just because of the limitation in scattering intensity;rather,it is the availability of a reliable vacuum furnace capable of reaching1300◦C that posed the greatest challenge.In this paper,we present,from in situ neutron scattering experiments,the evidence of thermally stable nanoclus-ters and show their connection to the improved creep properties.2.Experimental details2.1.Sample preparationTwo types of samples were investigated:14YWT with the composi-tion Fe–14Cr–3W+0.25Y2O3+0.4Ti(wt.%)and FNAN-1with the composition Fe–12.3Cr–3W+0.42Ti(wt.%).The former is a known nanostructured steel,whereas the latter is a base alloy containing no nanoclusters and used as a reference sam-ple.The14YWT alloy was fabricated by mechanical alloying followed by extrusion, whereas the FNAN-1alloy ingot was prepared by arc melting and drop casting into a copper mold.The14YWT powder was canned in mild steel,soaked,and then extruded at850◦C.There was no further consolidation(i.e.,no rolling or cold work-ing).In FNAN-1,the oxides werefloated to the ingot top as slag during casting without any residual Y retained in the alloy.All samples were given a standard heat treatment of1h at1000◦C in vacuum.2.2.In situ neutron scattering experimentsThe SANS experiment was carried out at Berlin Neutron Scattering Center, Helmholtz Center Berlin for Materials and Energy,using the V4instrument.The incident neutron wavelength was6˚A.The samples were cut into1-mm thick discs with a diameter of approximately8mm.An AS Scientific furnace,shown in Fig.1, with a maximum temperature of∼1600◦C,was used for the high-temperature mea-surements.Plate like specimens were mounted on a special sample holder,between two ceramic baffles,and heldfixed using a ceramic set screw.Heating was achieved by radiation with surround Nb heaters.SANS data were collected every∼25◦during heating to1400◦C,and at selected temperatures during cool down to room tem-perature.The temperature profile is plotted in Fig.2(a).For each temperature,two detector settings were used,with detectors at1and4m from the sample position, respectively,in order to cover a Q-range of0.01–0.3˚A−1.The total data collection time was10min for each temperature.The heating experiment was repeated with-out a sample for each detector setting,to determine the background scattering which also varied with the temperature.The high-temperature diffraction experiment was conducted at Intense Pulsed Neutron Source,Argonne National Laboratory,using the GPPD powder diffractome-ter.Heating was done with the“Miller”furnace.Cylindrical specimen was mounted on top of a ceramic pedestal which was then lowered into the furnace.After a rather fast temperature rise up to1000◦C with a heating rate of0.1◦C/s,both samples were subjected to consecutive stepwise annealing treatments covering the temperature range of1000–1300◦C with3h per50◦C step,during which time neutron diffraction data were collected.Additional high-temperature measurements were conducted using the new VULCAN diffractometer[20,21]at the Spallation Neutron Source[22]. In situ neutron diffraction was collected during continuous heating up to1300◦C.Fig.2.(a)Temperature profile for in situ SANS measurements.SANS data were collected every∼25◦during heating to1400◦C and at selected temperatures during cool down.At each temperature,the data collection was10min.(b)Differential scattering cross section per unit sample volume for alloy14YWT(with nanoclusters) at room temperature(blue triangles)and1412◦C(red solid circles).For comparison, room temperature SANS data for alloy FNAN-1containing no nanoclusters are also presented.(For interpretation of the references to color in this Fig.legend,the reader is referred to the web version of the article.)3.Experimental results3.1.Nanoscale particle size distributionStrong SANS intensity(over four decades)was observed in alloy14YWT,as shown in Fig.2(b).The experimental data were reduced to the differential scattering cross section per unit sample volume,d /d˝,following a standard data reduction procedure[23].For comparison,the room temperature data for the reference sam-ple FNAN-1are also plotted.These results confirm that,as in Refs.[11–13],the SANS intensity originates from scattering by nano-scale structure features formed during processing.At1400◦C,the low-Q portion of the SANS data moved towards lower Q, while the high-Q portion remained the essentially same or even increased slightly.Plotting SANS data in log–log scales revealed multiple length scales in14YWT alloys.As shown in the inset of Fig.3,three regimes in Q can be identified:0.01–0.03, 0.03–0.1,and0.1–0.3˚A−1,corresponding to three characteristic particle sizes.How-ever,modeling the SANS data with three particle size distribution functions will result in too many parameters that cannot be determined reliably.As the large par-ticles are not of interest in this study,only data for Q>0.03˚A−1corresponding to smaller particles were analyzed.The SANS data were therefore modeled with a bi-modal log-normal particle size distribution:f(D)=i=1,2N∗iD·s i√exp−1ln(D)−ln(D i)i2,(1)98X.L.Wang et al./Journal of Alloys and Compounds 529 (2012) 96–1013020100.0000.0050.0100.015P a r t i c l e S i z e D i s t r i b u t i o n F u n c t i o n [f (D )*V ]Diameter, D (nm)Fig.3.Bi-modal particle size distribution obtained by fitting the SANS data at1300◦C.The vertical axis is the volume fraction of the particle size distribution (weighted by scattering contrast),f (D )·V ,where V =( /6)D 3.The inset shows quality of the fit along with contributions by each particle size distribution.where D i is the centroid and s i is the standard deviation for each particle size dis-tribution.N ∗i is the number of particles N i weighted by the scattering contrast i ,i.e.,N ∗i =N i 2i .Satisfactory fits were obtained with this model,and an example of the particle size distribution is given in Fig.3.The maxima in the particle size distribution correspond to particles of diameters of 2nm (i =1)and 12nm (i =2),respectively.The contributions by each particle size distribution are also plottedin the inset of Fig.3.TEM experiments show that the larger particles (∼12nm)are either TiO 2or Y 2Ti 2O 7located mainly at grain boundaries.The 2-nm particles have been observed by atom probe tomography which showed that they are clusters composed of Y,Ti,and O,located both within the grains and at grain boundaries [15].An example of atom probe tomography study is shown in Fig.4for a 14YWT sample quenched after annealing for 1h at 1400◦C.Several nanometer sized Y–Ti–O clusters can be identified.Evidence for small,nanome-ter sized clusters was also reported by Hayashi et al.[24]using energy-filtered TEM.Note that SANS intensity contains scattering from both structural and magnetic heterogeneities.For a ferromagnetic material,the magnetic scattering comes from magnetic domain.Loffler et al.[17]showed that for nanostructured materials,the magnetic correlation length or domain size is at least 2–3times the grain size.For 14YWT,the typical grain size is ∼0.2–0.4m [4],so the magnetic domain is ∼0.5m.SANS from such length scale features typically show up at Q below 0.01˚A−1,very different from the Q region of our SANS data,0.01–0.3˚A−1.The presence of magnetic scattering intensity at low-Q does not affect our analysis,especially for the smaller 2-nm Y–Ti–O nanoclusters.10-410-310-210-1N *1 (a r b . u n i t )Temperature (C)Fig.5.Temperature dependence of N ∗1(see Eq.(1))for the 2-nm nanoclusters in 14YWT nanostructured ferritic alloy.The blue triangle data point was obtained aftercooling to room temperature.Reliable N ∗1couldnot be determined near the Curie temperature (T c ∼700◦C),so only data for up to 450◦C and above 1300◦C are pre-sented.(For interpretation of the references to color in this Fig.legend,the reader is referred to the web version of the article.)3.2.Thermal stability of the nanoclustersAs noted in Fig.2,the low-Q part of the SANS curve shifts slightly towards lower Q values at 1400◦C,suggesting limited growth of the 12-nm particles.Indeed,thefitted N ∗2decreases slightly,indicating a small degree of coarsening of the larger particles.This is consistent with (ex situ)TEM studies of annealed samples.However,our interest is in the 2-nm nanoclusters and their temperature dependence.In order to obtain stable fits for data sets at all temperatures,D 1and s 1were held fixed.Atom probe tomography experiments on samples annealed at 1000◦C showed noevidence of cluster growth.In Fig.5,we plot N ∗1,the number of the 2-nm clusters weighted by the scattering contrast 1,as a function of temperature.Significant magnetic critical scattering was observed due to the fluctuation of Fe magnetic moments across a Curie temperature of T c ∼700◦C.Here we need to emphasize that neither the 12-nm particles (TiO 2and Y 2Ti 2O 7)nor the 2-nm clusters (Y–Ti–O)are magnetic.In principle,the contribution by non-magnetic nanoclusters could be separated from the magnetic critical scattering,but this would require applying a magnetic field at elevated temperatures.Such an experimental environ-ment does not exist at present.Without applying a magnetic field,it is impossible to determine unambiguously the SANS intensity by the nanoclusters.For this reason,data close to T c cannot be discussed in a meaningful way and must be excluded.To determine the upper temperature limit of the magnetic critical scattering,a refer-ence alloy FNAN-1containing no nanoclusters was measured with a similar heatingFig.4.Atom maps produced by atom probe tomography on a 14YWT sample quenched from 1h annealing at 1400◦C.The size of the selected volume (i.e.,the box)is 20nm ×50nm,with a thickness of 5nm into the page.A grain-boundary is intersected,as indicated by the increased concentration of Cr atoms.Clustering of Y–Ti–O is evident.Note that the cluster is also slightly enriched in Cr.X.L.Wang et al./Journal of Alloys and Compounds 529 (2012) 96–101991400120010008006004002000.00.10.20.30.4I n t e n s i t y (a u )Temperature (C)Fig.6.SANS intensity for reference FNAN-1alloy without nanoclusters as function of temperature.The SANS intensity turned into a constant at temperatures above 1100◦C,marking the upper bound for magnetic critical scattering.profile.Above T c ,the SANS intensity at high Q for FNAN-1decreased monotonously with increasing temperature and turned into a constant for temperatures above ∼1100◦C.This is illustrated in Fig.6.Apparently,the magnetic critical scattering in the reference sample vanished at 1100◦C.Further evidence of this upper limit comes from the temperature dependence of the magnetic correlation length in pure iron.It has been established that as T c is approached from above,the magnetic critical scattering is characterized by a correlation length that grows exponentially with the inverse of temperature [25].Indeed,as Fig.7shows,the logarithm of the magnetic correlation length determined by Gersch et al.[26]for pure iron varies linearly withtemperature.At 850◦C,the magnetic correlation length is 3.2˚A.Extrapolating these data to 1000◦C shows a correlation length of less than 0.1˚A.From the analyses for pure iron and the reference alloy FNAN-1,we can safely conclude that the magneticcritical scattering is negligible above ∼1300◦C.Therefore in Fig.5,only N ∗1data for temperatures up to 450◦C and above 1300◦C are presented.It can be seen that N ∗1is essentially constant up to 450◦C.A dashed line is drawn as a guide to the parable values of N ∗1were found up to ∼1400◦C.Thus,despite the strong thermal fluctuations at this extreme temperature,a great num-ber of 2-nm nanoclusters still exist.It follows,therefore,that the 2-nm nanoclusters formed in 14YWT alloy are stable.Notice the blue triangle data point after cooling down to room temperature,further confirming the thermal stability of the nanoclus-ters.Limited coarsening can be seen,as the data point after cool down is slightly below that before heating.3.3.High temperature diffusionUnlike conventional ferric steel,14YWT alloys are thermally stable even at tem-peratures up to 1300◦C (0.87T m ,the melting temperature).This is illustrated in Fig.8(a)and (b),where the in situ neutron diffraction patterns for 14YWT and the nanoclusters-free FNAN-1are compared.After two cycles of heating,the diffraction pattern for 14YWT is virtually unchanged after 3h of annealing at 1300◦C.110010009008007000.1110100C o r r e l a t i o n L e n g t h (A )T (C)Fig.7.Magnetic correlation length determined for pure iron as a function of temper-ature.The data were taken from Ref.[26].The magnetic correlation length increases exponentially as the Cure temperature isapproached.Fig.8.(a)Temperature profile and diffraction data for 14YWT measured in situ at GPPD.The experiment covered two thermal cycles.Each step at high temperatures is approximately 3h.The vertical axis is the data file number.The left panel shows the temperature profile as a function of the data file number,whereas the right panel is a two-dimensional plot showing the evolution of neutron diffraction patterns with the data file number or temperature.(b)Temperature profile and diffraction data for FNAN-1measured in situ at GPPD.Each step at high temperatures is approximately 3h.The designation of the axes in (b)is the same as in (a).(c)Comparison of neu-tron diffraction data for 14YWT and FNAN-1collected in situ at 1300◦C.For GPPD,each data was sum over 3h.Data for VULCAN at the Spallation Neutron Source was collected for 4min in a vacuum furnace after 1h anneal at 1300◦C.Note that GPPD and VULCAN have different resolutions,so the peak widths are different.100X.L.Wang et al./Journal of Alloys and Compounds 529 (2012) 96–101Fig.9.Room temperature diffraction patterns of 14YWT (a)and FNAN-1(b)after step-wise heating (annealing)to 1300◦C.The horizontal axis is lattice spacing.The vertical axes designate the angle of the individual detectors.These detectors are grouped into detector banks.For details of the detector layout on GPPD,see Ref.[27].The nominal angle of each detector bank is given on the right side of the two-dimensional plot.Diffraction data from different detectors are stacked vertically (separated by the dark horizontal bands),with their diffraction peaks lined up in lattice spacing.With the furnace in place,only two of the detector banks (centered around ±90◦)were accessible due to the limited size of the furnace windows.The 14YWT alloy (a)is stable as evidenced from the powder diffraction lines.FNAN-1alloy (b)without nanoclusters experienced massive recrystallization and grain growth during heating.On the other hand,dramatic changes are seen for the diffraction peaks from FNAN-1.Most of the strong peaks in FNAN-1(e.g.,(200))are significantly weak-ened upon heating to 1150◦C,while (211)peak first decrease and then intensifies.In Fig.8(c),the diffraction patterns collected at 1300◦C are compared.While the diffraction pattern for nanocluster-free FNAN-1becomes very diffusive at high tem-peratures,alloy 14YWT retains the clean body-centered-cubic diffraction pattern up to a maximum annealing temperature of ∼1300◦C.The change of diffraction inten-sity with temperature,as shown in Fig.8(b)indicates that FNAN-1recrystallized upon heating and annealing at 1300◦C,see Section 4below for more details.Addi-tional data for 14YWT from the new VULCAN instrument at the Spallation Neutron Source are also included in Fig.8(c).Again,sharp diffraction peaks are identified,indicating that the sample is stable.Note that the VULCAN and GPPD have different resolutions,so the diffraction peak widths are different.Here,the fine resolution with VULCAN is evident.With the furnace in place,only a small range of reciprocal lattice space is covered due to the limited size of the furnace window.To confirm that the intensity change in FNAN-1is indeed due to recrystallization,we conducted additional measurements on GPPD on samples cooled to room temperature,without the furnace.Before presenting the full data set collected over all detectors,it is instructive to describe briefly how a time-of-flight diffractometer such as GPPD works.The detec-tor coverage for GPPD has been given in detail elsewhere [27],with the scattering angle ranging from 30◦to 150◦.For a time-of-flight diffractometer,each detector can collect a whole diffraction pattern,using an incident beam over a large wavelength range.Data from different detectors can be time-focused,i.e.,lined up with the same d-spacing.For powder samples (of random grains),the data from different detectors can be summed together to produce a single diffraction pattern.For materials with preferred grain orientations,data from different detectors no longer look the same.Analysis of the difference yields information on the texture of the sample.In Fig.9(a)and (b),diffraction data collected over all detectors on sam-ples annealed at 1300◦C are presented.It can be seen that recrystallization or grain growth in 14YWT is strongly suppressed,as its diffraction pattern remainspowder-like.In fact,the diffraction patterns recorded at room temperature before and after annealing are almost identical,demonstrating full recovery of the microstructure.On the other hand,FNAN-1alloy containing no nanoclusters expe-rienced massive recrystallization.Its diffraction pattern,after cooling back to room temperature,is spotty,with bright single crystal spots aligned along the weak poly-crystal lines,as shown in Fig.9(b).The contrasting images of Fig.9(a)and (b)show that in nanostructured steel,the grain boundary mobility is significantly slower.Since the grain boundary mobility is directly related to bulk diffusion,this result means that the diffusion of Fe atoms is slow,even at temperatures up to 1300◦C.4.DiscussionThe remarkable stability of Y–Ti–O nanoclusters is the result of strong chemical bonding that holds Y,Ti,and O together.First-principle calculations offered some clues as to why this is hap-pening [28].A new alloying state forms,the calculations show,with a binding energy between the stable states of TiO 2and Y 2Ti 2O 7.The formation of the new alloying state is facilitated by mechanical alloying,which created high density vacancies.Although the solu-bility of O in the body-center-cubic Fe lattice is extremely limited,O atoms binds strongly with vacancy,with a binding energy as high as −1.5eV.This greatly increases the solubility of O in the pres-ence of pre-existing vacancies.The presence of Y is essential for creating stable nanoclusters states,but the Y concentration must also be carefully controlled.Without Y,the stable alloying state is TiO 2oxide phase,with a heat of formation of −4.3eV per oxygen atom [28].In the presence of Y,the cluster state becomes compet-itive,and a small addition of Y actually promotes the formation of nanoclusters instead of TiO 2.With too much Y,however,the Y 2Ti 2O 7oxide phase,with a heat of formation of −5.6eV,is likely to precipitate.Thus,the condition for stable Y–Ti–O nanoclusters requires a well-dispersed Y concentration with more Ti than Y in the nanoclusters.Now that the nanoclusters are thermodynamically stable,and many of them remain at extreme temperatures up to 1400◦C,why are they thermally stable exhibiting minimal growth?To answer this question,we must consider both the thermodynamic driving force and growth kinetics.For normal particle growth,the pri-mary driving force is minimization of the interfacial energy [29].However,first-principle calculations suggest that the new metallic cluster is coherent with the Fe lattice.The neutron diffraction pat-terns in 14YWT remained body-centered-cubic like;no peaks from other phases were observed.These data support the predication by first-principle calculations.If the clusters are indeed coherent,the driving force for normal particle growth,due to high incoher-ent interfacial energy,no longer exists.The kinetics is governed by short-range and long-range transport of atoms that make up the cluster [30].The former involves the transfer of atoms across the particle boundary or interface,whereas the latter is by dif-fusion in which atoms are transported over distances of several atomic spacing.In Y–Ti–O nanoclusters,the atoms are held tightly by strong chemical bonding.Further growth requires concerted or collective migration of Y,Ti,and O-vacancy pairs.This requirement severely constraints the mobility of the desired chemical species.In order for an oxygen atom to move,for example,a vacancy must be created first.The energy required to break an O-vacancy pair is 1.5eV,far beyond the energy from thermal fluctuation,which is only ∼150meV at 1400◦C.Even if some chemical species are moved close to a cluster by long-range diffusion,the delicate chemistry requirement (e.g.,for Y as discussed earlier)can still prevent them to attach to the interface of the clusters limiting the growth.In nanostructured steel with minor N and C addition,the improvement in creep properties is attributed to a mechanism of grain boundary pinning by carbonitride precipitates [14].Hayashi et al.proposed a similar mechanism for the dramatically improved creep resistance in the present 14YWT alloy [24].Their analysis,X.L.Wang et al./Journal of Alloys and Compounds529 (2012) 96–101101based on microscopy and creep test results,suggests that the pin-ning effect of segregation and nanoclusters formation might be strong enough to prevent grain-boundary migration and grain-boundary sliding,even at test temperatures up to800◦C.There appears to be microscopic evidence from the high-temperature neutron diffraction experiments that supports the above proposed mechanism.As shown in Fig.9(a),recrystallization in14YWT alloys is strongly suppressed.This means that com-pared to cluster-free alloy FNAN-1,the grain-boundary mobility and hence the diffusion of Fe atoms in nanostructured steel is signif-icantly reduced.Indeed,O atoms in the lattice or grain boundaries serve as a sink for vacancies due to their strong binding energy.This will severely limit the diffusion of Fe atoms.Grain-boundary pin-ning by the coherent nanoclusters also contributes to the sluggish diffusion kinetics of Fe atoms.Notice that these data were collected after annealing at1300◦C.Even at this temperature,the mobility of Fe atoms is sufficiently slow to prevent massive recrystallization to occur.5.Concluding remarksIn summary,we have carried out a systematic investigation of the structure and thermal stability of a nanostructured ferritic alloy with in situ neutron scattering experiments.From the experimental results,we conclude the following:1.Nanoscale particles in the14YWT nanostructured ferritic alloyhave a bi-modal particle size distribution,with maxima around 2and12nm,respectively.2.The2-nm clusters survived up to1400◦C,suggesting thatthey are thermodynamically stable.The unusual stability of the nanoclusters is attributable to the unique chemistry,as thefirst-principle calculations predicted.3.The nanoclusters in14YWT are thermally stable,with littlegrowth even at high temperatures.The reduced cluster growth is attributed to the coherent nature of the cluster state and the chemistry requirement(e.g.,vacancies).4.The microstructure of the Fe matrix is stable,as evidenced fromthe lack of recrystallization at temperatures up to1300◦C.This result indicates that the mobility of grain boundaries or bulk diffusion is strongly suppressed.The creep rate of14YWT nanostructured ferritic alloy is six orders of magnitude slower,which,along with its incredible resis-tance against radiation damage[31,32],offers a great potential for use in advanced energy conversion systems.While the exact mechanisms of the low creep rate are not yet fully understood,the unusual thermal stability of the nanoclusters and the microstruc-ture of the Fe matrix must be playing a major role.The results of this study are expected to have a broad implication in understand-ing the emergent behavior of complex alloys and in the synthesis of new-generation nanostructured materials.Note added in proofAfter the submission of this manuscript,we became aware of the publication by Hirata et al.[33]which proved that the nan-oclusters discussed in this paper are indeed coherent with the bcc steel matrix.AcknowledgementsResearch sponsored by the Materials Sciences and Engineering Division,Office of Basic Energy Sciences,US Department of Energy. Atom probe tomography(MKM)was supported by ORNL’s Shared Research Equipment(SHaRE)User Facility,which is sponsored by the Office of Basic Energy Sciences,US Department of Energy.The Spallation Neutron Source was operated with support from the Sci-entific User Facilities Division,Office of Basic Energy Sciences,US Department of Energy.We thank Dr.D.T.Hoelzer for providing the 14YWT samples for this study.References[1]ai,T.Nishida,T.Okuda,T.Yoshitake,J.Nucl.Sci.Technol.35(1998)294–300.[2]rson,P.J.Maziasz,I.S.Kim,K.Miyahara,Scripta Mater.44(2001)359–364.[3]ler,E.A.Kenik,K.F.Russell,L.Heatherly,D.T.Hoelzer,P.J.Maziasz,Mater.Sci.Eng.A:Struct.Mater.Properties Microstruct.Process.353(2003) 140–145.[4]I.S.Kim,B.Y.Choi,Met.Mater.Int.8(2002)265–270.[5]R.L.Klueh,P.J.Maziasz,I.S.Kim,L.Heatherly,D.T.Hoelzer,N.Hashimoto,E.A.Kenik,K.Miyahara,J.Nucl.Mater.307(2002)773–777.[6]D.T.Hoelzer,J.Bentley,M.A.Sokolov,ler,G.R.Odette,M.J.Alinger,J.Nucl.Mater.367(2007)166–172.[7]I.S.Kim,B.Y.Choi,C.Y.Kang,T.Okuda,P.J.Maziasz,K.Miyahara,ISIJ Int.43(2003)1640–1646.[8]H.Kishimoto,M.J.Alinger,G.R.Odette,T.Yamamoto,J.Nucl.Mater.329(2004)369–371.[9]J.H.Schneibel,M.Heilmaier,W.Blum,G.Hasemann,T.Shanmugasundaram,Acta Mater.59(2011)1300–1308.[10]J.H.Schneibel,C.T.Liu,ler,ls,P.Sarosi,M.Heilmaier,D.Sturm,Scripta Mater.61(2009)793–796.[11]M.J.Alinger,Materials,University of California,Santa Barbara,2004.[12]M.J.Alinger,G.R.Odette,D.T.Hoelzer,J.Nucl.Mater.329(2004)382–386.[13]M.J.Alinger,G.R.Odette,D.T.Hoelzer,Acta Mater.57(2009)392–406.[14]M.Taneike,F.Abe,K.Sawada,Nature424(2003)294–296.[15]ler,C.L.Fu,M.Krcmar,D.T.Hoelzer,C.T.Liu,Front.Mater.Sci.China3(2009)9–14.[16]F.Bergner,A.Ulbricht,H.Hein,M.Kammel,J.Phys.Condensed Matter20(2008).[17]J.F.Loffler,H.B.Braun,W.Wagner,Phys.Rev.Lett.85(2000)1990–1993.[18]M.Ohnuma,J.Suzuki,S.Ohtsuka,S.W.Kim,T.Kaito,M.Inoue,H.Kitazawa,ActaMater.57(2009)5571–5581.[19]L.Yang,ler,X.L.Wang,C.T.Liu,A.D.Stoica,D.Ma,J.Almer,D.Shi,Adv.Mater.21(2009)305–308.[20]X.L.Wang,T.M.Holden,G.Q.Rennich,A.D.Stoica,P.K.Liaw,H.Choo,C.R.Hub-bard,Phys.B:Condensed Matter385(2006)673–675.[21]X.L.Wang,T.M.Holden,A.D.Stoica,K.An,H.D.Skorpenske,A.B.Jones,G.Q.Rennich,E.B.Iverson,Mater.Sci.Forum652(2010)105–110.[22]T.E.Mason,D.Abernathy,I.Anderson,J.Ankner,T.Egami,G.Ehlers,A.Ekkebus,G.Granroth,M.Hagen,K.Herwig,J.Hodges,C.Hoffmann,C.Horak,L.Horton,F.Klose,rese,A.Mesecar,D.Myles,J.Neuefeind,M.Ohl,C.Tulk,X.L.Wang,J.Zhao,Physica B385–386(2006)955–960.[23]U.Keiderling,Appl.Phys.A74(2002)S1455–S1457.[24]T.Hayashi,P.M.Sarosi,J.H.Schneibel,ls,Acta Mater.56(2008)1407–1416.[25]M.F.Collins,Magnetic Critical Scattering,Oxford University Press,Inc.,UK,1989.[26]H.A.Gersch,C.G.Shull,M.K.Wilkinson,Phys.Rev.103(1956)525–534.[27]Y.D.Wang,H.B.Tian,A.D.Stoica,X.L.Wang,P.K.Liaw,J.W.Richardson,Nat.Mater.2(2003)101–106.[28]C.L.Fu,M.Krcmar,G.S.Painter,X.Q.Chen,Phys.Rev.Lett.99(2007).[29]G.Gottstein,Physical Foundations of Materials Science,Springer,Berlin,2004.[30]R.D.Doherty,in:R.W.Cahn,P.Hason(Eds.),Physical Metallurgy,Elsevier Sci-ence B.V.,The Netherlands,1998.[31]G.R.Odette,M.J.Alinger,B.D.Wirth,Annu.Rev.Mater.Res.38(2008)471–503.[32]A.G.Certain,K.G.Field,T.R.Allen,ler,J.Bentley,J.T.Busby,J.Nucl.Mater.407(2010)2–9.[33]A.Hirata,T.Fujita,Y.R.Wen,J.H.Schneibel,C.T.Liu,M.W.Chen,Nat.Mater.10(2011)922–926.。