Synthesis of MoVTeNb Oxide Catalysts with Tunable Particle Dimensions
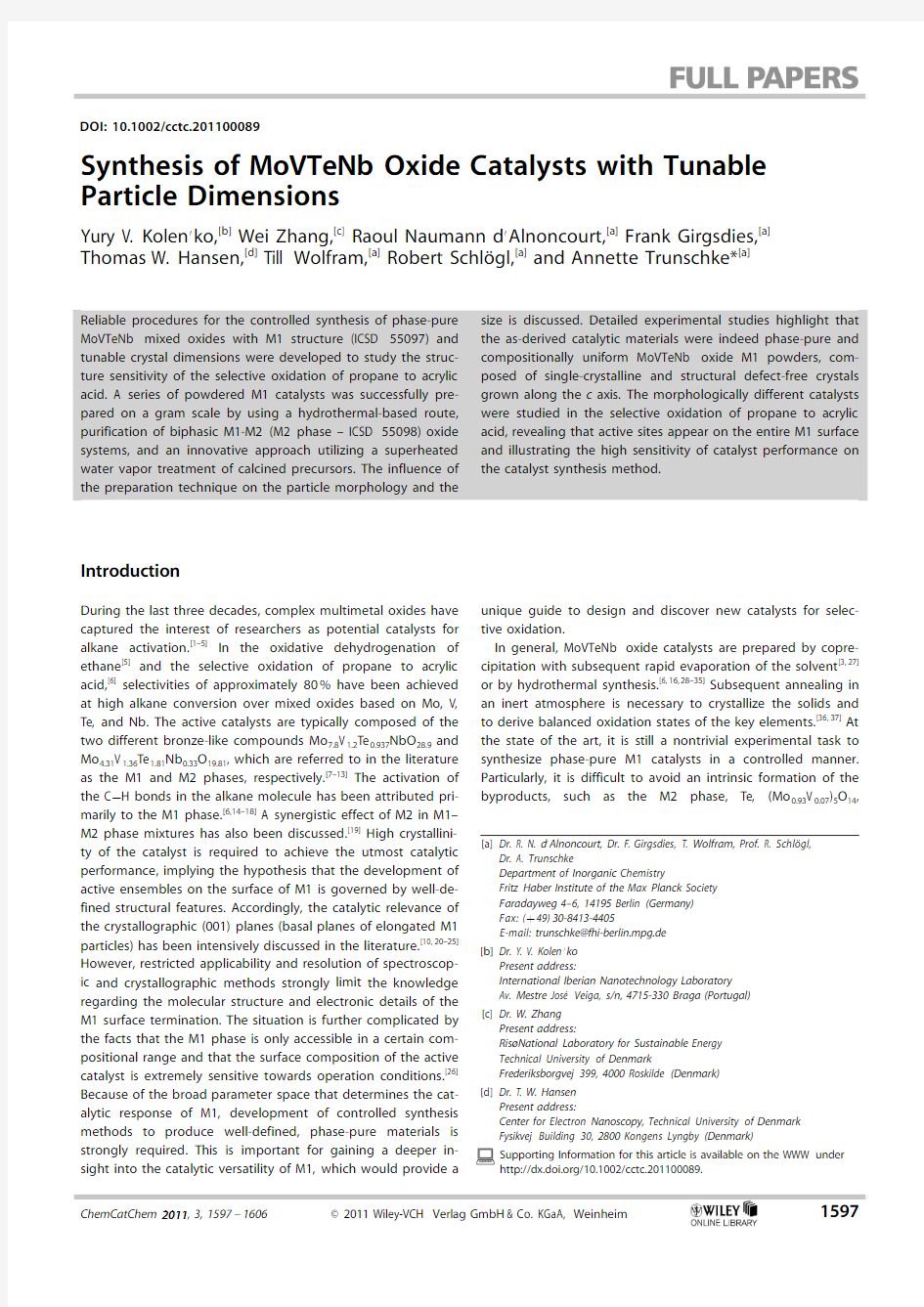

DOI:10.1002/cctc.201100089
Synthesis of MoVTeNb Oxide Catalysts with Tunable Particle Dimensions
Yury V.Kolen’ko,[b]Wei Zhang,[c]Raoul Naumann d’Alnoncourt,[a]Frank Girgsdies,[a] Thomas W.Hansen,[d]Till Wolfram,[a]Robert Schl?gl,[a]and Annette Trunschke*[a]
Introduction
During the last three decades,complex multimetal oxides have captured the interest of researchers as potential catalysts for alkane activation.[1–5]In the oxidative dehydrogenation of ethane[5]and the selective oxidation of propane to acrylic acid,[6]selectivities of approximately80%have been achieved at high alkane conversion over mixed oxides based on Mo,V, Te,and Nb.The active catalysts are typically composed of the two different bronze-like compounds Mo7.8V1.2Te0.937NbO28.9and Mo4.31V1.36Te1.81Nb0.33O19.81,which are referred to in the literature as the M1and M2phases,respectively.[7–13]The activation of the CàH bonds in the alkane molecule has been attributed pri-marily to the M1phase.[6,14–18]A synergistic effect of M2in M1–M2phase mixtures has also been discussed.[19]High crystallini-ty of the catalyst is required to achieve the utmost catalytic performance,implying the hypothesis that the development of active ensembles on the surface of M1is governed by well-de-fined structural features.Accordingly,the catalytic relevance of the crystallographic(001)planes(basal planes of elongated M1 particles)has been intensively discussed in the literature.[10,20–25] However,restricted applicability and resolution of spectroscop-ic and crystallographic methods strongly limit the knowledge regarding the molecular structure and electronic details of the M1surface termination.The situation is further complicated by the facts that the M1phase is only accessible in a certain com-positional range and that the surface composition of the active catalyst is extremely sensitive towards operation conditions.[26] Because of the broad parameter space that determines the cat-alytic response of M1,development of controlled synthesis methods to produce well-defined,phase-pure materials is strongly required.This is important for gaining a deeper in-sight into the catalytic versatility of M1,which would provide a unique guide to design and discover new catalysts for selec-tive oxidation.
In general,MoVTeNb oxide catalysts are prepared by copre-cipitation with subsequent rapid evaporation of the solvent[3,27] or by hydrothermal synthesis.[6,16,28–35]Subsequent annealing in an inert atmosphere is necessary to crystallize the solids and to derive balanced oxidation states of the key elements.[36,37]At the state of the art,it is still a nontrivial experimental task to synthesize phase-pure M1catalysts in a controlled manner. Particularly,it is difficult to avoid an intrinsic formation of the byproducts,such as the M2phase,Te,(Mo0.93V0.07)5O14, [a]Dr.R.N.d’Alnoncourt,Dr.F.Girgsdies,T.Wolfram,Prof.R.Schl?gl,
Dr.A.Trunschke
Department of Inorganic Chemistry
Fritz Haber Institute of the Max Planck Society
Faradayweg4–6,14195Berlin(Germany)
Fax:(+49)30-8413-4405
E-mail:trunschke@fhi-berlin.mpg.de
[b]Dr.Y.V.Kolen’ko
Present address:
International Iberian Nanotechnology Laboratory
Av.Mestre Jos?Veiga,s/n,4715-330Braga(Portugal)
[c]Dr.W.Zhang
Present address:
Ris?National Laboratory for Sustainable Energy
Technical University of Denmark
Frederiksborgvej399,4000Roskilde(Denmark)
[d]Dr.T.W.Hansen
Present address:
Center for Electron Nanoscopy,Technical University of Denmark
Fysikvej Building30,2800Kongens Lyngby
(Denmark)
Supporting Information for this article is available on the WWW under
https://www.360docs.net/doc/0516407420.html,/10.1002/cctc.201100089.
V 0.95Mo 0.97O 5,TeMo 5O 16,MoO 3,and MoO 2,during material syn-thesis.These facts motivated us to improve existing synthetic methods and to develop novel routes,enabling the production of single-phase M1and control over the particle size and mor-phology.The aim is to gain access to materials that will allow us to investigate the structure sensitivity of M1in the selective oxidation of propane to acrylic acid.Herein,we describe the successful preparation of high-quality M1catalysts in large batch sizes,exhibiting different particle dimensions and shapes,while keeping the chemical composition of the bulk almost constant.The catalysts are studied in the selective oxi-dation of propane to acrylic acid,addressing the structure sen-sitivity of the crystalline,single-phase M1MoVTeNb oxides in this reaction.
Results and Discussion
The majority of studies dealing with MoVTeNb mixed oxides focused on the catalytic properties of these materials.[29,38]For this reason,the material chemistry was explored less systemati-cally,and the phase purity of the synthesized products was not usually thoroughly verified.At the same time,the presence of metal oxide admixtures can play a considerable role in the catalytic activity and selectivity for the oxidation of propane to acrylic acid.[14–18]Such “phase purity”artifacts make it difficult to elucidate and understand the exact catalytic responses,masking the intrinsic behavior of the M1phase.Despite this compositional impact,it has been suggested that the morphol-ogy and the particle size of the M1phase influences the reac-tivity of M1as well,[39]although the synthesis of the M1crystals on various scales and forms still remains a challenge.Addition-ally,most of the reported routes to MoVTeNb mixed oxides are not well developed in terms of product purity,structural and chemical homogeneity,as well as viability of large scale pro-duction.To this end,a synthesis with particular emphasis on
control over the size and shape of the M1particles is attempt-ed in the current study by preparing a series of MoVTeNb oxide M1catalysts by utilizing a hydrothermal synthesis (Method 1),an adapted and improved precipitation/evapora-tion/crystallization/purification method (Method 2),and an in-novative route employing superheated water vapor treatment of an oxide precursor (Method 3);the resultant catalysts are re-ferred to as HT-M1,W-M1,and SH-M1,respectively.The phase composition,degree of crystallinity,unit cell parameters,aver-age width and length of the crystals,surface areas determined by using the Brunauer–Emmett–Teller method (A BET ),total pore volume,ratio of the surface areas of the basal planes and the lateral surface of the three as-derived catalysts,and catalytic conversions and selectivities are given in Table 1,whereas the compositional details for these materials are summarized in Table 2.
X-Ray diffraction (XRD)patterns of the catalyst precursors synthesized by using the three methods are presented in Figure 1.Hydrothermal synthesis at 423K for 48h (Method 1)resulted in the formation of a product exhibiting poor local or-dering along the c axis,as illustrated by weak (001)reflections from the orthorhombic MoVTeNb oxide M1phase (ICSD No.55097).A similar catalyst precursor,having short-range struc-tural ordering,is obtained after spray-drying the slurry with subsequent calcination at 548K (Method 2).In contrast,the XRD pattern of the product prepared in a similar fashion,but starting from a homogeneous complex solution (Method 3),in-dicates an essentially amorphous nature of the precursor.
The morphology evolution during the syntheses was ob-served by scanning electron microscopy (SEM,Figure S1–S3in the Supporting Information).The hydrothermally derived pre-cursor (Method 1)consisted of highly associated irregular mon-olithic structures with a lack of particulate structure regions or crystal faceting at the surface (Figure S1A in the Supporting In-formation),which is consistent with the poor crystallinity
of
A.Trunschke et al.
this catalyst precursor revealed by using XRD (Figure 1).The precursors of Method 2(Figure S2A in the Supporting Informa-tion)and Method 3(not shown)also exhibited a flat or feature-less morphology with a lack of particulate structure.
The XRD patterns from the products of the intermediate treatment steps of Methods 2and 3are shown in Figure 2.Thermal treatment of the catalyst precursor from Method 2at 873K in Ar leads to the formation of a mixture of two com-pounds:approximately 60wt %of the M1phase and approxi-mately 40wt %of the pseudo-hexagonal orthorhombic MoV-TeNb oxide phase (M2,ICSD No.55098),which is consistent with literature.[6]This biphasic precursor exhibits a rod-like (predominant)and a wide irregular plate-like particle morphol-ogy (Figure S2B in the Supporting Information).The interac-tion volume for the energy-dispersive X-ray spectroscopy (EDX)analysis hints that the rods are the M1phase,and the plates are the M2phase (insets in Figure S2B in the Supporting Infor-mation).Analogous to Baca et al.,[31]the H 2O 2washing is ap-plied to remove unwanted M2,and,according to the XRD,this procedure serves to effectively eliminate this admixture (Fig-ure 2A).Figure 2B shows an expanded view of the region,in which the strongest (220)peaks of M2phase for the samples obtained by using Method 2disappeared after washing with H 2O 2.This clearly demonstrates an entire removal of the M2admixture by washing with H 2O 2.Washing also leads to signifi-cant changes in the microstructure (Figure S2C in the Support-ing Information).In addition to the removal of the wide plate-like appearance (M2phase),the original rod-like crystals of the M1phase show erosion of the basal c surface of the rods (inset in Figure S2C in the Supporting Information).This is probably caused by an enhanced leaching of the elements,caused by H 2O 2,from the open channels terminated by the a b plane of M1and partially filled with Te atoms.
Superheated water vapor treatment at 773K for 2h of the precursor prepared by using Method 3yields a single-phase M1catalyst containing only a small amount (%5wt %)of ele-mental Te byproduct (ICDD No.36-1452;Figure 2A).However,
the powder XRD pattern of M1exhibits a strong line-broaden-ing effect,which is probably caused by the low crystallinity and the nanocrystalline nature of the product.SEM shows the presence of two different types of particle morphologies in the powder derived from superheated water vapor treatment:a rod-like morphology and the predominant particle-like appear-ance on a nanometer scale (Figure S3A in the Supporting In-formation).
Finally,the active catalysts were obtained by crystallization of the precursor materials through annealing at 873K in Ar for 2h.The particle morphology and the size of the resulting phase-pure M1catalysts is quite variable,depending on the applied synthesis method (Figures 3and S4in the Supporting Information).SEM observations of the thermally crystallized HT-M1(Figure 3A and Figure S1B in the Supporting Informa-tion)reveal that this sample consists of elongated sub-micro-meter-sized particles with a faceted cylindrical morphology.Many of these particles appear to have a fairly plate-like shape with an ellipsoidal basal plane.These particles
spontaneously
Figure 1.Powder XRD patterns of poorly crystalline catalyst precursors from Methods 1–3.Tick marks below the patterns correspond to the positions of the Bragg reflections expected for the M1
phase.
Figure 2.A)Comparison of the powder XRD patterns of the catalyst precur-sors after the respective treatments.The primary (101)reflection of the Te byproduct at 2q =27.68is denoted by ^,and the primary (220)reflection of the M2phase at 2q =28.38is denoted by *.B)Enlargement of the angular region from 2q =26–308(gray area in A),which is provided for clarification of the H 2O 2washing efficiency.Tick marks below the patterns correspond to the positions of the Bragg reflections expected for the M1phase.
MoVTeNb Oxide Catalysts
interlocked,thus generating a highly aggregated microstruc-ture of HT-M1.The crystals exhibit a rather nonuniform size distribution with an average width and length approximately 213and 421nm,respectively (Table 1,Figure S4A in the Sup-porting Information).Higher magnification images of this prod-uct clearly reveal the multistep structuring of the lateral sur-face of the cylindrical particles and a flat termination of the basal planes (inset in Figure S1B in the Supporting Informa-tion).W-M1particles from Method 2(Figure 3B)are nearly two times smaller than HT-M1crystals from Method 1,showing a nonuniform size distribution with an overall width and length of approximately 115and 199nm,respectively (Table 1,Fig-ure S4B in the Supporting Information).Again,the lateral sur-face exhibits a stepped nature,but the termination of the basal planes appears to be flat.
SEM images of the product produced by using Method 3are displayed in Figure 3C and Figure S3B in the Supporting Infor-mation.The SH-M1catalyst consists of cylindrical structures on a sub-micrometer scale and faceted,generally elongated nano-particles,exhibiting moderate aggregation.In contrast to the HT-M1and W-M1products,SH-M1crystals are considerably smaller in size with a nonuniform distribution.The average width and length of the particle is approximately 59and 180nm,respectively (Table 1,Figure S4C in the Supporting In-formation).
The XRD patterns for the hydrothermally derived catalyst crystallized through annealing at 873K in Ar for 2h prove the phase-purity of the HT-M1catalyst (Figure 4A).Similar results were previously obtained by several research groups.[32,33,35]Heat activation of the H 2O 2-washed powder does not lead to any significant changes in the phase composition;the as-pre-pared W-M1catalyst is single-phase M1(Figure 4A).Owing to
the liberation of Te through sublimation and phase crystalliza-tion during annealing,the same result was observed after ther-mal treatment of the precursor synthesized by the superheat-ed water vapor treatment (Figure 4A).Remarkably,the XRD patterns of all of the final products are very similar,and peaks related to the presence of any other crystalline phases,in par-ticular traces of the M2phase admixture,were not detected (Figure 4B).Deviations,especially with respect to the reference M1structure,[11]may be owed to differences in the metal-site occupancy because of varying chemical compositions.The lat-tice parameters extracted from the XRD data are listed in Table 1.Although the differences in the unit cell parameters are small,it can be concluded that all three derived catalysts are structurally very similar.
Using the XRD measurements employing rutile TiO 2SRM 674b as an internal standard,it is established that the degree of crystallinity of the derived M1catalysts is extremely high,lying in the range of 80–85wt %,depending on the prepara-tion method.The catalysts from Methods 2and 3exhibit slightly higher degrees of crystallinity (%85wt %)than the hy-drothermally prepared catalyst HT-M1(%80wt %).This
obser-Figure https://www.360docs.net/doc/0516407420.html,parison of the morphological features in SEM images of the catalysts A)HT-M1,B)W-M-1,and C)
SH-M1.
Figure 4.A)Comparison of the powder XRD patterns of HT-M1,W-M1,and SH-M1catalysts,which closely resemble the patterns of the M1phase with the expected Bragg reflections denoted by tick marks.B)Enlargement of the angular region from 2q =26–30(gray area in A),indicating the lack of the primary reflection of the most likely M2phase admixture.
A.Trunschke et al.
vation is probably related to the fact that the former solids were treated twice at high temperatures in the course of their preparation.This way,structurally more perfect products may be obtained because of enhanced annealing results in the re-duction of lattice defects and the conversion of surface reac-tion products.The remaining noncrystallinity of 15–20wt %is most likely a result of an intrinsic,structurally disordered sur-face layer of M1particles,as the surface region of any crystal-line material,regardless of phase purity,will not diffract in the same way as the bulk owing to relaxation of the crystal struc-ture.[40]Overall,all of the estimated values are in good agree-ment with an approximately 89.5wt %degree of crystallinity reported for the internal standard used in the XRD experi-ments (rutile TiO 2SRM 674b),[40]indicating excellent crystallinity of the synthesized catalysts.
A low-magnification transmission electron microscopy (TEM)image of the HT-M1catalyst prepared by using Method 1is shown in Figure 5A,presenting a section containing excep-tionally small particles.A representative HRTEM image along
the [65
ˉ0]zone axis is shown in Figure 5B,and the correspond-ing fast Fourier transform pattern is given as an inset.Both
reveal an overall crystallinity and M1structure of the HT-M1sample being present even for the smallest particles in the batch.The lattice fringes represent the (560)and (001)planes of the orthorhombic M1structure,indicating that the particles grow along the c axis.To investigate the chemical composition of the HT-M1particles,this catalyst is analyzed by using ele-ment-specific EDX mapping by using a scanning TEM(STEM),and the results are presented in Figure 5C.Low-magnification Mo,V,Te,Nb,and O EDX maps highlight the homogeneous distribution of the constituent elements inside the HT-M1crys-tals,confirming that the catalyst is indeed single-phase M1,devoid of any secondary clusters or precipitates.
A low-magnification TEM image of the catalyst W-M1shows the typical fine microstructure of the product from Method 2(Figure 6A).The sample consists of well-shaped submicrometer sized cylindrical particles.Figure 6
B displays a typical [110]*
electron diffraction pattern from a rod-like crystal.Electron dif-fraction reveals high crystallinity of the rods,which is also con-firmed by using XRD (Figure 4,Table 1).The spots of the elec-tron diffraction (ED)pattern can be completely indexed with reference to the orthorhombic Pba 2space group,using the M1phase unit cell parameters (ICSD No.55097).All of the ED patterns along the long axis of the rods have the same geome-try,revealing that each M1particle is a single crystal.From the orientation relation between image and diffraction,it also ap-pears that the W-M1rods are exclusively oriented with their long axis along [001],an indication of c -axis growth.
A representative HRTEM image of the W-M1catalyst is pre-sented in Figure 6C.In this figure,a M1crystal with well-devel-oped lattice fringes is seen,reflecting its good bulk
crystallinity.
Figure 5.A)Low-magnification TEM image,B)representative HRTEM image demonstrating the extended lattice fringes,and C)STEM image (together with the respective EDX mapping for the constituent elements V,Te,Nb,and O)of the HT-M1catalyst prepared by using Method
1.
Figure 6.A)A low-magnification TEM image and B)ED pattern along with the corresponding TEM image (inset)of the W-M1catalyst prepared by
using Method 2.C)A representative HRTEM image of the sample,showing a 1–2nm thick amorphous layer at the edge of M1particles.D)STEM image of a single crystal of W-M1together with the respective EDX mapping of the constituent elements (Mo,V,Te,Nb,and O).
MoVTeNb Oxide Catalysts
However,the surface of the particle is characterized by an ap-proximately 1–2nm thick restructured layer,which agrees fairly well with the XRD data on the degree of crystallinity (Table 1).Investigation of the individual M1particles with dis-tinct rod-like morphology by using EDX mapping at a high-magnification reveals that Mo,V,Te,Nb,and O are uniformly distributed throughout the crystals (Figure 6D),thus confirm-ing the lack of any compositional inhomogeneities in the cata-lyst synthesized by using Method 2.
In contrast to the HT-M1and W-M1catalysts,TEM shows the presence of two different types of crystallized structures in the SH-M1obtained by using Method 3.This catalyst is comprised of long rod-like particles and predominantly nanosized crystals with dimensions on the order of 10–25nm wide by 30–100nm long (Figure 7A).Electron diffraction of this sample shows a distinct ring pattern,typical for small particle cluster-ing (Figure 7B).The rings of the ED pattern correspond to the M1phase (ICSD No.55097,orthorhombic,Pba 2,a =21.134 ,b =26.658 ,c =4.015 ).Rings originating from a secondary phase are not present.
Representative HRTEM images from rod-like and nanocrystal-line structures of SH-M1are shown in Figure 7B and C.The two M1appearances exhibit well-crystallized bulk structures reflected in well-developed lattice fringes.Close inspection of the individual crystals on a large scale reveals the absence of any type of structural defects throughout the particles,but the surface of the crystals is always characterized by a disordered layer on the order of about 1nm.To verify the chemical com-position of the SH-M1catalyst,energy-filtered TEM (EFTEM)el-
emental maps were acquired in STEM mode.A major advant-age of this technique is a higher resolution than for EDX map-ping.The results are shown in Figure 8,wherein a TEM micro-graph of several particles of SH-M1together with the corre-
sponding EFTEM elemental maps for Mo,V,Te,Nb,and O are presented.The elemental maps confirm the presence of all five elements inside the particles as well as demonstrate that the elemental distribution of the M1catalytic material is very ho-mogeneous.
In summary,although using different starting element ratios,the resulting chemical compositions of the M1catalysts syn-thesized by using three different methods are very similar (Table 2).Furthermore,element-specific EDX mapping at differ-ent magnifications together with EFTEM clearly highlight that the chemical composition of the single-crystal M1particles is very uniform,and no indication of the presence of metal oxide clustering or segregation effects is found.TEM,ED,and HRTEM analyses confirm that the particles of HT-M1and W-M1are phase-pure M1single crystals,lacking any structural
defects,
Figure 7.A)A low-magnification TEM image and B)ED pattern of the SH-M1catalyst prepared by Method 3,along with representative HRTEM images of two different appearances of the sample:C)long sub-micrometer-sized rod-like crystals and D)the most abundant elongated nanosized
crystals.
Figure 8.Zero-loss TEM image of SH-M1catalyst together,with the corre-sponding EFTEM images at the Mo M 3-edge (393eV energy loss),V L 2,3-edges (513/520eV energy losses),Te M 5,4-edges (572/582eV energy losses),Nb M 3-edge (363eV energy loss)and O K -edge (532eV energy loss),demonstrating uniform distribution of the elements inside the M1crystals.
A.Trunschke et al.
and oriented with their long axis along the c axis.Moreover, these crystals are characterized by a multistep structure of the lateral surface and are interlocked.It is believed that the over-all interlocking of HT-M1and W-M1particles,and consequently the formation of compact packaged aggregates,is attributable to a hard-grained agglomeration of the respective catalyst pre-cursors,wherein spontaneous crystal growth from poorly crys-talline,but c-axis oriented templates,has occurred under ther-mal treatment.It is not possible for the time being to give a definitive answer as to where their different shapes and non-uniform particle size distributions originate.One possible ex-planation is that it could be caused by differences in the rate of the crystal growth during the crystallization process under the thermal treatment at873K in Ar.Another interesting thought is that it might be related to the retention of the pri-mary particle morphology of the nuclei(epitaxial growth),al-though,in the present study,the fine microstructure of the catalyst precursors was not analyzed.
The morphology and microstructure of the catalyst prepared by using an innovative synthesis(Method3)is very different from that of Methods1and2.SEM and TEM reveal that the su-perheated water vapor treatment with subsequent annealing at873K in Ar effectively extended the overall M1particle mor-phology and size to well-defined predominantly elongated nanosized crystals(SH-M1).Besides the most abundant forma-tion of the nanocrystalline M1,SEM and TEM also indicate the presence of rod-like particles in the as-prepared SH-M1.ED and HRTEM studies reveal orthorhombic M1crystal structures, a single-crystal nature and defect-free local structures of the rods and nanoparticles in the
SH-M1catalyst prepared by
using Method3.
The formation of M1nano-
crystals is somewhat surprising,
because the orthorhombic unit
cell of the M1phase is already
on a nanoscale(a and b parame-
ters more than2nm),thus
making the synthesis of nano-
sized M1,wherein the particles
should consist of only a few tens
of unit cells,difficult.It might be
possible that nanocrystalline M1
is formed readily because of
crystallization under highly non-
equilibrium,superheated water
vapor conditions(773K,
%20M Pa).[41,42]In addition,the
amorphous nature of the cata-
lyst precursor derived by decom-
position of the complex solution
(Figure1)could perhaps be re-
sponsible for the M1nanoparti-
cles appearance as well.There-
fore,the intensive growth of M1
crystals from a template that is
structurally poorly ordered along the c axis is suppressed in the case of Method3compared to Methods1and2.
The synthetic efforts described above resulted in a series of M1catalysts possessing different fractions of the terminating (001)plane,ranging between15and30%(Table1),and differ-ent specific surface areas,but identical crystal structures and almost equal chemical compositions.The catalysts were ex-plored in the selective oxidation of propane to acrylic acid at a reaction temperature of653K,applying a feed composition of C3H8/O2/H2O/N2=3:6:40:51.The products formed under these reaction conditions are propylene,acrylic acid,acetic acid,and carbon oxides.Figure9shows the selectivity as a function of propane conversion.
To gain catalytic data at similar conversions for reason of comparison,the gas hourly space velocity(GHSV)is varied in six steps in the range between2500and48000hà1.W-M1and SH-M1are characterized by a high and almost identical activity in the applied GHSV range.However,HT-M1is less active,and the lowest GHSV(2500hà1)has to be applied in case of HT-M1 to reach the same conversions observed with W-M1and SH-M1(approximately22%)at the highest GHSV(48000hà1).This fact can be partly rationalized by the lower A BET of HT-M1,but the result also indicates that the density of active sites seems to be different on the three catalysts.
Propylene is the first intermediate in the selective oxidation of propane to acrylic acid that is desorbed into the gas phase. Irrespective of the morphological differences between the three catalysts,a similar trend with respect to the selectivity of propylene is observed with increasing conversion(Figure
9),
Figure9.Selectivity of A)propylene,B)acrylic acid,C)acetic acid,and D)carbon oxides as a function of propane
conversion in the selective oxidation of propane at653K over the three morphologically different M1catalysts(~
HT-M1;&SH-M1);*W-M1);feed composition:C3H8/O2/H2O/N2=3:6:40:51vol%,P=0.1013M Pa.
MoVTeNb Oxide Catalysts
suggesting a related nature of the active sites for propane acti-vation on all catalysts.The acrylic acid profiles of the SH-M1and HT-M1catalysts are very similar despite the fact that differ-ent fractions of the terminating (001)plane occur in these ma-terials (Figure 9,Table 1).W-M1is more selective towards acryl-ic acid at higher conversions.
The propane conversion is shown in Figure 10as a function of contact time.Three different assumptions with respect to the “active mass W”have been made in Figure 10,resulting in three different plots of the same data set normalized either to the entire mass of the catalysts (Figure 10A),the surface area
of the (001)plane (Figure 10B),or the specific surface area (Figure 10C).At very low contact times,the three morphologi-cally different catalysts converge irrespective of the kind of representation,suggesting similar intrinsic properties of the active centers in propane activation.Although the fractions of the (001)surface area of the SH-M1and W-M1samples are quite different (15and 29%of the total surface area,respec-tively;Table 1),the two catalysts show similar dependency with respect to propane conversion,when the contact time is normalized to the mass (Figure 10A)or to the (001)surface area (Figure 10B).If the contact time is normalized to the spe-cific surface area,SH-M1and HT-M1show similar trends in ac-tivity,but W-M1is more active per surface area,suggesting dif-ferences in the surface termination (Figure 10C).A systematic correlation between the fraction of the terminating (001)planes and the activity is not observed,indicating that active sites are abundant on all terminating planes of the M1parti-cles.The present kinetic study shows that even though phase-pure and highly crystalline M1catalysts appear to have well-defined and uniform active surface centers and that the type
of termination and the total number of actives sites strongly depends on the synthesis method.
In summary,straightforward evidence for particle size effects
or structure sensitivity of M1,for example,change in the per-formance because of different ratios of exposed lattice planes
on changing the particle size,was not found.This is in agree-ment with previous findings based on model studies,in which the catalysts primarily exposed the (001)plane to the reacting molecules because the lateral surface was covered by a layer of silica.[25]The present results of propane oxidation performed at a broad range of residence times provided further evidence that the basal plane of M1did not show preferred catalytic performance.At higher propane conversion,internal mass transport limitations and heat transfer effects may superim-pose the intrinsic catalytic properties of the M1catalysts.
Conclusions
Single-phase MoVTeNb mixed oxide M1catalysts for propane oxidation were prepared in large batch sizes by using three
different solution-based meth-ods.These multistep approaches yielded M1materials exhibiting similar structural and composi-tional properties,but markedly different morphologies and di-mensions.It was found that sub-micrometer sized plate-like M1particles can be easily synthe-sized by using a hydrothermal-based method,but smaller in size and mostly continuous M1rods were readily produced by the purification route.Further-more,it was also shown that the synthesis method based on su-perheated water vapor treat-ment could generate a nanocrystalline M1phase.Systematic characterization supported the conclusion that the synthesized
M1products have a very homogeneous chemical composition and well-defined structure and microstructure.All three de-rived M1catalysts were active in the direct oxidation of pro-pane to acrylic acid.The different shapes of the primary cata-lyst particles enabled studies of the structure sensitivity of M1in propane oxidation.The identical intrinsic catalytic properties of M1catalysts with different fractions of surface area of the basal plane per mass catalyst gave clear evidence that not only the basal plane,but the entire surface of M1was involved in
the activation of propane.This observation is in line with the
picture of M1as a self-supporting catalyst,the crystalline
matrix of which generates an active two-dimensional surface layer of a thickness in the nanometer range that bears the cat-alytically active ensembles.[26]The chemical nature of this layer is subject of current research.
Experimental Section Starting materials Ammonium heptamolybdate(VI)tetrahydrate [(NH 4)6Mo 7O 24·4H 2O;GR for analysis Merck],molybdenum(VI)oxide (MoO 3;99.5%Fluka),telluric acid [Te(OH)6;97.5–102.5%Aldrich],vanadium(IV)
oxide sulfate pentahydrate (VOSO 4·5H 2O;95.0%Riedel-de Ha n),
ammonium (meta)vanadate(V)(NH 4VO 3;99.0%Fluka),vanadium(V)
oxide (V 2O 5;99.5%Riedel-de Ha n),ammonium niobate(V)oxalate hydrate (NH 4[NbO(C 2O 4)2]·x H 2O;99.99%H.C.Starck;20.17%of Nb),oxalic acid dihydrate (C 2H 2O 4·2H 2O;99.5%Roth),and
hydro-
Figure 10.Conversion of propane in the selective oxidation of propane at 653K over the three morphologically
different M1catalysts (~HT-M1;&SH-M1;*W-M1)as a function of the contact time W /F (W is active mass,F is
flow rate of educt gas).A)normalized to the mass of catalyst (W =m cat ),B)the surface area of the (001)plane (W =A (001)),and C)the specific surface area (W =A BET );feed composition:C 3H 8/O 2/H 2O/N 2=3:6:40:51vol %,P =0.1013M Pa.
A.Trunschke et al.
gen peroxide(H2O2;30%solution;GR for analysis Merck)were used as received.Ultrapure water was obtained by using the Milli-Q Synthesis System(MQ).
Synthesis
Method1:Phase-pure M1was synthesized by using a hydrother-mal-based route adapted from that of Celaya Sanfiz et al.[35]The synthesis proceeded by charging(NH4)6Mo7O24·4H2O(9.51mmol, 11.753g),VOSO4·5H2O(15.52mmol,3.928g),Te(OH)6(6.33mmol, 1.454g)and NH4[NbO(C2O4)2]·x H2O(4.56mmol, 2.101g)into a 400mL autoclave reactor made by Hastelloy C22(Premex AG)and backfilled with260mL of MQ water.The autoclave was sealed,and the precursor system with a nominal molar ratio Mo/V/Te/Nb of 1:0.233:0.095:0.069was subsequently homogenized in situ by stir-ring at343K for30min.Then,the autoclave was heated to423K at1.6K minà1,and kept at this temperature for48h under a con-stant stirring speed of300rpm and an autogenous pressure of ap-proximately0.93M Pa.After controlled cooling to298K at a rate of 1.6K minà1,the product was collected by centrifugation,washed with MQ water,and dried at368K.Finally,the resulting powder was annealed at873K(heating rate:15K minà1)for2h in an Ar flow by using a rotary tube furnace(Xerion).The catalyst yield was 47%(%6g;entry HT-M1,internal catalyst ID5511).
Method2:Single-phase M1was prepared by using a modified pro-cedure adapted from Ushikubo et al.,[7]which was followed by a washing route described by Baca et al.[31]First,(NH4)6Mo7O24·4H2O (92.86mmol,114.762g)was dissolved in MQ water(1.5L)at353K. Then,NH4VO3(195.00mmol,22.811g)was added and dissolved. Next,Te(OH)6(149.50mmol,34.33g)was added to the Mo/V-con-taining solution at313K.In parallel,the Nb-containing solution was prepared by dissolution of NH4[NbO(C2O4)2]·x H2O(81.25mmol, 37.441g)in MQ water(0.5L)at313K.This solution was then added to the Mo/V/Te-containing solution,and the solution volume was adjusted to2.5L by using MQ water.After stirring for 30min at313K,the resultant slurry with a nominal molar ratio Mo/V/Te/Nb of1:0.3:0.23:0.125was spray-dried by using a Büchi B-191mini spray-dryer.The as-prepared powder was calcined at 548K for1h in air flow and then annealed at873K in an Ar flow by using the rotary tube furnace for2h.Thus,a biphasic crystalline M1and M2MoVTeNb oxide was generated.Next,M1was isolated from M2through treatment of the mixed-phase powder by using a15%H2O2solution at room temperature using while stirring con-tinuously(400rpm)for24h.The solid-to-liquid ratio used was 0.04g mLà1.The washed product was collected by using vacuum filtration using a glass filter(porosity5),washed with MQ water, and dried at368K.Finally,the as-derived samples were heat-acti-vated at873K in an Ar flow for2h by using the rotary tube fur-nace.The catalyst yield was41%(%54g;entry W-M1,internal cat-alyst ID6059).
Method3:The title catalyst was also synthesized by using a novel route employing a superheated water vapor treatment.For this purpose,MoO3(80mmol,11.515g)and C2H2O4·2H2O(100mmol, 12.607g)were dissolved in MQ water(200mL)at353K.Then, Te(OH)6(12mmol,2.756g)was dissolved in the Mo-containing so-lution at333K.In parallel,a V-containing solution was prepared by dissolution of V2O5(9mmol,1.637g)together with C2H2O4·2H2O (27mmol,3.404g)in MQ water(100mL)at333K.This solution was added to the Mo/Te-containing solution,and then NH4[NbO-(C2O4)2]·x H2O(12.28mmol,5.659g)was added and dissolved at 313K to form a homogeneous oxalic complex solution with a nominal molar ratio Mo/V/Te/Nb of1:0.225:0.15:0.1535.Then,the solution was spray-dried by using a Büchi B-191mini spray-dryer.A light brown sticky product was quickly collected,and,owing to its instability,it was immediately calcined at548K in flowing air for 1h by using the rotary tube furnace.The as-derived precursor was then treated in superheated water vapor.The precursor powder (3g)and MQ water(2.7g)were repeatedly charged into a48mL stainless steel vessel,the vessel was capped,and placed inside a stainless steel bomb.The bomb was sealed and kept at773K under an in situ generated pressure of approximately20M Pa for 2h.The spatula-collected product of the superheated water vapor treatment was dried at368K and finally annealed at873K in an Ar flow for2h by using the rotary tube furnace.The catalyst yield was57%(%9g;entry SH-M1,internal catalyst ID5737). Characterization
The products were characterized by using powder X-ray diffraction (XRD)using a Bruker D8ADVANCED diffractometer and a STOE STADI-P transmission diffractometer,both equipped with Cu K a1ra-diation.The unit cell parameters were refined by least-square fit-ting of the diffraction peak positions using the M1structure(ortho-rhombic,space group Pba2[32];[ICSD55097])utilizing the Bruker program package TOPAS.The catalysts’degree of crystallinity was evaluated by applying Rietveld refinement with rutile TiO2SRM 674b purchased from NIST,USA,as an internal standard.[40]The morphology was studied by employing scanning electron micros-copy(SEM)using a Hitachi S-4800microscope operated at2kV. The relative Mo,V,Te,and Nb contents were determined by means of energy-dispersive X-ray spectroscopy(EDX)using an EDAX Gen-esis spectrometer attached to the microscope operated at15kV. The Mo,V,Te,and Nb contents were also measured by using in-ductively coupled plasma–optical emission spectrometry(ICP–OES) using a Varian Vista RL spectrometer;the O contents were estab-lished applying the carrier-gas hot extraction method(CGHE)using a LECO TC-436DR/5analyzer.All values were the average of at least three replications.Transmission electron microscopy(TEM), electron diffraction(ED),high-resolution TEM(HRTEM),energy-fil-tered TEM(EFTEM),and scanning TEM(STEM)investigations were performed by using Philips CM200-FEG and CM200-LaB6TEM/ STEM electron microscopes,operating at200kV and equipped with EDAX Genesis spectrometers.The samples were crushed,dis-persed in ethanol,and deposited on a holey carbon TEM grid.N2 physisorption was performed at liquid nitrogen temperatures on a Quantachrome Autosorb-6B analyzer.Prior to measurements,the samples were outgassed under vacuum at423K for2h.The spe-cific surface area(A BET)was calculated according to the multipoint Brunauer–Emmett–Teller method(BET)in the P/P0=0.05–0.3pres-sure range.The total pore volumes were estimated by using the amount of physisorbed nitrogen at a relative pressure of P/P0= 0.95.
Catalysis
The catalytic activities of HT-M1,W-M1and SH-M1were tested in the formation of acrylic acid from propane by using a ten-fold par-allel reactor set-up.For this purpose,the powdered catalyst(1g) was first pressed binder-free under approximately185M Pa, crushed,and sieved to a particle size of250–355m m.Then,125mg or30mg of each catalyst was diluted with silicon carbide(%1g, 250–355m m in size)and loaded into a tubular hastelloy reactors with an inner diameter of4mm.The feed was composed of C3H8, O2,H2O,and N2in a molar ratio of3:6:40:51.The reaction was car-ried out at653K and atmospheric pressure,whereas the gas
MoVTeNb Oxide Catalysts
hourly space velocity ranged(GHSV)between2500and48000hà1. Inlet and outlet gases were analyzed by performing on-line gas chromatography using an Agilent Technologies7890A GC system.
A Plot molesieve column and a PlotQ column coupled with a ther-mal conductivity detector were used for the analysis of O2,N2,CO, and CO2.Hydrocarbons and oxygenated products were monitored by using an HP-FFAP capillary column and a PlotQ column coupled with a flame ionization detector.The conversion and selectivity were calculated based on the total number of carbon atoms and the products found.
Acknowledgements
The authors thank E.Kitzelmann for her assistance with the XRD data collection,G.Lorenz and D.Brennecke for their help with the N2physisorption measurements,G.Weinberg for her help with the SEM analysis,Dr.G.Auffermann(MPI for Chemical Phys-ics of Solids,Dresden,Germany)for chemical analysis. Keywords:mixed metal oxides·structure sensitivity·propane·selective oxidation·structure–activity relationships
[1]E.M.Thorsteinson,T.P.Wilson,F.G.Young,P.H.Kasai,J.Catal.1978,52,
116–132.
[2]G.Centi,F.Cavani,F.Trifiro,in Selective Oxidation by Heterogeneous Cat-
alysis,Kluwer Academic,New York,Boston,Dordrecht,London, Moscow,2001,pp.363–495.
[3]M.M.Lin,Appl.Catal.A:Gen.2001,207,1–16.
[4]D.Vitry,J.L.Dubois,W.Ueda,J.Mol.Catal.A:Chem.2004,220,67–76.
[5]F.Cavani,J.H.Teles,ChemSusChem2009,2,508–534.
[6]P.Botella,E.Garcia-Gonzalez,A.Dejoz,J.M.Lopez Nieto,M.I.Vazquez,
J.Gonzalez-Calbet,J.Catal.2004,225,428–438.
[7]https://www.360docs.net/doc/0516407420.html,hikubo,K.Oshima,A.Kayou,M.Hatano,in Studies in Surface Sci-
ence and Catalysis,Vol.112,Elsevier,1997,pp.473–480.
[8]https://www.360docs.net/doc/0516407420.html,let,H.Roussel,A.Pigamo,J.L.Dubois,J.C.Jumas,Appl.
Catal.A:Gen.2002,232,77–92.
[9]H.Tsuji,Y.Koyasu,J.Am.Chem.Soc.2002,124,5608–5609.
[10]P.DeSanto,D.J.Buttrey,R.K.Grasselli,C.G.Lugmair,A.F.Volpe,B.H.
Toby,T.Vogt,Top.Catal.2003,23,23–38.
[11]P.DeSanto,Jr.,D.J.Buttrey,R.K.Grasselli,C.G.Lugmair,A.F.Volpe,Jr.,
B.H.Toby,T.Vogt,Z.Kristallogr.2004,219,152–165.
[12]H.Murayama,D.Vitry,W.Ueda,G.Fuchs,M.Anne,J.L.Dubois,Appl.
Catal.A:Gen.2007,318,137–142.
[13]W.D.Pyrz,D.A.Blom,N.R.Shiju,V.V.Guliants,T.Vogt,D.J.Buttrey,J.
Phys.Chem.C2008,112,10043–10049.
[14]M.Baca,A.Pigamo,J.L.Dubois,https://www.360docs.net/doc/0516407420.html,let,Top.Catal.2003,23,39–
46.
[15]J.M.Oliver,J.M.Lopez Nieto,P.Botella,A.Mifsud,Appl.Catal.A:Gen.
2004,257,67–76.
[16]W.Ueda,D.Vitry,T.Katou,Catal.Today2004,96,235–240.[17]P.Korovchenko,N.R.Shiju,A.K.Dozier,U.M.Graham,M.O.Guerrero-
Perez,V.V.Guliants,Top.Catal.2008,50,43–51.
[18]F.Ivars,B.Solsona,E.Rodríguez-Castellón,J.M.López Nieto,J.Catal.
2009,262,35–43.
[19]J.Holmberg,R.K.Grasselli,A.Andersson,Appl.Catal.A:Gen.2004,270,
121–134.
[20]K.Oshihara,T.Hisano,W.Ueda,Top.Catal.2001,15,153–160.
[21]R.K.Grasselli,D.J.Buttrey,P.DeSanto,J.D.Burrington,C.G.Lugmair,
A.F.Volpe,Jr.,T.Weingand,Catal.Today2004,91–92,251–258.
[22]V.V.Guliants,R.Bhandari,J.N.Al-Saeedi,V.K.Vasudevan,R.S.Soman,
O.Guerrero-Perez,M.A.Banares,Appl.Catal.A:Gen.2004,274,123–132.
[23]P.DeSanto,D.Buttrey,R.Grasselli,W.Pyrz,C.Lugmair,A.Volpe,T.Vogt,
B.Toby,Top.Catal.2006,38,31–40.
[24]J.B.Wagner,O.Timpe,F.A.Hamid,A.Trunschke,U.Wild,D.S.Su,R.K.
Widi,S.B.A.Hamid,R.Schl?gl,Top.Catal.2006,38,51–58.
[25]A.Celaya Sanfiz,T.W.Hansen,A.Sakthivel,A.Trunschke,R.Schl?gl,A.
Knoester,H.H.Brongersma,M.H.Looi,S.B.A.Hamid,J.Catal.2008, 258,35–43.
[26]A.Celaya Sanfiz,T.W.Hansen,D.Teschner,P.Schno¨rch,F.Girgsdies,A.
Trunschke,R.Schlo¨gl,M.H.Looi,S.B.A.Hamid,J.Phys.Chem.C2010, 114,1912–1921.
[27]M.M.Lin,Appl.Catal.A:Gen.2003,250,305–318.
[28]P.Botella,B.Solsona,A.Martinez-Arias,J.M.L.Nieto,Catal.Lett.2001,
74,149–154.
[29]P.Botella,J.M.Lopez Nieto,B.Solsona,A.Mifsud,F.Marquez,J.Catal.
2002,209,445–455.
[30]D.Vitry,Y.Morikawa,J.L.Dubois,W.Ueda,Appl.Catal.A:Gen.2003,
251,411–424.
[31]M.Baca,https://www.360docs.net/doc/0516407420.html,let,Appl.Catal.A:Gen.2005,279,67–77.
[32]F.Ivars,P.Botella, A.Dejoz,J.M.López Nieto,P.Concepción,M.I.
Vμzquez,Top.Catal.2006,38,59–67.
[33]W.Ueda,D.Vitry,T.Kato,N.Watanabe,Y.Endo,Res.Chem.Intermed.
2006,32,217–233.
[34]N.R.Shiju,V.V.Guliants,ChemPhysChem2007,8,1615–1617.
[35]A.Celaya Sanfiz,T.W.Hansen,F.Girgsdies,O.Timpe,E.R?del,T.Ressler,
A.Trunschke,R.Schl?gl,Top.Catal.2008,50,19–32.
[36]P.Beato,A.Blume,F.Girgsdies,R.E.Jentoft,R.Schl?gl,O.Timpe,A.
Trunschke,G.Weinberg,Q.Basher,F.A.Hamid,S.B.A.Hamid,E.Omar, L.Mohd Salim,Appl.Catal.A:Gen.2006,307,137–147.
[37]G.Y.Popova,T.V.Andrushkevich,L.S.Dovlitova,G.A.Aleshina,Y.A.
Chesalov,A.V.Ishenko,E.V.Ishenko,L.M.Plyasova,V.V.Malakhov,M.I.
Khramov,Appl.Catal.A:Gen.2009,353,249–257.
[38]D.Vitry,Y.Morikawa,J.L.Dubois,W.Ueda,Appl.Catal.A:Gen.2003,
251,411–424.
[39]K.Oshihara,Y.Nakamura,M.Sakuma,W.Ueda,Catal.Today2001,71,
153–159.
[40]D.L.Kaiser,R.L.Watters,Jr.,National Institute of Standards and Technol-
ogy,U.S.Department of Commerce,Gaithersburg,2007.
[41]B.L.Cushing,V.L.Kolesnichenko,C.J.O’Connor,Chem.Rev.2004,104,
3893–3946.
[42]A.A.Galkin,V.V.Lunin,Usp.Khim.2005,74,24–40.
Received:March11,2011
Published online on August29,2011
A.Trunschke et al.