The Discovery and Study of Nanocrystalline TiO2-(MoO3)
我们身边的纳米英语作文

我们身边的纳米英语作文Nanotechnology: Shaping the World Around Us.Nanotechnology, the manipulation of matter at theatomic and molecular scale, has emerged as a transformative force in various aspects of our lives. From healthcare and electronics to construction and energy, nanotechnology is revolutionizing industries and opening up new possibilities.Medical Advancements.One of the most significant applications of nanotechnology is in the medical field. Nanoparticles,which are particles with dimensions on the nanoscale, have unique properties that make them ideal for targeted drug delivery, disease diagnosis, and tissue engineering.Targeted Drug Delivery: Nanoparticles can beengineered to selectively deliver therapeutic drugs to specific cells or tissues, increasing drug efficacy whileminimizing side effects. This targeted approach has shown great promise in treating cancer, heart disease, and other life-threatening illnesses.Disease Diagnosis: Nanosensors, equipped with highly sensitive detectors, can swiftly and accurately diagnose diseases by detecting minute changes in biological markers. Early detection is crucial for timely intervention and improved patient outcomes.Tissue Engineering: Nanotechnology plays a vital role in tissue regeneration and repair. Biocompatible nanomaterials can act as scaffolds for growing new cells, facilitating the creation of tissues and organs for transplantation. This holds immense potential for addressing organ shortages and improving the quality oflife for patients.Electronic Revolution.Nanotechnology has also revolutionized the electronics industry. By manipulating materials at the nanoscale,engineers can create devices with enhanced performance, reduced energy consumption, and increased durability.Transistors and Memory Storage: Nano-sized transistors and memory storage devices enable faster processing speeds, lower power consumption, and increased storage capacity in electronic devices. This miniaturization trend is drivingthe development of smaller, more powerful computers, smartphones, and other gadgets.Flexible Electronics: Flexible nanomaterials make it possible to create bendable and foldable electronic devices, opening up new possibilities for wearable technology, such as smartwatches, fitness trackers, and flexible displays.Quantum Computing: Nanotechnology is essential for the advancement of quantum computing, which promises to revolutionize computing power and enable unprecedentedlevels of scientific discovery and technological innovation.Construction and Materials.Nanotechnology is also transforming the construction and materials industries. Nano-enabled materials and techniques offer enhanced strength, durability, and energy efficiency in buildings and infrastructure.High-Performance Construction Materials: Nanotechnology can strengthen construction materials like concrete and steel, making them lighter, stronger, and more resistant to wear and tear. This leads to longer-lasting, more earthquake-resistant structures.Smart Buildings: Nanosensors and nanotechnology-enhanced materials enable the creation of smart buildings that can monitor environmental conditions, optimize energy consumption, and adjust to occupants' needs. Thesebuildings contribute to energy savings, improved comfort, and overall well-being.Self-Cleaning Surfaces: Nanocoatings can impart self-cleaning properties to surfaces, reducing the need for frequent cleaning and maintenance. This is particularly beneficial in healthcare settings, food processingfacilities, and other hygiene-sensitive areas.Energy and Environment.Nanotechnology has significant implications for energy production and environmental protection. By harnessing the unique properties of nanomaterials, scientists are developing innovative solutions to address global energy challenges.Solar Energy: Nano-engineered solar cells can convert sunlight into electricity more efficiently, making solar energy more viable and accessible.Energy Storage: Nanotechnology enables the development of compact, high-capacity energy storage devices that support the integration of renewable energy sources into the power grid.Environmental Remediation: Nanoparticles can be used to capture and remove pollutants from air, water, and soil, contributing to a cleaner environment.Challenges and Considerations.While nanotechnology offers immense potential, it also comes with challenges and ethical considerations. It is essential to address these concerns responsibly to ensure the safe and ethical development and application of nanotechnology.Environmental and Health Impacts: The potential environmental and health impacts of nanomaterials need to be thoroughly assessed and managed to prevent any unintended consequences.Ethical Considerations: The widespread use of nanotechnology raises ethical questions regarding privacy, equity, and the potential misuse of technology.Regulatory Frameworks: Establishing appropriate regulatory frameworks is crucial to ensure the responsible development and use of nanotechnology while fostering innovation.Conclusion.Nanotechnology is a rapidly evolving field that is shaping the world around us in countless ways. Its applications span a wide range of industries, from medicine and electronics to construction and energy. By harnessing the power of the nanoscale, we can create innovative solutions that address global challenges, improve our quality of life, and pave the way for a brighter future. However, it is imperative to approach nanotechnology with responsibility and foresight, considering both itspotential benefits and risks. By fostering collaboration between scientists, policymakers, and ethicists, we can ensure that nanotechnology is used for the betterment of society and the preservation of our planet.。
纳米技术的作文450字
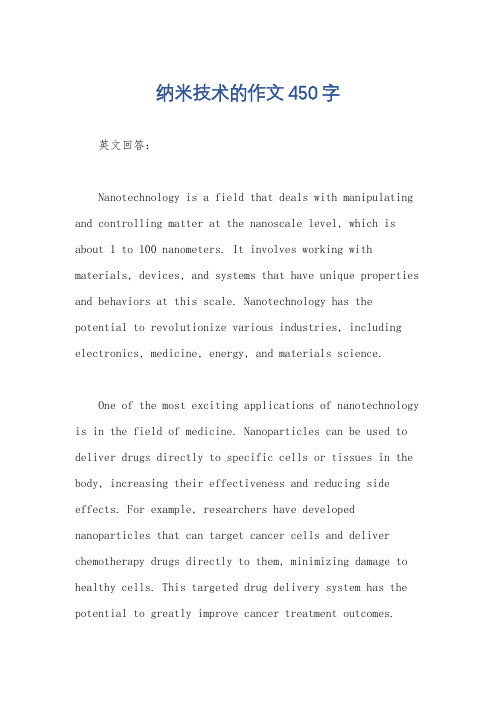
纳米技术的作文450字英文回答:Nanotechnology is a field that deals with manipulating and controlling matter at the nanoscale level, which is about 1 to 100 nanometers. It involves working with materials, devices, and systems that have unique properties and behaviors at this scale. Nanotechnology has the potential to revolutionize various industries, including electronics, medicine, energy, and materials science.One of the most exciting applications of nanotechnology is in the field of medicine. Nanoparticles can be used to deliver drugs directly to specific cells or tissues in the body, increasing their effectiveness and reducing side effects. For example, researchers have developed nanoparticles that can target cancer cells and deliver chemotherapy drugs directly to them, minimizing damage to healthy cells. This targeted drug delivery system has the potential to greatly improve cancer treatment outcomes.Another area where nanotechnology is making significant advancements is in electronics. Nanoscale materials and devices have unique properties that can be harnessed to create faster, smaller, and more efficient electronic devices. For instance, nanoscale transistors can be used to create faster and more powerful computer processors. Nanotechnology also plays a crucial role in the development of flexible and wearable electronics, such as smartwatches and fitness trackers.Furthermore, nanotechnology has the potential to revolutionize the energy sector. Nanomaterials can be used to improve the efficiency of solar cells, making them more cost-effective and viable as a renewable energy source. Additionally, nanotechnology can be used to develop more efficient batteries for energy storage, allowing for the widespread adoption of renewable energy technologies.In the field of materials science, nanotechnology has opened up new possibilities for creating stronger, lighter, and more durable materials. For example, carbon nanotubesare extremely strong and have a high thermal and electrical conductivity, making them ideal for applications in aerospace and automotive industries. Nanocomposites, which are materials made by combining nanoparticles with a matrix material, have also shown promise in improving the mechanical properties of materials.Overall, nanotechnology has the potential to revolutionize various industries and improve our quality of life. Its applications in medicine, electronics, energy,and materials science are just a few examples of the vast potential of this field. As researchers continue to explore and develop new nanoscale materials and devices, we can expect even more exciting advancements in the future.中文回答:纳米技术是一门涉及在纳米级别(约1到100纳米)上操控和控制物质的领域。
纳米技术的相关资料作文

纳米技术的相关资料作文英文回答:Nanotechnology is a field of science and technologythat deals with the manipulation of matter on an atomic and molecular scale. It involves the design, characterization, production, and application of structures, devices, and systems by controlling shape and size at the nanometer scale.Nanotechnology has a wide range of potential applications, including in medicine, electronics, energy production, and environmental protection. For example, in medicine, nanotechnology is being used to develop targeted drug delivery systems and more effective diagnostic tools. In electronics, it is enabling the creation of smaller, more powerful devices. And in energy production, nanotechnology is being used to improve the efficiency of solar panels and energy storage systems.Overall, nanotechnology has the potential to revolutionize many aspects of our lives, but it also raises ethical and societal concerns that need to be addressed asit continues to develop and advance.中文回答:纳米技术是一门处理原子和分子尺度上物质的科学和技术领域。
跟纳米东西有关的作文素材

跟纳米东西有关的作文素材英文回答:Nanotechnology is a rapidly advancing field that involves manipulating matter at the atomic and molecular level. It has the potential to revolutionize various industries, including electronics, medicine, energy, and materials science. There are several interesting aspects related to nanotechnology that can be used as writing material.One fascinating application of nanotechnology is in the field of medicine. Nanoparticles can be used to deliver drugs directly to targeted cells, increasing their effectiveness and reducing side effects. For example, researchers are developing nanocarriers that can transport chemotherapy drugs directly to cancer cells, minimizing damage to healthy cells. This targeted drug delivery system has the potential to greatly improve the treatment of various diseases.Another interesting area of nanotechnology is in the development of nanosensors. These tiny devices can detect and measure substances at the molecular level, enabling a wide range of applications. For instance, nanosensors can be used to detect pollutants in the environment, monitor glucose levels in diabetics, or even detect explosives in security screening. The ability to detect and measure substances at such a small scale opens up new possibilities for improving our daily lives.Furthermore, nanotechnology has the potential to revolutionize the energy sector. Researchers are exploring ways to use nanomaterials to improve the efficiency of solar panels, batteries, and fuel cells. By manipulating the properties of materials at the nanoscale, scientists can enhance their performance and make renewable energy sources more viable. This could lead to a significant reduction in our dependence on fossil fuels and a greener future.中文回答:纳米技术是一个快速发展的领域,涉及到在原子和分子水平上操纵物质。
纳米技术的发现作文300字

纳米技术的发现作文300字English Response:Discovery of Nanotechnology.Nanotechnology, a groundbreaking field of science, has revolutionized various industries with its microscopic wonders. The discovery of nanotechnology marks a pivotal moment in scientific history, opening doors to unimaginable possibilities.Initially, my fascination with nanotechnology stemmed from its potential in medicine. Nanoparticles, smaller than cells, possess unique properties that can be harnessed for targeted drug delivery. For instance, researchers have developed nanocarriers capable of transporting chemotherapy drugs directly to cancer cells while sparing healthy tissues. This precision medicine approach minimizes side effects and enhances treatment efficacy, offering hope to millions battling cancer worldwide.Moreover, the application of nanotechnology extends beyond medicine into electronics. Quantum dots, nanoscale semiconductor particles, have revolutionized display technology by producing vibrant and energy-efficient screens. These quantum dot displays boast superior color accuracy and brightness compared to traditional LCDs, enhancing the viewing experience for consumers worldwide.In addition to its practical applications, nanotechnology has sparked curiosity in fundamental research. The manipulation of materials at the nanoscale has led to the discovery of novel properties and phenomena. For instance, carbon nanotubes exhibit remarkable strength and conductivity, paving the way for advancements in materials science and engineering.The discovery of nanotechnology embodies human ingenuity and the relentless pursuit of knowledge. As we delve deeper into the nanoscale world, we uncover endless possibilities waiting to be explored and harnessed for the betterment of society.中文回答:纳米技术的发现。
科学发现作文

科学发现作文英文回答:Scientific discovery is a complex and multifaceted process that has been essential to the development of human knowledge and understanding. It involves the systematic observation, experimentation, and analysis of data to uncover new insights and principles about the natural world. The pursuit of scientific discovery has led to numerous breakthroughs that have transformed our understanding ofthe universe and have had a profound impact on daily life.One of the most significant aspects of scientific discovery is its objectivity and reliance on empirical evidence. Scientists strive to eliminate bias andsubjectivity by basing their conclusions on verifiable data obtained through observation and experimentation. This rigorous approach ensures that scientific discoveries are not merely opinions or beliefs but are instead rooted in evidence and supported by logical reasoning.The process of scientific discovery often begins with observation and the identification of patterns or anomalies in the natural world. These observations may lead to the formulation of hypotheses, which are tentative explanations that can be tested through experimentation. Experiments are designed to isolate variables and control conditions to determine the causal relationships between different factors. The results of experiments are carefully analyzed to either support or refute the hypothesis and contribute to the accumulation of scientific knowledge.Throughout the process of discovery, scientists engage in critical thinking and logical reasoning to evaluate evidence and draw conclusions. They consider multiple perspectives, question assumptions, and seek to replicate and verify findings to ensure their validity. Scientific discoveries are not static but are constantly refined and revised as new evidence emerges. This iterative process of inquiry and refinement is essential for the advancement of scientific knowledge.Scientific discoveries have had a transformative impact on human society, leading to technological advancements, medical breakthroughs, and a deeper understanding of our place in the universe. Examples of groundbreakingscientific discoveries include the development of the telescope, the discovery of DNA, and the understanding of the laws of thermodynamics. These discoveries have revolutionized our ability to observe and explore the cosmos, unravel the mysteries of life, and harness energy for practical applications.In addition to its practical benefits, scientific discovery also contributes to our intellectual and cultural well-being. It fosters curiosity, critical thinking, and a sense of wonder at the complexities of the natural world. Scientific discoveries inspire creativity, innovation, and a lifelong pursuit of knowledge. By expanding our understanding of the universe, science empowers us to make informed decisions, address global challenges, and shape a more enlightened and sustainable future.中文回答:科学发现。
纳米技术的发现英语作文300字
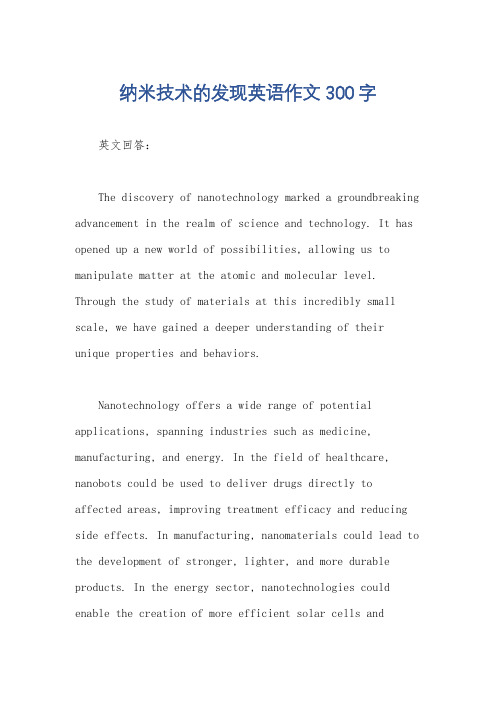
纳米技术的发现英语作文300字英文回答:The discovery of nanotechnology marked a groundbreaking advancement in the realm of science and technology. It has opened up a new world of possibilities, allowing us to manipulate matter at the atomic and molecular level. Through the study of materials at this incredibly small scale, we have gained a deeper understanding of their unique properties and behaviors.Nanotechnology offers a wide range of potential applications, spanning industries such as medicine, manufacturing, and energy. In the field of healthcare, nanobots could be used to deliver drugs directly to affected areas, improving treatment efficacy and reducing side effects. In manufacturing, nanomaterials could lead to the development of stronger, lighter, and more durable products. In the energy sector, nanotechnologies could enable the creation of more efficient solar cells andbatteries.However, it is important to acknowledge that nanotechnology also poses potential risks. The manipulation of matter at the nanoscale raises concerns about its safety and environmental impact. As this field continues to evolve, it is crucial that we carefully consider the ethical and societal implications of nanotechnology and take steps to mitigate any potential risks.中文回答:纳米技术的发现标志着科学技术领域的突破性进展。
纳米技术的了解30字作文

纳米技术的了解30字作文英文回答:Nanotechnology is the study and application of manipulating matter at the atomic and molecular level. It involves working with materials and devices that have unique properties and functions due to their small size. Nanotechnology has the potential to revolutionize various fields, including medicine, electronics, and energy.One example of nanotechnology is the development of nanomedicine. Scientists are using nanoparticles to deliver drugs directly to specific cells in the body, making treatments more targeted and effective. This canpotentially lead to better outcomes for patients with diseases like cancer.Another example is the use of nanomaterials in electronics. Nanowires, for instance, can be used to create smaller and more efficient electronic devices. This couldlead to the development of faster computers and smaller, more powerful smartphones.Nanotechnology also has applications in the energy sector. For example, researchers are exploring the use of nanomaterials to improve the efficiency of solar panels. By using nanoscale materials, it is possible to capture and convert sunlight more effectively, leading to increased energy production.中文回答:纳米技术是研究和应用在原子和分子水平上操纵物质的科学。
- 1、下载文档前请自行甄别文档内容的完整性,平台不提供额外的编辑、内容补充、找答案等附加服务。
- 2、"仅部分预览"的文档,不可在线预览部分如存在完整性等问题,可反馈申请退款(可完整预览的文档不适用该条件!)。
- 3、如文档侵犯您的权益,请联系客服反馈,我们会尽快为您处理(人工客服工作时间:9:00-18:30)。
The Discovery and Study of Nanocrystalline TiO2-(MoO3)Core-Shell MaterialsS.H.Elder,*,‡F.M.Cot,‡Y.Su,‡S.M.Heald,‡A.M.Tyryshkin,‡,†,§M.K.Bowman,‡Y.Gao,‡A.G.Joly,‡M.L.Balmer,‡Ana C.Kolwaite,‡K.A.Magrini,|and D.M.Blake| Contribution from The William R.Wiley En V ironmental Molecular Sciences Laboratory,Pacific Northwest National Laboratory,Richland,Washington99352,and the National Renewable Energy Laboratory, Golden,Colorado80401-3393Recei V ed August3,1999.Re V ised Manuscript Recei V ed January14,2000Abstract:Here we report the synthesis of a series of new nanocrystalline TiO2-(MoO3)core-shell materials whose photoabsorption energy(PE,the energy required to excite TiO2-core valence band electrons to MoO3-shell conduction band states)properties are correlated with both the nanoparticle size and the degree of chemical interaction between the TiO2core and the MoO3shell.The TiO2-(MoO3)nanoparticle size can be readily adjusted from80to40Å,and in this series,the PE decreases from2.88to2.60eV with decreasing particle size.The systematic PE red-shift exhibited by the core-shell materials is ascribed to the change in the relative position of the MoO3-shell conduction band as it evolves from less than a monolayer to a two monolayer shell.IntroductionIn the past decade,a new field of materials chemistry and physics has emerged that emphasizes the rational synthesis and study of nanocrystalline materials.Much of this work has focused on semiconductor nanoparticles1-65(refs1-39are work on non-oxide materials and refs40-65are TiO2related studies),which display a variety of fundamentally interesting photo-physical properties that are a direct result of their size and dimensionality.Because many semiconductor compounds have potential or demonstrated technological importance in photo-luminescence,solar energy conversion,and photocatalysis,the*Corresponding author(scotth.elder@).‡Pacific Northwest National Laboratory.†Institute of Chemical Kinetics and Combustion,Russian Academy of Science,Novosibrisk,Russia.§Current address:Chemistry Department,Princeton University,Prin-ceton,NJ08544.|National Renewable Energy Laboratory.(1)Brus,L.J.Phys.Chem.1986,90,2555.(2)Murray,C.B.;Norris,D.J.;Bawendi,M.G.J.Am.Chem.Soc. 1993,115,8706.(3)Alivisatos,A.P.Science1996,271,933.(4)Dabbousi,B.O.;Rodriguez-Viejo,J.;Mikulec,F.V.;Heine,J.R.; Mattoussi,H.Manuscript in preparation.(5)Ober,R.;Jensen,K.F.;Bewendi,M.G.J.Phys.Chem.1997,101, 9463.(6)Brus,L.E.Appl.Phys.A1991,53,465.(7)Alivisatos,A.P.J.Phys.Chem.1996,100,13226.(8)Weller,H.Ad V.Mater.1993,5,88.(9)Bawendi,M.G.;Steigerwald,M.L.;Brus,L.E.Annu.Re V.Phys. Chem.1990,41,477.(10)Bawendi,M.G.;Kortan,A.R.;Steigerwald,M.L.;Brus,L.E.J. Chem.Phys.1989,91,7282.(11)Chemsiddine,A.;Weller,H.Ber.Bunsen-Ges.Phys.Chem.1993, 97,636.(12)Micic,O.I.;Sprague,J.R.;Curtis,C.J.;Jones,K.M.;Machol,J. L.;Nozik,A.J.;Giessen,H.;Fluegel,B.;Mohos,G.;Peyghambarian N.J. Phys.Chem.1995,99,7754.(13)Tian,Y.;Newton,T.;Kotov,N.A.;Guldi;D.M.;Fendler,J.J. Chem.Phys.1996,100,8927.(14)Youn,H.C.;Baral,S.;Fendler,J.H.J.Phys.Chem.1988,92, 6320.(15)Kortan,A.R.;Hull,R.;Opila,R.L.;Bawendi,M.G.;Steigerwald, M.L.;Carroll,P.J.;Brus,L.E.J.Am.Chem.Soc.1990,112,1327.(16)Mews,A.;Eychmuller,A.;Giersig,M.;Schooss,D.;Weller,H.J. Phys.Chem.1994,98,934.(17)Danek,M.;Jensen,K.F.;Murray,C.B.;Bawendi,M.G.Chem. Mater,1996,8,173.(19)Wilson,W.L.;Szajowski,P.J.;Brus,L.E.Science1993,262, 1242.(20)Hines,M.A.;Guyot-Sionnest,P.J.Phys.Chem.1996,100,468.(21)Vossmeyer,T.;Katsikas,L.;Giersig,M.;Popovic,I.G.;Diesner, K.;Chemseddine,A.;Eychmuller,A.;Weller,H.J.Phys.Chem.1994, 98,7665.(22)Steigerwald,M.L.;Alivisatos,A.P.;Gibson,J.M.;Harris,T.D.; Kortan,R.;Muller,A.J.;Thayer,A.M.;Duncan,T.M.;Douglas,D.C.; Brus,L.E.J.Am.Chem.Soc.1988,110,3046.(23)Murray,C.B.Ph.D.Disseration,Massachusetts Institute of Technol-ogy,1995.(24)Brus,L.E.J.Chem.Phys.1983,79,5566.(25)Brus,L.E.J.Chem.Phys.1984,80,4403.(26)Norris,D.J.;Sacra,A.;Murray,C.B.;Bawendi,M.G.Phys.Re V. 1996,53,338.(27)Tagaki,H.;Ogawa,H.;Yamazaki,Y.;Ishizaki,A.;Nakagiri,T. Appl.Phys.Lett.1990,56,2379.(28)Brus,L.E.J.Phys.Chem.1994,98,3575.(29)Olshavsky,M.A.;Godstein,A.N.;Alivisatos,A.P.J.Am.Chem. Soc.1990,112,9438.(30)Ushida,H.;Curtis,C.J.;Nozik,A.J.J.Phys.Chem.1991,95, 5382.(31)Ushida,H.;Curtis,C.J.;Kamat,P.V.;Jones,K.M.;Nozik A.J. J.Phys.Chem.1992,96,1156.(32)Kher,S.S.;Wells,R.L.Chem.Mater.1994,6,2056.(33)Spahnel,L.;Haase,M.;Weller,H.;Henglein,A.J.Am.Chem.Soc. 1987,109,5649.(34)Eychmuller,A.;Hasselbarth,A.;Katsikas,L.;Weller,H.J.Lumin. 1991,48/49,745.(35)Hoener,C.F.;Allan,K.A.;Bard,A.J.;Champion,A.;Fox,M.A.;Mallouk,T.E.;Webber,S.E.;White,J.M.J.Phys.Chem.1992,96, 3812.(36)Eychmuller,A.;Mews,A.;Weller,H.Chem.Phys.Lett.1993,208, 59.(37)Danek,M.;Jensen,K.F.;Murray,C.B.;Bawendi,M.G.Appl. Phys.Lett.1994,65,2795.(38)Bowen Katari,J.E.;Colvin,V.L.;Alivisatos,A.P.J.Phys.Chem. 1994,98,4109.5138J.Am.Chem.Soc.2000,122,5138-5146tailoring of these properties by adjusting crystallite size and nanoarchitecture is an inviting prospect.66There has been extensive research activity focused on the synthesis and photophysical property characterization of nano-crystalline TiO2since the discovery that TiO2could photoelec-trolyze water to produce H2.67The stimulus behind this research is the potential for converting light to electrical energy(pho-tochromics and photovoltaics)or chemical energy(photocata-lytic splitting of water,photooxidation of harmful organics and microorganisms)by solar-driven band gap excitation of TiO2. Additionally,TiO2is inexpensive,nontoxic,and water stable, which makes it amenable for use in a wide range of processes with minimal environmental impact.Unfortunately,TiO2has physical property limitations with regard to its practical solar energy applications,most notably the band gap energy(3.2eV) is well outside the most intense region of the solar spectrum (centered at∼2.6eV).To this end,our research effort in this area is focused on the synthesis of new nanoarchitectured metal oxide materials with photophysical properties that can potentially be tuned for practical solar energy conversion. Experimental SectionPreparation of Core-Shell Materials.The TiO2-(MoO3)x core-shell materials are synthesized by a co-nucleation of metal oxide clusters at the surface of surfactant micelles.68The general reaction stoichiometry for the preparation of the TiO2-(MoO3)x materials is shown in eq1: As an example,for the synthesis of TiO2-(MoO3)0.18,4.8g(y)0.10) of(NH4)2Ti(OH)2(C3H4O3)2(Tyzor LA;DuPont)was combined with 4.9g of cetyltrimethylammonium chloride surfactant(CTAC,29wt %aqueous solution,Lonza).To this solution,30mL of a1.8mM Na4Mo8O26aqueous solution was added with vigorous stirring,which produced a voluminous white precipitate.The reaction was stirred at room temperature overnight,at70°C for24h,and at100°C for48h in a sealed Teflon reactor.The precipitate was isolated by washing and centrifuging several times with water,and the CTAC was removed by calcining in air at450°C for2h.The syntheses of TiO2-(MoO3)0.54 (y)0.25),TiO2-(MoO3)1.1(y)0.50),and TiO2-(MoO3)1.8(y)0.57) were accomplished in an analogous manner,and the chemical composi-tions were determined by elemental analysis.The quantity y(eq1) can be continuously varied between0and0.57,but the four core-shell compositions described above inclusively represent the range of structural and electronic properties displayed by the TiO2-(MoO3)x compounds.It is important to note that if no CTAC was included,or if we substituted the CTAC with NH4Cl,no precipitation reaction occurred at any point in the reaction steps.Furthermore,if no Mo8O264-(aq)was included in the reaction,only a white solid was produced,and the same observation was made if only Mo8O264-(aq) was used in reaction1.Only bulk,microcrystalline TiO2and R-MoO3 can be prepared for y>0.57,which is indicative of macroscopic phase separation.Techniques.X-ray powder diffraction(XRPD)data were collected from5to80°in2θwith0.05°steps/s with a Philips X’PERT-MPD diffractometer using Cu K R radiation.UV-vis diffuse+spectral reflectance(DSR)experiments were conducted using a CARY5G UV-vis-NIR spectrophotometer.The powder samples were smoothly compacted into a2-mm deep depression of a protruding sample holder and this was mounted onto an integrating sphere spectral collector.The data were collected between1000and 200nm at900nm/min with a2nm spectral bandwidth.BaSO4powder was used as a standard for the instrumental background correction.Electron paramagnetic resonance(EPR)measurements were made using a Bruker ESP380E X-band pulsed EPR spectrometer.Conven-tional CW-EPR measurements were made with100kHz field modula-tion and an Oxford Instruments ESR-910cryostat was inserted into a TE102rectangular cavity resonator.First derivative EPR spectra were double-integrated using the2D-WinEPR software package from Bruker to obtain relative numbers of spins.Pulsed EPR measurements of the two-pulse electron spin-echo decays were made using the Bruker pulsed ENDOR dielectric resonator in an Oxford CFG-935cryostat. The decay rates were extracted using the WinDS software package obtained from Dr.Andrei Astashkin and the Institute of Chemical Kinetics and Combustion of the Russian Academy of Science.The samples were loosely loaded into707-SQ quartz EPR tubes from Wilmad.The Mo and Ti K-edge measurements were made at the PNC-CAT insertion device beamline at the APS using Si(111)monochromator crystals.This line uses APS undulator A as a source,and the undulator is scanned to track the monochromator energy.For the Mo edge,powder samples were contained in thin-walled glass capillaries varying in(40)Bedja,I.;Kamat,P.V.J.Phys.Chem.1995,99,9182-9188.(41)Gopidas,K.R.;Bohorquez,M.;Kamat,P.V.J.Phys.Chem.1990, 94,6435-6440.(42)Joselevich,E.;Willner,I.J.Phys.Chem.1994,98,7628-7635.(43)Kormann,C.;Bahnemann,D.W.;Hoffman,M.R.J.Phys.Chem. 1988,92,5196-5201.(44)Choi,W.;Termin,A.;Hoffmann,M.J.Phys.Chem.1994,98, 13669-13679.(45)Duonghong,D.;Ramsden,J.;Gra¨tzel,M.J.Am.Chem.Soc.1982, 104,2977-2985.(46)Moser,J.;Gra¨tzel,M.;Gallay,R.Hel V.Chim.Acta1987,70,1596-1604.(47)Micic,O.I.;Zhang,Y.;Cromack,K.R.;Trifunac,A.D.;Thurnauer, M.C.J.Phys.Chem.1993,97,13284-13288.(48)Chen,L.X.;Rajh,T.;Wang,Z.;Thurnauer,M.C.J.Phys.Chem. B1997,101,10688-10697.(49)Thurnauer,M.C.;Rajh,T.;Tiede,D.M.Acta Chim.Scand.1997, 51,610-618.(50)Rajh,T.;Tiede,D.M.;Thurnauer,M.C.J.Non-Crystalline Solids 1996,205-207,815-820.(51)Rajh,T.;Ostafin,A.E.;Micic,O.L.;Tiede,D.M.;Thurnauer,M.C.J.Phys.Chem.1996,100,4538-4545.(52)Micic,O.L.;Zhang,Y.;Cromack,K.R.;Trifunac,A.D.;Thurnauer, M.C.J.Phys.Chem.1993,97,7277-7283.(53)Burfeindt,B.;Hannappel,T.;Storck,W.;Willig,F.J.Phys.Chem. 1996,100,16463-16465.(54)Vinodgopal,K.;Bedja,I.;Kamat,P.V.Chem.Mater.1996,8, 2180-2187.(55)Burnside,S.D.;Shklover,V.;Barbe´,C.;Comte,P.;Arendse,F.; Brooks,K.;Gra¨tzel,M.Chem.Mater.1998,10,2419-2425.(56)Trentler,T.J.;Denler,T.E.;Bertone,J.F.;Agrawal,A.;Colvin, V.L.J.Am.Chem.Soc.1999,121,1613-1614.(57)Howe,R.F.;Gra¨tzel,M.J.Phys.Chem.1985,89,4495-4499.(58)Borgarello,E.;Kiwi,J.;Gra¨tzel,M.;Pelizzetti,E.;Visca,M.J. Am.Chem.Soc.1982,104,2996-3002.(59)Gra¨tzel,M.;Howe,R.J.Phys.Chem.1990,94,2566-2572.(60)Ko¨lle,U.;Moser,J.;Gra¨tzel,M.Inorg.Chem.1985,24,2253-2258.(61)Cramer,S.P.;Hodgson,K.O.;Gillum,W.O.;Mortenson,L.E.J. Am.Chem.Soc.1977,100,3398-3408.(62)Bach,U.;Lup,D.;Comte,P.;Moser,J.E.;Weisso¨rtel,F.;Salbeck, J.;Spreitzer,H.;Gra¨tzel,M.Nature1998,395,583-585.(63)Shklover,V.;Haibach,T.;Bolliger,B.;Hochstrasser,M.;Erbudak, M.;Nissen,H.U.;Zakeeruddin,S.M.;Nazeeruddin,K.;Gra¨tzel,M.J. Solid State Chem.1997,132,60-72.(64)Shklover,V.;Nazeeruddin,M.K.;Zakeeruddin,S.M.;Barbe`,C.; Kay,A.;Haibach,T.;Steurer,W.;Hermann,R.;Nissen,H.U.;Gra¨tzel, M.Chem.Mater.1997,9,430-439.(65)Gerfin,T.;Gra¨tzel,M.;Walder,L.Prog.Inorg.Chem.1997,4, 345-391and references therein.(66)(a)Brunchez,M.;Marone,M.;Gin,P.;Weiss,S.;Alivisatos,A.P. Science1998,281,2013.(b)Chan,W.C.W.;Nie,S.Science1998,281, 2016.(c)O’Regan,B.;Gratzel,M.Nature1991,353,737.(d)Li,W.,et al.J.Phys.Chem.1998,102,5333.(e)Kavan,L.;Kratochvilova,K.;(1-y)(NH4)2Ti(OH)2(C3H4O3)2(aq)+(y/8)Na4Mo8O26(aq)+CTAC(aq)(1)(y e0.57)Nanocrystalline TiO2-(MoO3)Core-Shell Materials J.Am.Chem.Soc.,Vol.122,No.21,20005139rubbed onto adhesive tape.Typically,4layers of tape gave an absorption step of1-1.5for the Ti edge samples,and8layers sufficed for the Mo standards.Data were collected in both transmission and fluorescence mode,with the fluorescence signal used for the more dilute Mo samples. Helium-filled ion chambers were used for the transmission measure-ments,and an Ar-filled ion chamber for fluorescence.Care was taken to minimize harmonics by detuning the monochromator crystals.In all cases,the vertical beam height(0.1-0.7mm at52m from the source) was small enough that the monochromator resolution was dominated by the intrinsic crystal resolution.Typically,resolution was about0.8 eV at the Ti edge,and3eV at the Mo K-edge.The Mo L3-edge measurements were made at the SRC in Stoughton,WI.The beamline used was the Canadian DCM line that is part of the Canadian Synchrotron Radiation Facility.The double crystal monochromator used InSb(111)monochromator crystals with approximately0.5eV resolution at the Mo L3-edge.Samples were rubbed onto carbon tape,and a single layer was measured in a turbo pumped vacuum chamber at about10-7 Torr.Data were collected using total electron yield and fluorescence detection.In all cases the total yield signal was superior.The fluorescence measurements generally suffered from self-absorption distortions,but were useful in comparing with previous measurements on Mo standards that used fluorescence.The Raman spectra were collected on a Spex Triple Raman Spectrometer(Industries Model1977)with the488nm line of a COHERENT INNOVA306Ar+ion laser for excitation.The power at the sample was on the order of30mW or less.A LN/CCD detector (Princeton Instruments)was used with a typical exposure time of100 s and a slit width of200µm.Raman spectra were obtained at180°scattering geometry on pressed powders.The Raman peak positions were measured based on the reference of the known bands of anatase TiO2with an accuracy within(2cm-1.Spectral analysis was performed using commercial software(Galactic Industries Grams/32).Fluorescence spectra were collected using a SPEX Fluorolog fluorometer equipped with double monochromators for excitation and emission,a450W Xenon lamp,and a cooled photomultiplier tube detector.The samples were mounted on a fused silica plate and emission was collected in a backscattering geometry.Color glass cutoff filters were used in addition to the emission monochromators to help remove unwanted excitation light from the fluorescence spectra.The powders were tested for their photocatalytic oxidation activity against acetaldehyde.The details on the reactor and the experimental design for this testing are given elsewhere.69Briefly,the powders weresuspended in2-propanol(Aldrich Chemicals)and dropped via pipet onto a1.5in.wide by0.125in.thick quartz disk to form a nearly opaque layer.We obtained a fairly uniform coating,and catalyst amounts were generally about30-40mg.A reference disk with about 30mg of Degussa P25TiO2was used for comparison of photoactivity with both light sources.In a typical experiment the coated quartz disk was loaded and sealed into the photoreactor system,and the reactant gases were introduced.For these experiments,we used an initial concentration of50ppmv acetaldehyde in80%N2/20%O2synthetic air.When steady-state acetaldehyde concentration was reached,the catalyst-coated disk was illuminated and the outlet gas automatically sampled as ambient temperature photoreaction proceeded.A Hg lamp (6W black light at366nm)and a Xe lamp(150W at290-500nm) were used as illumination sources.Percent conversion of acetaldehyde (i.e.CH2O+O2f CO2+H2O)was calculated using the equation (C o-C reaction/C o)×100,where C o is the initial steady-state concentra-tion of acetaldehyde(ppmv)and C reaction is the steady state concentration during reaction.Transmission electron microscopy(TEM)data were obtained on a JEOL JEM-2010electron microscope.The samples were prepared by suspending the powders in ethanol and placing a drop of the solution on a holey carbon coated Cu grid.Nitrogen adsorption/desorption measurements were collected with the Quantachrome Autosorb6-B gas sorption system on degassed samples at77K.dissolution process was slow,but once fully dissolved they were diluted with deionized water and analyzed using a Perkin-Elmer Optima3000 D.V.ICP/AES analysis system.Results and DiscussionFor simplicity in the ensuing discussion we first describe how the MoO3monolayer coverage in the shell was calculated for the TiO2-(MoO3)x nomenclature.We calculated the MoO3 monolayer surface coverage by considering the elemental analysis data,the surface area of the powders(Table1),and the crystallographic structure of R-MoO3(Figure1).70The Mo surface density on the(010)plane of R-MoO3is6.8×1018 m-2,and this Mo surface density,combined with the measured surface area,was used to define the MoO3monolayer coverage for the shell.71For example,one MoO3monolayer is one layer of corner sharing MoO6octahedra,and two MoO3monolayers has the structure of one of the slabs oriented perpendicular to the b-axis of R-MoO3(see Figure1).We expected the color of the calcined TiO2-(MoO3)x powders to be white,or possibly very light yellow,because the transition metals are in their fully oxidized state(i.e.d0).In contrast,they displayed a variety of colors ranging from gray-green to green Table1.Elemental Analysis(mol%Mo)and Surface Area Data for the TiO2-(MoO3)x Core-Shell Materials Used To Calculate the Number of MoO3Monolayers(x1)a in the Shelly b mol%Mo surface area(m2/g)x1x2 0.1021250.180.12 0.25102000.540.56 0.5025205 1.1 1.20.5730150 1.8 1.7a The x2data are the calculated number of MoO3monolayers considering only the geometrical surface area of the spherical TiO2 cores(particle size determined from XRPD and TEM data)and a Mo surface packing density of6.8×1018/m2.b y refers to the reaction stoichiometry in eq1.Figure1.(A)A view of the R-MoO3crystal structure emphasizing the slabs comprised of corner(half a slab)and edge(full slab)sharing octahedra oriented perpendicular to the b-axis.(B)Looking down the b-axis of the R-MoO3structure.(C and D)Views of the anatase crystal structure for comparison.5140J.Am.Chem.Soc.,Vol.122,No.21,2000Elder et al.tion peaks that could be indexed on the TiO 2(anatase)unit cell,and based on the peak broadening,72the TiO 2was determined to be nanocrystalline,with the crystallite size decreasing as the MoO 3shell thickness increased.No crystalline molybdenum oxide phase was observed in the XRPD data.Images from high-resolution transmission electron microscopy (HRTEM)studies on these samples exhibited particles with well-defined lattice fringes (Figure 4).The lattice spacing of the crystallites (in Figure 4,both A and B)with non-crossed fringes measured 3.5(0.05Å,which corresponds to the distance between the (101)planes in anatase TiO 2.The TiO 2crystallite sizes measured in the HRTEM images were similar to those calculated from the XRPD data.Finally,there were no crystalline or large (g 10Å)amorphous molybdenum oxide domains evident in HRTEM data.We investigated the origin of the color variation in the core -shell compounds by collecting UV -visible diffuse +spectral reflectance (DSR)data to gain more understanding of how theFigure 2.A photograph of the TiO 2-(MoO 3)x compounds,along with PNNL-1(a material with the nominal composition of Zr 0.25Ti 0.75O 2,and containing 25ÅTiO 2nanocrystallites),bulk TiO 2,and bulk R -MoO 3to illustrate the variation incolor.Figure 3.X-ray powder diffraction data for the series of TiO 2-(MoO 3)x core -shell compounds.The lowest set of stick figure data are that reported for pure anatase TiO 2.The average TiO 2crystallite size was determined using the Scherrer equation and confirmed with HRTEM.The crystallite diameters are as follows:TiO 2-(MoO 3)0.18,80Å;TiO 2-(MoO 3)0.54,60Å;TiO 2-(MoO 3)1.1,50Å;and TiO 2-(MoO 3)1.8,40Å.Figure 4.High-resolution transmission electron micrograph of TiO 2-Nanocrystalline TiO 2-(MoO 3)Core -Shell Materials J.Am.Chem.Soc.,Vol.122,No.21,20005141evolving with composition and structure.The PEs were calculated based on a previously described method.73Briefly,an absorption coefficient (R )was calculated according to eq 2(where R is the reflectance measured in the DSR experiments):A plot of (R h ν)1/2vs h ν(Figure 5)was linear in the range of 2.6-3.3eV,and the intercept from the extrapolation of this linear portion gave the PE.It is immediately evident in Figure 5that the PE (i.e.the region of steep decrease in (R h ν)1/2)increasingly shifts to lower energy (longer wavelength)as the MoO 3monolayer coverage increases,and these energies are red-shifted relative to both bulk TiO 2(E g )3.2eV)and R -MoO 3(E g )2.9eV).Additionally,a plot of PE vs particle size (Figure 6)clearly shows that these energies for the TiO 2-(MoO 3)x compounds become progressively more red-shifted with de-creasing nanoparticle size.As a clarifying note,despite the increase in MoO 3shell thickness when going from TiO 2-(MoO 3)0.18to TiO 2-(MoO 3)1.8,the overall particle size (core +shell)decreases since the TiO 2core size decreases rapidly in this series,but the shell is never more than ∼6Åthick (see Figure 1).Theoretical and experimental work on II -VI and III -V core -shell nanoparticle systems indicate that E g is a function of both size quantization effects and the relative composition of the core -shell particle (i.e.relative thickness of the core and shell).74,75In the limiting case it is logical to expect the PE of a core -shell nanoparticle system to begreater than or equal to the smallest band gap material comprising the core -shell system.In addition to this,a PE blue-shift,relative to the band gap energies of the bulk materials,is expected when the core -shell particle size is in the quantum regime (i.e.,core diameter or shell thickness equal to or smaller than the Bohr radius of the valence/conduction band electron).Indeed,previous work demonstrates these two affects.74,75For these reasons we expected the PE for the TiO 2-(MoO 3)x core -shell materials to be greater than 2.9eV (E g for MoO 3),and likely greater than 3.2eV (E g for TiO 2)due to the dominantFigure 5.Plots of (R h ν)1/2vs h ν;R )-ln(R ),where R is the reflectance from the DSR measurements:(A)TiO 2-(MoO 3)0.18,PE )2.88eV;(B)TiO 2-(MoO 3)0.54,PE )2.79eV;(C)TiO 2-(MoO 3)1.1,PE )2.68eV;and (D)TiO 2-(MoO 3)1.8,PE )2.60eV.The intercept of the two linearly extrapolated lines gives the photoabsorption energy(PE).Figure 6.Photoabsorption energy (PE)as a function of TiO 2-(MoO 3)x core -shell diameter.For comparison,the band gap energy (E g )is 3.2eV for bulk TiO 2(anatase)and 2.9eV for bulk R -MoO 3.R )-ln(R )(2)5142J.Am.Chem.Soc.,Vol.122,No.21,2000Elder et al.of25-30Å.76In contrast,the TiO2-(MoO3)x PEs range from 2.88to2.60eV,approximately equal to or lower in energy than bulk MoO3,which places the PEs of TiO2-(MoO3)1.8in the most intense region of the solar spectrum.The charge-transfer absorption properties exhibited by the TiO2-(MoO3)x compounds appear to be fundamentally different than previously reported for the II-VI and III-V core-shell systems.Conversely,we did not observe the TiO2-(MoO3)x materials to fluoresce when they were photoexcited at energies above their PE edge,as opposed to a sample of pure TiO2that gave a characteristic fluorescence spectrum.77The lack of fluorescence is readily understood considering that the TiO2-(MoO3)x materials exhibit photochromic properties:they become blue/black in color when exposed to light under ambient conditions.We qualitatively studied this photochromism by irradiating each of the powders with monochromatic light,78and it was found that all of the samples turned blue/black when excited with light having420 nm(TiO2-(MoO3)0.18)eλe460nm(TiO2-(MoO3)1.8).Forcomparison,we found that bulk TiO2and MoO3exhibited photochromism,but only when irradiated with ultraviolet light (λ∼300nm).To the best of our knowledge this is the first report of TiO2or MoO3exhibiting visible-light induced pho-tochromism without first being blued by cathodic polarization.79 Considering the relative ease in which reduced molybdenum oxides are formed,generally called Magneli phases,80we collected EPR data on the TiO2-(MoO3)x compounds to deter-mine if paramagnetic molybdenum species played a role in the observed optical properties.Both CW-EPR spectra and profiles of the electron spin-echo intensity as a function of magnetic field were recorded between room temperature and5K for each sample.There was a single dominant EPR signal exhibiting roughly axial symmetry with g|)1.883and g⊥)1.93.The relative ordering of the g-values,g|and g⊥,is typical for Ti(III) in oxides,while the opposite is usually observed for Mo(V)in oxides.In addition,Ti(III)at the surface of an aqueous colloid of TiO2has g|)1.88and g⊥)1.925,making it likely the EPR signal from our samples is due to Ti(III)either at an exposed TiO2surface or at the Ti/Mo interface.57,59Assuming equal packing densities for each sample,the double integrals of the CW-spectra indicated that the number of spins was directly proportional to the Mo content.However,because the packing density of the samples in the EPR tubes was not known very accurately,double integration was not a precise method for determining absolute spin concentrations in the sample.Yet, the possibility that these centers might be responsible for the PE shifts made such data important.We therefore turned to measurements of the electron spin-spin relaxation to set upper limits on the absolute spin concentration.Interaction with nearby paramagnetic centers is one reason for decay of the two-pulse electron spin-echo and adds to the decay rate from other sources.The decay rate caused by nearby spins is well understood and for these samples is expected to be equal to R C‚C loc where R C∼(0.3-0.9)×10-13cm3/s and C loc is the local concentration of paramagnetic species.81The electron spin-echo decay rates were not obvi-ously related to Mo content and varied between0.4×106and 1.25×106s-1with little,if any,temperature dependence below 150K.Taking the fastest decay as an absolute upper bound on local paramagnetic concentration,which occurs in the sample with the highest Mo content(TiO2-(MoO3)1.8),gives a local concentration of paramagnetic centers of4×10-5Å-3.This corresponds to approximately one paramagnetic center per particle.The CW EPR measurements showed that the number of centers is10times less in TiO2-(MoO3)0.18,suggesting a local concentration10times lower in a particle with roughly10times larger volume,again giving an upper limit of approximately one paramagnetic center per particle.The actual local concentra-tion is probably at least an order of magnitude lower than this upper bound.We collected X-ray absorption near edge structure(XANES) data as a means to separately evaluate the Ti-O and Mo-O structural connectivity.The Ti K-edge and preedge data for all four TiO2-(MoO3)x compounds were nearly identical with that of the TiO2(anatase)standard.This supports the XRPD data and confirms that there is very little,if any,Mo in the anatase lattice:the TiO2and molybdenum oxide are in separate phases with an interface.The Mo K-edge XANES data(Figure7) clearly demonstrate that the edge and preedge features shift to lower energy in a regular fashion as the MoO3shell thickness increases,but the overall shape remains quite similar to that of R-MoO3.The shift in energy suggests a significant increase in the covalency of the Mo-O bonding,or O f Mo charge transfer, yet the overall Mo coordination is akin to that of R-MoO3. Mo L3-edge data were collected because they are particularly useful for determining the coordination symmetry(especially tetrahedral vs octahedral)about Mo.82-86For tetrahedrally or octahedrally coordinated Mo,the L3-edge data are characterized by two absorption peaks with an approximately2:3(e below t2)and3:2(t2g below e g)ratio in their intensities,respectively.(76)Elder,S.H.;Gao,Y,;Li,X.;Liu,J.;McCready,D.E.;Windisch,C.F.,Jr.Chem.Mater.1998,10,3140.(77)Poznyak,S.K.;Sviridov,V.V.;Kulak,A.I.;Samstov,M.P.J. Electroanal.Chem.1992,340,73.Smandek,B.;Gerischer,H.Electrochim. Acta1989,34,1411.Nakato,Y.;Tsumura,A.;Tsubomura,H.Chem.Phys. Lett.1982,85,387.(78)The photochromic experiments were conducted in a similar fashion as the fluorescence experiments.Monochromatic light from a450W xenon lamp was impinged on the samples under ambient conditions,and the color(81)Salikhov,K.M.;Tsvetkov,Y.D.In Time Domain Electron Spin Resonance;Kevan,L.,Schwartz,R.N.,Eds.;Wiley-Interscience:New York,1979;p231.(82)Hangchun,H.;Wachs,I.E.;Bare,S.R.J.Phys.Chem.1995,99, 10897-10910.(83)Hirofumi,A.;Tanka,T.;Funabiki,T.;Yoshida,S.;Eda,K.;Sotani, N.;Kudo,M.;Hasegawa,S.J.Phys.Chem.1996,100,19495-19501.Figure7.Mo K-edge XANES data for R-MoO3(0),TiO2-(MoO3)0.18 (4),TiO2-(MoO3)0.54(×),TiO2-(MoO3)1.1(O),TiO2-(MoO3)1.8(]),and MoO2(solid line).Nanocrystalline TiO2-(MoO3)Core-Shell Materials J.Am.Chem.Soc.,Vol.122,No.21,20005143。