压电纤维
压电结构纤维及复合材料完整版

压电结构纤维及复合材料完整版压电材料是指具有压电效应的功能材料,具有压电效应的材料在外加电场、机械应力或温度变化下,能够产生电荷分离和电荷累积,从而实现相应的机电耦合效应。
压电材料的应用非常广泛,包括传感器、驱动器、能量转换和微机电系统等领域。
压电材料主要有陶瓷和聚合物两种。
陶瓷压电材料具有优异的压电性能,但是脆性较大,不易加工成复杂形状,而聚合物压电材料则具有良好的可塑性和可加工性。
在聚合物压电材料中,压电结构纤维及复合材料是非常有潜力的应用材料。
压电结构纤维的制备主要通过改性聚合物纺丝方法来实现。
首先,选择适量的压电材料和聚合物基体进行混合,并通过化学方法将压电材料与聚合物基体进行键合,形成复合材料。
然后,将混合物进行熔融,并通过纺丝设备将熔融物拉伸成细丝状。
最后,经过拉伸、冷却和固化等过程,得到压电结构纤维。
压电结构纤维具有良好的可塑性和可加工性,可以通过编织、织物和编织等方式制备成各种形状的材料。
第一,良好的机电耦合效应。
压电材料在外加电场、机械应力或温度变化下能够产生电荷分离和电荷累积,从而实现相应的机电耦合效应。
压电结构纤维及复合材料能够将这种机电耦合效应发挥到极致,实现更高的敏感度和效率。
第二,良好的柔性和可塑性。
由于采用了聚合物基体和改性聚合物纺丝方法制备,压电结构纤维及复合材料具有良好的柔性和可塑性,可以根据需要制备成各种形状和尺寸的材料,非常适合于电子器件和结构件的应用。
第三,多功能性。
压电结构纤维及复合材料不仅具有压电性能,还可以具有其他功能,比如导电、抗静电、磁性和光学性能。
通过控制材料的组成和制备工艺,可以实现多种功能的组合,从而满足不同应用的需求。
第四,广泛的应用领域。
压电结构纤维及复合材料在传感器、驱动器、能量转换和微机电系统等领域具有广泛的应用潜力。
比如,可以制备出高性能的声发射传感器、振动能量收集器和柔性电子器件等,为人们的生活和工作提供更多便利。
总之,压电结构纤维及复合材料是一种具有良好机电耦合效应、柔性和可塑性的多功能材料,具有广泛的应用前景。
压电纤维发展现状
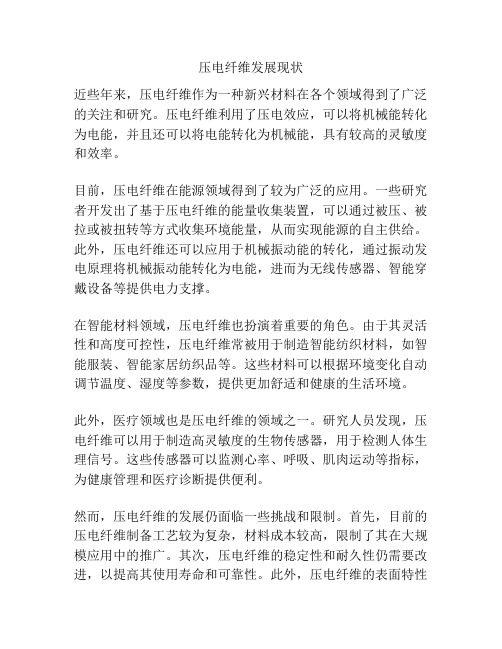
压电纤维发展现状
近些年来,压电纤维作为一种新兴材料在各个领域得到了广泛的关注和研究。
压电纤维利用了压电效应,可以将机械能转化为电能,并且还可以将电能转化为机械能,具有较高的灵敏度和效率。
目前,压电纤维在能源领域得到了较为广泛的应用。
一些研究者开发出了基于压电纤维的能量收集装置,可以通过被压、被拉或被扭转等方式收集环境能量,从而实现能源的自主供给。
此外,压电纤维还可以应用于机械振动能的转化,通过振动发电原理将机械振动能转化为电能,进而为无线传感器、智能穿戴设备等提供电力支撑。
在智能材料领域,压电纤维也扮演着重要的角色。
由于其灵活性和高度可控性,压电纤维常被用于制造智能纺织材料,如智能服装、智能家居纺织品等。
这些材料可以根据环境变化自动调节温度、湿度等参数,提供更加舒适和健康的生活环境。
此外,医疗领域也是压电纤维的领域之一。
研究人员发现,压电纤维可以用于制造高灵敏度的生物传感器,用于检测人体生理信号。
这些传感器可以监测心率、呼吸、肌肉运动等指标,为健康管理和医疗诊断提供便利。
然而,压电纤维的发展仍面临一些挑战和限制。
首先,目前的压电纤维制备工艺较为复杂,材料成本较高,限制了其在大规模应用中的推广。
其次,压电纤维的稳定性和耐久性仍需要改进,以提高其使用寿命和可靠性。
此外,压电纤维的表面特性
和界面相互作用等问题也需要进一步研究和解决。
总的来说,随着科技的不断进步和人们对高性能材料的需求不断增加,压电纤维有着广阔的发展前景。
在未来,压电纤维有望成为能源收集、智能材料和生物传感等领域的重要组成部分,为人们的生活和工作提供更多的便利和可能性。
无机压电纳米纤维材料的应用
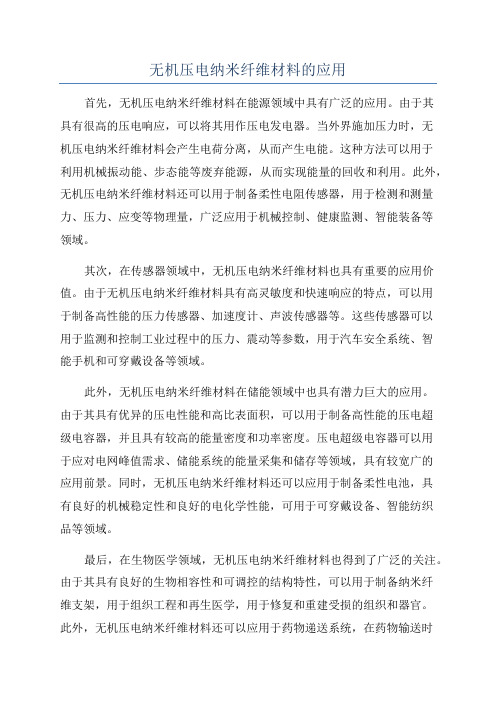
无机压电纳米纤维材料的应用首先,无机压电纳米纤维材料在能源领域中具有广泛的应用。
由于其具有很高的压电响应,可以将其用作压电发电器。
当外界施加压力时,无机压电纳米纤维材料会产生电荷分离,从而产生电能。
这种方法可以用于利用机械振动能、步态能等废弃能源,从而实现能量的回收和利用。
此外,无机压电纳米纤维材料还可以用于制备柔性电阻传感器,用于检测和测量力、压力、应变等物理量,广泛应用于机械控制、健康监测、智能装备等领域。
其次,在传感器领域中,无机压电纳米纤维材料也具有重要的应用价值。
由于无机压电纳米纤维材料具有高灵敏度和快速响应的特点,可以用于制备高性能的压力传感器、加速度计、声波传感器等。
这些传感器可以用于监测和控制工业过程中的压力、震动等参数,用于汽车安全系统、智能手机和可穿戴设备等领域。
此外,无机压电纳米纤维材料在储能领域中也具有潜力巨大的应用。
由于其具有优异的压电性能和高比表面积,可以用于制备高性能的压电超级电容器,并且具有较高的能量密度和功率密度。
压电超级电容器可以用于应对电网峰值需求、储能系统的能量采集和储存等领域,具有较宽广的应用前景。
同时,无机压电纳米纤维材料还可以应用于制备柔性电池,具有良好的机械稳定性和良好的电化学性能,可用于可穿戴设备、智能纺织品等领域。
最后,在生物医学领域,无机压电纳米纤维材料也得到了广泛的关注。
由于其具有良好的生物相容性和可调控的结构特性,可以用于制备纳米纤维支架,用于组织工程和再生医学,用于修复和重建受损的组织和器官。
此外,无机压电纳米纤维材料还可以应用于药物递送系统,在药物输送时可以通过施加压力、震动等方法来实现控制释放,具有较高的控制性和可调性。
综上所述,无机压电纳米纤维材料在能源、传感器、储能和生物医学等领域中具有广泛的应用价值。
随着纳米技术和制备技术的不断发展和改进,相信无机压电纳米纤维材料的应用前景将更加广阔,为各个领域中的科学研究和工程技术提供更多可能。
1-3型压电纤维复合材料薄壁梁-精品文档
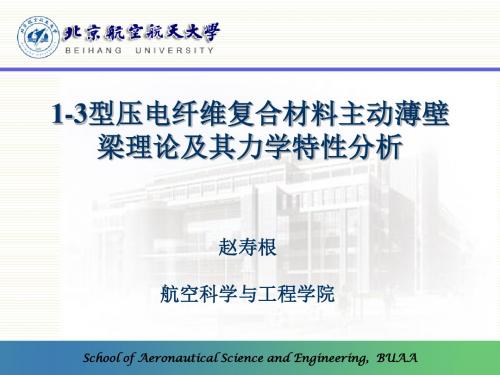
s n sn xn
同时注意另外两个应变
0 0 0 0
不为0。 (1)
x xs
(1) 薄壁梁上任意一点位移表达式的推导 设A为薄壁梁截面中线上任意的一点,它在整体坐标 系中坐标为(x0,y0,z0),在A点处有以改点为原点的流动 笛卡尔坐标系x-s-n,则薄壁梁截面上的任意一点B可以 用流动坐标系唯一确定,它在流动坐标系下的坐标为 (x0,s,n)。由以上可以推导出薄壁梁上任意点B的在整体 坐标系下的位移(u,v,w)与中面平动位移u0(x)、v0(x)和 w0(x)及其转角Φ x, Φ y和Φ z的关系为:
由于薄壁结构可以减轻结构自重,合理利用材料,并且 使得结构外形美观,所以工程中大量采用薄壁构件作为 承力部件。 其典型的应用领域有航空航天(机翼和直升机的旋翼是典 型的薄壁结构)、船舶、车辆、起重机和高层建筑等。薄 壁梁在工程中的使用,不可避免的会遇到振动、噪声和 结构优化等问题,由此也就引出了薄壁结构的振动和形 状控制等问题,特别是薄壁结构的扭转振动和扭转形状 控制。
采用虚拟操作面技术的飞机示意图
薄壁梁的研究进展
为了设计所需性能的含1-3型压电纤维复合材料的主动薄壁梁,就需要一 个可靠的,精度较高的和易于操作的主动梁分析模型。 Vasilan等研究了各向异性材料的薄壁梁自由扭转问题,其模型的主要特 点是假设结构受载时,剖面在固有平面内不变形,轴向应力和曲度为零; Gjelsvik A等结合圣维南原理假设闭室薄壁梁结构中面剪切变形的分布与 圣维南分布一致,建立了与开口剖面形式一致的闭室薄壁结构扭转综合 理论; Relifield等从能量原理推出了一个单闭室盒式薄壁梁模型,考虑了横向剪 切和约束扭转翘曲的影响; 邓采用高次翘曲理论对复合材料薄壁结构的限制扭转力学特性进行了分析 和研究。
智能压电纤维复合材料及其应用

智能压电纤维复合材料及其应用智能压电纤维复合材料是一种具有优异性能和广泛应用前景的新型材料。
它由压电陶瓷纤维和聚合物基体复合而成,结合了压电效应和材料弹性特性,具有优异的机械性能和电-机耦合性能。
本文将介绍智能压电纤维复合材料的制备方法、性能特点以及其在各个领域的应用。
智能压电纤维复合材料的制备方法多种多样,常见的包括热压法、浸渍法和电纺法等。
其中,热压法是最常用的制备方法之一。
该方法通过将预先制备好的压电陶瓷纤维和聚合物基体在高温高压条件下进行热压,使两者充分结合,形成复合材料。
浸渍法则是将聚合物基体浸渍到压电陶瓷纤维的空隙中,然后经过热处理固化。
电纺法则是将压电陶瓷纤维和聚合物基体同时溶解,通过高压电场的作用使两者同时喷射到收集器上,并在此过程中形成纤维复合材料。
这些制备方法各有优缺点,可以根据具体需求选择合适的制备方法。
智能压电纤维复合材料具有许多优异的性能特点。
首先,它具有优异的压电效应,能够将机械能转化为电能,实现电-机耦合。
其次,由于纤维增强的存在,它具有较高的强度和刚度,具备良好的抗拉、抗压性能。
此外,智能压电纤维复合材料还具有较高的温度稳定性和耐腐蚀性,能够在恶劣环境下长期稳定运行。
最重要的是,智能压电纤维复合材料具有良好的可塑性和可加工性,可以通过调整配方和制备工艺来实现对其性能的优化。
智能压电纤维复合材料在众多领域具有广泛的应用前景。
在航天航空领域,它可以应用于飞行器的结构健康监测和振动控制,实现飞行器的智能化和安全性能的提升。
在智能结构领域,它可以用于制备智能传感器和执行器,实现结构的自感知、自适应和自修复。
在医疗领域,它可以应用于人工关节、人工肌肉等医疗器械的制备,实现人体运动的仿生控制和康复治疗。
在能源领域,它可以用于制备微型发电装置,收集机械能并转化为电能,实现自供电。
智能压电纤维复合材料是一种具有优异性能和广泛应用前景的新型材料。
它通过压电效应和材料弹性特性的结合,具有优异的机械性能和电-机耦合性能。
生物压电材料的制备与应用
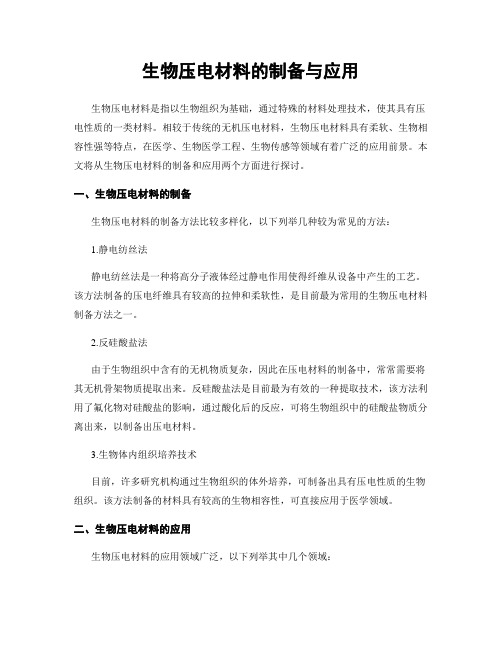
生物压电材料的制备与应用生物压电材料是指以生物组织为基础,通过特殊的材料处理技术,使其具有压电性质的一类材料。
相较于传统的无机压电材料,生物压电材料具有柔软、生物相容性强等特点,在医学、生物医学工程、生物传感等领域有着广泛的应用前景。
本文将从生物压电材料的制备和应用两个方面进行探讨。
一、生物压电材料的制备生物压电材料的制备方法比较多样化,以下列举几种较为常见的方法:1.静电纺丝法静电纺丝法是一种将高分子液体经过静电作用使得纤维从设备中产生的工艺。
该方法制备的压电纤维具有较高的拉伸和柔软性,是目前最为常用的生物压电材料制备方法之一。
2.反硅酸盐法由于生物组织中含有的无机物质复杂,因此在压电材料的制备中,常常需要将其无机骨架物质提取出来。
反硅酸盐法是目前最为有效的一种提取技术,该方法利用了氟化物对硅酸盐的影响,通过酸化后的反应,可将生物组织中的硅酸盐物质分离出来,以制备出压电材料。
3.生物体内组织培养技术目前,许多研究机构通过生物组织的体外培养,可制备出具有压电性质的生物组织。
该方法制备的材料具有较高的生物相容性,可直接应用于医学领域。
二、生物压电材料的应用生物压电材料的应用领域广泛,以下列举其中几个领域:1.医学生物压电材料的柔软性、生物相容性等特点,使得其在医学领域中有着广泛的应用。
例如,可应用于生物医学传感器、人工智能假肢等领域。
2.生物传感生物压电材料的压电性质可被用于生物传感和生物检测领域。
例如,可应用于血压、脉搏、呼吸等监测方面。
3.生物能源压电效应可将机械能转化为电能,因此生物压电材料可被应用于生物能源领域。
例如,可应用于超小型传感器等电能转化设备中。
总之,生物压电材料是一种具有广阔应用前景的材料。
未来,随着该材料的制备和应用技术的不断发展,其在医学、生物工程、能源等领域中的应用前景将会更加广阔。
压电纤维发展现状
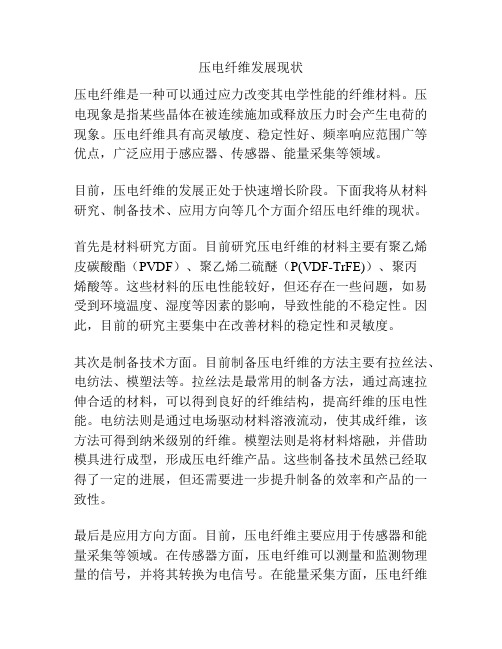
压电纤维发展现状压电纤维是一种可以通过应力改变其电学性能的纤维材料。
压电现象是指某些晶体在被连续施加或释放压力时会产生电荷的现象。
压电纤维具有高灵敏度、稳定性好、频率响应范围广等优点,广泛应用于感应器、传感器、能量采集等领域。
目前,压电纤维的发展正处于快速增长阶段。
下面我将从材料研究、制备技术、应用方向等几个方面介绍压电纤维的现状。
首先是材料研究方面。
目前研究压电纤维的材料主要有聚乙烯皮碳酸酯(PVDF)、聚乙烯二硫醚(P(VDF-TrFE))、聚丙烯酸等。
这些材料的压电性能较好,但还存在一些问题,如易受到环境温度、湿度等因素的影响,导致性能的不稳定性。
因此,目前的研究主要集中在改善材料的稳定性和灵敏度。
其次是制备技术方面。
目前制备压电纤维的方法主要有拉丝法、电纺法、模塑法等。
拉丝法是最常用的制备方法,通过高速拉伸合适的材料,可以得到良好的纤维结构,提高纤维的压电性能。
电纺法则是通过电场驱动材料溶液流动,使其成纤维,该方法可得到纳米级别的纤维。
模塑法则是将材料熔融,并借助模具进行成型,形成压电纤维产品。
这些制备技术虽然已经取得了一定的进展,但还需要进一步提升制备的效率和产品的一致性。
最后是应用方向方面。
目前,压电纤维主要应用于传感器和能量采集等领域。
在传感器方面,压电纤维可以测量和监测物理量的信号,并将其转换为电信号。
在能量采集方面,压电纤维可以通过机械振动或压力改变将能量转换为电能。
这些应用方向的发展为压电纤维的应用提供了广阔的发展前景。
总体来说,压电纤维正处于不断发展和突破的阶段,随着材料研究和制备技术的不断进步,压电纤维的性能将不断提升,应用领域也将不断拓展。
未来,我们可以期待压电纤维在智能材料、传感技术等领域的更广泛应用。
压电纤维复合材料的研究

( 蚰j gU i ri f eoat sadA t n u c , 蚰j g 10 6 N i n esyo A rnui n so t s N i 2 0 1 ) n v t c ra i n
A s a t I re a et c aigs a n t s f nedg ae lc o e (D b t c nod r ori eat t t i a ds eso t ii t e t d s I E)peolc i f e r t s h u n rn r i r t d er i eetci r z r b
维普资讯
・
计算材料学 ・
压 电纤 维 复 合 材 料 的研 究
刘永 刚 沈 星 赵 东标
201 ) 10 6
裘 进 浩
( 南京航空航 天大学 , 南京
文
摘
以提 高 交叉指 形 电极 压 电纤 维复合 材 料诱 导应 变和挟 持 应 力为 目的 , 用有 限元软 件 A S S分 采 NY
I tr ii td E e to e iz ee ti i e mp sts n edgt e l cr d sP e o l crc F b rCo o i a e
LuY ngn i oga g
S e ig hnXn
Z a ogio hoD nba
QuJ ho i i a n
sn e o fI r i r a i g wi t fI ig p r d o DE o nce sn d h o DE d v l me r to o b r mo g t e c mpo ie i n a ou a i ff e s a n o i h st .
与 传统 的平 面压 电 陶瓷驱 动器 相 比 , 电纤维 复合 材 压
- 1、下载文档前请自行甄别文档内容的完整性,平台不提供额外的编辑、内容补充、找答案等附加服务。
- 2、"仅部分预览"的文档,不可在线预览部分如存在完整性等问题,可反馈申请退款(可完整预览的文档不适用该条件!)。
- 3、如文档侵犯您的权益,请联系客服反馈,我们会尽快为您处理(人工客服工作时间:9:00-18:30)。
RECENT DEVELOPMENTS IN NASA PIEZOCOMPOSITE ACTUATOR TECHNOLOGYW. Wilkie U.S. Army Research Laboratory, Hampton, VA, USA D. Inman Virginia Polytechnic and State University, Blacksburg, Virginia, USA J. High NASA Langley Research Center, Hampton, Virginia, USA R. Williams NASA Jet Propulsion Laboratory, Pasadena, California, USA Abstract: In this paper, we present an overview of recent progress in the development of the NASA Macro-Fiber Composite (MFC) piezocomposite actuator device. This will include a brief history of the development of the MFC, a description of the standard manufacturing process used to fabricate MFC actuators, and a summary of ongoing MFC electromechanical characterization testing. In addition, we describe the development of a prototype single-crystal piezoelectric MFC device, and compare its performance with MFC actuator specimens utilizing conventional piezoceramic materials. Keywords: piezoelectric actuators, piezoelectric fiber composites Introduction Piezoelectric fiber composite actuators were originally developed as a means of overcoming many of the practical difficulties associated with using monolithic piezoceramic actuators in structural control applications [1]. Chief among these difficulties were brittleness of piezoceramic materials, poor conformability, particularly when applied to non-planar structures, nondirectional nature of strain actuation, and overall low strain energy density. To increase conformability, first generation piezocomposite actuators were manufactured using a layer of extruded piezoceramic fibers encased in a protective polymer matrix material. Strain energy density was improved by utilizing interdigitated electrodes to produce electrical fields in the plane of the actuator. In-plane electrical fields allow the piezoceramic elements to produce nearly twice the strain actuation, and four times the strain energy density, of a through-plane poled piezoceramic device. The NASA Langley Research Center Macro-Fiber Composite (MFC) actuator [2] was developed to alleviate many of the manufacturing and performance disadvantages associated with early piezocomposites [3]. The MFC (see Fig. 1) retains the most advantageous features of the early piezocomposite actuators, namely, high strain energy density, directional actuation, conformabilityFig. 1: NASA Macro-Fiber Composite actuator. and durability, yet incorporates several new features, chief among these being the use of lowcost fabrication processes that are uniform and repeatable [4, 5]. The principal components of the MFC and their arrangement in the actuator package are illustrated in Fig. 2. The piezoelectric fiber sheets used in assembling the MFC are machined from low-cost piezoceramic wafers using a computer-controlled dicing saw. The sheets are easily handled and allow the piezoceramic fibers to be precisely aligned within the actuator package. Producing and handling piezoceramic fibers in precision groups, versus individual pieces, minimizes variations in the active and passive properties of the actuatorFig. 2: General arrangement of Macro-Fiber Composite actuator components. package. Production costs associated with handling and manufacturing of individual piezoceramic fibers are also reduced. The flat surfaces of the piezoceramic fiber elements also permit direct contact with the interdigitated electrodes, which minimizes electric field attenuation by the low dielectric epoxy matrix. As a result, actuation performance of MFC piezocomposites tends to be greater (150%) than the earlier round piezoceramic fiber piezocomposites, which often suffer from poor or inconsistent electrode contact. The MFC has proven to be particularly useful in a variety of rotary-wing and fixed-wing aeronautical applications, ultra-lightweight spacecraft structures applications, and several recent automotive applications. In this paper, we present an overview of our most recent work in the development of the NASA MFC actuator device. In particular, we detail results from an extensive engineering properties characterization effort on laboratory reference MFC specimens, and describe our experiences in developing a single-crystal piezoelectric MFC actuator device. Engineering properties of MFC devices-1500Fig. 3: Typical reference MFC device used for electro-mechanical properties testing. Specimen is 140 mm x 25 mm x 0.3 mm, approx., including tabs. Measured maximum free-strain actuation capabilities of a typical reference MFC device are illustrated in Fig 4. This maximum peak-to-peak actuation strain of approximately 2000 parts-permillion in the longitudinal direction is typical for all NASA-standard MFC devices. The free-strain output of the MFC, as with most piezoceramic devices, varies considerably with the driving electric field amplitude. This variability in effective piezoelectric constants (d33, d31) is nonlinear, but repeatable as seen in Fig. 5.B B B B15001000500free strain, µε-2.E+06-1.E+060 0.E+00 -5001.E+062.E+063.E+064.E+06-1000longitudinal strain transverse strainWhile some MFC engineering properties, either estimated, or experimentally measured, have existed for some time, a complete and experimentally validated set of orthotropic mechanical properties, including low-field to high-field piezoelectric constants, has only recently become available [6]. These properties, which are summarized here, were determined by measurements performed on laboratory reference MFC devices (see Fig. 3). The reference MFC differs from typical MFC geometries in that piezoceramic fibers extend longitudinally beyond the edges of the active electrode field region. This is done to provide areas for bonding grip tabs to the specimens when conducting tensile mechanical tests. The reference MFC device is otherwise constructed using the standard MFC manufacturing method and materials.average electric field, V/mFig. 4: Maximum free-strain response of reference MFC device.6E-10effective piezoelectric constant, m/Vlongitudinal (d 33 )4E-102E-10transverse (-d 31 )0 0 500 1000 1500 2000 2500electrode voltage amplitude, V p-pFig. 5: Field amplitude dependence and repeatability of reference MFC piezoelectric constants; 1-σ error bars shown.Short-circuit stress-strain characteristics of the reference MFC under tensile loading conditions are shown in Fig. 6. In all cases, coupon-to-coupon variations in the elastic properties were small, indicating a high degree of repeatability in the MFC manufacturing process. This experimental program has allowed us to develop and validate several useful analytical micromechanics models for predicting MFC elastic properties [6]. These models most recently have been used to design application-specific MFC laminates, and perform parameter variations studies on the standard MFC package geometry.Fig. 7: Prototype PMN-PT single-crystal MFC piezocomposite actuator. Active area is 20 mm x 20 mm.2000longitudinal free strain, µε15001000500 PMN-PT PZT-5H PZT-5A0 0 500 1000 15002000electrode voltage, VFig. 6: Short-circuit tensile properties of reference MFC actuators. Single-crystal MFC piezocomposites Single crystal piezoelectric materials have been the subject of much recent attention due to their promising high-performance piezoelectric properties [7]. Only in the past several years have these materials become commercially available in sizes and quantities suitable for constructing large-scale actuator devices [8]. The authors have successfully constructed and tested one such single-crystal piezoelectric actuator utilizing standard MFC fabrication techniques [9]. A photograph of the prototype single-crystal MFC, which utilizes machined PMN-PT single-crystal fibers in place of conventional piezoceramic fibers, is shown in Fig. 7. Free strain performance of the prototype single crystal device was measured under quasistatic conditions and compared with geometrically identical devices which use conventional piezoceramic materials. Free-strain response of the PMN-PT specimen is compared with that of a reference specimen constructed with the MFC baseline PZT-5A material and a specimen utilizing an alternative PZT-5H ceramic material. Typical unipolar high-field quasistatic response is shown in Fig. 8.Fig. 8: Free-strain performance of single-crystal and piezoceramic MFC devices under quasistatic unipolar operation. The free-strain of the single-crystal piezocomposite was significantly greater than both of the piezoceramic reference specimens under all voltage conditions. This is qualitatively consistent with expectations based upon the bulk material piezoelectric d33 constants of the three specimens, although a large electric field amplitude dependence in effective d constants was observed (see Fig. 9), with a greater relative benefit realized with the single crystal material at lower driving electric fields.B B1000effective d3p, pm/V500PMN-PT PZT-5H PZT-5Ad330 PZT-5A PZT-5H PMN-PT -500 0 1 2 3 4d31electric field amplitude, kV/mm p-pFig. 9: Electric field amplitude dependence of piezoelectric constants for single crystal and standard piezoceramic MFC devices.Using the micromechanics models developed for the conventional MFC, and bulk material data for the PMN-PT single crystal materials, elastic properties of the single-crystal MFC device were estimated. These calculated properties, taken with the measured free-strain actuation behavior, allow us to estimate the useful working range of a single-crystal MFC when operating against an elastic constraint, e.g., when bonded to, or imbedded within, a nonactive material host structure (see Fig. 10).40References [1] Bent, A., “Active Fiber Composite Material Systems for Structural Control Applications,” Proceedings, SPIE’s 6th International Symposium on Smart Structures and Materials, Newport Beach, CA, March 1-5, 1999. [2] Wilkie, W., High, J., Mirick, P., Fox, R., Little, B., Bryant, R., Hellbaum, R., Jalink, A., "LowCost Piezocomposite Actuator for Structural Control Applications," Proceedings, SPIE’s 7th International Symposium on Smart Structures and Materials, Newport Beach, California, March 5-9, 2000. [3] Yoshikawa, S., Farrell, M., Warkentin, D., Jacques, R., Saarmaa, E., “Monolithic Piezoelectric Actuators and Vibration Dampers with Interdigitated Electrodes,” Proceedings, SPIE’s 6th International Symposium on Smart Structures and Materials, Newport Beach, CA, March 1-5, 1999. [4] High, J., Wilkie, W., "Method of Fabricating NASA-Standard Macro-Fiber Piezocomposite Actuators," NASA/TM-2003-212427, ARL TR 2833, June 2003. [5] Wilkie, W., et al, "Piezoelectric Macro-Fiber Composite Actuator and Method for Making Same," U.S. Patent No. 6,629,341, October 7, 2003. [6] Williams, R., Schultz, M., Hyer, M., Inman, D., Wilkie, W., "Nonlinear Tensile and Shear Behavior of Macro Fiber Composite Actuators," Journal of Composite Materials, accepted for publication, September, 2003. [7] Park, S-E., Shrout, T., “Ultrahigh Strain and Piezoelectric Behavior in Relaxor Based Ferroelectric Single Crystals,” Journal of Applied Physics, Vol 82, No. 4, August, 1997. [8] Rossetti, Jr., G., Pizzochero, A., Bent, A., “Recent Advances in Active Fiber Composites Technology,” Proceedings of the 2000 12th IEEE International Symposium on Applications of Ferroelectrics, pp. 753-756, 2000. [9] Wilkie, W., Inman, D., Lloyd, J., High, J., "Anisotropic Piezocomposite Actuator Incorporating Machined PMN-PT Single Crystal Fibers," SDM 12th AIAA/ASME/AHS Adaptive Structures Conference, Palm Springs, CA, 19-22 April 2004.induced stress, MPamaximum blocked-stress (inferred) 20 -cE33 (calculated)PZ TPMN -PT (±12 5 V)maximum free-strain (measured)-5 A(00 0-2 kV )PZ T5H(02k V)PMN-PT (0-2 kV)500100015002000induced strain, µεFig. 10: Estimated stress and strain actuation envelope of single-crystal and piezoceramic MFC devices. The triangular areas indicate the regions of induced stress and strain actuation producible for bipolar, low-voltage operation and unipolar, high-voltage operation. Dashed lines indicate the corresponding operational envelopes of the PZT-5A reference MFC and the PZT-5H alternate MFC device. This diagram suggests that the low stiffness (estimated) and high strain properties of the PMN-PT single crystal MFC make it best suited to low-stress, high strain applications. In particular, if only very low driving are available, vis, the smaller triangle region, the PMN-PT device can outperform the reference PZT-5A device under all circumstances. For higher induced stress applications, the piezoceramic devices, with their higher stiffness properties, will be preferred to the single-crystal MFC device studied here. Conclusions Our extensive electromechanical characterization effort of the NASA Macro-Fiber Composite actuator has shown that it has predictable and repeatable linear elastic properties, and repeatable non-linear actuation properties. The successful initial tests of a prototype single-crystal MFC device indicate that the manufacturing methods used to construct standard piezoceramic MFC actuators may be used without modification to construct practical single-crystal piezoelectric composite actuators with actuation properties superior to the conventional piezoceramic-based piezocomposite actuators.。